- 1Department of Biology, University of Nebraska Omaha, Omaha, NE, United States
- 2Institute of Clinical Medicine, Aarhus University, Aarhus, Denmark
- 3Department of Infectious Diseases, Aarhus University Hospital, Aarhus, Denmark
HIV reservoirs persist in infected individuals despite combination antiretroviral therapy and can be identified in secondary lymphoid tissues, in intestinal tissues, in the central nervous system as well as in blood. Clinical trials have begun to explore effects of small molecule interventions to perturb the latent viral infection, but only limited information is available regarding the impacts of HIV cure-related clinical interventions on viral reservoirs found in tissues. Of the 14 HIV cure-related clinical trials since 2012 that have evaluated the effects of small molecule interventions in vivo, four trials have examined the impacts of the interventions in peripheral blood as well as other tissues that harbor persistent HIV. The additional tissues examined include cerebral spinal fluid, intestines and lymph nodes. We provide a comparison contrast analyses of the data across anatomical compartments tested in these studies to reveal where peripheral blood analyses reflect outcomes in other tissues as well as where the data reveal differences between tissue outcomes. We also summarize the current knowledge on these topics and highlight key open questions that need to be addressed experimentally to move the HIV cure research field closer to the development of an intervention strategy capable of eliciting long-term antiretroviral free remission of HIV disease.
Introduction
Despite years of successful combination antiretroviral therapy (cART), viral replication rebounds almost inevitably in all HIV infected individuals upon cART cessation (Deeks et al., 2016; Margolis et al., 2016; Wong and Yukl, 2016). This is due to the presence of persistent viral reservoirs which are the greatest barrier to an HIV cure. Because of the relative ease with which peripheral blood can be collected, this is the most common anatomical compartment analyzed in clinical HIV studies (Archin et al., 2012, 2014, 2017; Elliott et al., 2014, 2015; Rasmussen et al., 2014; Spivak et al., 2014; Mothe et al., 2015; Søgaard et al., 2015; Gutierrez et al., 2016; Leth et al., 2016; Vibholm et al., 2017, 2019a; Saxena et al., 2019; Table 1). However, it is known that HIV (and SIV in non-human primates) persists in multiple organ systems throughout the body during cART and that peripheral blood reservoir findings may not accurately reflect reservoirs in tissue (Costiniuk and Jenabian, 2014; Lamers et al., 2016; Rose et al., 2016, 2018; Estes et al., 2017; Nolan et al., 2018). Thus, it is essential that the impacts of curative strategies in all relevant organ systems be defined.
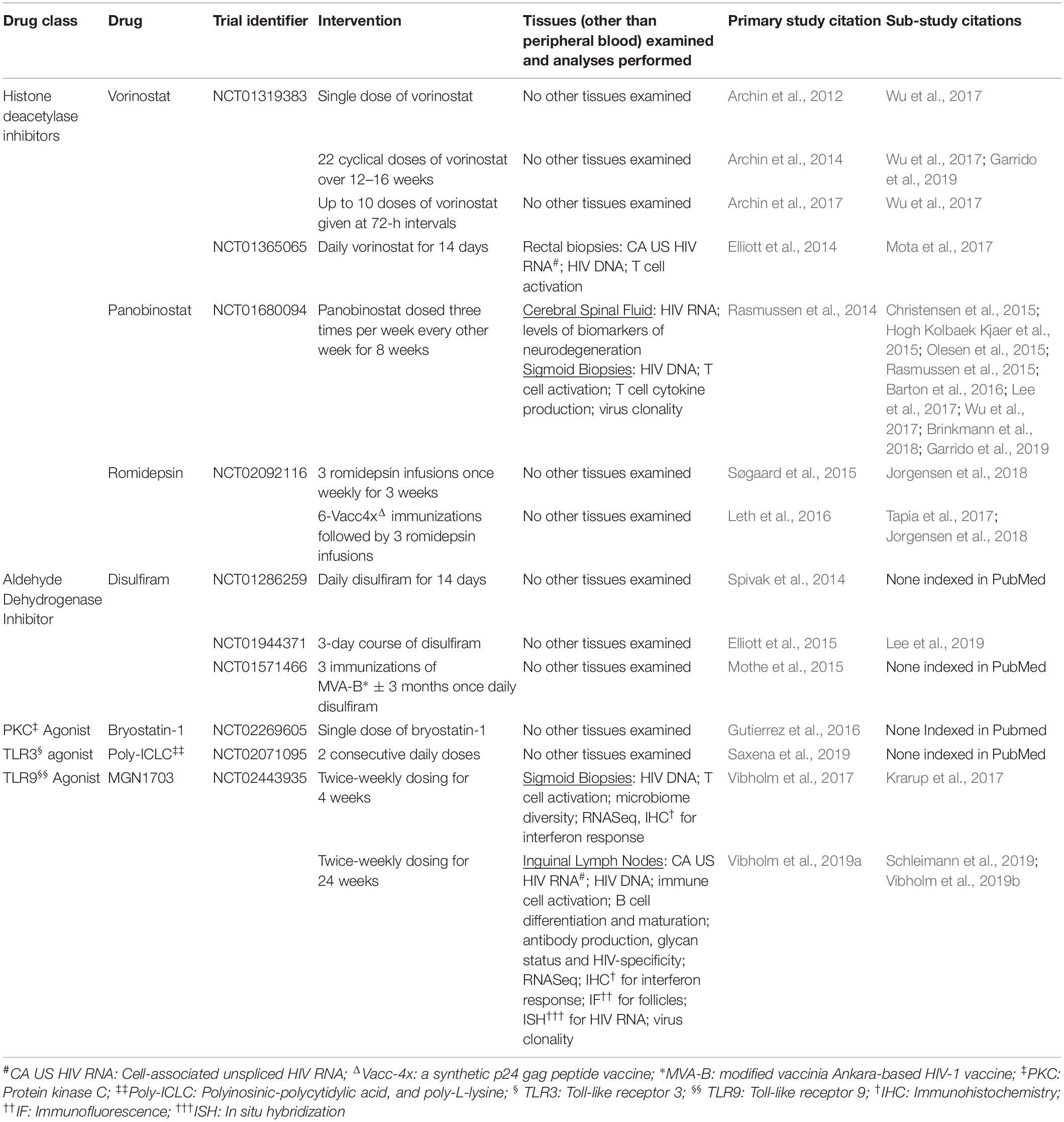
Table 1. Overview of tissue analyses in clinical studies dosing small molecule interventions in HIV cure-related context.
There are many unanswered questions regarding the impacts of HIV cure-related interventions on systemic HIV persistence. Among these are: Do findings in peripheral blood reflect outcomes in other tissues like the intestines and lymph nodes? and Do persistent viruses move freely between anatomical compartments? Studies have indicated that compartmentalization of HIV-infected cells into specific anatomical compartment and/or immunological sanctuaries occurs in untreated infections and that this compartmentalization persists during suppressive cART (Blackard, 2012). In addition, some of the drugs that have been tested in HIV cure trials may have reduced penetration into these compartments which may impede HIV cure efforts (Berg et al., 2004; Rasmussen et al., 2015). Therefore, we have focused this review on the impacts of HIV cure-related strategies onto clinical studies dosing small molecule interventions [e.g., histone deacetylase inhibitors, PKC agonist, disulfiram and toll-like receptor (TLR) agonists] and examining HIV persistence in tissues. Specifically, we review the impacts of such interventions on mechanisms that regulate HIV persistence in vivo as well as the immunological and virological impacts of these interventions in tissues other than peripheral blood. Other HIV cure related interventions including gene therapy approaches, stem cell transplants, antiretroviral intensification, therapeutic vaccines and broadly neutralizing antibody infusions have recently been reviewed elsewhere (Rasmussen and Sogaard, 2018; Caskey et al., 2019). Similarly, we callout several key animal model findings but do not elaborate upon animal model studies of HIV cure-related interventions as these data have also recently been reviewed elsewhere (Micci et al., 2015; Denton et al., 2016; Policicchio et al., 2016; Nixon et al., 2017; Honeycutt and Garcia, 2018; Whitney and Brad Jones, 2018).
Effects of HIV Cure Interventions in the Central Nervous System, Intestines, and Lymph Nodes
We and others have made efforts to complement peripheral blood analyses by defining the effects of various HIV cure-related interventions on HIV persistence within key tissues. In these trials, the intervention were either suberoylanilide hydroxamic acid (SAHA), panobinostat or the TLR9 agonist MGN1703 (Elliott et al., 2014; Rasmussen et al., 2014; Vibholm et al., 2017, 2019a). In the trial exploring SAHA as a latency reversing agent (LRA), the Lewin group examined rectal tissue biopsies (Elliott et al., 2014). In our panobinostat trial, we examined cerebral spinal fluid and sigmoid biopsies (Christensen et al., 2015; Rasmussen et al., 2015). And in our MGN1703 trials, we examined sigmoid biopsies and lymph nodes (Krarup et al., 2017; Schleimann et al., 2019). All these anatomical reservoir studies were longitudinal in design as they included analyses of samples at baseline as well as near the end of the dosing period for the interventional drug in the respective study.
Human and animal study data highlight the potential for the central nervous system to function as an HIV reservoir or sanctuary site for the virus during treatment (Clements et al., 2005; Barber et al., 2006; Churchill et al., 2009; Zink et al., 2010; Queen et al., 2011; Gray et al., 2014; Honeycutt et al., 2017, 2018). Investigators have examined the toxicity and latency reversal effects of multiple agents including panobinostat and romidepsin on primary astrocytes ex vivo (Gray et al., 2016). These agents were found to be non-toxic and capable of inducing viral transcription at therapeutic concentrations. Our study provides the only published in vivo human data on central nervous system effects of a latency reversal agent to date (Rasmussen et al., 2015). We found that repeated, cyclic treatment with panobinostat did not lead to central nervous system adverse effects according to cerebral spinal fluid biomarkers of inflammation and neurodegeneration. We also found that panobinostat did not sufficiently penetrate the central nervous system to detectable levels and that there were no treatment-associated changes in HIV reservoir detection in the cerebral spinal fluid (Rasmussen et al., 2015). This study represents a single foray into determining the in vivo effects of HIV cure interventions in the cerebral spinal fluid for one intervention. However, this finding may not be specific for panobinostat since it has been shown in non-human primates that the concentration of another latency reversal agent romidepsin in cerebral spinal fluid is only approximately 2% of the level found in plasma (Berg et al., 2004). Given the scarcity of data, drawing conclusions about distinct HIV cure-related intervention impacts in the central nervous system is premature.
The role of intestines in HIV persistence has been researched extensively in humans as well as non-human primates (Anton et al., 2003; Guadalupe et al., 2003; Brenchley et al., 2004; Mehandru et al., 2004; Li et al., 2005; Mattapallil et al., 2005; van Marle et al., 2007; Chun et al., 2008; Ciccone et al., 2010; North et al., 2010; Yukl et al., 2010a, b; Chege et al., 2011; Lerner et al., 2011; Evering et al., 2012; Horiike et al., 2012; Kline et al., 2013; Deere et al., 2014; Estes et al., 2017). In a clinical study of 14 days of repeated administration of the histone deacetylase inhibitor SAHA, HIV RNA levels in rectal CD4 + T cells were modestly increased and HIV DNA levels were unchanged (Elliott et al., 2014). This outcome was also realized in our studies of the sigmoid colon during panobinostat as well as MGN1703 dosing given that we did not observe cohort wide changes in the size of the HIV reservoir in either study (Christensen et al., 2015; Krarup et al., 2017). While SAHA had no impact on T cell activation in rectal tissue, panobinostat dosing was associated with a decreased frequency of CD69+ intestinal T cells (Elliott et al., 2014; Christensen et al., 2015). This was in contrast to findings in peripheral blood where T cell activation (CD69+) was found to be increased following the first doses of panobinostat (Brinkmann et al., 2018). Additionally, we observed that panobinostat increased IL-17A expression in the intestinal epithelium and IL-17a is known to induce the production of antimicrobial peptides that may help to maintain the intestinal epithelial barrier which is damaged during HIV infection (Brenchley et al., 2006; Liang et al., 2006; Christensen et al., 2015). With dosing of the TLR9 agonist MGN1703, a robust interferon response was noted in the sigmoid colon (Krarup et al., 2017). This interferon response in the intestine was quite distinct from that observed in the peripheral blood of the same individuals (Vibholm et al., 2017). Specifically, we observed that both type I and type II interferons were generated in the periphery of treated individuals but only a type I interferon response was detected in the sigmoid colon. The type I interferon response in the colon was associated with a trend toward improved intestinal microbiome species composition. Furthermore, we found that higher baseline levels of TLR9 expression in the intestine was associated with greater reductions in levels of integrated HIV DNA during MGN1703 treatment (Krarup et al., 2017). This result suggests that tissue-specific biomarkers may help determine which individuals will exhibit the strongest response to HIV cure-related interventions in future studies. Overall, these analyses examining the intestines of participants taking HIV cure-related interventions show that the intestines are an important anatomical site for study as the peripheral blood and intestines did not always exhibit similar responses to treatment in the examined parameters. Future studies will benefit from incorporating comprehensive intestinal biopsy analyses into the study plan.
The importance of lymphoid tissues, particularly lymph nodes, in HIV persistence is clear (Shen et al., 2003; Dinoso et al., 2009; Fukazawa et al., 2015; Banga et al., 2016; Deleage et al., 2016; Lorenzo-Redondo et al., 2016; Estes et al., 2017). Beyond the observation that lymph node tissues showed no changes in SIV reservoirs in non-human primates given SAHA (Del Prete et al., 2014), there has been no published data on the impacts of HIV cure-related interventions in vivo in lymph node tissues. To begin addressing this major knowledge gap in the field, we undertook a longitudinal study of inguinal lymph nodes in participants taking the TLR9 agonist MGN1703 for 24 weeks (Schleimann et al., 2019). We found that lymph nodes exhibited a potent interferon response to MGN1703 dosing as was observed in peripheral blood (Vibholm et al., 2019a). We also observed similarities between the lymph nodes and peripheral blood regarding significant changes in B cell differentiation and maturation levels in response to TLR9 agonist treatment. Related to these observations, we found that MGN1703 increased plasma IgG levels as well as increased AID expression in lymph nodes (Schleimann et al., 2019). Furthermore, after 24 weeks of MGN1703 dosing, plasma and lymph node IgG glycosylation patterns were significantly altered. Changes in glycosylation were associated with reductions in viral reservoir. This study revealing similarities between the peripheral blood and lymph node responses to MGN1703 is a beginning in the process of understanding the lymphoid tissue effects of HIV cure-related interventions.
No Evidence of Viral Compartmentalization in TLR9 Agonist Therapy Trial
While the direct in vivo impacts of HIV cure-related clinical interventions on latency controlling mechanisms have not been fully elucidated, there are multiple analyses that have focused on determining whether such interventions impact only clonal HIV isolates or reactivate a broad spectrum of persistent HIV isolates. These phylogenetic analyses performed with clinical trial samples have revealed that the histone deacetylase inhibitors SAHA, panobinostat and romidepsin reactivate latent viruses with unique sequence signatures as well as families of virus clones (Barton et al., 2016; Winckelmann et al., 2017, 2018). The panobinostat study also provided the first in vivo observation of a tissue-derived cell (i.e., a sigmoid colon lamina propria mononuclear cell) harboring an HIV provirus that matched plasma-derived rebound viruses isolated following analytical treatment interruption (Barton et al., 2016). Thus, these HIV cure-related interventions have broad latency reversing capacity in vivo in HIV infected individuals.
We recently examined the clonality of persistent virus in lymph nodes and compared these sequences to replication competent viruses that rebounded during an analytical treatment interruption (Vibholm et al., 2019b). We examined samples from our clinical trial in which participants received 24 weeks of repeated TLR9 agonist treatment. When we compared the latent viruses obtained from CD4+ T cells in peripheral blood and lymph nodes to viruses emerging during treatment interruption, we found there was no overlap between latent reservoir and rebound sequences. This was true even though 98% of intact or replication competent clonal sequences overlapped between these two anatomical compartments. Although rebound viruses were not derived from reservoirs detected in either blood or lymph node, we were able to show that rebound viruses were generated by recombination events between viruses within these two compartments (Vibholm et al., 2019b). This observation is consistent with peripheral blood data showing that recombination events are important during the emergence of rebound viremia (Lu et al., 2018). Whether the recombination events are due to improved viral fitness or escape of immune pressure is not yet known (Streeck et al., 2008; Ritchie et al., 2014). Overall, these data indicate that CD4+ T cells harboring latent HIV circulate between blood and lymphoid tissues during cART.
Conclusion
Understanding the regulation of HIV reservoir persistence is a high priority in the HIV cure research field. Since 2012, 14 HIV cure-related clinical trials have been published where the objective was to test the impacts of small molecule interventions designed to either cause infected cells to become visible to the immune system for clearance or to improve the ability of the immune system to clear infected cells. These clinical studies have yielded new insights into the effects of the interventions on the regulation of HIV persistence, particularly related to clonal populations of latently infected cells. In four of the trials, efforts were made to define the impacts of the respective intervention in the central nervous system, intestinal tissues and/or lymph nodes. Data from these four trials reveal key similarities between the peripheral blood and the organs. Furthermore, they highlight that observations made in peripheral blood are not always fully representative of the impacts made by interventions in the organs which also harbor persistent HIV reservoirs. Such differential responses highlight the importance of defining the impacts of curative strategies in all relevant organ systems including those reviewed herein as well as other applicable tissues such as spleen and bone marrow. With improved methods for analyzing tissue reservoirs, investigators will begin to overcome the limitations in studies that are due to the extreme rarity and heterogeneity of HIV infected cells in vivo in the setting of cART. Advancement of the HIV cure research agenda will benefit from a continued push to seek detailed explorations of infected cells both derived from peripheral blood as well as from organ sources. Thus, there is strong impetus to continue examining multiple organs in such trials.
Author Contributions
PD wrote the first draft the manuscript. OS and MT helped to wrote the manuscript. All authors read and approved the final manuscript.
Funding
We recognize the University of Nebraska at Omaha and the Novo Nordisk Foundation for support of this work. These agencies did not participate in analyses or the decision to publish.
Conflict of Interest Statement
The authors declare that the research was conducted in the absence of any commercial or financial relationships that could be construed as a potential conflict of interest.
References
Anton, P. A., Mitsuyasu, R. T., Deeks, S. G., Scadden, D. T., Wagner, B., Huang, C., et al. (2003). Multiple measures of HIV burden in blood and tissue are correlated with each other but not with clinical parameters in aviremic subjects. AIDS 17, 53–63. doi: 10.1097/00002030-200301030-00008
Archin, N. M., Bateson, R., Tripathy, M. K., Crooks, A. M., Yang, K. H., Dahl, N. P., et al. (2014). HIV-1 expression within resting CD4+ T cells after multiple doses of vorinostat. J. Infect. Dis. 210, 728–735. doi: 10.1093/infdis/jiu155
Archin, N. M., Kirchherr, J. L., Sung, J. A., Clutton, G., Sholtis, K., Xu, Y., et al. (2017). Interval dosing with the HDAC inhibitor vorinostat effectively reverses HIV latency. J. Clin. Invest. 127, 3126–3135. doi: 10.1172/JCI92684
Archin, N. M., Liberty, A. L., Kashuba, A. D., Choudhary, S. K., Kuruc, J. D., Crooks, A. M., et al. (2012). Administration of vorinostat disrupts HIV-1 latency in patients on antiretroviral therapy. Nature 487, 482–485. doi: 10.1038/nature11286
Banga, R., Procopio, F. A., Noto, A., Pollakis, G., Cavassini, M., Ohmiti, K., et al. (2016). PD-1(+) and follicular helper T cells are responsible for persistent HIV-1 transcription in treated aviremic individuals. Nat. Med. 22, 754–761. doi: 10.1038/nm.4113
Barber, S. A., Gama, L., Dudaronek, J. M., Voelker, T., Tarwater, P. M., and Clements, J. E. (2006). Mechanism for the establishment of transcriptional HIV latency in the brain in a simian immunodeficiency virus-macaque model. J. Infect. Dis. 193, 963–970. doi: 10.1086/500983
Barton, K., Hiener, B., Winckelmann, A., Rasmussen, T. A., Shao, W., Byth, K., et al. (2016). Broad activation of latent HIV-1 in vivo. Nat. Commun. 7:12731. doi: 10.1038/ncomms12731
Berg, S. L., Stone, J., Xiao, J. J., Chan, K. K., Nuchtern, J., Dauser, R., et al. (2004). Plasma and cerebrospinal fluid pharmacokinetics of depsipeptide (FR901228) in nonhuman primates. Cancer Chemother. Pharmacol. 54, 85–88. doi: 10.1007/s00280-004-0766-5
Blackard, J. T. (2012). HIV compartmentalization: a review on a clinically important phenomenon. Curr. HIV Res. 10, 133–142. doi: 10.2174/157016212799937245
Brenchley, J. M., Price, D. A., Schacker, T. W., Asher, T. E., Silvestri, G., Rao, S., et al. (2006). Microbial translocation is a cause of systemic immune activation in chronic HIV infection. Nat. Med. 12, 1365–1371.
Brenchley, J. M., Schacker, T. W., Ruff, L. E., Price, D. A., Taylor, J. H., Beilman, G. J., et al. (2004). CD4+ T cell depletion during all stages of HIV disease occurs predominantly in the gastrointestinal tract. J. Exp. Med. 200, 749–759. doi: 10.1084/jem.20040874
Brinkmann, C. R., Hojen, J. F., Rasmussen, T. A., Kjaer, A. S., Olesen, R., Denton, P. W., et al. (2018). Treatment of HIV-infected individuals with the histone deacetylase inhibitor panobinostat results in increased numbers of regulatory t cells and limits ex vivo lipopolysaccharide-induced inflammatory responses. mSphere 3:e00616-17. doi: 10.1128/mSphere.00616-17
Caskey, M., Klein, F., and Nussenzweig, M. C. (2019). Broadly neutralizing anti-HIV-1 monoclonal antibodies in the clinic. Nat. Med. 25, 547–553. doi: 10.1038/s41591-019-0412-8
Chege, D., Sheth, P. M., Kain, T., Kim, C. J., Kovacs, C., Loutfy, M., et al. (2011). Sigmoid Th17 populations, the HIV latent reservoir, and microbial translocation in men on long-term antiretroviral therapy. AIDS 25, 741–749. doi: 10.1097/QAD.0b013e328344cefb
Christensen, A. B., Dige, A., Vad-Nielsen, J., Brinkmann, C. R., Bendix, M., Ostergaard, L., et al. (2015). Administration of panobinostat is associated with increased IL-17A mRNA in the intestinal epithelium of HIV-1 patients. Mediators Inflamm. 2015:120605. doi: 10.1155/2015/120605
Chun, T. W., Nickle, D. C., Justement, J. S., Meyers, J. H., Roby, G., Hallahan, C. W., et al. (2008). Persistence of HIV in gut-associated lymphoid tissue despite long-term antiretroviral therapy. J. Infect. Dis. 197, 714–720. doi: 10.1086/527324
Churchill, M. J., Wesselingh, S. L., Cowley, D., Pardo, C. A., Mcarthur, J. C., Brew, B. J., et al. (2009). Extensive astrocyte infection is prominent in human immunodeficiency virus-associated dementia. Ann. Neurol. 66, 253–258. doi: 10.1002/ana.21697
Ciccone, E. J., Read, S. W., Mannon, P. J., Yao, M. D., Hodge, J. N., Dewar, R., et al. (2010). Cycling of gut mucosal CD4+ T cells decreases after prolonged anti-retroviral therapy and is associated with plasma LPS levels. Mucosal Immunol. 3, 172–181. doi: 10.1038/mi.2009.129
Clements, J. E., Li, M., Gama, L., Bullock, B., Carruth, L. M., Mankowski, J. L., et al. (2005). The central nervous system is a viral reservoir in simian immunodeficiency virus–infected macaques on combined antiretroviral therapy: a model for human immunodeficiency virus patients on highly active antiretroviral therapy. J. Neurovirol. 11, 180–189. doi: 10.1080/13550280590922829
Costiniuk, C. T., and Jenabian, M. A. (2014). The lungs as anatomical reservoirs of HIV infection. Rev. Med. Virol. 24, 35–54. doi: 10.1002/rmv.1772
Deeks, S. G., Lewin, S. R., Ross, A. L., Ananworanich, J., Benkirane, M., Cannon, P., et al. (2016). International AIDS Society global scientific strategy: towards an HIV cure 2016. Nat. Med. 22, 839–850. doi: 10.1038/nm.4108
Deere, J. D., Kauffman, R. C., Cannavo, E., Higgins, J., Villalobos, A., Adamson, L., et al. (2014). Analysis of multiply spliced transcripts in lymphoid tissue reservoirs of rhesus macaques infected with RT-SHIV during HAART. PLoS One 9:e87914. doi: 10.1371/journal.pone.0087914
Del Prete, G. Q., Shoemaker, R., Oswald, K., Lara, A., Trubey, C. M., Fast, R., et al. (2014). Effect of suberoylanilide hydroxamic acid (SAHA) administration on the residual virus pool in a model of combination antiretroviral therapy-mediated suppression in SIVmac239-infected indian rhesus macaques. Antimicrob. Agents Chemother. 58, 6790–6806. doi: 10.1128/AAC.03746-14
Deleage, C., Wietgrefe, S. W., Del Prete, G., Morcock, D. R., Hao, X. P., Piatak, M., et al. (2016). Defining HIV and SIV reservoirs in lymphoid tissues. Pathog. Immun. 1, 68–106.
Denton, P. W., Sogaard, O. S., and Tolstrup, M. (2016). Using animal models to overcome temporal, spatial and combinatorial challenges in HIV persistence research. J. Transl. Med. 14:44. doi: 10.1186/s12967-016-0807-y
Dinoso, J. B., Rabi, S. A., Blankson, J. N., Gama, L., Mankowski, J. L., Siliciano, R. F., et al. (2009). A simian immunodeficiency virus-infected macaque model to study viral reservoirs that persist during highly active antiretroviral therapy. J. Virol. 83, 9247–9257. doi: 10.1128/JVI.00840-09
Elliott, J. H., Mcmahon, J. H., Chang, C. C., Lee, S. A., Hartogensis, W., Bumpus, N., et al. (2015). Short-term administration of disulfiram for reversal of latent HIV infection: a phase 2 dose-escalation study. Lancet HIV 2, e520–e529. doi: 10.1016/S2352-3018(15)00226-X
Elliott, J. H., Wightman, F., Solomon, A., Ghneim, K., Ahlers, J., Cameron, M. J., et al. (2014). Activation of HIV transcription with short-course vorinostat in HIV-infected patients on suppressive antiretroviral therapy. PLoS Pathog. 10:e1004473. doi: 10.1371/journal.ppat.1004473
Estes, J. D., Kityo, C., Ssali, F., Swainson, L., Makamdop, K. N., Del Prete, G. Q., et al. (2017). Defining total-body AIDS-virus burden with implications for curative strategies. Nat. Med. 23, 1271–1276. doi: 10.1038/nm.4411
Evering, T. H., Mehandru, S., Racz, P., Tenner-Racz, K., Poles, M. A., Figueroa, A., et al. (2012). Absence of HIV-1 evolution in the gut-associated lymphoid tissue from patients on combination antiviral therapy initiated during primary infection. PLoS Pathog. 8:e1002506. doi: 10.1371/journal.ppat.1002506
Fukazawa, Y., Lum, R., Okoye, A. A., Park, H., Matsuda, K., Bae, J. Y., et al. (2015). B cell follicle sanctuary permits persistent productive simian immunodeficiency virus infection in elite controllers. Nat. Med. 21, 132–139. doi: 10.1038/nm.3781
Garrido, C., Tolstrup, M., Sogaard, O. S., Rasmussen, T. A., Allard, B., Soriano-Sarabia, N., et al. (2019). In-vivo administration of histone deacetylase inhibitors does not impair natural killer cell function in HIV+ individuals. AIDS 33, 605–613. doi: 10.1097/qad.0000000000002112
Gray, L. R., On, H., Roberts, E., Lu, H. K., Moso, M. A., Raison, J. A., et al. (2016). Toxicity and in vitro activity of HIV-1 latency-reversing agents in primary CNS cells. J. Neurovirol. 22, 455–463. doi: 10.1007/s13365-015-0413-4
Gray, L. R., Roche, M., Flynn, J. K., Wesselingh, S. L., Gorry, P. R., and Churchill, M. J. (2014). Is the central nervous system a reservoir of HIV-1? Curr. Opin. HIV AIDS 9, 552–558. doi: 10.1097/COH.0000000000000108
Guadalupe, M., Reay, E., Sankaran, S., Prindiville, T., Flamm, J., Mcneil, A., et al. (2003). Severe CD4+ T-cell depletion in gut lymphoid tissue during primary human immunodeficiency virus type 1 infection and substantial delay in restoration following highly active antiretroviral therapy. J. Virol. 77, 11708–11717. doi: 10.1128/jvi.77.21.11708-11717.2003
Gutierrez, C., Serrano-Villar, S., Madrid-Elena, N., Perez-Elias, M. J., Martin, M. E., Barbas, C., et al. (2016). Bryostatin-1 for latent virus reactivation in HIV-infected patients on antiretroviral therapy. AIDS 30, 1385–1392. doi: 10.1097/QAD.0000000000001064
Hogh Kolbaek Kjaer, A. S., Brinkmann, C. R., Dinarello, C. A., Olesen, R., Ostergaard, L., Sogaard, O. S., et al. (2015). The histone deacetylase inhibitor panobinostat lowers biomarkers of cardiovascular risk and inflammation in HIV patients. AIDS 29, 1195–1200. doi: 10.1097/QAD.0000000000000678
Honeycutt, J. B., and Garcia, J. V. (2018). Humanized mice: models for evaluating NeuroHIV and cure strategies. J. Neurovirol. 24, 185–191. doi: 10.1007/s13365-017-0567-3
Honeycutt, J. B., Liao, B., Nixon, C. C., Cleary, R. A., Thayer, W. O., Birath, S. L., et al. (2018). T cells establish and maintain CNS viral infection in HIV-infected humanized mice. J. Clin. Invest. 128, 2862–2876. doi: 10.1172/JCI98968
Honeycutt, J. B., Thayer, W. O., Baker, C. E., Ribeiro, R. M., Lada, S. M., Cao, Y., et al. (2017). HIV persistence in tissue macrophages of humanized myeloid-only mice during antiretroviral therapy. Nat. Med. 23, 638–643. doi: 10.1038/nm.4319
Horiike, M., Iwami, S., Kodama, M., Sato, A., Watanabe, Y., Yasui, M., et al. (2012). Lymph nodes harbor viral reservoirs that cause rebound of plasma viremia in SIV-infected macaques upon cessation of combined antiretroviral therapy. Virology 423, 107–118. doi: 10.1016/j.virol.2011.11.024
Jorgensen, S., Leth, S., Sommerfelt, M., Ostergaard, L., Tolstrup, M., and Sogaard, O. S. (2018). Differences in antiretroviral regimens do not impact safety or level of latency reversal in persons receiving romidepsin. AIDS 32, 1729–1731. doi: 10.1097/qad.0000000000001904
Kline, C., Ndjomou, J., Franks, T., Kiser, R., Coalter, V., Smedley, J., et al. (2013). Persistence of viral reservoirs in multiple tissues after antiretroviral therapy suppression in a macaque RT-SHIV model. PLoS One 8:e84275. doi: 10.1371/journal.pone.0084275
Krarup, A. R., Abdel-Mohsen, M., Schleimann, M. H., Vibholm, L., Engen, P. A., Dige, A., et al. (2017). The TLR9 agonist MGN1703 triggers a potent type I interferon response in the sigmoid colon. Mucosal Immunol. 11, 449–461. doi: 10.1038/mi.2017.59
Lamers, S. L., Rose, R., Maidji, E., Agsalda-Garcia, M., Nolan, D. J., Fogel, G. B., et al. (2016). HIV DNA is frequently present within pathologic tissues evaluated at autopsy from combined antiretroviral therapy-treated patients with undetectable viral loads. J. Virol. 90, 8968–8983. doi: 10.1128/JVI.00674-16
Lee, S. A., Elliott, J. H., Mcmahon, J., Hartogenesis, W., Bumpus, N. N., Lifson, J. D., et al. (2019). Population pharmacokinetics and pharmacodynamics of disulfiram on inducing latent HIV-1 transcription in a phase IIb trial. Clin. Pharmacol. Ther. 105, 692–702. doi: 10.1002/cpt.1220
Lee, W. S., Kristensen, A. B., Rasmussen, T. A., Tolstrup, M., Ostergaard, L., Sogaard, O. S., et al. (2017). Anti-HIV-1 ADCC antibodies following latency reversal and treatment interruption. J. Virol. 91:e00603-17. doi: 10.1128/JVI.00603-17
Lerner, P., Guadalupe, M., Donovan, R., Hung, J., Flamm, J., Prindiville, T., et al. (2011). The gut mucosal viral reservoir in HIV-infected patients is not the major source of rebound plasma viremia following interruption of highly active antiretroviral therapy. J. Virol. 85, 4772–4782. doi: 10.1128/jvi.02409-10
Leth, S., Schleimann, M. H., Nissen, S. K., Hojen, J. F., Olesen, R., Graversen, M. E., et al. (2016). Combined effect of Vacc-4x, recombinant human granulocyte macrophage colony-stimulating factor vaccination, and romidepsin on the HIV-1 reservoir (REDUC): a single-arm, phase 1B/2A trial. Lancet HIV 3, e463–e472. doi: 10.1016/S2352-3018(16)30055-8
Li, Q., Duan, L., Estes, J. D., Ma, Z. M., Rourke, T., Wang, Y., et al. (2005). Peak SIV replication in resting memory CD4+ T cells depletes gut lamina propria CD4+ T cells. Nature 434, 1148–1152. doi: 10.1038/nature03513
Liang, S. C., Tan, X. Y., Luxenberg, D. P., Karim, R., Dunussi-Joannopoulos, K., Collins, M., et al. (2006). Interleukin (IL)-22 and IL-17 are coexpressed by Th17 cells and cooperatively enhance expression of antimicrobial peptides. J. Exp. Med. 203, 2271–2279. doi: 10.1084/jem.20061308
Lorenzo-Redondo, R., Fryer, H. R., Bedford, T., Kim, E. Y., Archer, J., Kosakovsky Pond, S. L., et al. (2016). Persistent HIV-1 replication maintains the tissue reservoir during therapy. Nature 530, 51–56. doi: 10.1038/nature16933
Lu, C. L., Pai, J. A., Nogueira, L., Mendoza, P., Gruell, H., Oliveira, T. Y., et al. (2018). Relationship between intact HIV-1 proviruses in circulating CD4(+) T cells and rebound viruses emerging during treatment interruption. Proc. Natl. Acad. Sci. U.S.A. 115, E11341–E11348. doi: 10.1073/pnas.1813512115
Margolis, D. M., Garcia, J. V., Hazuda, D. J., and Haynes, B. F. (2016). Latency reversal and viral clearance to cure HIV-1. Science 353:aaf6517. doi: 10.1126/science.aaf6517
Mattapallil, J. J., Douek, D. C., Hill, B., Nishimura, Y., Martin, M., and Roederer, M. (2005). Massive infection and loss of memory CD4+ T cells in multiple tissues during acute SIV infection. Nature 434, 1093–1097. doi: 10.1038/nature03501
Mehandru, S., Poles, M. A., Tenner-Racz, K., Horowitz, A., Hurley, A., Hogan, C., et al. (2004). Primary HIV-1 infection is associated with preferential depletion of CD4+ T lymphocytes from effector sites in the gastrointestinal tract. J. Exp. Med. 200, 761–770. doi: 10.1084/jem.20041196
Micci, L., Mcgary, C. S., and Paiardini, M. (2015). Animal models in HIV cure research. J. Virus Erad. 1, 17–22.
Mota, T. M., Rasmussen, T. A., Rhodes, A., Tennakoon, S., Dantanarayana, A., Wightman, F., et al. (2017). No adverse safety or virological changes 2 years following vorinostat in HIV-infected individuals on antiretroviral therapy. AIDS 31, 1137–1141. doi: 10.1097/QAD.0000000000001442
Mothe, B., Climent, N., Plana, M., Rosas, M., Jimenez, J. L., Munoz-Fernandez, M. A., et al. (2015). Safety and immunogenicity of a modified vaccinia Ankara-based HIV-1 vaccine (MVA-B) in HIV-1-infected patients alone or in combination with a drug to reactivate latent HIV-1. J. Antimicrob. Chemother. 70, 1833–1842. doi: 10.1093/jac/dkv046
Nixon, C. C., Mavigner, M., Silvestri, G., and Garcia, J. V. (2017). In vivo models of human immunodeficiency virus persistence and cure strategies. J. Infect. Dis. 215, S142–S151. doi: 10.1093/infdis/jiw637
Nolan, D. J., Rose, R., Rodriguez, P. H., Salemi, M., Singer, E. J., Lamers, S. L., et al. (2018). The spleen is an HIV-1 sanctuary during combined antiretroviral therapy. AIDS Res. Hum. Retroviruses 34, 123–125. doi: 10.1089/AID.2017.0254
North, T. W., Higgins, J., Deere, J. D., Hayes, T. L., Villalobos, A., Adamson, L., et al. (2010). Viral sanctuaries during highly active antiretroviral therapy in a nonhuman primate model for AIDS. J. Virol. 84, 2913–2922. doi: 10.1128/JVI.02356-09
Olesen, R., Vigano, S., Rasmussen, T. A., Sogaard, O. S., Ouyang, Z., Buzon, M., et al. (2015). Innate immune activity correlates with CD4 T cell-associated HIV-1 DNA decline during latency-reversing treatment with panobinostat. J. Virol. 89, 10176–10189. doi: 10.1128/JVI.01484-15
Policicchio, B. B., Pandrea, I., and Apetrei, C. (2016). Animal models for HIV cure research. Front. Immunol. 7:12. doi: 10.3389/fimmu.2016.00012
Queen, S. E., Mears, B. M., Kelly, K. M., Dorsey, J. L., Liao, Z., Dinoso, J. B., et al. (2011). Replication-competent simian immunodeficiency virus (SIV) gag escape mutations archived in latent reservoirs during antiretroviral treatment of SIV-infected macaques. J. Virol. 85, 9167–9175. doi: 10.1128/JVI.00366-11
Rasmussen, T. A., and Sogaard, O. S. (2018). Clinical interventions in HIV cure research. Adv. Exp. Med. Biol. 1075, 285–318. doi: 10.1007/978-981-13-0484-2_12
Rasmussen, T. A., Tolstrup, M., Brinkmann, C. R., Olesen, R., Erikstrup, C., Solomon, A., et al. (2014). Panobinostat, a histone deacetylase inhibitor, for latent-virus reactivation in HIV-infected patients on suppressive antiretroviral therapy: a phase 1/2, single group, clinical trial. Lancet HIV 1, e13–e21. doi: 10.1016/S2352-3018(14)70014-1
Rasmussen, T. A., Tolstrup, M., Moller, H. J., Brinkmann, C. R., Olesen, R., Erikstrup, C., et al. (2015). Activation of latent human immunodeficiency virus by the histone deacetylase inhibitor panobinostat: a pilot study to assess effects on the central nervous system. Open Forum Infect. Dis. 2:ofv037. doi: 10.1093/ofid/ofv037
Ritchie, A. J., Cai, F., Smith, N. M., Chen, S., Song, H., Brackenridge, S., et al. (2014). Recombination-mediated escape from primary CD8+ T cells in acute HIV-1 infection. Retrovirology 11:69. doi: 10.1186/s12977-014-0069-9
Rose, R., Lamers, S. L., Nolan, D. J., Maidji, E., Faria, N. R., Pybus, O. G., et al. (2016). HIV maintains an evolving and dispersed population in multiple tissues during suppressive combined antiretroviral therapy in individuals with cancer. J. Virol. 90, 8984–8993. doi: 10.1128/JVI.00684-16
Rose, R., Nolan, D. J., Maidji, E., Stoddart, C. A., Singer, E. J., Lamers, S. L., et al. (2018). Eradication of HIV from tissue reservoirs: challenges for the cure. AIDS Res. Hum. Retroviruses 34, 3–8. doi: 10.1089/AID.2017.0072
Saxena, M., Sabado, R. L., La Mar, M., Mohri, H., Salazar, A. M., Dong, H., et al. (2019). Poly-ICLC, a tlr3 agonist, induces transient innate immune responses in patients with treated HIV-Infection: a randomized double-blinded placebo controlled trial. Front. Immunol. 10:725. doi: 10.3389/fimmu.2019.00725
Schleimann, M. H., Kobberø, M. L., Vibholm, L. K., Kjaer, K., Giron, L. B., Busman-Sahay, K., et al. (2019). TLR9 agonist MGN1703 enhances B cell differentiation and function in lymph nodes. EBioMedicine 45, 328–340. doi: 10.1016/j.ebiom.2019.07.005
Shen, A., Zink, M. C., Mankowski, J. L., Chadwick, K., Margolick, J. B., Carruth, L. M., et al. (2003). Resting CD4+ T lymphocytes but not thymocytes provide a latent viral reservoir in a simian immunodeficiency virus-Macaca nemestrina model of human immunodeficiency virus type 1-infected patients on highly active antiretroviral therapy. J. Virol. 77, 4938–4949. doi: 10.1128/jvi.77.8.4938-4949.2003
Søgaard, O. S., Graversen, M. E., Leth, S., Olesen, R., Brinkmann, C. R., Nissen, S. K., et al. (2015). The depsipeptide romidepsin reverses HIV-1 latency in vivo. PLoS Pathog. 11:e1005142. doi: 10.1371/journal.ppat.1005142
Spivak, A. M., Andrade, A., Eisele, E., Hoh, R., Bacchetti, P., Bumpus, N. N., et al. (2014). A pilot study assessing the safety and latency-reversing activity of disulfiram in HIV-1-infected adults on antiretroviral therapy. Clin. Infect. Dis. 58, 883–890. doi: 10.1093/cid/cit813
Streeck, H., Li, B., Poon, A. F., Schneidewind, A., Gladden, A. D., Power, K. A., et al. (2008). Immune-driven recombination and loss of control after HIV superinfection. J. Exp. Med. 205, 1789–1796. doi: 10.1084/jem.20080281
Tapia, G., Hojen, J. F., Okvist, M., Olesen, R., Leth, S., Nissen, S. K., et al. (2017). Sequential Vacc-4x and romidepsin during combination antiretroviral therapy (cART): immune responses to Vacc-4x regions on p24 and changes in HIV reservoirs. J. Infect. 75, 555–571. doi: 10.1016/j.jinf.2017.09.004
van Marle, G., Gill, M. J., Kolodka, D., Mcmanus, L., Grant, T., and Church, D. L. (2007). Compartmentalization of the gut viral reservoir in HIV-1 infected patients. Retrovirology 4:87. doi: 10.1186/1742-4690-4-87
Vibholm, L., Schleimann, M. H., Hojen, J. F., Benfield, T., Offersen, R., Rasmussen, K., et al. (2017). Short-course toll-like receptor 9 agonist treatment impacts innate immunity and plasma viremia in individuals with human immunodeficiency virus infection. Clin. Infect. Dis. 64, 1686–1695. doi: 10.1093/cid/cix201
Vibholm, L. K., Konrad, C. V., Schleimann, M. H., Frattari, G., Winckelmann, A., Klastrup, V., et al. (2019a). Effects of 24 week toll-like receptor 9 agonist treatment in HIV-1+ individuals: a single-arm, phase 1B/2A trial. AIDS 33, 1315–1325. doi: 10.1097/QAD.0000000000002213
Vibholm, L. K., Lorenzi, J. C. C., Pai, J. A., Cohen, Y. Z., Oliveira, T. Y., Barton, J. P., et al. (2019b). Characterization of intact proviruses in blood and lymph node from HIV-infected individuals undergoing analytical treatment interruption. J. Virol. 93, e1920–18. doi: 10.1128/JVI.01920-18
Whitney, J. B., and Brad Jones, R. (2018). In vitro and in vivo models of HIV latency. Adv. Exp. Med. Biol. 1075, 241–263. doi: 10.1007/978-981-13-0484-2_10
Winckelmann, A., Barton, K., Hiener, B., Schlub, T. E., Shao, W., Rasmussen, T. A., et al. (2017). Romidepsin-induced HIV-1 viremia during effective antiretroviral therapy contains identical viral sequences with few deleterious mutations. AIDS 31, 771–779. doi: 10.1097/QAD.0000000000001400
Winckelmann, A., Morcilla, V., Shao, W., Schleimann, M. H., Hojen, J. F., Schlub, T. E., et al. (2018). Genetic characterization of the HIV-1 reservoir after Vacc-4x and romidepsin therapy in HIV-1 infected individuals. AIDS 32, 1793–1802. doi: 10.1097/QAD.0000000000001861
Wong, J. K., and Yukl, S. A. (2016). Tissue reservoirs of HIV. Curr. Opin. HIV AIDS 11, 362–370. doi: 10.1097/coh.0000000000000293
Wu, G., Swanson, M., Talla, A., Graham, D., Strizki, J., Gorman, D., et al. (2017). HDAC inhibition induces HIV-1 protein and enables immune-based clearance following latency reversal. JCI Insight 2:e92901. doi: 10.1172/jci.insight.92901
Yukl, S. A., Gianella, S., Sinclair, E., Epling, L., Li, Q., Duan, L., et al. (2010a). Differences in HIV burden and immune activation within the gut of HIV-positive patients receiving suppressive antiretroviral therapy. J. Infect. Dis. 202, 1553–1561. doi: 10.1086/656722
Yukl, S. A., Shergill, A. K., Mcquaid, K., Gianella, S., Lampiris, H., Hare, C. B., et al. (2010b). Effect of raltegravir-containing intensification on HIV burden and T-cell activation in multiple gut sites of HIV-positive adults on suppressive antiretroviral therapy. AIDS 24, 2451–2460. doi: 10.1097/QAD.0b013e32833ef7bb
Zink, M. C., Brice, A. K., Kelly, K. M., Queen, S. E., Gama, L., Li, M., et al. (2010). Simian immunodeficiency virus-infected macaques treated with highly active antiretroviral therapy have reduced central nervous system viral replication and inflammation but persistence of viral DNA. J. Infect. Dis. 202, 161–170. doi: 10.1086/653213
Keywords: HIV cure, viral persistence, latency, tissues, interventional clinical trials
Citation: Denton PW, Søgaard OS and Tolstrup M (2019) Impacts of HIV Cure Interventions on Viral Reservoirs in Tissues. Front. Microbiol. 10:1956. doi: 10.3389/fmicb.2019.01956
Received: 02 July 2019; Accepted: 08 August 2019;
Published: 21 August 2019.
Edited by:
Maria J. Buzon, Vall d’Hebron Research Institute (VHIR), SpainReviewed by:
Alberto Bosque, George Washington University, United StatesMaria Salgado, IrsiCaixa, Spain
Copyright © 2019 Denton, Søgaard and Tolstrup. This is an open-access article distributed under the terms of the Creative Commons Attribution License (CC BY). The use, distribution or reproduction in other forums is permitted, provided the original author(s) and the copyright owner(s) are credited and that the original publication in this journal is cited, in accordance with accepted academic practice. No use, distribution or reproduction is permitted which does not comply with these terms.
*Correspondence: Paul W. Denton, pdenton@unomaha.edu