- 1State Key Laboratory of Animal Nutrition, China Agricultural University, Beijing, China
- 2Ministry of Agriculture and Rural Affairs Feed Industry Centre, China Agricultural University, Beijing, China
- 3Beijing Key Laboratory of Bio-Feed Additives, Beijing, China
Natural microcin J25 (MccJ25) represent promising alternatives to traditional antibiotics for the treatment of drug-resistant infections. However, little is known about the antibacterial activity of recombinant MccJ25 against foodborne pathogens. Here, the activity of recombinant MccJ25 was examined using a matrix of conditions in order to assess the efficacy of recombinant MccJ25 as a mitigation against foodborne pathogens, such as Salmonella species and Escherichia coli (E. coli) O157:H7. Results showed that recombinant MccJ25 displayed excellent antimicrobial activity against these foodborne pathogens, including clinical isolates of Salmonella and E. coli, as well as clinical antibiotic-resistant Salmonella and E. coli isolates with different minimal inhibitory concentrations. In addition, antimicrobial activity curves and Live/Dead assay evidenced that recombinant MccJ25 harbors strong bactericidal activity against Salmonella and E. coli O157:H7. Notably, recombinant MccJ25 also had great potency and induced fast mortality against different growth phase of Salmonella and E. coli. The stability analysis results showed that the activity of recombinant MccJ25 was not influenced by temperatures as high as 121°C. Varying the pH from 2.0 to 9.0 did not appear to affect the activity of recombinant MccJ25. Under the challenge of several proteases, simulated gastrointestinal fluids and serum, recombinant MccJ25 still maintained exceptionally strong antimicrobial activity. Significant reductions in Salmonella Pullorum levels were also achieved in food biological environments, such as milk, egg and meat. Moreover, we demonstrated that recombinant MccJ25 appeared to act by inducing membrane breaks, thinning, and disintegration in the Salmonella Pullorum cytoplasmic membrane. Taken together, these results indicated that recombinant MccJ25 could be an effective alternative for mitigating and prevention of Salmonella and E. coli infection in food, animal and agriculture applications.
Introduction
The most notorious and common pathogenic Escherichia coli (E. coli) O157:H7, Salmonella Typhimurium and Salmonella Pullorum strains are primary enteric pathogens that not only inhibit human and animal health but also cause food poisoning and food-related epidemics around the world (Sable et al., 2000; Ma et al., 2016; Fan et al., 2017). Especially, fresh produce, such as milk, meat, and egg, is increasingly related to the incidence of foodborne illness, and outbreaks associated with fresh produce consumption by human or animals have risen substantially recently (Centers for Disease Control and Prevention [CDC], 2010, 2013). As reported, from 1990 to 2005, around 800 outbreaks sickened over 34,000 people because of produce-associated foodborne illness (DeWaal and Bhuiya, 2007).
Contaminated food and water are possible sources of produce-related disease transmission because food and water resources can serve as natural reservoirs for Salmonella and E. coli (Black, 1990; Rajabi et al., 2011). It is estimated that over 50% of produce-related illnesses are caused by Salmonella and E. coli, which is the leading cause of both hospitalizations and deaths associated with bacterial foodborne illness (Centers for Disease Control and Prevention [CDC], 2011). In terms of controlling food or food processing water quality is needed to ensure the safety of human and animals. Commonly used antibiotics are now unsuccessful (Wlodarska et al., 2011; Cho et al., 2012; Rabanal et al., 2015) and are challenged by antibiotic-resistant pathogens (Moreno et al., 1995; Falagas et al., 2012; Penders et al., 2013). Therefore, there is an urgent need to encourage the produce industry to find more efficient and less toxic antimicrobial agents alternatives for treating bacterial infections, particularly those caused by foodborne pathogens, and to ensure human health, food safety, and animal health.
Nature AMP MccJ25 has attracted much attention as a potential agent for both humans and animals and as a food preservative due to its strong antimicrobial activity in vitro and in vivo (Black, 1990; Sassone-Corsi et al., 2016; Forkus et al., 2017). But, in recent years, chemical synthesis of AMPs is extreme costly and is not scalable for large-scale AMP production. Recombinant expression was always used to produce AMPs in many laboratories (Cao et al., 2018; Wei et al., 2018; Kim et al., 2019; Meng et al., 2019). Recombinant AMP MccJ25 can be large-scale produced by an MccJ25 expression vector and exhibit antimicrobial activity. For example, recombinant AMP MccJ25 exerted significant antimicrobial activity against Salmonella, Shigella, and E. coli (Yu et al., 2018a). Additionally, recombinant AMP MccJ25 was shown to effectively reduce E. coli in live piglets (Yu et al., 2017) and in healthy mice (Yu et al., 2018b). Recombinant AMP MccJ25 also did not cause toxic risk in vivo or in vitro (Yu et al., 2018a, b).
The effects of recombinant AMP MccJ25 on ETEC K88 were examined previously, but this research did not determine efficacy for different conditions and strains (Yu et al., 2018a). Additionally, the mode of action, antimicrobial properties in different biological or incubation conditions, and potential use of recombinant MccJ25 against S. Pullorum remains unclear. Therefore, it is critical for us to investigate the comprehensive antimicrobial activity of recombinant AMP in different environments. In the present study, we examined the anti-Salmonella and E. coli O157:H7 activity of recombinant AMP MccJ25 for various environmental conditions in order to evaluate its potential as an antimicrobial agent in food, agricultural, and animal practices. This assessment was performed to evaluate its potential as an antimicrobial agent for clinical studies and commercial use to treat bacterial infections and ensure food safety and animal health.
Materials and Methods
Preparation of Recombinant MccJ25
Recombinant MccJ25 was expressed and purified as has been described previously (Yu et al., 2018a), with minor modifications. A recombinant expression vector pMJ25 was constructed according to our lab standard protocol. Then, the recombinant expression vector was transformed into chemically competent E. coli BL21. The recombinant strain, E. coli BL21, was inoculated into a sucrose compound-based medium supplemented with the antibiotics kanamycin and ampicillin in 200 mL shaking flasks at 37°C at 200 rpm for 12 h. After expression, culture supernatants were harvested by centrifugation at 4°C and 12,000 rpm for 10 min. The recombinant MccJ25 was purified by HPLC, and the purity of the recombinant MccJ25 was greater than 99.95%. The recombinant MccJ25 concentrated stock solution was prepared in endotoxin-free water and stored at −20°C until use.
Measurement of Minimum Inhibitory Concentration (MIC) and Minimum Bactericidal Concentration (MBC)
Four standard Salmonella enterica (S. enterica) serotype strains and E. coli O157:H7 are listed in Table 1 and were used to detect activity in Recombinant MccJ25 optimization studies. These strains were obtained from the China Institute of Veterinary Drug Control in Beijing. The recombinant MccJ25 sensitivity of the additional isolates was also examined for the strains listed in Table 2. These strains were obtained from intestinal contents of weaned pigs with diarrhea, diseased poultry and cattle (strains were provided by Beijing Bio-feed Additive Key Laboratory) as well as clinical sources (Dr. Wu Congming, College of Veterinary Medicine, China Agricultural University). The clinical source strains, referred to as CAU, were antibiotic (lincomycin and tilmicosin)-resistant Salmonella and E. coli isolates.
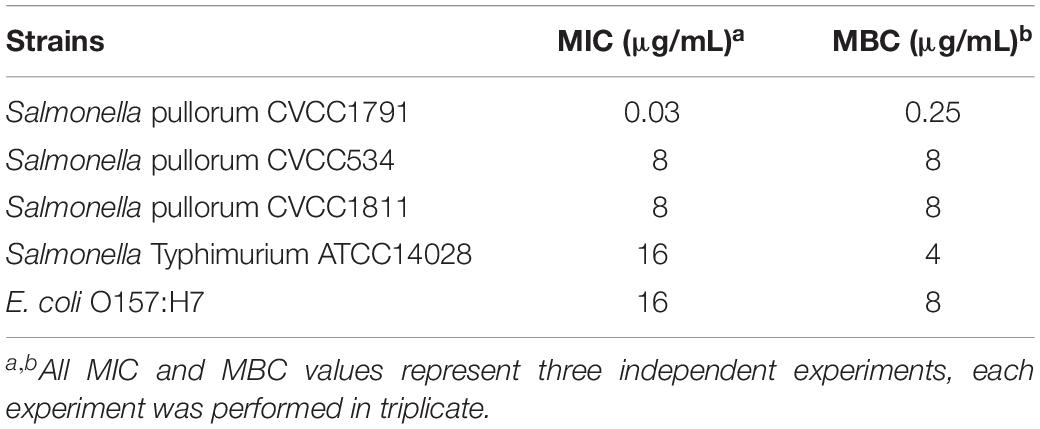
Table 1. Comparison of minimal inhibitory concentration (MIC) and minimum bactericidal concentration (MBC) of recombinant MccJ25 among Salmonella serotypes and E. coli O157:H7.
These strains were stored at −80°C in Luria broth (LB) and were prepared and autoclaved with 45% glycerol. For each experiment, Salmonella and E. coli strains were individually plated onto XLT4 and MacConkey agar (Beijing Land Bridge Technology Co., Beijing, China) for isolation. Single colonies were then inoculated into 10 mL of LB. Cultures were incubated at 37°C with overnight shaking (200 rpm) to reach a stationary phase with approximately 1 × 109 colony-forming units (CFU)/mL. They were subsequently reinoculated into new test tubes with 10 mL of LB at 37°C for the indicated time to obtain 1 × 108 CFU/mL log phase bacteria.
The MIC is described as the lowest concentration of the recombinant MccJ25 capable of preventing visible growth of the bacteria in susceptibility tests by broth dilution, as described previously (CLSI, 2012; Wei et al., 2018; Meng et al., 2019). The MBC is the first drug dilution with a 99.9% reduction in the initial bacterial concentration. MBC was determined in LB medium. Bacterial concentrations were adjusted to approximately 5 × 106 CFU/mL. The concentrations of the recombinant MccJ25 ranged from 0.125 to 256 μg/mL. MIC and MBC were determined from three independent experiments, each experiment was performed in triplicate.
Antimicrobial Activity Curves and Live/Dead Viability Assay
To further address the activity of recombinant MccJ25, antimicrobial activity curves and Live/Dead viability assay were performed. A single colony of S. Pullorum CVCC1791, S. Typhimurium ATCC14082, and E. coli O157:H7 was inoculated into 5 mL of LB. The culture was then incubated at 37°C with shaking at 200 rpm overnight. The following day, the culture was diluted 1:100 in fresh LB and again incubated at 37°C with shaking at 200 rpm. For antimicrobial activity, the bacteria were grown until they reached log phase. Approximately 5 × 104 CFU/mL bacteria were inoculated into 2 mL of LB containing different concentrations of the recombinant MccJ25 (0, 1 × MIC, 2 × MIC, and 4 × MIC). The cultures (0.1 mL) were serially diluted with PBS and plated on LB and TSA agar at 0, 2, 4, and 6 h. The plates were incubated at 37°C overnight to count colony. Colonies were counted to determine CFU/mL. The experiment was conducted with two replicates in three independent trials.
A Live/Dead BacLight Bacterial Viability Kit 7 (Molecular Probes, Inc., Eugene) was used to test bacterial viability. Briefly, 5 × 104 CFU/mL S. Pullorum, S. Typhimurium, and E. coli O157:H7 were inoculated into 1 mL of LB containing recombinant MccJ25 at the MIC level specific for each pathogen. Bacterial cultures were incubated at 37°C for 2 h and then incubated in the dark at ambient temperature with SYTO 9 and propidium iodide for 15 min. Bacteria were observed using a fluorescence microscope (EVOS XL Cell Imaging System).
Antimicrobial Activity of Recombinant MccJ25 Against Pathogens in Different Growth Phases
The effect of growth phase on the anti-Salmonella and -E. coli O157:H7 activity of recombinant MccJ25 was determined by the viable plate counting method, as described previously (Ma et al., 2016; Fan et al., 2017), with minor modifications. Cultures of the strains in the early-log phase, late-log phase and stationary phase were washed, concentrated and diluted with sterilized phosphate-buffered saline (PBS) to approximately 107 CFU/mL. Then, 1 mL was incubated individually in 9 mL of PBS to reach an approximate 106 CFU/mL final concentration. The bacterial cells were then inoculated into 5 mL of LB containing different concentrations of MccJ25 (0, 1 × MIC, 2 × MIC, and 4 × MIC). At 0, 12, 24, and 48 h post incubation, the cultures (0.1 mL) were serially diluted with PBS and plated on LB and TSA agar. The plates were incubated at 37°C overnight to count colony. Colonies were counted to determine CFU/mL. The experiment was conducted with two replicates in three independent trials.
Antimicrobial Activity of Recombinant MccJ25 Against Pathogens in Biological Products
To further investigate the effects of recombinant MccJ25 on Salmonella and E. coli O157:H7 in different biological food products, sterile skim milk, egg yolk and mincemeat (pork) extract were collected and used as described previously with minor modifications (Sable et al., 2000). The skim milk, egg and mincemeat samples were purchased from a Carrefour store in Beijing. Specimens were kept on ice packs in coolers until they reached the laboratory, where they were stored at 4°C. The skim milk (SKM) and mincemeat supernatant (SMS) were autoclave sterilized at 121°C for 20 min. After autoclaving, the SKM and SMS samples were stored at 4°C for further use. The eggs were sterilized with 75% ethanol and transferred into an exceptionally clean bench to collect the egg yolk (SEY). The SEY was diluted 1:1 with sterile distilled water. Biological product samples were mixed with a final concentration of 4 × MIC of recombinant MccJ25 solution in sterile saline water (0.85%). They were then inoculated with S. Pullorum, S. Typhimurium and E. coli O157:H7 at a final concentration of 106 CFU/mL. Sterilized saline water (SSW) with the recombinant MccJ25 was utilized for comparison. This assay was performed to obtain the desired quantity of cells. The mixtures were tested after 24 h of incubation at 37°C with shaking (250 rpm). After incubation, Salmonella species and E. coli O157:H7 were serially diluted with PBS and plated on LB and TSA agar. The plates were incubated at 37°C overnight to count colony. Colonies were counted to determine CFU/mL. The experiment was conducted with two replicates in three independent trials.
The Effect of Temperature and pH on the Anti-Salmonella Activity of Recombinant MccJ25
Assays of the antibacterial activity of recombinant MccJ25 at different temperatures (25, 37, 45, 55, 65, 75, 85, and 95°C for 20 min) and different pH values (pH 2.0, 3.0, 4.0, 5.0, 6.0, 7.0, 8.0, and 9.0 for 2 h) were performed. The assays were conducted as described previously (Chen et al., 2009; Meng et al., 2019), with slight modifications. LB agar inoculated with the indicator bacteria S. Pullorum (approximately 105 viable cells per mL) was poured over plates fitted with Oxford cups to make wells. 150 μL of 1 × MIC final concentration of recombinant MccJ25 samples previously treated under various conditions were added to the wells. The agar overlays were incubated overnight at 37°C, and the inhibition zones were recorded.
Effect of a Single Protease on the Antimicrobial Activity of Recombinant MccJ25 Against Salmonella
The resistance of recombinant MccJ25 to pepsin, trypsin and chymotrypsin was evaluated as described previously (Chen et al., 2009; Meng et al., 2019) with slight modification. The indicator Salmonella was prepared (approximately 1 × 107 CFU/mL), and 1 mL of bacterial inoculum was transferred into 9 mL of each medium with the recombinant MccJ25 and into corresponding control media without the recombinant MccJ25. LB agar inoculated with the indicator bacteria S. Pullorum (approximately 105 viable cells per mL) was poured over plates that had been fitted with Oxford cups to make wells. Then, 150 μL of a 1 × MIC final concentration of recombinant MccJ25 that had been previously treated under various conditions for 2 and 4 h were added to the wells. The agar overlays were incubated overnight at 37°C, and the inhibition zones were recorded.
Determination of Antimicrobial Activity of Recombinant MccJ25 in Biological Conditions
Biological fluids (SGF; SIF) and serum were prepared as described previously (Liu et al., 2013; Xia et al., 2015). Then, assays of the antibacterial activity of recombinant MccJ25 in SGF, SIF and serum were performed. S. Pullorum was grown in Mueller-Hinton broth (MHB, Difco Laboratories, Detroit, MI, United States). One milliliter of bacterial inoculum was transferred into 9 mL of each medium with the recombinant MccJ25 and into corresponding control medium without the recombinant MccJ25. LB agar inoculated with the indicator bacteria S. Pullorum (approximately 105 viable cells per mL) was poured over plates fitted with Oxford cups to make wells. Hundred and fifty μL of a 1 × MIC final concentration of recombinant MccJ25 samples that had previously been treated with SGF, SIF and serum for 2, 4, and 6 h, respectively, were added to the wells. The agar overlays were incubated overnight at 37°C, and the inhibition zones were recorded.
Anti-Salmonella Mechanism Study
To assess the alteration of S. Pullorum cell membrane morphology and ultrastructure, SEM and TEM were performed as described previously, with minor modifications (Wang et al., 2017; Meng et al., 2019). For SEM sample preparation, approximately 2 × 106 S. Pullorum cells were incubated at 37°C for 1 h with the recombinant MccJ25 at 1× and 4 × MIC levels. The control was performed without the recombinant MccJ25. After incubation, the cells were harvested, mixed and centrifuged at 8,000 × g for 3 min. The bacterial pellets were washed three times in PBS and centrifuged at 3,000 × g for 10 min. The bacterial cells were resuspended in 500 μL of PBS and supplemented with 2.5% fresh glutaraldehyde at 4°C overnight. Thereafter, the bacterial pellets were postfixed in 1% osmium tetroxide, dehydrated, and coated with gold-palladium. They were observed by SEM (EVO MA10 XVP, Carl Zeiss).
For TEM sample preparation, (1) two milliliters of 2 × 106 cells of S. Pullorum broth cultured in the absence or presence of 1×, 2× and 4× MIC of the recombinant MccJ25 were incubated and collected at 1 h. (2) After that, approximately 2 × 106 S. Pullorum cells were incubated at 37°C for 1 h with the recombinant MccJ25 at 2 × MIC for 2, 3, and 4 h. They were then centrifuged at 5,000 × g for 5 min. Pretreatment of bacterial samples was conducted in the same way as the SEM treatment. Finally, the samples were placed in capsules containing embedding medium and heated at 70°C for approximately 9 h. Specimens were stained with uranyl acetate and alkaline lead citrate for 15 min. They were then observed by TEM (Model JEM-1230, JEOL, Japan).
Statistical Analysis
Microbiological population results were log transformed for statistical analysis. The results are expressed as the mean ± standard error of the mean (SEM). Data were analyzed by one-way ANOVA with the use of GraphPad Prism 6 (GraphPad Software Inc., San Diego, CA, United States). Tukey’s post hoc test was applied to determine differences between treatment means. All data were visualized using GraphPad Prism 6. Statistical significance was expressed using P < 0.05.
Results
Variability in Recombinant MccJ25 Sensitivity Among Salmonella and E. coli Strains
As shown in Table 1, recombinant MccJ25 activity was tested for four standard Salmonella strains, including the serotypes Pullorum (n = 3) and Typhimurium (n = 1), and for E. coli O157:H7. The results are shown by MIC and MBC. Recombinant MccJ25 was effective and showed the lowest MIC (0.03 μg/mL) and MBC (0.25 μg/mL) against S. Pullorum CVCC1791. In comparison, the MICs and MBCs of recombinant MccJ25 against S. Pullorum CVCC534, S. Pullorum CVCC1811, S. Typhimurium ATCC14028 and E. coli O157:H7 were 8/8, 8/8, 16/4 and 16/8 μg/mL, respectively. Additionally, all pathogens treated with recombinant MccJ25 at various MBC levels (Table 1) were killed. Bactericidal activity is presented as MBC and is defined as eliminating 99.9% of the bacteria of the tested species.
Similar to foodborne E. coli O157:H7, Salmonella is one of the most common pathogens associated with foodborne disease worldwide. Salmonella strains included isolates from clinical sources (n = 8) and are presented in Table 2. Recombinant MccJ25 showed strong antimicrobial activity (low MIC and MBC) against these clinically relevant Salmonella strains. For bactericidal activity, the results showed that most of the pathogens treated with recombinant MccJ25 at different MBC levels (Table 2) were killed. These assays indicated that the recombinant MccJ25 exerted antibacterial and bactericidal activity against the foodborne pathogens Salmonella and E. coli O157:H7.
Antimicrobial Activity of Recombinant MccJ25
To clarify the effectiveness of the antimicrobial activity of recombinant MccJ25, we performed a time-course antimicrobial assay. Killing curves for pathogenic S. Pullorum (Figure 1A), S. Typhimurium (Figure 1B), and E. coli O157:H7 (Figure 1C) in the presence of recombinant MccJ25 were determined. These pathogens were collected every 2 h during incubation to count viable cells for 6 h. Recombinant MccJ25 eliminated the above pathogens after 6 h of incubation when a final concentration of 4 × MIC was applied (Figure 1). However, for the pathogen S. Pullorum (Figure 1A), when the concentration was lowered to 1 × MIC, recombinant MccJ25 killed all bacteria after 4 h and did not exhibit regrowth after 8 h of incubation. Therefore, the Killing curve data confirmed that recombinant MccJ25 has a stronger antimicrobial activity against S. Pullorum than against S. Typhimurium and E. coli O157:H7.
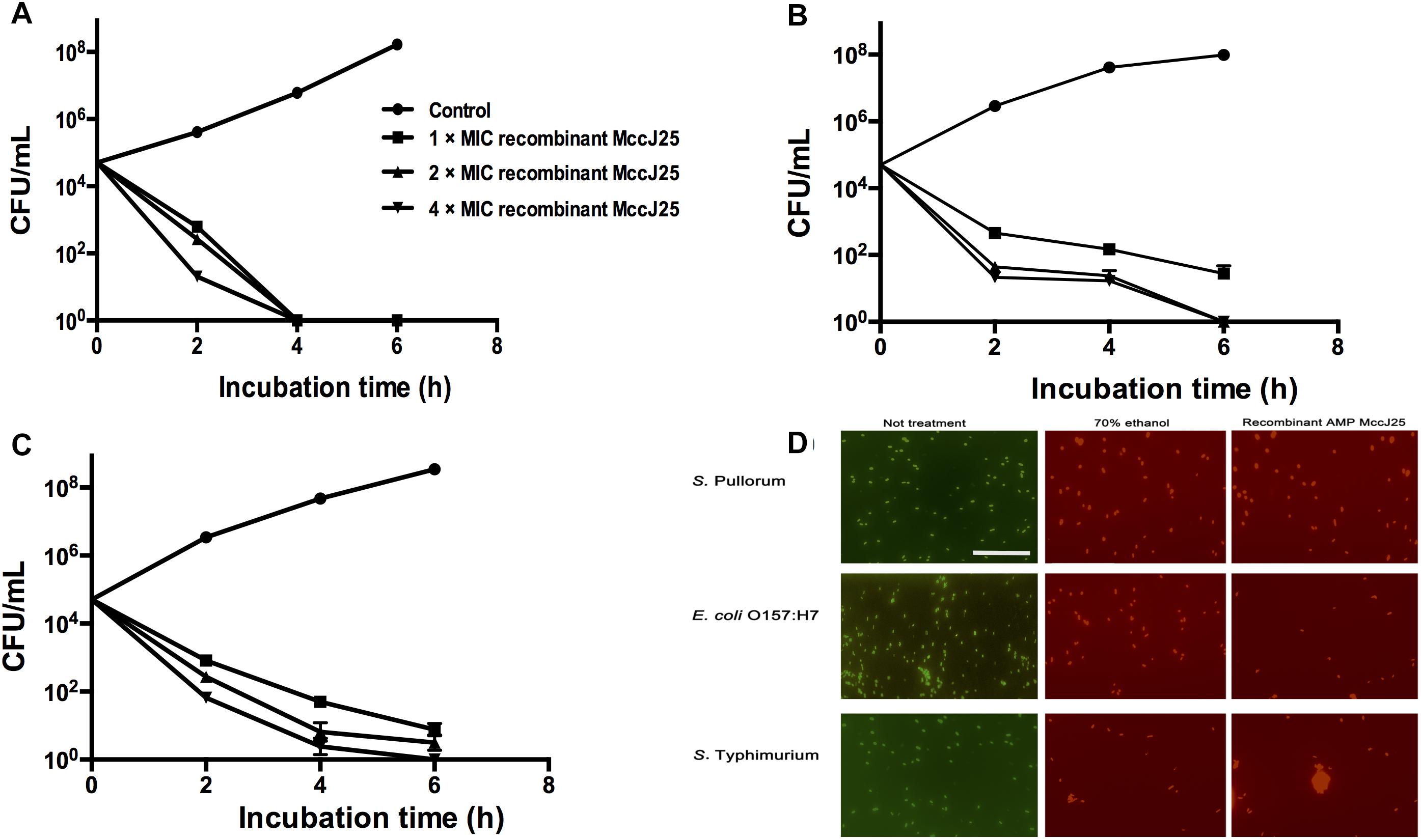
Figure 1. Recombinant MccJ25 kills foodborne pathogens. Killing curves of S. Pullorum (A), E. coli O157:H7 (B), and S. Typhimurium (C) for different concentrations of recombinant MccJ25. Survival of bacteria was compared at 0, 2, 4, and 6 h post inoculation. Viable cells were determined by plate counts, and mean CFU/mL ± SEMs is shown for three individual experiments. n = 6. (D) Fluorescent micrograph of cells treated with 0% recombinant MccJ25 (left), 70% ethanol (middle), or recombinant MccJ25 at the MIC level (right). The white bar indicates 20 μm. The results shown are representative of three independent experiments.
Additionally, to further investigate the bactericidal activity of recombinant MccJ25 against S. Pullorum, S. Typhimurium and E. coli O157:H7, we conducted a Live/Dead antimicrobial assay. As shown in Figure 1D, live bacteria without treatment emit green fluorescence, which are stained with SYTO 9, while dead bacteria treated with 70% ethanol treatment emit red fluorescence, which are stained with propidium iodide, as this dye penetrates into the cytosol through the damaged membranes. Recombinant MccJ25 had excellent bactericidal activity against all challenging microorganisms tested.
The Effects of Recombinant MccJ25 on Different Phase Cultures of Salmonella and E. coli O157:H7
The antimicrobial activity of a range of recombinant MccJ25 concentrations against Salmonella and E. coli O157:H7 was examined at 12, 24, and 48 h at different growth phases, including the early-log phase, late-log phase and stationary phase. Regardless of the bacterial growth phase, recombinant MccJ25 exhibited significant antimicrobial activity against S. Pullorum, S. Typhimurium, and E. coli O157:H7 (Figure 2). S. Pullorum cells at early-log (Figure 2A1), late-log (Figure 2A2), and stationary phase (Figure 2A3) were effectively killed by recombinant MccJ25. Early- or late-log phase cells of S. Pullorum were reduced to undetectable levels within 24 h when treated with 8 μg/mL recombinant MccJ25 and by 12 h when treated with 4 × MIC of recombinant MccJ25 (Figures 2A1,A2). Moreover, there was a reduction in stationary phase cells of S. Pullorum to non-detectable levels within 24 h at concentrations of 16 μg/mL recombinant MccJ25 and by 48 h with 8 μg/mL recombinant MccJ25.
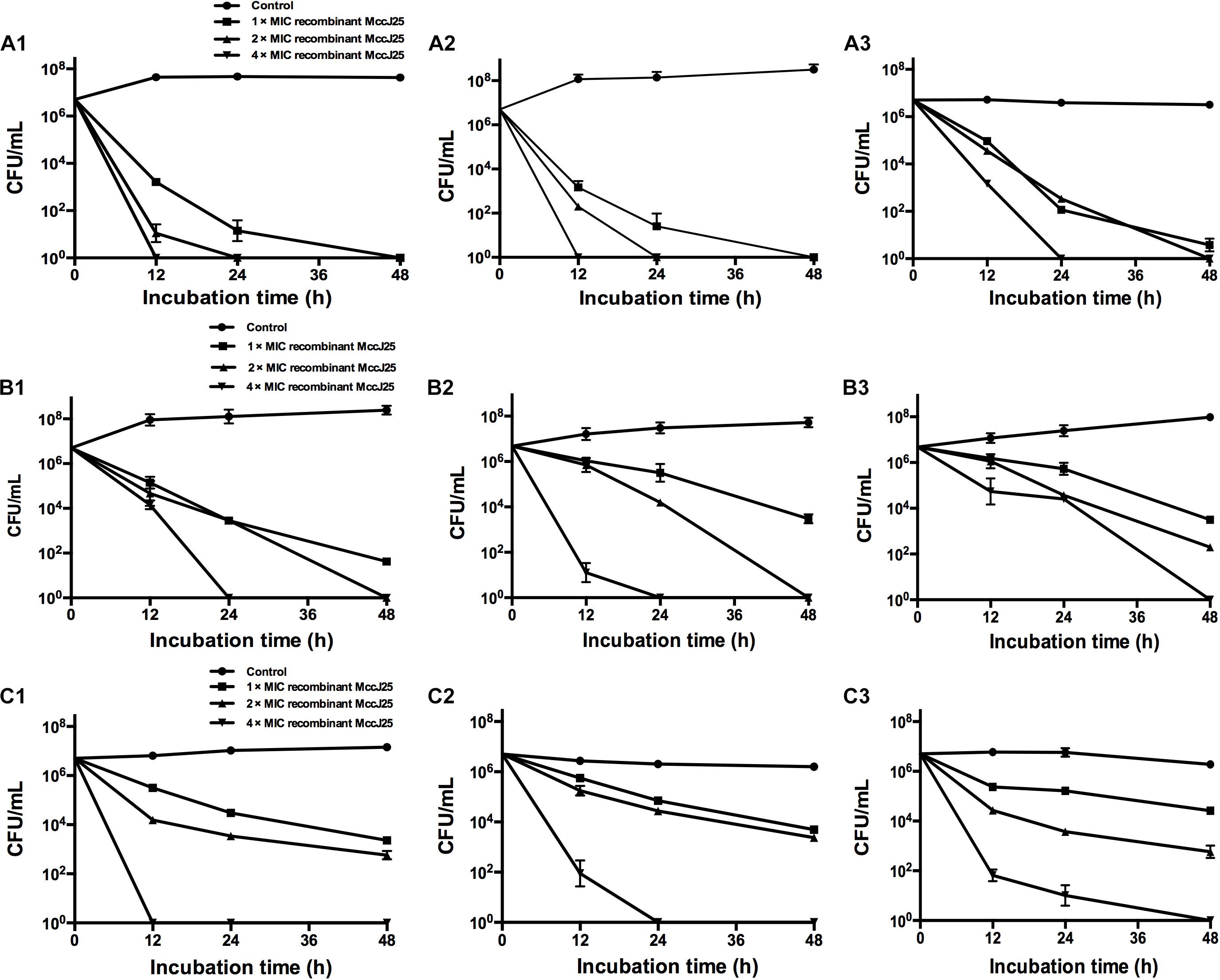
Figure 2. Recombinant MccJ25 exerts strong antimicrobial activity against different growth phase foodborne pathogens. S. Pullorum (A1–A3), S. Typhimurium (B1–B3), and E. coli O157:H7 (C1–C3) were grown to early-log (A1, B1, C1), late-log (A2, B2, C2), and stationary (A3, B3, C3) phases. Then, approximately 107 CFU/mL bacteria were inoculated into LB medium containing different concentrations of recombinant MccJ25. Survival of strains was compared at 0, 12, 24, and 48 h post inoculation. Salmonella and E. coli levels (mean CFU/mL ± SEMs) were determined from three individual experiments. n = 6.
When the early and late-log phase cells of S. Typhimurium were incubated with recombinant MccJ25 at various concentrations, S. Typhimurium was killed within 24 h at 4 × MIC recombinant MccJ25 and by 48 h with 2 × MIC recombinant MccJ25 (Figures 2B1,B2). However, S. Typhimurium exhibited significantly greater resistance to recombinant MccJ25 in the stationary phase than in the log phase. Additionally, reduction to non-detectable levels required 4 × MIC recombinant MccJ25 at 48 h (Figure 2B3). However, a reduction in early- and late-log phase E. coli O157:H7 to non-detectable levels within 12 and 24 h, respectively, required a concentration of 4 × MIC recombinant MccJ25 (Figures 2C1,C2). Bacteria exhibited significantly greater resistance to recombinant MccJ25 in the stationary phase than in the early-log and late-log phases, as well as a reduction in non-detectable levels requiring 4 × MIC recombinant MccJ25 and 48 h (Figure 2C3). Although the antimicrobial activity decreased in the different phases for the different strains, recombinant MccJ25 still exerted immense antimicrobial activity against all pathogens.
Recombinant MccJ25’s Bactericidal Capacity in Biological Products
The efficacy of recombinant MccJ25 against inoculated Salmonella and E. coli O157:H7 in different food samples was tested. When the three products contained 4 × MIC recombinant MccJ25 and all pathogen concentrations were 103 CFU/mL, no viable bacteria were detected after 24 h (0 CFU in three 100 μL product samples, data not shown). With all pathogenic bacteria concentrations at 104 CFU/mL, we obtained the same results (no bacteria were detected, data not shown) for the three products. However, when the concentration of all pathogens was 106 CFU/mL, Salmonella and E. coli O157:H7 cultures inoculated into the three biological products (SKM, SEY, and SMS) with 4 × MIC recombinant MccJ25 decreased significantly. S. Pullorum reductions of approximately 104, 8 × 103, and 7 × 103 CFU/mL were achieved for SKM, SEY, and SMS, respectively. Furthermore, an approximately 103 CFU/mL reduction in S. Typhimurium and E. coli O157:H7 was achieved for SKM, SEY, and SMS (Figure 3).
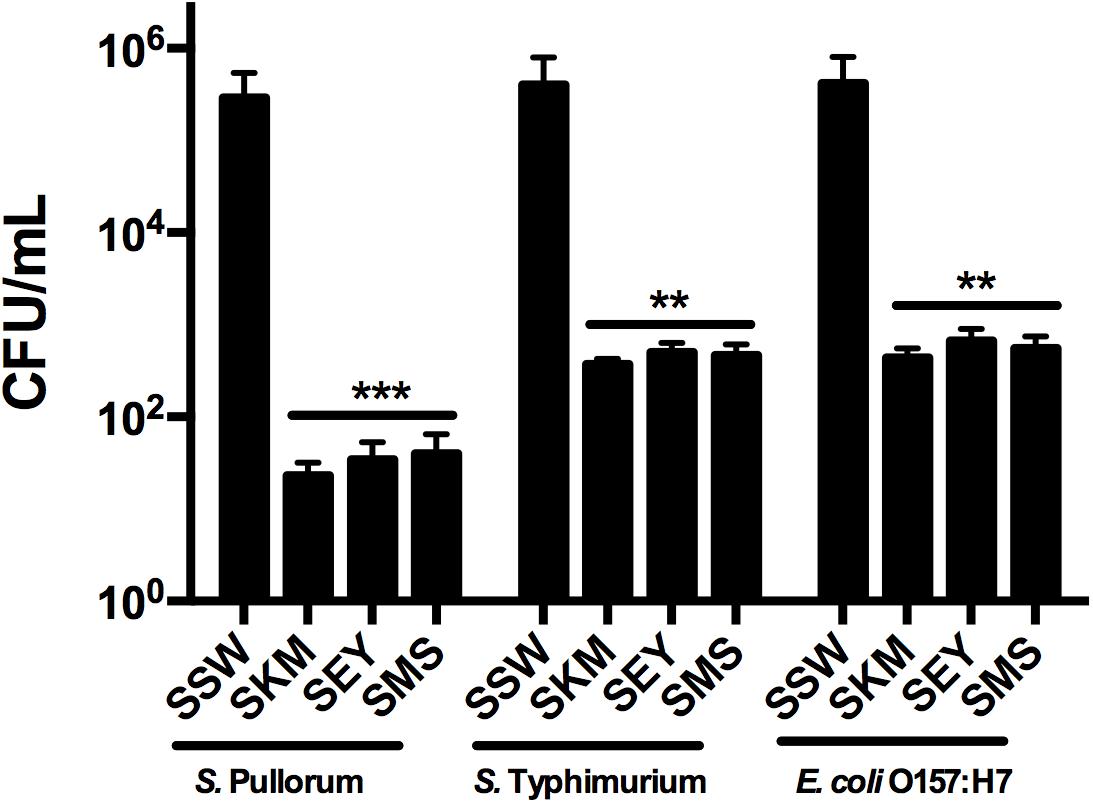
Figure 3. Antimicrobial activity of recombinant MccJ25 against foodborne pathogens in biological products. Approximately 5 × 106 CFU/mL bacteria were inoculated into autoclaved skim milk (SKM), mincemeat supernatant (SMS), and sterilized egg yolk (SEY). CFU Reduction in bacteria after 24 h in SKM, SMS, and SEY containing 4 × MIC recombinant MccJ25. Viable cells were determined by plate counts, and mean CFU/mL ± SEMs is shown for three individual experiments. n = 6. The asterisk denotes a significant difference in comparison with the control group (∗∗P < 0.01, ∗∗∗P < 0.001).
Overall Evaluation of All Incubation Biological and Biochemical Parameters
To further investigate whether recombinant MccJ25 maintained antimicrobial activity in vivo, an S. Pullorum strain was used as the indicator pathogen because the data presented in the above experiments showed stronger antimicrobial activity against S. Pullorum than against either E. coli O157:H7 or S. Typhimurium. The bactericidal potency of recombinant MccJ25 for S. Pullorum was tested under different conditions, as summarized in Figures 4A–F. The results of the thermal stability test showed that temperatures as high as 121°C for 20 min had no influence on the effect of recombinant MccJ25 against S. Pullorum (Figure 4A). Consistent with the thermal stability results, varying the pH from 2.0 to 9.0 also had no effect on the effect on recombinant MccJ25 activity against S. Pullorum (Figure 4B). Moreover, under the challenge of three proteases (pepsin, trypsin, and chymotrypsin) for 2 and 4 h, recombinant MccJ25 maintained its antimicrobial activity, as shown in Figure 4C.
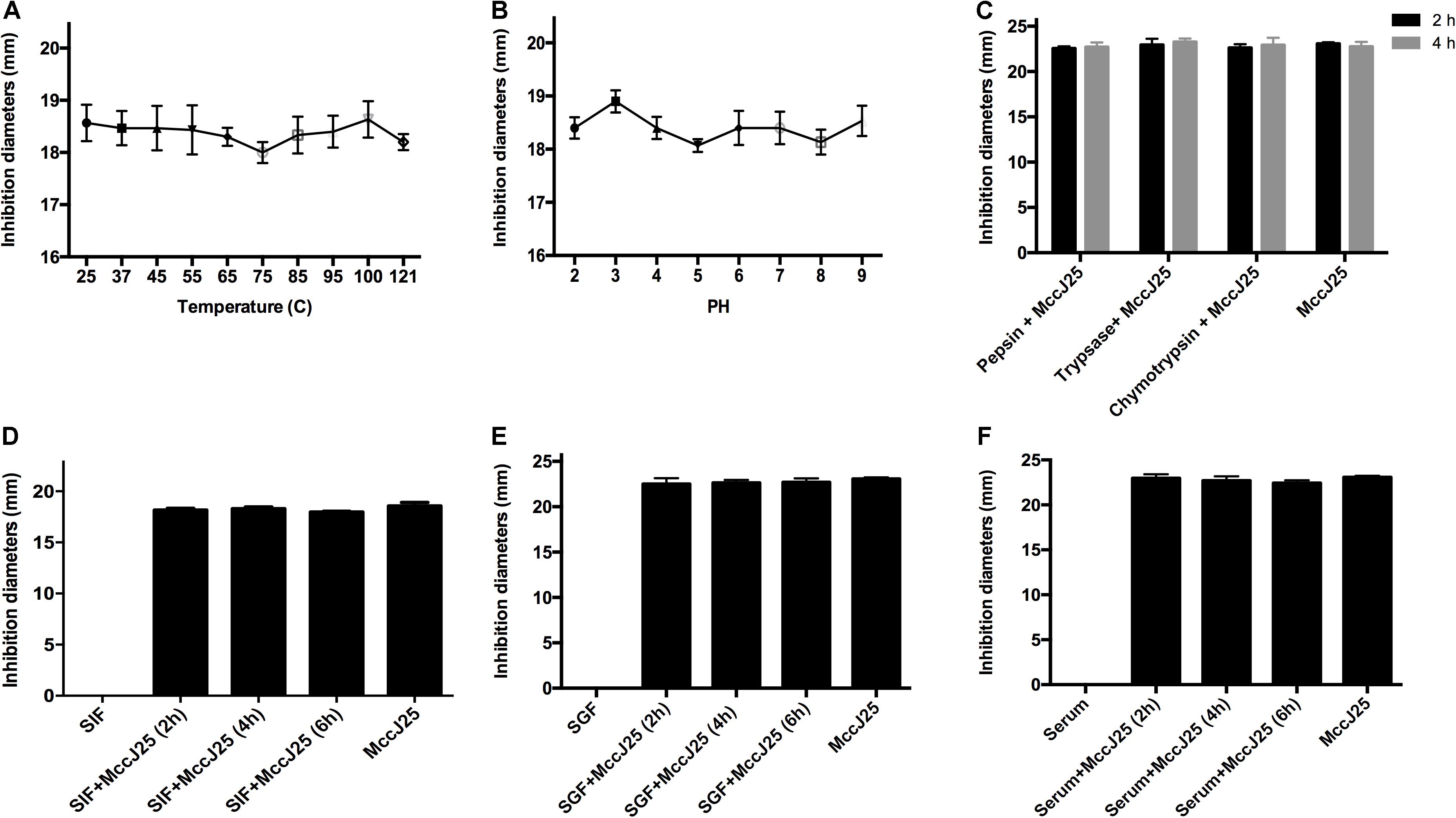
Figure 4. Effects of temperature (A), pH (B), and proteases (C) on inhibition of Salmonella by recombinant MccJ25, as well as antimicrobial activity of recombinant MccJ25 against Salmonella in the presence of SGF (D), SIF (E), and serum (F). The inhibition zone method was used to assess the antimicrobial activity of recombinant MccJ25. The graphs were derived from average values for three replicate experiments and almost identical triplicate sets of data. No significant difference was detected among treatments (P > 0.05).
To further assess the therapeutic potential of recombinant MccJ25, we first assayed its effect in the presence of simulated gastrointestinal fluids and serum. When incubated with SGF (Figure 4D), SIF (Figure 4E) or serum (Figure 4F), recombinant MccJ25 still inhibited the growth of S. Pullorum in a manner similar to that of recombinant MccJ25 alone. These results suggest that recombinant MccJ25 is stable in the gastrointestinal tract and remains effective.
Disruption of Bacterial Membrane Integrity
SEM and TEM have been used to directly observe the effects of AMPs on the cell morphology and integrity of bacteria. In the present study, the microbicidal properties of recombinant MccJ25 were measured by observing the morphology and integrity of the S. Pullorum cell membrane. As shown in Figure 5, untreated cells in the control group had a smooth and bright surface as well as intact cell morphology. Treatment with 1 or 4 × MIC recombinant MccJ25 for 1 h, which did not inhibit the growth of S. Pullorum, resulted in minimal membrane roughening, enlargement, and some filiferous adhesions in a small amount of cells (Figure 5A).
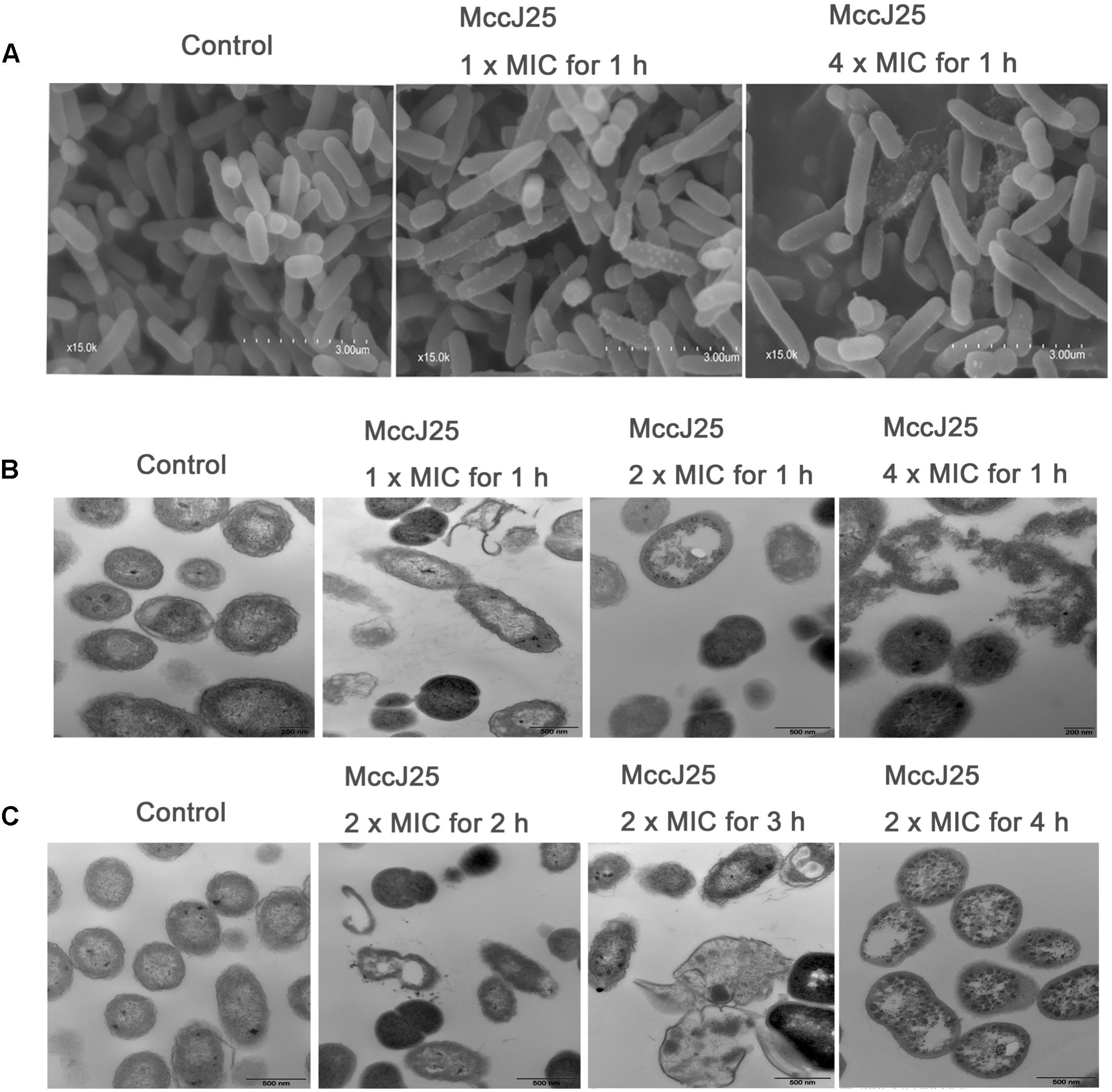
Figure 5. SEM (A) and TEM (B,C) micrographs of S. Pullorum. For SEM, bacteria were treated with recombinant MccJ25 at 1 and 4 × MIC for 1 h. The scale bar is 3 μm. For TEM, bacteria were treated with recombinant MccJ25 at 2 × MIC for 2, 3, and 4 h. The control was performed without recombinant MccJ25. The scale bar is 0.5 μm. Data are the means ± SEMs of three independent experiments.
TEM was also used to explore the morphology and intracellular alteration of the bacteria (Figures 5B,C). The results showed that the integrity of the cytoplasmic membrane of S. Pullorum after treatment with 1, 2, or 4 × MIC recombinant MccJ25 for 1 h was adversely affected (Figure 5B). Compared to the control, recombinant MccJ25 significantly disrupted the membrane of S. Pullorum, and some cytoplasmic clear zones were visible. Leakage of intracellular contents was also observed in recombinant MccJ25-treated S. Pullorum cells. However, 2 × MIC recombinant MccJ25 treatment of bacteria for different times (2, 3, and 4 h) showed deformed small septa, invisible cytoplasmic membranes, heterogeneous electron density, electron-light regions and thinned cell walls. Total leakage of cytoplasmic contents was also evident (Figure 5C).
Discussion
The number of foodborne pathogens has increased rapidly in recent years, especially those of Salmonella and E. coli species (Ma et al., 2016; Fan et al., 2017; Yi et al., 2017). Current treatments are limited due to the loss of antimicrobial agent efficacy and the slow development of new drugs and antibiotics (Ling et al., 2015; Rabanal et al., 2015; Yi et al., 2017). Practical solutions for these pathogens in food, animals and humans are not available (Hancock and Sahl, 2006). Therefore, the development of alternative antimicrobial agents for human health, food safety, and animal health is of critical importance. Nowadays, recombinant AMPs is promising alternatives to conventional antibiotics for the treatment of pathogens infections because of chemical synthesis is not suited for synthesis of large AMPs and is not scalable for large-scale production for clinical studies and commercial use (Cao et al., 2018; Wei et al., 2018; Kim et al., 2019; Meng et al., 2019).
The effects of recombinant MccJ25 on survival and growth of Salmonella and E. coli in different environments were examined in order to ascertain its potential as a antimicrobial agent for various domains applications. The results of assaying the antimicrobial activity of recombinant MccJ25 showed that sensitivity to the effects of recombinant MccJ25 varied among the different Salmonella strains and was not related to serotype. This finding is because differences were observed within strains of the same Pullorum serotype. Among all the tested bacteria, recombinant MccJ25 had the lowest MIC and MBC against S. Pullorum. These results corroborate our previous study. This finding indicates that recombinant MccJ25 inhibits S. Pullorum, as determined by an inhibition zone assay (Yu et al., 2018a). However, recombinant MccJ25 inhibits pathogenic bacteria E. coli O157:H7 and S. Typhimurium with an MIC of 16 μg/mL. This result could be directly attributed to the mechanism of action of MccJ25. This finding is consistent with previous studies that have found that MccJ25 has different mechanisms of action against different gram-negative bacteria and the same gram-negative bacteria at different MICs (Rintoul et al., 2001; Mukhopadhyay et al., 2004; Bellomio et al., 2007; Chirou et al., 2008). Additionally, previous reports have shown that microcins and recombinant AMPs exhibit different antimicrobial features and activity against gram-negative bacteria (Thomas et al., 2004; Severinov et al., 2007; Wei et al., 2018; Meng et al., 2019).
To further understand the effectiveness of the antimicrobial effect of recombinant MccJ25, we conducted a time-course and Live/Dead antimicrobial assay. The effects of recombinant MccJ25 on the survival and growth of S. Pullorum, S. Typhimurium and E. coli O157:H7 were examined to ascertain its potential as an antimicrobial agent and bioactive compound for food, animal or agricultural applications. The antimicrobial activity of recombinant MccJ25 was concentration dependent, as antimicrobial activity increased when more recombinant MccJ25 was added to the cultures. Our results corroborate previous studies indicating that recombinant MccJ25 has a different spectrum of activity against pathogens. They also showed that bacteria had varying sensitivities to the microcins (Hancock and Sahl, 2006; Ling et al., 2015). Additionally, Han et al. (2011) have shown that six different AMPs derived from five different animal species have different time curves for E. coli mortality. In addition, recombinant MccJ25 breaks bacterial cell walls, as shown by Live/Dead assays staining with propidium iodide, suggesting that recombinant MccJ25 may have a mode of action to kill pathogens similar to that of other AMPs or nano/microparticles (Han et al., 2011; Ling et al., 2015; Ma et al., 2016).
Some studies have shown that the antimicrobial activity of antimicrobial agents is unrelated to bacterial cell wall synthesis; however, they do disrupt bacterial cell walls (Ma et al., 2016; Fan et al., 2017). To examine this hypothesis, we employed bacterial cells at different growth phases for evaluating bactericidal activity. Although Salmonella strains and E. coli O157:H7 varied in their sensitivity, different concentrations of recombinant MccJ25 eliminated log phase (early- and late-log) bacteria for all strains examined at different indicated hours. Compared with log phase pathogens, stationary phase cells were more resistant to recombinant MccJ25, yet dramatic reductions were still achieved. It is known that stationary phase bacteria are more resistant than log phase cells to various antimicrobial treatments, including high pressure, heat, and biocides (Lee et al., 1994; Tabak et al., 2007; Cherchi and Gu, 2011). Manas and Mackey (2004) have also shown that stationary phase bacteria are stable because of intact outer membranes with higher protein and lipid ratios, lower unsaturated fatty acid content, and thicker cell walls than those of log phase bacteria. Although bacteria in food, the gut or water may be in the stationary phase due to nutrient limitations and stress, a reduction in Salmonella and E. coli O157:H7 cells in the stationary phase would still be feasible at high concentrations of recombinant MccJ25 or with long incubation times.
Thus, we carried out further studies of recombinant MccJ25 growth-inhibiting properties based on the above experiments on different biological food products and incubation conditions. In Sable et al.’s (2000) study, purified MccJ25 completely inhibition of the growth of 104 CFU/mL E. coli O157:H7 required a concentration of 50 μg/mL in egg yolk. This result was because of the richness of the egg yolk medium. However, in our study, we found that 4 × MIC recombinant MccJ25 could halt the growth of 103 and 104 CFU/mL Salmonella and E. coli O157:H7 in different food products. Furthermore, in the rich egg yolk medium, 4 × MIC recombinant MccJ25 could halt the growth of E. coli O157:H7 and Salmonella. The reason for this discrepancy may be a possible variation in the purification approach, the purity of MccJ25, or the design of the expression vector. Moreover, when the concentration of Salmonella and E. coli O157:H7 reached 106 CFU/mL in food products, 4 × MIC recombinant MccJ25 did not halt the growth of bacteria but did significantly reduce their number. Recombinant MccJ25 exerted stronger antimicrobial activity for the target Salmonella strain in the three food products than for E. coli O157:H7.
The data presented in the above experiments reveal the possibility of using recombinant MccJ25 to control pathogens. As we know, for any application of antimicrobial agents in food, humans or livestock, it is critical to identify any factors that could interfere with its activity, such as temperature, pH or proteases (Chen et al., 2009; Liu et al., 2013). A major limitation of AMPs is their low stability toward proteases (Chen et al., 2009; Han et al., 2011; Liu et al., 2013). This characteristic limits the practical usage of many peptides as drugs or food additives for both animals and humans. In the present study, the antimicrobial activity of recombinant MccJ25 against S. Pullorum was retained after varying temperature, pH, pepsin, trypsin and chymotrypsin treatments. These findings are consistent with prior reports that indicate MccJ25 is stable in complex conditions (Rosengren et al., 2003; Rebuffat et al., 2004). Additionally, the in vitro antimicrobial activity of recombinant MccJ25 was evaluated in simulated gastrointestinal environments and serum. This assessment was done to understand the fate of recombinant MccJ25 when it is orally administered. Our study reveals that recombinant MccJ25 exerts antibacterial activity in murine serum, in the stomach and in the intestinal tract. This observation is consistent with previous reports that some AMPs or micro-nano biomaterials have a stable bactericidal effect that is retained in SGF, SIF and serum (Liu et al., 2013; Xia et al., 2015; Ma et al., 2016).
We further confirmed the antimicrobial activities of recombinant MccJ25 by SEM and TEM. In our study, we found that recombinant MccJ25 has an acute negative effect on inner membrane integrity. As observed by TEM, the cytoplasmic membrane thinned, broke, and disintegrated. Abnormal separation was also observed. To our knowledge, this study was the first time that recombinant MccJ25 was observed to lead to lysis of the S. Pullorum cytoplasmic membrane and release of fluid and debris. Previous published studies have reported that MccJ25 disrupts membrane integrity in S. enterica Newport, in liposomes, and in uncharged phospholipid monolayers. However, these properties have been reported to be specific to S. enterica serovars and have been observed at concentrations greater than those of the MICs (Rintoul et al., 2001). Overall, our findings are consistent with an acting mechanism confined to the cytoplasmic membrane. These macroscopic effects indicate that these peptides might kill cells in different ways. Moreover, most AMPs disrupt membranes or induce pore/ion channel formation in the cytoplasmic membranes of susceptible bacteria (Han et al., 2011; Li et al., 2017; Wang et al., 2017). Additionally, some AMPs have multiple potential targets in bacteria, including the DNA, RNA, cell wall, cytoplasmic membrane, and various enzymes (Lee et al., 2016). On the other hand, our previous study has been shown that recombinant MccJ25 suppress intestinal inflammation and improve intestinal epithelial barrier function independently of their antimicrobial activity (Yu et al., 2018a). Consistent with the previous study, in the present study, we also found that recombinant MccJ25 inhibited S. Pullorum invasion and attenuated S. Pullorum-induced decreases in TEER and increases in FD4 and LDH levels (Supplementary Figure S2). To further investigate, the antibacterial activity of recombinant MccJ25 treatments on IPEC-J2 cells against S. Pullorum may be attributed to tight junction proteins (TJPs) expression (Supplementary Figures S1, S3). These results provide further evidence that recombinant MccJ25 is a candidate for clinical therapeutic use. For the determination of TJPs expression, the primers used are listed in Supplementary Table S1.
To summarize, this work showed that recombinant MccJ25 exhibits the greatest antimicrobial activity against different phases of Salmonella and E. coli, as well as harbors strong antimicrobial activity in various environments, thus mimicking real-world situations. In particular, examining the activity of recombinant MccJ25 against Salmonella and E. coli O157:H7 in food products showed the potential of recombinant MccJ25 as an antimicrobial agent or bioactive compound in the food industry. Recombinant MccJ25 has advantages over toxic chemicals commonly used for food and animal application due to its lack of toxicity, high bio-degradability, cost, availability, and reduced public health concerns. Although we identified relatively resistant strains of Salmonella and E. coli, high efficacy of recombinant MccJ25 (MIC was less or equal to 6.25 μg/mL) was found for all strains. Overall, the results of our study show great potential of recombinant MccJ25 to treat pathogens infections diseases caused by Salmonella and E. coli, especially multidrug resistant microorganisms, without adverse side effects. These data also support further investigations for larger scale application of a recombinant MccJ25 that offers broad range antimicrobial activity and low environmental impact.
Author Contributions
SQ, XZ, and QS obtained the financial support. SQ, XZ, and HY designed and oversaw the study. HY, NL, and LL performed the cells experiment and cultured the cells. HY, SC, and YW conducted and performed the qPCR. HY, GW, and SH analyzed the data. XD provided the recombinant MccJ25 sample. HY wrote the manuscript with contributions from all other authors. All authors read and approved the final manuscript. SQ is the guarantor of this work.
Funding
This work was supported by the National Key Research and Development Program of China (Grant Number 2016YFD0501308), Agro-scientific Research in the Public Interest (Grant Number 201403047), and Research and Application of Key Technologies for Enterobactercin New Veterinary Drug Product Development (Grant Number Z171100001317017).
Conflict of Interest Statement
The authors declare that the research was conducted in the absence of any commercial or financial relationships that could be construed as a potential conflict of interest.
Acknowledgments
The authors would like to express special thanks to American Journal Experts for scrutinizing and correcting the language.
Supplementary Material
The Supplementary Material for this article can be found online at: https://www.frontiersin.org/articles/10.3389/fmicb.2019.01954/full#supplementary-material
Abbreviations
AMPs, antimicrobial peptides; E. coli, Escherichia coli; ETEC, enterotoxigenic E. coli; MBC, minimum bactericidal concentration; MccJ25, microcin J25; MIC, minimum inhibitory concentration; PBS, phosphate buffered saline; SEM, scanning electron microscopy; SEY, sterilized egg yolk; SGF, simulated gastric fluid; SIF, simulated intestinal fluid; SKM, sterilized skim milk; SMS, sterilized mincemeat supernatant; S. Pullorum, Salmonella Pullorum; S. Typhimurium, Salmonella Typhimurium; TEM, transmission electron microscopy; TSA, Trypticase Soy agar; XLT4, xylose lysine tergitol 4.
References
Bellomio, A., Vincent, P. A., de Arcuri, B. F., Farias, R. N., and Morero, R. D. (2007). Microcin J25 has dual and independent mechanisms of action in Escherichia coli: RNA polymerase inhibition and increased superoxide production. J. Bacteriol. 189, 4180–4186. doi: 10.1128/JB.00206-07
Black, R. E. (1990). Epidemiology of travelers’ diarrhea and relative importance of various pathogens. Rev. Infect. Dis. 1, S73–S79.
Cao, J. C., Fuente-Nunez, C. D. L., Ou, R. W., Torossian, T. M. D., Santosh, G., Pande, S. G., Sinskey, A. G., et al. (2018). Yeast-Based synthetic biology platform for antimicrobial peptide production. ACS Synth. Biol. 7, 896–902. doi: 10.1021/acssynbio.7b00396
Centers for Disease Control and Prevention [CDC] (2010) Surveillance for Foodborne Disease Outbreaks, United States. Morbidity and Mortality Weekly Report 59.31, 973-979. Available at: http://www.cdc.gov/foodsafety/fdoss/surveillance/index.html [accessed May 6, 2016].
Centers for Disease Control and Prevention [CDC] (2011) National Enteric Disease Surveillance: Salmonella Surveillance Overview. Available at: http://www.cdc.gov/nationalsurveillance/PDFs/NationalSalmSurveillOverview_508/ [accessed April 23, 2016].
Centers for Disease Control and Prevention [CDC] (2013) Estimates of Foodborne Illness in the United States. Available at: http://www.cdc.gov/foodborneburden/ [accessed November 5, 2015].
Chen, X., Zhu, F. M., Cao, Y. H., and Qiao, S. Y. (2009). Novel expression vector for secretion of cecropin AD in Bacillus subtilis with enhanced antimicrobial activity. Antimicrob. Agents Chemother. 53, 3683–3689. doi: 10.1128/AAC.00251-09
Cherchi, C., and Gu, A. Z. (2011). Effect of bacterial growth stage on resistance to chlorine disinfection. Water Sci. Technol. 64, 7–13. doi: 10.2166/wst.2011.536
Chirou, M. N., Bellomio, A., Dupuy, F., Arcuri, B., Minahk, C., and Morero, R. (2008). Microcin J25 induces the opening of the mitochondrial transition pore and cytochrome c release through superoxide generation. FEBS J. 275, 4088–4096. doi: 10.1111/j.1742-4658.2008.06550.x
Cho, I., Yamanishi, S., Cox, L., Methe ì, B. A., Zavadil, J., Li, K., et al. (2012). Antibiotics in early life alter the murine colonic microbiome and adiposity. Nature 488, 621–626. doi: 10.1038/nature11400
CLSI (2012). Methods for Dilution Antimicrobial Susceptibility Tests for Bacteria That Grow Aerobically; Approved Standard - 9th ed. M07-A9 2012, 32. Wayne, PA: Clinical and Laboratory Standards Institute.
DeWaal, C. S., and Bhuiya, F. (2007). Outbreaks by the Numbers: Fruits and vegetables 1990–2005. Poster Presented at 2007 Annual Meeting of the International Association for Food Protection, Lake Buena Vista, FL, USA. Available at: https://cspinet.org/foodsafety/IAFPPoster.pdf [accessed June 7, 2016].
Falagas, M. E., Tansarli, G. S., Rafailidis, P. I., Kapaskelis, A., and Vardakas, K. Z. (2012). Impact of antibiotic MIC on infection outcome in patients with susceptible gram-negative bacteria: a systematic review and meta-analysis. Antimicrob. Agents Chemother. 56, 4214–4222. doi: 10.1128/AAC.00663-12
Fan, Y., Ginn, A., Kang, M., Jeong, K. C., and Wright, A. C. (2017). Application of chitosan microparticles for mitigation of Salmonella in agricultural water. J. Appl. Microbiol. 123, 1346–1358. doi: 10.1111/jam.13566
Forkus, B., Ritter, S., Vlysidis, M., Geldart, K., and Kaznessis, Y. N. (2017). Antimicrobial probiotics reduce Salmonella enterica in turkey gastrointestinal tracts. Sci. Rep. 7:40695. doi: 10.1038/srep40695.
Han, F. F., Liu, Y. F., Xie, Y. G., Gao, Y. H., Luan, C., and Wang, Y. Z. (2011). Antimicrobial peptides derived from different animals: comparative studies of antimicrobial properties, cytotoxicity and mechanism of action. World J. Microbiol. Biotechnol. 27, 1847–1857. doi: 10.1007/s11274-010-0643-9
Hancock, R. E., and Sahl, H. G. (2006). Antimicrobial and host-defense peptides as new anti-infective therapeutic strategies. Nat. Biotechnol. 24, 1551–1557. doi: 10.1038/nbt1267
Kim, D. S., Kim, S. W., Song, J. M., Kim, S. Y., and Kwon, K. C. (2019). A new prokaryotic expression vector for the expression of antimicrobial peptide abaecin using SUMO fusion tag. BMC Biotechnol. 19:13. doi: 10.1186/s12896-019-0506-x
Lee, I. S., Slonczewski, J. L., and Foster, J. W. (1994). A low-pH-inducible, stationary-phase acid tolerance response in Salmonella typhimurium. J. Bacteriol. 176, 1422–1426. doi: 10.1128/jb.176.5.1422-1426.1994
Lee, J. K., Seo, C. H., Luchian, T., and Parka, Y. Y. (2016). Antimicrobial peptide CMA3 derived from the CA-MA hybrid peptide: antibacterial and anti-inflammatory activities with low cytotoxicity and mechanism of action in Escherichia coli. Antimicrob. Agents Chemother. 60, 495–506. doi: 10.1128/AAC.01998-15
Li, Z. Z., Mao, R. Y., Teng, D., Hao, Y., Chen, H. X., Wang, X. M., et al. (2017). Antibacterial and immunomodulatory activities of insect defensins-DLP2 and DLP4 against multidrug-resistant Staphylococcus aureus. Sci. Rep. 7:12124. doi: 10.1038/s41598-017-10839-4
Ling, L. L., Schneider, T., Peoples, A. J., Spoering, A. L., Engels, I., Conlon, B. P., et al. (2015). A new antibiotic kills pathogens without detectable resistance. Nature 517, 455–459. doi: 10.1038/nature14098
Liu, Y. F., Xia, X., Xu, L., and Wang, Y. Z. (2013). Design of hybrid β-hairpin peptides with enhanced cell specificity and potent anti-inflammatory activity. Biomaterials 34, 237–250. doi: 10.1016/j.biomaterials.2012.09.032
Ma, Z. X., Kim, D., Adesogan, A. T., Ko, S., Galvao, K. N. A., and Jeong, K. C. (2016). Chitosan microparticles expert broad-spectrum antimicrobial activity against antibiltic-resistant microorganisms without increasing resistance. ACS Appl. Mater. Interfaces 8, 10700–10709. doi: 10.1021/acsami.6b00894
Manas, P., and Mackey, B. M. (2004). Morphological and physiological changes induced by high hydrostatic pressure in exponential- and stationary-phase cells of Escherichia coli: relationship with cell death. Appl. Environ. Microbiol. 70, 1545–1554. doi: 10.1128/aem.70.3.1545-1554.2004
Meng, D. M., Li, W. J., Shi, L. Y., Lv, Y. J., Sun, X. Q., Hu, J. C., et al. (2019). Expression, purification and characterization of a recombinant antimicrobial peptide hispidalin in Pichia pastoris. Protein. Expr. Purif. 160, 19–27. doi: 10.1016/j.pep.2019.03.007
Moreno, F., San-Millan, J. L., Herna ìndez-Chico, C., and Kolter, R. (1995). Microcins. Biotechnol. 28, 307–321.
Mukhopadhyay, J., Sineva, E., Knight, J., Levy, R. M., and Ebright, R. H. (2004). Antibacterial peptide microcin J25 inhibits transcription by binding within and obstructing the RNA polymerase secondary channel. Mol. Cell. 14, 739–751. doi: 10.1016/j.molcel.2004.06.010
Penders, J., Stobberingh, E. E., Savelkoul, P. H., and Wolffs, P. F. (2013). The human microbiome as a reservoir of antimicrobial resistance. Front. Microbiol. 4:87. doi: 10.3389/fmicb.2013.00087
Rabanal, F., Grau-Campistany, A., Vila-Farres, X., Gonzalez-Linares, J., Borras, M., Vila, J., et al. (2015). A bioinspired peptide scaffold with high antibiotic activity and low in vivo toxicity. Sci. Rep. 5:10558. doi: 10.1038/srep10558
Rajabi, M., Jones, M., Hubbard, M., Rodrick, G., and Wright, A. C. (2011). Distribution and genetic diversity of Salmonella enterica in the upper Suwannee River. Int. J. Food Microbiol. 2011:461321. doi: 10.1155/2011/461321
Rebuffat, S., Blond, A., Destoumieux-Garzon, D., Goulard, C., and Peduzzi, J. (2004). Microcin J25, from the macrocyclic to the lasso structure: implications for biosynthetic, evolutionary and biotechnological perspectives. Curr. Protein Pept. Sci. 5, 383–391. doi: 10.2174/1389203043379611
Rintoul, M. R., de Arcuri, B. F., Salomon, R. A., Farias, R. N., and Morero, R. D. (2001). The antibacterial action of microcin J25: evidence for disruption of cytoplasmic membrane energization in Salmonella newport. FEMS. Microbiol. Lett. 204, 265–270. doi: 10.1111/j.1574-6968.2001.tb10895.x
Rosengren, K. J., Clark, R. J., Daly, N. L., Goransson, U., Jones, A., and Craik, D. J. (2003). Microcin J25 has a threaded sidechain-to-backbone ring structure and not a head-to-tail cyclized backbone. J. Am. Chem. Soc. 125, 12464–12474. doi: 10.1021/ja0367703
Sable, S., Pons, A. M., Gendron-Gaillard, S., and Cottenceau, G. (2000). Antibacterial activity evaluation of microcin J25 against diarrheagenic Escherichia coli. Appl. Environ. Microb. 66, 4595–4597. doi: 10.1128/AEM.66.10.4595-4597.2000
Sassone-Corsi, M., Nuccio, S. P., Liu, H., Hernandez, D., Vu, C. T., Takahashi, A. A., et al. (2016). Microcins mediate competition among Enterobacteriaceae in the inflamed gut. Nature 540, 280–283. doi: 10.1038/nature20557
Severinov, K., Semenova, E., Kazakov, A., Kazakov, T., and Gelfand, M. S. (2007). Low-molecular-weight post-translationally modified microcins. Mol. Microbiol. 65, 1380–1394. doi: 10.1111/j.1365-2958.2007.05874.x
Tabak, M., Scher, K., Hartog, E., Romling, U., Matthews, K. R., Chikindas, M. L., et al. (2007). Effect of triclosan on Salmonella typhimurium at different growth stages and in biofilms. FEMS Microbiol. Lett. 267, 200–206. doi: 10.1111/j.1574-6968.2006.00547.x
Thomas, X., Destoumieux-Garzon, D., Peduzzi, J., Afonso, C., Blond, A., Birlirakis, N., et al. (2004). Siderophore peptide, a new type of post-translationally modified antibacterial peptide with potent activity. J. Biol. Chem. 279, 28233–28242. doi: 10.1074/jbc.M400228200
Wang, S., Wang, Q., Zeng, X., Ye, Q., Huang, S., Yu, H., et al. (2017). Use of the antimicrobial peptide sublancin with combined antibacterial and immunomodulatory activities to protect against methicillin-resistant Staphylococcus aureus infection in mice. J. Agric. Food. Chem. 65, 8595–8605. doi: 10.1021/acs.jafc.7b02592
Wei, X. B., Wu, R. J., Zhang, L. L., Ahmad, B., Si, D. Y., and Zhang, R. J. (2018). Expression, purification, and characterization of a novel hybrid peptide with potent antibacterial activity. Molecules 23:E1491. doi: 10.3390/molecules23061491
Wlodarska, M., Willing, B., Keeney, K. M., Menendez, A., Bergstrom, K. S., Gill, N., et al. (2011). Antibiotic treatment alters the colonic mucus layer and predisposes the host to exacerbated Citrobacter rodentium-induced colitis. Infect. Immun. 79, 1536–1545. doi: 10.1128/IAI.01104-10
Xia, X., Zhang, L., and Wang, Y. Z. (2015). The antimicrobial peptide cathelicidin- BF could be a potential therapeutic for Salmonella typhimurium infection. Microbiol. Res. 171, 45–51. doi: 10.1016/j.micres.2014.12.009
Yi, H. B., Hu, W. Y., Chen, S., Lu, Z. Q., and Wang, Y. Z. (2017). Cathelicidin- WA improves intestinal epithelial barrier function and enhances host defence against enterohemorrhhagic Escherichia coli O157:H7 infection. J. Immunol. 198, 1696–1705. doi: 10.4049/jimmunol.1601221
Yu, H. T., Ding, X. L., Li, N., Zhang, X. Y., Zeng, X. F., Wang, S., et al. (2017). Dietary supplemented antimicrobial peptide microcin J25 improves the growth performance, apparent total tract digestibility, fecal microbiota, and intestinal barrier function of weaned pigs. J. Anim. Sci. 95, 5064–5076. doi: 10.2527/jas2017.1494
Yu, H. T., Ding, X. L., Shang, L. J., Zeng, X. F., Liu, H. B., Li, N., et al. (2018a). Protective ability of biogenic antimicrobial peptide Microcin J25 against enterotoxigenic Escherichia Coli-induced intestinal epithelial dysfunction and inflammatory responses in IPEC-J2 cells. Front. Cell. Infect. Microbiol. 8:242. doi: 10.3389/fcimb.2018.00242
Yu, H. T., Shang, L. J., Zeng, X. F., Li, N., Liu, H. B., Cai, S., et al. (2018b). Risks related to high-dosage recombinant antimicrobial peptide microcin J25 in mice model: intestinal microbiota, intestinal barrier function, and immune regulation. J. Agric. Food Chem. 66, 11301–11310. doi: 10.1021/acs.jafc.8b03405
Keywords: Salmonella, Escherichia coli O157:H7, recombinant microcin J25, antimicrobial activity, biological environments, stability, mitigation
Citation: Yu H, Li N, Zeng X, Liu L, Wang Y, Wang G, Cai S, Huang S, Ding X, Song Q and Qiao S (2019) A Comprehensive Antimicrobial Activity Evaluation of the Recombinant Microcin J25 Against the Foodborne Pathogens Salmonella and E. coli O157:H7 by Using a Matrix of Conditions. Front. Microbiol. 10:1954. doi: 10.3389/fmicb.2019.01954
Received: 10 February 2019; Accepted: 08 August 2019;
Published: 27 August 2019.
Edited by:
Steven L. Cobb, Durham University, United KingdomReviewed by:
César de la Fuente, Massachusetts Institute of Technology, United StatesShankar Thangamani, Midwestern University College of Veterinary Medicine, United States
Copyright © 2019 Yu, Li, Zeng, Liu, Wang, Wang, Cai, Huang, Ding, Song and Qiao. This is an open-access article distributed under the terms of the Creative Commons Attribution License (CC BY). The use, distribution or reproduction in other forums is permitted, provided the original author(s) and the copyright owner(s) are credited and that the original publication in this journal is cited, in accordance with accepted academic practice. No use, distribution or reproduction is permitted which does not comply with these terms.
*Correspondence: Shiyan Qiao, qiaoshiyan@cau.edu.cn