- 1School of Environment and Science, Environmental Futures Research Institute, Griffith University, Nathan, QLD, Australia
- 2Queensland Micro- and Nanotechnology Centre (QMNC), Nathan, QLD, Australia
Plant pathogens reduce global crop productivity by up to 40% per annum, causing enormous economic loss and potential environmental effects from chemical management practices. Thus, early diagnosis and quantitation of the causal pathogen species for accurate and timely disease control is crucial. Botrytis Gray Mold (BGM), caused by Botrytis cinerea and B. fabae, can seriously impact production of temperate grain legumes separately or within a complex. Accordingly, several immunogenic and molecular probe-type protocols have been developed for their diagnosis, but these have varying levels of species-specificity, sensitivity and consequent usefulness within the paddock. To substantially improve speed, accuracy and sensitivity, advanced nanoparticle-based biosensor approaches have been developed. These novel methods have made enormous impact toward disease diagnosis in the medical sciences and offer potential for transformational change within the field of plant pathology and disease management, with early and accurate diagnosis at the point-of-care in the field. Here we review several recently developed diagnostic tools that build on traditional approaches and are available for pathogen diagnosis, specifically for Botrytis spp. diagnostic applications. We then identify the specific gaps in knowledge and current limitations to these existing tools.
Introduction
Legumes are the member of third largest plant family, Fabaceae, which comprises over 20,000 species are grown in a broad spectrum of climatic conditions and soil categories (Cernay et al., 2016). Legume crops help to build soil fertility by fixing nitrogen, act as a disease break for cereal and oilseed crops are staple foods, providing a significant source of protein, dietary fiber, carbohydrates and dietary minerals, as well as essential amino acids. Several represent high value cash export food crops, together representing 27% of the total global crop production (Smýkal et al., 2015).
Grain legumes are susceptible to attack by numerous pathogens, including bacteria, fungi, viruses to nematodes and parasitic plants, resulting in large global economic disaster. In particular, necrotrophic fungal pathogens such as Ascochyta spp., Botrytis spp., Colletotrichum spp. and others cause widespread disease and severe crop loss. Botrytis gray mold (BGM) of temperate grain legumes is caused by the fungal species Botrytis cinerea and Botrytis fabae separately or in a complex. The disease occurs particularly in dense, early-sown crops, when there are prolonged wet periods. The fungus can affect all above ground plant parts, resulting in tissue necrosis, flower and pod abortion, and seed shriveling and marking1. The loss in faba bean (Vicia faba L.) and lentil (Lens culinaris Medik.) from attack from Botrytis spp. in Australia alone during a conducive year is around AUD $1.4 million and AUD $1.0 million, respectively (Murray and Brennan, 2012).
Large economic and environmental savings could be made through early, fast and accurate diagnosis of the pathogen when the inoculum is in sufficient levels to cause an epidemic. This would lead to strategic timing and potential reduction in amounts and/or numbers of fungicide applications. To date, several immunogenic and molecular probe-type diagnostic methods have been developed for B. cinerea (Gachon and Saindrenan, 2004; Suarez et al., 2005; Chilvers and Lindsey, 2006; Rigotti et al., 2006; Diguta et al., 2010; Fernández-Baldo et al., 2011; Duan et al., 2014; Fan et al., 2015). However, their ability to discriminate between Botrytis ssp. and hence their usefulness in the paddock is unclear. Meanwhile, these existing methods, together with those based on whole-genome-sequencing and polymerase chain reaction (PCR) amplification are time-consuming and offer varying levels of sensitivity (Fan et al., 2015).
Necrotrophic Fungal Pathogens of Grain Legumes
Necrotrophic fungi kill the plant tissue during invasion and subsequently live saprotrophically on the dead cells. Important temperate grain legumes such as chickpea (Cicer arietinum L.), lentil, field pea (Pisum sativum) and faba bean are impacted by major necrotrophic fungi belonging to the Ascochyta spp., Botrytis spp., Colletotrichum spp. and Phoma spp. Together, these represent a $1.9 million per year loss in lentil, a $2.9 million per year loss in faba bean, a $5.2 million per year loss in chickpea and a $20.0 million per year loss in field peas (Murray and Brennan, 2012). Losses occur through direct seed loss and reduced marketability as well as disease management including chemical and cultural methods and through to the cost of breeding for resistant cultivars. Disease management costs would be reduced through rapid, sensitive and accurate pathogen identification. Additionally, several of the above-mentioned pathogens infect across-species, have similar disease symptoms on the plants and may be difficult to discriminate under a microscope due to very similar conidia spore morphology Therefore, a faster and highly accurate, species-specific, diagnosis would effectively enable a faster and more accurate response for management.
Diagnostic Methods Currently Used for Necrotrophic Fungi of Temperate Grain Legumes
Traditional diagnostic methods are mainly based on visual inspection of structural characters like shape and size of conidia, spores and cultural characters like growth rate and colony color (Taylor and Ford, 2007). However, these methods are relatively slow, often taking over a week to obtain as well as requiring a skilled pathologist to reliably identify the organism. Rapid diagnosis is required for the appropriate disease control measures to prevent an epidemic within an entire crop.
More recently, diagnostic assays for plant pathogens have been developed using nucleic acid sequences. Molecular approaches, mainly PCR-based, have been used extensively for fungal pathogens detection (Martin et al., 2000; Schaad and Frederick, 2002). Phan et al. (2002) designed a selective and sensitive diagnostic marker to differentiate Ascochyta rabiei of chickpea from other host specific Ascochyta spp. using ribosomal nucleotide sequence data with a detection limit of 0.1 pg of pathogen DNA.
Advanced molecular detection techniques such as the real time polymorphic chain reaction, loop mediated isothermal amplification (LAMP) method have also been developed (Notomi et al., 2000). Bayraktar et al. (2016) developed a real time PCR method to detect down to 1 pg of A. rabiei gDNA, a limit of just 0.1 pg of A. rabiei gDNA was detected with a LAMP assay, with the advantage of the visualization of color change with the naked eye (Chen et al., 2016).
Case Study: Detection of Botrytis spp. of Temperate Grain Legumes
Incidence and Global Impact of Botrytis Gray Mold Diseases on Grain Legumes
The major staple grain legume, chickpea, is impacted by the foliar disease BGM, also known as chocolate spot (Pande et al., 2009). The disease on chickpea is attributed solely to B. cinerea and the first occurrence was noted in India by Shaw and Ajrekar (1915) subsequently by Butler and Bisby (1931). The disease remains a major issue in Australia, Argentina, Bangladesh, India, Nepal, and Pakistan (Davidson et al., 2007) where complete crop losses were reported under conducive environmental conditions (Pande et al., 2009). BGM on chickpea were also been reported in Colombia, Chile, Canada, Mexico, Hungary, Spain, Myanmar, Turkey, and Vietnam (Pande et al., 2006).
Botrytis Gray Mold is also a major constraint to lentil production in Australia, Nepal, Bangladesh, Canada, Pakistan, Colombia, New Zealand, India, Morocco, Syria, and the United States (Davidson et al., 2007). On lentil, the disease is caused by co-existing and unknown mixed ratios of B. cinerea and B. fabae (Lindbeck et al., 2008, 2009). In Australia, severe epidemics with losses exceeding 50% were first reported in 1999 (Davidson et al., 2007). Similarly, BGM on faba bean is caused by both B. cinerea and B. fabae with yield losses in excess of 90% (Beyene et al., 2016). BGM also impacts production of several other grain legumes including field pea (Kraft and Pfleger(eds), 2001) and mung bean (Li et al., 2016).
Management and Control of Botrytis Gray Mold (BGM) on Grain Legumes
Botrytis Gray Mold is difficult to manage since the causal pathogens are soil, seed, and air borne (Davidson et al., 2007). Extensive screening of chickpea and lentil germplasm for reaction to the fungi has failed to identify high levels of resistance (Pande et al., 2006; Davidson et al., 2007). Similarly, only moderate levels of quantitative resistance have been reported in faba bean (Bouhassan et al., 2004; Tivoli et al., 2006). Therefore, fungicides remain a major component of BGM disease management strategy. However, these are often used only after symptoms are visible, at which point a substantial epidemic may already be well underway, requiring multiple subsequent foliar sprays at regular intervals.
Frequent use of fungicides can lead to the development of isolate population with loss of sensitivity to chemicals with multiple modes of action and the spread of these isolates is of huge concern to the horticultural and cropping industries (Rupp et al., 2017). Also, prolonged, repeated and over-spraying potentially through lack or misdiagnosis of the causal pathogen, can cause environmental contamination with substantial toxicological concern (Komárek et al., 2010). Informed timing of application, optimally prior to symptomology and in combination with climatic predictors greatly enhances fungicide efficacy (Eikemo et al., 2013). Indeed, early detection of the causal agents of BGM remains the most important step in effective and targeted management of the disease in the field and in greenhouse systems (Mertely et al., 2002).
Diagnostics for Botrytis spp.
As necrotrophic fungi Botrytis ssp. can survive from one season to another and are readily dispersed by wind or water. Infections are recognizable by their gray conidial structures within infected crop canopies but only once an advanced epidemic is underway. Several methods have been developed to identify these fungal species as follows:
Traditional Methods
Botrytis species have traditionally been identified through non-precise, time-consuming and non-sensitive visual symptomology, plate culturing and histopathological methods (Dewey and Yohalem, 2007). The later stages of infection by Botrytis spp. are easily recognized followed by the gray and/or brown conidial structures on the surface of infected host (Khazaeli et al., 2010). However, the initial or dormant and endophytic stages of infections are difficult to detect in non-symptomatic material (Molly and Grant-Downton, 2015). Also, the morphologies of conidiospores of Botrytis ssp. are often indistinct (Harrison, 1988; Beever and Weeds, 2007) and the use of a microscope is somewhat impractical for field diagnostics (Ray et al., 2017).
Immunoassay-Based Diagnostics
Several immunoassay methods have been developed to diagnose and quantify to a lower levels of plant pathogens. By far the most commonly used method for Botrytis ssp. species has been enzyme-linked immunosorbent assays (Dewey and Yohalem, 2007). By raising the antisera in rabbits, Ricker et al. (1991) applied plate-trapped antigen-immunosorbent assays to determine levels of Botrytis antigens in infected grapes juice. The same protocol, with the genus-specific monoclonal antibody (MAB) BC-12.CA4, was used to detect and quantify B. cinerea in pear stems (Meyer et al., 2000), strawberries (Mehli et al., 2005), grape berries (Obanor et al., 2004), raspberries (Dewey et al., 2000) and latent B. aclada infections in onion leaves (Yohalem et al., 2004). Production of MAB for Botrytis spp. is now somewhat routine2. The laboratory-based plate-trapped antigen ELISA (Meyer et al., 2000)was time-consuming, involving an overnight antigen coating step with extracts from infected plant tissues followed by sequential incubations with a monoclonal antibody, a secondary enzyme conjugated anti-mouse antibody and a substrate with washings between each step (Molly and Grant-Downton, 2015). By increasing the concentration of the secondary reporter antibody, the total time involved for diagnosis was subsequently reduced to 20 min (Dewey et al., 2000). Meanwhile, a microtiter immune-spore trapping device (MTIST device), that uses a suction system to directly trap air particulates by impaction in microtiter wells., was used together with the BC12.CA4 antibody for rapid detection and quantification of B. cinerea (Kennedy et al., 2000), Fernández-Baldo et al. (2011) then used BC-12.CA4 within a competitive ELISA quantification of B. cinerea in apple, table grape and pear tissues. In this assay, Botrytis-ssp. antigens present in extracts from infected tissues compete with “purified” B. cinerea antigens immobilized on the surface of microtiter plates using a cross linking agent. Although the assay takes about 40 min with a detection limit of 0.97 μg/ml, including in non-symptomatic infections, the BC12.CA4 antibody was subsequently shown not to discriminate among Botrytis species (Binder, 2014).
Molecular-Based Diagnostics
As an alternative to traditional and immunogenic approaches, unique diagnostics for individual Botrytis spp. may potentially be developed using molecular capture probes designed to individualized targets (DNA sequence in the first instance), that are stable and robust in terms of evolutionary potential. Accordingly, several nucleic acid-based probe targets have been described for the detection of B. cinerea including to the ribosomal intergenic spacer (IGS) region (Suarez et al., 2005), β-tubulin (Brouwer et al., 2003; Mehli et al., 2005; Spotts et al., 2008), cutinase A (Gachon and Saindrenan, 2004) and RNA helicase (Celik et al., 2009) genes, as well as ad hoc sequence characterised amplified region (SCAR) sequences (Rigotti et al., 2002; Suarez et al., 2005; Cadle-Davidson, 2008). However, the use of some ribosomal sequences for diagnostic purposes have been criticized due to non-stable inheritance (Suarez et al., 2005) and assays targeting specific genes like the cutinase A and β-tubulin from B. cinerea have been characterized to cross-react with close relatives such as B. fabae (Suarez et al., 2005; Spotts et al., 2008).
Depending on sequence deviation in the nuclear ribosomal IGS region Chilvers et al. (2007) developed a real-time PCR assay with primer set, LR12R/CNS1, for the quantification of Botrytis aclada, Botrytis allii, and Botrytis byssoidea in onion seed. Subsequently a TaqMan real-time PCR assay was developed by Carisse et al. (2009) with the primer set, B_squa_up221/B_squa_lo361. This was used to quantify the conidia of Botrytis squamosa, causal agent of botrytis leaf blight of onion. Similarly for diagnosis of B. cinerea on grapes, Diguta et al. (2010) developed a real-time PCR assay was based on IGS sequence with a detection limit of 6.3 pg DNA (corresponding to ∼540 genomes). Meanwhile, Botrytis fabiopsis, a newly characterized species causing BGM on broad bean in central China, was identified (Zhang et al., 2010). Fan et al. (2015) used three primer sets, Bc-f/Bc-r, Bfa-f/Bfa-r, and Bfab-f/Bfab-r, from the necrosis and ethylene-inducing protein 1 (NEP1) gene, to discriminate B. cinerea, from B. fabae, and B. fabiopsis in broad bean. These were species-specific in both single and multiplex PCR assays with the detection limits of 40, 40, and 400 pg (∼869, ∼869, and ∼8690 genomes) of purified gDNA, respectively. Furthermore, the existence of broad bean gDNA in the PCR reaction at 1:1000 (Botrytis gDNA/broad bean gDNA (wt/wt) had insignificant effect on diagnosis of the presence of Botrytis spp. The multiplex PCR assay could detect all the three Botrytis spp. in both artificially and naturally infected broad bean leaves (Fan et al., 2015).
To improve the portability of molecular diagnostic assays, a DNA amplification technique known as LAMP was developed, which amplifies nucleic acid with high selectivity, sensitivity and quickly under isothermal conditions (Notomi et al., 2000). LAMP products can be visualized with the naked eye by adding DNA-intercalating dyes, metal-ion indicators (Goto et al., 2009), CuSO4 (Zoheir and Allam, 2011), calcein (Tomita et al., 2008) or by measuring the increase in turbidity derived from magnesium pyrophosphate formation to infer increases in amplified DNA concentration (Mori et al., 2001). LAMP products may be assessed in a real-time detection format on a portable, low power platform, such as Genie I (OptiGene, United Kingdom) (Tomlinson et al., 2010). LAMP probes have been designed for B. cinerea targeting the ribosomal IGS (Tomlinson et al., 2010) with a detection limit of 6.5 pg (∼140 genomes) using hydroxynaphthol blue dye (HNB). LAMP assays for B. cinerea have also been developed based on the Bcos5 gene with a detection limit of 10–3 ng/mL (∼2.17 genomes; Duan et al., 2014) and was patented (CN103276057A;CN104293957A). Although, diagnostic methods using molecular probes alone have proven to be rapid and have high specificity and sensitivity, there remain limitations in detecting pathogens at low titers in materials at early infection stages (Beever and Weeds, 2007; Herbert et al., 2008; Khater et al., 2017). Also, false positive results are possible through cross-amplification of PCR-generated fragments of non-targets not considered during assay development. Further, false negatives may result from mis-amplification of target DNA (Khater et al., 2017). Consequently, further improvements to the robustness of molecular diagnostics systems are needed, which may include the use of biosensor devices.
Biosensor-Advanced DIAGNOSTIC APPROACHES FOR RAPID, SPECIFIC AND SENSITIVE PATHOGEN DIAGNOSTICS
A biosensor is an analytical device used to detect target molecules by producing a readout signal proportional to the specific interaction of an analyte with a recognition element (Mascini and Tombelli, 2008). In recent years, biosensors have been broadly used as diagnostic tools in environmental, food and clinical analysis, as well as in plant pathogen diagnosis and analysis (Mehrotra, 2016; Khater et al., 2017). Different types of sensor formats have been utilized for pathogen diagnosis and analysis of various biological elements at the level of tissue, microorganism, cell receptor, organelle, enzyme, antibody and nucleic acid. This has created new opportunities for highly sensitive protein detection in the cancer industry (Chikkaveeraiah et al., 2012; Rama and Costa-García, 2016; Marques et al., 2018), for detecting food borne pathogens (Wang et al., 2012; Xu et al., 2016), and biological and chemical contaminants in the agricultural sector (Linting et al., 2012; Tran et al., 2012; Regiart et al., 2017).
Biosensor Technologies Used in the Agricultural Sector
Electrochemical immunoassays with nanostructured surfaces, nanoparticle labels and magnetic beads have created new opportunities for thoroughly sensitive detection of biological and chemical contaminants in the agricultural sector (Linting et al., 2012; Tran et al., 2012; Regiart et al., 2017). In this device, an electrochemical immunosensor produces an electroactive signal that is detected by transducers through the use of an antibody that is incorporated into a bio-recognition layer to generate a measurable signal. Label-free electrochemical immunosensors with acceptable stability and reproducibility have been applied for pesticide analysis in crop samples (Tran et al., 2012; Liu et al., 2014). For the detection of atrazine, a widely used pesticide in agriculture, Liu et al. (2014) developed a simple, specific, sensitive electrochemical immunosensor that is characterized by cyclic voltammetry (CV) and electrochemical impedance spectroscopy (EIS) using ferricyanide as an electrochemical redox indicator. This biosensor uses immobilized gold nanoparticles (GNPs) on a gold electrode surface and under favorable condition the limit of detection for atrazine is just 0.016 ng/mL (Liu et al., 2016).
As an electroactive label, horseradish peroxidase (HRP) exhibits good stability and solubility to catalyze substrates to produce quantitative electrochemical signals (Zhao et al., 2011). By utilizing the favorable conductivity of the large surface area of gold nanoparticles (AuNPs) and high-quality catalytic ability of HRP, a dual amplified electrochemical immunosensor has been developed for accurate and sensitive diagnosis of Pantoea stewartii subsp. Stewartia of maize, with a detection limit of 7.8 × 103 cfu/mL. This was 20-fold more sensitive than conventional ELISA (Zhao et al., 2011).
Meanwhile, glass-poly(dimethylsiloxane) (PDMS) microfluidic sensors comprise a glass substrate that provides a thermally stable and amenable platform for sensing of a target molecule by deposition of the electrodes by sputtering (Hatamie et al., 2015). For these biosensors, zinc oxide nano rods are fabricated on non-commercial electrodes and the glass substrate is coated with a gold layer. The sensing is then determined through voltammetric changes during antibody capture. This type of biosensor has the advantages of: low cost, durability, chemical inertness, optical transparency and automation (Casanova-Moreno et al., 2017). By utilizing this technique, the bacterial pathogen Xanthomonas arboricola was detected very quickly and at 1.5 × 102 cfu/mL in walnuts, based on the covalent immobilization of a MAB (Regiart et al., 2017).
Optical biosensors offer the advantage of label-free real time detection of chemical and biological substances by exploiting the interaction of an optical field with a bio-recognition element (Damborský et al., 2016). The central point of this method is the ability to detect the target microorganism via color change, without any analytical instrument. This has immense portability and the generation of an instant result. However, the major drawback is low sensitivity, for example a detection limit of just 5 × 104 cfu/mL for Escherichia coli (Pöhlmann et al., 2014) compared to the limit of detection by electrochemical assay of 32 cfu/mL (Li et al., 2015).
Fluorescence-based immunosensors are used to measure the concentration and intensity of target analyte fluorescence to which fluorescent molecules bind directly or indirectly (Holford et al., 2012). For this, an external short-wavelength light source is required to initiate electronic transitions in a molecule for producing longer wavelengths of light. The fluorescent bioreceptor and optical transducer combination was used in the fluorescent-based optical biosensor to diagnose Phytophthora palmivora, Ustilago maydis, Colletotrichum lindemuthianum, Aspergillus nidulans like plant pathogenic fungi (Ray et al., 2017). Charlermroj et al. (2013) used this technique to develop a microsphere immunoassay to detect important plant pathogens like, watermelon silver mottle virus (WSMoV), fruit blotch bacterium (Acidovorax avenae subsp. Citrulli), melon yellow spot virus (MYSV) and chilli vein-banding mottle virus (CVbMV). Antibody correlated to fluorescence-coded magnetic microsphere for each plant pathogen used to capture corresponding pathogen. The presence of the pathogens were identified by secondary R-phycoerythrin (RPE)-labeled antibodies with the limit of detection of 6 × 105 cfu/ml, 1.0 ng/ml, 20.5 ng/ml and 35.3 ng/ml, respectively (Charlermroj et al., 2013). Though this assay showed relatively sensitivity, it requires a multi-channel fluorescence reader (Khater et al., 2017). Gold nanoparticle/multi-walled carbon nanotube (Nano-Au/C-MWCNT) based label free immunosensor was used to detect capsicum chlorosis virus (CaCV) in bell pepper which showed 800–1000 times more sensitivity than ELISA (Sharma et al., 2017).
Biosensors have been developed to detect pathogens through surface enhanced Raman spectroscopy (SERS) and surface plasmon resonance (SPR) (Tokel et al., 2014; Yoo and Lee, 2015). The SPR sensor technology uses electromagnetic waves that can be stimulated by light at gold sensor surface (Skottrup et al., 2008). The incoming light, associate with the gold interface, at angles greater than the critical angle and the reflected light exhibits a characteristic devaluation due to resonant energy transfer from the incoming photons to the surface plasmons (Skottrup et al., 2008). Skottrup et al. (2008) compared SPR-based biosensors for the detection of plant pathogens and noted a diversity in sensitivity level including those developed for Fusarium culmorum, Puccinia striiformis and Phytophthora infestans at 0.06 pg/ml (∼2 spores/ml), 3.1 × 105 spores/ml and 2.2 × 106 spores/ml, respectively (Skottrup et al., 2007a, b; Torrance et al., 2006; Zezza et al., 2006, respectively). Meanwhile, Mendes et al. (2009) reported a SPR-based immunosensor for detecting soybean rust on leaves whereby an antibody to Phakopsora pachyrhizi was covalently immobilized on a gold substrate via a self-assembled monolayer (SAM) of thiols using cystamine-coupling chemistry. This biosensor had a detection limit of 8 μg/ml (9.26 × 106 spores/ml). The ability of real-time and label-free detection of targets is the major advantage of using SPR in pathogen detection, however, low sensitivity and specificity due to the high non-specific adsorption of non-targets onto the sensor surface remains a major hurdle.
Accordingly, SERS has been used in various biosensor-based pathogen detection techniques because of its single molecule-level sensitivity, molecular selectivity and quenching insensitivity (Yoo and Lee, 2016). This method provided a 10–100 times narrower spectral widths than previous methods and minimized overlapping between different labels (Bantz et al., 2011). Gold nanoparticle probes with versatile Raman dyes have also become popular for development of SERS-based immunoassays (Xu et al., 2004).
Although the power of nano-biosensors for the timely, accurate and at-point-of-contact diagnostics is clear, there remain major challenges to their use that are related to the isolation and capture of specific targets from biological and environmental backgrounds and in the way in which the target capture is relayed as a signal of detection.
Challenges Involved in Developing an In-Field Nano-Biosensor for Botrytis spp.
Biological Challenges
Sampling and In-Field Processing
Though biosensors showed higher sensitivities compared to traditional, immunogenic or molecular assays, majority have been tested with synthetic samples and in in vitro. Many are yet to be validated in field, which incorporates optimization of target extraction from the host tissue (Ray et al., 2017). A quick sample processing of samples is also a requirement. The BGM target will be fungal spores and/or mycelia, dependant on the stage of infection or epidemic. Airborne spores are the major source of inoculum generally blown in through prevailing air currents from neighboring crops. However, the pathogens also survive between seasons as hardened bodies of mycelial sclerotia in the soil and on infected plant trash (previously harvested stems) (Lindbeck et al., 2009). Therefore, careful consideration must be given to capturing the target organism, potentially via monitoring and surveillance using aerial trapping or screening of plant surfaces. Consideration should also be given to determining how many spores/genomes of a pathogen are required to lead to a disease epidemic under optimal climatic and host conditions to ensure that a quantitative diagnostic is realistically translatable to informing disease management decision (González-Domínguez et al., 2015).
Consideration should be given to the potential for biological interference from the sample used for diagnosis. In the case of BGM, the pathogen is both external and internal to the host plant tissue, existing as mycelia on the foliage and as conidiophores within foliar lesions at later stages of the infection cycle. If the diagnostic is applied to an infected plant sample which is then used as the complete sample (containing both fungus and plant), the assay may be impacted by the noise of the plant itself which contains a variety of nucleic acids and polymers. These plant cell components have the potential to cause subsequent loss of sensitivity with diagnostic processes from inaccurate or reduced molecular probe binding as well as causing electromagnetic interference (McCann et al., 1997). Also, if using a molecular probe, potential co-extraction of high content of tannins, polysaccharides, and polyphenols may lead to oxidation and degradation of the target fungal gDNA sequences, again leading to failure or loss of detection sensitivity (Arruda et al., 2017).
Technical Challenges
Several pre-analytical steps involved in DNA, RNA, or whole pathogen isolation and detection may significantly affect the sensitivity and accuracy of a nano-biosensor as follows:
Background Interference
In many assay designs, target nucleic acids are initially captured and isolated by a pre-isolation step where they are hybridized to a complementary capture probe. However, the capture of a specific nucleic acid (DNA or RNA) target is greatly reduced in accuracy when present in a background that is populated with highly homologous non-target sequences, i.e., plant sequences from the same family as the target fungal sequence. Accordingly, probes must be exact and able to robustly differentiate even single nucleotide polymorphisms (SNPs). The biological challenge from highly-heterologous samples may also result in the technical challenge of non-specific absorption of molecules to the sensor surface inducing false responses (Islam et al., 2017). Therefore, a suitable blocking/antifouling agent such as mercapto-ethanol, poly (ethylene glycol), mercaptohexanol or bovine serum albumin is often used (Gooding, 2002; Islam et al., 2017). Electro biochemical sensors are also impacted by the potential non-specific adsorption of non-target nucleic acids onto the electrode surface (Lucarelli et al., 2004), which can lower hybridization efficiency to less than 10% (Levicky et al., 1998). Again, this is greatly improved by exposing the DNA modified surface to a blocking agent (Gooding, 2002; Islam et al., 2017).
Target Capture Efficiency
The dynamics of the specific nucleic acid hybridization plays a crucial role in the specificity and sensitivity of a hybridization-based DNA nano-biosensor (Liu et al., 2012). The capture probe, which is complementary to the single-stranded target sequence, detects the target gDNA by hybridization onto the recognition interface (Gooding, 2002). For this to be accurate, the probe sequence must be designed unique to the target. During the hybridization, if the capture probe and the target sequences are not permitted to coil around each other, if so they will not hybridize efficiently (Gooding, 2002). Some other factors such as end point immobilization of probe strand (Levicky et al., 1998), density and surface chemistry of probe strand (Gooding, 2002) also play key roles in determining the hybridization efficiency.
Limits of Probe Specificity and Sensitivity
Different construction of capture probes affects the hybridization efficiency of biosensor which is an important factor in the specificity and sensitivity of the biosensor (Liu et al., 2012). Ranging 18–25 nucleotides, usually allows higher level of specificity in hybridization reaction where excessive length of capture probe often results unfavorable yields (Lucarelli et al., 2008). Due to the spatial hindrance effect, a probe cannot capture a target analyte if the density is too low or too high (Ding et al., 2013). Therefore, surface coverage of the probe DNA is a crucial issue. Too few binding sites (<10–12 cm–2) will result in a reduced detection sensitivity.
Biosensors Developed for Botrytis spp. Detection
Electrochemical Biosensors
For Botrytis spp. detection in onion, Binder (2014) developed an electrochemical immunosensor using screen printed gold electrodes (SPGE) primed with horseradish peroxidase (HRP) that were able to detect 34 ng/ml (∼7 × 105 spores/ml). Most recently, a fast (60 min) and sensitive (214 pM or ∼3 × 108 spores/μl) diagnostic protocol was developed for the detection of Pseudomonas syringae from infected plant samples via rapid isothermal amplification of target sequences by RPA followed by gold nanoparticle-based electrochemical stimulus with differential pulse voltammetry (DPV) (Lau et al., 2017; Figure 1). Briefly, the isothermally amplified targets were first incubated with the AuNPs/DNA probe. The mixture was then incubated with streptavidin-coated magnetic beads. After performing a magnetic collection of the beads, the mixture was resuspended in PBS and denatured at 95°C and following a second magnetic separation, the supernatant was transferred to an electrode surface for DPV signal capture.
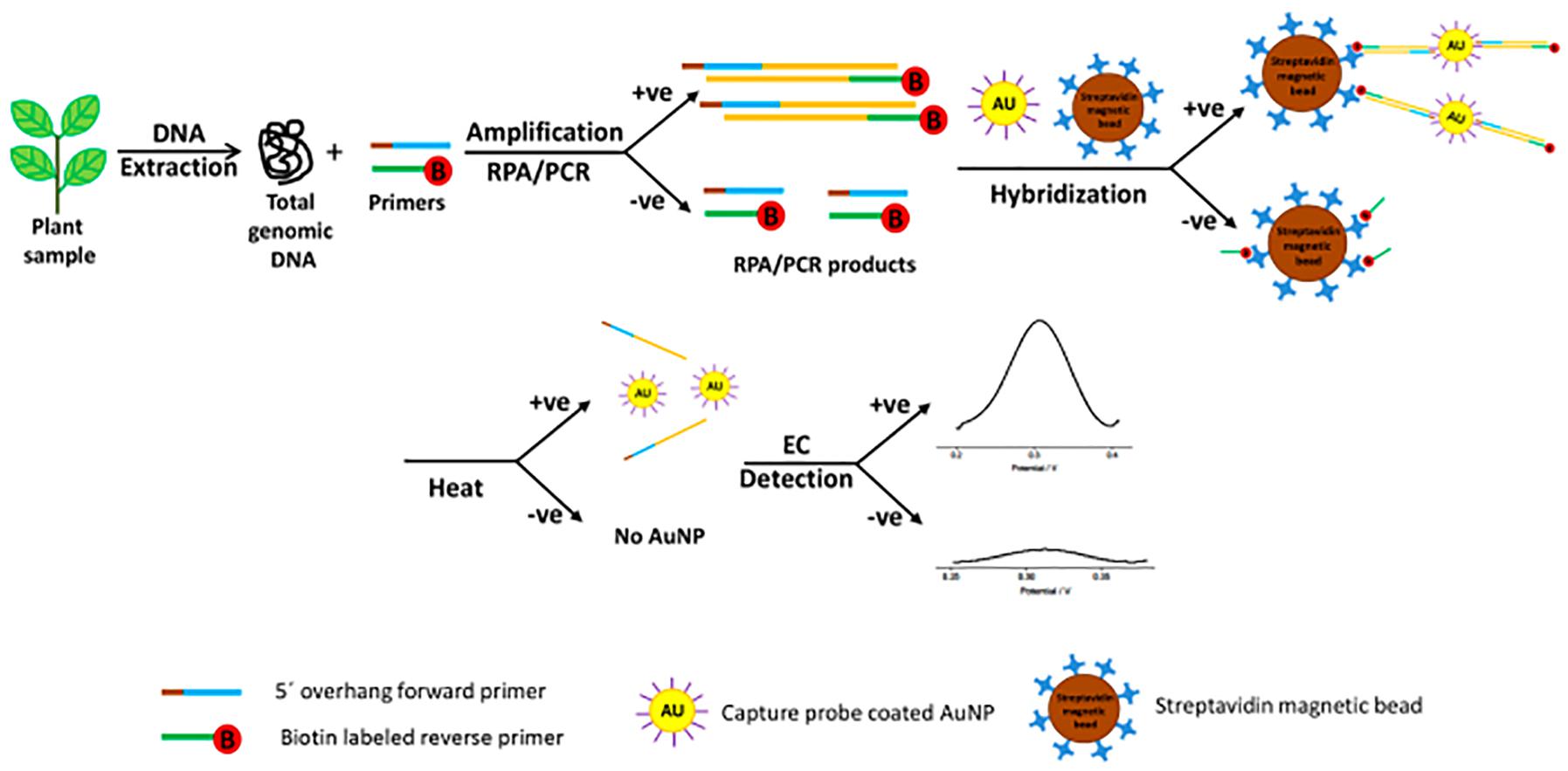
Figure 1. Schematic representation of the electrochemical bioassay for plant pathogen diagnosis involving incubation of the amplified target, AuNPs-DNA probes and streptavidin beads (reproduced with permission from Lau et al., 2017).
Optical Biosensors
A novel assay was developed to detect isothermally amplified DNA of important plant pathogens, including Botrytis spp. (Wee et al., 2015). The DNA-mediated bridging flocculation assay develops to detect the presence of the target pathogen gDNA, which is amplified to produce high molecular weight DNA amplicons (Figure 2; Wee et al., 2015). This method provided a yes/no pathogen presence result for immediate informed disease risk management and was used to detect B. cinerea at very early infection stages, when symptoms were just visible to the naked eye with the detection limit of less than 0.3 pg/μl (∼ 6 spores/μl) (Wee et al., 2015).
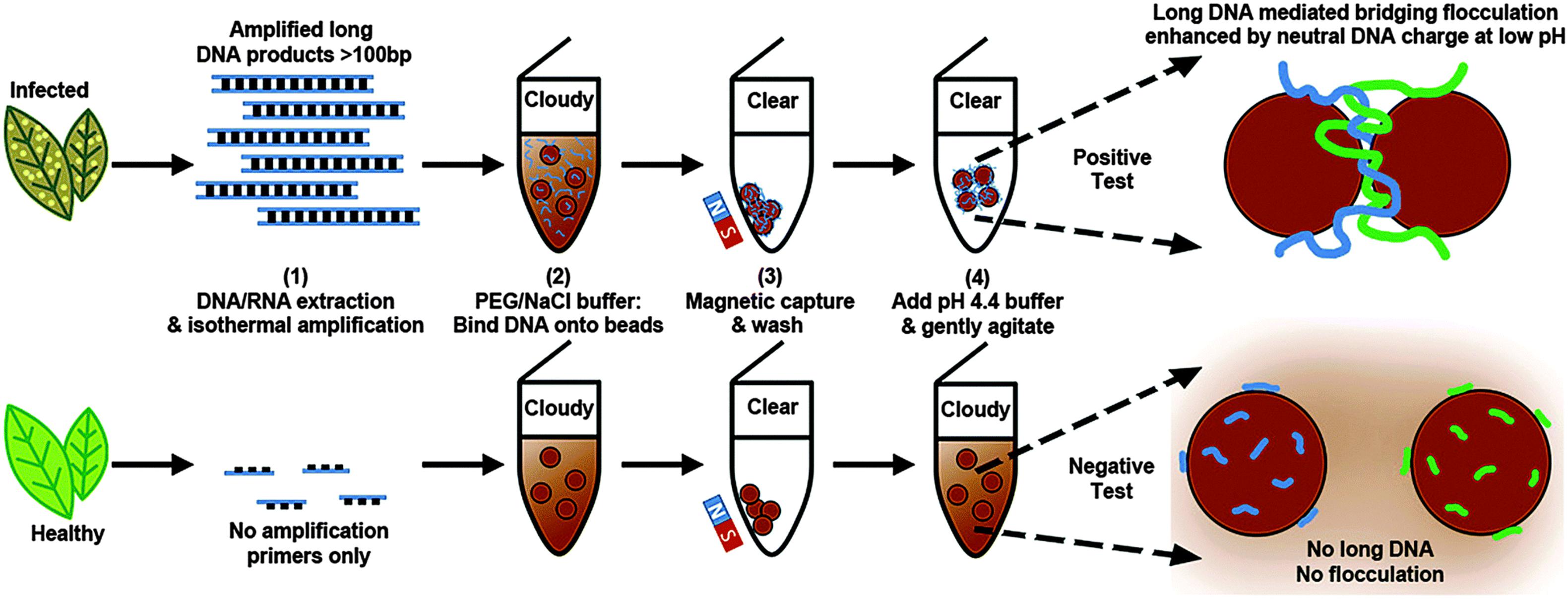
Figure 2. Schematic representation of the DNA-based bridging flocculation assay (reproduced with permission from Wee et al., 2015).
Recently, B. cinerea, P. syringae, and Fusarium oxysporum were detected in Arabidopsis thaliana and tomato plants via a multiplex point-of-care diagnostic platform developed using a combination of SERS and RPA (Figure 3; Lau et al., 2016). The method was faster and 100 times more sensitive than PCR alone and could detect just two B. cinerea genomes.
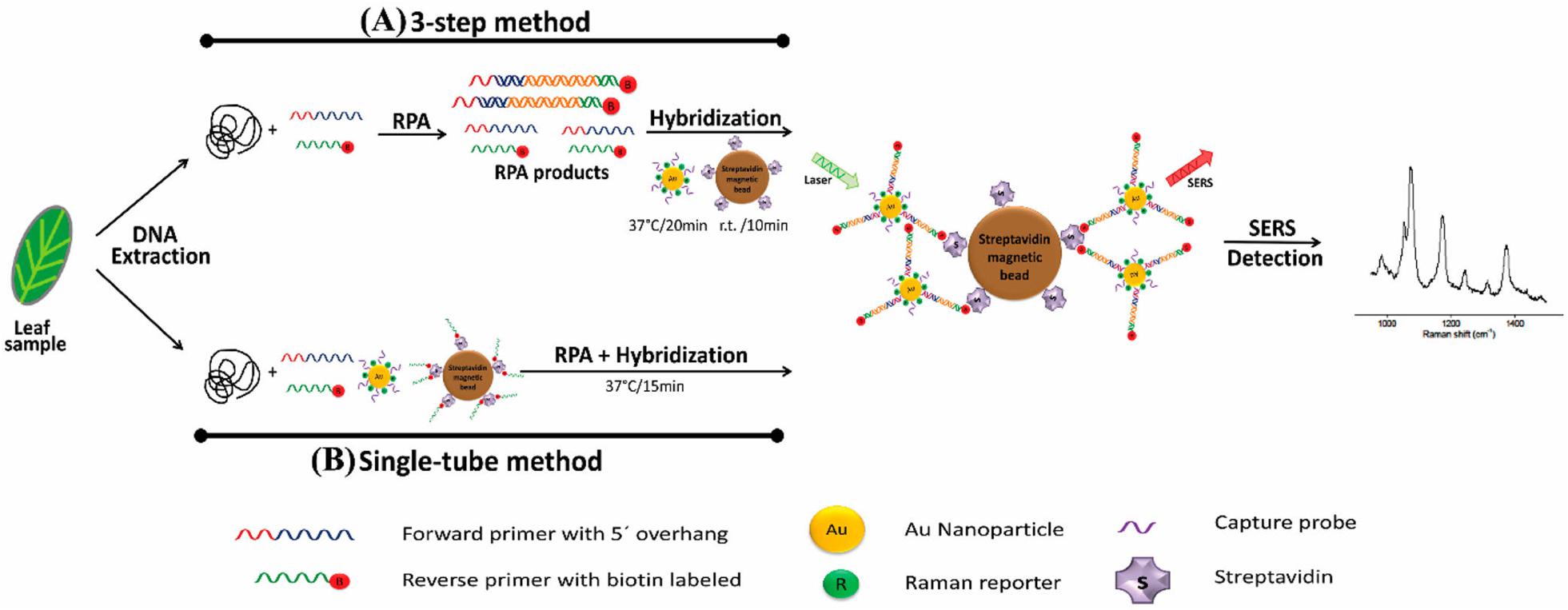
Figure 3. Schematic representation of RPA/SERS multiplex assay (reproduced with permission from Lau et al., 2016). (A) Genomic DNA was extracted using modified Solid Phase Reversible Immobilization (SPRI) method, followed by recombinase polymerase amplification (RPA) using specific biotinylated capture probe. After amplification, biotin/RPA/SERS products were captured by streptavidin magnetic beads. (B) For faster and simpler assessment, SERS nanotags were amplified and hybridized in a single tube.
Although showing rapid and accurate result, a large limitation of SERS biosensors is the requirement for bulky optical instruments, such as lasers, optical microscopes, detectors and monochromators (Yoo and Lee, 2015). These do not translate well into the field environment. Therefore, scope remains for development of a rapid, accurate, inexpensive and sensitive nano-biosensor for plant pathogens.
Summary and Conclusion
There are a range of technologies used in advance diagnostic assays for plant pathogens. Each of these has advantages and biological and technical limitations that relate to specificity, sensitivity, speed to result, quantification, portability, and cost. Recent advances in electromagnetic nano-biosensors coupled with species-specific molecular probes has the potential to overcome many of these limitations. Ultimately, a practicable diagnostic method would instantly inform growers on the presence and quantity of the target pathogen and be able to accurately detect at the threshold of spore density to create an epidemic. Unfortunately, the currently even the most advanced Botrytis species diagnostics do not meet these criteria, being either non-specific or lacking in sensitivity, and/or unable to provide a portable and instant result (Table 1). Therefore, the challenge remains to provide such a tool to the cropping industries at a cost low enough to be commercially viable for wide uptake to guide informed disease management, specifically for necrotrophic fungal pathogens to aid in the use and timing of fungicide application.
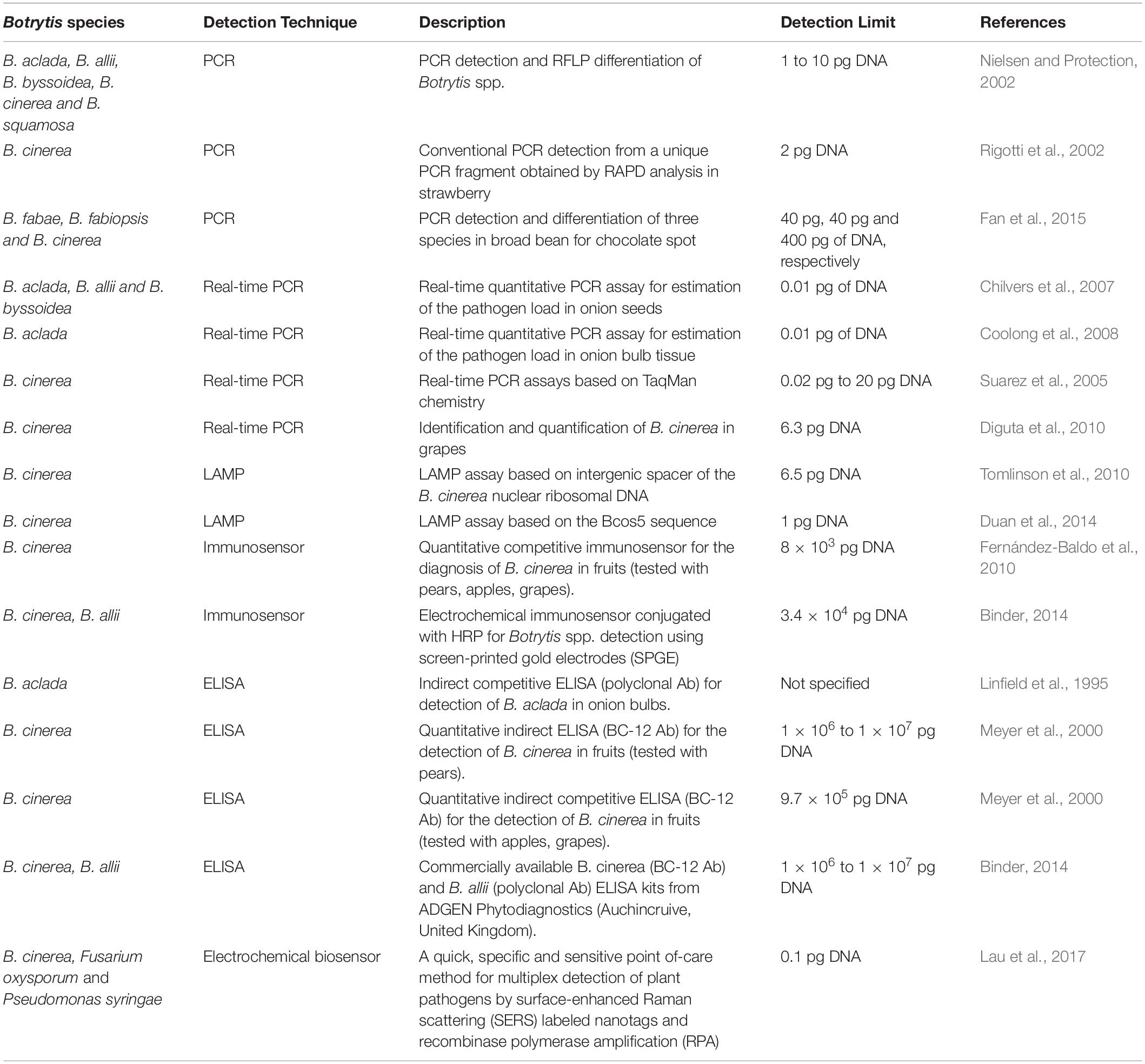
Table 1. Summary of published diagnostic methods and their detection limits for crop disease-related Botrytis species.
Author Contributions
All authors wrote and revised the manuscript, and approved it for publication.
Funding
The authors acknowledge Griffith University for providing the Ph.D. scholarship to MB and AW Howard Memorial Trust for the additional funds to MB for her research.
Conflict of Interest Statement
The authors declare that the research was conducted in the absence of any commercial or financial relationships that could be construed as a potential conflict of interest.
Acknowledgments
The authors acknowledge Chemical Communications, Analytical Chemistry, and Scientific Reports for permission to reproduce figures.
Footnotes
- ^ http://www.pulseaus.com.au/about/australian-pulse-industry
- ^ http://dshb.biology.uiowa.edu/Botrytis-cinerea
References
Arruda, S. R., Pereira, D. G., Silva-Castro, M. M., Brito, M. G., and Waldschmidt, A. M. (2017). An optimized protocol for DNA extraction in plants with a high content of secondary metabolites, based on leaves of Mimosa tenuiflora (Willd.) Poir. (Leguminosae). Genet. Mol. Res. 16:gmr16039063. doi: 10.4238/gmr16039063
Bantz, K. C., Meyer, A. F., Wittenberg, N. J., Im, H., Kurtuluş, Ö, Lee, S. H., et al. (2011). Recent progress in SERS biosensing. Phys. Chem. Chem. Phys. 13, 11551–11567. doi: 10.1039/c0cp01841d
Bayraktar, H., Zer, G. O., Aydoğan, A., and Palacıoğlu, G. (2016). Determination of Ascochyta blight disease in chickpea using real-time PCR. J. Plant Dis. Prot. 123, 109–117. doi: 10.1007/s41348-016-0017-0
Beever, R. E., and Weeds, P. L. (2007). “Taxonomy and genetic variation of botrytis and botryotinia,” in Botrytis: Biology, Pathology and Control, eds Y. Elad, B. Williamson, P. Tudzynski, and N. Delen (Dorldrecht: Springer), 29–52. doi: 10.1007/978-1-4020-2626-3_3
Beyene, A. T., Derera, J., Sibiya, J., and Fikre, A. (2016). Gene action determining grain yield and chocolate spot (Botrytis fabae) resistance in faba bean. Euphytica 207, 293–304. doi: 10.1007/s10681-015-1536-7
Binder, M. (2014). Development of a Botrytis Specific Immunosensor: Towards Using PCR Species Identification. Ph. D Thesis, University of Cranfield, Cranfield.
Bouhassan, A., Sadiki, M., and Tivoli, B. (2004). Evaluation of a collection of faba bean (Vicia faba L.) genotypes originating from the Maghreb for resistance to chocolate spot (Botrytis fabae) by assessment in the field and laboratory. Euphytica 135, 55–62. doi: 10.1023/B:EUPH.0000009540.98531.4d
Brouwer, M., Lievens, B., Van Hemelrijck, W., Van Den Ackerveken, G., Cammue, B. P. A., and Thomma, B. P. H. J. (2003). Quantification of disease progression of several microbial pathogens on Arabidopsis thaliana using real-time fluorescence PCR. FEMS Microbiol. Lett. 228, 241–248. doi: 10.1016/s0378-1097(03)00759-6
Butler, E. J., and Bisby, G. R. (1931). The fungi of India. Imp. Coun. Agri. Res. India Sci. Mono. 18:237.
Cadle-Davidson, L. (2008). Monitoring pathogenesis of natural Botrytis cinerea infections in developing grape berries. Am. J. Enol. Vitic. 59, 387–395.
Carisse, O., Tremblay, D. M., Lévesque, C. A., Gindro, K., Ward, P., and Houde, A. (2009). Development of a TaqMan real-time PCR assay for quantification of airborne conidia of Botrytis squamosa and management of botrytis leaf blight of onion. Phytopathology 99, 1273–1280. doi: 10.1094/PHYTO-99-11-1273
Casanova-Moreno, J., To, J., Yang, C. W. T., Turner, R. F. B., Bizzotto, D., and Cheung, K. C. (2017). Fabricating devices with improved adhesion between PDMS and gold-patterned glass. Sens. Actuators B Chem. 246, 904–909. doi: 10.1016/j.snb.2017.02.109
Celik, M., Kalpulov, T., Zutahy, Y., Ish-shalom, S., Lurie, S., and Lichter, A. (2009). Quantitative and qualitative analysis of Botrytis inoculated on table grapes by qPCR and antibodies. Postharvest Biol. Technol. 52, 235–239. doi: 10.1016/j.postharvbio.2008.10.007
Cernay, C., Pelzer, E., and Makowski, D. (2016). Data descriptor: a global experimental dataset for assessing grain legume production. Sci. Data 3:160084. doi: 10.1038/sdata.2016.84
Charlermroj, R., Himananto, O., Seepiban, C., Kumpoosiri, M., Warin, N., Oplatowska, M., et al. (2013). Multiplex detection of plant pathogens using a microsphere immunoassay technology. PLoS One 8:e62344. doi: 10.1371/journal.pone.0062344
Chen, X., Ma, L., Qiang, S., and Ma, D. (2016). Development of a loop-mediated isothermal amplification method for the rapid diagnosis of Ascochyta rabiei L. in chickpeas. Sci. Rep. 6:25688. doi: 10.1038/srep25688
Chikkaveeraiah, B. V., Bhirde, A. A., Morgan, N. Y., Eden, H. S., and Chen, X. (2012). Electrochemical immunosensors for detection of cancer protein biomarkers. ACS Nano 6, 6546–6561. doi: 10.1021/nn3023969
Chilvers, M. I., du Toit, L. J., Akamatsu, H., and Peever, T. L. (2007). A real-time, quantitative PCR seed assay for Botrytis spp. that Cause Neck Rot of Onion. Plant Dis. 91, 599–608. doi: 10.1094/PDIS-91-5-0599
Chilvers, M. I., and Lindsey, J. (2006). Detection and identification of Botrytis species associated with neck rot, scape blight, and umbel blight of onion. Plant Heal. Prog. 7. doi: 10.1094/PHP-2006-1127-01-DG
Coolong, T. W., Walcott, R. R., and Randle, W. M. (2008). A quantitative real-time polymerase chain reaction assay for Botrytis aclada in onion bulb tissue. Am. Soc. Hortic. Sci. 43, 408–413. doi: 10.21273/hortsci.43.2.408
Damborský, P., Švitel, J., and Katrlík, J. (2016). Optical biosensors. Essays Biochem. 60, 91–100. doi: 10.1042/EBC20150010
Davidson, J. A., Pande, S., Bretag, T. W., Lindbeck, K. D., and Krishna-Kishore, G. (2007). “Biology and management of Botrytis spp. in legume crops,” in Botrytis: Biology, Pathology and Control, eds Y. Elad, B. Williamson, P. Tudzynski, and N. Delen (Dordrecht: Springer), 295–318. doi: 10.1007/978-1-4020-2626-3_16
Dewey, F. M., Ebeler, S. E., Adams, D. O., Noble, A. C., and Meyer, U. M. (2000). Quantification of Botrytis in grape juice determined by a monoclonal antibody-based immunoassay. Am. J. Enol. Vitic. 51, 276–282.
Dewey, F. M., and Yohalem, D. (2007). “Detection, quantification and immunolocalisation of Botrytis species,” in Botrytis: Biology, Pathology and Control, eds Y. Elad, B. Williamson, P. Tudzynski, and N. Delen (Dordrecht: Springer), 181–194. doi: 10.1007/978-1-4020-2626-3_11
Diguta, C. F., Rousseaux, S., Weidmann, S., Bretin, N., Vincent, B., Guilloux-Benatier, M., et al. (2010). Development of a qPCR assay for specific quantification of Botrytis cinerea on grapes. FEMS Microbiol. Lett. 313, 81–87. doi: 10.1111/j.1574-6968.2010.02127.x
Ding, L., Bond, A. M., Zhai, J., and Zhang, J. (2013). Utilization of nanoparticle labels for signal amplification in ultrasensitive electrochemical affinity biosensors: a review. Anal. Chim. Acta 797, 1–12. doi: 10.1016/j.aca.2013.07.035
Duan, Y.-B., Ge, C.-Y., Zhang, X.-K., Wang, J.-X., and Zhou, M.-G. (2014). Development and evaluation of a novel and rapid detection assay for Botrytis cinerea based on loop-mediated isothermal amplification. PLoS One 9:e111094. doi: 10.1371/journal.pone.0111094
Eikemo, H., Strømeng, G. M., and Stensvand, A. (2013). Evaluation of two models to predict grey mould in strawberry. Eur. J. Hortic. Sci. 78, 40–46.
Fan, X., Zhang, J., Yang, L., Wu, M., Chen, W., and Li, G. (2015). Development of PCR-Based assays for detecting and differentiating three species of Botrytis infecting Broad Bean. Plant Dis. 99, 691–698. doi: 10.1094/PDIS-07-14-0701-RE
Fernández-Baldo, M. A., Fernández, J. G., Pereira, S. V., Messina, G. A., Salinas, E., Raba, J., et al. (2011). Development of an indirect competitive enzyme-linked immunosorbent assay applied to the Botrytis cinerea quantification in tissues of postharvest fruits. BMC Microbiol. 11:220. doi: 10.1186/1471-2180-11-220
Fernández-Baldo, M. A., Messina, G. A., Sanz, M. I., and Raba, J. (2010). Microfluidic immunosensor with micromagnetic beads coupled to carbon-based screen-printed electrodes (SPCEs) for determination of Botrytis cinerea in tissue of fruits. J. Agric. Food Chem. 58, 11201–11206. doi: 10.1021/jf1025604
Gachon, C., and Saindrenan, P. (2004). Real-time PCR monitoring of fungal development in Arabidopsis thaliana infected by Alternaria brassicicola and Botrytis cinerea. Plant Physiol. Biochem. 42, 367–371. doi: 10.1016/j.plaphy.2004.04.001
González-Domínguez, E., Caffi, T., Ciliberti, N., and Rossi, V. (2015). A mechanistic model of Botrytis cinerea on grapevines that includes weather, vine growth stage, and the main infection pathways. PLoS One 10:e0140444. doi: 10.1371/journal.pone.0140444
Gooding, J. J. (2002). Electrochemical DNA hybridization biosensors. Electroanalysis 14, 1149–1156. doi: 10.1002/1521-4109(200209)14:17<1149::aid-elan1149>3.0.co;2-8
Goto, M., Honda, E., Ogura, A., Nomoto, A., and Hanaki, K. I. (2009). Colorimetric detection of loop-mediated isothermal amplification reaction by using hydroxy naphthol blue. Biotechniques 46, 167–172. doi: 10.2144/000113072
Harrison, J. G. (1988). The biology of Botrytis spp. on Vicia beans and chocolate spot disease-a review. Plant Pathol. 37, 168–201. doi: 10.1111/j.1365-3059.1988.tb02064.x
Hatamie, A., Echresh, A., Zargar, B., Nur, O., and Willander, M. (2015). Fabrication and characterization of highly-ordered Zinc Oxide nanorods on gold/glass electrode, and its application as a voltammetric sensor. Electrochim. Acta 174, 1261–1267. doi: 10.1016/j.electacta.2015.06.083
Herbert, K., Powell, K., Mckay, A., Hartley, D., Herdina, Ophel-Keller, K., et al. (2008). Developing and testing a diagnostic probe for grape phylloxera applicable to soil samples. J. Econ. Entomol. 101, 1934–1943. doi: 10.1603/0022-0493-101.6.1934
Holford, T. R. J., Davis, F., and Higson, S. P. J. (2012). Recent trends in antibody based sensors. Biosens. Bioelectron. 34, 12–24. doi: 10.1016/j.bios.2011.10.023
Islam, M. N., Masud, M. K., Haque, M. H., Hossain, M. S., Al Yamauchi, Y., Nguyen, N.-T., et al. (2017). RNA biomarkers: diagnostic and prognostic potentials and recent developments of electrochemical biosensors. Small Methods 1:1700131. doi: 10.1002/smtd.201700131
Kennedy, R., Wakeham, A. J., Byrne, K. G., Meyer, U. M., and Dewey, F. M. (2000). A new method to monitor airborne inoculum of the fungal plant pathogens Mycosphaerella brassicicola and Botrytis cinerea. Appl. Environ. Microbiol. 66, 2996–3003. doi: 10.1128/AEM.66.7.2996-3003.2000
Khater, M., de la Escosura-Muñiz, A., and Merkoçi, A. (2017). Biosensors for plant pathogen detection. Biosens. Bioelectron. 93, 72–86. doi: 10.1016/j.bios.2016.09.091
Khazaeli, P., Zamanizadeh, H., Morid, B., and Bayat, H. (2010). Morphological and molecular identification of Botrytis Cinerea causal agent of gray mold in rose greenhouses in Centeral Regions of Iran. Int. J. Agric. Sci. Res. 1, 19–24.
Komárek, M., Čadková, E., Chrastný, V., Bordas, F., and Bollinger, J. C. (2010). Contamination of vineyard soils with fungicides: a review of environmental and toxicological aspects. Environ. Int. 36, 138–151. doi: 10.1016/j.envint.2009.10.00
Kraft, J. M., and Pfleger, F. L. (eds) (2001). Compendium of Pea Diseases and Pests. St. Paul, MN: American Phytopathological Society (APS Press).
Lau, H. Y., Wang, Y., Wee, E. J. H., Botella, J. R., and Trau, M. (2016). Field demonstration of a multiplexed point-of-care diagnostic platform for plant pathogens. Anal. Chem. 88, 8074–8081. doi: 10.1021/acs.analchem.6b01551
Lau, H. Y., Wu, H., Wee, E. J. H., Trau, M., Wang, Y., and Botella, J. R. (2017). Specific and sensitive isothermal electrochemical biosensor for plant pathogen DNA detection with colloidal gold nanoparticles as probes. Sci. Rep. 7:38896. doi: 10.1038/srep38896
Levicky, R., Herne, T. M., Tarlov, M. J., and Satija, S. K. (1998). Using self-assembly to control the structure of DNA monolayers on gold: a neutron reflectivity study. J. Am. Chem. Soc. 120, 9787–9792. doi: 10.1021/ja981897r
Li, Y., Deng, J., Fang, L., Yu, K., Huang, H., Jiang, L., et al. (2015). A novel electrochemical DNA biosensor based on HRP-mimicking hemin/G-quadruplex wrapped GOx nanocomposites as tag for detection of Escherichia coli O157: H7. Biosens. Bioelectron. 63, 1–6. doi: 10.1016/j.bios.2014.07.012
Li, Y., Sun, S., Du, C., Xu, C., Zhang, J., Duan, C., et al. (2016). A new disease of mung bean caused by Botrytis cinerea. Crop Prot. 85, 52–56. doi: 10.1016/j.cropro.2016.03.020
Lindbeck, K. D., Bretag, T. W., and Ford, R. (2009). Survival of Botrytis spp. on infected lentil and chickpea trash in Australia. Australas. Plant Pathol. 38, 399–407. doi: 10.1071/AP09015
Lindbeck, K. D., Bretag, T. W., and Materne, M. A. (2008). Field screening in Australia of lentil germplasm for resistance to botrytis grey mould. Australas. Plant Pathol. 37, 373–378. doi: 10.1071/AP08012
Linfield, C. A., Kenny, S. R., and Lyons, N. F. (1995). A serological test for detecting Botrytis allii, the cause of neck rot of onion bulbs. Ann. Appl. Biol. 126, 259–268. doi: 10.1111/j.1744-7348.1995.tb05364.x
Linting, Z., Ruiyi, L., Zaijun, L., Qianfang, X., Yinjun, F., and Junkang, L. (2012). An immunosensor for ultrasensitive detection of aflatoxin B1 with an enhanced electrochemical performance based on graphene/conducting polymer/gold nanoparticles/the ionic liquid composite film on modified gold electrode with electrodeposition. Sensors Actuators B Chem. 174, 359–365. doi: 10.1016/j.snb.2012.06.051
Liu, A., Wang, K., Weng, S., Lei, Y., Lin, L., Chen, W., et al. (2012). Development of electrochemical DNA biosensors. TrAC Trends Anal. Chem. 37, 101–111. doi: 10.1016/j.trac.2012.03.008
Liu, X., Li, W.-J., Li, L., Yang, Y., Mao, L.-G., and Peng, Z. (2014). A label-free electrochemical immunosensor based on gold nanoparticles for direct detection of atrazine. Sensors Actuators B Chem. 191, 408–414. doi: 10.1016/j.snb.2013.10.033
Liu, X., Shuai, H. L., Liu, Y. J., and Huang, K. J. (2016). An electrochemical biosensor for DNA detection based on tungsten disulfide/multi-walled carbon nanotube composites and hybridization chain reaction amplification. Sensors Actuators B Chem. 235, 603–613. doi: 10.1016/j.snb.2016.05.132
Lucarelli, F., Marrazza, G., Turner, A. P. F., and Mascini, M. (2004). Carbon and gold electrodes as electrochemical transducers for DNA hybridisation sensors. Biosens. Bioelectron. 19, 515–530. doi: 10.1016/S0956-5663(03)00256-2
Lucarelli, F., Tombelli, S., Minunni, M., Marrazza, G., and Mascini, M. (2008). Electrochemical and piezoelectric DNA biosensors for hybridisation detection. Anal. Chim. Acta. 609, 139–159. doi: 10.1016/j.aca.2007.12.035
Marques, R. C. B., Costa-Rama, E., Viswanathan, S., Nouws, H. P. A., Costa-García, A., Delerue-Matos, C., et al. (2018). Voltammetric immunosensor for the simultaneous analysis of the breast cancer biomarkers CA 15-3 and HER2-ECD. Sensors Actuators B Chem. 255, 918–925. doi: 10.1016/j.snb.2017.08.107
Martin, R. R., Delano, J., and Lévesque, A. (2000). Impacts of molecular diagnostic technologies on plant disease management. Annu. Rev. Plant Phytopathol. 38, 207–239. doi: 10.1146/annurev.phyto.38.1.207
Mascini, M., and Tombelli, S. (2008). Biosensors for biomarkers in medical diagnostics. Biomarkers 13, 637–657. doi: 10.1080/13547500802645905
McCann, M. C., Chen, L., Roberts, K., Kemsley, E. K., Sene, C., Carpita, N. C., et al. (1997). Infrared microspectroscopy: sampling heterogeneity in plant cell wall composition and architecture. Physiol. Plant. 100, 729–738. doi: 10.1034/j.1399-3054.1997.1000337.x
Mehli, L., Kjellsen, T. D., Dewey, F. M., and Hietala, A. M. (2005). A case study from the interaction of strawberry and Botrytis cinerea highlights the benefits of comonitoring both partners at genomic and mRNA level. New Phytol. 168, 465–474. doi: 10.1111/j.1469-8137.2005.01526.x
Mehrotra, P. (2016). Biosensors and their applications - a review. J. Oral Biol. Craniofacial Res. 6, 153–159. doi: 10.1016/j.jobcr.2015.12.002
Mendes, R. K., Carvalhal, R. F., Stach-Machado, D. R., and Kubota, L. T. (2009). Surface plasmon resonance immunosensor for early diagnosis of Asian rust on soybean leaves. Biosens. Bioelectron. 24, 2483–2487. doi: 10.1016/j.bios.2008.12.033
Mertely, J. C., MacKenzie, S. J., and Legard, D. E. (2002). Timing of fungicide applications for Botrytis cinerea based on development stage of strawberry flowers and fruit. Plant Dis. 86, 1019–1024. doi: 10.1094/PDIS.2002.86.9.1019
Meyer, U. M., Spotts, R. A., Univer-, O. S., River, H., Dewey, F. M., Sciences, P., et al. (2000). Detection and quantification of Botrytis cinerea by ELISA in pear stems during cold storage. Plant Dis. 84, 1099–1103. doi: 10.1094/PDIS.2000.84.10.1099
Molly, F. M. D., and Grant-Downton, R. (2015). “Botrytis-biology, detection and quantification,” in Botrytis - The Fungus, the Pathogen and its Management in Agricultural Systems, eds S. Fillinger, and Y. Elad (Cham: Springer), 17–34. doi: 10.1007/978-3-319-23371-0_2
Mori, Y., Nagamine, K., Tomita, N., and Notomi, T. (2001). Detection of loop-mediated isothermal amplification reaction by turbidity derived from magnesium pyrophosphate formation. Biochem. Biophys. Res. Commun. 289, 150–154. doi: 10.1006/bbrc.2001.5921
Murray, G. M., and Brennan, J. P. (2012). The Current and Potential Costs from Diseases of Pulse Crops in Australia. Canberra, VIC: Grains Resaerch and Development Cooperation.
Nielsen, K., and Protection, C. (2002). PCR detection and RFLP differentiation of Botrytis species associated with neck rot of onion. Plant Dis. 86, 682–686. doi: 10.1094/PDIS.2002.86.6.682
Notomi, T., Okayama, H., Masubuchi, H., Yonekawa, T., Watanabe, K., Amino, N., et al. (2000). Loop-mediated isothermal amplification of DNA. Nucleic Acids Res. 28:E63. doi: 10.1093/nar/28.12.e63
Obanor, F. O., Williamson, K., Mundy, D. C., Wood, P. N., and Walter, M. (2004). Optimisation of PTA-ELISA detection and quantification of Botrytis cinerea infections in grapes. New Zeal. Plant Prot. 57, 130–137.
Pande, S., Galloway, J., Gaur, P. M., Siddique, K. H. M., Tripathi, H. S., Taylor, P., et al. (2006). Botrytis grey mould of chickpea: a review of biology, epidemiology, and disease management. Aust. J. Agric. Res. 57, 1137–1150. doi: 10.1071/AR06120
Pande, S., Sharma, M., Kumari, S., Gaur, P., Chen, W., Kaur, L., et al. (2009). “Integrated foliar diseases management of legumes,” in Proceedings of the International Conference on Grain Legumes: Quality Improvement, Value Addition and Trade, (Kanpur), 143–161.
Phan, H. T. T., Ford, R., Bretag, T., and Taylor, P. W. J. (2002). A rapid and sensitive polymerase chain reaction (PCR) assay for detection of Ascochyta rabiei, the cause of ascochyta blight of chickpea. Australas. Plant Pathol. 31, 31–39. doi: 10.1071/AP01056
Pöhlmann, C., Dieser, I., and Sprinzl, M. (2014). A lateral flow assay for identification of Escherichia coli by ribosomal RNA hybridisation. Analyst 139, 1063–1071. doi: 10.1039/c3an02059b
Rama, E. C., and Costa-García, A. (2016). Screen-printed electrochemical immunosensors for the detection of cancer and cardiovascular biomarkers. Electroanalysis 28, 1700–1715. doi: 10.1002/elan.201600126
Ray, M., Ray, A., Dash, S., Mishra, A., Achary, K. G., Nayak, S., et al. (2017). Fungal disease detection in plants: traditional assays, novel diagnostic techniques and biosensors. Biosens. Bioelectron. 87, 708–723. doi: 10.1016/j.bios.2016.09.032
Regiart, M., Rinaldi-Tosi, M., Aranda, P. R., Bertolino, F. A., Villarroel-Rocha, J., Sapag, K., et al. (2017). Development of a nanostructured immunosensor for early and in situ detection of Xanthomonas arboricola in agricultural food production. Talanta 175, 535–541. doi: 10.1016/j.talanta.2017.07.086
Ricker, R. W., Marois, J. J., Dlott, J. W., Bostock, R. M., and Morrison, J. C. (1991). Immunodetection and quantification of Botrytis cinerea on harvested wine grapes. Phytopathology 81, 404–411. doi: 10.1094/Phyto-81-404
Rigotti, S., Gindro, K., Richter, H., and Viret, O. (2002). Characterization of molecular markers for specific and sensitive detection of Botrytis cinerea Pers.: Fr. in strawberry (Fragaria × ananassa Duch.) using PCR. FEMS Microbiol. Lett. 209, 169–174. doi: 10.1016/s0378-1097(02)00491-3
Rigotti, S., Viret, O., and Gindro, K. (2006). Two new primers highly specific for the detection of Botrytis cinerea Pers.: Fr. Phytopathol. Mediterr. 45, 253–260.
Rupp, S., Weber, R. W. S., Rieger, D., Detzel, P., and Hahn, M. (2017). Spread of Botrytis cinerea strains with multiple fungicide resistance in german horticulture. Front. Microbiol. 7:2075. doi: 10.3389/fmicb.2016.02075
Schaad, N. W., and Frederick, R. D. (2002). Real-time PCR and its application for rapid plant disease diagnostics. Can. J. Plant Pathol. 24, 250–258. doi: 10.1080/07060660209507006
Sharma, A., Kaushal, A., and Kulshrestha, S. (2017). A Nano-Au/C-MWCNT based label free amperometric immunosensor for the detection of capsicum chlorosis virus in bell pepper. Arch. Virol. 162, 2047–2052. doi: 10.1007/s00705-017-3293-5
Shaw, F. J. W., and Ajrekar, S. L. (1915). The genus Rhizoctonia in India. Dep. Agric. Indian Bot. Surv. 7, 177–194.
Skottrup, P., Hearty, S., Frøkiaer, H., Leonard, P., Hejgaard, J., O’Kennedy, R., et al. (2007a). Detection of fungal spores using a generic surface plasmon resonance immunoassay. Biosens. Bioelectron. 22, 2724–2729. doi: 10.1016/j.bios.2006.11.017
Skottrup, P., Nicolaisen, M., and Justesen, A. F. (2007b). Rapid determination of Phytophthora infestans sporangia using a surface plasmon resonance immunosensor. J. Microbiol. Methods 68, 507–515. doi: 10.1016/j.mimet.2006.10.011
Skottrup, P. D., Nicolaisen, M., and Justesen, A. F. (2008). Towards on-site pathogen detection using antibody-based sensors. Biosens. Bioelectron. 24, 339–348. doi: 10.1016/j.bios.2008.06.045
Smýkal, P., Coyne, C. J., Ambrose, M. J., Maxted, N., Schaefer, H., Blair, M. W., et al. (2015). Legume crops phylogeny and genetic diversity for science and breeding. CRC Crit. Rev. Plant Sci. 34, 43–104. doi: 10.1080/07352689.2014.897904
Spotts, R. A., Wallis, K. M., Serdani, M., O’Gorman, D. T., and Sholberg, P. L. (2008). Methodology for determining relationships between inoculum concentration of Botrytis cinerea and Penicillium expansum and stem end decay of pear fruit. Plant Dis. 92, 451–455. doi: 10.1094/PDIS-92-3-0451
Suarez, M. B., Walsh, K., Boonham, N., O’Neill, T., Pearson, S., and Barker, I. (2005). Development of real-time PCR (TaqMan®) assays for the detection and quantification of Botrytis cinerea in planta. Plant Physiol. Biochem. 43, 890–899. doi: 10.1016/j.plaphy.2005.07.003
Taylor, P. W. J., and Ford, R. (2007). “Diagnostics, genetic diversity and pathogenic variation of Ascochyta blight of cool season food and feed legumes,” in Ascochyta Blights of Grain Legumes, eds B. Tivoli, A. Baranger, F. J. Muehlbauer, and B. M. Cooke (Dordrecht: Springer), doi: 10.1007/978-1-4020-6065-6_13
Tivoli, B., Baranger, A., Avila, C. M., Banniza, S., Barbetti, M., Chen, W., et al. (2006). Screening techniques and sources of resistance to foliar diseases caused by major necrotrophic fungi in grain legumes. Euphytica 147, 223–253. doi: 10.1007/s10681-006-3131-4
Tokel, O., Inci, F., and Demirci, U. (2014). Advances in plasmonic technologies for point of care applications. Chem. Rev. 114, 5728–5752. doi: 10.1021/cr4000623
Tomita, N., Mori, Y., Kanda, H., and Notomi, T. (2008). Loop-mediated isothermal amplification (LAMP) of gene sequences and simple visual detection of products. Nat. Protoc. 3, 877–882. doi: 10.1038/nprot.2008.57
Tomlinson, J. A., Dickinson, M. J., and Boonham, N. (2010). Detection of Botrytis cinerea by loop-mediated isothermal amplification. Lett. Appl. Microbiol. 51, 650–657. doi: 10.1111/j.1472-765X.2010.02949.x
Torrance, L., Ziegler, A., Pittman, H., Paterson, M., Toth, R., and Eggleston, I. (2006). Oriented immobilisation of engineered single-chain antibodies to develop biosensors for virus detection. J. Virol. Methods 134, 164–170. doi: 10.1016/j.jviromet.2005.12.012
Tran, H. V., Yougnia, R., Reisberg, S., Piro, B., Serradji, N., Nguyen, T. D., et al. (2012). A label-free electrochemical immunosensor for direct, signal-on and sensitive pesticide detection. Biosens. Bioelectron. 31, 62–68. doi: 10.1016/j.bios.2011.09.035
Wang, Y., Ye, Z., and Ying, Y. (2012). New trends in impedimetric biosensors for the detection of foodborne pathogenic bacteria. Sensors 12, 3449–3471. doi: 10.3390/s120303449
Wee, E. J. H., Lau, H. Y., Botella, J. R., and Trau, M. (2015). Re-purposing bridging flocculation for on-site, rapid, qualitative DNA detection in resource-poor settings. Chem. Commun. 51, 5828–5831. doi: 10.1039/C4CC10068A
Xu, M., Wang, R., and Li, Y. (2016). Rapid detection of Escherichia coli O157:H7 and Salmonella typhimurium in foods using an electrochemical immunosensor based on screen-printed interdigitated microelectrode and immunomagnetic separation. Talanta 148, 200–208. doi: 10.1016/j.talanta.2015.10.082
Xu, S., Ji, X., Xu, W., Li, X., Wang, L., Bai, Y., et al. (2004). Immunoassay using probe-labelling immunogold nanoparticles with silver staining enhancement via surface-enhanced Raman scattering. Analyst 129, 63–68. doi: 10.1039/b313094k
Yohalem, D. S., Nielsen, K., Green, H., and Jensen, D. F. (2004). Biocontrol agents efficiently inhibit sporulation of Botrytis aclada on necrotic leaf tips but spread to adjacent living tissue is not prevented. FEMS Microbiol. Ecol. 47, 297–303. doi: 10.1016/S0168-6496(03)00299-X
Yoo, S. M., and Lee, S. Y. (2015). Optical biosensors for the detection of pathogenic microorganisms. Trends Biotechnol. 34, 7–25. doi: 10.1016/j.tibtech.2015.09.012
Yoo, S. M., and Lee, S. Y. (2016). Optical biosensors for the detection of pathogenic microorganisms. Trends Biotechnol. 34, 7–25. doi: 10.1016/j.tibtech.2015.09.01
Zezza, F., Pascale, M., Mulè, G., and Visconti, A. (2006). Detection of Fusarium culmorum in wheat by a surface plasmon resonance-based DNA sensor. J. Microbiol. Methods 66, 529–537. doi: 10.1016/j.mimet.2006.02.003
Zhang, J., Wu, M.-D., Li, G.-Q., Yang, L., Yu, L., Jiang, D.-H., et al. (2010). Botrytis fabiopsis, a new species causing chocolate spot of broad bean in central China. Mycologia 102, 1114–1126. doi: 10.3852/09-217
Zhao, J., Zhang, Y., Li, H., Wen, Y., Fan, X., Lin, F., et al. (2011). Ultrasensitive electrochemical aptasensor for thrombin based on the amplification of aptamer-AuNPs-HRP conjugates. Biosens. Bioelectron. 26, 2297–2303. doi: 10.1016/j.bios.2010.09.056
Keywords: Botrytis gray mold, biosensor, diagnosis, legumes, nanotechnology, nano-biosensor
Citation: Bilkiss M, Shiddiky MJA and Ford R (2019) Advanced Diagnostic Approaches for Necrotrophic Fungal Pathogens of Temperate Legumes With a Focus on Botrytis spp.. Front. Microbiol. 10:1889. doi: 10.3389/fmicb.2019.01889
Received: 05 December 2018; Accepted: 30 July 2019;
Published: 14 August 2019.
Edited by:
Luis F. Larrondo, Pontifical Catholic University of Chile, ChileReviewed by:
Ram Prasad, Amity University, IndiaEvelyn Silva-Moreno, Universidad Autónoma de Chile, Chile
Copyright © 2019 Bilkiss, Shiddiky and Ford. This is an open-access article distributed under the terms of the Creative Commons Attribution License (CC BY). The use, distribution or reproduction in other forums is permitted, provided the original author(s) and the copyright owner(s) are credited and that the original publication in this journal is cited, in accordance with accepted academic practice. No use, distribution or reproduction is permitted which does not comply with these terms.
*Correspondence: Rebecca Ford, cmViZWNjYS5mb3JkQGdyaWZmaXRoLmVkdS5hdQ==