- 1Instituto de Biología Funcional y Genómica, Consejo Superior de Investigaciones Científicas, Universidad de Salamanca, Salamanca, Spain
- 2Department of Biochemistry and Medical Chemistry, University of Pécs Medical School, Pécs, Hungary
- 3Department of Medical Microbiology and Immunology, University of Pécs Medical School, Pécs, Hungary
A series of 4-(arylmethylene)-3-isochromanones have been prepared with base-catalyzed Knoevenagel condensation starting from 3-isochromanone and aromatic aldehydes. The outcome of the reaction- the isomeric composition of the products depends on the aromatic aldehyde applied. These reactions afforded mostly the more stable E-diastereoisomer, but some condensations resulted in the Z-diastereoisomer or mixture of the stereoisomers (1–16). The products showed antifungal effect against some pathogenic fungi. We wanted to extend this study and to synthesize a new generation of 4-(arylmethylene)-3-isochromanones. These condensations led mostly to E-diastereoisomers (17–30). The structure verifications were performed by FT IR, 1H and13C NMR methods. Both the 1–16 and the novel 17–30 compounds have been screened against the three yeast models, fission yeast Schizosaccharomyces pombe (wild-type, and pbr1-6 and pbr1-8 mutants resistant to specific cell wall synthesis inhibitors), budding yeast Saccharomyces cerevisiae (wild-type and pbr1-1) and pathogenic yeast Candida albicans (wild-type, ATCC 26555, 90028 and SC5314). Osmotic protection with sorbitol attenuated the in vivo inhibition in living cells suggesting a cell wall-specific antifungal effect. Moreover, the S. pombe wild-type and mutant strains were tested for their resistant or sensitive in vitro β(1,3)-glucan synthase (GS) activity. We found both in vivo in living cells and in vitro in the enzymatic GS assay a synergistic effect of higher sensitivity of the pbr1 mutants resistant to the specific GS inhibitors papulacandins and echinocandins. These results may provide new insights into new strategies of combined antifungal therapy of GS inhibitors directed against spontaneous mutants resistant to echinocandins.
Introduction
In our previous studies we have dealt with the synthesis of some E-2-arylmethylene-1-tetralones and E-2-heteroarylmethylene-1-tetralones applying base-catalyzed aldol condensation. These compounds were examined for their antimycotic activity using some clinical isolates of the pathogenic fungi Candida albicans, Cryptococcus neoformans, etc. Some of them showed a high antifungal activity with low MIC (Minimal Inhibitory Concentration) values of 1.5 μg/mL and several of the tested compounds exerted better activity than the commercial antifungal standards (Al-Nakib et al., 2001). With the aim of finding new and better specific antifungal inhibitors, we wished to expand these investigations to the analogous α,β-unsaturated heterocyclic ketones. Therefore the principle of the natural product based synthesis was utilized and a molecular library of 4-(arylmethylene)-3-isochromanones was prepared via base-catalyzed Knoevenagel condensations, i.e., the first generation of isochromanones (1—16, see in Figure 1 and Table 1) (Lóránd et al., 2002). Using phenolic aldehydes –with ortho-OH group- in this reaction resulted in the formation of the corresponding coumarines (12, 15) due to a second intramolecular reaction (Figure 2) (Lóránd et al., 2002). (Compounds of isochromane skeleton occur in the nature as the tricyclic fusarubin, showing antibacterial and antifungal effect) (Ruelius and Gauhe, 1950). Several members of the first generation of 4-(arylmethylene)-3-isochromanones showed excellent antifungal activity using some clinical isolates of C. albicans, C. neoformans, etc. (Lóránd et al., 1998). In order to collect a larger molecular library for biological investigations we planned to prepare a new class of isochromanones. We wished to explore the antifungal activity of the two families of isochromanones. The in vivo inhibitory capacity of the first family against clinical isolates of C. albicans or C. neoformans has been shown (Lóránd et al., 1998). Thus our aim was to extend or investigate the in vivo inhibitory capacity on the living cells of both families of isochromanones and to study the possible in vitro inhibitory capacity of both families of the β(1,3)-glucan synthase (GS) activity in order to find new families of specific fungal cell wall synthesis inhibitors. The cell wall is an essential structure for the fungal cells that is absent in animal cells (Free, 2013; Cortés et al., 2016; Gow et al., 2017; Hopke et al., 2018) therefore representing a very useful target in the search for new, more efficient and selective drugs for the treatment of invasive fungal infections without causing toxicity in the animal host cells (Bal, 2010; Hector and Bierer, 2011; Brown et al., 2012; Kathiravan et al., 2012; Cortés et al., 2019). In this study, we used the following wild-type or papulacandin and echinocandin resistant mutant strains: Schizosaccharomyces pombe wild-type, and pbr1-8 and pbr1-6 resistant mutants (Castro et al., 1995; Martins et al., 2011), Saccharomyces cerevisiae wild-type and pbr1-1 resistant mutant (Castro et al., 1995), and several C. albicans wild-type strains.
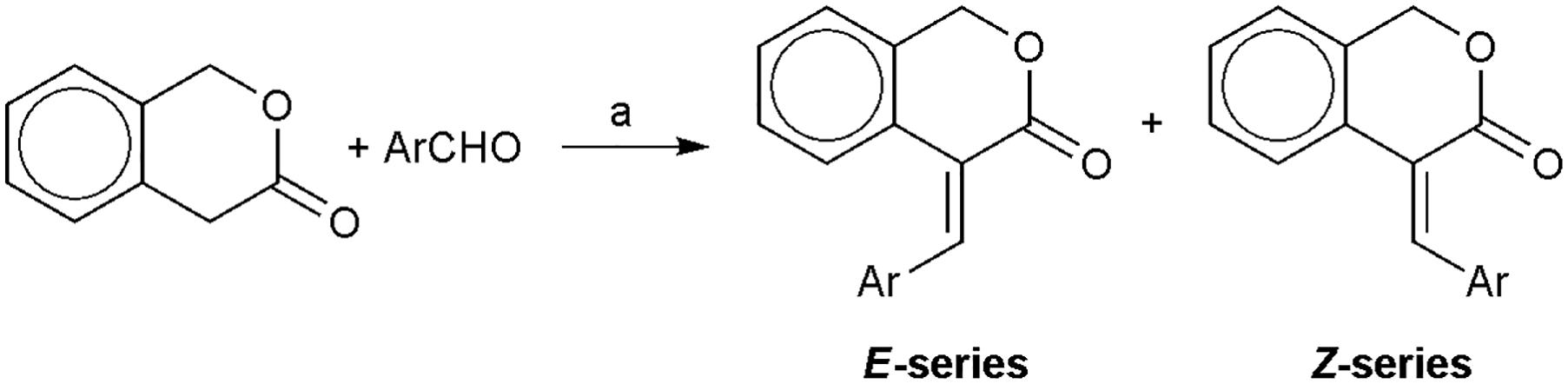
Figure 1. Synthetic route of the 4-(arylmethylene)-3-isochromanones; Reagents and conditions: a) piperidine, 140°C, Ar.
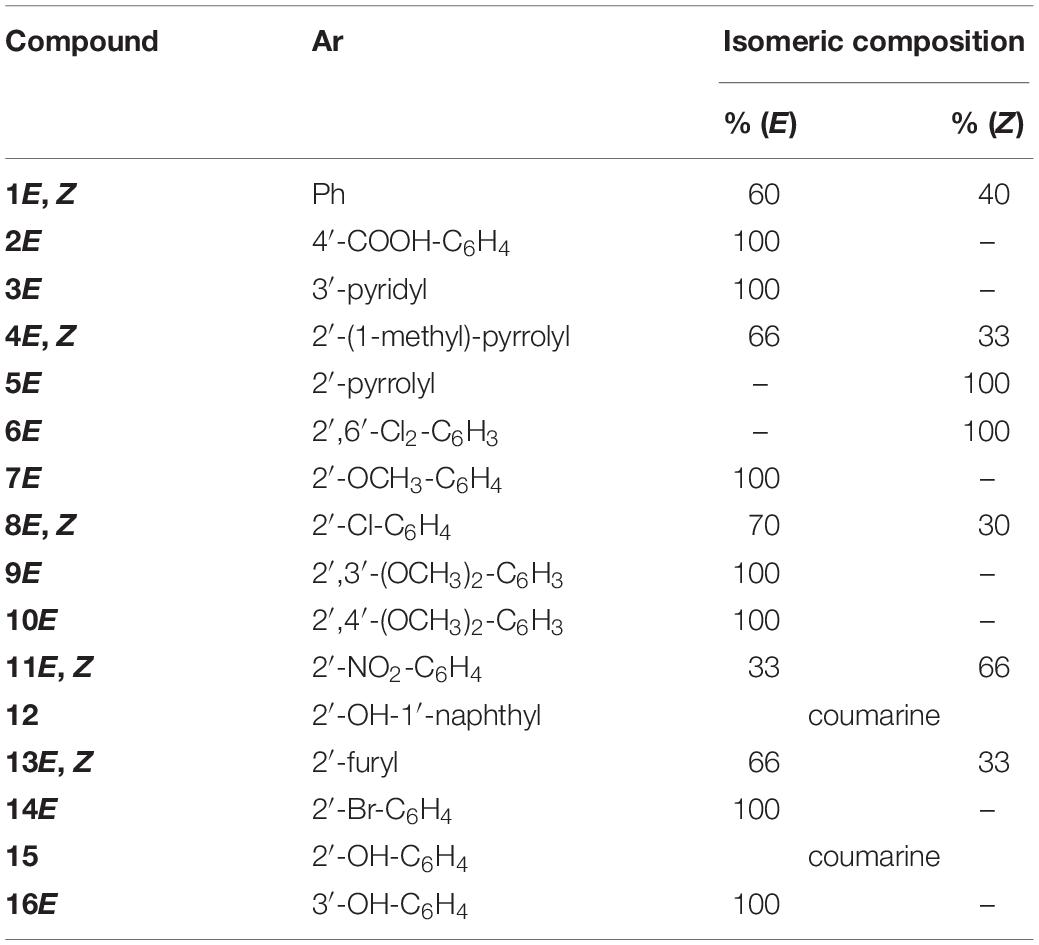
Table 1. Structure and isomeric composition of the first generation of second generation of isochromanones (1–16).
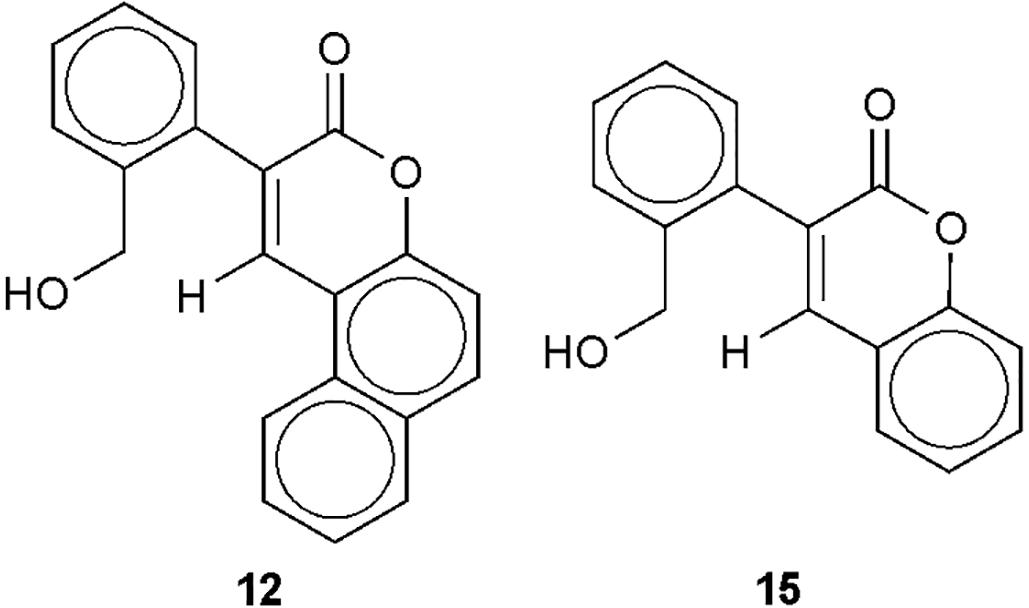
Figure 2. The structure of coumarines (12,15) formed in the condensations with some hydroxyaldehydes.
Materials and Methods
General Procedures for the Synthesis of Compounds
All of the reagents were purchased from Sigma-Aldrich Company and were used without further purifications. The purification of the novel compounds was performed with column chromatography on Sigma-Aldrich silica gel (pore size: 60 Å, particle size 230–400 mesh). The analytical thin-layer chromatography was performed on Merck silica gel plates (60 F254) and the eluents used are described in the next chapter. NMR spectra were recorded on a Bruker Avance III Ascend 500 spectrometer (500/125 MHz for 1H/13C); chemical shifts are referenced to residual solvent signals. Measurements were performed at a probe temperature of 298 K in solution with an appropriate solvent. The FT IR spectra were run on an Impact 400 (Nicolet) FT IR spectrophotometer in KBr pellets using a KBr pellet as the background reference spectrum. Infrared spectra were obtained between 400 and 4000 cm–1 with a spectral resolution of 4 cm–1 Melting points were measured with a Boethius hot plate apparatus and are uncorrected. The spectroscopic data of the title compounds can be found in the Supplementary Material.
Synthesis of 4-Arylmethylene-3-Isochromanones (17–30) (Lóránd et al., 2002)
The equimolar mixture of 3-isochromanone (3.38 mmol) and the corresponding aromatic aldehyde (3.38 mmol) was heated (140°C) in the presence of catalytic amount of piperidine (four drops) in argon atmosphere under stirring for 1 h. Then, the reaction mixture was taken up in ethanol and cooled down. The crystals separated were filtered off and washed with cold ethanol. The products were purified by means of column chromatography. (See details at the compounds.) The conversion was always 100%.
Synthesis of E-4-[(4′-Nitrophenyl)Methylene]-3- Isochromanone (17E) and Z-4-[4′-Nitrophenyl)- Methylene]-3-Isochromanone (17Z)
Obtained from a mixture of 3-isochromanone (0.50 g; 3.38 mmol) and 4-nitrobenzaldehyde (0.51 g, 3.38 mmol) with the above method. The reaction mixture was separated by column chromatography (silica gel, CH2Cl2/CH3OH = 10:0.01) to afford 17E (85%) and 17Z (15%) based on separation (0.69 g, 72%). 17E Rf = 0.37 (silica gel, dichloromethane/methanol = 10:0.05), yellow crystalline solid from methanol. m.p. 145–146°C. IR νmax (cm–1) (KBr) 1726 (st, C = O). 1H NMR (500 MHz, dmso-d6) δ (ppm) 5.47 (s, 2H), 7.13 (d, J = 7.6 Hz, 1H), 7.20 (t, J = 7.2 Hz, 1H), 7.38 (dt, J = 7.6, 0.7 Hz, 1H), 7.47 (d, J = 7.6 Hz, 1H), 7.72 (d, J = 8.7 Hz, 2H), 7.79 (s, 1H), 8.21 (d, J = 8.7 Hz, 2H).13C NMR. (125 MHz, dmso-d6) δ (ppm) 68.8, 123.7, 125.8, 127.0, 128.1, 128.2, 129.0, 129.1, 130.4, 133.5, 135.0, 141.3, 147.2, 167.1, 3J(Hα-C3) = 7.1 Hz. Anal Calcd for C16H11NO4: C, 68.33; H, 3.94; Found: C, 68.22; H, 4.11.
Z-4-[(4′-nitrophenyl)methylene]-3-isochromanone (17Z)
It is a yellow crystalline solid from methanol. m.p. 202°C (dec.). IR νmax (cm–1) (KBr) 1723 (st, C = O). Anal Calcd for C16H11N1O4: C, 68.33, H, 3.94; Found: C, 68.12; H, 4.20.
Synthesis of E- 4-[(2′,3′,4′-Trimethylphenyl)Methylene]-3-Isochromanone (18E)
Prepared from a mixture of 3-isochromanone (0.50 g; 3.38 mmol) and mesitaldehyde (98%) (0.51 g, 3.37 mmol) with the above method (0.82 g, 89%). Rf = 0.52 (silica gel, dichloromethane/methanol = 10:0.01), m.p.155°C (dec.) yellow crystalline solid from methanol. IR νmax (cm–1) (KBr) 1726 (st, C = O). Anal Calcd for C19H18O2: C, 81.99; H, 6.52; Found: C, 82.18; H, 6.65.
Synthesis of E-4-[(4′-Methylphenyl)Methylene]- 3-Isochromanone (19E)
Prepared from a mixture of 3-isochromanone (0.50 g; 3.38 mmol) and p-tolualdehyde (0.41 g, 3.38 mmol) with the above method. The reaction mixture was purified via column chromatography (silica gel, toluene/ethyl acetate = 95.5:4.5) to afford 19E (0.49 g, 58%). Rf = 0.49 (silica gel, toluene/ethyl acetate = 10:1), m.p.125–26°C, yellow crystalline solid from methanol. IR νmax (cm–1) (KBr) 1716 (st, C = O). Anal Calcd for C17H14O2: C, 81.58; H, 5.64; Found: C, 80.69; H, 5.70.
Synthesis of E-4-[(2′-Methylphenyl)Methylene]- 3-Isochromanone (20E)
Prepared from a mixture of 3-isochromanone (0.50 g; 3.38 mmol) and o-tolualdehyde (0.41 g, 3.38 mmol) with the above method. The reaction mixture was purified via column chromatography (silica gel, toluene/ethyl acetate = 95.5:4.5) to afford 20E (0.42 g, 50%). Rf = 0.49 (silica gel, toluene/ethyl acetate = 10:1), m.p.78°C (dec.), yellow crystalline solid from methanol. IR νmax (cm–1) (KBr) 1722 (st, C = O). Anal Calcd for C17H14O2: C, 81.58; H, 5.64; Found: C, 81.70; H, 5.79.
Synthesis of E-4-[(4′-Methoxyphenyl)Methylene]-3-Isochromanone (21E)
Prepared from a mixture of 3-isochromanone (0.50 g; 3.38 mmol) and 4-anisaldehyde (0.47 g, 3.34 mmol) with the above method. The reaction mixture was purified via column chromatography (silica gel, toluene/ethyl acetate = 95.5:4.5) to afford 21E (0.73 g, 81%). Rf = 0.43 (silica gel, toluene/ethyl acetate = 10:1), m.p.129–131°C, yellow crystalline solid from methanol. IR νmax (cm–1) (KBr) 1707 (st, C = O). Anal Calcd for C17H14O3: C, 76.68; H, 5.30; Found: C, 76.79; H, 5.49.
Synthesis of E-4-[(3′-Methoxyphenyl)Methylene]-3-Isochromanone (22E)
Prepared from a mixture of 3-isochromanone (0.50 g; 3.38 mmol) and 3-anisaldehyde (0.47 g, 3.34 mmol) with the above method. The reaction mixture was purified via column chromatography (silica gel, toluene/ethyl acetate = 95.5:4.5) to afford 22E (0.59g, 66%), Rf = 0.43 (silica gel, toluene/ethyl acetate = 10:1), m.p.102–103°C, yellow crystalline solid from methanol. IR νmax (cm–1) (KBr) 1719 (st, C = O). Anal Calcd for C17H14O3: C, 76.68; H, 5.30; Found: C, 76.79; H, 5.48.
Synthesis of E-4-[(4′-Chlorophenyl)Methylene]- 3-Isochromanone (23E)
Prepared from a mixture of 3-isochromanone (0.50 g; 3.38 mmol) and 4-chlorobenzaldehyde (0.48 g, 3.34 mmol) with the above method. The reaction mixture was purified via column chromatography (silica gel, toluene/ethyl acetate = 95.5:4.5) to afford 23E (0.68 g, 74%), Rf = 0.44 (silica gel, toluene/ethyl acetate = 10:1), m.p.163–165°C, yellow crystalline solid from methanol. IR νmax (cm–1) (KBr) 1716 (st, C = O). Anal Calcd for C16H11ClO2: C, 70.99; H, 4.10; Found: C, 71.15; H, 4.22.
Synthesis of E-4-[(3′-Nitrophenyl)Methylene]- 3-Isochromanone (24E) and Z-4-[(3′-Nitrophenyl) -Methylene]-3-Isochromanone (24Z)
Obtained from a mixture of 3-isochromanone (0.50 g; 3.38 mmol) and 3-nitrobenzaldehyde (0.51 g, 3.38 mmol) with the above method. The reaction mixture was separated by column chromatography (silica gel, dichloromethane/ methanol = 10:0.05) to afford 65% 24E and 35% 24Z stereoisomers (0.56 g, 58%). 24E: Rf = 0.55 (silica gel, dichloromethane/methanol = 10:0.1), m.p. 183°C, yellow crystalline solid from methanol. IR νmax (cm–1) (KBr) 1720 (st, C = O). Anal Calcd for C16H11N1O4: C, 68.33; H, 3.94; Found: C, 68.22; H, 4.19.
Z-4-[(3′-nitrophenyl)methylene]-3-isochromanone (24Z)
Rf = 0.65 (silica gel, dichloromethane/methanol = 10:0.1), Yellow crystals from methanol, m.p.178–179°C, IR νmax (cm–1) (KBr) 1724 (st, C = O), Anal Calcd for C16H11NO4: C, 68.33; H, 3.94; Found: C, 68.47; H, 4.17.
Synthesis of E-4-[(3′,4′,5′-Trimethoxyphenyl)Methylene]-3-Isochromanone (25E)
Prepared from a mixture of 3-isochromanone (0.50 g; 3.38 mmol) and 3,4,5-trimethoxybenzaldehyde (95%) (0.70 g, 3.38 mmol) with the above method. The reaction mixture was purified via column chromatography (silica gel, toluene/ethyl acetate = 95.5:4.5) to give 25E (0.75 g, 68%), Rf = 0.34 (silica gel, toluene/ethyl acetate = 8:2), m.p.165–167°C, yellow crystalline solid from methanol. IR νmax (cm–1) (KBr) 1723 (st, C = O). Anal Calcd for C19H18O5: C, 69.93; H, 5.56; Found: C, 70.11; H, 5.64.
Synthesis of E-4-[(3′,4′-Dimethoxyphenyl)Methylene]-3-Isochromanone (26E)
Prepared from a mixture of 3-isochromanone (0.50 g; 3.38 mmol) and veratraldehyde (95%) (0.60 g, 3.43 mmol) with the above method. The reaction mixture was purified via column chromatography (silica gel, toluene/ethyl acetate = 95.5:4.5) to give 26E as a faint yellow crystalline solid from methanol (0.71g, 64%), Rf = 0.36 (silica gel, toluene/ethyl acetate = 8:2), m.p.129–131°C. IR νmax (cm–1) (KBr) 1717 (st, C = O). Anal Calcd for C18H16O4: C, 72.96; H, 5.44; Found: C, 73.09; H, 5.58.
Synthesis of E-4-[(3′,4′-Methylenedioxyphenyl)Methylene]-3-Isochromanone (27E)
Obtained from a mixture of 3-isochromanone (0.50 g; 3.38 mmol) and piperonal (0.51 g, 3.37 mmol) with the method above. The reaction mixture was separated by column chromatography (silica gel, toluene/ethyl acetate = 95.5:4.5) to afford 40% 27E and 60 % 27Z stereoisomers (0.63 g, 66%). 27E Rf = 0.42 (silica gel, toluene/ethyl acetate = 10:1), m.p.153–155°C, yellow crystalline solid from methanol. IR νmax (cm–1) (KBr) 1716 (st, C = O). Anal Calcd for C17H12O4: C, 72.85; H, 4.32; Found: C, 72.95; H, 4.50.
Z-4-[(3′,4′-methylenedioxyphenyl)methylene]-3-isochromanone (27Z)
Rf = 0.43 (silica gel, toluene/ethyl acetate = 10:1), m.p.157–158°C, yellow crystalline solid from methanol. IR νmax (cm–1) (KBr) 1715 (st, C = O). Anal Calcd for C17H12O4: C, 72.85; H, 4.32; Found: C, 72.99; H, 4.48.
Synthesis of E-4-[(3′- Chlorophenyl)Methylene]-3-Isochromanone (28E)
Obtained from a mixture of 3-isochromanone (0.50 g; 3.38 mmol) and 3-chlorobenzaldehyde (95%) (0.50 g, 3.38 mmol) with the above method. The reaction mixture was separated by column chromatography (silica gel, dichloromethane) to afford 80% 28E and 20% 28Z (0.61 g, 67%). 28E Rf = 0.37 (silica gel, dichloromethane), m.p.132–133°C, yellow crystalline solid from methanol. IR νmax (cm–1) (KBr) 1724 (st, C = O). Anal Calcd for C16H11ClO2: C, 70.99; H, 4.10; Found: C, 71.18; H, 4.23.
Z-4-[(3′-chlorophenyl)methylene]-3-isochromanone (28Z)
Rf = 0.49 (silica gel, dichloromethane), m.p 116–117°C, yellow crystalline solid from methanol. IR νmax (cm–1) (KBr) 1721 (st, C = O). Anal Calcd for C16H11ClO2: C, 70.99; H, 4.10; Found: C, 71.22; H, 4.31.
Synthesis of E-4-[(4′- Hydroxyphenyl)Methylene]-3-Isochromanone (29E)
Prepared from a mixture of 3-isochromanone (0.50 g; 3.38 mmol) and 4-hydroxybenzaldehyde (0.41 g, 3.38 mmol) with the above method. The reaction mixture was purified via column chromatography (silica gel, dichloromethane/methanol = 10:0.05) to give 29E as a faint yellow crystalline solid from methanol (0.62 g, 73%). Rf = 0.14 (silica gel, dichloromethane/methanol = 10:0.15), m.p. 212–213°C. IR νmax (cm–1) (KBr) 3444 (st, OH), 1703 (st, C = O). Anal Calcd for C16H12O3: C, 76.18; H, 4.79; Found: C, 76.30; H, 4.91.
Synthesis of E- 4-[(4′-Hydroxy-3′-Methoxyphenyl)Methylene]-3-Isochromanone (30E)
Prepared from a mixture of 3-isochromanone (0.50 g; 3.38 mmol) and vanillin (0.51 g, 3.38 mmol) with the above method. The reaction mixture was purified via column chromatography (silica gel, dichloromethane/methanol = 10:0.05) to give 30E as a faint yellow crystalline solid from methanol (0.60 g, 63%), Rf = 0.24 (silica gel, dichloromethane/methanol = 10:0.1), m.p. 140–141°C. IR νmax (cm–1) (KBr) 3346 (st, OH), 1696 (st C = O). Anal Calcd for C17H14O4: C, 72.33; H, 5.00; Found: C, 72.41; H, 5.12.
Biological Assays
Antifungal Assay
Strains used in these experiments: C. albicans wild-type ATCC 26555, C. albicans wild-type SC5314 (ATCC MYA-2876), C. albicans wild-type ATCC 90028, S. cerevisiae wild-type CVX12-3A (MATα ura3-373-251-328 his4-34 leu2-3-112), S. cerevisiae MATα pbr1-1 leu1 ura3-373-251-328, S. pombe wild-type 972 h–, S. pombe pbr1-8 leu1-32 h–, S. pombe pbr1-6 leu1-32 h–.
The antifungal activity of isochromanones was determined with agar dilution method by using Sabouraud-chloramphenicol agar as previously published (Zacchino et al., 1997, 1999). Stock solutions of tested compounds (10 mg/mL in DMSO) were diluted to afford serial 2-fold dilutions that were added to each medium yielding in concentrations ranging from 0.10 to 250 μg/ mL. The final concentration of DMSO in the assay was not higher than 2.5%.
As standard amphotericin B was applied (Inj. FUNGIZONE 50mg Bristol-Myers Squibb Hungary Kft.). The antifungal caspofungin was a generous gift from Merck Sharp & Dohme.
Determination of MIC (Minimum Inhibitory Concentration) Value by Macro and Micro Tube Dilution Method (Alfa et al., 1993; Washington et al., 2006; Espinel-Ingroff and Pfaller, 2007)
Standard YEPD (Yeast Extract, Peptone, Dextrose) (Sherman, 1990), YEPD+S (YEPD with 1.2M sorbitol), YES (Yeast Extract, Dextrose and Supplements of Adenine, Histidine, Leucine, Uracil and Lysine) (Alfa et al., 1993) and YES+S (YES with 1.2 M sorbitol) (Ribas et al., 1991; Ishiguro et al., 1997), media were used for determination of MIC values of the tested compounds. A stock solution of 10 mg/ml of each tested compounds was prepared in DMSO and serial dilutions in DMSO were prepared. The final concentration of DMSO was 2% in the YEPD medium. This concentration of DMSO does not influence the viability and sensitivity of the tested yeast strains. The serial dilutions of compounds were dispensed into test tubes with 1 ml of YEPD or YEPD+S to make final concentrations of 200, 100, 50, 25, 12.5, 6.26, 3.125, 1.6, 0.8 and 0.4 μg/ml. The tested yeast strains were previously grown in YEPD, YEPD+S, YES or YES+S agar plates at 30°C for 48 h.
For inoculation of YEPD or YEPD+S macro test tubes a suspension of tested fungus was produced with 105 cfu/ml (cfu - colony forming unit). 10 μl of suspension was used for inoculation of each tube. After 48 h incubation at 30°C the tubes were checked. The MIC value is the lowest concentration of the tested compound that could inhibit the fungal growth and the medium remained clear. From each tube 10 μl culture was plated on YEPD agar medium to check the contamination. The MIC tests were carried out in three parallels.
Micro-culture assays of large numbers of samples were analyzed as described) (Martins et al., 2011). Essentially early log-phase cells were grown in YEPD, YEPD+S, YES or YES+S (1.2 M sorbitol) medium at 30°C, and diluted to cell density of 4 X 106 cells/ml in YEPD, YEPD+S, YES or YES+S medium and aliquots of 100 μl were dispensed into each small test tube (3 ml test tube size). Then, another 100 μl of YEPD, YEPD+S, YES or YES+S with the corresponding dilution of each compound at 2X concentration (4% DMSO) was added. The final volume was 200 μl, with the cells diluted to of 2 X 106 cells/ml and each compound diluted to 1X final concentration, containing increasing concentrations of antifungal (0, 1, 2, 5, 10, 20, 50, 100 and 200 μg/ml) or an equivalent volume of solvent (2% DMSO). The cells were grown at 30°C in an orbital roller and turbidity was analyzed after 24 and 48 h of incubation. The MIC was determined as the minimal concentration of tested compound that produced complete cell growth inhibition and kept the medium clear. The MIC values were calculated from at least three independent experiments.
According to the previous experiments of the authors the GS-specific antifungals showed the following in vivo MIC values – in brackets – for the different S. pombe strains given in μg/ml: S. pombe wild-type: papulacandin (5), enfumafungin (10), pneumocandin (5), caspofungin (10); S. pombe pbr1-8 mutant: papulacandin (>100), enfumafungin (>100), pneumocandin (>100), caspofungin (50); S. pombe pbr1-6 mutant: papulacandin (>100), enfumafungin (>100), pneumocandin (50), caspofungin (30) (Martins et al., 2011). In this study we have used caspofungin as a standard control of the in vivo MIC values in S. pombe, S. cerevisiae and C. albicans cells.
Enzyme Preparation and β(1,3)-Glucan Synthase Assay
β(1,3)-D-glucan synthase assay was executed as published previously) (Ishiguro et al., 1997; Martins et al., 2011). Cell extracts were obtained from early log-phase cells grown in YES medium at 28°C. Membrane enzyme extracts were resuspended in buffer A (50 mM Tris-HCl, pH 7.5, 1 mM EDTA, and 1 mM β-mercaptoethanol) containing 33% glycerol and 50 μM GTPγS and stored at −80°C. The standard assay mixture contained 5 μl of enzyme (15–25 μg protein), 150 μM GTPγS, and 2 μl of increasing concentrations of tested compound (0, 0.01, 0.02, 0.05, 0.1, 0.2, 0.5, 1, 2, 5, 10 and 20 μg in 2 μl of DMSO, from dilutions of a stock solution of 10 mg/ml in DMSO and kept at −20°C) or an equivalent volume of 2 μl of solvent DMSO, in a total volume of 40 μl. The reaction was incubated for 30 min at 30°C and stopped by addition of 1 ml 10% trichloroacetic acid. The IC50 was determined as the concentration of tested compound that produced half-maximal inhibitory concentration of the in vitro GS activity and the IC30 was the concentration of tested compound that produced 30% inhibition of the maximal activity. All reactions were carried out in duplicate, and the values were calculated from three independent cell cultures.
According to the previous experiments of the authors the control antifungal caspofungin used in this study showed the following in vitro IC50 values – in brackets - of the enzymatic GS activity for the different S. pombe strains given in μg/ml: S. pombe wild-type: caspofungin (0.3); S. pombe pbr1-8 mutant: caspofungin (250); S. pombe pbr1-6 mutant: caspofungin (150) (Martins et al., 2011). In this study we have used caspofungin as a standard control of the IC50 and IC30 values for in vitro assays of enzymatic GS activity.
Results and Discussion
Synthesis and Structure Elucidation
The selection of the aromatic aldehydes was based on the existing structure-antimicrobial activity relationships. In addition, we wanted to study the effect of the aromatic substituent on the stereo-composition of the reaction mixture. The synthetic route was a solvent free one step method starting from 3-isochromanone and the corresponding aromatic aldehyde using catalytic amount of piperidine (Lóránd et al., 2002). (Under more basic conditions the 3-isochromanone ring undergoes hydrolysis) (Barbier, 1987). To avoid the air oxidation the condensations were carried out under argon atmosphere at 140°C. With this procedure the degradation of the starting 3-isochromanone can be avoided and good yields (50–80%) can be achieved. By this route we have prepared the second generation of isochromanones (17–30, see in Table 2 and Figure 1) with different substitution patterns- with both electron-withdrawing and electron-donating groups in the aromatic nucleus.
The degree of conversion was in each case 100%. These condensations generally yield from the two possible stereoisomers solely the E-stereoisomer. However, in the case of sterically hindered educts the Z-stereoisomer forms (Dimmock and Wong, 1976; Rossi et al., 2010). In four cases a mixture of E/Z-isomers was obtained (17, 24, 27 and 28). Regarding the influence of the aromatic substituents, compounds 17, 24 and 28 contain electron-withdrawing substituents in the aromatic ring.
The structure of the title compounds was supported by the FT IR spectra, too. The strongest band of the FT IR spectra belongs to the CO stretching vibrations lying mostly in the region of 1715–1726 cm–1 with the exception of compounds 21, 29–30. Similar absorption maxima of the CO stretching were observed at analogous compounds – 11E –Z (Keresztury et al., 2004). The decreased νCO frequency of the two hydroxyl compounds (29–30) can be explained with the intermolecular hydrogen bond decreasing the absorption maxima (Silverstein et al., 2015).
The main problem of the structure verification is the distinction between the E-Z-stereoisomers. The 1H- and 13C-APT NMR measurements of the substances achieved spectra in good accordance with the expected structures. As the stereochemistry of the double bond required further corroboration proven to be necessary earlier (Lóránd et al., 2002), with the help of the 2D HSQMBC technique (Williamson et al., 2000), the αH-C3 heteronuclear coupling constants 3J (see Table 3 and Figure 3) were determined. The E- and Z-possibilities of the same molecular framework had significantly and characteristically different proton-carbon coupling constants (see Table 3) allowing the confirmation of the expected geometries.
Biological Part
Antifungal Assays and Structure-Activity Relationships
To study the structure-antifungal activity in the two molecular library of isochromanones (1E-16E and 17E-30E) different type of structures were studied. The effect of the structural changes on the antifungal effect was investigated. Thus the aromatic groups were either homo- or heteroaromatic, the substituents of the aromatic ring were selected as both electron withdrawing and electron donating ones. As for the influence of the stereochemical factor, the tested compounds are of E- or Z-configuration around the exocyclic double bond.
A series of yeasts was chosen for the antifungal screening as wild-type and mutants resistant to the specific fungal cell wall GS inhibitors papulacandins and echinocandins (Cortés et al., 2019): fission yeast S. pombe wild-type (972 h–) and pbr1-8 and pbr1-6 resistant mutants, budding yeast S. cerevisiae wild-type and pbr1-1 resistant mutant, and the pathogenic dimorphic fungus C. albicans wild-type (ATCC 26555, SC5314 and ATCC 90028) (Castro et al., 1995; Martins et al., 2011). Four types of complete media were used for the fungal culture in the screening: standard YEPD, YES, YEPD+S (1.2 M sorbitol) and YES+S media were applied for the vegetative growth of yeast cells. Sorbitol is an osmotic stabilizer, and YEPD+S or YES+S media were used for the analysis of osmotic protection, either in cell wall deficient mutants or against the lethal antifungal effects (Ribas et al., 1991; Castro et al., 1995; Frost et al., 1995; Cortés et al., 2012; Muñoz et al., 2013).
The screening of the first generation of isochromanones was carried out in YEPD and YEPD+S media (1E-16E). The fungal strains analyzed are: C. albicans wild-type ATCC 26555, SC5314 and ATCC 90028, S. cerevisiae wild-type CVX12-3A and pbr1-1 mutant, and S. pombe wild-type (972h–), pbr1-8 mutant and pbr1-6 mutant. The results are summarized in Table 4. The bold numbers in Tables 4–6 indicate the more efficient compounds. In general, under these conditions the tested compounds (1E-16E) showed a high activity. The weak or moderate efficacy against the C. albicans wild-type strains was observed by some substances (3E, 4Z, 5Z, 10E, 15, and 16E). The efficiency toward the S. pombe pbr1-6 mutant strain was the highest inhibitory activity, except 7E, 11E, 12 and 14E. The highest activity was observed at the 1E- phenyl derivative, 3E –pyridyl derivative, 4Z- methylpyrrolyl compound, 5Z- pyrrolyl derivative, 8Z-2-chloro derivative. As regards the other S. pombe pbr1-8 mutant strain, the antifungal activity of the tested compounds was slightly lower, but the substances with the best activity are partly identical to the best compounds mentioned with pbr1-6, (i.e., 1E, 3E, 4Z, 5Z).
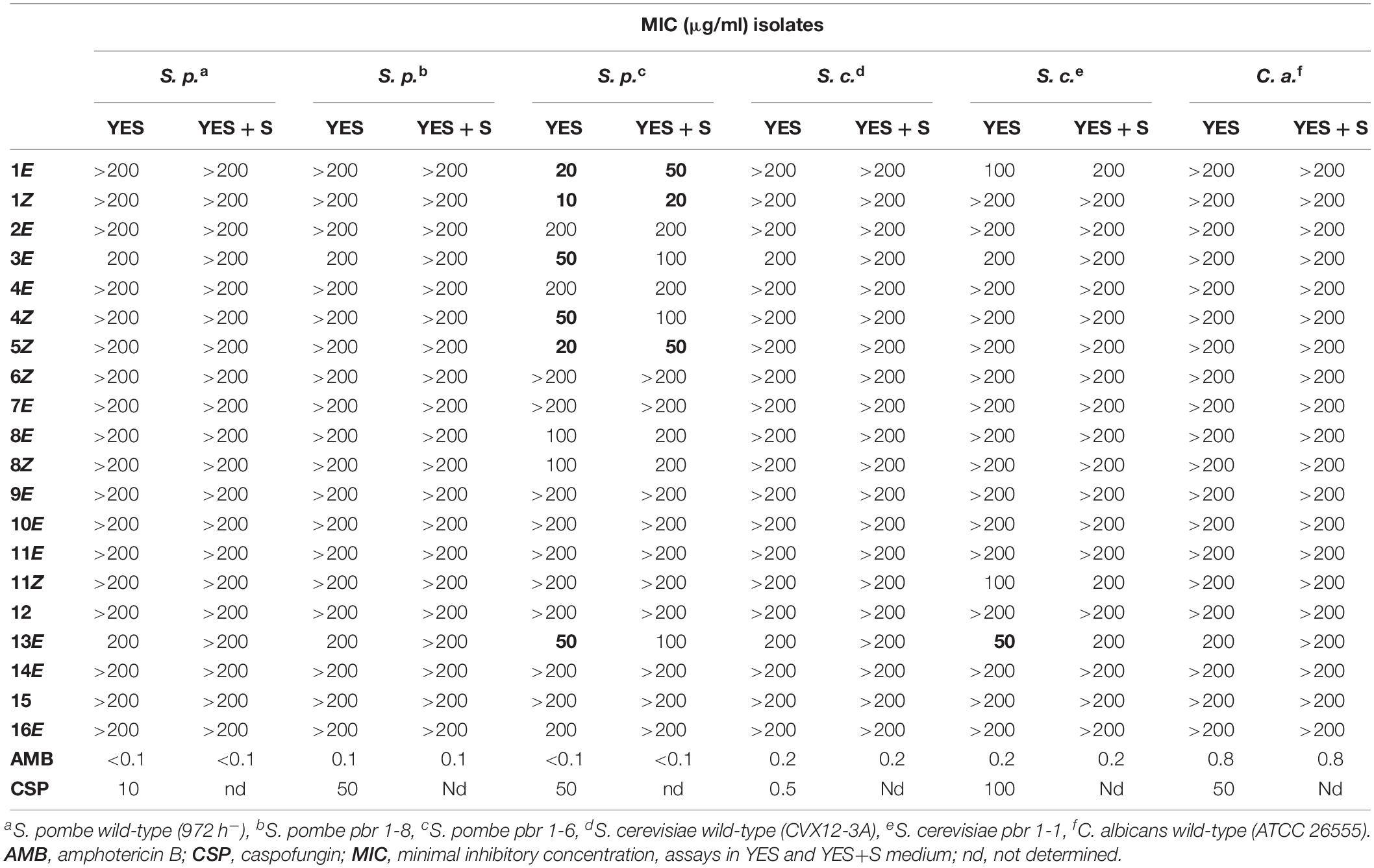
Table 5. MIC values of the first generation of isochromanones (1E–16E) against different yeast strains.
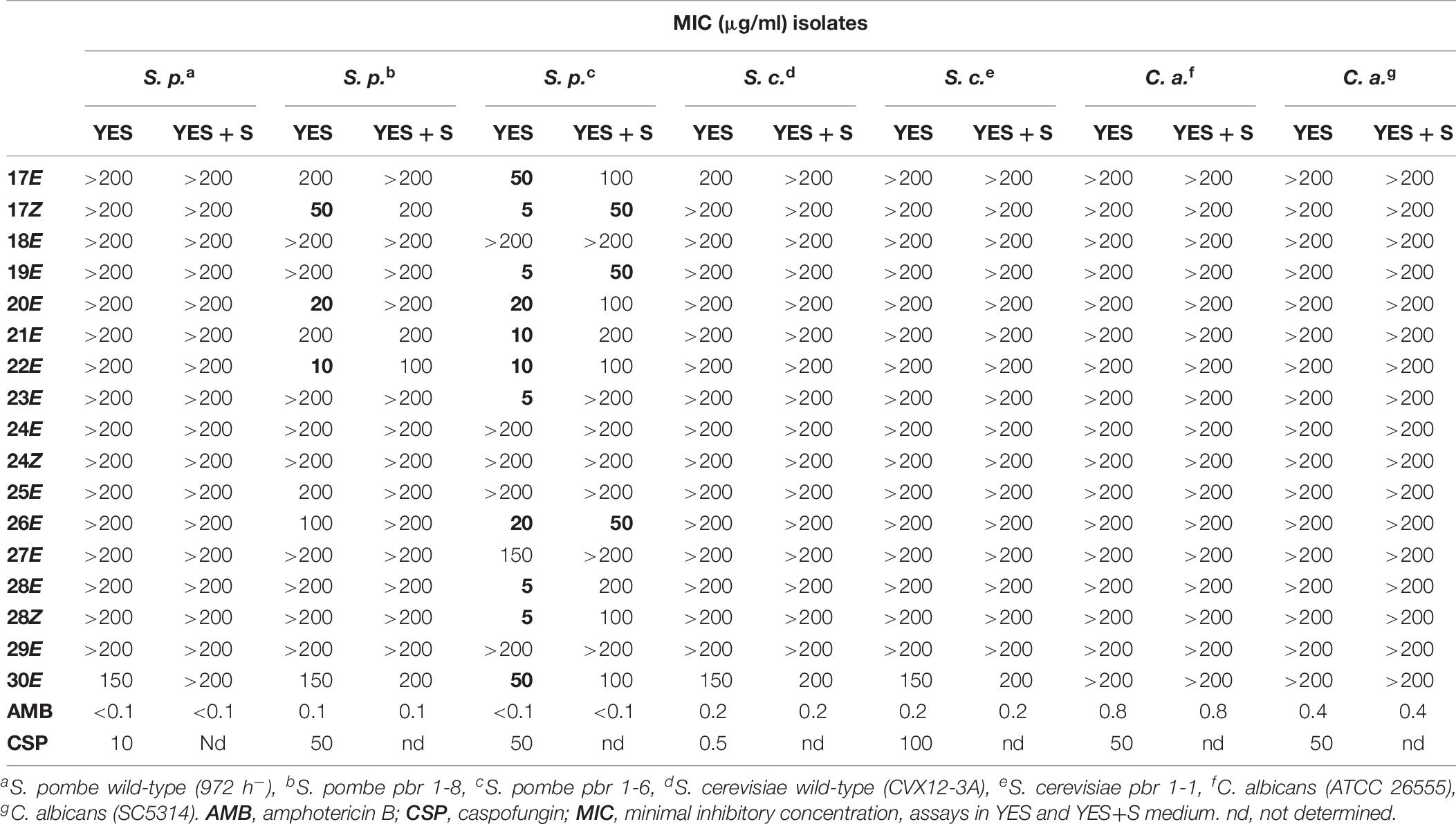
Table 6. MIC values of the second generation of isochromanones (17E-30E) against different yeast strains.
This family of isochromanones showed weak or moderate antifungal activity toward the C. albicans wild-type strains (3E, 4Z, 5Z, 9E and 16E), and 4Z- methylpyrrolyl compound proved to be the most active one
Next, the first generation of isochromanones (1E-16E) was also screened in YES medium (Table 5) and exerted a weaker antifungal potency than in YEPD medium and also than the second family of isochromanones in YES medium (17E-30E) (Table 6). The strains are partly different from the previously mentioned ones (Tables 5, 6) analyzing two wild type C. albicans strains because they behaved similarly in YEPD. It is remarkable that there was no effect against either the C. albicans, the S. cerevisiae, or the S. pombe wild-type strains, except with 30E. Interestingly, the S. cerevisiae and more specially the S. pombe pbr1-6 and pbr1-8 mutants resistant to specific fungal cell wall inhibitors showed different degrees of sensitivity. The results of these antifungal assays in YES medium are displayed in Tables 5, 6.
The S. pombe pbr1-6 mutant strain was the more vulnerable one, as several isochromanones of the first generation exerted a medium or good antifungal effect (e.g., 1E-1Z- phenyl derivatives, 3E –pyridyl derivative, 4Z –methylpyrrolyl, 5Z - pyrrolyl compound and 13E furyl derivative). The S. cerevisiae strains were less sensitive toward the isochromanones, from the first generation of the isochromanones almost only one substance, the 13E furyl derivative - the most efficient antifungal from this series- proved to be efficient and 1E and 11E to be slightly efficient against the S. cerevisiae pbr1-1 mutant strains.
We wanted to see the bioactivity of the second family of isochromanones to see if they had a better antifungal activity in YES with respect to WT and pbr mutants. This family of compounds (17E-30E), generally showed higher antifungal activity than the first generation (Tables 5, 6). With the exception of 30E- a vanillin derivative – all of the compounds were inefficient against the wild-type strains of S. pombe, S. cerevisiae and C. albicans. Several substances proved to be very active specially against the S. pombe pbr1-6 mutant strain, some compounds with electron withdrawing groups as 17Z -4-nitrophenyl derivative, 23E -4-chlorophenyl derivative, 28E-28Z -3-chlorophenyl derivatives, but some substances with electron donating groups also exerted an excellent antifungal potency as 19E -4-methylphenyl derivative, 21E 4-methoxyphenyl derivative. Some compounds showed a medium antifungal potency (as 20E, 26E). As regards the antifungal activity of the E-Z stereoisomers, there was a difference in the potency of 17E and 17Z, the latter was ten times more efficient.
Most of the previously mentioned compounds showed antifungal activity also against the S. pombe pbr1-8 mutant strain, although in some cases it was weaker being the most efficient: 17Z -4-nitrophenyl derivative, 20E -2-methylphenyl derivative, 22E- 3-methoxyphenyl derivative. Out of the second generation of isochromanones the 30E – vanillin derivative - had the broadest spectrum showing antifungal activity against all of the strains except the C. albicans wild-type strains.
The type of medium i. e. composition, incubation times and temperature can influence the growth and detectability of fungi from the clinical samples and the MIC values of the tested strains (Als et al., 2010; Cruz et al., 2013; Balouiri et al., 2016). The Sabouraud and YEPD media are effective and most useful as media for subculture (Washington et al., 2006). Modified RPMI medium is proposed as a standard medium for determination of MIC values of fungi (Washington et al., 2006; Espinel-Ingroff and Pfaller, 2007; Clinical and Laboratory Standard Institute, 2008). In our experiments we used the standard YES and YEPD media and they were optimal for our experimental strains and assays.
Summarizing the results of the antifungal screenings, the most efficient compounds with activity and the lowest MIC values against several strains are the following compounds ordered by their antifungal activity: 1E- phenyl derivative, 3E –pyridyl derivative, 4Z- methylpyrrolyl compound, 5Z- pyrrolyl derivative, 8Z-2-chloro derivative, 9E – 2,3-dihydroxy derivative and the 15 coumarine from the first family, from the second family the 17Z -4-nitrophenyl, 19E -4-methylphenyl, 23E -4-chlorophenyl and 28E-28Z -3-chlorophenyl derivatives.
Mode of Action
In order to explore the mechanism of action of the tested compounds, the osmotic protection against the antifungal effect was analyzed. The inhibitory effect of the compounds in YEPD and YES media were compared with their inhibitory effect in YEPD or YES medium containing 1.2 M sorbitol (YEPD+S or YES+S). These media have been used to detect osmotically fragile mutants that can be rescued in the presence of sorbitol. These mutants present defects in their cell wall synthesis and composition (Ribas et al., 1991; Cortés et al., 2012; Muñoz et al., 2013). Similarly, the osmotic protection can partially protect the cells against the specific cell wall synthesis antifungals (Frost et al., 1995). Therefore, we tested in YEPD+S and YES+S the inhibitory effect of the compounds that showed some in vivo inhibition in YES medium (Tables 4–6). In all of the cases sorbitol protected the cells increasing the in vivo MIC of the tested compound (Tables 4–6), suggesting that the mode of action of the compounds could be inhibiting the synthesis of essential cell wall polymers, as it has been described for the specific GS inhibitors papulacandins and echinocandins (Martins et al., 2011; Brown et al., 2012; Kathiravan et al., 2012; Cortés et al., 2019). To explore this possibility of the mode of action of the tested drugs, their putative inhibitory capacity of the enzymatic GS activity was also examined in vitro (Tables 7, 8). According to these tests, the first family of isochromanones acted as poor inhibitor of this enzyme, compounds 1E – 1Z showed weak inhibitory effect against the in vitro GS of S. pombe pbr1-6 mutant strain (Table 7). Other compounds that had no in vivo effect showed some weak in vitro inhibitory effect detected as IC30 (30% of the maximal inhibitory concentration) but not as IC50 (half-maximal inhibitory concentration), indicating that some in vitro inhibition was present but it never or hardly reached the 50% of inhibition. Interestingly, the in vitro inhibition was higher in the GS enzyme of pbr1-6 mutant, as observed in vivo in living cells. All these data are in accordance with their weak antifungal effect.
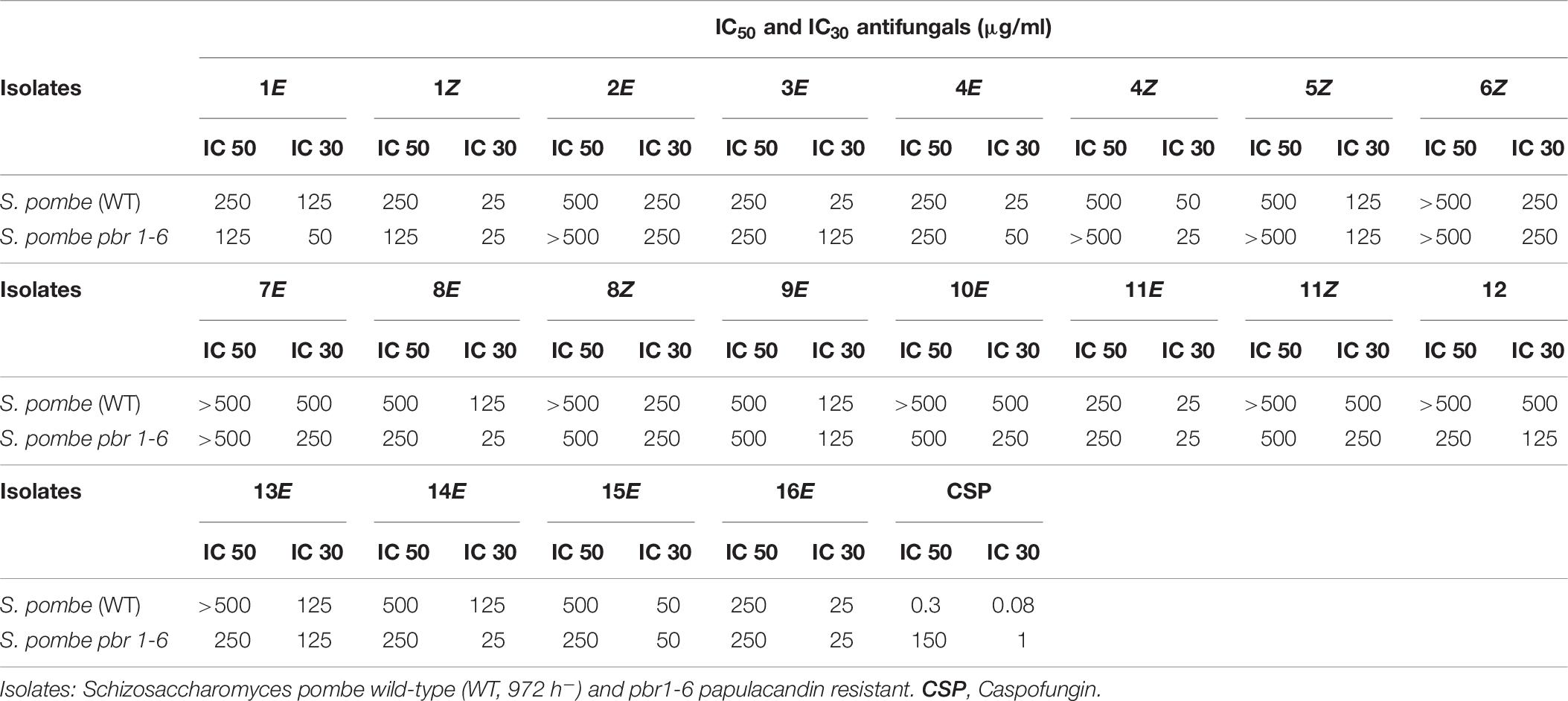
Table 7. Inhibitory capacity of the first generation of isochromanones of the β(1-3)glucan synthase (in vitro assay) expressed as IC50 and IC30 values.
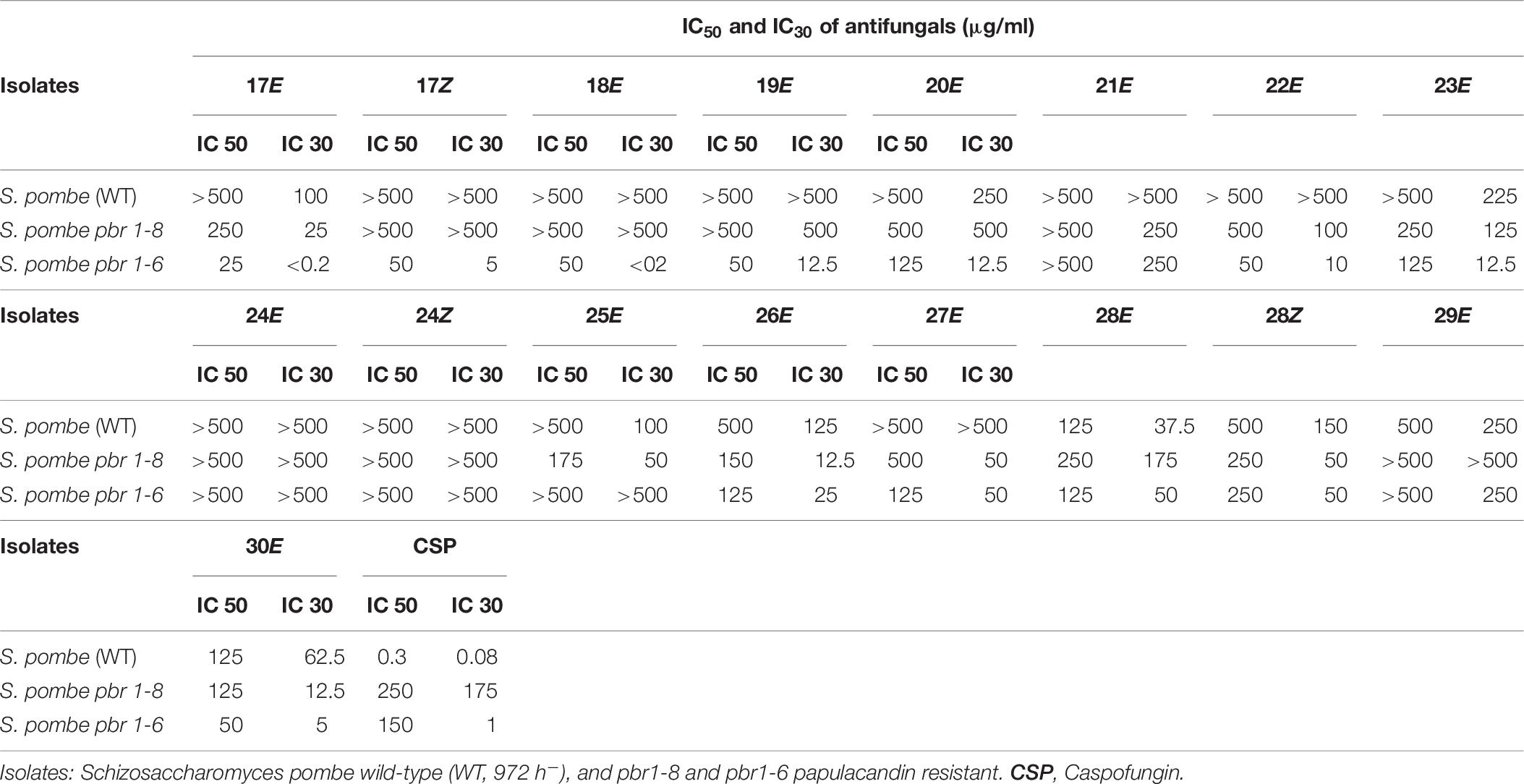
Table 8. Inhibitory capacity of the second generation of isochromanones of the β(1-3)glucan synthase (in vitro assay) expressed as IC50 and IC30 values.
Regarding the second generation of isochromanones, they produced a much higher inhibitory effect against the GS of S. pombe pbr1-6 mutant strain (as 17E–17Z – 4-nitrophenyl, 18E – 2,4,6-trimethylphenyl, 19E – 4-methylphenyl, 22E – 3-methoxyphenyl and the 30E - vanillin derivative, Table 8). The inhibition of pbr1-6 GS was even stronger considering the IC30 value compared to that of wild-type GS. Concerning the influence of the aromatic moieties there are both electron withdrawing and electron donating ones among these substituents. The inhibition of these compounds on the other S. pombe pbr1-8 mutant strain was slight (17E, 23E, 25E, 26E, 28E, 28Z, and 30E). As before, the effect was more pronounced considering the IC30. Finally, the wild-type GS also showed a noticeable sensitivity to drug 30E. These promising results open new possibilities of antifungal strategies that will require, however, to be explored in deep with echinocandin resistant mutants of pathogenic fungi in future studies.
Implications
A synthetic route yielding differently substituted E- and Z-arylidene-3-isochromanones was applied. These condensations generally afforded the E-isomer, but the aromatic substituent has an impact on the stereocomposition of the reaction mixture. The NMR measurements (the αH-C3 heteronuclear coupling constants) afforded unequivocal proof for the steric assignments.
The antifungal effect of the two generation of isochromanones against wild-type and mutant yeast strains showed big difference in the antifungal efficacy. The first generation of isochromanones were less efficient toward the wild-type and mutant strains. Several compounds of the second generation of isochromanones as 17E-Z, 19E, 21E, 23E, 26E, 28E-Z of different substitution pattern showed good or medium activity mainly against the S. pombe pbr1-6 and some against the pbr1-8 mutant strain. A similar effect with some compounds 1E, 11Z, 13E and 30E was also observed with S. cerevisiae wild-type and pbr1-1 mutant. The C. albicans wild-type strains showed sensitivity toward the isochromanones (3E, 4Z, 5Z 9E and 16E) in YEPD medium but were not sensitive in YES medium. The stereochemical factors – E-Z isomerism or substitution pattern of the aromatic ring had an impact on the bioactivity. The first generation of isochromanones was screened using different culture media and the MIC values obtained were different. These experiments demonstrated the effect of the medium on antifungal activity. At last the mode of action was also investigated. According to the results our isochromanones proved to be weak inhibitor of the wild-type GS enzyme but potent inhibitors of the echinocandin resistant GS enzymes.
Susceptibility to antimicrobial drugs depends on the isolated strains and the technique used for determination of susceptibility. The first and very sensitive group of our tested yeast strains was isolated in Kuwait more than 20 years ago (Lóránd et al., 1998). There are different endemic strains in these countries, and there are different inhibition protocols that could show different sensitivities. The type of medium (YES, YEPD, or Casamino medium in the first test), time and temperature of strains incubation, test methods (micro and macro tube dilution, micro plate dilution or E – test) used for determination of the antifungal sensitivity can influence the MIC values. We have to take into consideration these facts when trying to compare MIC values of strains isolated in Kuwait more than 20 years ago with standard laboratory strains either wild-type or isolated mutants resistant to GS antifungals.
Independently of the sensitivity test used, we demonstrated that the isochromanones inhibit the synthesis of cell wall β(1,3)-glucan and that this inhibition is specially enhanced in mutants resistant to the GS inhibitors papulacandins and echinocandins, indicating that isochromanones present a different mechanism of action than the described cell wall β(1,3)-glucan inhibitors papulacandins, acidic terpenoids and echinocandins (Martins et al., 2011; Cortés et al., 2019). This higher sensitivity of the mutants resistant to echinocandins may provide new insights into new strategies of combined antifungal therapy using echinocandins and isochromanones, directed against the spontaneous emergence of mutants resistant to echinocandins.
Author Contributions
JR, MC, and TL designed the experiments and revised the manuscript. TL performed the synthesis of the test compounds and the FT-IR examinations, RB, MC and BK performed the biological experiments and MC analyzed the biological data. AA and GG-F conducted the structure verifications by NMR methods. JR, TL, RB, GG-F, and AA wrote the manuscript.
Funding
RB acknowledges support through a postdoctoral fellowship from the Brazilian Ministry of Education. This work was supported by the Grants BIO2015-69958-P and PGC2018-098924-B-I00 from the Ministerio de Ciencia, Innovación y Universidades (MICINN, Spain) and CSI068P17 and “Escalera de Excelencia” CLU-2017-03 from the Junta de Castilla y León/FEDER (Spain). This study was also financed by the research Grants GINOP-2.3.2-15-2016-00049 and EFOP-3.6.3-VEKOP-16-2017-00009.
Conflict of Interest Statement
The authors declare that the research was conducted in the absence of any commercial or financial relationships that could be construed as a potential conflict of interest.
Acknowledgments
The authors thank Krisztina Sajti for her efficient technical assistance and Zoltán Berente for help with the NMR measurements. The present scientific contribution is dedicated to the 650th anniversary of the foundation of the University of Pécs, Hungary.
Supplementary Material
The Supplementary Material for this article can be found online at: https://www.frontiersin.org/articles/10.3389/fmicb.2019.01692/full#supplementary-material
References
Alfa, C., Fantes, P., Hyams, J., Mc Leod, M., and Warbrick, E. (eds) (1993). Experiments with Fission Yeast: A Laboratory Course Manual. Cold Spring Harbor, NY: Cold Spring Harbor Laboratory.
Al-Nakib, T. M., Lóránd, T., Földesi, A., and Varghese, R. (2001). The in vitro antimycotic activity and acute toxicity of third generation benzylidenetetralones and heteroarylidenetetralones. Med. Principles Prac. 10, 191–196. doi: 10.1159/000050368
Als, S., Sancak, B., Hascelik, G., and Arikan, S. (2010). Influence of different susceptibility testing methods and media on determination of the relevant fluconazole minimum inhibitory concentrations for heavy trailing Candida isolates with low-high phenotype. Mycoses 53, 475–480. doi: 10.1111/j.1439-0507.2009.01739.x
Bal, A. M. (2010). The echinocandins three useful choices or three too many? Int. J. Antimicrob. Agents 5, 13–18. doi: 10.1016/j.ijantimicag.2009.09.011
Balouiri, M., Sadiki, M., and Ibnsouda, S. K. (2016). Methods for in vitro evaluating antimicrobial activity: a review. J. Pharmaceut. Anal. 6, 71–79. doi: 10.1016/j.jpha.2015.11.005
Barbier, M. (1987). Direct access to 4-Substituted 3-Isochromanones by the pH controlled addition of aldehydes. Heterocycles 26, 421–425. doi: 10.3987/R-1987-02-0421
Brown, G. D., Denning, D. W., Gow, N. A., Levitz, S. M., Netea, M. G., and White, T. C. (2012). Hidden killers: human fungal infections. Sci. Transl. Med. 4:165rv13. doi: 10.1126/scitranslmed.3004404
Castro, C., Ribas, J. C., Valdivieso, M. H., Varona, R., del Rey, F., and Durán, A. (1995). Papulacandin B resistance in budding and fission yeasts: isolation and characterization of a gene involved in (1,3)(-D-Glucan Synthesis in Saccharomyces cerevisiae. J. Bacteriol. 177, 5732–5739. doi: 10.1128/jb.177.20.5732-5739
Clinical and Laboratory Standard Institute (2008). Reference Method for Broth Dilution Antifungal Susceptibility Testing of Yeasts: Approved Standard M27-A3. Wayne PA: Clinical and Laboratory Standard Institute.
Cortés, J. C. G., Curto, M. A., Carvalho, V. S. D., Pérez, P., and Ribas, J. C. (2019). The fungal cell wall as a target for the development of new antifungal therapies. Biotechnol. Adv. doi: 10.1016/j.biotechadv.2019.02.008 [Epub ahead of print].
Cortés, J. C. G., Ramos, M., Osumi, M., Pérez, P., and Ribas, J. C. (2016). The cell biology of fission yeast septation. Microbiol. Mol. Biol. Rev. 80, 779–791. doi: 10.1128/MMBR.00013-16
Cortés, J. C. G., Sato, M., Muñoz, J., Moreno, M. B., Clemente-Ramos, J. A., Ramos, M., et al. (2012). Fission yeast Ags1 confers the essential septum strength needed for safe gradual cell abscission. J. Cell Biol. 198, 637–656. doi: 10.1083/jcb.201202015
Cruz, R. C., Werneck, S. M. C., Oliveira, C. S., Santos, P. C., Soares, B. M., Santos, D. A., et al. (2013). Influence of different media, incubation times, and temperatures for determining the MICs of seven antifungal agents against Paracoccidioides brasiliensis by microdilution. J. Clin. Microbiol. 51, 436–443. doi: 10.1128/JCM.02231-12
Dimmock, J. R., and Wong, M. L. C. (1976). Bioactivities and potential uses in drug design of acyclic (,β-unsaturated ketones. Can. J. Pharm. Sci. 11, 35–53. doi: 10.1002/jps.2600730812
Espinel-Ingroff, A. V., and Pfaller, M. A. (2007). “Susceptibility test methods: yeast and filamentous fungi,” in Manual of Clinical Microbiology, 9th Edn, Vol. 2, ed. P. R. Murray (Washington DC: ASM Press), 1972.
Free, S. J. (2013). Fungal cell wall organization and biosynthesis. Adv. Genet. 81, 33–82. doi: 10.1016/B978-0-12-407677-8.00002-6
Frost, D., Brandt, K., Cugier, D., and Goldman, R. (1995). A whole-cell Candida albicans assay for the detection of inhibitors towards fungal cell wall synthesis and assembly. J. Antibiot. 48, 306–310. doi: 10.7164/antibiotics.48.306
Gow, N. A. R., Latge, J. P., and Munro, C. A. (2017). The fungal cell wall: structure, biosynthesis, and function. Microbiol. Spectr. 5:FUNK–0035–2016. doi: 10.1128/microbiolspec.FUNK-0035-2016
Hector, R. F., and Bierer, D. E. (2011). New β-glucan inhibitor as antifungal drugs. Expert Opin. Ther. Pat. 21, 1597–1610. doi: 10.1517/13543776.2011.603899
Hopke, A., Brown, A. J. P., Hall, R. A., and Wheeler, R. T. (2018). Dynamic fungal cell wall architecture in stress adaptation and immune evasion. Trends Microbiol. 26, 284–295. doi: 10.1016/j.tim.2018.01.007
Ishiguro, J., Saitou, A., Duran, A., and Ribas, J. C. (1997). cps11, a Schizosaccharomyces pombe Gene Homolog of Saccharomyces cerevisiae FKS genes whose mutation confers hypersensitivity to cyclosporin A and papulacandin B. J. Bacteriol. 179, 7653–7662. doi: 10.1128/jb.179.24
Kathiravan, M. K., Salake, A. B., Chothe, A. S., Dudhe, P. B., Watode, R. P., Mukta, M. S., et al. (2012). The biology and chemistry of antifungal agents: a review. Bioorg. Med. Chem. 20, 5678–5698. doi: 10.1016/j.bmc.2012.04.045
Keresztury, G., Holly, S., István, K., Sundius, T., and Lóránd, T. (2004). Analysis of Vibrational Spectra of some new E- and Z-4-Arylidene-3-isochromanones – Part 2. Isomers and Conformers of the 2’-pyrrolyl and 2’-nitrophenyl derivative. J. Biochem. Biophys. Methods 61, 107–118. doi: 10.1016/j.jbbm.2004.04.012
Lóránd, T., Al Nakib, T. M., and Forgó, P. (1998). “Synthesis and antifungal study of some new E- and Z-4-arylidene-3-isochromanones,” in Proceedings of the Federal of European Chemical Societies 9th FECHEM Conference on Heterocycles in Bio-organic Chemistry, (Aussois). 70.
Lóránd, T., Forgó, P., Földesi, A., Õsz, E., and Prókai, L. (2002). Improved Solvent-free synthesis and structure elucidation of (E)- and (Z)-4-Arylmethylene-3-isochromanones. Eur. J. Org. Chem. 2002, 2996–3003. doi: 10.1002/1099-0690(200209)2002:17<2996::AID-EJOC2996<3.0.CO;2-G
Martins, I. M., Cortés, J. C. G., Muñoz, J., Moreno, M. B., Ramos, M., Clemente-Ramos, J. A., et al. (2011). Differential activities of three families of specific β(1,3)-glucan synthase inhibitors in wild-type and resistant strains of fission yeast. J. Biol. Chem. 286, 3484–3496. doi: 10.1074/jbc.M110.174300
Muñoz, J., Cortés, J., Sipiczki, M., Ramos, M., Clemente-Ramos, J., Moreno, M., et al. (2013). Extracellular cell wall β(1,3) glucan is required to couple septation to actomyosin ring contraction. J. Cell Biol. 203, 265–282. doi: 10.1083/jcb.201304132
Ribas, J. C., Díaz, M., Durán, A., and Pérez, P. (1991). Isolation and characterization of Schizosaccharomyces pombe mutants defective in cell wall (1,3)-beta-D-glucan. J. Bacteriol. 173, 3456–3462. doi: 10.1128/jb.173.11.3456-3462.1991
Rossi, D., Baraglia, A. C., Serra, M., Azzolina, O., and Collina, S. (2010). An efficient procedure based on a MW-assisted Horner-Wadsworth-Emmons reaction for: the synthesis of (Z)-3,3-trisubstituted-alpha,beta-unsaturated esters. Molecules 15, 5928–5942. doi: 10.3390/molecules15095928
Ruelius, H. W., and Gauhe, A. (1950). Über einen Oxynaphthochinon-schwefelsäure-ester aus Fusarien. Justus Liebigs Ann. Chem. 569, 38–59. doi: 10.1002/jlac.19505700110
Sherman, F. (1990). “Getting started with yeast,” in Guide to Yeast Genetics and Molecular Biology, Vol. 194, eds C. Guthrie and G. R. Fink (San Diego, CA: Academic Press), 3. doi: 10.1016/0076-6879(91)94004-v
Silverstein, R. M., Webster, F. X., Kiemle, D., and Bryce, D. L. (2015). Spectrometric Identification of Organic Compounds, 5th Edn. Hoboken, NJ: Wiley, 76.
Washington, W. Jr., Allen, S., Janda, W., Koneman, E., Procop, G., Schreckenberger, P., et al. (2006). Koneman’s Color Atlas and Textbook of Diagnostic Microbiology, 6th Edn. Philadelphia, PA: Williams Lippincott., and Wilkins Lippincott, 1165.
Williamson, R. T., Márquez, B. L., Gerwick, W. H., and Kövér, K. E. (2000). One- and two-dimensional gradient-selected HSQMBC NMR experiments for the efficient analysis of long-range heteronuclear coupling constants. Magn. Reson. Chem. 38, 265–273. doi: 10.1002/(sici)1097-458x(200004)38:4<265::aid-mrc637>3.0.co;2-#
Zacchino, S. A., Lopez, S. N., Pezzenati, G., Furlan, R. L., Santecchia, C. B., Munoz, L., et al. (1999). In Vitro evaluation of antifungal properties of phenylpropanoids and related compounds acting against dermatophytes. J. Nat. Prod. 63, 1353–1357. doi: 10.1021/np9805443
Keywords: antifungals, inhibitors, glucan synthase, echinocandin, cell wall
Citation: Berzaghi R, Agócs A, Curto MA, Gulyás-Fekete G, Kocsis B, Ribas JC and Lóránd T (2019) Novel Cell Wall Antifungals Reveal a Special Synergistic Activity in pbr1 Mutants Resistant to the Glucan Synthesis Antifungals Papulacandins and Echinocandins. Front. Microbiol. 10:1692. doi: 10.3389/fmicb.2019.01692
Received: 20 November 2018; Accepted: 09 July 2019;
Published: 24 July 2019.
Edited by:
Carlos Pelleschi Taborda, University of São Paulo, BrazilReviewed by:
Johanna Rivera, Albert Einstein College of Medicine, United StatesLuis Antonio Pérez-García, Universidad Autónoma de San Luis Potosí, Mexico
Copyright © 2019 Berzaghi, Agócs, Curto, Gulyás-Fekete, Kocsis, Ribas and Lóránd. This is an open-access article distributed under the terms of the Creative Commons Attribution License (CC BY). The use, distribution or reproduction in other forums is permitted, provided the original author(s) and the copyright owner(s) are credited and that the original publication in this journal is cited, in accordance with accepted academic practice. No use, distribution or reproduction is permitted which does not comply with these terms.
*Correspondence: Tamás Lóránd, dGFtYXMubG9yYW5kQGFvay5wdGUuaHU=