- 1Key Laboratory of Animal Feed and Nutrition of Zhejiang Province, Institute of Feed Science, College of Animal Sciences, Zhejiang University, Hangzhou, China
- 2College of Animal Science and Technology, Qingdao Agricultural University, Qingdao, China
- 3Key Laboratory of High Efficient Processing of Bamboo of Zhejiang Province, China National Bamboo Research Center, Hangzhou, China
- 4Department of Hepatobiliary Surgery II, Guangdong Provincial Research Center of Artificial Organ and Tissue Engineering, Zhujiang Hospital of Southern Medical University, Guangzhou, China
- 5State Key Laboratory of Organ Failure Research, Southern Medical University, Guangzhou, China
Obesity and the related liver diseases are prevalent around the world. Although probiotics have been shown to prevent obesity through multiple ways, only few researches investigated the lipid-lowering effects of probiotic Bacillus. Moreover, the limited results consistently suggested that Bacillus regulated genes related to lipogenesis and oxidation, but no further exploration was made. Our previous study revealed that Bacillus amyloliquefaciens SC06 has a potent antioxidant capacity in vitro. The aim of this study is to investigate the effects of SC06 on obesity and the associated liver injury of high-fat diet (HFD)-fed-mice and its underlying mechanism. By feeding normal chow (NC), NC+SC06, HFD, and HFD+SC06 to mice, we found that SC06 improved body weight gain, hepatic steatosis, and glucose metabolism of HFD-mice. Furthermore, SC06 also increased the antioxidant capacity of mice through Nrf2/Keap1 signaling pathway. High-throughput sequencing of 16S rRNA gene showed that HFD changed the gut microbiota dramatically, while HFD+SC06 decreased the ratio of Firmicutes/Bacteroidetes and increased TM7 abundance. More differences were also found in lower taxa. Altogether, SC06 is a potential probiotic that decreases HFD-related lipid accumulation and liver injury via regulating the antioxidant capacity and host gut microbiota.
Introduction
With more than 1.4 billion overweight or obese adults (Hall et al., 2015), obesity has become a major threat to public health in both developed and developing countries (Haslam and James, 2005). In the United States, more than 36% of adults are obese with a body mass index (BMI) greater than 30 kg/m2 (Ogden et al., 2014). The mean BMI in Chinese adults also increased from 22.7 kg/m2 in 2004 to 23.7 kg/m2 in 2010 with the prevalence of obesity increased from 3.3% in 2004 to 5.2% in 2010 (Jiang Y. et al., 2015). Therefore, it is now beginning to replace under-nutrition and infectious diseases as the most significant contributor to ill health (Dietz et al., 2015).
Obesity and obesity-related metabolic diseases are caused by multiple factors, including diet, gene, environment and mentality (Kopelman, 2000). High fat and sugar intake are the main causes of obesity (Tappy and Le, 2010). Although the mechanisms that underlie high-fat diet (HFD)-induced physiopathologic changes have yet to be elucidated, it is unveiled that HFD regulates the genes encoding enzymes and influences nutrient-sensing signal (Kim et al., 2004; Birse et al., 2010). Associated with obesity, non-alcoholic fatty liver disease (NAFLD) is the major reason for abnormal liver function worldwide (Li et al., 2014). Approximately 5% of NAFLD individuals develops hepatic inflammation and fibrosis (Barreyro et al., 2015). Obesity is also linked to oxidative stress (Furukawa et al., 2004; Charradi et al., 2013). Multiple possible sources of oxidant stress in the fatty liver may constitute the “second hit” for cellular injury in non-alcoholic steatohepatitis (Carmiel-Haggai et al., 2004). Therefore, it is believed that antioxidants may be effective agents in treating active steatohepatitis through attenuating the secondary injury. Foster et al. (2011) found that antioxidant atorvastatin combined with vitamin C and E was effective in reducing the hepatic steatosis in individuals with NAFLD. S-adenosylmethionine, which generates glutathione (GSH), also had the potential to treat NAFLD (Musso et al., 2013).
During the past decades, it became clear that gut microbiota is instrumental in the control of host energy metabolism. Germ-free mice had about 40% less total body fat than the “normal” mice (Backhed et al., 2004). The conventionalization contributed to a 60% increase in body fat of conventionalized germ-free mice with the gut microbiota from the “normal” mice. Besides, the interactions between liver and gut play a critical role in NAFLD onset and progression (Jumpertz et al., 2011). Although the involvement of gut microbiota in NAFLD pathogenesis is complex, several reports demonstrated that intestinal bacteria may cause NAFLD via modulating low-grade inflammation, energy homeostasis, choline metabolism, bile acid homeostasis and endogenous ethanol generation (Kirpich et al., 2015).
Probiotics are non-pathogenic bacteria that exert a beneficial influence on host health (Rajput et al., 2013). As the widely studied probiotics, Lactobacillus and Bifidobacteria can improve lipid metabolism through assimilating cholesterol, binding cholesterol of cellular surface and deconjugating bile (Kumar et al., 2012). Moreover, Lactobacillus consumption can also alter the gut microbiota community structure and enrich the operational taxonomic units that are negatively correlated with metabolic syndrome phenotypes, including NAFLD (Compare et al., 2012; Wang et al., 2015; Park et al., 2017). However, as far as we know, only few studies explored the effects of Bacillus on lipid metabolism, and the limited results of these studies were simply obtained by detecting gene expressions and serum indexes (Do et al., 2015; Lei et al., 2015). Besides, it is worth noting that the lowered lipid accumulation induced by Bacillus was found to be accompanied by the increase of antioxidation (Do et al., 2015; Lei et al., 2015). Our previous study implied that Bacillus amyloliquefaciens SC06 (SC06) markedly elevated the antioxidant capacity of porcine intestinal epithelial cells (Wang et al., 2017). As oxidative stress is an obvious phenomenon in obesity, we hypothesis that SC06 may also prevent obesity and associated liver injury by regulating the antioxidant capacity and gut microbiota of hosts. In this study, we assessed the preventive effects of SC06 on HFD-induced obesity, liver injury and oxidative stress in mice and analyzed the intestinal microbiota structure.
Materials and Methods
Bacteria
Bacillus amyloliquefaciens SC06 (SC06) cells were stored in China Center for Type Culture Collection (No. M 2012280). The culture and preparation of SC06 was referred to previous study (Wang et al., 2017). Briefly, SC06 powder (108 cfu/g) was prepared by Microbiology and Genetic Engineering Laboratory, Institute of Feed Sciences, Zhejiang University, China). SC06 was cultured on Luria-Bertani media, kept at 37°C for 24 h and shaken at 180 r/min. Pure bacterial cells were collected after centrifugation at 5000 g for 10 min at 4°C. Then, these cells were washed twice with sterile 0.85% sodium chloride solution. Ultimately, the culture purity and identification were constantly checked by the spreading plate method (Nikoskelainen et al., 2003).
Animals and Diets
The experimental procedure was illustrated in Supplementary Figure S1. Sixty male C57BL/6J mice (6 weeks old, n = 15 per group) were obtained from Slac Laboratory Animal Co., Ltd. (Shanghai, China) and fed on normal chow diet for 1 week to adapt to the environment. Thereafter, animals were divided into four groups and fed with normal chow (NC group, 3616 Kcal/Kg energy), NC supplemented with 0.1% (w/w) SC06 powder (NC+SC06 group), HFD (HFD group, 80% NC, 0.5% cholesterol, 6.3% lard, 13% dried egg yolk, and 0.2% cholate, 4270 Kcal/Kg energy) and HFD supplemented with 0.1% (w/w) SC06 powder (HFD+SC06 group) for 8 weeks. During the preparation of the SC06 powder, starch was used to dilute SC06 and the same amount of starch was also added to the NC and HFD groups to compensate for the difference in nutrient composition of the diets. Normal chow diet was purchased from Xietong Organism Co., Ltd. (Nanjing, China). The nutritional constitutes of HFD was based on previous study (Xin et al., 2014). NC+SC06, HFD, and HFD+SC06 diets were all prepared by Xietong Organism Co., Ltd. (Nanjing, China). Mice were housed in standard plastic cages (three mice per cage) and maintained under a 12-h light-dark cycle at constant temperature and humidity [(23 ± 1)°C and (55 ± 5)%, respectively]. Mice body weight and food intake were recorded. The mass of white fat, including the perirenal fat, subcutaneous fat and epididymal fat was weighed. The experiment was approved by and performed in accordance with the guidelines of the ethics committee of Zhejiang University.
Insulin Sensitivity
Oral glucose tolerance test (OGTT) and insulin tolerance test (ITT) were performed at the 7th week and 8th week, respectively. Before OGTT test, mice were fasted 8 h and were then given 2 g/Kg glucose orally. Blood glucose levels were determined with an Accu-chek glucose meter (Roche Diagnostics, Almere, Netherlands) at 0, 15, 30, 60, and 120 min. Before the ITT test, mice were fasted 4 h and insulin (0.75 U/kg) was injected intraperitoneally. Blood glucose levels were determined with an Accu-chek glucose meter (Roche Diagnostics, Almere, The Netherlands) at 0, 15, 30, 60, and 120 min.
Western-Blotting Analysis
Liver tissues were resuspended in lysis buffer (Biotime Biotechnology, China), ground and rocked for 30 min on ice. Crude lysates were then centrifuged at 12000 rpm for 10 min. Western-blotting analysis was performed according to previous study (Wang et al., 2017).
Biochemical Evaluation of Serum
The activities of glutamic-oxalacetic transaminase (GOT), glutamic-pyruvic transaminase (GPT), total-antioxidant capacity (T-AOC), superoxidase (SOD), and catalase (CAT) in serum were determined using commercial kits (Nanjing Jiancheng Bioengineering Institute, China) according to the instructions of the manufacturer. Interleukin (IL)-6, IL-1β, tumor necrosis factor (TNF)-α, and leptin concentrations were detected using a commercial ELISA kit (Raybiotech, GA, United States) according to the instructions of the manufacturer.
Biochemical Evaluation of Liver
T-AOC, CAT activities as well as malonaldehyde (MDA), GSH levels in livers were determined using commercial kits (Nanjing Jiancheng Bioengineering Institute, China) according to the instructions of the manufacturer.
Hematoxylin and Eosin, and Oil Red O Staining
Hematoxylin and eosin staining were performed according to previous study (Xin et al., 2014). For oil red O staining, 4-μm sections were cut from frozen optimal-cutting-temperature samples, affixed to microscope slides, and allowed to air-dry overnight at room temperature. The tissue sections were stained in fresh oil red O for 10 min and rinsed in water.
Reactive Oxygen Species (ROS) Detection
Frozen liver sections were incubated with 5 mol/L Dihydroethidium (Sigma-Aldrich, United States) for 30 min (37°C). After washes with PBS, the ROS level in the tissue was measured by confocal microscopy (Leica, Germany).
TUNEL Staining
The cell apoptosis in the liver was determined by TUNEL according to the manufacturer’s instructions In Situ Cell Death Detection Kit (KeyGen BioTECH, China).
Transmission Electron Microscopy (TEM)
Liver tissue was harvested after perfusion with PBS, then fixed and processed as described previously (Stolz et al., 1999). After dehydration, thin sections were stained with uranyl acetate and lead citrate for observation under a 1024 × 1024 pixel CCD camera system (AMT Corp., United States).
Fecal DNA Extraction and Illumina Miseq Sequencing
Genomic DNA was extracted using a TIANamp Stool DNA Kit according to manufacturer’s protocols (Tiangen Biotech, China). The V4 hypervariable region of 16S rRNA gene was amplified using the primer pair 515F (5′-GTGCCAGCMGCCGCGGTAA-3′) and 806R (5′-GGACTACHVGGGTWTCTAAT-3′), and MiSeq sequencing were performed by RiboBio Co., Ltd. (Guangzhou, China). All the DNA datasets have been submitted to the NCBI Sequence Read Archive database under the accession number SRP128678.
Statistical Analysis
The data are presented as the means ± SD with respect to the number of samples (n) in each group. Statistical significance between multiple treatment groups was determined using analysis of variance (ANOVA). Tukey’s method was used to perform multiple comparison of means. P-value less than 0.05 was considered as statistical significance.
Results
SC06 Treatment Attenuated Obesity and Insulin Resistance
Compared with NC, NC+SC06 did not alter body weight significantly. HFD-fed mice gained more weight from week 5 than NC-fed mice (p < 0.05), but HFD+SC06 significantly relieved the HFD-induced body weight increases (p < 0.05, Figure 1A). Besides, the daily energy intake of mice in HFD group was increased compared with NC group (p < 0.05), while HFD+SC06 decreased energy intake significantly (p < 0.05, Figure 1B). Perirenal and subcutaneous partial fat weights were not changed in NC+SC06-fed mice compared to NC-fed mice, but were higher in mice receiving HFD (p < 0.05). Although weights of the perirenal, subcutaneous and epididymal fat were consistently decreased in HFD-fed mice received SC06, only subcutaneous fat weight reduced significantly (p < 0.05, Figure 1C). Furthermore, histological analysis of perirenal, subcutaneous and epididymal adipose tissues showed that the size of adipocytes in the HFD group was greater than that in NC, but the size of adipocytes in the HFD+SC06 group was less than that in HFD group (Figures 1D–F). In addition, HFD significantly increased glucose levels during the OGTT (p < 0.05, Figure 1G), and HFD+SC06 elevated the clearance of glucose slightly. Moreover, HFD worsened the insulin resistance slightly, while HFD+SC06 marginally improved the insulin sensitivity (p = 0.058).
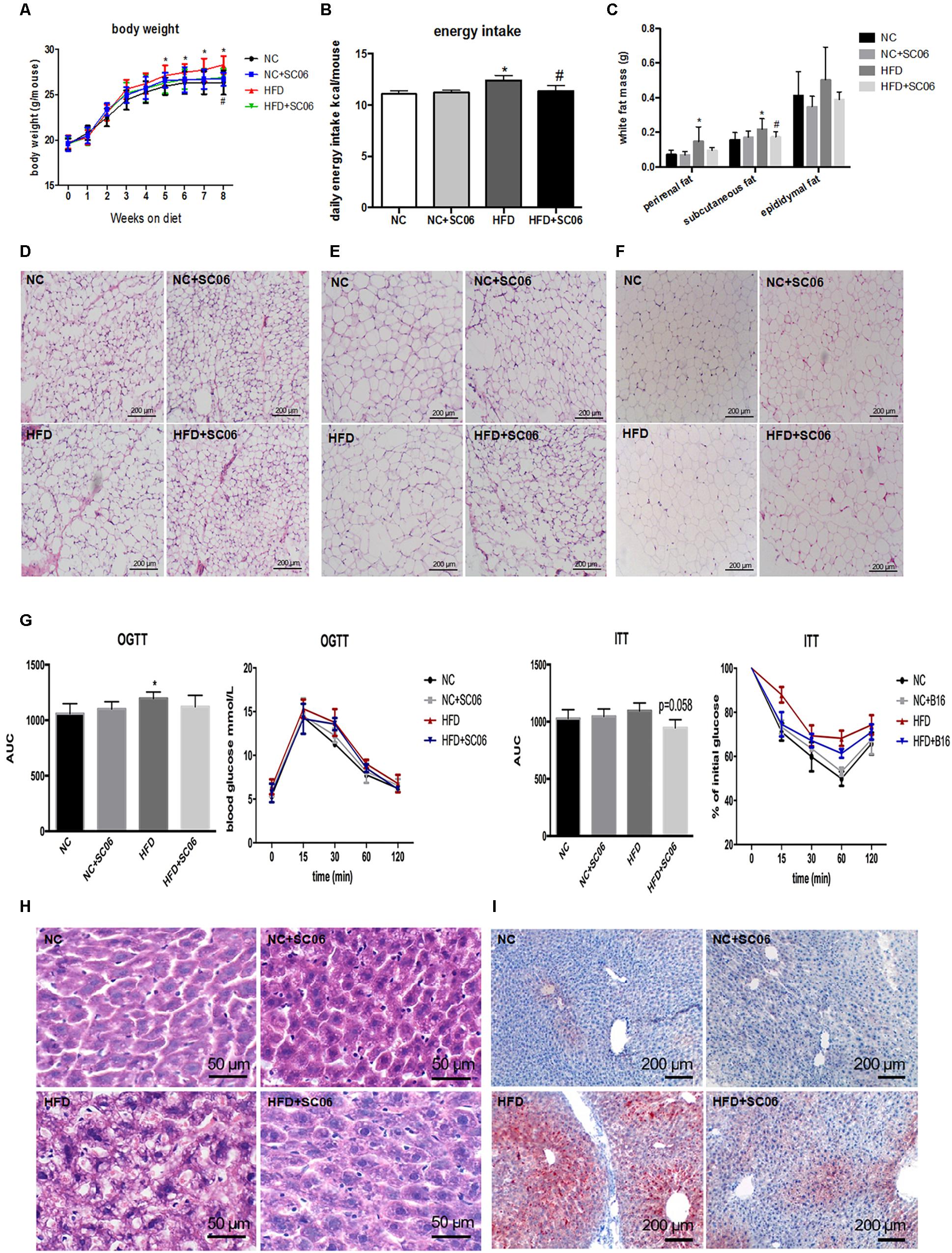
Figure 1. SC06 attenuated HFD-induced obesity, insulin resistance and hepatic steatosis. (A) Body weight, (B) daily energy intake, (C) partial white fat mass, (D) subcutaneous adipocyte morphology, (E) perirenal adipocyte morphology, (F) epididymal adipocyte morphology, (G), OGTT and ITT, AUC means area under the curve. (H) HE liver sections, (I) oil-red O liver section. Results are expressed as means ± SD (n = 15 for body weight, energy intake, and fat mass; n = 4 for tissue morphology). Differences between groups were determined by one-way ANOVA followed by Tukey’s t-test. Significant differences between HFD versus NC are indicated as ∗p < 0.05. Significant differences between HFD versus HFD+SC06 are indicated as #p < 0.05.
SC06 Treatment Alleviated Liver Steatosis and Injury of HFD-Induced Mice
The HE and oil-red O liver sections from HFD mice exhibited visible intracellular vacuolization and marked lipid accumulation. Compared with the HFD group, the degree of hepatic steatosis was appreciably decreased by SC06 treatment (Figures 1H,I). The results of GOT and GPT activities showed that HFD or/and SC06 had no significant effect on GOT activity. However, GPT activity was significantly enhanced in HFD group (p < 0.01), but decreased slightly in HFD+SC06 (Figure 2A). Additionally, TUNEL results indicated that HFD enhanced liver cell apoptosis, which was reduced by SC06 treatment (Figure 2B). The mitochondrial TEM revealed that HFD seriously induced mitochondrial abnormalities, including swelling and disappearance of cristae, which were effectively alleviated by administration SC06 (Figure 2C).
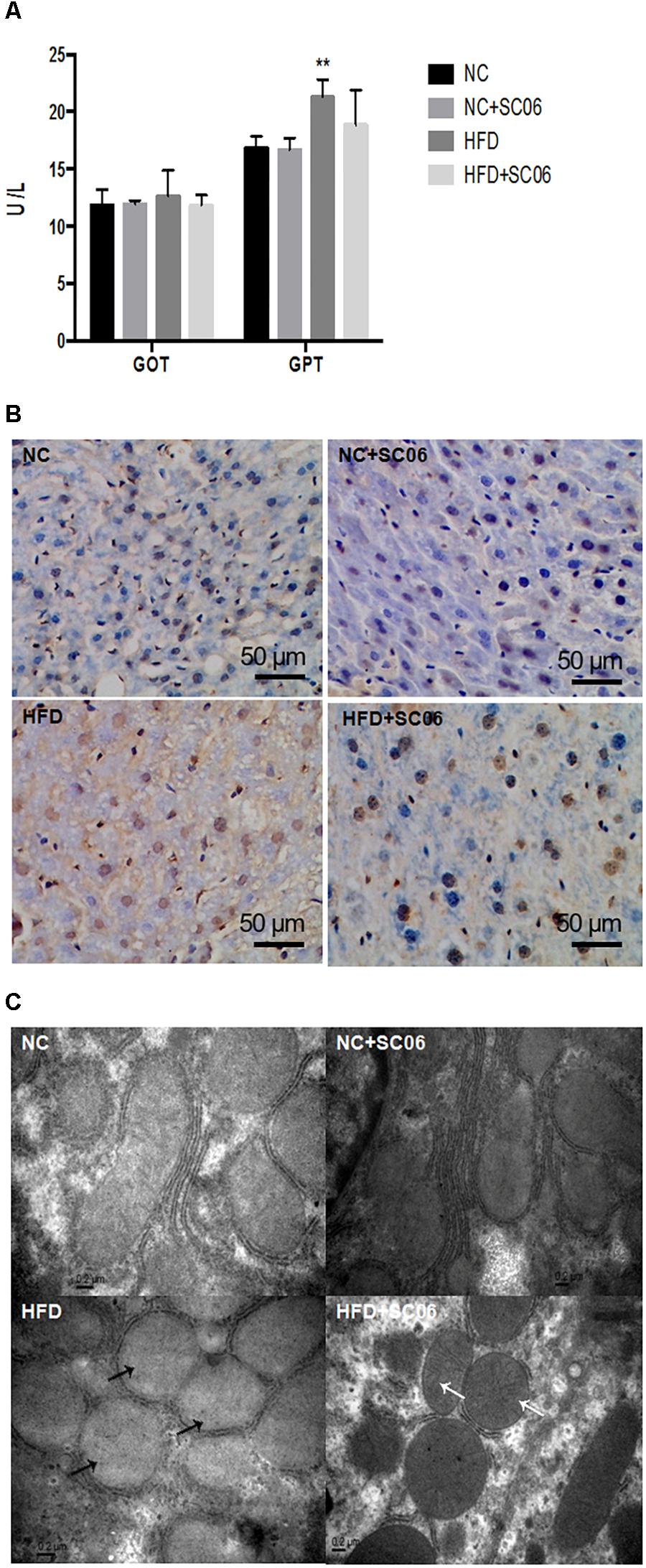
Figure 2. SC06 attenuated liver lesion. (A) GOT and GPT activities, (B) TUNEL sections, (C) electron microscopic sections. The black arrows indicate the abnormal mitochondria, including the disappearance of cristae and swelling. The white arrows indicate the normal mitochondrial. Results are expressed as means ± SD. Differences between groups were determined by one-way ANOVA followed by Tukey’s t-test (n = 6 for GOT and GPT; n = 4 for TUNEL and TEM). Significant differences between HFD versus ND are indicated as ∗∗p < 0.01.
SC06 Treatment Decreased the Secretion of Inflammatory Factors and Adipokine of HFD-Induced Mice
No significant changes were found for serum levels of IL-6, IL-1β, TNF-α and leptin between NC and NC+SC06 groups (Figures 3A–D). As expected, leptin, IL-6 as well as TNF-α levels were significantly elevated in HFD group (p < 0.05), but lowered in HFD+SC06 (Figures 3A–C) (p < 0.05). However, IL-1β level was not altered significantly in HFD and/or HFD+SC06 groups (Figure 3D).
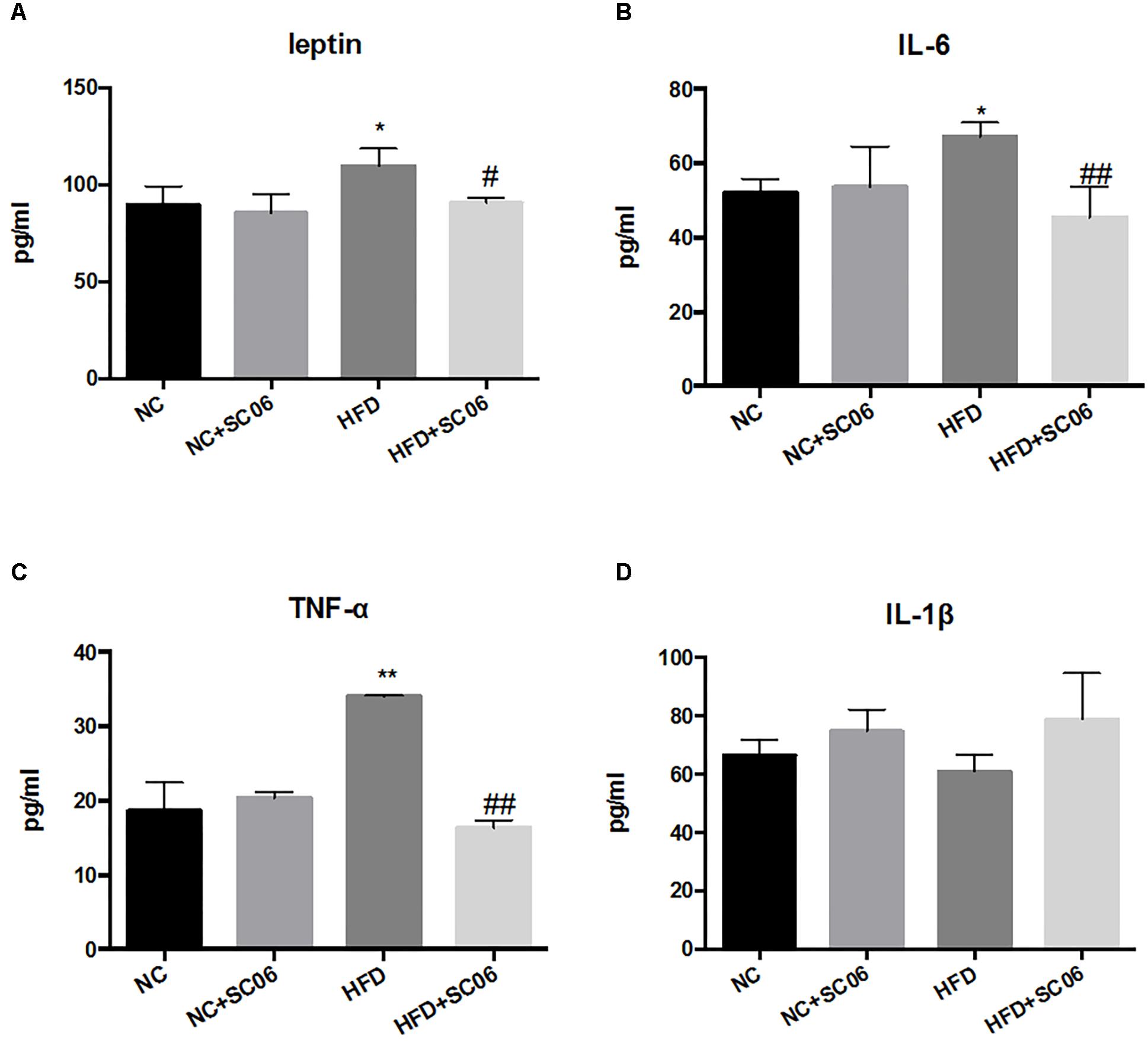
Figure 3. SC06 modulated secretion of inflammatory factors and adipokine. (A) Leptin, (B) IL-6, (C) TNF-α, (D) IL-1β. Results are expressed as means ± SD. Differences between groups were determined by one-way ANOVA followed by Tukey’s t-test (n = 6). Significant differences between HFD versus NC are indicated as ∗p < 0.05, ∗∗p < 0.01. Significant differences between HFD versus HFD+SC06 are indicated as #p < 0.05, ##p < 0.01.
SC06 Relieved Liver Oxidative Stress of HFD-Induced Mice
Table 1 shows that mice fed with NC+SC06 exhibited higher hepatic CAT activity (p < 0.05). Whereas, NC+SC06 had no significant effects on MDA, T-AOC, GSH levels and SOD activity in liver. HFD significantly increased MDA content (p < 0.05), which was lowered significantly by HFD+SC06 (p < 0.05).
Nrf2-Keap1 is a well-characterized pathway that responds to oxidative stress and regulates genes of phase II detoxifying enzymes through binding with antioxidant-responsive element (Turnbaugh et al., 2008; Jones et al., 2015). Figure 4A shows that Nrf2 expression was marginally increased in mice feeding NC+SC06 (p = 0.054). Meanwhile, the phosphorylation of Nrf2 was significantly up-regulated in HFD group compared to NC (p < 0.05). Compared to HFD, mice receiving HFD+SC06 showed down-regulated p-Nrf2 expression (p < 0.05). No significant differences were observed in Keap1 expressions among all groups (Figure 4A).
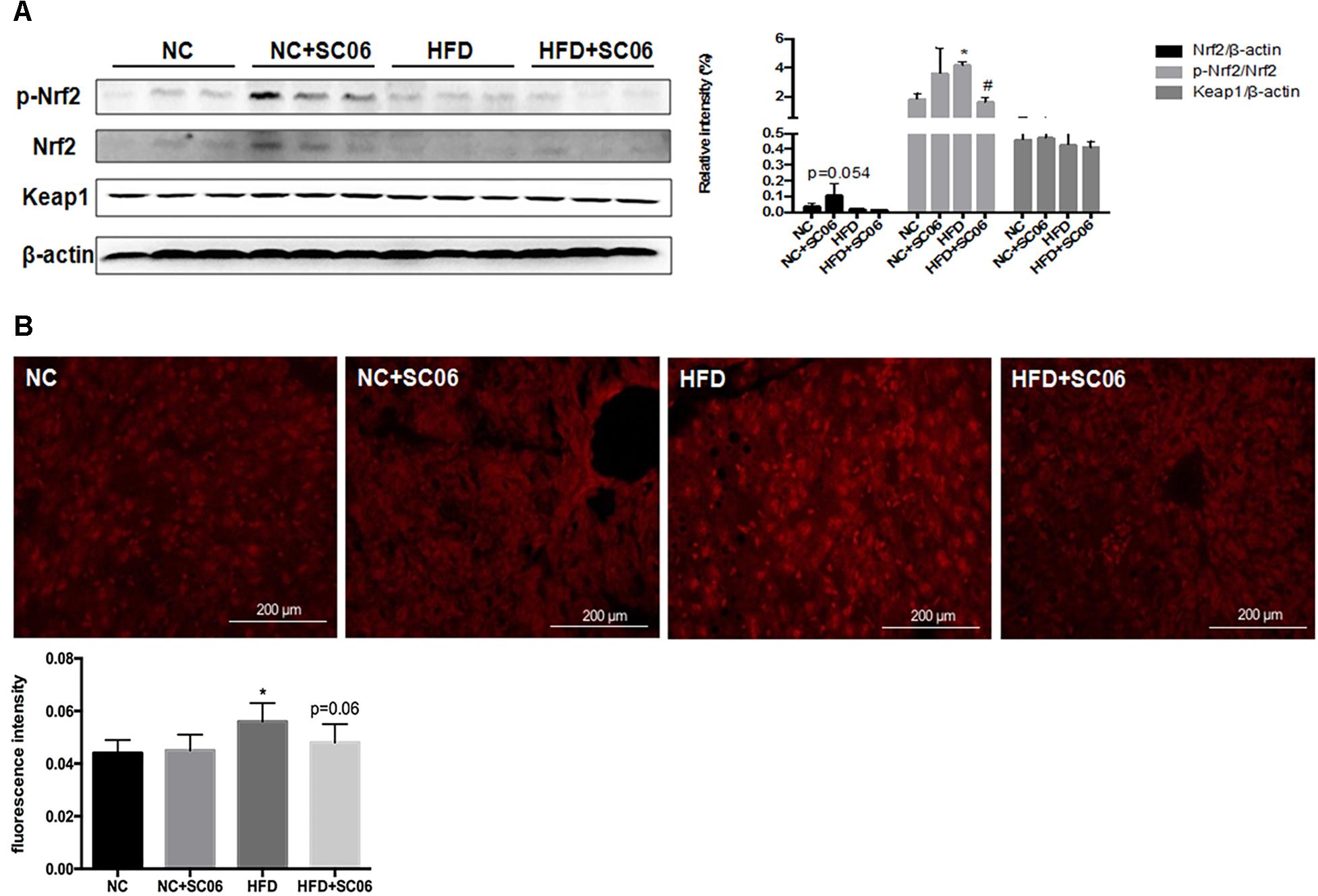
Figure 4. SC06 regulated Nrf2/Keap1 signaling pathway and ROS level. (A) Total protein levels of Keap1 and β-actin as well as the phosphorylated and total protein levels of Nrf2 in mice livers were determined using Abs recognizing phospho-specific or total protein, (B) ROS frozen sections. Fluorescence intensity was quantified by ImageJ software. Results are expressed as means ± SD. Differences between groups were determined by one-way ANOVA followed by Tukey’s t-test (n = 6). Significant differences between HFD versus ND are indicated as ∗p < 0.05. Significant differences between HFD versus HFD+SC06 are indicated as #p < 0.05.
Oxidative stress is derived either from an increase in ROS production or decreased levels of ROS scavenging. According to Figure 4B, HFD elevated ROS levels in liver (p < 0.05), but HFD+SC06 inhibited the elevated ROS concentration induced by HFD, although this was not statistically significant (p = 0.06).
Overall Structural Modulation of Gut Microbiota After SC06 Treatment
α-diversity (richness and evenness) of the communities was measured by Simpson’s, Goods_coverage’s, Chao1’s and Observed_species’ indexes, respectively (Figure 5). After 2- and 4-week HFD feeding, α-diversity was much higher than that of NC (p < 0.05). However, at the 8th week, there was no difference between the two groups (Figures 5A,B,D). In addition, as shown by the Simpson’s index, we observed that HFD+SC06 increased the α-diversity obviously at the 2nd week (p < 0.05) and the 4th week (p = 0.053), but not at the 8th week (Figure 5A). Principal component analysis revealed that gut microbiota in HFD group deviated from NC structure. At the 8th week, the gut bacterial composition profile in HFD+SC06 changed toward control group (Supplementary Figure S2).
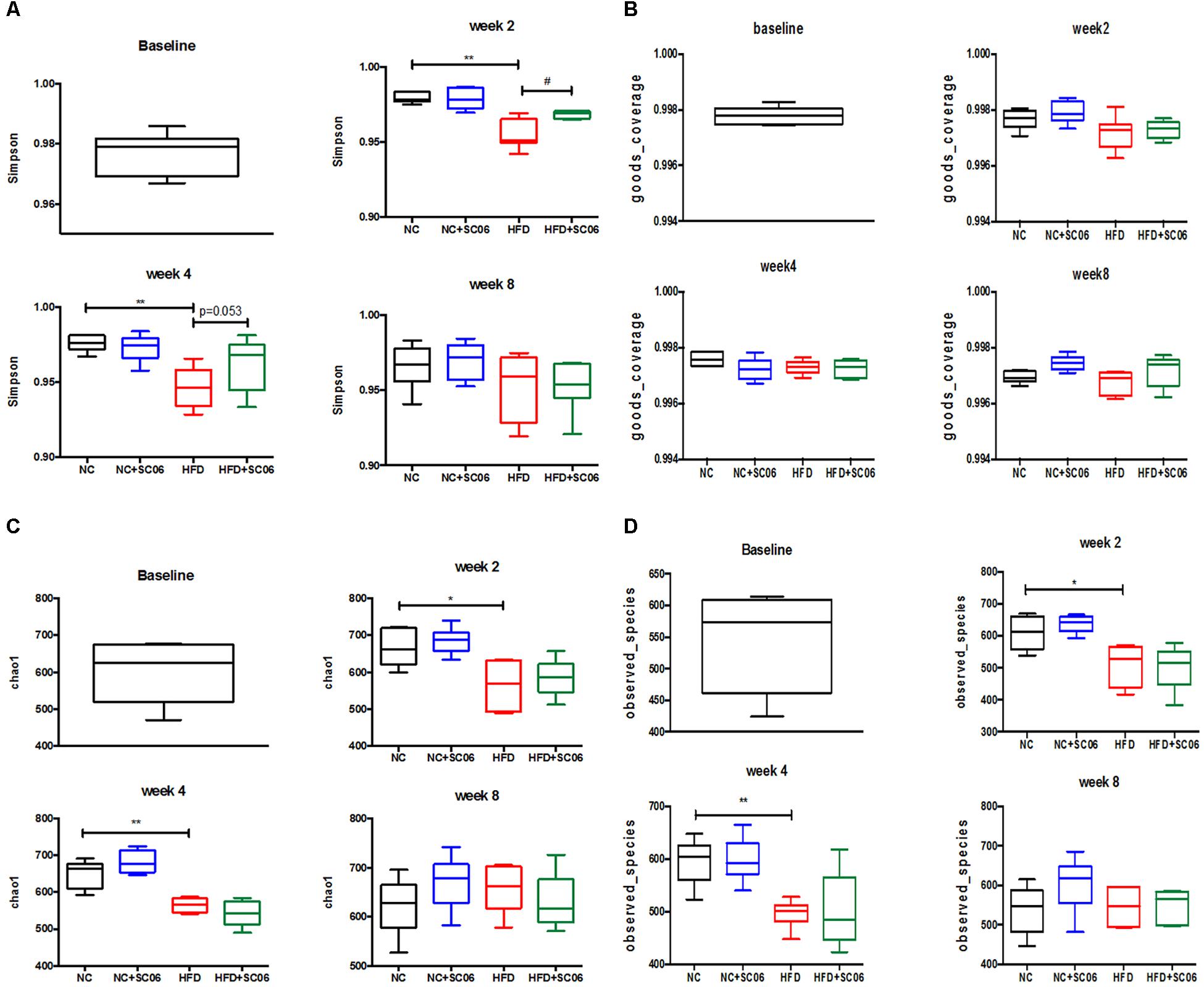
Figure 5. Changes in a-diversity of gut microbiota communities, as measured by (A) Simpson’s, (B) Goods_coverage’s, (C) Chao1’s, and (D) Observed_species’ Index Calculator. Differences between groups were determined by one-way ANOVA followed by Tukey’s t-test (n = 5). Significant differences between HFD versus NC are indicated as ∗p < 0.05, ∗∗p < 0.01. Significant differences between HFD versus HFD+SC06 are indicated as #p < 0.05.
Histograms illustrating the gut microbiota structure revealed the microbial species and their relative abundance (Figure 6). As obesity is characterized by a decrease in Bacteroidetes and an increase of Firmicutes (Nicholson et al., 2012). In the present study, we first determined the ratio of Firmicutes/Bacteroidetes. At the 4th week, HFD was associated with a significant increase in Firmicutes/Bacteroidetes (p < 0.05). Moreover, HFD+SC06 lowered Firmicutes/Bacteroidetes obviously compared to HFD-fed mice (Figure 6A). At phylum level, we also noticed that HFD increased Proteobacteria and TM7, especially at the 4th week (p < 0.05), while HFD+SC06 reduced TM7 abundance at the 4th week (p = 0.06). On the contrary, Verrucomicrobia almost disappeared in both HFD and HFD+SC06 groups (Figures 6B,C and Supplementary Figure S3). At family level, we found that S24-7 family accounted for the majority. At the 4th and 8th weeks, Clostridiaceae relative abundance was increased by HFD but inhibited by HFD+SC06 (p < 0.05) (Figure 6C and Supplementary Figure S3B). Furthermore, although most genera were unclassified, we also found that the genera profiles in HFD groups were distinct from that of NC groups. It is worth noting that increased Prevotella in HFD group was inhibited by HFD+SC06 at the 2nd week (p < 0.05) (Figure 6D and Supplementary Figure S3C). To compare community characteristics in more detail, heatmaps were constructed representing the relative abundance of species (Supplementary Figure S4). Results showed that most members of the gut microbiota belonging to S24-7 genus. The altered species in HFD group were seldom returned to normal by SC06 treatment at the 2nd and 4th weeks. However, some of the decreased S24-7 species (S24-7_346870, 315430, 264734, etc.) in mice receiving HFD were reversed in HFD+SC06 group at the 8th week.
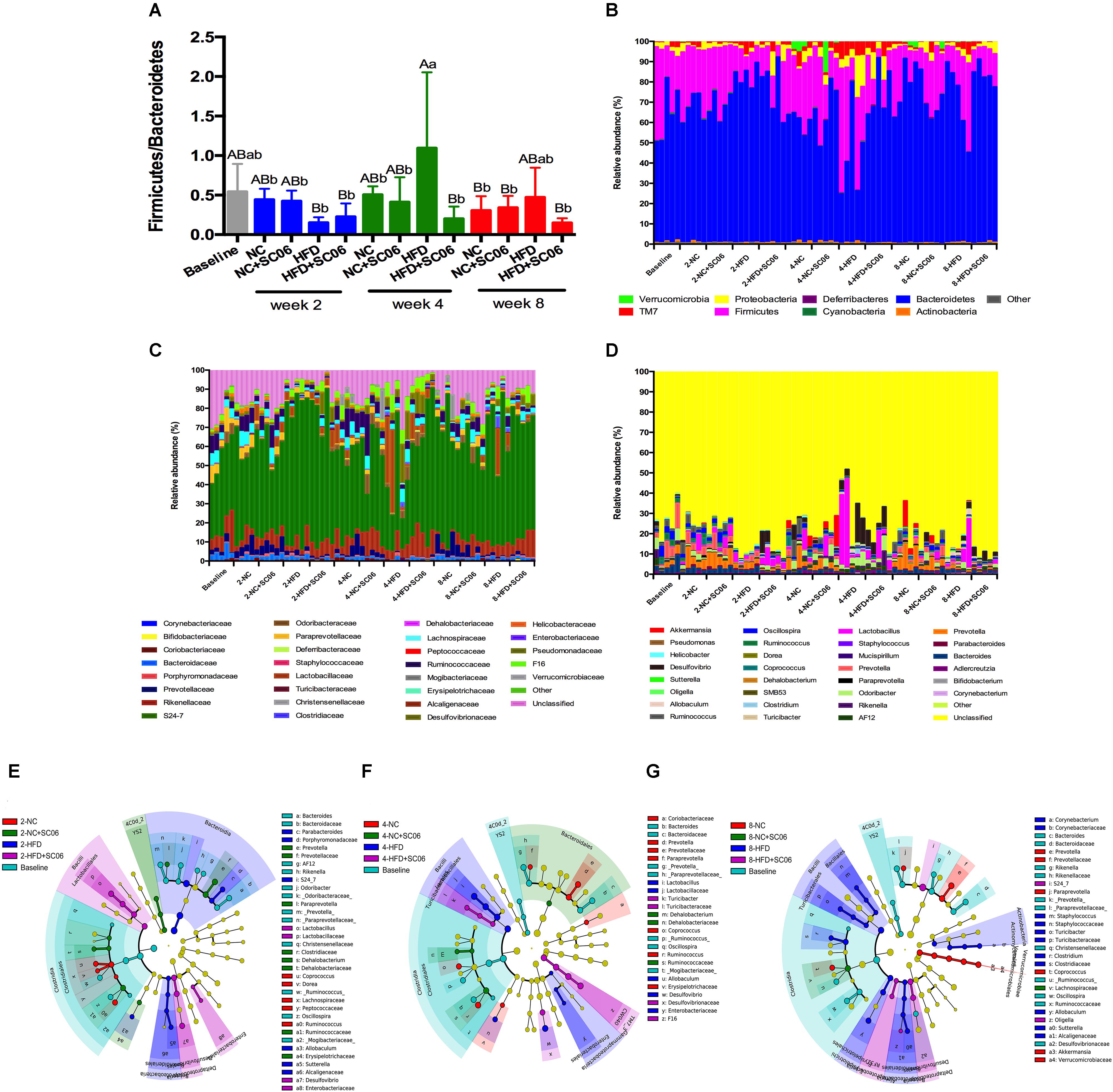
Figure 6. SC06 administration changed gut microbiota. (A) The ratio between Firmicutes and Bacteroidetes of gut microbiota, (B) phyla, (C) families, (D) genus of mice. Cladogram plotted from LeFSe analysis showing the taxonomic levels represented by rings with phyla in the outermost the ring and genera in the innermost ring. Each circle is a member within that level. (E) Week 2, (F) week 4, and (G) week 8.
LeFSe depicted time course changes of the dominant microbiota among groups (Figures 6E–G). We observed that at the 2nd week, the predominant intestinal flora in HFD were Bacteroidia and Betaproteobacteria. However, as time went on, branches of Bacilli became the major ones in HFD group at both 4th and 8th weeks. With HFD+SC06 feeding for different time points, the branches of Desulfovibrio, S24-7 and F16 were identified as novel superior microbiota (Figure 6).
Discussion
Consistent with previous research (Xin et al., 2014), here we found that using the same HFD also successfully induced mice obesity and the related metabolic disorder, indicated by higher fat deposition, insulin resistance and hepatic steatosis. However, SC06 treatment effectively improved these features of metabolic syndrome. Coelomate animals coevolve with a diverse range of gut microbiota (Nicholson et al., 2012). The coevolution between host and gut microorganisms have led to a mutualistic relationship in which the microbiota contributes to many host physiological processes and in turn, hosts provide niches and nutrients for microbial survival (Kamada et al., 2013). When the mutualistic relationship is disrupted, the disordered gut microbiota leads to diseases (Littman and Pamer, 2011). Obesity and associated metabolic disorders are characterized by “low-grade” inflammation (Hotamisligil, 2006). Changes in gut microbiota and epithelial functions may play a crucial role in inflammation associated with obesity (Cani et al., 2008). In this study, with the reduction of obesity, HFD+SC06 also significantly decreased the concentration of IL-6 and TNF-α compared to that of HFD. Thus, the role of SC06 in improving obesity and inflammation promoted us to figure out whether SC06 also attenuated gut bacterial dysbiosis. In mouse, the major distal gut microbiota are members belonging to Bacteroidetes and Firmicutes (Ley et al., 2005). Many studies have shown that the increased ratio of Firmicutes and Bacteroidetes is associated with the obesity phenotype (Ley et al., 2005; Moschen et al., 2012). In agreement with these findings, we observed that compared to NC group, Firmicutes/Bacteroidetes in HFD increased about twofold, which was decreased efficiently by SC06 administration. We also noticed that HFD increased relative abundance of TM7 and Proteobacteria at the 4th week, but decreased Verrucomicrobia and enriched TM7 was suppressed by SC06. In a previous report, obese individuals contained lower Verrucomicrobia abundance as well (Clarke et al., 2012). It was also suggested that Nlrp6-deficient mice with overrepresentation of Prevotellaceae and TM7 increased susceptibility to liver injury when on a methionine-choline-deficient to induce NASH (Elinav et al., 2011). TM7 can induce changes that result in inflammation in the colon and adipose tissues as well (Henao-Mejia et al., 2012). Differences were found not only at phyla level but also at all lower taxa levels among groups. Although it is shown that Clostridiaceae families were lowered in diet-induced obese mice (Clarke et al., 2013), a recent study found that Clostridiaceae was enriched in NAFLD patients (Jiang W.W. et al., 2015). Here, we found the increased Clostridiaceae induced by HFD was suppressed by SC06 treatment. Additionally, we also identified bacterial species whose abundance were altered by HFD and barely returned to control levels with SC06 approaches at the 2nd and 4th weeks. However, the abundance of species belonging to S24-7, including S24-7_346870, 315430 and 264734, was reversed by probiotic treatment at the 8th week. Bacteroidales S24-7 is a butyrate-producing bacterium associated with intestinal epithelial cell health (Villanueva-Millan et al., 2015). Qiu et al. (2017) believed S24-7 is negatively correlated with trimethylamine N-oxide, which can enhance accumulation of macrophage cholesterol and foam cell formation. On the contrary, Wu et al. (2017) considered S24-7 as the bad gut microbiota. Based on our results, we speculate that the increase of specific S24-7 strains might exert beneficial effect on the host. However, we didn’t notice significant differences for S24-7 abundance at family level between HFD and HFD+SC06 groups.
Because of the anatomical position and its unique vascular system, the liver is susceptible to the microbial products from the gut (Seo and Shah, 2012). Derangement of the gut microbiota occurs in a large percentage of patients with chronic liver disease, including NAFLD and NASH (Compare et al., 2012). The close interplay exists between the gut and liver is named “gut-liver axis.” Here, we also examined the liver injury in obese mice. HFD obviously increased GPT activity in the serum and elevated lipid accumulation, apoptosis and mitochondrial injury in the liver, which were reversed effectively in HFD+SC06. Lipid peroxidation and secondary cellular injury are the dominant mechanism in the transition from relatively stable hepatic steatosis to potentially progressive steatohepatitis in NAFLD. Oxidation of excessive fatty acids generates ROS that damage organelles and stimulate signaling pathways leading to fibrosis and cellular injury (Chang et al., 2006). Through analyzing the hepatic oxidation, we found that NC+SC06 significantly increased liver CAT activity. What’s more, oxidative stress was induced by HFD, but inhibited by SC06 administration, which was represented by the decreased hepatic ROS level and MDA concentration. Moreover, Nrf2 is a transcriptional activator that regulates cytoprotective gene expressions (Ishii et al., 2000). During oxidative stress, Nrf2 is released from its cytosolic repressor Keap1. The phosphorylated Nrf2 translocates into the nucleus where it binds to antioxidant reaction element residing within the promoter regions of many antioxidant and phase II genes (Lee and Johnson, 2004). According to our results, NC+SC06 elevated Nrf2 level to enhance the antioxidant ability. As a stress factor, HFD also significantly increased the phosphorylation of Nrf2 to resist the oxidative stress induced by diet. In the previous study, compounds with antioxidant capacity could activate Nrf2 pathway in combination with HFD (Feng et al., 2017). However, in the present study, with HFD+SC06 treatment, p-Nrf2 expression was markedly decreased. As far as our concerned, since the ROS and MDA levels were reduced in HFD+SC06 treated mice, it is not necessary for hepatocytes to activate more Nrf2 and increase antioxidase activities to combat with oxidative stress. Additionally, recent studies demonstrated Nrf2 ablation in mice adipose tissue prevented diet-induced obesity (Zhang et al., 2016), and mice lacking Nrf2 had more energy expenditure and resisted to diet-induced obesity (Schneider et al., 2016). Thus, as far as we concerned, the obvious inactivation of Nrf2 induced by HFD+SC06 might also protect against HFD-induced lipid accumulation.
Epithelial function is important for the development of NAFLD and obesity (Raybould, 2012). In a previous research, we suggested that SC06 was able to elevate the oxidation resistant capacity of intestinal porcine epithelial cell line (Wang et al., 2017), and the present study has provided novel information regarding the effects of SC06 on gut microbiota, hepatic steatosis and oxidative stress in mice (Figure 7).
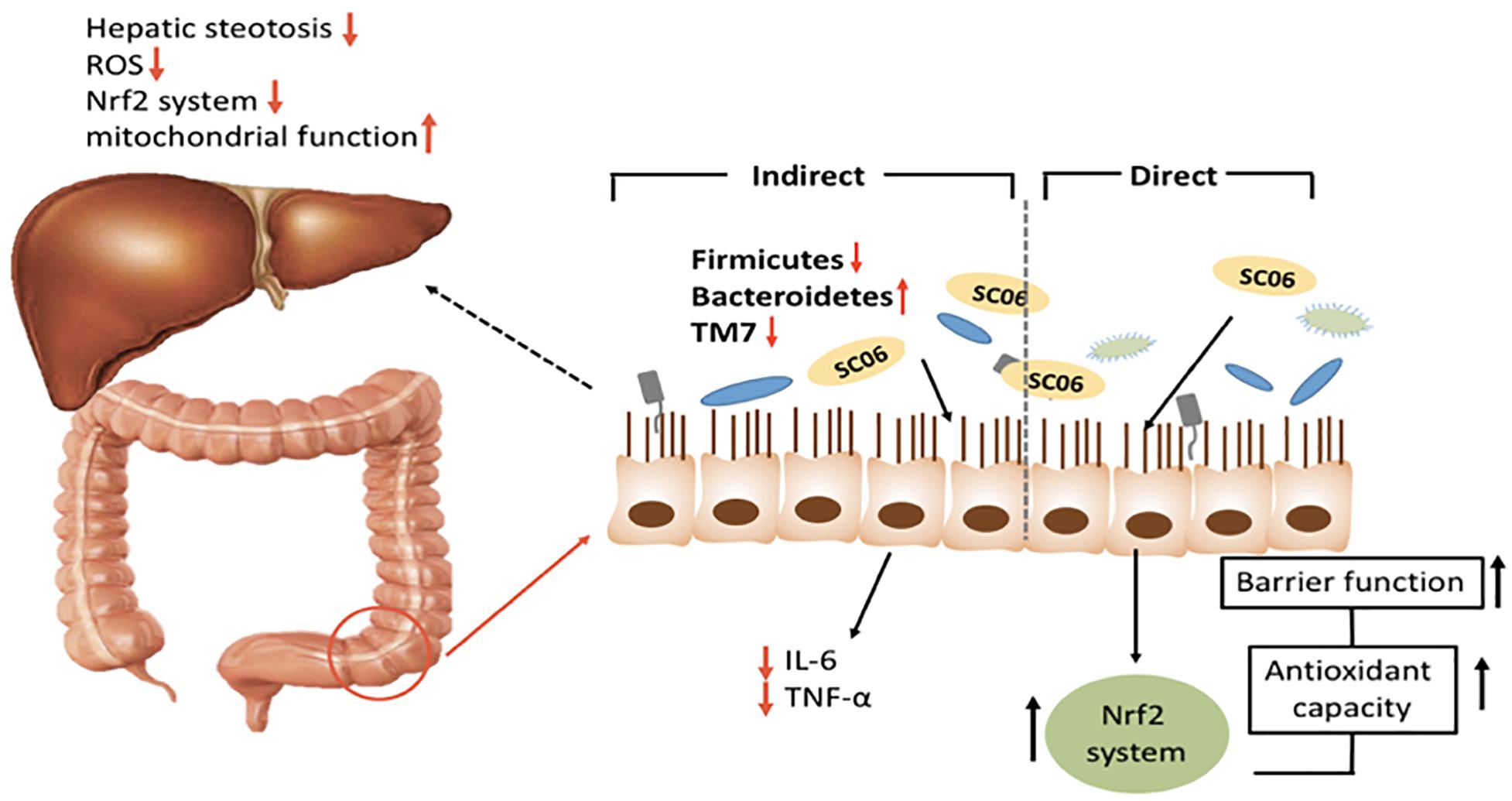
Figure 7. Graphical summary of multiple roles of SC06 in improving HFD-induced obesity and liver injury.
Taken together, we propose that SC06 protected mice from HFD-induced obesity and liver injury through (1) directly improving the epithelial function by enhancing intestinal epithelial cell antioxidant capacity, (2) decreasing inflammation, lowering hepatic oxidative damage and (3) regulating gut microbiota composition, especially Bacteroides, Firmicutes, TM7 phyla and species belonging to S24-7. These findings may aid in the application of probiotic Bacillus in the food industry to improve human and animal’s health. However, further investigation about the correlations among the decreased obesity, oxidative stress and gut bacterial composition are needed.
Author Contributions
WL and YPW designed the experiments. YPW, HX, and XX performed the animal husbandry. BW, XZ, and JN analyzed the 16S rRNA data. YW and XM did the animal experiments. YW wrote the final manuscript. All authors approved and contributed to the final version of the manuscript.
Funding
This study was supported by the National Natural Science Foundation of China (Nos. 31672460 and 31472128), the National High-Tech R&D Program (863) of China (No. 2013AA102803D), the Major Science and Technology Project of Zhejiang Province (No. 2006C12086), and the Key Laboratory of Animal Feed and Nutrition of Zhejiang Province (No. 201802).
Conflict of Interest Statement
The authors declare that the research was conducted in the absence of any commercial or financial relationships that could be construed as a potential conflict of interest.
Supplementary Material
The Supplementary Material for this article can be found online at: https://www.frontiersin.org/articles/10.3389/fmicb.2019.01161/full#supplementary-material
References
Backhed, F., Ding, H., Wang, T., Hooper, L. V., Koh, G. Y., Nagy, A., et al. (2004). The gut microbiota as an environmental factor that regulates fat storage. Proc. Natl. Acad. Sci. U.S.A. 101, 15718–15723. doi: 10.1073/pnas.0407076101
Barreyro, F. J., Holod, S., Finocchietto, P. V., Camino, A. M., Aquino, J. B., Avagnina, A., et al. (2015). The pan-caspase inhibitor Emricasan (IDN-6556) decreases liver injury and fibrosis in a murine model of non-alcoholic steatohepatitis. Liver Int. 35, 953–966. doi: 10.1111/liv.12570
Birse, R. T., Choi, J., Reardon, K., Rodriguez, J., Graham, S., Diop, S., et al. (2010). High-fat-diet-induced obesity and heart dysfunction are regulated by the TOR pathway in Drosophila. Cell. Metab. 12, 533–544. doi: 10.1016/j.cmet.2010.09.014
Cani, P. D., Bibiloni, R., Knauf, C., Neyrinck, A. M., Neyrinck, A. M., Delzenne, N. M., et al. (2008). Changes in gut microbiota control metabolic endotoxemia-induced inflammation in high-fat diet-induced obesity and diabetes in mice. Diabetes 57, 1470–1481. doi: 10.2337/db07-1403
Carmiel-Haggai, M., Cederbaum, A. I., and Nieto, N. (2004). A high-fat diet leads to the progression of non-alcoholic fatty liver disease in obese rats. FASEB J. 18, 136–138. doi: 10.1096/fj.04-2291fje
Chang, C. Y., Argo, C. K., Al-Osaimi, A. M., and Caldwell, S. H. (2006). Therapy of NAFLD: antioxidants and cytoprotective agents. J. Clin. Gastroenterol. 40(Suppl. 1), S51–S60.
Charradi, K., Elkahoui, S., Limam, F., and Aouani, E. (2013). High-fat diet induced an oxidative stress in white adipose tissue and disturbed plasma transition metals in rat: prevention by grape seed and skin extract. J. Physiol. Sci. 63, 445–455. doi: 10.1007/s12576-013-0283-6
Clarke, S. F., Murphy, E. F., Nilaweera, K., Ross, P. R., Shanahan, F., O’Toole, P. W., et al. (2012). The gut microbiota and its relationship to diet and obesity: new insights. Gut Microbes 3, 186–202. doi: 10.4161/gmic.20168
Clarke, S. F., Murphy, E. F., O’Sullivan, O., Ross, R. P., O’Toole, P. W., Shanahan, F., et al. (2013). Targeting the microbiota to address diet-induced obesity: a time dependent challenge. Plos One 8:e65790. doi: 10.1371/journal.pone.0065790
Compare, D., Coccoli, P., Rocco, A., Nardone, O. M., De Maria, S., Carteni, M., et al. (2012). Gut-liver axis: the impact of gut microbiota on non alcoholic fatty liver disease. Nutr. Metab. Cardiovas. 22, 471–476. doi: 10.1016/j.numecd.2012.02.007
Dietz, W. H., Baur, L. A., Hall, K., Puhl, R. M., Taveras, E. M., Uauy, R., et al. (2015). Management of obesity: improvement of health-care training and systems for prevention and care. Lancet 385, 2521–2533. doi: 10.1016/S0140-6736(14)61748-7
Do, H. J., Chung, J. H., Hwang, J. W., Kim, O. Y., Lee, J. Y., and Shin, M. J. (2015). 1-Deoxynojirimycin isolated from Bacillus subtilis improves hepatic lipid metabolism and mitochondrial function in high-fat-fed mice. Food Chem. Toxicol. 75, 1–7. doi: 10.1016/j.fct.2014.11.001
Elinav, E., Strowig, T., Kau, A. L., Henao-Mejia, J., Thaiss, C. A., Booth, C. J., et al. (2011). NLRP6 inflammasome regulates colonic microbial ecology and risk for colitis. Cell 145, 745–757. doi: 10.1016/j.cell.2011.04.022
Feng, X. J., Yu, W., Li, X. D., Zhou, F. F., Zhang, W. L., Shen, Q., et al. (2017). Apigenin, a modulator of PPAR gamma, attenuates HFD-induced NAFLD by regulating hepatocyte lipid metabolism and oxidative stress via Nrf2 activation. Biochem. Pharmacol. 136, 136–149. doi: 10.1016/j.bcp.2017.04.014
Foster, T., Budoff, M. J., Saab, S., Ahmadi, N., Gordon, C., and Guerci, A. D. (2011). Atorvastatin and antioxidants for the treatment of nonalcoholic fatty liver disease: the St Francis heart study randomized clinical trial. Am. J. Gastroenterol. 106, 71–77. doi: 10.1038/ajg.2010.299
Furukawa, S., Fujita, T., Shimabukuro, M., Iwaki, M., Yamada, Y., Nakajima, Y., et al. (2004). Increased oxidative stress in obesity and its impact on metabolic syndrome. J. Clin. Invest. 114, 1752–1761. doi: 10.1172/JCI21625
Hall, J. E., do Carmo, J. M., da Silva, A. A., Wang, Z., and Hall, M. E. (2015). Obesity-induced hypertension interaction of neurohumoral and renal mechanisms. Circ. Res. 116, 991–1006. doi: 10.1161/Circresaha.116.305697
Haslam, D. W., and James, W. P. T. (2005). Obesity. Lancet 366, 1197–1209. doi: 10.1016/S0140-6736(05)67483-1
Henao-Mejia, J., Elinav, E., Jin, C., Hao, L., Mehal, W. Z., Strowig, T., et al. (2012). Inflammasome-mediated dysbiosis regulates progression of NAFLD and obesity. Nature 482, 179–185. doi: 10.1038/nature10809
Hotamisligil, G. S. (2006). Inflammation and metabolic disorders. Nature 444, 860–867. doi: 10.1038/nature05485
Ishii, T., Itoh, K., Takahashi, S., Sato, H., Yanagawa, T., Katoh, Y., et al. (2000). Transcription factor Nrf2 coordinately regulates a group of oxidative stress-inducible genes in macrophages. J. Biol. Chem. 275, 16023–16029. doi: 10.1074/jbc.275.21.16023
Jiang, W. W., Wu, N., Wang, X. M., Chi, Y. J., Zhang, Y. Y., Qiu, X. Y., et al. (2015). Dysbiosis gut microbiota associated with inflammation and impaired mucosal immune function in intestine of humans with non-alcoholic fatty liver disease. Sci. Rep. 5:8096. doi: 10.1038/srep08096
Jiang, Y., Xu, Y., Bi, Y. F., Wang, L. M., Zhang, M., Zhou, M. G., et al. (2015). Prevalence and trends in overweight and obesity among Chinese adults in 2004-10: data from three nationwide surveys in China. Lancet 386:77. doi: 10.1016/S0140-6736(15)00658-3
Jones, R. M., Desai, C., Darby, T. M., Luo, L., Wolfarth, A. A., Scharer, C. D., et al. (2015). Lactobacilli modulate epithelial cytoprotection through the Nrf2 Pathway. Cell Rep. 12, 1217–1225. doi: 10.1016/j.celrep.2015.07.042
Jumpertz, R., Le, D. S., Turnbaugh, P. J., Trinidad, C., Bogardus, C., Gordon, J. I., et al. (2011). Energy-balance studies reveal associations between gut microbes, caloric load, and nutrient absorption in humans. Am. J. Clin. Nutr. 94, 58–65. doi: 10.3945/ajcn.110.010132
Kamada, N., Seo, S. U., Chen, G. Y., and Nunez, G. (2013). Role of the gut microbiota in immunity and inflammatory disease. Nat. Rev. Immunol. 13, 321–335. doi: 10.1038/nri3430
Kim, S., Sohn, I., Ahn, J. I., Lee, K. H., Lee, Y. S., and Lee, Y. S. (2004). Hepatic gene expression profiles in a long-term high-fat diet-induced obesity mouse model. Gene 340, 99–109. doi: 10.1016/j.gene.2004.06.015
Kirpich, I. A., Marsano, L. S., and McClain, C. J. (2015). Gut-liver axis, nutrition, and non-alcoholic fatty liver disease. Clin. Biochem. 48, 923–930. doi: 10.1016/j.clinbiochem.2015.06.023
Kumar, M., Nagpal, R., Kumar, R., Hemalatha, R., Verma, V., Kumar, A., et al. (2012). Cholesterol-lowering probiotics as potential biotherapeutics for metabolic diseases. Exp. Diabetes Res. 2012:902917. doi: 10.1155/2012/902917
Lee, J. M., and Johnson, J. A. (2004). An important role of Nrf2-ARE pathway in the cellular defense mechanism. J. Biochem. Mol. Biol. 37, 139–143. doi: 10.5483/bmbrep.2004.37.2.139
Lei, K., Li, Y. L., Wang, Y., Wen, J., Wu, H. Z., Yu, D. Y., et al. (2015). Effect of dietary supplementation of Bacillus subtilis B10 on biochemical and molecular parameters in the serum and liver of high-fat diet-induced obese mice. J. Zhejiang Univ. Sci. B 16, 487–495. doi: 10.1631/jzus.B1400342
Ley, R. E., Backhed, F., Turnbaugh, P., Lozupone, C. A., Knight, R. D., and Gordon, J. I. (2005). Obesity alters gut microbial ecology. Proc. Natl. Acad. Sci. U.S.A. 102, 11070–11075. doi: 10.1073/pnas.0504978102
Li, C., Nie, S. P., Zhu, K. X., Ding, Q., Li, C., Xiong, T., et al. (2014). Lactobacillus plantarum NCU116 improves liver function, oxidative stress and lipid metabolism in rats with high fat diet induced non-alcoholic fatty liver disease. Food Funct. 5, 3216–3223. doi: 10.1039/c4fo00549j
Littman, D. R., and Pamer, E. G. (2011). Role of the commensal microbiota in normal and pathogenic host immune responses. Cell Host Microbe 10, 311–323. doi: 10.1016/j.chom.2011.10.004
Moschen, A. R., Wieser, V., and Tilg, H. (2012). Dietary factors: major regulators of the gut’s microbiota. Gut Liver 6, 411–416. doi: 10.5009/gnl.2012.6.4.411
Musso, G., Anty, R., and Petta, S. (2013). Antioxidant therapy and drugs interfering with lipid metabolism: could they be effective in NAFLD patients? Curr. Pharm. Design. 19, 5297–5313. doi: 10.2174/1381612811319290010
Nicholson, J. K., Holmes, E., Kinross, J., Burcelin, R., Gibson, G., Jia, W., et al. (2012). Host-gut microbiota metabolic interactions. Science 336, 1262–1267. doi: 10.1016/j.chom.2008.02.015
Nikoskelainen, S., Ouwehand, A. C., Bylund, G., Salminen, S., and Lilius, E. M. (2003). Immune enhancement in rainbow trout (Oncorhynchus mykiss) by potential probiotic bacteria (Lactobacillus rhamnosus). Fish Shellfish Immunol. 15, 443–452. doi: 10.1016/S1050-4648(03)00023-8
Ogden, C. L., Carroll, M. D., and Flegal, K. M. (2014). Prevalence of obesity in the United States. JAMA J. Am. Med. Assoc. 312:189. doi: 10.1001/jama.2014.6219
Park, S., Ji, Y., Jung, H. Y., Park, H., Kang, J., Choi, S. H., et al. (2017). Lactobacillus plantarum HAC01 regulates gut microbiota and adipose tissue accumulation in a diet-induced obesity murine model. Appl. Microbiol. Biotechnol. 101, 1605–1614. doi: 10.1007/s00253-016-7953-2
Qiu, L., Yang, D., Tao, X. Y., Yu, J., Xiong, H., and Wei, H. (2017). Enterobacter aerogenes ZDY01 attenuates choline-induced trimethylamine N-Oxide levels by remodeling gut microbiota in mice. J. Microbiol. Biotechnol. 27, 1491–1499. doi: 10.4014/jmb.1703.03039
Rajput, I. R., Li, W. F., Li, Y. L., Jian, L., and Wang, M. Q. (2013). Application of probiotic (Bacillus subtilis) to enhance immunity, antioxidation, digestive enzymes activity and hematological profile of Shaoxing Duck. Pak. Vet. J. 33, 69–72.
Raybould, H. E. (2012). Gut microbiota, epithelial function and derangements in obesity. J. Physiol. 590, 441–446. doi: 10.1113/jphysiol.2011.222133
Schneider, K., Valdez, J., Nguyen, J., Vawter, M., Galke, B., Kurtz, T. W., et al. (2016). Increased energy expenditure, Ucp1 expression, and resistance to diet-induced obesity in mice lacking nuclear factor-erythroid-2-related transcription factor-2 (Nrf2). J. Biol. Chem. 291, 7754–7766. doi: 10.1074/jbc.m115.673756
Seo, Y. S., and Shah, V. H. (2012). The role of gut-liver axis in the pathogenesis of liver cirrhosis and portal hypertension. Clin. Mol. Hepatol. 18, 337–346. doi: 10.3350/cmh.2012.18.4.337
Stolz, D. B., Ross, M. A., Salem, H. M., Mars, W. M., Michalopoulos, G. K., and Enomoto, K. (1999). Cationic colloidal silica membrane perturbation as a means of examining changes at the sinusoidal surface during liver regeneration. Am. J. Pathol. 155, 1487–1498. doi: 10.1016/s0002-9440(10)65464-8
Tappy, L., and Le, K. A. (2010). Metabolic effects of fructose and the worldwide increase in obesity. Physiol. Rev. 90, 23–46. doi: 10.1152/physrev.00019.2009
Turnbaugh, P. J., Baeckhed, F., Fulton, L., and Gordon, J. I. (2008). Diet-induced obesity is linked to marked but reversible alterations in the mouse distal gut microbiome. Cell Host Microbe 3, 213–223. doi: 10.1016/j.chom.2008.02.015
Villanueva-Millan, M. J., Perez-Matute, P., and Oteo, J. A. (2015). Gut microbiota: a key player in health and disease. A review focused on obesity. J. Physiol. Biochem. 71, 509–525. doi: 10.1007/s13105-015-0390-3
Wang, J. J., Tang, H., Zhang, C. H., Zhao, Y. F., Derrien, M., Rocher, E., et al. (2015). Modulation of gut microbiota during probiotic-mediated attenuation of metabolic syndrome in high fat diet-fed mice. ISME J. 9, 1–15. doi: 10.1038/ismej.2014.99
Wang, Y., Wu, Y. P., Wang, Y. B., Fu, A. K., Gong, L., Li, W. F., et al. (2017). Bacillus amyloliquefaciens SC06 alleviates the oxidative stress of IPEC-1 via modulating Nrf2/Keap1 signaling pathway and decreasing ROS production. Appl. Microbiol. Biotechnol. 101, 3015–3026. doi: 10.1007/s00253-016-8032-4
Wu, Q. Z., Zhang, H. C., Wang, P. G., and Chen, M. (2017). Evaluation of the efficacy and safety of Ganoderma lucidum mycelium-fermented liquid on gut microbiota and its impact on cardiovascular risk factors in human. Rsc. Adv. 7, 45093–45100. doi: 10.1039/c7ra08087e
Xin, J. G., Zeng, D., Wang, H. S., Ni, X. Q., Yi, D., Pan, K. C., et al. (2014). Jing, B. Preventing non-alcoholic fatty liver disease through Lactobacillus johnsonii BS15 by attenuating inflammation and mitochondrial injury and improving gut environment in obese mice. Appl. Microbiol. Biotechnol. 98, 6817–6829. doi: 10.1007/s00253-014-5752-1
Keywords: Bacillus amyloliquefaciens, high-fat diet, liver injury, gut microbiota, oxidative stress
Citation: Wang Y, Wu Y, Wang B, Xu H, Mei X, Xu X, Zhang X, Ni J and Li W (2019) Bacillus amyloliquefaciens SC06 Protects Mice Against High-Fat Diet-Induced Obesity and Liver Injury via Regulating Host Metabolism and Gut Microbiota. Front. Microbiol. 10:1161. doi: 10.3389/fmicb.2019.01161
Received: 04 September 2018; Accepted: 07 May 2019;
Published: 28 May 2019.
Edited by:
Haitao Lu, Shanghai Jiao Tong University, ChinaReviewed by:
Maria de los Angeles Serradell, National Council for Scientific and Technical Research (CONICET), ArgentinaAlex Galanis, Democritus University of Thrace, Greece
Copyright © 2019 Wang, Wu, Wang, Xu, Mei, Xu, Zhang, Ni and Li. This is an open-access article distributed under the terms of the Creative Commons Attribution License (CC BY). The use, distribution or reproduction in other forums is permitted, provided the original author(s) and the copyright owner(s) are credited and that the original publication in this journal is cited, in accordance with accepted academic practice. No use, distribution or reproduction is permitted which does not comply with these terms.
*Correspondence: Xiaoping Zhang, emhhbmd4aWFvcGluZzQwM0BnbWFpbC5jb20=; Jiajia Ni, bmlqaWFqaWEyMDA1QDEyNi5jb20=; Weifen Li, d2VpZmVubGlAemp1LmVkdS5jbg==