- 1Guangdong Province Key Laboratory of Microbial Signals and Disease Control, College of Agriculture, South China Agricultural University, Guangzhou, China
- 2Sugarcane Research Institute, Guangxi Academy of Agricultural Sciences, Nanning, China
- 3State Key Laboratory of Conservation and Utilization of Subtropical Agro-Bioresources, Guangxi University, Nanning, China
The basidiomycetous fungus Sporisorium scitamineum causes a serious sugarcane smut disease in major sugarcane growing areas. Sexual mating is essential for infection to the host; however, its underlying molecular mechanism has not been fully studied. In this study, we identified a conserved farnesyltransferase (FTase) β subunit Ram1 in S. scitamineum. The ram1Δ mutant displayed significantly reduced mating/filamentation, thus of weak pathogenicity to the host cane. The ram1Δ mutant sporidia showed more tolerant toward cell wall stressor Congo red compared to that of the wild-type. Transcriptional profiling showed that Congo red treatment resulted in notable up-regulation of the core genes involving in cell wall integrity pathway in ram1Δ sporidia compared with that of WT, indicating that Ram1 may be involved in cell wall integrity regulation. In yeast the heterodimeric FTase is responsible for post-translational modification of Ras (small G protein) and a-factor (pheromone). We also identified and characterized two conserved Ras proteins, Ras1 and Ras2, respectively, and a MAT-1 pheromone precursor Mfa1. The ras1Δ, ras2Δ and mfa1Δ mutants all displayed reduced mating/filamentation similar as the ram1Δ mutant. However, both ras1Δ and ras2Δ mutants were hypersensitive to Congo red while the mfa1Δ mutant was the same as wild-type. Overall our study displayed that RAM1 plays an essential role in S. scitamineum mating/filamentation, pathogenicity, and cell wall stability.
Introduction
Sugarcane smut disease caused by the basidiomycetous fungus Sporisorium scitamineum is one of the most severe diseases in world-wide sugarcane growing areas. The three-stage morphological transitions are most prominent during the smut fungus life cycle: the haploid sporidia switch to dikaryotic hyphal growth to infect the plant cane; in the later growth period, diploid teliospores form within the host stem; and after teliospore germination, haploid sporidia are generated by successive budding (Taniguti et al., 2015). Therefore switching from non-pathogenic yeast-like sporidia to pathogenic hypha through sexual mating between two compatible sporidia is a prerequisite for infection. In the phytopathogenic basidiomycete model fungus, Ustilago maydis, biallelic a locus and multiallelic b locus comprise the tetrapolar mating system. The a locus encodes pheromone precursor MFA1/2 and receptor PRA1/2, controlling sporidial recognition and fusion during the sexual mating process. The b locus encodes bE and bW proteins forming a heterodimeric transcription factor, activating the filamentation (Hartmann et al., 1996; Kahmann and Kamper, 2004). In S. scitamineum mfa2 knock-out mutants in MAT-2 background were mating-deficient (Lu et al., 2017), and b locus deletion mutants were defective in filamentous growth (Yan et al., 2016a), suggesting common molecular characters between S. scitamineum and U. maydis. However, the molecular mechanisms of S. scitamineum mating/filamentation and/or pathogenicity remain largely unclear.
The biogenesis of mature pheromones has been well elaborated in Saccharomyces cerevisiae for its pheromone a-factor (Michaelis and Barrowman, 2012), during which the a-factor precursor undergoes a sequential processing by three separate modules, namely the C-terminal processing (prenylation by farnesyltransferase Ram1/Ram2, proteolysis by endoproteases Ste24 or Rce1, and Carboxymethylation by methyltransferase Ste14), the N-terminal proteolytic cleavage by endoproteases Ste24 and Axl1, and non-classical export by the ATP binding cassette (ABC) transporter Ste6 (Young et al., 2005; Paumi et al., 2009). The prenylation of the secreted a-factor-like pheromones is a common feature of ascomycetes and basidiomycetes (Brown and Casselton, 2001; Whiteway, 2011), including U. maydis (Spellig et al., 1994; Koppitz et al., 1996), Ustilago hordei (Kosted et al., 2000), Cryptococcus neoformans (Shen et al., 2002), Schizophyllum commune (Fowler et al., 1999), and Sporisorium reilianum (Schirawski et al., 2005).
Prenylation is a post-translational modification in which hydrophobic groups are added to the C-terminus of the protein (Michaelis and Barrowman, 2012). Modification of proteins at C-terminal cysteine residue(s) by the isoprenoids farnesyl (C15) and geranylgeranyl (C20) as lipid donors is essential for the biological function of a number of eukaryotic proteins (Leach and Brown, 2012). Three highly conserved prenyltransferases have been described in eukaryotic cells, and are responsible for these protein modifications: protein farnesyltransferase (FTase) and protein geranyltransferase (GGTase) types I and II. β subunit Ram1 (Ras and a-factor maturation) and α subunit Ram2 comprising the heterodimeric FTase (Omer and Gibbs, 1994). Ultimately, yeast and mammalian Ras proteins, yeast a-factor, and the mammalian nuclear scaffold protein lamin B, share a common motif CAAX (“C” is cysteine, “A” is often an aliphatic amino acid, and “X” is any residue except Phe or Leu) at their C-terminus, which are farnesylated by Ram1/Ram2 (Leung et al., 2006). The biological functions of FTase have been investigated by characterizing RAM1-deletion mutants in S. cerevisiae (He et al., 1991), C. neoformans (Vallim et al., 2004; Esher et al., 2016), Candida albicans (Song and White, 2003) and Aspergillus fumigatus (Qiao et al., 2017), while the RAM2 gene seems to be essential. However, the RAM1 function has not been reported in S. scitamineum.
In this study, we identified and characterized conserved RAM1, RAS1, RAS2, and MFA1 genes in S. scitamineum. The ram1Δ, ras1Δ, ras2Δ, and mfa1Δ mutants all displayed reduced mating/filamentation compared with wild-type, which could be differentially restored by exogenous addition of Synthetic (farnesylated) Mfa1 peptide or cAMP. The ram1Δ mutant displayed enhanced tolerance, while the ras1Δ and ras2Δ mutants were hypersensitive toward cell wall stressor Congo red. However, and the mfa1Δ mutant was comparable with WT in stress tolerance to Congo red. In summary, we here report that the FTase β subunit Ram1 as a critical virulence factor in sugarcane smut fungus S. scitamineum. Ram1 and Ras proteins are both involved in cell wall integrity pathway of S. scitamineum but maybe of different signaling pathway.
Materials and Methods
Multiple Sequence Alignment and Phylogenetic Analysis
Amino acid sequences were aligned by ClustalX 2.0 (Thompson et al., 1994) with the following parameters: pairwise alignment parameters (gap opening = 10, gap extension = 0.1) and multiple alignment parameters (gap opening = 10, gap extension = 0.2, transition weight = 0.5, delay divergent sequences = 25%). The alignment was phylogenetically analyzed with maximum likelihood by MEGA 6.0 (Kenaley et al., 2018), using a Le-Gasquel amino acid replacement matrix with 1,000 bootstrap replications. The tree graph was viewed in Tree-View1.1. The alignment of amino acid sequence was adjusted in BioEdit software.
Strains and Growth Conditions
Two S. scitamineum wild-type haploid MAT-1 (a1b1) and MAT-2 (a2b2) isolates were obtained from teliospores on sugarcane in Guangdong providence, China (Yan et al., 2016c), and stored locally. The culture medium used in this study included YePSA medium (yeast extract 1%, peptone 2%, sucrose 2%, and agar 2%) and YePSL medium (yeast extraction 1%, peptone 2%, sucrose 2%). For mating and stress tolerance assays, S. scitamineum strains were grown in shake culture in YePSL at 28°C for 12 h. The sporidia were harvested via centrifugation and washed twice in distilled water, and concentrations adjusted to obtain OD600 = 1.0 for serial 10-fold dilutions in distilled water (Krombach et al., 2018). The diluted sporidial suspensions of compatible mating type were mixed together in equal volume and plated on YePSA, incubated in dark at 28°C for 48 h before assessment, and photographed. For stress tolerance assessment, the sporidial culture at OD600 = 1.0 and its serial 10-fold dilutions were spotted on MM medium (Chakraborty et al., 1991) in the absence or presence of stress inducers, including 20 μg/mL Congo red (GENVIEW, DC241), 25 μg/mL calcofluor white (Sigma, 18909), 20 μg/mL SDS (GENVIEW, GS286), 1 M Sorbitol (TGI, S0065), 0.5 mM H2O2 (Damao, 7722841), and incubated in dark at 28°C for 48 h before assessment and photographing. For testing the effects of exogenous cyclic AMP (cAMP; Sigma, A9501) was added to MM plates to reach final concentrations of 2.5 or 5 mM. For testing the effect of MAT-1 pheromone, exogenous synthetic Mfa1 (Sigma, with or without farnesylation at C-terminal CAAX motif) was added to MM to reach a final concentration of 1 or 10 μg/mL. For colony counting, the S. scitamineum strains were shake cultured in YePSL at 28°C for 12 h, before harvesting. Sporidia were washed twice and diluted in distilled water to OD600 = 1.0, from which serial 10-fold dilutions were generated in distilled water to an OD600 of 10–5. An aliquot of 150 μL of this diluted sporidial suspension was incubated on solid medium at 28°C, and the colony forming unit (CFU) was counted after 48 h. At least three independent biological replicates of colony counting were performed each time.
Strains for Gene Deletion and Complementation
Deletion of targeted genes (listed in Supplementary Table S1) follows the same strategy previously described (Li et al., 2014). For generation of deletion mutants, the 1–1.5 kb left and 1–1.5 kb right borders of each targeted gene were PCR-amplified from S. scitamineum wild-type genomic DNA separately, and two overlapping HPT fragments (for hygromycin resistance) were amplified from pDAN (HYGR). These PCR products were used as templates in fusion PCR to generate two PCR fragments separately containing the left or right border of targeted genes with the truncated, partial-overlapped HPT fragments. These two fusion-PCR products were transformed into S. scitamineum wild-type MAT-1 protoplasts via polyethylene glycol (PEG)-mediated transformation (Deng et al., 2018).
For RAM1, RAS1, RAS2, and MFA1 complementation, a fragment containing the 1–1.5 kb 5′ flanking sequence, whole coding sequence and 1 kb 3′ flanking sequence of the respective gene was PCR amplified with wild-type genomic DNA as template, using the primers pair listed in Supplementary Table S2. This fragment was inserted into the engineered pEX2 (ZeocinR) derived plasmid (Williams and Birnbaum, 1988) through homologous recombination by using ClonExpress II One Step Cloning Kit (Vazyme, C112-01), to form the complementary plasmid pEX2-RAM1, pEX2-RAS1, or pEX2-RAS2, respectively. The complementary plasmid was, respectively, transformed into the corresponding deletion mutant via PEG-mediated transformation and screened by Zeocin resistance, and confirmed by PCR and Southern blot analysis.
For construction of eGFP-RAM1 plasmid, coding sequence of eGFP was PCR amplified using pEX1 (Sun et al., 2014) as template, and 1818 bp of RAM1 coding sequence was PCR amplified using genomic DNA of wild-type strain as template. These two PCR amplified fragments were then fused by PCR amplification and cloned into the engineered pEX2 (ZeocinR) derived plasmid, driven by a constitutive (G3PD) promoter. The two fusion homologous fragments were PCR amplified as one fragment containing HPT-LB fused with complete G3PD-eGFP-RAM1 sequence and partially overlapped fragments of the zeocin gene, and the other containing partially overlapped fragments of the zeocin gene and HPT-RB. This construct was used to replace the hygromycin gene (HPT) in the ram1Δ mutant, with complementary eGFP-RAM1 coding sequence and zeocin resistance gene. Primers used in this study are listed in Supplementary Table S2.
Nucleic Acid Manipulation
Fungal genomic DNA was extracted using a modified SDS method (Yan et al., 2016b). PCR amplification was performed using KOD High-Fidelity DNA Polymerase FX (TOYOBO, KEX-101). Purification of DNA fragments was done using a Gel Extraction Kit (Omega, D2500-02) or a Cycle Pure Kit (Omega, D6492-02). Homologous recombination for fragment ligation with the plasmid was performed using Exnase Multis (Vazyme, C113-01). Total RNA was extracted with Trizol (Invitrogen), and the PrimeScript RT Master Mix (TAKARA, RR036A) was used for cDNA synthesis. NANODROP ONE (Thermo scientific) was used for measuring concentrations and purity testing. In Southern blot assay, restriction enzymes (HindIII, XbaI, and SpeI) used for genomic DNA digestion were from New England Biolabs (United States). For probe preparation, the PCR amplified fragment was purified using Cycle Pure Kit (Omega, D6492-02), and labeled with digoxin using DIG-High Prime DNA Labeling and Detection Starter Kit I (Roche, 11745832910). Probe hybridization was performed using a DIG Probe Synthesis Kit (Roche, 11636090910) and detected by DIG Nucleic Acid Detection Kit (Roche, 11175 041910).
Plant Infection and Fungal Biomass Assessment
Strains were grown in YePSL medium under shake culture conditions at 28°C for 12 h. The sporidia were harvested via centrifugation and resuspended in distilled water to a final OD600 of 1.0. Sporidial suspension (1 mL) was syringe-injected into the 3-week-old seedlings of the highly susceptible sugarcane cultivar ROC22 (Guangxi Province, China). Mock inoculations were carried out by injecting distilled water. The sugarcane stem tissue used for S. scitamineum biomass evaluation was collected at 3 days post inoculation (dpi), and quantification of relative fungal biomass in infected sugarcane stem tissue was performed using the fungal ACTIN gene as reference (Brefort et al., 2014). The sugarcane glyceraldehyde dehydrogenase (GAPDH) gene served as reference for normalization (Su et al., 2016). The black whip symptoms were evaluated for disease rating (Chang et al., 2018). Three biological repeats, each containing three technical replicas for each sample, were performed.
Quantitative Real-Time PCR
Quantitative reverse transcription polymerase chain reaction (qRT-PCR) was performed on a qTOWER3G (Analytik Jena) using the TB Green Premix Ex Tag (TAKARA, RR820A). The qRT-PCR was run with the following settings: 95°C/3 min – (95°C/10 s – 60°C/30 s –72°C/30 s) × 40 cycles and 72°C 10 s. Relative expression values were calculated with the 2–ΔΔCt method (Livak and Schmittgen, 2001), using ACTIN as internal control. Three biological repeats, each containing three technical replicas for each sample, were performed. Primers used in this study are listed in Supplementary Table S3.
Protoplast Production Assay
For protoplast generation assay the cultured S. scitamineum sporidia (OD600 ≈ 0.6) were harvested, and incubated with the lyzing solution (the lyzing enzyme, Sigma L1412, was dissolved in SCS solution comprised of 20 mM trisodium citrate and 1M D-sorbitol, at pH 5.8, to reach a concentration of 15 mg/mL) and incubated for 20 min (Chang et al., 2018). The lysis was stopped by placing the reaction tube on ice. The protoplasts production were examined by microscopy (OLYMPUS, CX21) and counted using a hemacytometer (Jeon et al., 2008). Three biological repeats, each containing three technical replicas for each sample, were performed.
Malondialdehyde (MDA) Content Measurement
Sporisorium scitamineum sporidia (OD600 ≈ 0.1) were harvested, and 150 μL of sporidial suspension was used for culturing on MM plates with or without 25 μg/mL SDS treatment for 48 h at 28°C before determination of MDA content. The sporidia samples were gently scraped from the plates and homogenized in an ice bath with 2.0 mL of 5% trichloroacetic acid solution (m/v), and then centrifuged at 10,000 rpm for 15 min at 4°C. Approximately 2.0 mL of the resulting supernatant was mixed with 1.0 mL of 5% trichloroacetic acid containing 0.67% thiobarbituric acid solution (m/v), and the container heated in boiling water for 5 min. The mixture was rapidly transferred to an ice bath, and measured for its absorbance at 532 nm using a spectrophotometer (METASH, UV5100B). Non-specific turbidity of the sample was corrected by subtracting its absorbance at 600 nm. The MDA concentration was calculated and expressed as μmol/g FW (Lanubile et al., 2015).
Sporidial Staining and Microscopy
Sporisorium scitamineum sporidia (OD600 ≈ 1.0) were harvested via centrifugation and washed twice, before further processing and staining. The sporidial suspension with or without SDS in full 200 μg/mL SDS treatment for 10 min was harvested and washed twice in distilled water, and subjected to staining with propidium iodide PI (Sigma, 25535-16-4), by incubating with 60 μg/mL PI solution (in DPBS, Dulbecco’s Phosphate-Buffered Saline: 2.67 mM KCl, 1.47 mM KH2PO4, 138.00 mM NaCl, and 8.10 mM Na2HPO4) for 15 min in the dark (Silva et al., 2005). The stained sporidia were washed twice and placed on a slide for examination under an epifluorescent microscope (OLYMPUS, BX53) using a DAPI filter. For Concanavalin A (ConA) staining, 100 μL of sporidial suspension was collected and stained in 100 μg/mL ConA type VI conjugated to FITC (Sigma, C7642) for 45 min as described (Li et al., 2014). The stained sporidia were washed twice and observed by epifluorescent microscopy using a GFP filter. For staining with the fluorescent brightener calcofluor white (CFW), 100 μL of sporidial suspension were collected and stained in 10 μg/mL fluorescent brightener (calcofluor white; Sigma, 18909) as described (Krombach et al., 2018), and examined by epifluorescent microscopy using a DAPI filter. Microscopic images were taken with a digital camera (OLYMPUS, DP80) equipped with the microscope.
Intercellular cAMP Extraction and Detection
Intracellular cAMP of S. scitamineum sporidia were extracted and detected by cAMP Enzyme Immunoassay Kit (Sigma, CA201), using the procedure previous reported (Chang et al., 2018).
Results
Identification of a Conserved Farnesyltransferase β Subunit Encoding Gene RAM1
A tBLASTx search with Neurospora crassa Ram1 protein (XM_953714.2:176-1807) as the query revealed the presence of one putative RAM1 gene in S. scitamineum. A phylogenetic analysis of the putative S. scitamineum Ram1 protein and previously characterized Ram1 proteins from other fungi, including smut fungi and ascomycetous fungi, indicated the presence of two distinct phylogenetic clades (Figure 1). S. scitamineum Ram1 was 87% identical to putative Sporisorium reilianum Ram1, 78% to U. maydis Ram1, 34% to C. neoformans Ram1, and 34% to A. fumigatus Ram1, respectively. RAM1 is predicted to encode a peptide of 606 amino acids, with two prenyltransferase domains and one squalene oxidase repeat (PF00432.16) at positions of 139–180 and 207–250 amino acid in S. scitamineum. The domain and amino acid sequences of Ram1 proteins were highly conserved among all smut fungi analyzed (Supplementary Figure S1A).
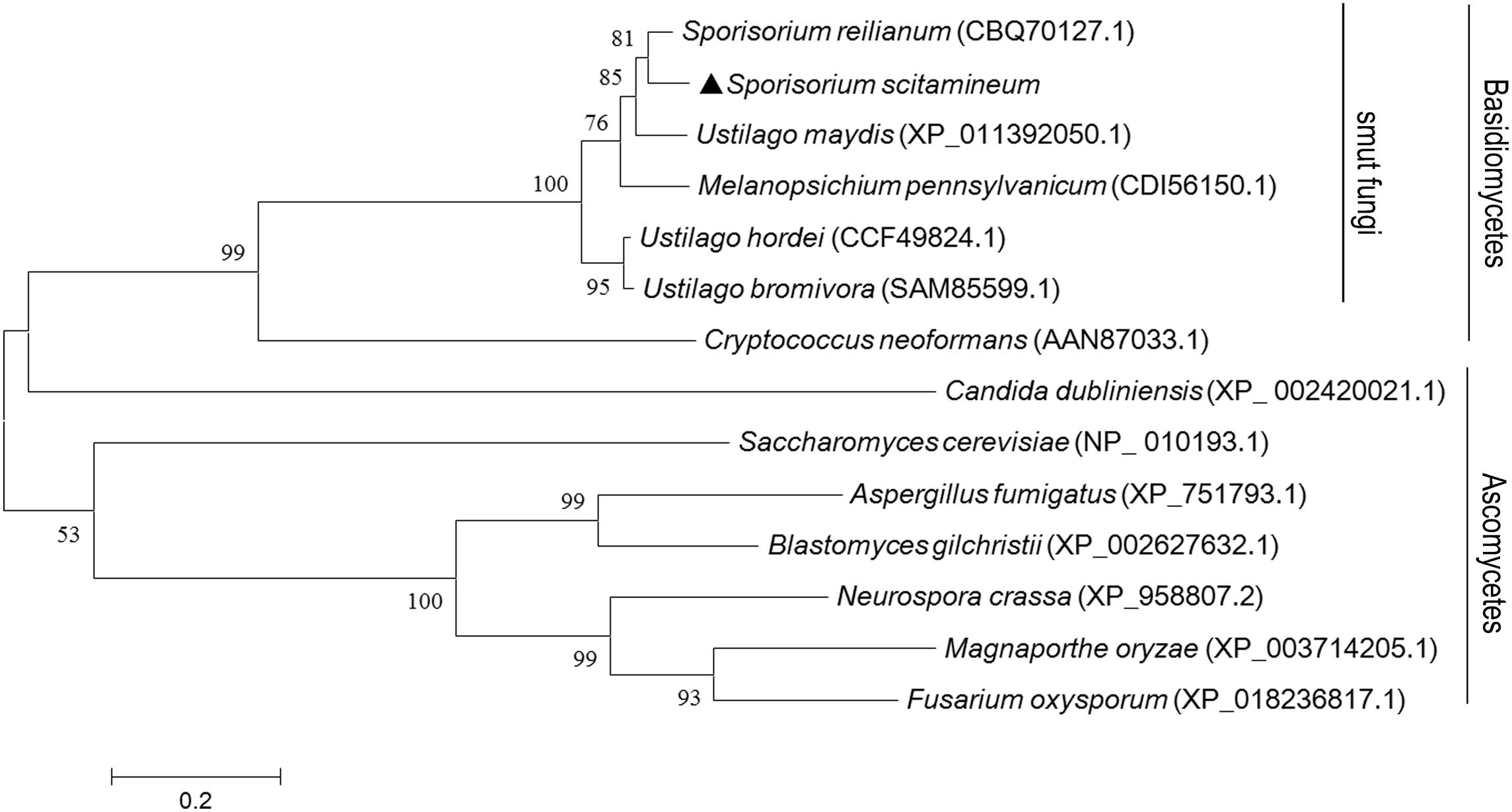
Figure 1. Phylogenetic analysis and amino acid sequences arrangement with Sporisorium scitamineum Ram1 protein and its orthologs. Phylogenetic analysis of fungal orthologous Ram1 proteins. Amino acid sequences were aligned with ClustalX 2.0 (Thompson et al., 1994) with the following parameters: pairwise alignment parameters (gap opening = 10, gap extension = 0.1) and multiple alignment parameters (gap opening = 10, gap extension = 0.2, transition weight = 0.5, delay divergent sequences = 25%). The alignment was phylogenetically analyzed with maximum likelihood by MEGA 6.0 (Kenaley et al., 2018), by using a Le-Gasquel amino acid replacement matrix with 1,000 bootstrap replications. S. scitamineum Ram1 was denoted by a solid triangle.
RAM1 Is Required for S. scitamineum Mating/Filamentation
To evaluate the impact of RAM1 on mating and growth, a ram1Δ mutant and its complementation strain RAM1c were generated. The number of insertion copy was verified by Southern blotting using HPT fragment as probe (Supplementary Figure S1B), and the replacement of RAM1 gene was also confirmed by Southern blotting using locus-specific probe (Supplementary Figure S1C). The re-introduction of RAM1 gene was verified by PCR amplification (Supplementary Figure S1D). For haploid vegetative growth, the ram1Δ colony surface appeared drier than that of the wild-type MAT-1 or RAM1c strain when grown on YePSA (Figure 2A: left panel), but the growth speed of sporidia was indistinguishable among these three strains when cultured in YePS liquid medium (Figure 2B). The mating of ram1Δ sporidia with compatible wild-type MAT-2 was obviously reduced, compared to that of wild-type or RAM1c strains (Figure 2A: right panel). Furthermore, we found that mixing of two ram1Δ of compatible mating types displayed a complete blockage of mating and filamentous growth/filamentation (Supplementary Figure S2A). qRT-PCR analysis was performed for assessing expression of genes related to fungal mating and filamentation in mutants and wild-type. The results showed that in ram1Δ sporidia, the a locus genes MFA1 and PRA1, and the b locus genes bE1 and bW1 were all comparable to that in the complementary or wild-type strains, while PRF1, the master transcriptional regulator for mating and filamentation, was significantly down-regulated (Supplementary Figure S2B). Overall these results showed that Ram1 is required for S. scitamineum mating and filamentation.
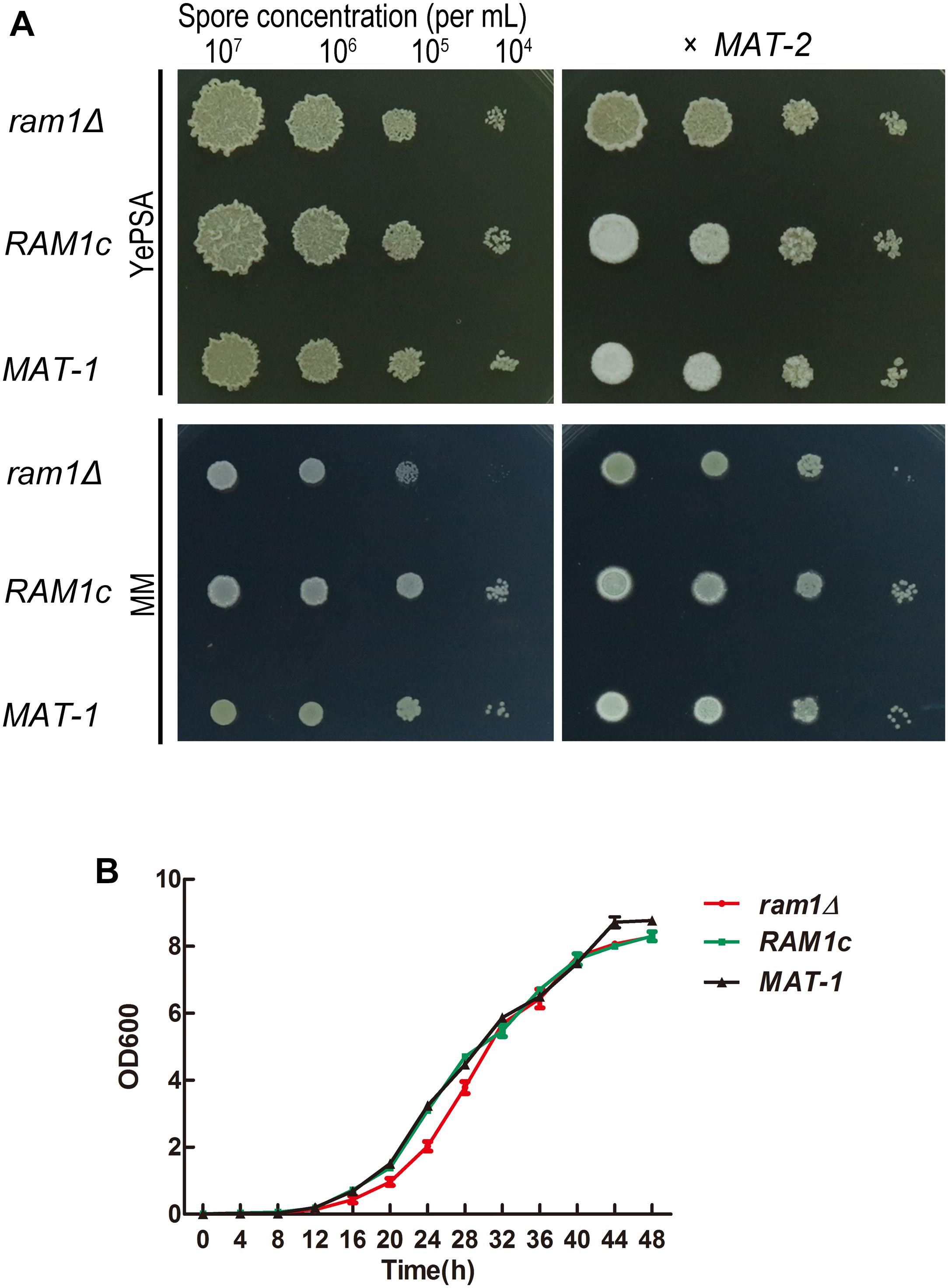
Figure 2. RAM1 is required for S. scitamineum sexual mating. (A) Haploid (left) and mating (right, x MAT-2) colony of ram1Δ, wild-type MAT-1 and the complementation strain RAM1c. A sporidial suspension (1 μL) of OD600 = 1.0, and the serial 10-fold dilutions of indicated strains, were spotted on YePSL or MM plates and incubated at 28°C for 24 h, before examination. (B) Saprophytic growth curve of ram1Δ, wild-type MAT-1 and the complementation strain RAM1c. For the growth curve, strains were cultured in YePSL over a period of 48 h. Three biological replicates, each containing three technical replicates for each sample, were performed.
Expression of RAM1 During Development of Sporidia to Hyphal in S. scitamineum
To determine the potential function of RAM1 at the haploid sporidia switch to dikaryotic hyphal growth, we assessed transcriptional profile of RAM1 gene every 12 h over a period of 72 h in haploid or mating condition using qRT-PCR. In wild-type sporidia, the expression of RAM1 was elevated with cultural time. The maximal expression level occurred at around 60 h, of more than 10-fold compared to that at 12 h. Then we examined the expression of RAM1 gene from 12 to 72 h in dikaryotic hyphal stage. The results showed that the expression of RAM1 was obviously reduced compared to that in sporidia stage (Figure 3A). We also found the pattern of pheromone precursor gene MFA1 was similar to that of RAM1 (Figure 3B), but the elevated expression initially started at 48 h and reached the maximum at around 60 h (Figures 3A,B).
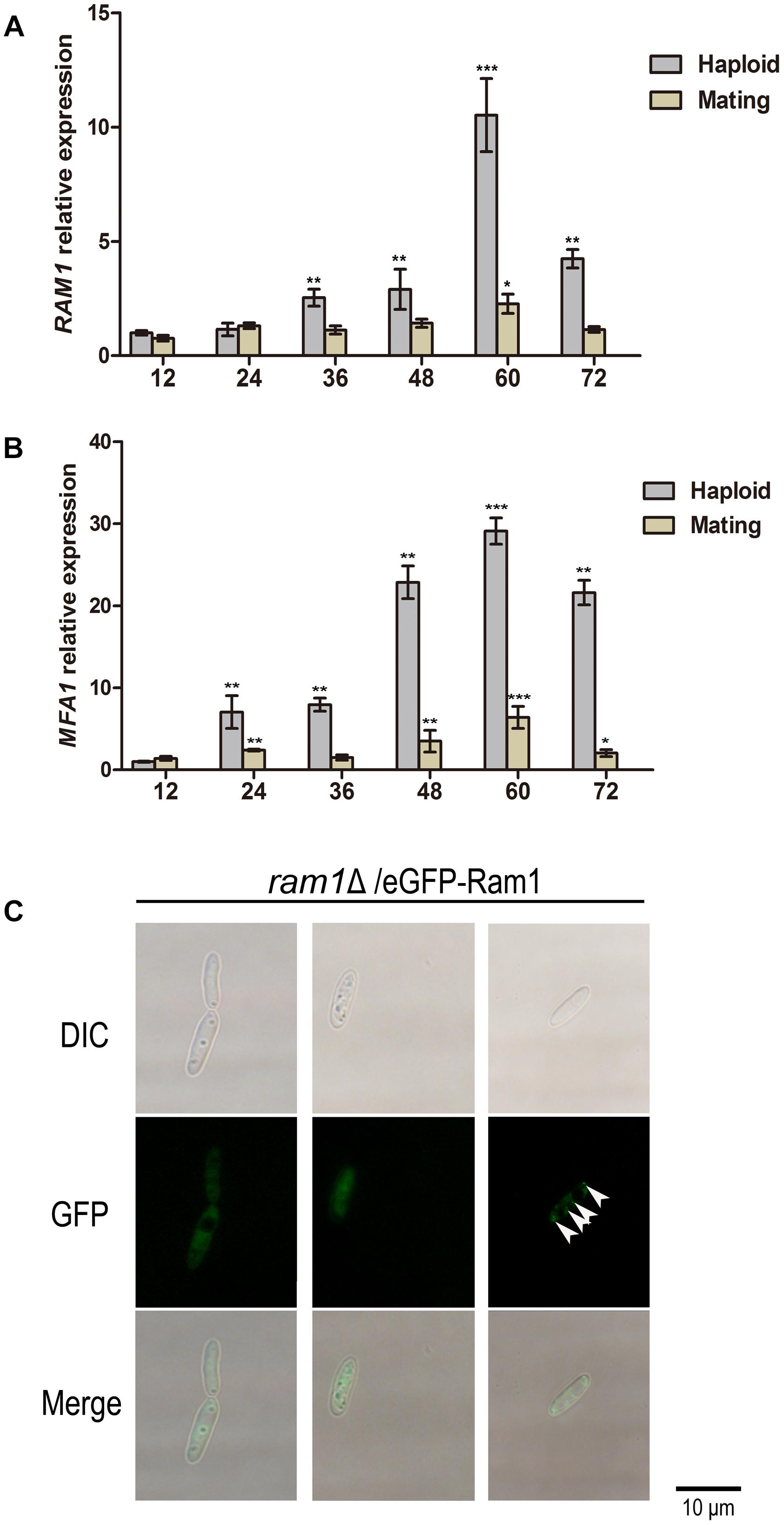
Figure 3. Transcriptional profile of RAM1 gene and MFA1 gene and subcellular localization of Ram1 protein in S. scitamineum. (A) qRT-PCR analysis of RAM1 expression in wild-type strain under sporidial growth or mating condition on YePSA plate. RAM1 expression in 12 h was set as 1 and the relative gene expression fold change in a period of 72 h was calculated with 2–ΔΔCt method (Livak and Schmittgen, 2001), using ACTIN as internal control. Statistical significance of the expression data was determined at ∗∗∗p < 0.005, **p < 0.01, and *p < 0.05 using Student’s t-test, and error bars represent ± standard deviation values. (B) qRT-PCR analysis of MFA1 expression in wild-type strain under sporidial growth or mating condition on YePSA plate. MFA1 expression in 12 h was set as 1 and the relative gene expression fold change in a period of 72 h was calculated with 2–ΔΔCt method (Livak and Schmittgen, 2001), using ACTIN as internal control. Statistical significance of the expression data was determined at ∗∗∗p < 0.005, **p < 0.01, and *p < 0.05 using Student’s t-test, and error bars represent ± standard deviation values. (C) Localization of eGFP-Ram1 fusion proteins in the ram1Δ sporidia. Sporidial suspension of OD600 = 1.0 were spotted on YePSA plates and incubated at 28°C for 24 h, before epifluorescent microscopic observation and imaging. Arrows denote punctate structure visualized by eGFP-Ram1. Scale bar = 10 μm.
To observe the subcellular localization of Ram1 protein, a copy of eGFP-RAM1 fusion fragment was transferred into the ram1Δ mutant. The mating ability was restored in the resulting ram1Δ/eGFP-Ram1 strain, indicating that the eGFP-Ram1 fusion protein is functional. By epifluorescent microscopy, we observed that the eGFP-Ram1 signal localized in the cytosol of sporidial cells (Figure 3C), with occasional punctate structure in the cytoplasm (Figure 3C, arrows). These data imply that RAM1 plays an important role in development of sporidia.
Mfa1 Farnesylation Could Partially Restore ram1Δ Mating/Filamentation Defect
The S. cerevisiae and U. maydis pheromone precursor Mfa1 undergoes C-terminal farnesylation before its maturation and functioning as a hormone in compatible sporidial recognition and induction of sexual mating (Ashby and Rine, 1995; Koppitz et al., 1996). Therefore we intended to investigate whether the mating/filamentation defect of the ram1Δ was due to failure of the Mfa1 precursor in farnesylation (Spellig et al., 1994; Ashby and Rine, 1995). In S. scitamineum, the MFA1 gene codes a 41-amino-acid pheromone precursor in MAT-1 (CAI59747.1), which contains a predicted farnesylation motif CTIA at its C-terminal. We generated mfa1Δ mutants and its complementation strains MFA1c with wild-type MAT-1 background. The mutants and complementation strains were verified by PCR and Southern blotting (Supplementary Figure S3). As expected the mating of the mfa1Δ mutant with compatible mating type MAT-2 was completely blocked when cultured on YePSA media, and significant reduced on MM plates, compared to that of wild-type or MFA1c strains (Figure 4A). To confirm that such mating/filamentation defect was caused by loss of functional (farnesylated) a-pheromone encoded by MFA1, we generated and supplied the synthetic Mfa1 peptide with or without C-terminal farnesylation to MM plates to reach a final concentration of 1 or 10 μg/mL, and tested its effect on restoring mating/filamentation of the mfa1Δ or ram1Δ mutant. We found that the farnesylated Mfa1 peptide could effectively restore mating/filamentation in the mfa1Δ mutant at the concentration of 10 μg/mL, and only partially restored that of the ram1Δ mutant (Figure 4B). In contrast, addition of the Mfa1 peptide without farnesylation failed to rescue the defective mating/filamentation in ram1Δ or mfa1Δ mutant (Figure 4B). Putting together, these results suggested that Ram1 is likely required for farnesylation of pheromone precursor Mfa1, which is essential for S. scitamineum mating/filamentation.
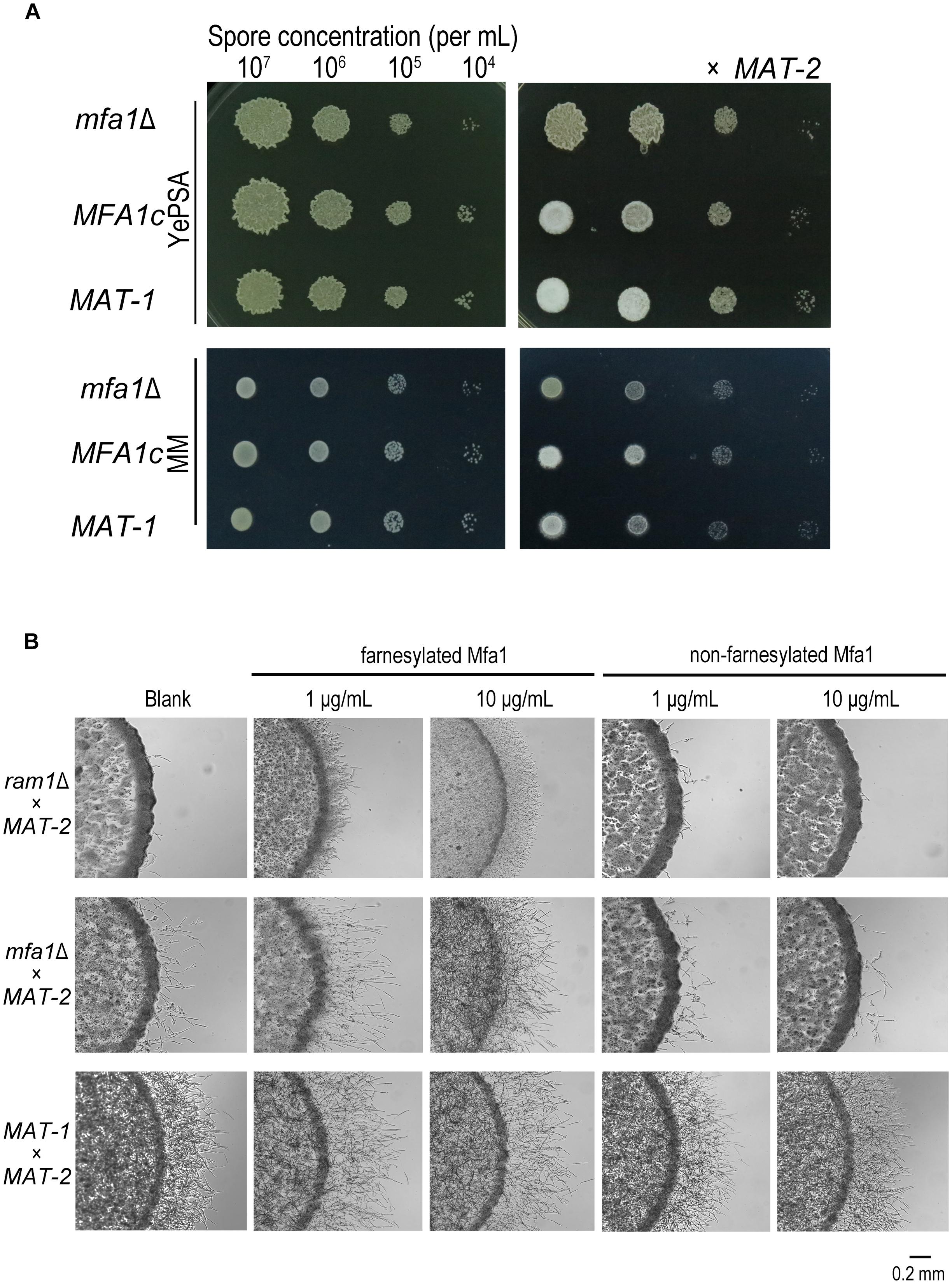
Figure 4. Mfa1 farnesylation could partially restore ram1Δ mating/filamentation defect in S. scitamineum. (A) Haploid (left) and mating (right, x MAT-2) colony of m1Δ and wild-type MAT-1. 1 μL sporidia suspension of OD600 = 1.0, and the serial 10-fold dilutions of indicated strains, were spotted on YePSA or MM plates and incubated at 28°C for 24 h. (B) Mating/filamentation assay with synthetic (farnesylated or non-farnesylated) Mfa1 exogenously supplied to MM plates to reach a final concentration of 1 μg/mL or 10 μg/mL. 1 μL sporidial suspension (of the mutants ram1Δ, mfa1Δ or wild-type strain) of OD600 = 1.0 were, respectively, mixed with compatible mating partner and cultured on MM plates at 28°C for 24 h.
RAM1 Is Required for Full Pathogenicity of S. scitamineum
To test whether the RAM1 is required for S. scitamineum pathogenicity, we inoculated the mixture of ram1Δ × MAT-2 sporidia by injection, into a susceptible sugarcane seedling, and evaluated the disease symptoms. Inoculation with the wild-type MAT-1 × MAT-2 or the RAM1c × MAT-2 combination served as positive control, and sterile water as mock. The ram1Δ × MAT-2 combination displayed significantly reduced pathogenicity, with only around 10% of the infected seedlings showing black whip symptoms at 200 dpi. In contrast, the wild-type MAT-1 and MAT-2 mixture lead to 70% of the infected seedlings with black whip symptoms, while that in the RAM1c and wild-type MAT-2 mixture was approximately 50% (Figure 5A). Consistently, relative fungal biomass was significantly lower at 3 dpi in seedling stems inoculated with ram1Δ × MAT-2, compared to that inoculated with the wild-type or complementary strain combinations (Figure 5B). These data suggest that Ram1 is also essential for full pathogenicity of S. scitamineum.
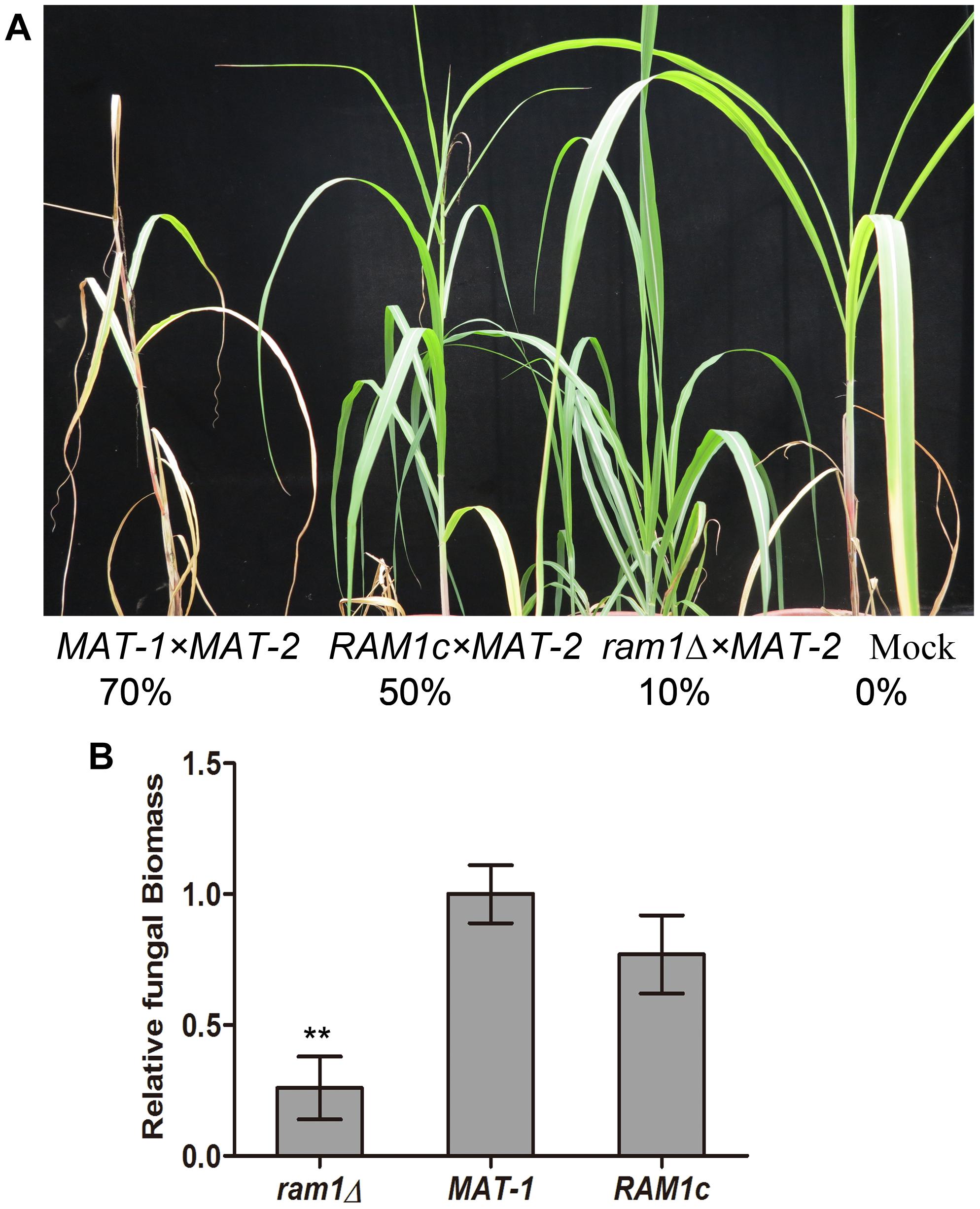
Figure 5. RAM1 is required for S. scitamineum pathogenicity. (A) The susceptible sugarcane cultivar ROC22 was inoculated with mixed sporidia (1:1) of the indicated wild-type or mutant combination through injection at the 5–6-leaf seedling stage, and the symptoms of ‘black whip’ were assessed and documented at 200 days post inoculation (dpi). The infection assays were performed with at least 20 seedlings. Percentage of plants with ‘black whip’ symptoms is illustrated. (B) Colonization of the plant tissue by ram1Δ, wild-type MAT-1, or the complementation strain RAM1c. Relative fungal biomass was determined by qRT-PCR with S. scitamineum genomic DNA isolated from infected sugarcane stems at 3 dpi. The fungal ACTIN gene was used for estimation of relative fungal biomass through 2–ΔΔCt method (Livak and Schmittgen, 2001), normalized to the plant GADPH gene. Barchart depicts the average ± standard error (SE) derived from three biological replicates. Significance of differences between wild-type MAT-1 and ram1Δ was calculated using Student’s t-test, **p < 0.01.
RAS Genes Are Required for S. scitamineum Mating and Filamentation
It has been reported that Ram1/Ram2 FTase complex controls fungal differentiation and/or pathogenicity via post-translational modifications of Ras proteins, the small G proteins playing an important role in cell signaling (Omer and Gibbs, 1994). Therefore we wondered whether defects mating and filamentation defects in the ram1Δ were related to Ras protein modification and the Ras-mediated signaling pathway. A BLASTP search with U. maydis Ras1 protein (XP_011386972.1) as the query revealed the presence of one putative RAS1 gene in S. scitamineum, which is predicted to encode a protein of 215 amino acids, with one RAS superfamily domain (PF00071) from amino acids 11–172. A BLASTP search with U. maydis Ras2 (XP_011387629.1) as the query revealed the presence of one putative RAS2 gene in S. scitamineum, encoding a protein of 192 amino acids, with one RAS superfamily (PF00071) from amino acids 5–173. S. scitamineum Ras1 and Ras2 shared 58% identity from Ras1 amino acids 10–171 covering the entire RAS domain, which indicated the conservation of Ras proteins at the amino acid sequence level. However, this indicated the other portions outside of the RAS domain of the Ras1 and Ras2 proteins probably serve other functions. A phylogenetic analysis of S. scitamineum Ras1 and Ras2 proteins with predicted or characterized Ras proteins from other basidiomycetous or ascomycetous fungi indicates that Ras1 and Ras2 proteins are divergent with each other, but is respective well conserved within its own group (Supplementary Figure S4).
To further investigate the function of S. scitamineum RAS genes, we generated ras1Δ and ras2Δ mutants, and their complementation strains RAS1c, RAS2c, both with wild-type MAT-1 background, and verified by Southern blotting and PCR (Supplementary Figures S5, S6). The ras1Δ haploid colonies were obviously smaller and wetter than that of the wild-type MAT-1 strain (Figure 6A, left panel). The compatibility of ras1Δ with MAT-2 sporidia was obviously reduced compared to that of wild-type MAT-1 and the RAS1 complementation strain, which displayed normal filament formation after mating (Figures 6A right panel, 6B). The ras2Δ haploid colonies were also obviously smaller and wetter than that of the wild-type MAT-1 strain (Figure 6A, left panel). However, the mating of ras2Δ with MAT-2 sporidia was only mildly reduced, and the mating of complementation strains was normal (Figures 6A right panel, 6B). Exogenous applications of cAMP (concentrations from 2.5 to 5 mM) could enhance (but not fully restore) sexual mating of mutants of ras1Δ or ras2Δ but not the one of ram1Δ and mfa1Δ (Figure 6C). This result indicates that cAMP signaling may be at downstream of Ras proteins, but not sufficient for fully restore Ram1 or Mfa1 function. Exogenous applications of synthetic (farnesylated) Mfa1 (concentrations 1 or 10 μg/mL) only or together with 5 mM cAMP couldn’t restore sexual mating of mutants of ram1Δ, ras1Δ, or ras2Δ except for mfa1Δ, synthetic (non-farnesylated) Mfa1 couldn’t restore mating/filamentation in these four mutants (Figure 6C). The phenotype of mating defect in ras1Δ or ras2Δ mutants were slighter than that in ram1Δ imply function of Ras1/Ras2 proteins are probably redundant in regulating mating/filamentation in S. scitamineum.
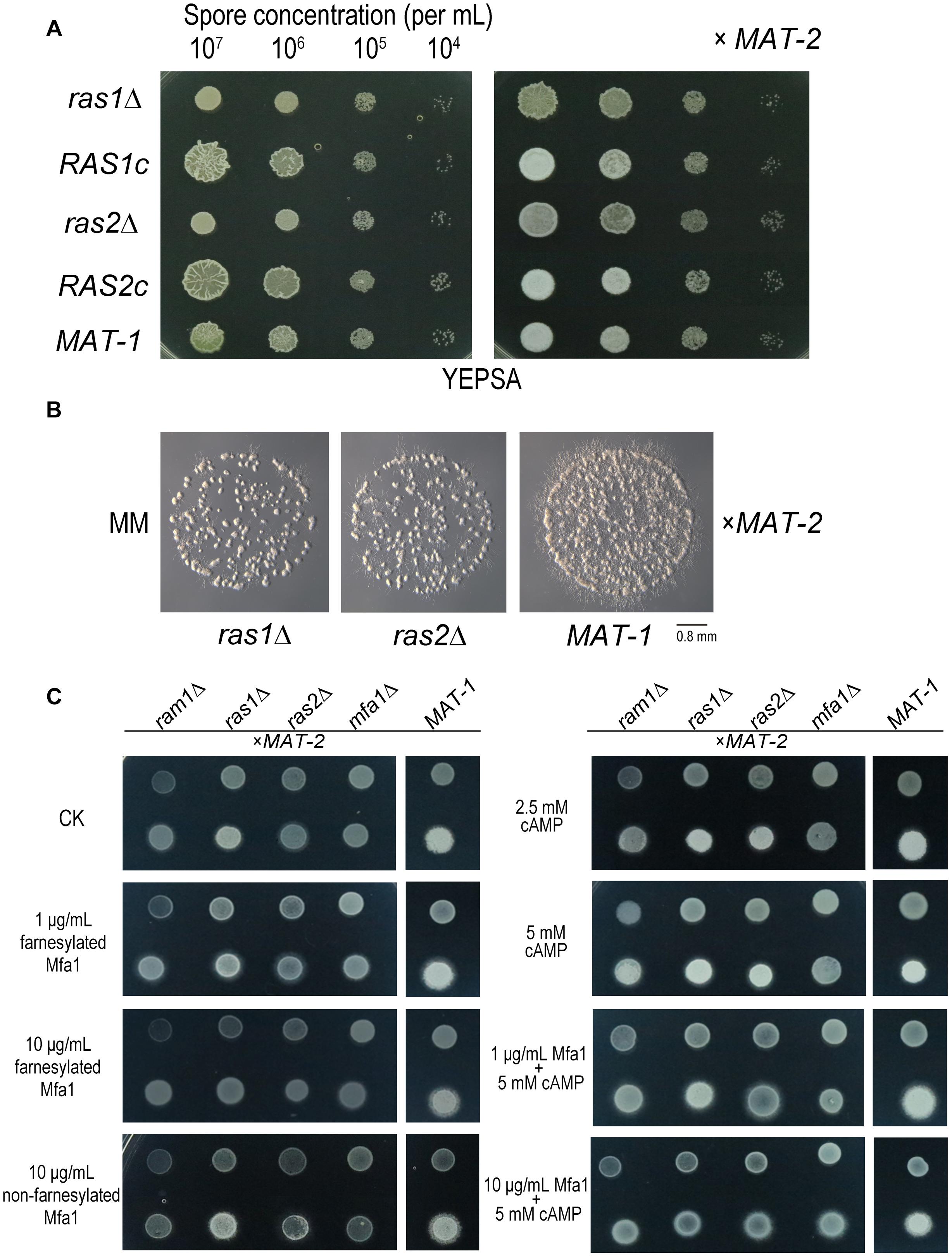
Figure 6. RAS1 and RAS2 are required for S. scitamineum mating/filamentation. (A) Haploid (left) or mating (right, x MAT-2) colonies of ras1Δ, RAS1c, ras2Δ, RAS2c, and wild-type MAT-1. One microliter sporidial suspension of OD600 = 1.0, and serial 10-fold dilutions, of indicated strains, were spotted on YePSA plates and incubated at 28°C for 24 h. (B) Microscopic observation of mating colonies of ras1Δ, ras2Δ, and wild-type MAT-1 on MM plates with 1 μL sporidial suspension (OD600 = 0.1) and incubated for 12 h at 28°C. Scale bar = 0.8 mm. (C) Haploid (upper) and mating (lower, x MAT-2) colony of ras1Δ, ras2Δ, ram1Δ, and mfa1Δor wild-type MAT-1 on MM plates with 1 μL OD600 = 1.0 sporidia suspension with exogenous cAMP of 0, 2.5, or 5 mM, synthetic (farnesylated) Mfa1 exogenously supplied to MM plates to reach a final concentration of 1 or 10 μg/mL, synthetic (non-farnesylated) Mfa1 exogenously supplied to MM plates to reach a final concentration of 10 μg/mL at 28°C for 24 h.
Ram1 and Ras Signaling Are Involved in S. scitamineum Cell Wall Integrity
Next we evaluated the stress tolerance of the ram1Δ, ras1Δ, ras2Δ, and mfa1Δ mutants, toward 20 μg/mL Congo red (cell wall stress), 20 μg/mL SDS (membrane stress), 25 μg/mL CFW (cell wall stress), 1 M Sorbitol (osmotic stress) and 0.5 mM H2O2 (oxidative stress). The mutants ram1Δ, ras1Δ, and ras2Δ displayed greater growth inhibition by treatment with SDS (20 μg/mL) or H2O2 (0.5 mM) than the wild-type MAT-1 strain, and the tolerance abilities were restored in the complementation strains (Supplementary Figures S7–S9). Both ras1Δ and ras2Δ mutants were hypersensitive to CR (20 μg/mL) while ram1Δ mutant was not hypersensitive (Supplementary Figure S7). With respect to osmotic stress sensitivity against sorbitol (1 M), all the mutant strains were indistinguishable from the wild-type strain (Supplementary Figure S7). On the other hand, the mfa1Δ mutant displayed no obvious difference in tolerance toward all these testing stressors, compared to that of WT (Supplementary Figure S7).
Given that the ram1Δ mutant was hypersensitive toward SDS, we intended to measure malondialdehyde (MDA) content of S. scitamineum sporidia, which indicates the degree of cell membrane damage (Li et al., 1998). The results showed that the ram1Δ sporidia contained notably higher MDA content than that of the wild-type MAT-1 or the complementation strain after SDS treatment (Figure 7A), which may account for reduced tolerance to SDS in the ram1Δ. It has been reported that SDS strongly increases solubilization of membrane lipid, leading to lipid peroxidation and cell membrane damage (He et al., 2005). MDA is the final product, and also an important indicator, of lipid oxidation (Lanubile et al., 2015), hence it is expected that the increased MDA content indicates cell membrane damage caused by SDS treatment. Correspondingly, the ram1Δ sporidia displayed notable propidium iodide (PI) positive staining compared to that of the wild-type or the complementation strains, after treatment with 200 μg/mL SDS for 10 min (Figure 7B), suggesting that the cell membrane of ram1Δ sporidia was weaker than that of the wild-type or the complementation strains.
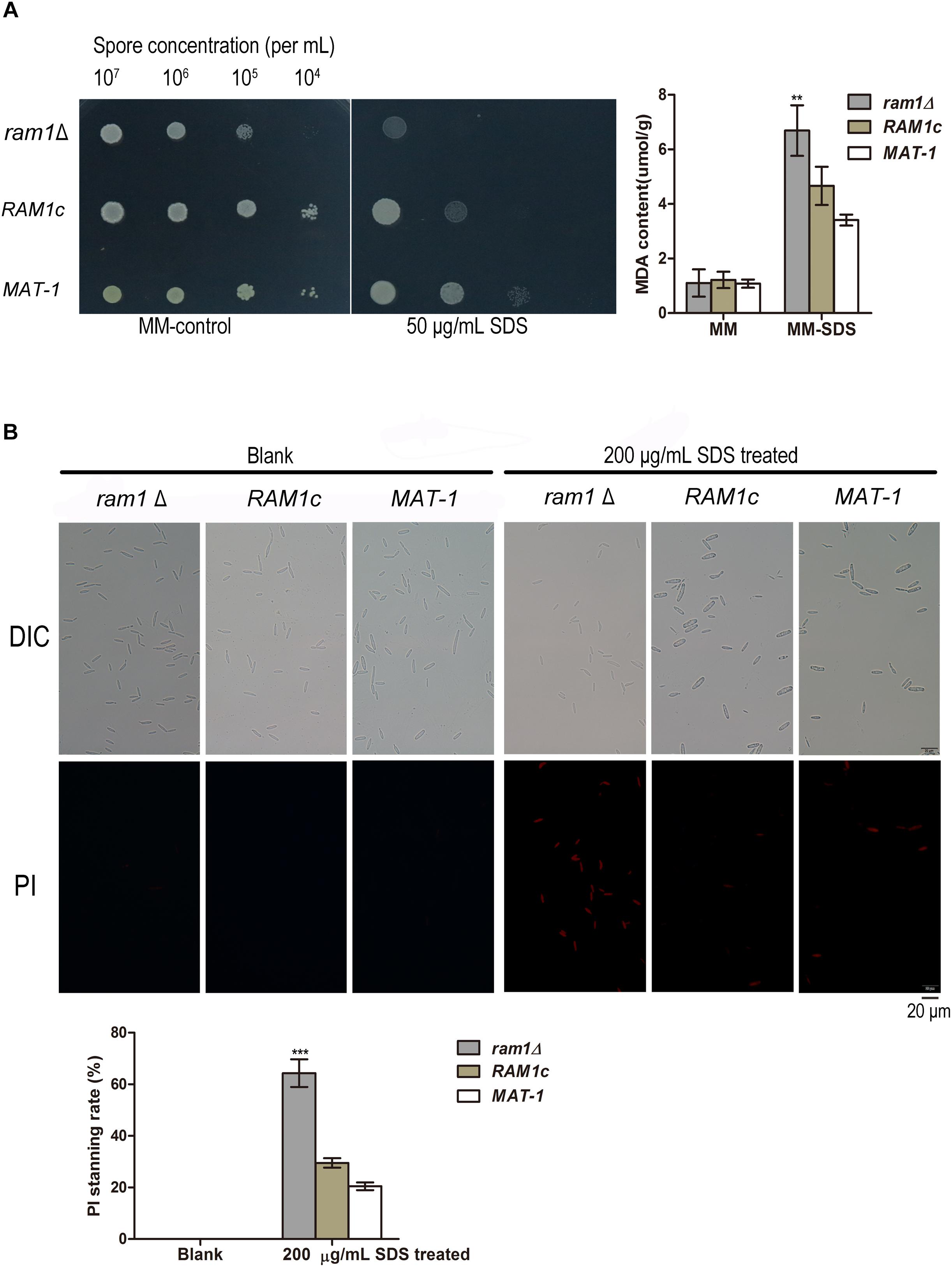
Figure 7. RAM1 is required for membrane stability of S. scitamineum sporidia. (A) Malondialdehyde (MDA) content of ram1Δ, wild-type MAT-1 and the complementation strain RAM1c with or without treatment of 50 μg/mL SDS. Barchart on the right panel depicts the average ± standard error (SE) derived from three independent biological replicates. Significance of differences between wild-type MAT-1 and ram1 was calculated by Student’s t-test, **p < 0.01. (B) Microscopic examination (upper panel) of ram1Δ, wild-type MAT-1 and the complementation strain RAM1c with Propidium iodide (PI) staining after 200 μg/mL SDS treatment for 10 min. PI staining was assessed after 15 min of staining. Scale bar = 20 mm. Barchart on the lower panel depicts the average ± standard error (SE) derived from three independent replicates. Significance of differences between wild-type MAT-1 and ram1Δ was calculated using a Student’s t-test, ***p < 0.005.
On MM media supplemented with 20 μg/mL Congo red (CR), the ram1Δ mutant displayed no obvious difference in growth compared to the wild-type strain (Supplementary Figure S7). We further verified the inhibition effect of CR by colony counting from the sporidial suspension on the MM plates with or without 50 μg/mL CR. Growth inhibition of ram1Δ mutant on CR-supplemented MM plate was 71.94% while that of wild-type or the complementation strain was 98%, of that on the MM plate without CR (Figure 8A). This indicates that the ram1Δ mutant was more tolerant toward CR compared to the wild-type or the complementation strains. To test cell wall integrity, an equal amount of sporidia from the ram1Δ mutant, the RAM1c and wild-type MAT-1 strains were treated with 15 mg/mL cell wall lyzing enzyme at 28°C for 20 min. The percentage of protoplasts generated was viewed as an indicator of cell wall integrity. The result showed that the RAM1c and wild-type MAT-1 sporidia were hypersensitive to the enzyme treatment, producing more protoplasts than the ram1Δ mutant (Figure 8B). This also suggested that the cell wall integrity was enhanced in the ram1Δ mutant, and thus it was more tolerant to CR. Furthermore, we assessed the expression of the genes involved in the cell wall integrity (CWI) pathway by qRT-PCR, in the ram1Δ mutant in comparison of the wild-type MAT-1, with or without treatment of 25 μg/mL CR. Our results showed that MID2 and WSC1 (membrane proteins as main sensors) (Philip and Levin, 2001), ROM2 (GEF which sensors interacted with) (Ozaki et al., 1996), RHO1 (small GTPase) (Schmelzle et al., 2002), PKC1 (protein kinase c) (Paravicini and Friedli, 1996), BCK1 (MAPKKK) (Lee and Levin, 1992), MKK1 (MAPKK) (Kim et al., 2008), SLT2 (MAPK) (Rui and Hahn, 2007), RLM1 (downstream transcription factors) (Jung et al., 2002), FKS3 and SMI1 (glycan synthase) (Lesage and Bussey, 2006) were all significantly up-regulated in the ram1Δ mutant treated with CR, compared to wild-type MAT-1 sporidia (Figure 8C). This result indicated that the RAM1 gene may regulate CWI via transcriptional regulation of the genes involved in this signaling pathway.
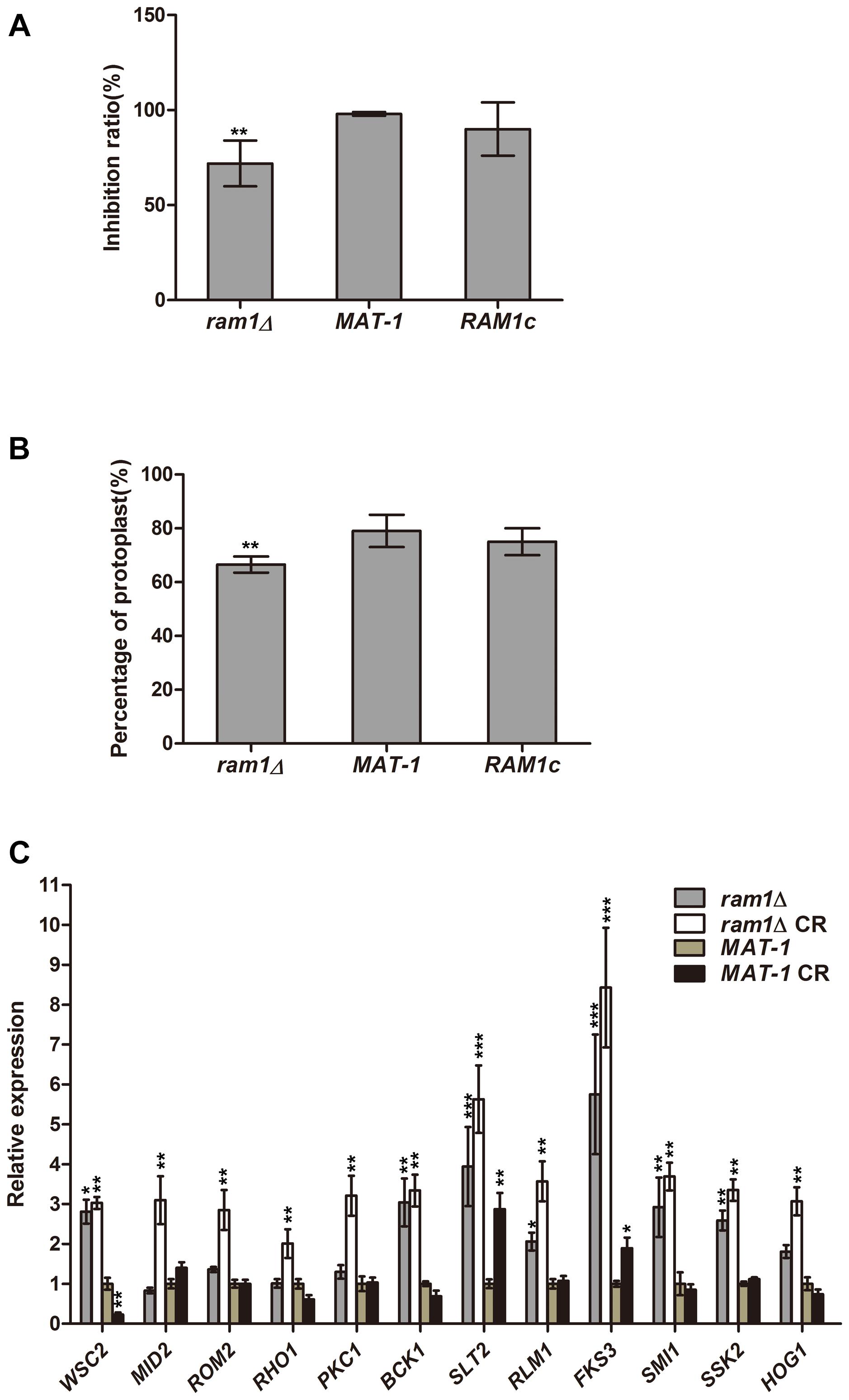
Figure 8. RAM1 regulates S. scitamineum cell wall integrity. (A) Growth inhibition calculated by number of colonies formed following streaking of ram1Δ, wild-type MAT-1 and the complementation strain RAM1c sporidial suspension (OD = 10–5; 150 mL), on MM plates with or without 50 μg/mL Congo red (CR). Statistical significance of the inhibition was determined at **p < 0.01 using Student’s t-test, and mean ± standard deviation was derived from three independent biological replicates. (B) Enzymatic digestion and protoplast production assay with ram1Δ, wild-type MAT-1 and the complementation strain RAM1c. Protoplast production was counted at 20 min post lyzing enzyme incubation. Statistical significance of the protoplast production was determined at **p < 0.01 using Student’s t-test, and mean ± standard deviation was derived from three independent biological replicates. (C) qRT-PCR analysis with genes involved in cell wall integrity pathway in ram1Δ and wild-type MAT-1, with or without treatment of 25 μg/mL CR. Gene expression in the wild-type MAT-1 strain (untreated) was set as 1 and the relative gene expression fold change in other strains/conditions was calculated with 2–ΔΔCt method (Livak and Schmittgen, 2001), using ACTIN as internal control. Barchart depicts the average ± standard error (SE) derived from three independent biological replicates. Statistical significance of the expression data was determined at ***p < 0.005, **p < 0.01, *p < 0.05 using Student’s t-test, and error bars represent ± standard deviation values.
On the other hand, the ras1Δ and ras2Δ mutants displayed hypersensitivity to cell wall stressors CR, CFW, and SDS, and the tolerance abilities were restored in the complementation strains (Supplementary Figures S7, S9). We further investigated whether Ras1 and/or Ras2 affect the cell wall integrity by conA and CFW staining. ConA is lectin specifically binding to internal and non-reducing terminal α-d-mannosyl groups and thus is routinely used to assay the presence of mannan moieties in fungal cell walls (Maddi et al., 2012). Our results showed that the ras1Δ mutant exhibited weaker fluorescence staining by conA, indicating reduced conA binding sites, while the ras2Δ mutant displayed indistinguishable conA staining compared to that of the wild-type (Figure 9A). For CFW staining, the ras1Δ mutant exhibited a significantly brighter staining pattern than the wild-type, and the ras2Δ mutant was slightly brighter than wild-type MAT-1 (Figure 9A). This result implied that the cell wall permeability toward CFW dye was enhanced in the ras1Δ and the ras2Δ mutants, but not in wild-type.
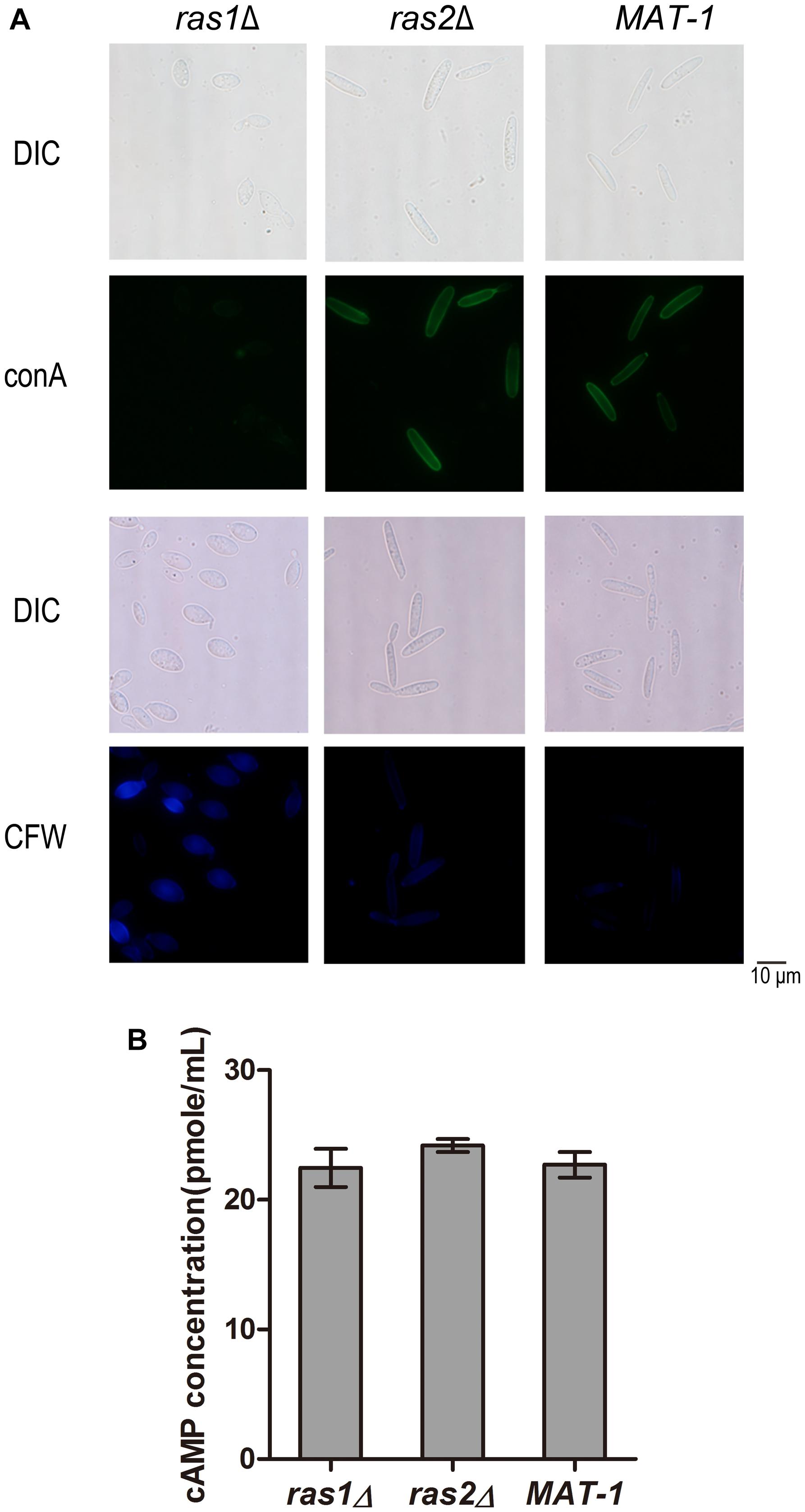
Figure 9. RAS1 and RAS2 regulates S. scitamineum cell wall integrity. (A) Microscopic examination of ras1Δ, ras2Δ, and wild-type MAT-1 stained with 100 μg/mL Concanavalin A (conA, type VI conjugated to FITC), or with 10 μg/mL fluorescent brightener calcofluor white (CFW). Scale bar = 10 mm. (B) Measurement of intracellular cAMP in 2-day-old sporidia of strains ras1Δ, ras2Δ and wild-type MAT-1, cultured on YePSA plates at 28°C for 24 h. The cAMP concentration was calculated and expressed as pmol/mL. Barchart depicts the average ± standard error (SE) derived from three independent replicates. Statistical analysis was performed by Student’s t-test.
In U. maydis, both Ras1 and Ras2 regulate intercellular cAMP level (Nadal et al., 2008; Zhu et al., 2009). In C. neoformans, intercellular cAMP level regulates the cell wall integrity pathway (Donlin et al., 2014). To furthermore evaluate the role of cAMP in cell wall integrity we measured the intercellular cAMP concentration in the ras1Δ and ras2Δ mutants in comparison with wild-type. The results showed no obvious differences in the intercellular cAMP concentrations between the ras1Δ and the ras2Δ mutants and the wild-type strain (Figure 9B). We proposed that Ras1/Ras2-regulating CWI pathway is likely independent of cAMP/PKA signaling pathway.
Overall, in this section we found that Ram1 may negatively, and Ras proteins positively, regulate CWI pathway in S. scitamineum. The a-factor Mfa1 may not be involved in S. scitamineum CWI regulation.
Discussion
The predominant function of FTase composed of Ram1 and Ram2 is conserved among eukaryotes (Omer and Gibbs, 1994). In our study, the ram1Δ mutant is viable, whereas the ram2Δ mutants could not be generated although repeated attempts were made, which is consistent with what has been reported in S. cerevisiae, C. neoformans, and C. albicans that the RAM2 gene is essential while RAM1 is not (Alspaugh et al., 2000; Song and White, 2003; He et al., 2005). It remains a possibility that some suppressors can directly or indirectly enhance cross-prenylation of certain CAAX substrates to replace the function of Ram1 but not Ram2. S. cerevisiae Ram1 is associated to sexual mating and survival in stressful conditions including high temperature, but is not essential for haploid growth (Michaelis and Barrowman, 2012). However, C. neoformans Ram1 is involved in diploid growth (Nadal et al., 2008). In A. fumigatus, ramAΔ mutants resulted in growth abnormalities including impaired hyphal branching, delayed conidial germination, reduced conidial viability, and aberrant distribution of nuclei in growing hyphae (Fortwendel et al., 2004). In this study, we found that the virulence of the S. scitamineum ram1Δ mutant (mixed with compatible wild-type sporidia) was significantly reduced compared to the wild-type strain, possibly resulting from reduced mating/filamentation and/or weakening stress resistance. For the Ram1 subcellular localization, we generated an eGFP-Ram1 strain and found it localized in the cytosol of sporidial cells. We made attempts to use the native promoter fused with eGFP-Ram1, but we found such ectopic expression of RAM1 was less than 20% compared to wild-type. Relative expression of RAM1 driven by G3PD promoter in ram1Δ/eGFP-Ram1 strain was about 70% compared to that of wild-type and the mating ability was restored (data not shown). Therefore, we believe in the localization of Ram1 protein was original not GFP-artifactual. S. scitamineum ram1Δ mutant showed notably down-regulation of the PRF1 gene, encoding a master transcription factor governing mating and filamentation, which may account for its defect in mating/filamentation. However, we did not find down-regulation of the a or b locus genes, which were shown to be transcriptionally induced by Prf1 in U. maydis mating/filamentation (Hartmann et al., 1999). We infer that S. scitamineum may possess redundant (un-identified) regulator of the a or b locus genes’ transcription, which may not depend on Ram1 function.
In S. cerevisiae, a-factor precursor (with CVIA C-terminal motif), Ras1 (with CIIC C-terminal motif), Ras2 (with CIIS C-terminal motif), G protein gamma subunit Ste18 (with CTLM C-terminal motif) are farnesylated by the FTase composed of Ram1 and Ram2 (Omer and Gibbs, 1994; Flom et al., 2008; Michaelis and Barrowman, 2012). Currently, fungal Ras1 and Ras2 are known to be involved in regulating not only growth, development and conidiation, but also virulence and tolerance to oxidation, cell wall disturbance, or heat sensitivity in either independent or interactive manners (Mosch et al., 1996; Lee and Kronstad, 2002; Bluhm et al., 2007; Xie et al., 2013). Disruption of fungal Ras2 has been previously reported in several fungi and also in this study, but deletion of RAS1 gene was only reported in haploid pathogenic fungus A. fumigatus (Fortwendel et al., 2004; Boyce et al., 2005). It was reported that in U. maydis or C. albicans, both Ras1 and Ras2 regulate intracellular cAMP level, with Ras1 as cAMP-dependent, and Ras2 as MAPK-dependent (Nadal et al., 2008; Zhu et al., 2009). In this study, we successfully deleted RAS1 gene in S. scitamineum. Both S. scitamineum ras1Δ and ras2Δ mutants showed defective sexual mating which could enhance but not restore obviously by exogenous cAMP. To evaluate whether the concentration of intercellular cAMP of ras1Δ and ras2Δ mutants changed, ELISA method was conducted and the results showed no obvious differences in the intercellular cAMP concentrations between the ras1Δ and the ras2Δ mutants and the wild-type strain. Similarly, in S. pombe and Schizophyllum commune, deletion of RAS1 couldn’t reduce the cAMP; in Fusarium graminearum, the intercellular cAMP level was not affected by deletion of RAS2 (Hughes et al., 1993; Yamagishi et al., 2004; Bluhm et al., 2007). On the other hand, we found that exogenous addition of synthetic (farnesylated) Mfa1 peptide could effectively restore mating/filamentation in the S. scitamineum mfa1Δ mutant, and partially restore that in the ram1Δ ras1Δ and ras2Δ mutants; synthetic (non-farnesylated) Mfa1 couldn’t restore mating/filamentation in these four mutants. We infer that both Mfa1 farnesylation and Ras-mediated signaling pathway are critical for S. scitamineum mating/filamentation, and may both depend on Ram1 function. It would be worth generating and assessing mislocalization of Ras and Mfa1 proteins by comparing their phenotype with the ram1Δ mutant in the future.
Fungal cell wall allows exchange of the compounds and signals between environment and fungal cells, or to build parasitical structures. Cell wall integrity (CWI) signal pathway is highly conserved in eukaryotic organisms, and is involved in stress tolerance and fungal pathogenicity (Hamel et al., 2012). We found that the ram1Δ sporidia were more tolerant to cell wall disrupting agent Congo red (CR), and CR treatment could induce a significantly higher up-regulation of several conserved CWI genes, especially Slt2 (MAPK) and Fks3 (glucan synthase), compared to that of wild-type sporidia. These results together demonstrate that Ram1 likely regulates the cell wall integrity in S. scitamineum.
In S. scitamineum, both ras1Δ and ras2Δ mutants were hypersensitive toward cell wall disrupt agents and cell membrane disrupt agents. In C. neoformans, intracellular cAMP level regulates the cell wall integrity pathway (Donlin et al., 2014), however, we found no obvious difference in terms of intracellular cAMP concentration of S. scitamineum ras1Δ or ras2Δ mutant compared to that of the wild-type strain. Sensitivity toward cell wall stressor Congo red was opposite in the ras1Δ or ras2Δ sporidia (hypersensitive) compared to that of ram1Δ mutant (tolerant). Both ras1Δ and ras2Δ were hypersensitive toward cell wall stressors, which supported the Ras involved in cell wall integrity pathway. But CFW dye on ras2Δ has almost no intensity but ras1Δ has strong intensity. From our unpublished data, three kinase genes involving in cell wall integrity pathway deletion mutants had slightly intensity by CFW dye, as the ras2Δ showed. We speculate the Ras1 and Ras2 involve in CWI pathway but have different compensation mechanism for cell wall composition. Overall, these results suggested that Ras may regulate S. scitamineum cell wall integrity through a signaling pathway independent or parallel of the cAMP/PKA pathway.
In summary, our study revealed a possible regulatory function of Ram1 by farnesylation of a-factor and/or Ras proteins, which are both critical for S. scitamineum mating/filamentation and/or stress tolerance.
Data Availability
All datasets generated for this study are included in the manuscript and/or the Supplementary Files.
Author Contributions
YD, BC, CC, and ZJ conceived and designed the experiments. SS, CC, EC, MY, and LL performed the experiments. YD, SS, CC, and ZJ analyzed the data and wrote the manuscript.
Funding
This research was supported by the National 973 Program of China (2015CB150600) and National Natural Science Foundation of China (31672091).
Conflict of Interest Statement
The authors declare that the research was conducted in the absence of any commercial or financial relationships that could be construed as a potential conflict of interest.
Acknowledgments
We are grateful for the critical reading and helpful comments on our manuscript by Prof. Tom Hsiang (University of Guelph).
Supplementary Material
The Supplementary Material for this article can be found online at: https://www.frontiersin.org/articles/10.3389/fmicb.2019.00976/full#supplementary-material
References
Alspaugh, J. A., Cavallo, L. M., Perfect, J. R., and Heitman, J. (2000). RAS1 regulates filamentation, mating and growth at high temperature of Cryptococcus neoformans. Mol. Microbiol. 36, 352–365. doi: 10.1046/j.1365-2958.2000.01852.x
Ashby, M. N., and Rine, J. (1995). Ras and a-factor converting enzyme. Methods Enzymol. 250, 235–251. doi: 10.1016/0076-6879(95)50076-50076
Bluhm, B. H., Zhao, X., Flaherty, J. E., Xu, J. R., and Dunkle, L. D. (2007). RAS2 regulates growth and pathogenesis in Fusarium graminearum. Mol. Plant-Microbe Interact. 20, 627–636. doi: 10.1094/MPMI-20-6-0627
Boyce, K. J., Hynes, M. J., and Andrianopoulos, A. (2005). The Ras and Rho GTPases genetically interact to co-ordinately regulate cell polarity during development in Penicillium marneffei. Mol. Microbiol. 55, 1487–1501. doi: 10.1111/j.1365-2958.2005.04485.x
Brefort, T., Tanaka, S., Neidig, N., Doehlemann, G., Vincon, V., and Kahmann, R. (2014). Characterization of the largest effector gene cluster of Ustilago maydis. PLoS Pathog. 10:e1003866. doi: 10.1371/journal.ppat.1003866
Brown, A. J., and Casselton, L. A. (2001). Mating in mushrooms: increasing the chances but prolonging the affair. Trends Genet. 17, 393–400. doi: 10.1016/S0168-9525(01)02343-2345
Chakraborty, B. N., Patterson, N. A., and Kapoor, M. (1991). An electroporation-based system for high-efficiency transformation of germinated conidia of filamentous fungi. Can. J. Microbiol. 37, 858–863. doi: 10.1139/m91-147
Chang, C., Cai, E., Deng, Y. Z., Mei, D., Qiu, S., Chen, B., et al. (2018). cAMP/PKA signaling pathway regulates redox homeostasis essential for Sporisorium scitamineum mating/filamentation and virulence. Environ. Microbiol. 21, 959–971. doi: 10.1111/1462-2920.14496
Deng, Y. Z., Zhang, B., Chang, C., Wang, Y., Lu, S., Sun, S., et al. (2018). The MAP kinase SsKpp2 is required for mating/filamentation in Sporisorium scitamineum. Front. Microbiol. 9:2555. doi: 10.3389/fmicb.2018.02555
Donlin, M. J., Upadhya, R., Gerik, K. J., Lam, W., VanArendonk, L. G., Specht, C. A., et al. (2014). Cross talk between the cell wall integrity and cyclic AMP/protein kinase A pathways in Cryptococcus neoformans. Mbio 5:e01573-14. doi: 10.1128/mBio.01573-1514
Esher, S. K., Ost, K. S., Kozubowski, L., Yang, D. H., Kim, M. S., Bahn, Y. S., et al. (2016). Relative contributions of prenylation and postprenylation processing in Cryptococcus neoformans pathogenesis. mSphere 1:e00084-15. doi: 10.1128/mSphere.00084-15
Flom, G. A., Lemieszek, M., Fortunato, E. A., and Johnson, J. L. (2008). Farnesylation of ydj1 is required for in vivo interaction with Hsp90 client proteins. Mol. Biol. Cell 19, 5249–5258. doi: 10.1091/mbc.E08-04-0435
Fortwendel, J. R., Panepinto, J. C., Seitz, A. E., Askew, D. S., and Rhodes, J. C. (2004). Aspergillus fumigatus rasA and rasB regulate the timing and morphology of asexual development. Fungal Genet. Biol. 41, 129–139. doi: 10.1016/j.fgb.2003.10.004
Fowler, T. J., DeSimone, S. M., Mitton, M. F., Kurjan, J., and Raper, C. A. (1999). Multiple sex pheromones and receptors of a mushroom-producing fungus elicit mating in yeast. Mol. Biol. Cell 10, 2559–2572. doi: 10.1091/mbc.10.8.2559
Hamel, L. P., Nicole, M. C., Duplessis, S., and Ellis, B. E. (2012). Mitogen-activated protein kinase signaling in plant-interacting fungi: distinct messages from conserved messengers. Plant Cell 24, 1327–1351. doi: 10.1105/tpc.112.096156
Hartmann, H. A., Kahmann, R., and Bolker, M. (1996). The pheromone response factor coordinates filamentous growth and pathogenicity in Ustilago maydis. EMBO J. 15, 1632–1641. doi: 10.1002/j.1460-2075.1996.tb00508.x
Hartmann, H. A., Krüge, J., Lottspeich, F., and Kahmann, R. (1999). Environmental signals controlling sexual development of the corn Smut fungus Ustilago maydis through the transcriptional regulator Prf1. Plant Cell. 11, 1293–1305. doi: 10.2307/3870750
He, B., Chen, P., Chen, S. Y., Vancura, K. L., Michaelis, S., and Powers, S. (1991). RAM2, an essential gene of yeast, and RAM1 encode the two polypeptide components of the farnesyltransferase that prenylates a-factor and Ras proteins. Proc. Natl. Acad. Sci. U.S.A. 88, 11373–11377. doi: 10.1073/pnas.88.24.11373
He, Y. L., Liu, X. Z., and Huang, B. R. (2005). Changes in protein content, protease activity, and amino acid content associated with heat injury in creeping bentgrass. J. Am. Soc. Hortic. Sci. 130, 842–847. doi: 10.21273/JASHS.130.6.842
Hughes, D. A., Ashworth, A., and Marshall, C. J. (1993). Complementation of byr1 in fission yeast by mammalian MAP kinase kinase requires coexpression of Raf kinase. Nature 364, 349–352. doi: 10.1038/364349a0
Jeon, J., Goh, J., Yoo, S., Chi, M. H., Choi, J., Rho, H. S., et al. (2008). A putative MAP kinase kinase kinase, MCK1, is required for cell wall integrity and pathogenicity of the rice blast fungus, Magnaporthe oryzae. Mol. Plant Microbe Interact. 21, 525–534. doi: 10.1094/MPMI-21-5-0525
Jung, U. S., Sobering, A. K., Romeo, M. J., and Levin, D. E. (2002). Regulation of the yeast Rlm1 transcription factor by the Mpk1 cell wall integrity MAP kinase. Mol. Microbiol. 46, 781–789. doi: 10.1046/j.1365-2958.2002.03198.x
Kahmann, R., and Kamper, J. (2004). Ustilago maydis: how its biology relates to pathogenic development. New Phytol. 164, 31–42. doi: 10.1111/j.1469-8137.2004.01156.x
Kenaley, S. C., Quan, M., Aime, M. C., and Bergstrom, G. C. (2018). New insight into the species diversity and life cycles of rust fungi (Pucciniales) affecting bioenergy switchgrass (Panicum virgatum) in the Eastern and Central United States. Mycol. Prog. 17, 1251–1267.
Kim, K. Y., Truman, A. W., and Levin, D. E. (2008). Yeast mpk1 mitogen-activated protein kinase activates transcription through Swi4/Swi6 by a noncatalytic mechanism that requires upstream signal. Mol. Cell. Biol. 28, 2579–2589. doi: 10.1128/MCB.01795-1797
Koppitz, M., Spellig, T., Kahmann, R., and Kessler, H. (1996). Lipoconjugates: structure-activity studies for pheromone analogues of Ustilago maydis with varied lipophilicity. Int. J. Pept. Protein Res. 48, 377–390. doi: 10.1111/j.1399-3011.1996.tb00855.x
Kosted, P. J., Gerhardt, S. A., Anderson, C. M., Stierle, A., and Sherwood, J. E. (2000). Structural requirements for activity of the pheromones of Ustilago hordei. Fungal Genet. Biol. 29, 107–117. doi: 10.1006/fgbi.2000.1191
Krombach, S., Reissmann, S., Kreibich, S., Bochen, F., and Kahmann, R. (2018). Virulence function of the Ustilago maydis sterol carrier protein 2. New Phytol. 220, 553–566. doi: 10.1111/nph.15268
Lanubile, A., Maschietto, V., De Leonardis, S., Battilani, P., Paciolla, C., and Marocco, A. (2015). Defense responses to mycotoxin-producing fungi Fusarium proliferatum, F. subglutinans, and Aspergillus flavus in kernels of susceptible and resistant maize genotypes. Mol. Plant Microbe Interact. 28, 546–557. doi: 10.1094/MPMI-09-14-0269-R
Leach, M. D., and Brown, A. J. (2012). Posttranslational modifications of proteins in the pathobiology of medically relevant fungi. Eukaryot. Cell 11, 98–108. doi: 10.1128/EC.05238-5211
Lee, K. S., and Levin, D. E. (1992). Dominant mutations in a gene encoding a putative protein kinase (BCK1) bypass the requirement for a Saccharomyces cerevisiae protein kinase C homolog. Mol. Cell. Biol. 12, 172–182. doi: 10.1128/mcb.12.1.172
Lee, N., and Kronstad, J. W. (2002). ras2 controls morphogenesis, pheromone response, and pathogenicity in the fungal pathogen Ustilago maydis. Eukaryot. Cell 1, 954–966. doi: 10.1128/EC.1.6.954-966.2002
Lesage, G., and Bussey, H. (2006). Cell wall assembly in Saccharomyces cerevisiae. Microbiol. Mol. Biol. Rev. 70, 317–343. doi: 10.1128/MMBR.00038-35
Leung, K. F., Baron, R., and Seabra, M. C. (2006). Thematic review series: lipid posttranslational modifications. geranylgeranylation of Rab GTPases. J. Lipid Res. 47, 467–475. doi: 10.1194/jlr.R500017-JLR200
Li, D., Yang, B., and Mehta, J. L. (1998). Ox-LDL induces apoptosis in human coronary artery endothelial cells: role of PKC, PTK, bcl-2, and Fas. Am. J. Physiol. 275(Pt 2), H568–H576. doi: 10.1016/S0002-9149(98)00337-333
Li, M. H., Xie, X. L., Lin, X. F., Shi, J. X., Ding, Z. J., Ling, J. F., et al. (2014). Functional characterization of the gene FoOCH1 encoding a putative alpha-1,6-mannosyltransferase in Fusarium oxysporum f. sp. cubense. Fungal Genet. Biol. 65, 1–13. doi: 10.1016/j.fgb.2014.01.005
Livak, K. J., and Schmittgen, T. D. (2001). Analysis of relative gene expression data using real-time quantitative PCR and the 2(-Delta Delta C(T) Method. Methods 25, 402–408. doi: 10.1006/meth.2001.1262
Lu, S., Shen, X., and Chen, B. (2017). Development of an efficient vector system for gene knock-out and near in-cis gene complementation in the sugarcane smut fungus. Sci. Rep. 7:3113. doi: 10.1038/s41598-017-03233-3237
Maddi, A., Fu, C., and Free, S. J. (2012). The Neurospora crassa dfg5 and dcw1 genes encode alpha-1,6-mannanases that function in the incorporation of glycoproteins into the cell wall. PLoS One 7:e38872. doi: 10.1371/journal.pone.0038872
Michaelis, S., and Barrowman, J. (2012). Biogenesis of the Saccharomyces cerevisiae pheromone a-factor, from yeast mating to human disease. Microbiol. Mol. Biol. Rev. 76, 626–651. doi: 10.1128/MMBR.00010-12
Mosch, H. U., Roberts, R. L., and Fink, G. R. (1996). Ras2 signals via the Cdc42/Ste20/mitogen-activated protein kinase module to induce filamentous growth in Saccharomyces cerevisiae. Proc. Natl. Acad. Sci. U.S.A. 93, 5352–5356. doi: 10.2307/39435
Nadal, M., Garcia-Pedrajas, M. D., and Gold, S. E. (2008). Dimorphism in fungal plant pathogens. FEMS Microbiol. Lett. 284, 127–134. doi: 10.1111/j.1574-6968.2008.01173.x
Omer, C. A., and Gibbs, J. B. (1994). Protein prenylation in eukaryotic microorganisms: genetics, biology and biochemistry. Mol. Microbiol. 11, 219–225. doi: 10.1111/j.1365-2958.1994.tb00302.x
Ozaki, K., Tanaka, K., Imamura, H., Hihara, T., Kameyama, T., Nonaka, H., et al. (1996). Rom1p and Rom2p are GDP/GTP exchange proteins (GEPs) for the Rho1p small GTP binding protein in Saccharomyces cerevisiae. EMBO J. 15, 2196–2207. doi: 10.1002/j.1460-2075.1996.tb00573.x
Paravicini, G., and Friedli, L. (1996). Protein-protein interactions in the yeast PKC1 pathway: Pkc1p interacts with a component of the MAP kinase cascade. Mol. Gen. Genet. 251, 682–691. doi: 10.1007/BF02174117
Paumi, C. M., Chuk, M., Snider, J., Stagljar, I., and Michaelis, S. (2009). ABC transporters in Saccharomyces cerevisiae and their interactors: new technology advances the biology of the ABCC (MRP) subfamily. Microbiol. Mol. Biol. Rev. 73, 577–593. doi: 10.1128/mmbr.00020-29
Philip, B., and Levin, D. E. (2001). Wsc1 and Mid2 are cell surface sensors for cell wall integrity signaling that act through Rom2, a guanine nucleotide exchange factor for Rho1. Mol. Cell. Biol. 21, 271–280. doi: 10.1128/MCB.21.1.271-280.2001
Qiao, J., Song, Y., Ling, Z., Liu, X., and Fang, H. (2017). ram1 gene, encoding a subunit of farnesyltransferase, contributes to growth, antifungal susceptibility to amphotericin B of Aspergillus fumigatus. Med. Mycol. 55, 883–889. doi: 10.1093/mmy/myx002
Rui, O., and Hahn, M. (2007). The Slt2-type MAP kinase Bmp3 of botrytis cinerea is required for normal saprotrophic growth, conidiation, plant surface sensing and host tissue colonization. Mol. Plant Pathol. 8, 173–184. doi: 10.1111/j.1364-3703.2007.00383.x
Schirawski, J., Heinze, B., Wagenknecht, M., and Kahmann, R. (2005). Mating type loci of Sporisorium reilianum: novel pattern with three a and multiple b specificities. Eukaryot. Cell 4, 1317–1327. doi: 10.1128/EC.4.8.1317-1327.2005
Schmelzle, T., Helliwell, S. B., and Hall, M. N. (2002). Yeast protein kinases and the RHO1 exchange factor TUS1 are novel components of the cell integrity pathway in yeast. Mol. Cell. Biol. 22, 1329–1339. doi: 10.1128/MCB.22.5.1329-1339.2002
Shen, W. C., Davidson, R. C., Cox, G. M., and Heitman, J. (2002). Pheromones stimulate mating and differentiation via paracrine and autocrine signaling in Cryptococcus neoformans. Eukaryot. Cell 1, 366–377. doi: 10.1128/EC.1.3.366-377.2002
Silva, R. D., Sotoca, R., Johansson, B., Ludovico, P., Sansonetty, F., Silva, M. T., et al. (2005). Hyperosmotic stress induces metacaspase- and mitochondria-dependent apoptosis in Saccharomyces cerevisiae. Mol. Microbiol. 58, 824–834. doi: 10.1111/j.1365-2958.2005.04868.x
Song, J. L., and White, T. C. (2003). RAM2: an essential gene in the prenylation pathway of Candida albicans. Microbiology 149(Pt 1), 249–259. doi: 10.1099/mic.0.25887-25880
Spellig, T., Bolker, M., Lottspeich, F., Frank, R. W., and Kahmann, R. (1994). Pheromones trigger filamentous growth in Ustilago maydis. EMBO J. 13, 1620–1627. doi: 10.1002/j.1460-2075.1994.tb06425.x
Su, Y., Xu, L., Wang, Z., Peng, Q., Yang, Y., Chen, Y., et al. (2016). Comparative proteomics reveals that central metabolism changes are associated with resistance against Sporisorium scitamineum in sugarcane. BMC Genomics 17:800. doi: 10.1186/s12864-016-3146-3148
Sun, L., Yan, M., Ding, Z., Liu, Y., Du, M., Xi, P., et al. (2014). Improved dominant selection markers and co-culturing conditions for efficient Agrobacterium tumefaciens-mediated transformation of Ustilago scitaminea. Biotechnol. Lett. 36, 1309–1314. doi: 10.1007/s10529-014-1486-1485
Taniguti, L. M., Schaker, P. D., Benevenuto, J., Peters, L. P., Carvalho, G., Palhares, A., et al. (2015). Complete genome sequence of Sporisorium scitamineum and biotrophic interaction transcriptome with sugarcane. PLoS One 10:e0129318. doi: 10.1371/journal.pone.0129318
Thompson, J. D., Higgins, D. G., and Gibson, T. J. (1994). CLUSTAL W: improving the sensitivity of progressive multiple sequence alignment through sequence weighting, position-specific gap penalties and weight matrix choice. Nucleic Acids Res. 22, 4673–4680. doi: 10.1007/978-1-4020-6754-9_3188
Vallim, M. A., Fernandes, L., and Alspaugh, J. A. (2004). The RAM1 gene encoding a protein-farnesyltransferase beta-subunit homologue is essential in Cryptococcus neoformans. Microbiology 150(Pt 6), 1925–1935. doi: 10.1099/mic.0.27030-27030
Williams, S. A., and Birnbaum, M. J. (1988). The rat facilitated glucose transporter gene. transformation and serum-stimulated transcription initiate from identical sites. J. Biol. Chem. 263, 19513–19518.
Xie, X. Q., Guan, Y., Ying, S. H., and Feng, M. G. (2013). Differentiated functions of Ras1 and Ras2 proteins in regulating the germination, growth, conidiation, multi-stress tolerance and virulence of Beauveria bassiana. Environ. Microbiol. 15, 447–462. doi: 10.1111/j.1462-2920.2012.02871.x
Yamagishi, K., Kimura, T., Suzuki, M., Shinmoto, H., and Yamaki, K. J. (2004). Elevation of intracellular cAMP levels by dominant active heterotrimeric G protein alpha subunits ScGP-A and ScGP-C in homobasidiomycete, Schizophyllum commune. Biosci. Biotechnol. Biochem. 68, 1017–1026. doi: 10.1271/bbb.68.1017
Yan, M., Zhu, G., Lin, S., Xian, X., Chang, C., Xi, P., et al. (2016a). The mating-type locus b of the sugarcane smut Sporisorium scitamineum is essential for mating, filamentous growth and pathogenicity. Fungal Genet. Biol. 86, 1–8. doi: 10.1016/j.fgb.2015.11.005
Yan, M. X., Cai, E. P., Zhou, J. N., Chang, C. Q., Xi, P. G., Shen, W. K., et al. (2016b). A dual-color imaging system for sugarcane smut fungus Sporisorium scitamineum. Plant Dis. 100, 2357–2362. doi: 10.1094/PDIS-02-16-0257-SR
Yan, M. X., Dai, W. J., Cai, E. P., Deng, Y. Z., Chang, C. Q., Jiang, Z. D., et al. (2016c). Transcriptome analysis of Sporisorium scitamineum reveals critical environmental signals for fungal sexual mating and filamentous growth. BMC Genomics 17:354. doi: 10.1186/s12864-016-2691-2695
Young, S. G., Fong, L. G., and Michaelis, S. (2005). Prelamin A, Zmpste24, misshapen cell nuclei, and progeria–new evidence suggesting that protein farnesylation could be important for disease pathogenesis. J. Lipid Res. 46, 2531–2558. doi: 10.1194/jlr.R500011-JLR200
Keywords: cell wall integrity, mating, RAM1, Ras, Sporisorium scitamineum
Citation: Sun S, Deng Y, Cai E, Yan M, Li L, Chen B, Chang C and Jiang Z (2019) The Farnesyltransferase β-Subunit Ram1 Regulates Sporisorium scitamineum Mating, Pathogenicity and Cell Wall Integrity. Front. Microbiol. 10:976. doi: 10.3389/fmicb.2019.00976
Received: 13 March 2019; Accepted: 18 April 2019;
Published: 08 May 2019.
Edited by:
Fausto Almeida, University of São Paulo, BrazilReviewed by:
Andrei Steindorff, Joint Genome Institute and Lawrence Berkeley National Laboratory, United StatesJarrod R. Fortwendel, The University of Tennessee Health Science Center, United States
Copyright © 2019 Sun, Deng, Cai, Yan, Li, Chen, Chang and Jiang. This is an open-access article distributed under the terms of the Creative Commons Attribution License (CC BY). The use, distribution or reproduction in other forums is permitted, provided the original author(s) and the copyright owner(s) are credited and that the original publication in this journal is cited, in accordance with accepted academic practice. No use, distribution or reproduction is permitted which does not comply with these terms.
*Correspondence: Changqing Chang, Y2hhbmdjcUBzY2F1LmVkdS5jbg== Zide Jiang, emRqaWFuZ0BzY2F1LmVkdS5jbg==