- 1Department of Biochemistry and Molecular Biology, School of Basic Medical Sciences, Anhui Medical University, Hefei, China
- 2Anhui Provincial Key Laboratory of Microbiology and Parasitology, Anhui Medical University, Hefei, China
- 3Department of Anesthesiology, The First Affiliated Hospital of Anhui Medical University, Hefei, China
Toxoplasma gondii is an obligate intracellular parasite responsible for toxoplasmosis, which can cause severe disease in the fetus and immunocompromised individuals. Developing an effective vaccine is crucial to control this disease. Macrophage migration inhibitory factor (MIF) has gained substantial attention as a pivotal upstream cytokine to mediate innate and adaptive immune responses. Homologs of MIF have been discovered in many parasitic species, and one homolog of MIF has been isolated from the parasite Toxoplasma gondii. In this study, the recombinant Toxoplasma gondii MIF (rTgMIF) as a protein vaccine was expressed and evaluated by intramuscular injection in BALB/c mice. We divided the mice into different dose groups of vaccines, and all immunizations with purified rTgMIF protein were performed at 0, 2, and 4 weeks. The protective efficacy of vaccination was analyzed by antibody assays, cytokine measurements and lymphoproliferative assays, respectively. The results obtained indicated that the rTgMIF vaccine elicited strong humoral and cellular immune responses with high levels of IgG antibody and IFN-γ production compared to those of the controls, in addition to slight higher levels of IL-4 production. After vaccination, a stronger lymphoproliferative response was also noted. Additionally, the survival time of mice immunized with rTgMIF was longer than that of the mice in control groups after challenge infection with virulent T. gondii RH tachyzoites. Moreover, the number of brain tissue cysts in vaccinated mice was reduced by 62.26% compared with the control group. These findings demonstrated that recombinant TgMIF protein is a potential candidate for vaccine development against toxoplasmosis.
Introduction
Toxoplasma gondii is an obligate intracellular protozoan that belongs to the phylum Apicomplexa. This parasite can infect a wide range of warm-blooded mammals, including humans, livestock and birds. According to the statistics, approximately one-third of the world’s population is seropositive (Petersen et al., 2001; Munoz et al., 2011). The parasite causes severe symptoms in immunocompromised patients, as well as abortions in infected pregnant women (Martin et al., 2004; Ching et al., 2016). Moreover, the obtained results from the epidemiologic survey show that the prevalent disease of T. gondii has caused large economic losses to the stock-raising industry (Dubey et al., 2005; Wang et al., 2016).
Currently, medications (pyrimethamine, sulfadiazine, and spiramycin) can control T. gondii acute infections, but they are unable to eliminate the chronic infection completely (Montoya and Liesenfeld, 2004; Innes, 2010). Vaccinations are considered to be an effective mean to prevent infection with the parasite. To date, inactivated and attenuated vaccines, subunit vaccines, DNA vaccines and recombinant protein vaccines have been developed to prevent infection by T. gondii. However, inactivated and attenuated vaccines are not sufficiently efficacious against this ubiquitous pathogen. In addition, it has been recognized that DNA vaccines generate only weak immune responses when used in higher primates and humans (Kur et al., 2009; Liu et al., 2012). Toxovax®, the only available commercial vaccine, is based on the live-attenuated T. gondii S48 strain and is licensed only for use in sheep to avoid congenital toxoplasmosis (Buxton and Innes, 1995). However, due to the risk of reverting to a virulent wild type, live vaccines need to be further explored in food-producing animals and humans. Thus, it is imperative for us to develop a valuable and practical vaccine against toxoplasmosis, and an effective vaccine will be beneficial to the livestock industry by reducing economic losses (Qu et al., 2013).
Macrophage migration inhibitory factor (MIF) is a pro-inflammatory mammalian cytokine with enzymatic activity of tautomerase and oxidoreductase. MIF has been demonstrated to be involved in innate and adaptive immune responses by stimulating the production of pro-inflammatory mediators, such as TNF-α and IL-8. MIF was found to sustain macrophage survival and proinflammatory function by suppressing activation-induced, p53-dependent apoptosis (Mitchell et al., 2002; Calandra and Roger, 2003; Larson and Horak, 2006; Hertelendy et al., 2018). The cytokines affected by MIF are involved in the pathogenesis of infectious and non-infectious inflammatory diseases (de Oliveira Gomes et al., 2011; Gomes et al., 2018). In a previous study, this conserved protein homolog has been found in different phyla, including many parasitic organisms, and it was capable of facilitating the host immune responses during infection (Zang et al., 2002; Flores et al., 2008; Vermeire et al., 2008; Sommerville et al., 2013; Sparkes et al., 2017). Considering the important roles of MIF, we hypothesized that the homolog of MIF from Toxoplasma gondii (TgMIF) could be utilized as a candidate vaccine against Toxoplasma infection.
The aim of this study was evaluating the mouse immunoreaction after injecting the rTgMIF protein vaccine and examining the efficacy of TgMIF as a vaccine candidate against acute and chronic T. gondii infection.
Materials and Methods
Ethics Statement
Ethical permission was obtained from the Institutional Review Board of the Institute of Biomedicine at Anhui Medical University (permit number 20180016), which records and regulates all research activities in the school. This study was carried out in strict accordance with the recommendations of the Guide for the Care and Use of Laboratory Animals of Anhui Medical University. The experiments were subjected to approval by the Ethics Committee of the Animal Experiments of Anhui Medical University. The approval from the Institutional Review Board includes permission for using mice under anesthesia, and all of the experimental procedures were performed in strict accordance with the recommendations in the Guide for the Care and Use of Laboratory Animals of the National Institutes of Health.
Animals and Parasites
Six- to eight-week-old female BALB/c mice were purchased from the Anhui Medical University Laboratory Animal Center. All mice were bred under specific-pathogen-free conditions and had free access to food and tap water. The virulent wild-type RH strain (Type 1) and PRU strain (Type 2) were propagated in our laboratory. The tachyzoites of RH were harvested from human foreskin fibroblast cells. The PRU strain of T. gondii used in this study was maintained by passage of cysts in Kunming mice.
TgMIF Antibody Preparation
Specific TgMIF antibody was obtained by subcutaneous immunizations of a rabbit with 1 mg of the recombinant protein separated on sodium dodecyl sulfate polyacrylamide gel electrophoresis (SDS–PAGE) and transferred to nitrocellulose, which was then crushed in Freund’s complete adjuvant and injected. Two further injections were performed at 3-week intervals in Freund’s incomplete adjuvant. The rabbit was then bled, and the specific antibodies were affinity purified on the recombinant protein that was electrophoresed and transferred onto nitrocellulose for subsequent Western blot analysis.
Expression and Purification of Recombinant TgMIF and Tg14-3-3
Amplification of the open reading frame encoding T. gondii MIF (GenBankTM ID XP_002368429.1) was achieved through RT-PCR of the whole T. gondii (ME49 strain) RNA. The gene sequence encoding TgMIF was inserted into the prokaryotic expression vector pET28a (Novagen). Amplification of the open reading frame encoding T. gondii 14-3-3 (GenBankTM ID AB012775) was achieved through RT-PCR of the whole T. gondii (RH strain) RNA. The gene sequence encoded Tg14-3-3 was inserted into the prokaryotic expression vector pET28a (Novagen). To express the recombinant protein, the pET-28a-TgMIF or pET-28a-Tg14-3-3 plasmid was transformed into Rosetta (DE3) host bacteria cells (Transgen, CN), and recombinant protein expression was induced with 0.1 mM IPTG at 30°C for 4 h with constant shaking at 220 rpm. Then, the bacterial cells were harvested by centrifugation at 12,000 rpm for 5 min at 4°C. The obtained pellets were resuspended in lysis buffer (50 mM NaH2PO4, 300 mM NaCl, 10 mM imidazole, pH 8.0), the bacteria suspension was sonicated at a speed of 300 W/s on ice, and the total ultrasonic time was 10 min at 5 s interval. In the next step, the lysate was centrifuged at 12,000 × g for 20 min to separate the supernatant and bacteria debris. The level of rTgMIF expression was analyzed by 15% SDS–PAGE electrophoresis and Coomassie blue R-250 staining.
The subsequent purification steps were implemented at 4°C. The separated supernatants were collected and mixed with a purification column containing 1 ml Ni2+-NTA agarose (Qiagen, Germany). The complex was incubated for 1 h with gentle rotating. After binding, the binding protein was washed with wash buffer 1 (50 mM NaH2PO4, 300 mM NaCl, 20 mM imidazole, pH 8.0) and wash buffer 2 (50 mM NaH2PO4, 300 mM NaCl, 40 mM imidazole, pH8.0) for removing other unrelated proteins. Subsequently, the target protein was eluted with elution buffer (50 mM NaH2PO4, 300 mM NaCl, 300 mM imidazole, pH 8.0). The eluted rTgMIF protein was collected and dialyzed overnight against PBS to remove unwanted salt ions. The efficiency of purification was analyzed via 15% SDS–PAGE.
Western Blotting
The rTgMIF and rTg14-3-3 were prepared as described above. The supernatants were separated on 15% SDS–PAGE gels and then electrophoretically transferred to PVDF membranes (GE Healthcare). The membranes were blocked in 5% skim milk for 1 h at room temperature and then incubated at 4°C overnight with the rabbit anti-TgMIF primary antibody (1:1000). The PVDF membrane was subsequently incubated with anti-rabbit HRP-IgG (Beyotime, China) for 1 h at room temperature, and chemiluminescence was detected using an ECL blot detection system (Engreen, China).
rTgMIF Immunization
Initially, 6- to 8-week-old female BALB/c mice (n = 240) were divided into six groups with 40 mice per group at random. We set two negative groups: a blank control and PBS, and four immunization groups (protein doses of 2.5, 5.0, 10.0, or 20.0 μg). The doses of immunization were referenced according to previously studies (Pinzan et al., 2015; Ching et al., 2016; Sonaimuthu et al., 2016; Czarnewski et al., 2017). For the experimental group, all mice in different groups were intramuscularly injected with 2.5, 5.0, 10.0, or 20.0 μg of rTgMIF emulsified in Freund’s adjuvant, respectively. As negative controls, the mice were intramuscularly injected with 100 μl sterile PBS added with adjuvant. Different immunization samples were emulsified with complete/incomplete Freud’s adjuvant (C/IFA) at a 1:1 ratio before immunization. The first immunization was with Freund’s complete adjuvant, and the next two immunizations were with Freund’s incomplete adjuvant for booster injections. Mice were immunized using the same protocol on days 1, 15, and 29. Blood serum samples were collected from the immunized mice in each group via tail-bleeding on days 0, 14, 28, and 42. Sera samples were separated and stored at -80°C until evaluation of the levels of specific antibodies.
Antibody Detection
Serum samples were harvested on days 0, 14, 28, and 42 and stored at -80°C. The level of antigen-specific IgG was evaluated by enzyme-linked immunosorbent assays (ELISAs). In brief, polystyrene 96-well flat-bottom microtiter plates were coated with 10 μg/mL rTgMIF diluted in 100 μl coating buffer (50 mM carbonate buffer, pH 9.6) at 37°C for 1 h, and then incubated overnight at 4°C. The plates were washed five times with PBS containing 0.05% Tween20 (PBST, pH7.4) and then we blocked the plates with 1% BSA for 1 h at 37°C. Thereafter, after washing five times with PBST, they were incubated with sera diluted in 0.1% BSA-PBST (1:50 for IgG) for 1 h at 37°C. After washing, 100 μl of HRP-conjugated goat anti-mouse IgG (ProteinTech Group, Inc., United States) diluted in PBST (1:1000) was added to the wells and incubated for 1 h at 37°C. Then, the plates were washed, and the immune complexes were represented by incubating with TMB (100 μl/well, Beyotime Biotechnology) for 20 min. The enzyme reaction was terminated by adding 2 M H2SO4. The optical density (OD) was measured with an ELISA reader at 450 nm. All sera samples were measured in triplicate.
Lymphocyte Proliferation Assays
Two weeks after the last immunization, three mice per group were anesthetized and sacrificed. The spleens were harvested under aseptic conditions in PBS and passed through a 70 μm wire mesh sieve to generate a single cell suspension. The splenic lymphocytes were disposed of using standard lymphocyte isolation lysis buffer. Splenocytes were cultured in 96-well microplates at a density of 2 × 105 cells per well in RPMI-1640 containing 10% FBS. The spleen cells were stimulated with 10 μg/mL rTgMIF (positive control) or medium alone (negative control) for 72 h at 37°C in a 5% CO2 incubator. Splenocytes proliferation assay was conducted by using a Cell Counting Kit-8 (Beyotime Biotechnology, CN). The stimulation index (SI) was expressed using the formula OD450 rTgMIF/OD450M, which was the ratio of the OD450 of stimulated cells to the OD450 of unstimulated cells.
Cytokine Assays
To detect the levels of cytokine production, spleen cells were obtained from three mice in each group as described. For these experiments, 1.5 × 106 cells/well were seeded into 24-well plates and stimulated with 10 μg/mL rTgMIF. Cell-free supernatants were harvested to measure IL-4 activity at 24 and 96 h for quantification of IFN-γ after poststimulation, respectively. All assays were performed with commercial ELISA kits according to the manufacturer’s instructions (Shanghai Enzyme-linked Biotechnology Co., Ltd., China). The levels of IFN-γ and IL-4 were determined by reference to standard curves constructed with known amounts of mouse recombinant IFN-γ and IL-4. The analysis was performed with data from three independent experiments.
Challenge Infection
The best immune group was selected to infect with T. gondii. A total of 30 mice per group were randomly chosen and challenged with 1 × 103 T. gondii RH strain tachyzoites diluted in 100 μl PBS by intraperitoneal injection 2 weeks after the final immunization. The survival time of the challenged mice was recorded within 30 days, and their survival rate was calculated until there was a fatal outcome for all animals. To evaluate the protection efficiency of the vaccine against chronic toxoplasmosis, three mice per group were orally challenged with 20 tissue cysts of the PRU strain as a model of experimental chronic toxoplasmosis. One month after infection, brains of mice from each group were homogenized in 1 ml PBS. The number of cysts per brain was determined by three samples of 10 μl aliquots of each homogenized brain under an optical microscope.
Statistical Analysis
Statistical analysis and graphics were performed following the procedures of GraphPad Prism 5.0. All data, including antibody levels and lymphocyte proliferation assays, cytokine production and brain cyst loadings were compared by one-way ANOVA. The survival time was evaluated by the Kaplan-Meier method and compared with the log-rank test. The value of p < 0.05 was considered to be statistically significant.
Results
Epitope Analysis
The ORF of TgMIF is 351 bp, encoding a protein of 116 amino acids with a predicted molecular weight of 13 kDa and an isoelectric point of 8.91. DNASTAR was used to analyze the protein of TgMIF for surface probability, antigenic index, and hydrophilic plot, as well as flexible region. The results revealed that most regions of the TgMIF protein were in hydrophilicity plots and flexible regions, and TgMIF exhibited ideal surface probability and an antigenic index that indicated it was a promising prospect for producing vaccines (Figure 1).
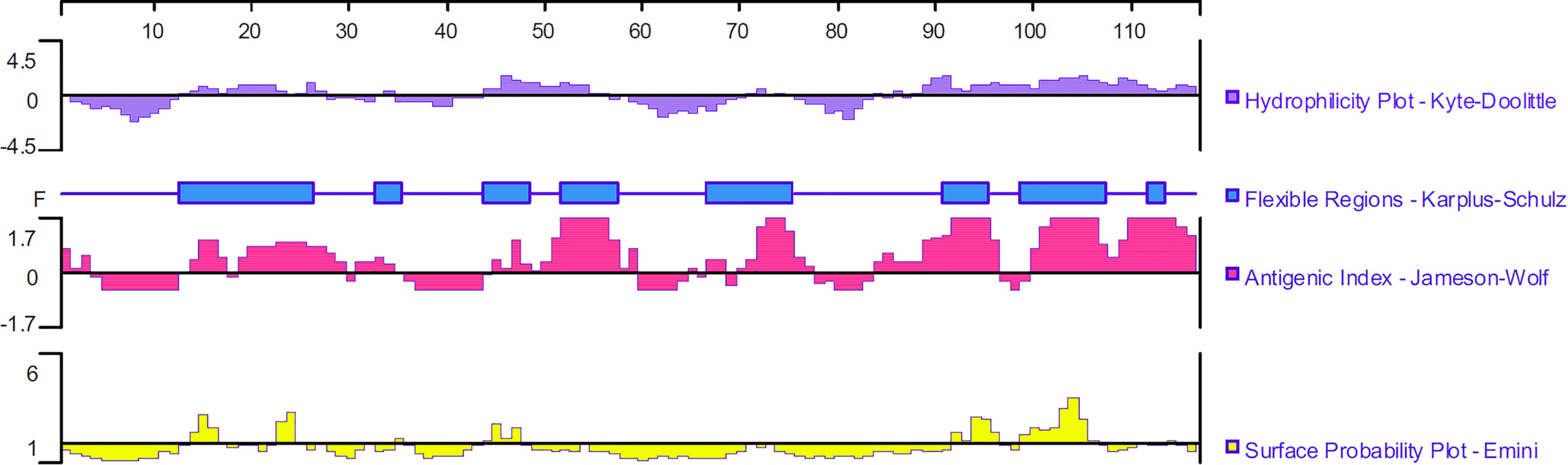
Figure 1. Linear-B cell epitopes of TgMIF predicted by DNASTAR in hydrophilicity plot, flexible regions, antigenic index, and surface probability rules.
Recombinant TgMIF Expression and Purification
The recombinant TgMIF was expressed in E. coli and the soluble recombinant protein was purified by Ni2+-NTA agarose. SDS-PAGE analysis indicated that the rTgMIF had a molecular weight of approximately 13 kDa (Figure 2A). In addition, the specificity of prokaryotic expression of rTgMIF was identified by the polyclonal antibody against TgMIF as a band of approximately 13 kDa, which was consistent with the deduced size. In addition, another purified recombinant parasite protein, Tg14-3-3, was not identified by the TgMIF antibody (Figure 2B). Thus, these results indicated that rTgMIF had been successfully expressed and purified.
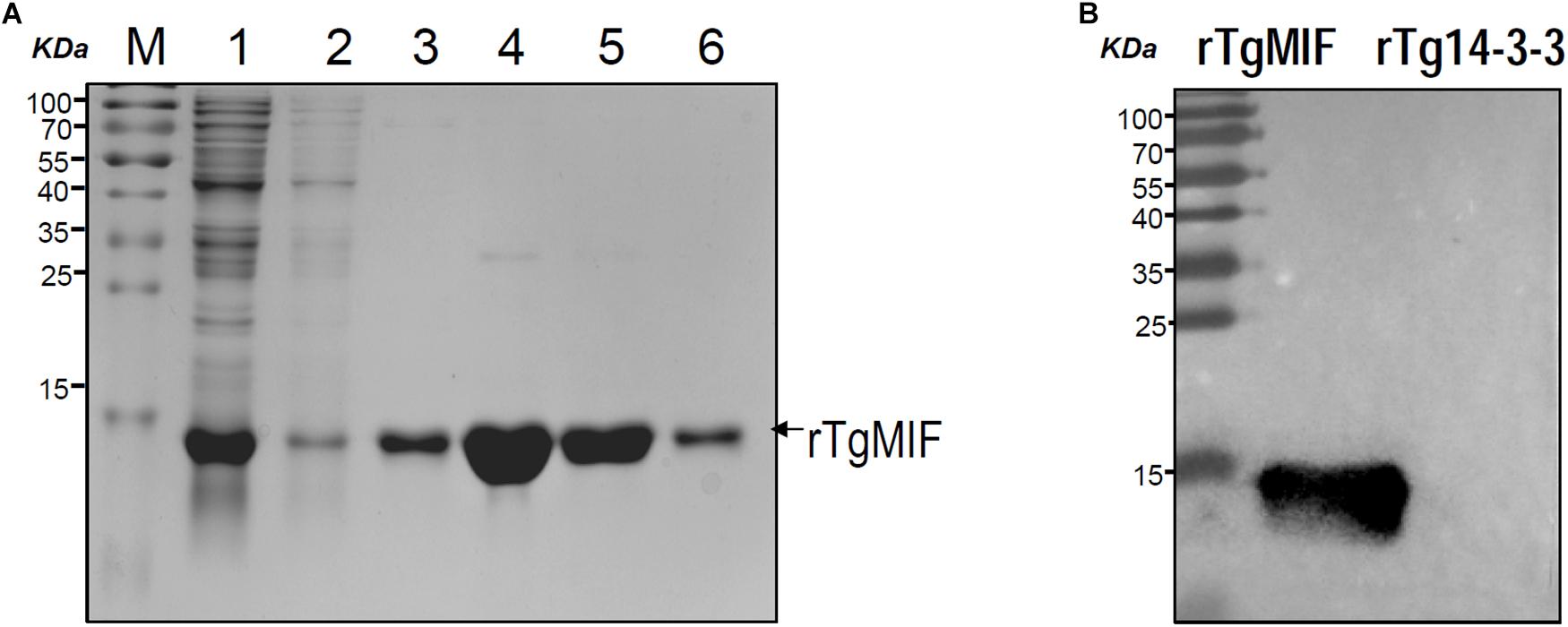
Figure 2. SDS-PAGE and western blotting analysis of the purified rTgMIF protein. (A) rTgMIF protein was purified and stained with Coomassie blue. The protein band of rTgMIF was approximately 13 kDa. M: protein marker; Lane 1: total bacterial lysate of pET-28a-TgMIF; Lanes 2–3: wash 1 and wash 2, the purified protein was washed, and the washing product was detected; Lanes 4–6: elution product, the purified rTgMIF was eluted three times, Elution 1, Elution 2, and Elution 3. (B) The purified rTgMIF and rTg14-3-3 were detected through western blotting by anti-TgMIF polyclonal antibody. M: protein molecular weight marker; rTgMIF and rTg14-3-3 purified through Ni2+-charged column chromatography and after dialysis.
Humoral Immune Response Elicited by the Immunized Mice
To evaluate the levels of specific anti-T. gondii antibodies in sera, IgG from different groups were tested by ELISA on the preinjection, second, fourth, and sixth week after inoculation. The results revealed that IgG remained stable, and there was no difference in the blank control and PBS groups. However, the levels of IgG were significantly increased in the sera of mice immunized with rTgMIF compared with the control groups (p < 0.001). Moreover, the level of IgG tended to increase along with the continuous injection. The results also indicated that 5 μg rTgMIF induced a humoral immune response in the second week, and other doses induced a response in the fourth week. Moreover, a dose of 5 μg rTgMIF elicited the maximum level of IgG (Figure 3).
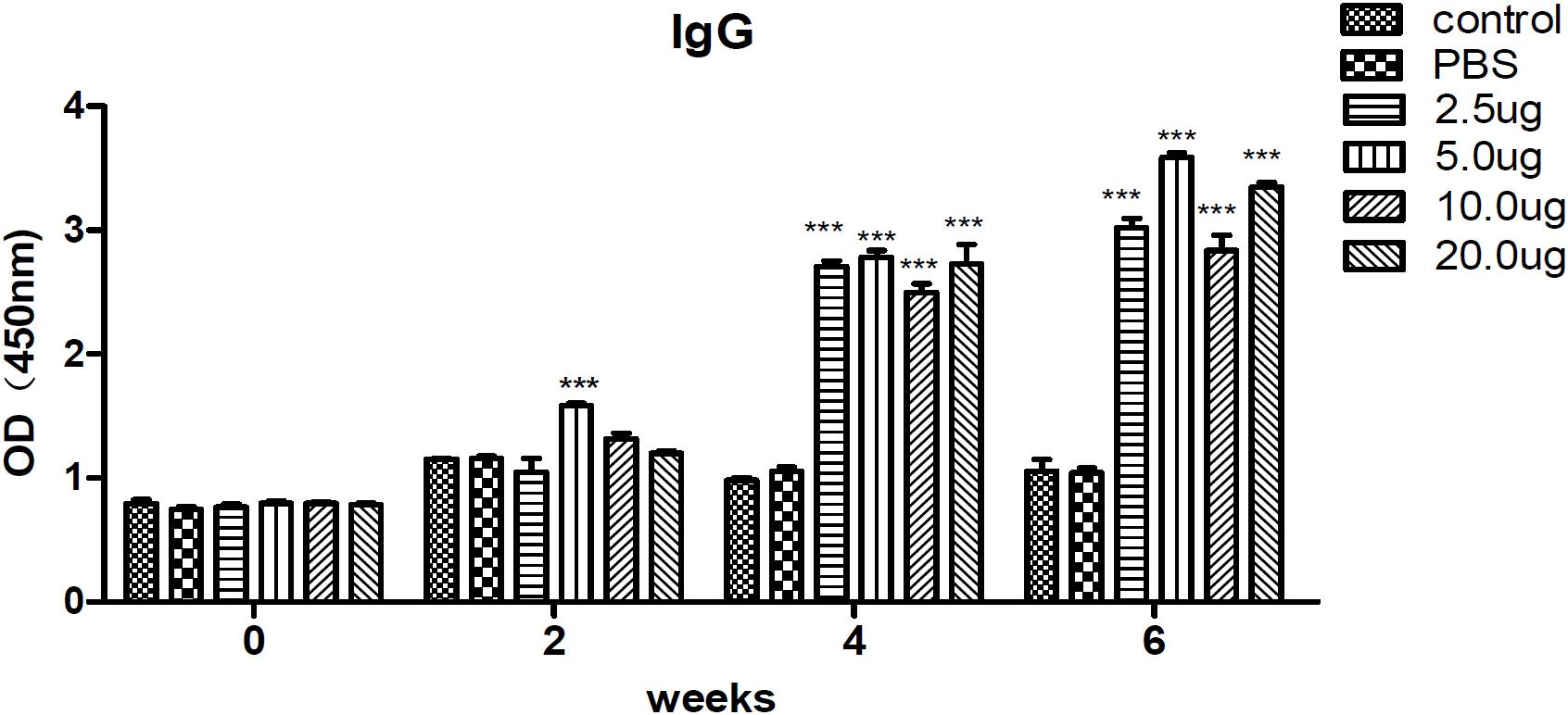
Figure 3. Detection of specific IgG antibodies in mice sera. IgG antibodies were determined and evaluated by ELISA. Serum samples of each group were collected and detected on the preinjection, second, fourth, and sixth week after immunization. The results are expressed as the mean ± SD (n = 3). Statistical differences are indicated by ∗∗∗ (highly significant; p < 0.001) compared with the PBS group.
Evaluation of Lymphocyte Proliferation
Splenocytes from each group were cultured to evaluate the antigen-specific lymphocyte proliferative response by CCK-8 assay. As expected, there was no significant difference between the two control groups (the blank and PBS control groups). However, lymphocyte proliferation in vitro assays revealed that splenic lymphocytes from mice immunized with 5 μg or 10 μg rTgMIF had a significantly higher proliferative response compared with the blank or PBS control groups (Figure 4). These results suggested that immunization with rTgMIF induced antigen-specific lymphocytes in mice.
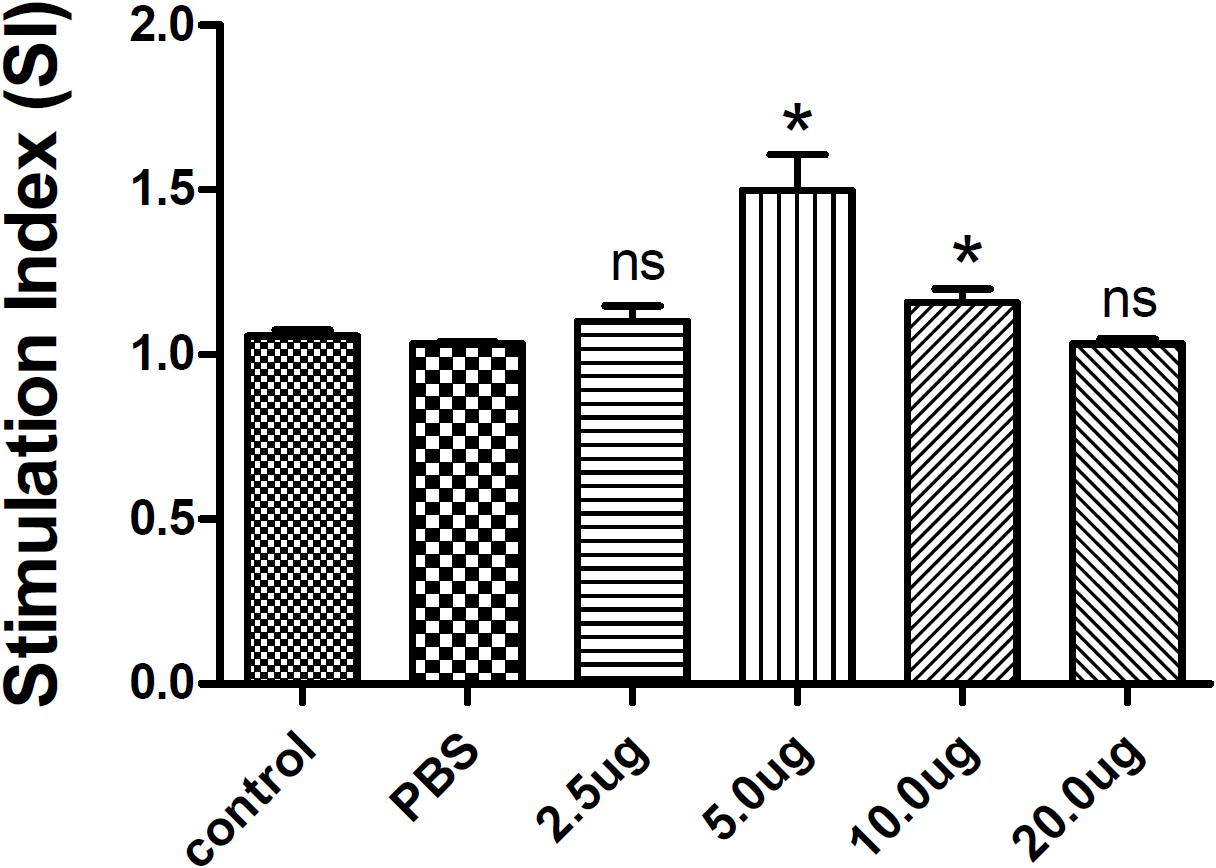
Figure 4. Splenocyte proliferation assay in mice. Two weeks after the last immunization, spleen lymphocytes were collected from vaccinated mice. The proliferative response was evaluated by CCK-8 assay. The results are expressed as the stimulation index (SI) ± SD (n = 3). Statistical differences are represented by ∗(p < 0.05) in comparison with the PBS control group. ns: no significant.
Expression of Cytokines
The cell-mediated immunity induced in the immunized mice was evaluated by measuring the levels of cytokines released in the supernatants of cultures of rTgMIF-stimulated spleen cells. The levels of IFN-γ and IL-4 secreted from the spleen cells of all of the rTgMIF-immunized mice were increased compared with the PBS group, and 5 μg of rTgMIF elicited cell-mediated immunity more efficiently compared to the other dosages (Figure 5). It is known that IFN-γ favors Th1-type immune responses, whereas IL-4 is correlated with a Th2-type response. The rTgMIF vaccine induced high levels of IFN-γ and slight higher low IL-4 levels, indicating that a bias toward the Th1-type immune response was induced by rTgMIF vaccines. Therefore, these data indicated that the cellular immune response induced by rTgMIF was oriented toward a Th1 profile in the immunized mice.
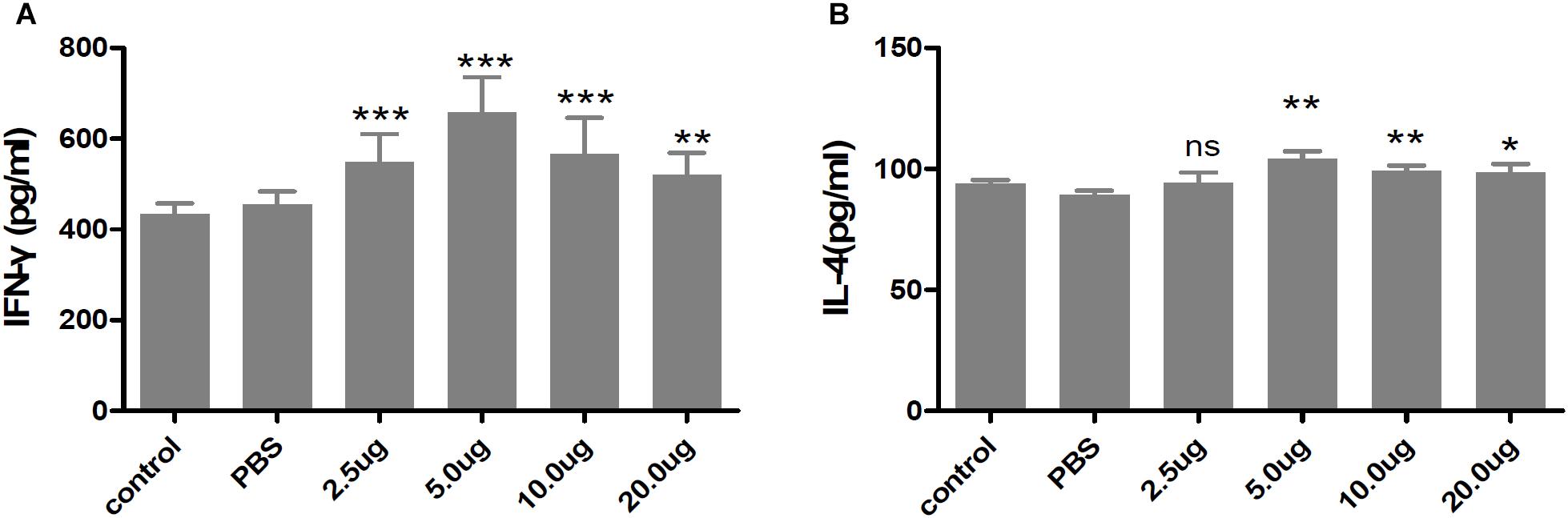
Figure 5. Cytokines secreted by splenocytes of mice immunized with rTgMIF protein. (A) IFN-γ. (B) IL-4. Each bar represents the mean ± SD (n = 9). ∗∗∗p < 0.001, ∗∗p < 0.01, ∗p < 0.05, ns: no significant.
Evaluation of Protective Activity in BALB/c Mice
To evaluate whether the rTgMIF protein could confer effective protection against acute toxoplasmosis, different groups were injected with 1 × 103 tachyzoites of the virulent RH strain intraperitoneally. The survival curves of mice from different groups are shown in Figure 6. Mice in the groups of blank control and PBS died from 7 to 10 days after the challenge, and there were no survivors in either group. The two groups showed no significant difference in survival time (median survival of 8 and 9 days, respectively). It was revealed that the mice immunized with 5 μg rTgMIF (median survival of 11 days) had a significantly prolonged survival rate in comparison to the two control groups (blank control or PBS) (p < 0.0001, respectively). Surprisingly, 25% of the immunized mice survived to the 30th day. These results demonstrated that rTgMIF conferred some degree of protection against T. gondii acute infection in mice.
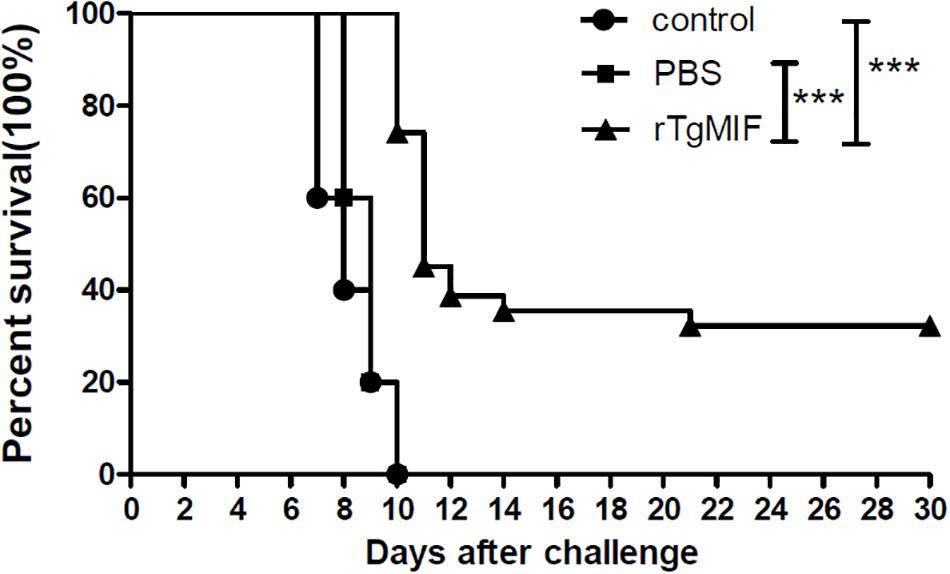
Figure 6. Survival rates of the immunized BALB/c mice. Three groups of mice (n = 30) were challenged with 1 × 103 tachyzoites of T. gondii virulent RH strain intraperitoneally 2 weeks after the last immunization. Mice immunized with rTgMIF exhibited a significant increase in the survival days (median survival of 11 days) in comparison to the blank control or PBS group (median survival of 8 and 9 days, respectively). ∗∗∗p < 0.001, Gehan–Breslow–Wilcoxon tests.
Furthermore, the protein vaccine was evaluated against T. gondii chronic infection. Mice were orally challenged with 20 cysts of the T. gondii PRU strain. One month after infection, the number of brain cysts was counted. As shown in Figure 7, the number of cysts in mice immunized with rTgMIF (1333.33 ± 352.8) was significantly lower compared with the groups of blank control (4400 ± 916.5) and PBS (3533.33 ± 290.6) (p < 0.01). The number of brain cysts in vaccinated mice showed a 62.26% reduction compared with the PBS group. In addition, the size of the brain cysts in vaccinated mice was obviously smaller than that in the two control groups (data not shown). These results revealed that rTgMIF protein enhanced the immunity of mice against T. gondii chronic challenge.
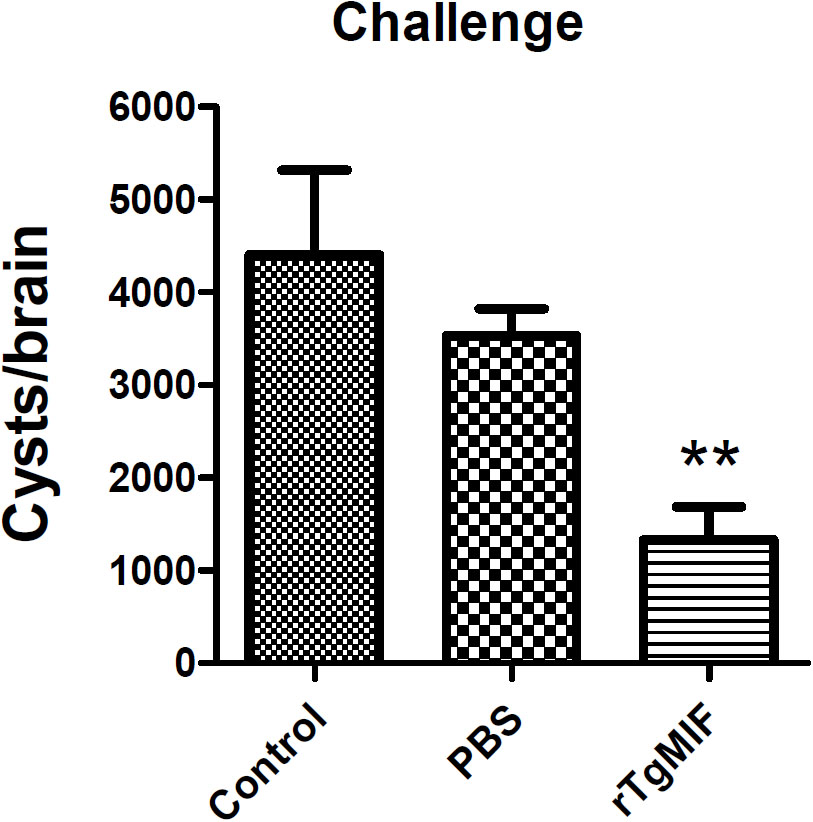
Figure 7. Total brain cysts in mice after challenge infection. Mice were orally challenged with 20 tissue cysts of the T. gondii PRU strain 2 weeks after the last immunization. The number of brain cysts was counted, and the results are expressed as the mean ± SD (n = 3). Statistical differences are represented by ∗∗ (p < 0.01).
Discussion
Toxoplasma gondii is a ubiquitous intracellular apicomplexan parasite that infects many species of animals from diverse geographic regions; thus, it is necessary to develop effective vaccines to prevent and control the spread of toxoplasmosis. The only commercially live attenuated vaccine, Toxovax®, derived from the attenuated tachyzoites of the T. gondii S48 strain, has been used to reduce abortion in sheep (Buxton and Innes, 1995). In addition, genetically modified parasites, such as the uracil auxotroph mutant, also have yielded promising results in the experimental setting (Fox and Bzik, 2002, 2010, 2015; Xia et al., 2018). However, these vaccines may revert to a pathogenic strain and therefore are not suitable for human use. To date, considerable research has been conducted on DNA vaccines or DNA-cocktail vaccines, with few protein vaccines being tested (Yan et al., 2012; Chen et al., 2013, 2015; Hassan et al., 2014; Zhang et al., 2015; Zhang N.Z. et al., 2018; Zhang Z. et al., 2018). It is well-known that protein vaccines elicit strong humoral responses by inducing antigen-specific antibodies. In this work, a recombinant protein vaccine containing the ORF of TgMIF was prepared.
Migration inhibitory factor is an ancient pro-inflammatory mammalian cytokine with multiple functions, such as pro-inflammatory, chemotactic and growth-promoting activities. In addition, MIF has gained substantial attention as a pivotal upstream mediator of innate and adaptive immune responses. The proinflammatory activity of MIF has been implicated in a diverse range of biological processes including activation of ERK/MAPK pathways and inhibition of the anti-inflammatory actions of glucocorticoids. MIF has also been shown to induce the production of downstream pro-inflammatory gene expression, such as TNF-α, IL-8, and IL-17 (Calandra and Roger, 2003; Larson and Horak, 2006; Hertelendy et al., 2018). MIF plays an important role in host defense against a variety of microorganisms including protozoan parasites (Satoskar et al., 2001; Calandra and Roger, 2003; Magalhaes et al., 2009; Cavalcanti et al., 2011). Previous study revealed that MIF is essential for controlling parasites burden with increasing inflammatory response and tissue damage after oral infection with T. gondii (Cavalcanti et al., 2011). Additionally, it is revealed that that MIF-induced early dendritic cell maturation and IL-12 production mediate resistance to T. gondii infection (Terrazas et al., 2010). Recent study suggested that the host NADPH oxidase 4 is required for the generation of MIF and host defense against T. gondii infection (Kim et al., 2017). Therefore, the host MIF participates in anti-T. gondii defense. Interestingly, some microbial pathogens also express a MIF-like protein. Homologs of MIF have been discovered in many parasitic species infecting mammalian hosts and it has been suggested that parasites express MIF to manipulate the host immune response during infection (Zang et al., 2002; Flores et al., 2008; Vermeire et al., 2008; Sparkes et al., 2017). However, not surprisingly, MIF encoded by the hosts and microbial pathogens display distinct immunoregulatory properties. For example, Chicken macrophages treated with chicken MIF (C.MIF) protein expressed increased levels of IL-6, IL-17, and decreased level of IL-8, whereas Eimeria-encoded MIF (E.MIF) treatment only down-regulated IL-8. Moreover, E.MIF, but not C.MIF, enhanced protection against experimental Eimeria infection (Jang et al., 2011). Interspecies sequence alignments revealed that TgMIF has 26% identity with mammalian MIFs from host species (Sommerville et al., 2013). In this study, the protein sequence of TgMIF was analyzed by DNASTAR. The results suggested that most regions of the TgMIF protein were in hydrophilicity plots and flexible regions, and TgMIF had an excellent antigenic index and surface probability, indicating the positive antigenicity of TgMIF. Moreover, TgMIF shows 99.7% nucleotide sequence identity and 100% identity of amino acids from all three strains, indicating it can be considered as a vaccine candidate against various strains of T. gondii. Recently, Plasmodium-encoded MIF ortholog was found to be a good vaccine conferring complete protection against malaria re-infection (Baeza Garcia et al., 2018). Therefore, we speculate that MIF ortholog from T. gondii may be an ideal material for producing an anti-T. gondii protein vaccine.
In our study, 25% of immunized mice survived to the 30th day after challenge infection with type 1 RH tachyzoites, whereas there were no survivors in the control groups. These results suggested that rTgMIF protein triggered a protective effect against type 1 acute toxoplasmosis. In chronic infection with type 2 PRU bradyzoites, the data indicated that the rTgMIF protein vaccine caused a 62.26% reduction in parasite brain cysts compared to the control groups (p < 0.01). Therefore, these results revealed that the rTgMIF protein induced a protective effect against type 1 and 2 strains.
Cytokines are critical to promote T helper cell activation. Previous studies have shown that IFN-γ is associated with a Th1 immune response, whereas IL-4 is associated with a Th2 response (Romagnani, 1994; Abbas et al., 1996). IL-4 counteracts the secretion of IFN-γ and facilitates B cell generation, differentiation, and maturation (Chapoval et al., 2010; Bao and Cao, 2014). Most importantly, IFN-γ is a crucial mediator of immune responses against T. gondii during the whole period of infection (Dupont et al., 2012; Yarovinsky, 2014). In our study, IFN-γ and IL-4 were quantitatively tested to evaluate the protective effect of the vaccine. Our results revealed that 5 μg of rTgMIF evoked higher levels of IFN-γ and slight higher levels of IL-4 from immunized mice in comparison with other groups. Therefore, our findings are consistent with previous reports that mice immunized with recombinant proteins generated a mixed Th1/Th2-type immune response (Wang et al., 2014; Ching et al., 2016; Sonaimuthu et al., 2016). Meanwhile, many studies have also shown that a Th1-predominant immune response is more critical for effective protection against T. gondii infection (Lieberman et al., 2004; Kemball et al., 2007; Suzuki et al., 2011; Dupont et al., 2012; Sturge et al., 2013; Lopez-Yglesias et al., 2018). The rTgMIF vaccine induced high levels of IFN-γ and slight higher IL-4 levels, indicating that a bias toward the Th1-type immune response was induced by rTgMIF vaccines. As is well-known, specific IgG antibodies exert an important role in controlling T. gondii infection in cooperation with macrophages for resisting secondary invasion (Johnson et al., 2004; Dupont et al., 2012; Wang et al., 2013, 2017). The IgG antibody mainly inhibits the adhesion of the parasite to host cell receptors and eliminates the parasites (Zhou and Wang, 2017). Therefore, we analyzed a specific IgG antibody. The dose-dependent results indicated that anti-MIF IgG antibodies were generated at 2 weeks after preinjection with 5 μg rTgMIF. The levels of IgG antibodies from the immunized groups were higher compared with mice of the control groups (p < 0.0001). These findings indicated that the vaccine could trigger stronger humoral and cellular immune responses against T. gondii infection.
To further evaluate the protective effect of rTgMIF protein vaccines against T. gondii infection, the best immunization dose (5 μg rTgMIF) was utilized to perform the experiment. Prolonged survival was observed in immunized BALB/c mice, with approximately 25% of the immunized mice remaining alive at the end of the analysis period. In chronic infection challenge, the data indicated that the rTgMIF protein vaccine caused a 62.26% reduction in the number of parasite brain cysts compared to the PBS group (p < 0.01), which were higher than previous studies using other antigens (Hu et al., 2017; Yang et al., 2017; Zhou and Wang, 2017; Gao et al., 2018). The result suggested that rTgMIF protein could strongly induce protective effect against chronic toxoplasmosis. Together these results revealed that the rTgMIF protein provided partial but effective protection against both chronic and acute T. gondii infection.
Conclusion
Our research demonstrated that rTgMIF successfully triggered a strong and specific immune response, and the vaccinated BALB/c mice had a certain protective effect against acute and chronic T. gondii infection. Therefore, rTgMIF should be considered to be a potential vaccine candidate against toxoplasmosis. Complete protection could be achieved by binding TgMIF to other immunogenic antigens against T. gondii challenge in the future.
Author Contributions
JD and JS conceived and designed the experiments. KL, HW, LC, HC, and MW performed the experiments. KL, LC, HW, HC, MW, RA, DC, and LY analyzed the data. KL, HW, LC, MW, and JD wrote and critically revised the manuscript. All authors read and approved the final version of the manuscript.
Funding
This work was supported by the National Natural Science Foundation of China (81871674, 81672046 to JD), and the Academic and Technology Leaders reserve candidate Fund of Anhui Province (2016H080 to JD); the key project of outstanding young talent support program in Anhui province university (gxyqZD2016047 to JD); the National Natural Science Foundation of Anhui Province (1708085MH190 to LC).
Conflict of Interest Statement
The authors declare that the research was conducted in the absence of any commercial or financial relationships that could be construed as a potential conflict of interest.
References
Abbas, A. K., Murphy, K. M., and Sher, A. (1996). Functional diversity of helper T lymphocytes. Nature 383, 787–793. doi: 10.1038/383787a0
Baeza Garcia, A., Siu, E., Sun, T., Exler, V., Brito, L., Hekele, A., et al. (2018). Neutralization of the plasmodium-encoded MIF ortholog confers protective immunity against malaria infection. Nat. Commun. 9:2714. doi: 10.1038/s41467-018-05041-7
Bao, Y., and Cao, X. (2014). The immune potential and immunopathology of cytokine-producing B cell subsets: a comprehensive review. J. Autoimmun. 55, 10–23. doi: 10.1016/j.jaut.2014.04.001
Buxton, D., and Innes, E. A. (1995). A commercial vaccine for ovine toxoplasmosis. Parasitology 110, S11–S16.
Calandra, T., and Roger, T. (2003). Macrophage migration inhibitory factor: a regulator of innate immunity. Nat. Rev. Immunol. 3, 791–800. doi: 10.1038/nri1200
Cavalcanti, M. G., Mesquita, J. S., Madi, K., Feijo, D. F., Assuncao-Miranda, I., Souza, H. S., et al. (2011). MIF participates in Toxoplasma gondii-induced pathology following oral infection. PLoS One 6:e25259. doi: 10.1371/journal.pone.0025259
Chapoval, S., Dasgupta, P., Dorsey, N. J., and Keegan, A. D. (2010). Regulation of the T helper cell type 2 (Th2)/T regulatory cell (Treg) balance by IL-4 and STAT6. J. Leukoc. Biol. 87, 1011–1018. doi: 10.1189/jlb.1209772
Chen, J., Huang, S. Y., Li, Z. Y., Yuan, Z. G., Zhou, D. H., Petersen, E., et al. (2013). Protective immunity induced by a DNA vaccine expressing eIF4A of Toxoplasma gondii against acute toxoplasmosis in mice. Vaccine 31, 1734–1739. doi: 10.1016/j.vaccine.2013.01.027
Chen, J., Li, Z. Y., Petersen, E., Huang, S. Y., Zhou, D. H., and Zhu, X. Q. (2015). DNA vaccination with genes encoding Toxoplasma gondii antigens ROP5 and GRA15 induces protective immunity against toxoplasmosis in Kunming mice. Expert Rev. Vaccines 14, 617–624. doi: 10.1586/14760584.2015.1011133
Ching, X. T., Fong, M. Y., and Lau, Y. L. (2016). Evaluation of immunoprotection conferred by the subunit vaccines of GRA2 and GRA5 against acute toxoplasmosis in BALB/c Mice. Front. Microbiol. 7:609. doi: 10.3389/fmicb.2016.00609
Czarnewski, P., Araujo, E. C. B., Oliveira, M. C., Mineo, T. W. P., and Silva, N. M. (2017). Recombinant TgHSP70 immunization protects against Toxoplasma gondii brain cyst formation by enhancing inducible nitric oxide expression. Front. Cell. Infect. Microbiol. 7:142. doi: 10.3389/fcimb.2017.00142
de Oliveira Gomes, A., de Oliveira Silva, D. A., Silva, N. M., de Freitas Barbosa, B., Franco, P. S., Angeloni, M. B., et al. (2011). Effect of macrophage migration inhibitory factor (MIF) in human placental explants infected with Toxoplasma gondii depends on gestational age. Am. J. Pathol. 178, 2792–2801. doi: 10.1016/j.ajpath.2011.02.005
Dubey, J. P., Hill, D. E., Jones, J. L., Hightower, A. W., Kirkland, E., Roberts, J. M., et al. (2005). Prevalence of viable Toxoplasma gondii in beef, chicken, and pork from retail meat stores in the United States: risk assessment to consumers. J. Parasitol. 91, 1082–1093. doi: 10.1645/GE-683.1
Dupont, C. D., Christian, D. A., and Hunter, C. A. (2012). Immune response and immunopathology during toxoplasmosis. Semin. Immunopathol. 34, 793–813. doi: 10.1007/s00281-012-0339-3
Flores, M., Saavedra, R., Bautista, R., Viedma, R., Tenorio, E. P., Leng, L., et al. (2008). Macrophage migration inhibitory factor (MIF) is critical for the host resistance against Toxoplasma gondii. FASEB J. 22, 3661–3671. doi: 10.1096/fj.08-111666
Fox, B. A., and Bzik, D. J. (2002). De novo pyrimidine biosynthesis is required for virulence of Toxoplasma gondii. Nature 415, 926–929. doi: 10.1038/415926a
Fox, B. A., and Bzik, D. J. (2010). Avirulent uracil auxotrophs based on disruption of orotidine-5′-monophosphate decarboxylase elicit protective immunity to Toxoplasma gondii. Infect. Immun. 78, 3744–3752. doi: 10.1128/IAI.00287-10
Fox, B. A., and Bzik, D. J. (2015). Nonreplicating, cyst-defective type II Toxoplasma gondii vaccine strains stimulate protective immunity against acute and chronic infection. Infect. Immun. 83, 2148–2155. doi: 10.1128/IAI.02756-14
Gao, Q., Zhang, N. Z., Zhang, F. K., Wang, M., Hu, L. Y., and Zhu, X. Q. (2018). Immune response and protective effect against chronic Toxoplasma gondii infection induced by vaccination with a DNA vaccine encoding profilin. BMC Infect. Dis. 18:117. doi: 10.1186/s12879-018-3022-z
Gomes, A. O., Barbosa, B. F., Franco, P. S., Ribeiro, M., Silva, R. J., Gois, P. S. G., et al. (2018). Macrophage migration inhibitory factor (MIF) prevents maternal death, but contributes to poor fetal outcome during congenital toxoplasmosis. Front. Microbiol. 9:906. doi: 10.3389/fmicb.2018.00906
Hassan, I. A., Wang, S., Xu, L., Yan, R., Song, X., and Li, X. (2014). DNA vaccination with a gene encoding Toxoplasma gondii deoxyribose phosphate aldolase (TgDPA) induces partial protective immunity against lethal challenge in mice. Parasit. Vectors 7, 431. doi: 10.1186/1756-3305-7-431
Hertelendy, J., Reumuth, G., Simons, D., Stoppe, C., Kim, B. S., Stromps, J. P., et al. (2018). Macrophage migration inhibitory factor - a favorable marker in inflammatory diseases? Curr. Med. Chem. 25, 601–605. doi: 10.2174/0929867324666170714114200
Hu, L. Y., Zhang, N. Z., Zhang, F. K., Wang, M., Gao, Q., Wang, J. L., et al. (2017). Resistance to chronic Toxoplasma gondii infection induced by a DNA vaccine expressing GRA16. Biomed. Res. Int. 2017, 1295038. doi: 10.1155/2017/1295038
Innes, E. A. (2010). Vaccination against Toxoplasma gondii: an increasing priority for collaborative research? Expert Rev. Vaccines 9, 1117–1119. doi: 10.1586/erv.10.113
Jang, S. I., Lillehoj, H. S., Lee, S. H., Kim, D. K., Pages, M., Hong, Y. H., et al. (2011). Distinct immunoregulatory properties of macrophage migration inhibitory factors encoded by Eimeria parasites and their chicken host. Vaccine 29, 8998–9004. doi: 10.1016/j.vaccine.2011.09.038
Johnson, L. L., Lanthier, P., Hoffman, J., and Chen, W. (2004). Vaccination protects B cell-deficient mice against an oral challenge with mildly virulent Toxoplasma gondii. Vaccine 22, 4054–4061. doi: 10.1016/j.vaccine.2004.03.056
Kemball, C. C., Pack, C. D., Guay, H. M., Li, Z. N., Steinhauer, D. A., Szomolanyi-Tsuda, E., et al. (2007). The antiviral CD8+ T cell response is differentially dependent on CD4+ T cell help over the course of persistent infection. J. Immunol. 179, 1113–1121. doi: 10.4049/jimmunol.179.2.1113
Kim, J. H., Lee, J., Bae, S. J., Kim, Y., Park, B. J., Choi, J. W., et al. (2017). NADPH oxidase 4 is required for the generation of macrophage migration inhibitory factor and host defense against Toxoplasma gondii infection. Sci. Rep. 7:6361. doi: 10.1038/s41598-017-06610-4
Kur, J., Holec-Gasior, L., and Hiszczynska-Sawicka, E. (2009). Current status of toxoplasmosis vaccine development. Expert Rev. Vaccines 8, 791–808. doi: 10.1586/erv.09.27
Larson, D. F., and Horak, K. (2006). Macrophage migration inhibitory factor: controller of systemic inflammation. Crit. Care 10:138. doi: 10.1186/cc4899
Lieberman, L. A., Banica, M., Reiner, S. L., and Hunter, C. A. (2004). STAT1 plays a critical role in the regulation of antimicrobial effector mechanisms, but not in the development of Th1-type responses during toxoplasmosis. J. Immunol. 172, 457–463. doi: 10.4049/jimmunol.172.1.457
Liu, Q., Singla, L. D., and Zhou, H. (2012). Vaccines against Toxoplasma gondii: status, challenges and future directions. Hum. Vaccin. Immunother. 8, 1305–1308. doi: 10.4161/hv.21006
Lopez-Yglesias, A. H., Burger, E., Araujo, A., Martin, A. T., and Yarovinsky, F. (2018). T-bet-independent Th1 response induces intestinal immunopathology during Toxoplasma gondii infection. Mucosal Immunol. 11, 921–931. doi: 10.1038/mi.2017.102
Magalhaes, E. S., Paiva, C. N., Souza, H. S., Pyrrho, A. S., Mourao-Sa, D., Figueiredo, R. T., et al. (2009). Macrophage migration inhibitory factor is critical to interleukin-5-driven eosinophilopoiesis and tissue eosinophilia triggered by Schistosoma mansoni infection. FASEB J. 23, 1262–1271. doi: 10.1096/fj.08-124248
Martin, V., Supanitsky, A., Echeverria, P. C., Litwin, S., Tanos, T., De Roodt, A. R., et al. (2004). Recombinant GRA4 or ROP2 protein combined with alum or the gra4 gene provides partial protection in chronic murine models of toxoplasmosis. Clin. Diagn. Lab. Immunol. 11, 704–710. doi: 10.1128/CDLI.11.4.704-710.2004
Mitchell, R. A., Liao, H., Chesney, J., Fingerle-Rowson, G., Baugh, J., David, J., et al. (2002). Macrophage migration inhibitory factor (MIF) sustains macrophage proinflammatory function by inhibiting p53: regulatory role in the innate immune response. Proc. Natl. Acad. Sci. U.S.A. 99, 345–350. doi: 10.1073/pnas.012511599
Montoya, J. G., and Liesenfeld, O. (2004). Toxoplasmosis. Lancet 363, 1965–1976. doi: 10.1016/s0140-6736(04)16412-x
Munoz, M., Liesenfeld, O., and Heimesaat, M. M. (2011). Immunology of Toxoplasma gondii. Immunol. Rev. 240, 269–285. doi: 10.1111/j.1600-065X.2010.00992.x
Petersen, E., Pollak, A., and Reiter-Owona, I. (2001). Recent trends in research on congenital toxoplasmosis. Int. J. Parasitol. 31, 115–144. doi: 10.1016/s0020-7519(00)00140-5
Pinzan, C. F., Sardinha-Silva, A., Almeida, F., Lai, L., Lopes, C. D., Lourenco, E. V., et al. (2015). Vaccination with recombinant microneme proteins confers protection against experimental toxoplasmosis in mice. PLoS One 10:e0143087. doi: 10.1371/journal.pone.0143087
Qu, D., Han, J., and Du, A. (2013). Evaluation of protective effect of multiantigenic DNA vaccine encoding MIC3 and ROP18 antigen segments of Toxoplasma gondii in mice. Parasitol. Res. 112, 2593–2599. doi: 10.1007/s00436-013-3425-0
Romagnani, S. (1994). Lymphokine production by human T cells in disease states. Annu. Rev. Immunol. 12, 227–257. doi: 10.1146/annurev.iy.12.040194.001303
Satoskar, A. R., Bozza, M., Rodriguez Sosa, M., Lin, G., and David, J. R. (2001). Migration-inhibitory factor gene-deficient mice are susceptible to cutaneous Leishmania major infection. Infect. Immun. 69, 906–911. doi: 10.1128/IAI.69.2.906-911.2001
Sommerville, C., Richardson, J. M., Williams, R. A., Mottram, J. C., Roberts, C. W., Alexander, J., et al. (2013). Biochemical and immunological characterization of Toxoplasma gondii macrophage migration inhibitory factor. J. Biol. Chem. 288, 12733–12741. doi: 10.1074/jbc.M112.419911
Sonaimuthu, P., Ching, X. T., Fong, M. Y., Kalyanasundaram, R., and Lau, Y. L. (2016). Induction of protective immunity against toxoplasmosis in BALB/c mice vaccinated with Toxoplasma gondii Rhoptry-1. Front. Microbiol. 7:808. doi: 10.3389/fmicb.2016.00808
Sparkes, A., De Baetselier, P., Roelants, K., De Trez, C., Magez, S., Van Ginderachter, J., et al. (2017). The non-mammalian MIF superfamily. Immunobiology 222, 473–482. doi: 10.1016/j.imbio.2016.10.006
Sturge, C. R., Benson, A., Raetz, M., Wilhelm, C. L., Mirpuri, J., Vitetta, E. S., et al. (2013). TLR-independent neutrophil-derived IFN-gamma is important for host resistance to intracellular pathogens. Proc. Natl. Acad. Sci. U.S.A. 110, 10711–10716. doi: 10.1073/pnas.1307868110
Suzuki, Y., Sa, Q., Gehman, M., and Ochiai, E. (2011). Interferon-gamma- and perforin-mediated immune responses for resistance against Toxoplasma gondii in the brain. Expert Rev. Mol. Med. 13:e31. doi: 10.1017/S1462399411002018
Terrazas, C. A., Juarez, I., Terrazas, L. I., Saavedra, R., Calleja, E. A., and Rodriguez-Sosa, M. (2010). Toxoplasma gondii: impaired maturation and pro-inflammatory response of dendritic cells in MIF-deficient mice favors susceptibility to infection. Exp. Parasitol. 126, 348–358. doi: 10.1016/j.exppara.2010.03.009
Vermeire, J., Cho, Y., Lolis, E., Bucala, R., and Cappello, M. (2008). Orthologs of macrophage migration inhibitory factor from parasitic nematodes. Trends Parasitol. 24, 355–363. doi: 10.1016/j.pt.2008.04.007
Wang, H. L., Li, Y. Q., Yin, L. T., Meng, X. L., Guo, M., Zhang, J. H., et al. (2013). Toxoplasma gondii protein disulfide isomerase (TgPDI) is a novel vaccine candidate against toxoplasmosis. PLoS One 8:e70884. doi: 10.1371/journal.pone.0070884
Wang, H. L., Zhang, T. E., Yin, L. T., Pang, M., Guan, L., Liu, H. L., et al. (2014). Partial protective effect of intranasal immunization with recombinant Toxoplasma gondii rhoptry protein 17 against toxoplasmosis in mice. PLoS One 9:e108377. doi: 10.1371/journal.pone.0108377
Wang, J. L., Huang, S. Y., Behnke, M. S., Chen, K., Shen, B., and Zhu, X. Q. (2016). The past, present, and future of genetic manipulation in Toxoplasma gondii. Trends Parasitol. 32, 542–553. doi: 10.1016/j.pt.2016.04.013
Wang, S., Zhang, Z., Wang, Y., Gadahi, J. A., Xu, L., Yan, R., et al. (2017). Toxoplasma gondii elongation factor 1-Alpha (TgEF-1alpha) is a novel vaccine candidate antigen against toxoplasmosis. Front. Microbiol. 8:168. doi: 10.3389/fmicb.2017.00168
Xia, N., Zhou, T., Liang, X., Ye, S., Zhao, P., Yang, J., et al. (2018). A lactate fermentation mutant of toxoplasma stimulates protective immunity against acute and chronic toxoplasmosis. Front. Immunol. 9:1814. doi: 10.3389/fimmu.2018.01814
Yan, H. K., Yuan, Z. G., Song, H. Q., Petersen, E., Zhou, Y., Ren, D., et al. (2012). Vaccination with a DNA vaccine coding for perforin-like protein 1 and MIC6 induces significant protective immunity against Toxoplasma gondii. Clin. Vaccine Immunol. 19, 684–689. doi: 10.1128/CVI.05578-11
Yang, W. B., Zhou, D. H., Zou, Y., Chen, K., Liu, Q., Wang, J. L., et al. (2017). Vaccination with a DNA vaccine encoding Toxoplasma gondii ROP54 induces protective immunity against toxoplasmosis in mice. Acta Trop. 176, 427–432. doi: 10.1016/j.actatropica.2017.09.007
Yarovinsky, F. (2014). Innate immunity to Toxoplasma gondii infection. Nat. Rev. Immunol. 14, 109–121. doi: 10.1038/nri3598
Zang, X., Taylor, P., Wang, J. M., Meyer, D. J., Scott, A. L., Walkinshaw, M. D., et al. (2002). Homologues of human macrophage migration inhibitory factor from a parasitic nematode. Gene cloning, protein activity, and crystal structure. J. Biol. Chem. 277, 44261–44267. doi: 10.1074/jbc.M204655200
Zhang, N. Z., Gao, Q., Wang, M., Elsheikha, H. M., Wang, B., Wang, J. L., et al. (2018). Immunization With a DNA vaccine cocktail encoding TgPF, TgROP16, TgROP18, TgMIC6, and TgCDPK3 genes protects mice against chronic toxoplasmosis. Front. Immunol. 9:1505. doi: 10.3389/fimmu.2018.01505
Zhang, Z., Li, Y., Wang, M., Xie, Q., Li, P., Zuo, S., et al. (2018). Immune protection of rhoptry protein 21 (ROP21) of Toxoplasma gondii as a DNA vaccine against toxoplasmosis. Front. Microbiol. 9:909. doi: 10.3389/fmicb.2018.00909
Zhang, N. Z., Xu, Y., Wang, M., Petersen, E., Chen, J., Huang, S. Y., et al. (2015). Protective efficacy of two novel DNA vaccines expressing Toxoplasma gondii rhomboid 4 and rhomboid 5 proteins against acute and chronic toxoplasmosis in mice. Expert Rev. Vaccines 14, 1289–1297. doi: 10.1586/14760584.2015.1061938
Keywords: T. gondii, toxoplasmosis, TgMIF, protein vaccine, protective efficacy
Citation: Liu K, Wen H, Cai H, Wu M, An R, Chu D, Yu L, Shen J, Chen L and Du J (2019) Protective Effect Against Toxoplasmosis in BALB/c Mice Vaccinated With Toxoplasma gondii Macrophage Migration Inhibitory Factor. Front. Microbiol. 10:813. doi: 10.3389/fmicb.2019.00813
Received: 26 December 2018; Accepted: 01 April 2019;
Published: 24 April 2019.
Edited by:
José Roberto Mineo, Federal University of Uberlândia, BrazilReviewed by:
Marcelo Torres Bozza, Federal University of Rio de Janeiro, BrazilShenyi He, Shandong University, China
Gang Lu, Taishan Medical University, China
Copyright © 2019 Liu, Wen, Cai, Wu, An, Chu, Yu, Shen, Chen and Du. This is an open-access article distributed under the terms of the Creative Commons Attribution License (CC BY). The use, distribution or reproduction in other forums is permitted, provided the original author(s) and the copyright owner(s) are credited and that the original publication in this journal is cited, in accordance with accepted academic practice. No use, distribution or reproduction is permitted which does not comply with these terms.
*Correspondence: Lijian Chen, chenlijian77@126.com Jian Du, dujane@163.com; dujian@ahmu.edu.cn
†These authors have contributed equally to this work