- 1CIBIO/InBIO, Centro de Investigação em Biodiversidade e Recursos Genéticos da Universidade do Porto, Porto, Portugal
- 2Laboratoire d’Eco-Ethologie, Institut de Biologie, Université de Neuchâtel, Neuchâtel, Switzerland
- 3Computational Biology Institute, Department of Epidemiology and Biostatistics, Milken Institute School of Public Health, The George Washington University, Ashburn, VA, United States
The broadstripe cleaning goby Elacatinus prochilos has two alternative ecotypes: sponge-dwellers, which live in large groups and feed mainly upon nematode parasites; and coral-dwellers, that live in small groups or in solitude and behave as cleaners. Recent studies focusing on the skin and gut microbiomes of tropical fish showed that microbial communities are influenced mainly by diet and host species. Here, we compare the skin and gut microbiomes of the Caribbean broadstripe cleaning goby E. prochilos alternative ecotypes (cleaners and non-cleaners) from Barbados and predict that different habitat use and behavior (cleaning vs. non-cleaning) will translate in different bacterial profiles between the two ecotypes. We found significant differences in both alpha- and beta-diversity of skin and gut microbiomes belonging to different ecotypes. Importantly, the skin microbiome of obligate cleaners showed greater intra-sample diversity and harbored a significantly higher prevalence of potential fish pathogens. Likewise, potential pathogens were also more prevalent in the gut of obligate cleaners. We suggest that habitat use, diet, but also direct contact with potential diseased clientele during cleaning, could be the cause for these patterns.
Introduction
Cleaner fish are pivotal players in marine ecosystems, providing a valuable service to other fish (known as clients) by removing ectoparasites and dead or damaged tissue (Côté, 2000). Classically described as “doctors of the sea,” these usually smaller-sized fish are conspicuously colorful organisms (Cheney et al., 2009) and are easily identified by client species. Clients then visit their territories (referred as cleaning stations) to be relieved of parasites, and to gain in stress reduction and other putative fitness benefits (Ros et al., 2011; Soares et al., 2011). Indeed, these cleaning interactions influence client growth rate (Clague et al., 2011; Waldie et al., 2011) and affect local populations mobility contributing to the diversity and abundance of juvenile and adult fish (Bshary, 2003; Grutter et al., 2003; Waldie et al., 2011). Additionally, the presence of cleaners is known to influence habitat selection (e.g., processes of settlement and recruitment) of young reef fishes (Sun et al., 2015a,b). Cleaners are primary agents in these mutualistic exchanges, choosing when and how to inspect clients, and determining the quality of these inspections. They can, for instance, select the number and size of the parasites they ingest (Grutter, 1997), decide whether to provide or not physical contact (tactile stimulation, Bshary and Würth, 2001) or the amount of bites given to fish mucus, which is harmful to the clients because mucus protects fish from disease and sunburn (Bshary and Grutter, 2002; Eckes et al., 2015). While there seems to be a great benefit of having such a decisive role, there is also vulnerability when one needs to wait for the food (clients) to visit. For instance, the amount of potential clientele could be determinant to the number of visits and dietary value may fluctuate, as parasite load of clients may change from reef to reef (Cheney and Côté, 2005), but also amongst client species (Soares et al., 2007) or between seasons (Grutter, 1994). Moreover, some clients could actually eat the cleaner (Côté, 2000), and cleaners are not indifferent to the risk involved (Soares et al., 2012). Finally, clients may also be vectors of parasites, bacterial contamination, and disease to cleaners (Grutter, 2002; Jones et al., 2004; Sasal et al., 2005). For example, cleaner fish (e.g., Ctenolabrus rupestris and Centrolabrus exoletus) used in salmon farms can become infected by pathogenic Vibrio sp. and Aeromonas salmonicida, as well as by pancreatic necrosis virus (Treasurer and Laidler, 1994), although little empirical evidence is available on this matter.
Bacterial consortia play an important role at all biological scales from individuals to ecosystems (McFall-Ngai et al., 2013; Gibbons and Gilbert, 2015; Delgado-Baquerizo et al., 2016). Fish microbiome studies have generally shown a high degree of host specificity and specialization across organs (e.g., Lowrey et al., 2015; Pratte et al., 2018; Rosado et al., 2019), as well as a close association between microbiome composition and host ecology. Specifically, host factors (ontogeny and genetic background), environment and diet are considered to be the main drivers of the bacteria consortia present in the fish gut (Tarnecki et al., 2017; Egerton et al., 2018) and skin (Larsen et al., 2013; Chiarello et al., 2018). Recent studies have also revealed crucial connections between animal microbiomes and social behavior; those studies have shown that social interactions and physical proximity can modulate the composition and function of animal microbiomes (White et al., 2010; Koch and Schmid-Hempel, 2011; Tung et al., 2015), and that the microbiome affects social communication by influencing the host central nervous system and peripheral chemical communication (Sharon et al., 2010; Theis et al., 2013; Venu et al., 2014). In fact, microbial transfer between socially interacting partners is beginning to be considered a key driver in the cost–benefit calculus of group network interactions (Koch and Schmid-Hempel, 2011; Tung et al., 2015). While microbe transmission may be beneficial by promoting disease resistance (Endt et al., 2010; Stecher et al., 2010; Koch and Schmid-Hempel, 2011), it may also facilitate pathogen transmission between interacting hosts (Garrett et al., 2010; Elinav et al., 2011). In this respect, cleaners could become good animal models to study the role of microbiome in mutualistic behavior, as both partners come in close physical contact during interactions, allowing for direct microbe transmission (skin-to-skin) and potentially also modulating the gut microbiome of cleaners (Soares et al., 2019).
In gobies, cleaner species occur exclusively in the genus Elacatinus, which include 27 described species that are distributed throughout the western Atlantic Ocean, from North Carolina (United States) to Brazil (Colin, 1975, 2010; Taylor and Hellberg, 2005, 2006; Randall and Lobel, 2009). In Elacatinus gobies there is an association of cleaning behavior with habitat, morphology, and color (Taylor and Hellberg, 2005). The absence of cleaning is associated with sponge-dwelling, while the occurrence of cleaning is related to inhabiting other substrata (Rüber et al., 2003; Taylor and Hellberg, 2005), ideally (but not exclusively) live-coral (Sazima et al., 2000, 2008; Whiteman and Côté, 2002). These alternative ecotypes may also occur intra-specifically (Côté and Soares, 2011), like in the case of the broadstripe cleaning goby E. prochilos (Böhlke and Robins, 1968), which in Barbados is known to occupy sponges (sponge-dwellers) and other alternative substrates (mostly live coral) – herein referred to as coral-dwellers (Whiteman and Côté, 2004b). Remarkable changes occur between these two ecotypes of E. prochilos; sponge-dwellers live in variable (10 up to 80 individuals), dominance-structured groups of conspecifics, foraging on Haplosyllis polychaete worms which parasitize sponges (Colin, 1975; Whiteman and Côté, 2004a). Coral-dwellers, however, are most frequently found in solitary, paired (usually a male-female couple) or in smaller groups of conspecifics, and rely heavily on client-gleaned material as food source (Arnal and Côté, 2000; Whiteman and Côté, 2002). This intraspecific alternative system has been found in other fish species such as Elacatinus evelynae from St. Croix, United States Virgin Islands (White et al., 2007) and Elacatinus figaro from Brazil (Rocha et al., 2000).
Here, we compare the bacterial communities from the skin and gut of the Barbadian E. prochilos alternative ecotypes (sponge- vs. coral-dwelling) sampled in two different localities (biological replicates), to test the hypothesis that different habitat use and behavior will lead to different bacterial profiles in the two ecotypes. Specifically, we hypothesize that the microbiome of cleaners will be enriched by potential pathogens due to frequent contact with diseased clients. To accomplish this aim we will couple high-throughput sequencing of the bacterial 16S rRNA gene V4 region with amplicon sequence variant analysis.
Materials and Methods
Sample Collection and DNA Extraction
Twenty-three E. prochilos specimens were collected in two reefs located on the west coast of Barbados (13 km apart): Speightstown (sponge-dwellers N = 6; coral-dwellers N = 6) (13°15′31.8′′N 59°38′42.6′′W) and Batts Rock (sponge-dwellers N = 6; coral-dwellers N = 5) (13°08′12.6′′N 59°38′16.2′′W). Sponge-dwellers formed groups of 5 up to 80 individuals and were associated to giant barrel sponges (Xestospongia muta) in the patch reef zone (6–10 m depth). In contrast, coral-dwellers were either solitary or in pairs, mainly associated to live coral, but also other substrates like coralline algae and dead coral from the spurs and grooves zone (3–8 m deep). Sponge-dwellers and coral-dwellers were usually found from 50 to 100 m apart. Fish were captured by SCUBA using individual hand nets or plastic bags and transported to the lab inside sealed plastic bags. In the lab, fish were carefully removed from the bags, and without further manipulation, had their skin swiped with cotton swabs at least two times on each body side. Gloves were used during the procedure. Fish were then sacrificed with an overdose of clove oil mixture. Specimens and cotton swabs were then immediately frozen and kept at -20°C until further analysis. Three weeks later, fish were dissected with sterile material and the whole gut was taken. DNA from 23 skin to 23 guts was extracted using the PowerSoil DNA Isolation Kit (QIAGEN, Netherlands), following the manufacturer’s protocol. DNA concentration and quality was measured in a NanoDropTM 2000 Spectrophotometer (Thermo Fisher Scientific, United States). Each DNA sample was PCR amplified for the V4 hypervariable region of the 16S rRNA gene (∼250 bp) using the primers F515/R806 developed by Caporaso et al. (2011). This gene region has been widely used to characterize the bacterial communities from vertebrates (Earth Microbiome Project, Gilbert et al., 2014), including fish (Llewellyn et al., 2015; Carlson et al., 2017; Nielsen et al., 2017; Chiarello et al., 2018). Amplicon libraries were prepared using the Dual-Index Sequencing Strategy in Kozich et al. (2013) and sequenced in a single run of the Illumina MiSeq sequencing platform at the Center for Microbial Systems of the University of Michigan Medical School (United States).
Data and Statistical Analyses
Raw FASTQ files were analyzed using the Quantitative Insights Into Microbial Ecology 2 (QIIME2; release 2018.4) platform. Clean sequences were aligned against the Silva (132) reference database (Quast et al., 2012) with DADA2 pipeline (Callahan et al., 2016). Samples were rarefied to the minimum read count and two feature tables containing amplicon sequence variants (ASVs) from the skin and gut were constructed. The core microbiome was assessed for the skin and gut considering ASVs present in 100% of the samples from each tissue. For the most abundant ASVs in each tissue (>1% of representative sequences), a heatmap was created using the -p-normalize option in QIIME2, which normalizes the feature table by adding a pseudocount of 1 and uses the log10 frequency for the phylum and genus levels.
Bacterial taxonomic alpha-diversity (intra-sample) was calculated using Shannon, Fisher, Faith’s phylogenetic diversity (PD), Evenness, and Simpson indices as implemented in the R package phyloseq (McMurdie and Holmes, 2013). Species beta-diversity (inter-sample) was estimated using Bray–Curtis and phylogenetic Unifrac (unweighted and weighted) distances. Dissimilarity between samples was assessed by principal coordinates analysis (PCoA).
Differences in alpha-diversity across habitat and locality were analyzed by performing a linear model analysis and model effects were evaluated by using 1,000 residual randomizations in a permutation procedure using the R package RRPP (Collyer et al., 2015). Beta-diversity differences across locality and habitat were assessed using permutational analysis of variance (1,000 permutations), as implemented in the adonis function of the vegan R package. Differences in community composition between ecotypes were tested using linear regression models for the most abundant taxa (with >1% representative sequences).
Results
Taxonomic Composition and Core Bacterial Communities in E. prochilos
A total of 954,109 raw reads were generated (537,084 for the skin and 417,025 for the gut), with a minimum of 8,991 reads per sample and a maximum of 39,640. These sequences corresponded to 1,155 unique ASVs, from which 662 and 579 were found in the skin and the gut of E. prochilos, respectively.
Twenty-two of the 25 bacterial phyla were detected in the skin, but only five were represented by more than 1% of sequences (Figure 1 and Supplementary Table S1). Members of Proteobacteria (80% of the sequences), Bacteroidetes (7.1%), and Firmicutes (2.2%) occurred in all individuals (Supplementary Table S1), thus forming the core bacterial communities of the skin. Members of Tenericutes (1.6% of the sequences) only occurred in coral-dwellers (but only in 6 out of 11 individuals) (Figure 1 and Supplementary Table S1). Eleven families dominated the core bacterial communities of the skin, with Pseudomonadaceae (ca. 18.5% of the sequences) and Beijerinckiaceae (ca. 47.2%) being the most abundant (Supplementary Table S1). At the genus level, 10 identified genera were represented by >1% of the sequences, with Methylobacterium (41.8% of the sequences), Pseudomonas (18.5%), and Janthinobacterium (1.5%) comprising the core microbiome (Figure 1). It is worth noticing that potential pathogens from the genera Photobacterium and Vibrio, were more prevalent in coral-dwellers, with the first genus only occurring in this ecotype (Figure 1 and Supplementary Table S1).
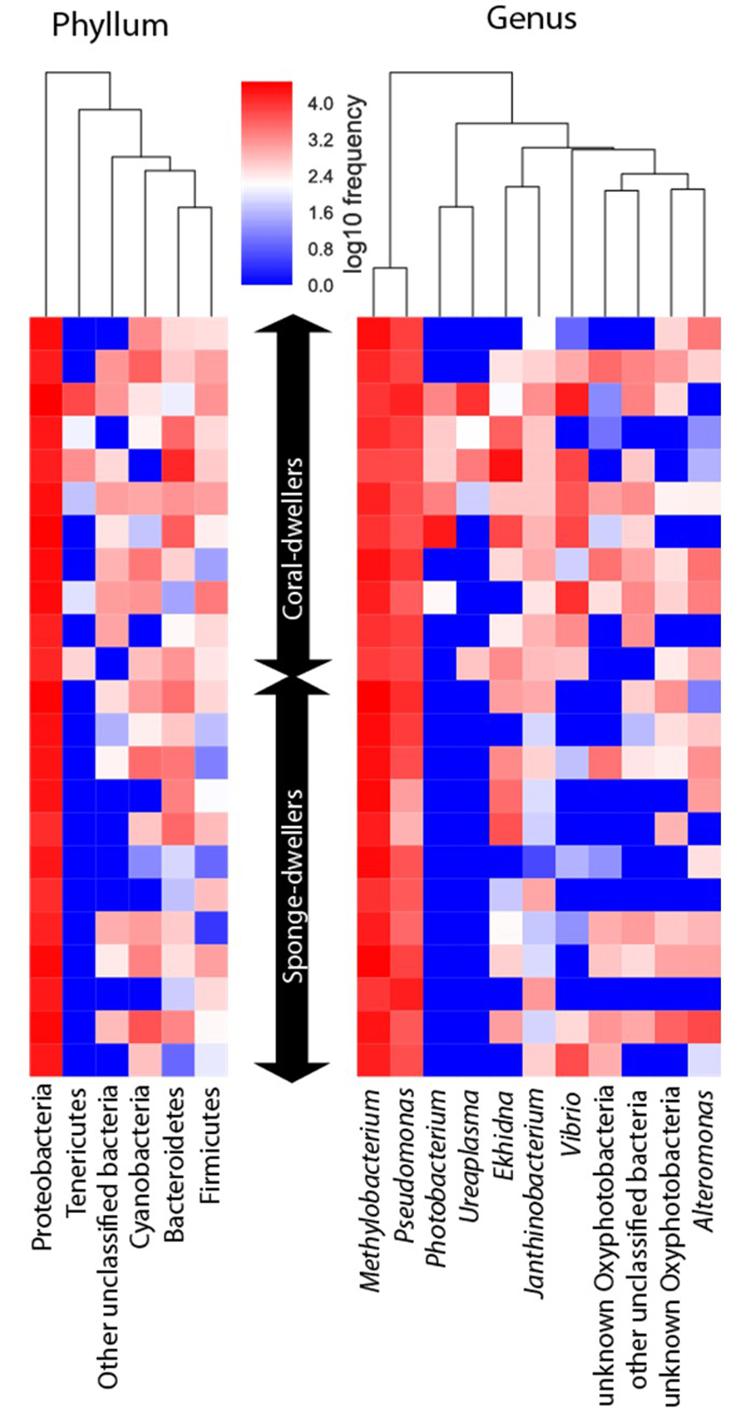
Figure 1. Heatmap depicting absolute abundance of ASVs identified at the phylum and genus levels for the skin microbiome of the two ecotypes.
Nineteen phyla were detected in the gut, but only six were represented by more than 1% of the sequences (Figure 2 and Supplementary Table S2). Only ASVs belonging to Proteobacteria (68% of the sequences) were found in all individuals sampled (Figure 2 and Supplementary Table S2). The core bacterial communities present in the gut were only composed by Beijerinckiaceae, which accounted for 50% of the sequences (Figure 2 and Supplementary Table S2). Seven genera were considered abundant in the gut (Figure 2 and Supplementary Table S2) and from these only Methylobacterium (Beijerinckiaceae) (ca. 50% of the sequences) was present in all individuals. As in the skin, potential pathogens from Photobacterium and Vibrio had higher prevalence in the coral-dwellers (Figure 2).
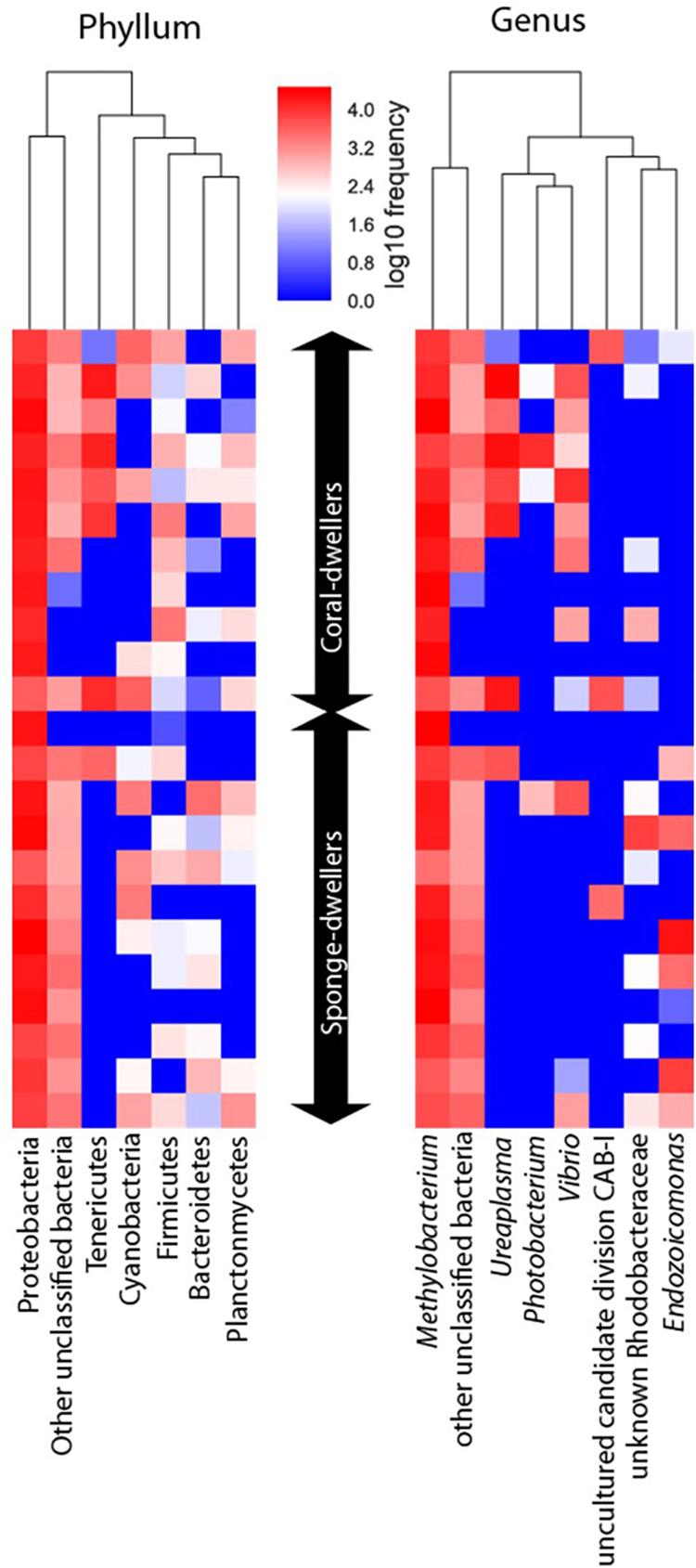
Figure 2. Heatmap depicting absolute abundance of ASVs identified at the phylum and genus levels for the gut microbiome of the two ecotypes.
Bacterial Diversity Analyses
Skin microbiome alpha-diversity was significantly different between ecotypes, with coral-dwellers showing consistently higher alpha-diversity indices as assessed by Shannon (F = 13.786, p = 0.002), Simpson (F = 20.162, p = 0.001), and Evenness (F = 17.807, p = 0.001) indices (see Figure 3 and Supplementary Tables S3, S4). Locality or the interaction term locality∗ecotype had no effect on microbial alpha diversity. Significant differences in beta-diversity were also found between ecotypes using phylogenetic Unifrac weighted (R2 = 0.224 and p = 0.005) and Bray–Curtis (R2 = 0.098 and p = 0.007) distances (Supplementary Table S3 and Figure 4A). Similarly, sampling localities also showed significant differences in beta-diversity for the Bray–Curtis distance (R2 = 0.087, p = 0.018) (Supplementary Table S3 and Figure 4A).
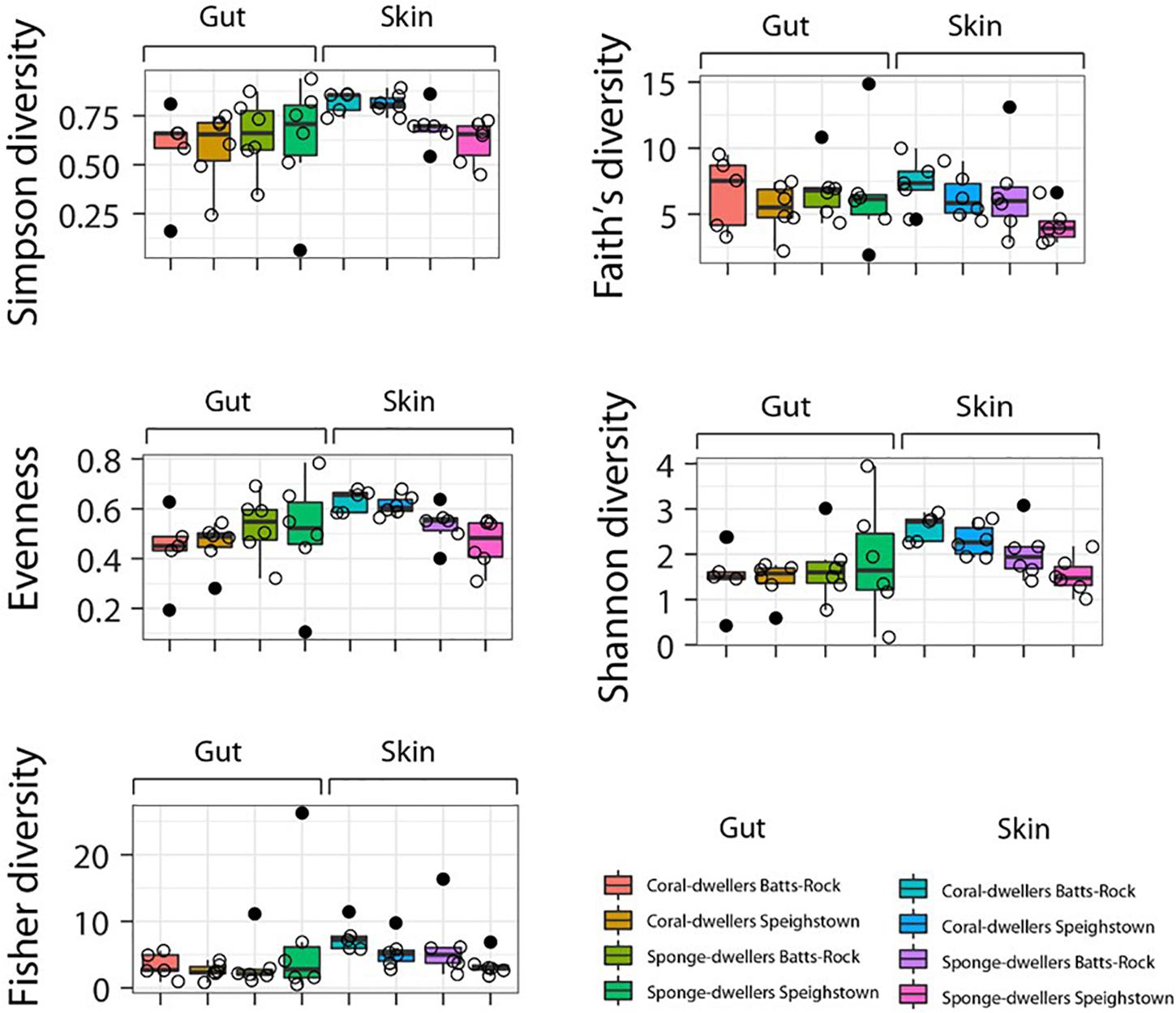
Figure 3. Box-plots depicting mean values and standard deviations of alpha diversity indices for the skin and gut microbiome per locality and ecotype.
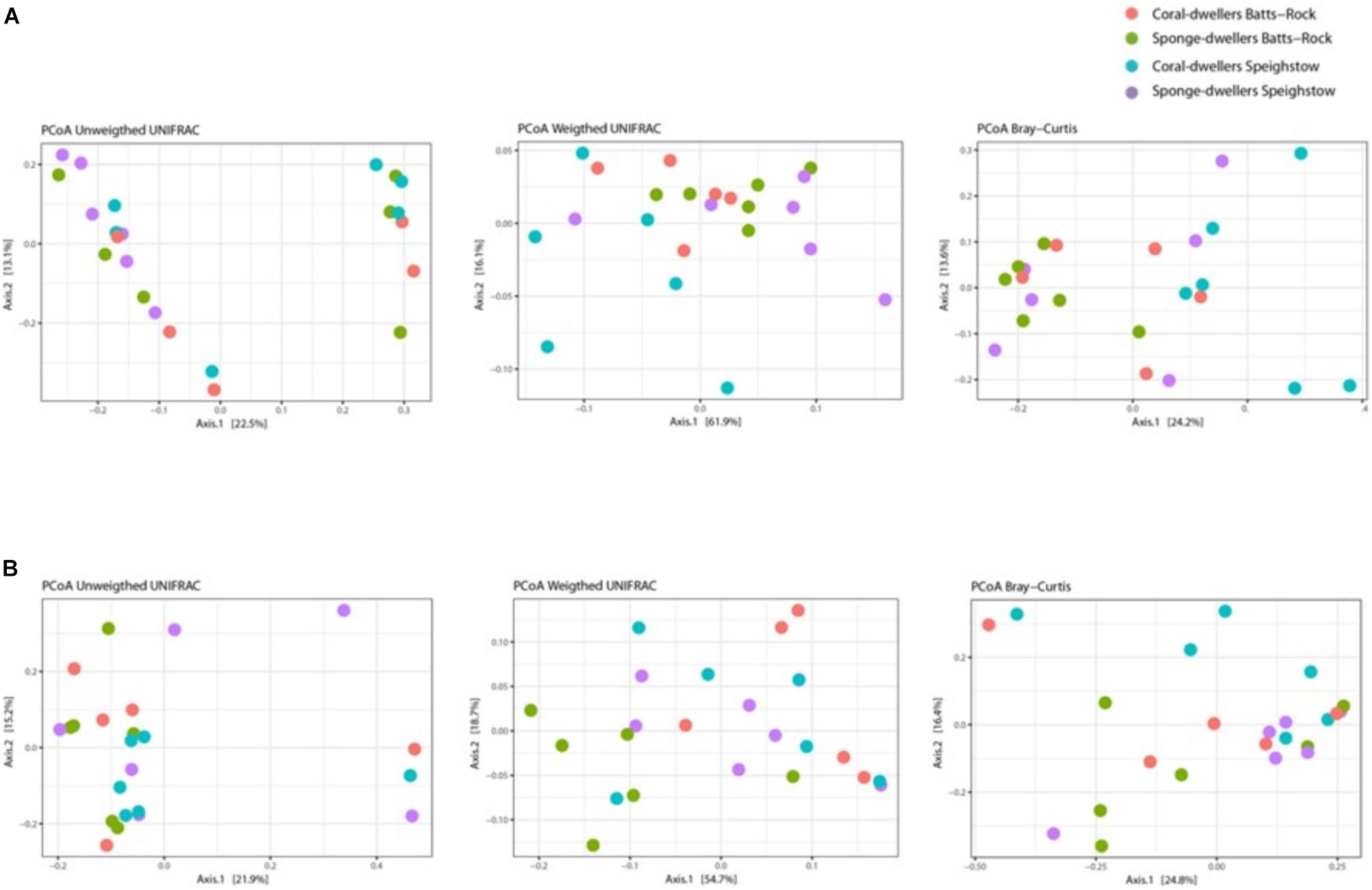
Figure 4. (A) PCoA plots depicting weighted and unweighted Unifrac distances and Bray-Curtis distances for the skin microbiome; and (B) PCoA plots depicting weighted and unweighted Unifrac distances and Bray-Curtis distances for the gut microbiome.
Analysis of mean taxa proportions showed significant differences in the abundance of Vibrionaceae (F-statistics = 6.057, p = 0.023) and Beijerinckiaceae (F-statistics = 19.34, p < 0.0001) between ecotypes. Within these two families, the abundance of Vibrio (Vibrionaceae, F-statistics = 5.443, p = 0.030) and Methylobacterium (Beijerinckiaceae, F-statistics = 9.32, p < 0.0001) varied also significantly between ecotypes (Supplementary Table S1).
For the gut microbiome, locality, ecotype, and the interaction between the two factors had no effect in any of the alpha diversity indices (see Figure 3 and Supplementary Tables S3, S4). However, the analysis of beta-diversity revealed significant differences between ecotypes (R2 = 0.125, p = 0.038) and ecotype∗locality for one of the three distances (phylogenetic Unifrac weighted) tested (R2 = 0.116; p = 0.037) (Supplementary Table S3, and Figure 4B). Analysis of mean taxa proportions showed significant differences between ecotypes for Ureaplasma (Mycoplasmataceae, Tenericutes) (F-statistics = 6.105; p = 0.022), which was almost exclusive of the coral-dwelling ecotype (Supplementary Table S1).
Discussion
The present study describes the core bacterial communities of the skin and gut of E. prochilos and the differences between two alternative ecotypes (cleaners vs. non-cleaners) in Barbados. Overall, the skin microbiome of coral-dweller obligate cleaners showed consistently greater intra-sample diversity and harbored a significantly higher prevalence of potential fish pathogens confirming our initial hypotheses.
Taxonomic Composition and Core Bacterial Communities in E. prochilos
The skin core microbiome of E. prochilos was more diverse than that of the gut, with the former comprised of Proteobacteria, Bacteroidetes, and Firmicutes, whereas the latter only encompassed Proteobacteria of mainly the Methylobacterium genus (Beijerinckiaceae). The bacterial profiles reported here for the skin and gut microbiomes of E. prochilos are similar to those previously published for other teleosts (e.g., McDonald et al., 2012; Larsen et al., 2014; Lokesh and Kiron, 2016; Carda-Diéguez et al., 2017; Rosado et al., 2019), including other coral reef fish (Chiarello et al., 2018).
Differences in Bacterial Diversity Between E. prochilos Ecotypes
We found significant intraspecific differences in the bacterial diversity of the skin between the two E. prochilos ecotypes. The skin microbiome of coral-dwellers had higher alpha diversity (intra-sample) when compared to sponge-dwellers, although observed differences were not always statistically significant. These differences most likely reflect ecotype-specific differences in habitat use, but also behavior, since coral-dwellers depend almost exclusively on client-derived ectoparasites, mucus and tissue for food, coming into frequent contact with other fish species (e.g., Côté and Soares, 2011). Sponge-dwellers, however, by preying on other items, limit their contact with heterospecifics (Whiteman and Côté, 2002). We hence hypothesize that bacterial diversity in the skin of E. prochilos can increase by horizontal transfer of bacteria from frequent contact with fish clients. Microbial exchanges via social contact have been reported in several organisms such as chimpanzees (Moeller et al., 2016), ants (Ivens et al., 2018), baboons (Tung et al., 2015), bumblebees (Koch and Schmid-Hempel, 2011), and humans (Kort et al., 2014). In fish, the impact of social transmission on microbiome composition is still unclear, however, there is empirical evidence suggesting that co-housing could have a diluting effect on microbiome differences driven by host genotype (Burns et al., 2016). Importantly, bacterial pathogens have been reported to be transmitted to cleaning fish through contact with diseased clients (Treasurer and Laidler, 1994).
Compared with sponge-dwellers, the skin microbiome of coral-dweller cleaners was significantly enriched with Vibrionaceae (more than 6-fold), a bacterial family known to encompass several fish pathogens (see for example Austin, 2011 for a review). Within this family, ASVs from Vibrio and Photobacterium, had higher prevalence in the cleaner ecotype. These two genera are known to harbor numerous pathogens able to infect fish worldwide, including tropical species (Landsberg, 1995; Panek, 2005; Gomez-Gil et al., 2007). Indeed, the abundance of Vibrio was significantly higher in the skin of coral-dwellers. This supports our initial hypothesis that pathogen transmission may occur from diseased clients.
Taxon differences found in the gut were more subtle. Among the most abundant taxa in the gut, only one ASV belonging to Ureaplasma varied significantly between ecotypes. Nonetheless, the interaction between sampling locality and ecotype had an effect on beta-diversity (Weighted Unifrac). This is somewhat surprising since several studies have shown that diet has a significant effect on the gut microbiome composition of fish (see the reviews by Tarnecki et al., 2017; Egerton et al., 2018).
Despite the important ecological role cleaner fish play in marine ecosystems, results from previous studies have provided strong evidence that adopting a non-cleaning lifestyle has some evolutionary advantages, and that sponge-dwelling may be a conservative strategy; for instance, adult E. prochilos in Barbados, have been mostly found in sponges regardless of coral habitat availability (Whiteman and Côté, 2004b). This suggests a preference for this habitat or, at least, the existence of potential constraints to adopt a cleaner lifestyle. Importantly, White et al. (2007) found that, overall, immature sponge-dwelling E. evelynae gobies grew faster than immature coral-dwellers, and the latter seemed to disappear at higher rates than non-cleaning sponge dwellers. The underlying causes for these disappearances were then suggested to be a result of emigration or were related to higher mortality rates due to predation by clients, which led to the hypothesis that being a cleaner is a riskier and less reliable mode of life, depending heavily on the quality and abundance of clients and more vulnerable to predation (White et al., 2007). Moreover, parasite transmission from diseased clients may also cause this apparent higher mortality, thus representing another negative consequence of adopting a cleaning behavior (Grutter, 2002; Jones et al., 2004). The results from the present study suggest that a higher load of bacterial pathogens may be acquired through cleaning engagement and may help explain the patterns found by White et al. (2007), although more research and data are needed to further confirm this hypothesis.
Conclusion
The results from this study showed that the bacterial communities of the skin of the two alternative ecotypes of E. prochilos can be distinguished using 16S rRNA gene sequences, even amongst fish captured only 10 s of meters apart. Furthermore, the skin microbiome of coral-dwelling gobies (cleaners) harbors higher bacterial diversity, including a significantly higher proportion of potential fish pathogens (e.g., Vibrio and Photobacterium). We propose that habitat use, diet and social engagement, due to frequent physical contact with potential diseased clients, could lead to significant differences in the diversity and abundance of pathogenic bacteria between cleaner and non-cleaner ecotypes of E. prochilos.
Ethics Statement
This study was carried out in accordance with the recommendations of the Coastal Zone Management Unit (CZMU) in Barbados. The protocol was approved by the Minister of Environment on behalf of the CZUM (permit reference number: CZ01/9/9).
Author Contributions
RX, MS, and RM conceived the work. RM collected the fish. RX and JS conducted the laboratory work. RX, MP-L, and DR conducted data analysis. All authors contributed to writing the manuscript.
Funding
RX, MS, MP-L, AV, and DR were currently supported by FCT under the Programa Operacional Potencial Humano – Quadro de Referência Estratégico Nacional funds from the European Social Fund and Portuguese Ministério da Educação e Ciência (MS: SFRH/BPD/109433/2015; RX: IF/00359/2015; AV: SFRH/BPD/77487/2011; DR: SFRH/BD/117943/2016). RM was supported by a scholarship from the Conselho Nacional de Desenvolvimento Científico e Tecnológico do Brazil (CNPq). Research was supported by the European Regional Development Fund (ERDF) through COMPETE program and by National Funds through FCT – Foundation for Science and Technology (projects PTDC/MAR-BIO/0902/2014- POCI-01-0145- FEDER-016550; and PTDC/BIA-MIC/27995/2017- POCI-01-0145-FEDER-027995).
Conflict of Interest Statement
The authors declare that the research was conducted in the absence of any commercial or financial relationships that could be construed as a potential conflict of interest.
Acknowledgments
We thank J. Germain, S. Tide, H. Valles, L. Waterman, and R. Bshary for logistical support and help with the gobies collection, and S. Cardoso for helping with the manuscript format. We also thank the staff of the Bellairs Research Institute for logistical support.
Supplementary Material
The Supplementary Material for this article can be found online at: https://www.frontiersin.org/articles/10.3389/fmicb.2019.00716/full#supplementary-material
References
Arnal, C., and Côté, I. M. (2000). Diet of broadstripe cleaning gobies on a Barbadian reef. J. Fish Biol. 57, 1075–1082. doi: 10.1111/j.1095-8649.2000.tb02213.x
Austin, B. (2011). Taxonomy of bacterial fish pathogens. Vet. Res. 42:20. doi: 10.1186/1297-9716-42-20
Böhlke, J. E., and Robins, C. R. (1968). Western Atlantic seven-spined gobies, with descriptions of ten new species and a new genus, and comments on Pacific relatives. Proc. Acad. Nat. Sci. Phila. 120, 45–174.
Bshary, R. (2003). The cleaner wrasse, Labroides dimidiatus, is a key organism for reef fish diversity at Ras Mohammed National Park, Egypt. J. Anim. Ecol. 72, 169–176. doi: 10.1046/j.1365-2656.2003.00683.x
Bshary, R., and Grutter, A. S. (2002). Asymmetric cheating opportunities and partner control in a cleaner fish mutualism. Anim. Behav. 63, 547–555. doi: 10.1006/anbe.2001.1937
Bshary, R., and Würth, M. (2001). Cleaner fish Labroides dimidiatus manipulate client reef fish by providing tactile stimulation. Proc. R. Soc. Lond. B Biol. Sci. 268, 1495–1501. doi: 10.1098/rspb.2001.1495
Burns, A. R., Stephens, W. Z., Stagaman, K., Wong, S., Rawls, J. F., Guillemin, K., et al. (2016). Contribution of neutral processes to the assembly of gut microbial communities in the zebrafish over host development. ISME J. 10, 655–664. doi: 10.1038/ismej.2015
Callahan, B. J., McMurdie, P. J., Rosen, M. J., Han, A. W., Johnson, A. J. A., and Holmes, S. P. (2016). DADA2: high-resolution sample inference from Illumina amplicon data. Nat. Methods 13, 581–583. doi: 10.1038/nmeth.3869
Caporaso, J. G., Lauber, C. L., Walters, W. A., Berg-Lyons, D., Lozupone, C. A., Turnbaugh, P. J., et al. (2011). Global patterns of 16S rRNA diversity at a depth of millions of sequences per sample. Proc. Natl. Acad. Sci. U.S.A. 108(Suppl. 1), 4516–4522. doi: 10.1073/pnas.1000080107
Carda-Diéguez, M., Ghai, R., Rodríguez-Valera, F., and Amaro, C. (2017). Wild eel microbiome reveals that skin mucus of fish could be a natural niche for aquatic mucosal pathogen evolution. Microbiome 5:162. doi: 10.1186/s40168-017-0376-1
Carlson, J. M., Leonard, A. B., Hyde, E. R., Petrosino, J. F., and Primm, T. P. (2017). Microbiome disruption and recovery in the fish Gambusia affinis following exposure to broad-spectrum antibiotic. Infect. Drug Resist. 10, 143–154. doi: 10.2147/IDR.S129055
Cheney, K. L., and Côté, I. M. (2005). Mutualism or parasitism? The variable outcome of cleaning symbioses. Biol. Lett. 1, 162–165. doi: 10.1098/rsbl.2004.0288
Cheney, K. L., Grutter, A. S., Blomberg, S. P., and Marshall, N. J. (2009). Blue and yellow signal cleaning behaviour in coral reef fishes. Curr. Biol. 19, 1283–1287. doi: 10.1016/j.cub.2009.06.028
Chiarello, M., Auguet, J. C., Bettarel, Y., Bouvier, C., Claverie, T., Graham, N. A. J., et al. (2018). Skin microbiome of coral reef fish is highly variable and driven by host phylogeny and diet. Microbiome 6:147. doi: 10.1186/s40168-018-0530-4
Clague, G. E., Cheney, K. L., Goldizen, A. W., McCormick, M. I., Waldie, P. A., and Grutter, A. S. (2011). Long-term cleaner fish presence affects growth of a coral reef fish. Biol. Lett. 7, 863–865. doi: 10.1098/rsbl.2011.0458
Colin, P. L. (1975). The Neon Gobies: The Comparative Biology of the Gobies of the Genus Gobiosoma, Subgenus Elacitunus (Pisces: Gobiidae) in the Tropical Western North Atlantic Ocean. Neptune, NJ: T. H. F. Publications.
Colin, P. L. (2010). Fishes as living tracers of connectivity in the tropical western North Atlantic: I. Distribution of the neon gobies, genus Elacatinus (Pisces: Gobiidae). Zootaxa 2370, 36–52. doi: 10.11646/zootaxa.2370.1.2
Collyer, M. L., Sekora, D. J., and Adams, D. C. (2015). A method for analysis of phenotypic change for phenotypes described by high-dimensional data. Heredity 115, 357–365. doi: 10.1038/hdy.2014.75
Côté, I. M. (2000). Evolution and ecology of cleaning symbioses in the Sea. Oceanogr. Mar. Biol. Rev. 38, 311–355.
Côté, I. M., and Soares, M. C. (2011). “Gobies as cleaners,” in The Biology of Gobies, eds R. A. Patzner, J. L. van Tassell, M. Kovaciĉ, and B. G. Kapoor (Enfield, NH: Science Publishers), 563–592.
Delgado-Baquerizo, M., Maestre, F. T., Reich, P. B., Jeffries, T. C., Gaitan, J. J., Encinar, D., et al. (2016). Microbial diversity drives multifunctionality in terrestrial ecosystems. Nat. Commun. 7:10541. doi: 10.1038/ncomms10541
Eckes, M., Dove, S., Siebeck, U. E., and Grutter, A. S. (2015). Fish mucus versus parasitic gnathiid isopods as sources of energy and sunscreens for a cleaner fish. Coral Reefs 34, 823–833. doi: 10.1007/s00338-015-1313-z
Egerton, S., Culloty, S., Whooley, J., Stanton, C., and Ross, R. P. (2018). The gut microbiota of marine fish. Front. Microbiol. 9:873. doi: 10.3389/fmicb.2018.00873
Elinav, E., Strowig, T., Henao-Mejia, J., and Flavell, R. A. (2011). Regulation of the an-timicrobial response by NLR proteins. Immunity 34, 665–679. doi: 10.1016/j.immuni.2011.05.007
Endt, K., Stecher, B., Chaffron, S., Slack, E., Tchitchek, N., Benecke, A., et al. (2010). The microbiota mediates pathogen clearance from the gut lumen after non-typhoidal Salmonella diarrhea. PLoS Pathog. 6:e1001097. doi: 10.1371/journal.ppat.1001097
Garrett, W. S., Gordon, J. I., and Glimcher, L. H. (2010). Homeostasis and inflammation in the intestine. Cell 140, 859–870. doi: 10.1016/j.cell.2010.01.023
Gibbons, S. M., and Gilbert, J. A. (2015). Microbial diversity - exploration of natural ecosystems and microbiomes. Curr. Opin. Genet. Dev. 35, 66–72. doi: 10.1016/j.gde.2015.10.003
Gilbert, J. A., Jansson, J. K., and Knight, R. (2014). The earth microbiome project: successes and aspirations. BMC Biol. 12:69. doi: 10.1186/s12915-014-0069-1
Gomez-Gil, B., Fajer-Avila, E., and García-Vargas, F. (2007). Vibrios of the spotted rose snapper Lutjanus guttatus Steindachner, 1869 from northwestern Mexico. J. Appl. Microbiol. 102, 1518–1526. doi: 10.1111/j.1365-2672.2006.03199.x
Grutter, A. S. (1994). Spatial and temporal variations of the ectoparasites of seven reef fish species from Lizard Island and Heron Island, Australia. Mar. Ecol. Prog. Ser. 115, 21–30. doi: 10.3354/meps115021
Grutter, A. S. (1997). Size-selective predation by the cleaner fish Labroides dimidiatus. J. Fish Biol. 50, 1303–1308. doi: 10.1111/j.1095-8649.1997.tb01654.x
Grutter, A. S. (2002). Cleaning symbioses from the parasites’ perspective. Parasitology 124, 65–81. doi: 10.1017/S0031182002001488
Grutter, A. S., Murphy, J. M., and Choat, J. H. (2003). Cleaner fish drives local fish diversity on coral reefs. Curr. Biol. 13, 64–67. doi: 10.1016/S0960-9822(02)01393-3
Ivens, A. B. F., Gadau, A., Kiers, E. T., and Kronauer, D. J. C. (2018). Can social partnerships influence the microbiome? Insights from ant farmers and their trophobiont mutualists. Mol. Ecol. 27, 1898–1914. doi: 10.1111/mec.14506
Jones, C. M., Grutter, A. S., and Cribb, T. H. (2004). Cleaner fish become hosts: a novel form of parasite transmission. Coral Reefs 23, 521–529. doi: 10.1007/s00338-004-0411-0
Koch, H., and Schmid-Hempel, P. (2011). Socially transmitted gut microbiota protect bumble bees against an intestinal parasite. PNAS 108, 19288–19292. doi: 10.1073/pnas.1110474108
Kort, R., Caspers, M., van de Graaf, A., van Egmond, W., Keijser, B., and Roeselers, G. (2014). Shaping the oral microbiota through intimate kissing. Microbiome 2:41. doi: 10.1186/2049-2618-2-41
Kozich, J. J., Westcott, S. L., Baxter, N. T., Highlander, S. K., and Schloss, P. D. (2013). Development of a dual-index sequencing strategy and curation pipeline for analyzing amplicon sequence data on the MiSeq Illumina sequencing platform. Appl. Environ. Microbiol. 79, 5112–5120. doi: 10.1128/AEM.01043-13
Landsberg, J. H. (1995). Tropical reef-fish disease outbreaks and mass mortalities in Florida, USA: what is the role of dietary biological toxins? Dis. Aquat. Anim. 22, 83–100. doi: 10.3354/dao022083
Larsen, A., Tao, Z., Bullard, S. A., and Arias, C. R. (2013). Diversity of the skin microbiota of fishes: evidence for host species specificity. FEMS Microbiol. Ecol. 85, 483–494. doi: 10.1111/1574-6941.12136
Larsen, A. M., Mohammed, H. H., and Arias, C. R. (2014). Characterization of the gut microbiota of three commercially valuable warmwater fish species. J. Appl. Microbiol. 116, 1396–1404. doi: 10.1111/jam.12475
Llewellyn, M. S., McGinnity, P., Dionne, M., Letourneau, J., Thonier, F., Carvalho, G. R., et al. (2015). The biogeography of the Atlantic salmon (Salmo salar) gut microbiome. ISME J. 10, 1280–1284. doi: 10.1038/ismej.2015.189
Lokesh, J., and Kiron, V. (2016). Transition from freshwater to seawater reshapes the skin-associated microbiota of Atlantic salmon. Sci. Rep. 6:19707. doi: 10.1038/srep19707
Lowrey, L., Woodhams, D. C., Tacchi, L., and Salinas, I. (2015). Topographical mapping of the rainbow trout (Oncorhynchus mykiss) microbiome reveals a diverse bacterial community with antifungal properties in the skin. Appl. Environ. Microbiol. 81, 6915–6925. doi: 10.1128/AEM.01826-15
McDonald, R., Schreier, H. J., and Watts, J. E. M. (2012). Phylogenetic analysis of microbial communities in different regions of the gastrointestinal tract in panaque nigrolineatus, a wood-Eating fish. PLoS One 7:e48018. doi: 10.1371/journal.pone.0048018
McFall-Ngai, M., Hadfield, M. G., Bosch, T. C. G., Carey, H. V., Domazet-Lošo, T., Douglas, A. E., et al. (2013). Animals in a bacterial world, a new imperative for the life sciences. PNAS 110, 3229–3236. doi: 10.1073/pnas.1218525110
McMurdie, P. J., and Holmes, S. (2013). Phyloseq: an R package for reproducible interactive analysis and graphics of microbiome census data. PLoS One 8:e61217. doi: 10.1371/journal.pone.0061217
Moeller, A. H., Foerster, S., Wilson, M. L., Pusey, A. E., Hahn, B. H., and Ochman, H. (2016). Social behavior shapes the chimpanzee pan-microbiome. Sci. Adv. 2:e1500997. doi: 10.1126/sciadv.1500997
Nielsen, S., Walburn, J. W., Vergés, A., Thomas, T., and Egan, S. (2017). Microbiome patterns across the gastrointestinal tract of the rabbitfish Siganus fuscescens. PeerJ 5:e3317. doi: 10.7717/peerj.3317
Panek, F. M. (2005). Epizootics and disease of coral reef fish in the tropical Western Atlantic and Gulf of Mexico. Rev. Fish. Sci. 13, 1–21. doi: 10.1080/10641260590885852
Pratte, Z. A., Besson, M., Hollman, R. D., and Stewart, F. J. (2018). The gills of reef fish support a distinct microbiome influenced by host-specific factors. Appl. Environ. Microbiol. 84:e00063-18. doi: 10.1128/AEM.00063-18
Quast, C., Pruesse, E., Yilmaz, P., Gerken, J., Schweer, T., Yarza, P., et al. (2012). The SILVA ribosomal RNA gene database project: improved data processing and web-based tools. Nucleic Acids Res. 41, D590–D596. doi: 10.1093/nar/gks1219
Randall, J. E., and Lobel, P. S. (2009). A literature review of the sponge-dwelling gobiid fishes of the genus Elacatinus from the western Atlantic, with description of two new Caribbean species. Zootaxa 2133, 1–19. doi: 10.5281/zenodo.274944
Rocha, L. A., Rosa, I. L., and Feitoza, B. M. (2000). Sponge-dwelling fishes of northeastern Brazil. Environ. Biol. Fish. 59, 453–458. doi: 10.1023/A:1026584708092
Ros, A. F. H., Lusa, J., Meyer, M., Soares, M., Oliveira, R. F., Brossard, M., et al. (2011). Does access to the bluestreak cleaner wrasse Labroides dimidiatus affect indicators of stress and health in resident reef fishes in the Red Sea? Horm. Behav. 59, 151–158. doi: 10.1016/j.yhbeh.2010.11.006
Rosado, D., Pérez-Losada, M., Severino, R., Cable, J., and Xavier, R. (2019). Characterization of the skin and gill microbiomes of the farmed seabass (Dicentrarchus labrax) and seabream (Sparus aurata). Aquaculture 500, 57–64. doi: 10.1016/j.aquaculture.2018.09.063
Rüber, L., van Tassell, J. L., and Zardoya, R. (2003). Rapid speciation and ecological divergence in the american seven-spined gobies (Gobiidae, Gobiosomatini) inferred from a molecular phylogeny. Evolution 57, 1584–1598. doi: 10.1111/j.0014-3820.2003.tb00366.x
Sasal, P., Thomas, F., and Grutter, A. S. (2005). “Behavioural aspects of parasitism,” in Marine Parasitology, ed. K. Rohde (Wallingford, CT: CABI Publishing), 259–278.
Sazima, I., Carvalho, A., and Sazima, C. (2008). A new cleaner species of Elacatinus (Actinopterygii: Gobiidae) from the Southwestern Atlantic. Zootaxa 1932, 27–32. doi: 10.5281/zenodo.184869
Sazima, I., Sazima, C., Francini, R. B., and Moura, R. L. (2000). Daily cleaning activity and diversity of clients of the barber goby, Elacatinus figaro, on rocky reefs in southeastern Brazil. Environ. Biol. Fish. 59, 69–77. doi: 10.1023/A:1007655819374
Sharon, G., Segal, D., Ringo, J. M., Hefetz, A., Ziber-Rosenberg, I., and Rosenberg, E. (2010). Commensal bacteria play a role in mating preference of Drosophila melanogaster. PNAS 107, 20051–20056. doi: 10.1073/pnas.1009906107
Soares, M. C., Bshary, R., Cardoso, S. C., Côté, I. M., and Oliveira, R. F. (2012). Face your fears: cleanerfish inspect predators despite being stressed by them. PLoS One 7:e39781. doi: 10.1371/journal.pone.0039781
Soares, M. C., Cable, J., Lima-Maximino, M., Maximino, C., and Xavier, R. (2019). Using fish models to investigate the links between microbiome and social behaviour: the next step for translational microbiome research? Fish Fish. (in press). doi: 10.1111/faf.12366
Soares, M. C., Cardoso, S. C., and Côté, I. M. (2007). Client preferences by Caribbean cleaning gobies: food, safety or something else? Behav. Ecol. Sociobiol. 61:1015. doi: 10.1007/s00265-006-0334-6
Soares, M. C., Oliveira, R. F., Ros, A. F. H., Grutter, A. S., and Bshary, R. (2011). Tactile stimulation lower stress in fish. Nat. Commun. 2:534. doi: 10.1038/ncomms1547
Stecher, B., Chaffron, S., Käppeli, R., Hapfelmeier, S., Freedrich, S., Weber, T. C., et al. (2010). Like will to like: abundances of closely related species can predict susceptibility to intestinal colonization by pathogenic and commensal bacteria. PLoS Pathog. 6:e1000711. doi: 10.1371/journal.ppat.1000711
Sun, D., Cheney, K. L., Werminghausen, J., McClure, E. C., Meekan, M. G., McCormick, M. I., et al. (2015a). Cleaner wrasse influence habitat selection of young damselfish. Coral Reefs 35, 427–436. doi: 10.1007/s00338-015-1391-y
Sun, D., Cheney, K. L., Werminghausen, J., Meekan, M. G., McCormick, M. I., Cribb, T. H., et al. (2015b). Presence of cleaner wrasse increases the recruitment of damselfishes to coral reefs. Biol. Lett. 11:20150456. doi: 10.1098/rsbl.2015.0456
Tarnecki, A. M., Burgos, F. A., Ray, C. L., and Arias, C. R. (2017). Fish intestinal microbiome: diversity and symbiosis unravelled by metagenomics. J. Appl. Microbiol. doi: 10.1111/jam.13415 [Epub ahead of print].
Taylor, M. S., and Hellberg, M. E. (2005). Marine radiations at small geographic scales: speciation in neotropical reef gobies (Elacatinus). Evolution 59, 374–385. doi: 10.1554/04-590
Taylor, M. S., and Hellberg, M. E. (2006). Comparative phylogeography of a genus of coral reef fishes: biogeographical, and genetical concordance in the Caribbean. Mol. Ecol. 15, 695–707. doi: 10.1111/j.1365-294X.2006.02820.x
Theis, K. R., Venkataraman, A., Dycus, J. A., Koonter, K. D., Schmitt-Matzen, E. N., Wagner, A. P., et al. (2013). Symbiotic bacteria appear to mediate hyena social odors. PNAS 110, 19832–19837. doi: 10.1073/pnas.1306477110
Treasurer, J. W., and Laidler, L. A. (1994). Aeromonas salmonicida infection in wrasse (Labridae), used as cleaner fish, on an Atlantic salmon. Salmo salar L., farm. J. Fish. Dis. 17, 155–161. doi: 10.1111/j.1365-2761.1994.tb00208.x
Tung, J., Barriero, L. B., Burns, M. B., Grenier, J. C., Lynch, J., Grieneisen, L. E., et al. (2015). Social networks predict gut microbiome composition in wild baboons. eLife 4:e05224. doi: 10.7554/eLife.05224
Venu, I., Durisko, Z., Xu, J., and Dukas, R. (2014). Social attraction mediated by fruit flies’ microbiome. J. Exp. Biol. 217(Pt 8), 1346–1352. doi: 10.1242/jeb.099648
Waldie, P. A., Blomberg, S. P., Cheney, K. L., Goldizen, A. W., and Grutter, A. S. (2011). Long-Term Effects of the cleaner fish Labroides dimidiatus on coral reef fish communities. PLoS One 6:e21201. doi: 10.1371/journal.pone.0021201
White, J., Mirleau, P., Danchin, E., Mulard, H., Hatch, S. A., Heeb, P., et al. (2010). Sexually transmitted bacteria affect female cloacal assemblages in a wild bird. Ecol. Lett. 13, 1515–1524. doi: 10.1111/j.1461-0248.2010.01542.x
White, J. W., Grigsby, C. J., and Warner, R. R. (2007). Cleaning behaviour is riskier and less profitable than an alternative strategy for a facultative cleaner fish. Coral Reefs 26, 87–94. doi: 10.1007/s00338-006-0161-2
Whiteman, E. A., and Côté, I. M. (2002). Cleaning activity of two Caribbean cleaning gobies: intra- and interspecific comparisons. J. Fish Biol. 60, 1443–1458. doi: 10.1111/j.1095-8649.2002.tb02439.x
Whiteman, E. A., and Côté, I. M. (2004a). Dominance hierarchies in group-living cleaning gobies: causes and foraging consequences. Anim. Behav. 67, 239–247. doi: 10.1016/j.anbehav.2003.04.006
Keywords: E. prochilos, ecotypes, pathogens, coral-dwellers, sponge-dwellers, social contamination
Citation: Xavier R, Mazzei R, Pérez-Losada M, Rosado D, Santos JL, Veríssimo A and Soares MC (2019) A Risky Business? Habitat and Social Behavior Impact Skin and Gut Microbiomes in Caribbean Cleaning Gobies. Front. Microbiol. 10:716. doi: 10.3389/fmicb.2019.00716
Received: 29 October 2018; Accepted: 21 March 2019;
Published: 09 April 2019.
Edited by:
Suhelen Egan, The University of New South Wales, AustraliaReviewed by:
Ravindra Pal Singh, National Agri-Food Biotechnology Institute, IndiaAymé Spor, UMR Agroécologie, France
Copyright © 2019 Xavier, Mazzei, Pérez-Losada, Rosado, Santos, Veríssimo and Soares. This is an open-access article distributed under the terms of the Creative Commons Attribution License (CC BY). The use, distribution or reproduction in other forums is permitted, provided the original author(s) and the copyright owner(s) are credited and that the original publication in this journal is cited, in accordance with accepted academic practice. No use, distribution or reproduction is permitted which does not comply with these terms.
*Correspondence: Marta C. Soares, marta.soares@cibio.up.pt; martinha.soares@gmail.com