- 1Laboratory of Genomics and Bioinformatics, Center of Genomics and Systems Biology, Institute of Biological Sciences, Federal University of Pará, Belém, Brazil
- 2Department of Biology and CESAM, University of Aveiro, Aveiro, Portugal
- 3Federal Rural University of Amazon, Capanema, Brazil
Aquatic systems have been described as antibiotic resistance reservoirs, where water may act as a vehicle for the spread of resistant bacteria and resistance genes. We evaluated the occurrence and diversity of third generation cephalosporin-resistant gram-negative bacteria in a lake in the Amazonia region. This water is used for human activities, including consumption after appropriate treatment. Eighteen samples were obtained from six sites in October 2014. Water quality parameters were generally within the legislation limits. Thirty-three bacterial isolates were identified as Escherichia (n = 7 isolates), Acinetobacter, Enterobacter, and Klebsiella (n = 5 each), Pseudomonas (n = 4), Shigella (n = 3), and Chromobacterium, Citrobacter, Leclercia, Phytobacter (1 isolate each). Twenty nine out of 33 isolates (88%) were resistant to most beta-lactams, except carbapenems, and 88% (n = 29) were resistant to antibiotics included in at least three different classes. Among the beta-lactamase genes inspected, the blaCTX–M was the most prevalent (n = 12 positive isolates), followed by blaTEM (n = 5) and blaSHV (n = 4). blaCTX–M–15 (n = 5), blaCTX–M–14 (n = 1) and blaCTX–M–2 (n = 1) variants were detected in conserved genomic contexts: blaCTX–M–15 flanked by ISEcp1 and Orf477; blaCTX–M–14 flanked by ISEcp1 and IS903; and blaCTX–M–2 associated to an ISCR element. For 4 strains the transfer of blaCTX–M was confirmed by conjugation assays. Compared with the recipient, the transconjugants showed more than 500-fold increases in the MICs of cefotaxime and 16 to 32-fold increases in the MICs of ceftazidime. Two isolates (Escherichia coli APC43A and Acinetobacter baumannii APC25) were selected for whole genome analysis. APC43A was predicted as a E. coli pathogen of the high-risk clone ST471 and serotype O154:H18. blaCTX–M–15 as well as determinants related to efflux of antibiotics, were noted in APC43A genome. A. baumannii APC25 was susceptible to carbapenems and antibiotic resistance genes detected in its genome were intrinsic determinants (e.g., blaOXA–208 and blaADC–like). The strain was not predicted as a human pathogen and belongs to a new sequence type. Operons related to metal resistance were predicted in both genomes as well as pathogenicity and resistance islands. Results suggest a high dissemination of ESBL-producing bacteria in Lake Água Preta which, although not presenting characteristics of a strongly impacted environment, contains multi-drug resistant pathogenic strains.
Introduction
Bacterial resistance to antibiotics is currently one of the most serious public health concerns. The environment and particularly aquatic systems have been pointed as important reservoirs of resistance (Baquero et al., 2008; Taylor et al., 2011; Marti et al., 2014). These settings bring together indigenous bacterial communities and bacteria resulting from anthropogenic contamination, creating a milieu that may promote horizontal gene transfer (Pei and Gunsch, 2009; Jiao et al., 2017). Furthermore, significant quantities of contaminants accumulate in polluted aquatic systems and some of these contaminants were implicated in the selection of resistant bacteria (e.g., antibiotics, metals, disinfectants) (Henriques et al., 2016; Jiao et al., 2017). The environment was also confirmed as the origin of some of the most successfully widespread antibiotic resistance genes (e.g., blaCTX–M and blaOXA–48; Poirel et al., 2002; Tacão et al., 2018). These evidences urgently ask to better understand the ecology of antibiotic resistance and the factors involved in resistance selection in aquatic systems. Dissemination of antibiotic resistance in these systems is particularly relevant when water is used for purposes that facilitate the transmission of bacteria to humans, namely for consumption, irrigation, recreational activities and fishing. Increasing our understanding of antibiotic resistance in specific aquatic systems is essential to suggest and implement mitigation strategies.
Nowadays, the spread of resistance to third generation cephalosporins in gram-negative bacteria is one of the major concerns in terms of antibiotic resistance. These antibiotics have great human health importance being often the first choice for the treatment of infectious diseases caused by gram-negative bacteria. Nevertheless, the levels of resistance to third generation cephalosporins have been increasing, and in several countries have reached levels that threaten their usefulness (WHO, 2014; ECDC, 2017). The most common and successful mechanism of resistance is the production of extended-spectrum beta-lactamases. According to a recent World Health Organization report, ESBL-producing Enterobacteriaceae are a critical human health concern (WHO, 2014). ESBLs can be classified into Ambler’s classes A (e.g., TEM, SHV, CTX-M, PER, VEB, GES) and D (OXA) (Ambler, 1980). Among these, enzymes of the CTX-M family are currently globally disseminated, often found in pathogenic bacteria of the family Enterobacteriaceae, and associated with mobile genetic elements (Bevan et al., 2017). In Brazil, CTX-M-producing bacteria have been frequently reported in hospital settings, with the most common variants being CTX-M-15 and CTX-M-2 (Rocha et al., 2016).
The problematic summarized above demands from the authorities measures to contain the spread of resistance to antibiotics. Aquatic environments may be one of the most important intervention areas. The occurrence of ESBL genes, including blaCTX–M, in different aquatic systems has been reported in several countries (Tacão et al., 2012; Zurfluh et al., 2013; Alves et al., 2014; Nascimento et al., 2017). In Brazilian aquatic systems, clinically relevant bacteria producing CTX-M enzymes have been recently described, e.g., in lakes (Nascimento et al., 2017), rivers (de Oliveira et al., 2017), wastewater (Dropa et al., 2016) and coastal water (Sellera et al., 2017). For the measures to be effective further studies are required to reveal which bacteria and which resistance and transfer mechanisms are present in these settings. There is a need to address different geographic areas, particularly ecologically relevant aquatic systems whose water is used for human activities.
In this work, we collected samples in an Amazonian lake. Water from this lake is used for water supply, irrigation and recreational activities (Santos et al., 2015). Gram-negative bacteria resistant to antibiotics were selected and mechanisms of resistance were characterized. The occurrence of genetic platforms that may contribute to multi-drug resistance in these bacteria (i.e., integrons) was also assessed. Two isolates belonging to species of public health interest (i.e., Escherichia coli and Acinetobacter baumanii) were selected for whole genome sequencing and analysis.
Materials and Methods
Sampling and Sample Analysis
Lake Água Preta (1°25′7.849″S, 48°26′19.02″W) is an Amazonian mesotrophic lake located in the Utinga State Park, Pará, Brazil. It is located near a densely populated area that includes the city of Belém (population of approximately 1.5 million). This lake was chosen considering its importance in water supply, irrigation and recreational activities. It has great ecological relevance in the Amazonian area (Santos et al., 2015). The lake has a surface area of approximately 7 km2 and a maximum depth of 8.5 m. There are no relevant agricultural or livestock activities on the banks of the lake. There is, however, a record of untreated wastewater discharges resulting from a large number of illegal homes in the vicinity of the lake. Six sampling points were selected (Figure 1). One liter of water was collected in triplicate at each sampling point in October 2014. Samples were collected in 1 L polypropylene flasks, packed in an isothermal box with ice, and sent to the Faculty of Sanitary and Environmental Engineering laboratory, Federal University of Pará, Brazil. Water samples collected for microbiological analysis were stored in previously sterilized polypropylene flasks of 250 mL. Sampling and analytical methods were performed according to the procedures and recommendations described in Standards Methods for the Examination of Water and Wastewater (Rice et al., 2012). Physico-chemical parameters such as pH, conductivity, temperature, dissolved oxygen and salinity were analyzed at the sampling points by potentiometry using a multi-parametric probe (556 MPS; YSI, United States). The following parameters were determined by UV spectrophotometry (UV DR 2800; HACH, Germany): turbidity, total solids, true color, apparent color, total phosphorous, total nitrogen, total iron, chemical oxygen demand (COD), and the concentration of the ions nitrite, nitrate, ammonia, chloride, aluminum, manganese, nickel, cadmium, copper, zinc and sulfate. Biochemical oxygen demand (BOD) was determined using a manometric respirometric test in the equipment BODTrack II (HACH, United States). The Most Probable Number (MPN) of total coliforms and E. coli was determined using the chromogenic substrate Colilert 18/QUANTI-TRAY (IDEXX Laboratories, United States) according to the manufacturers’ protocol. Odor intensity was measured using sensorial panel, while alkalinity and acidity were determined by titrimetry.
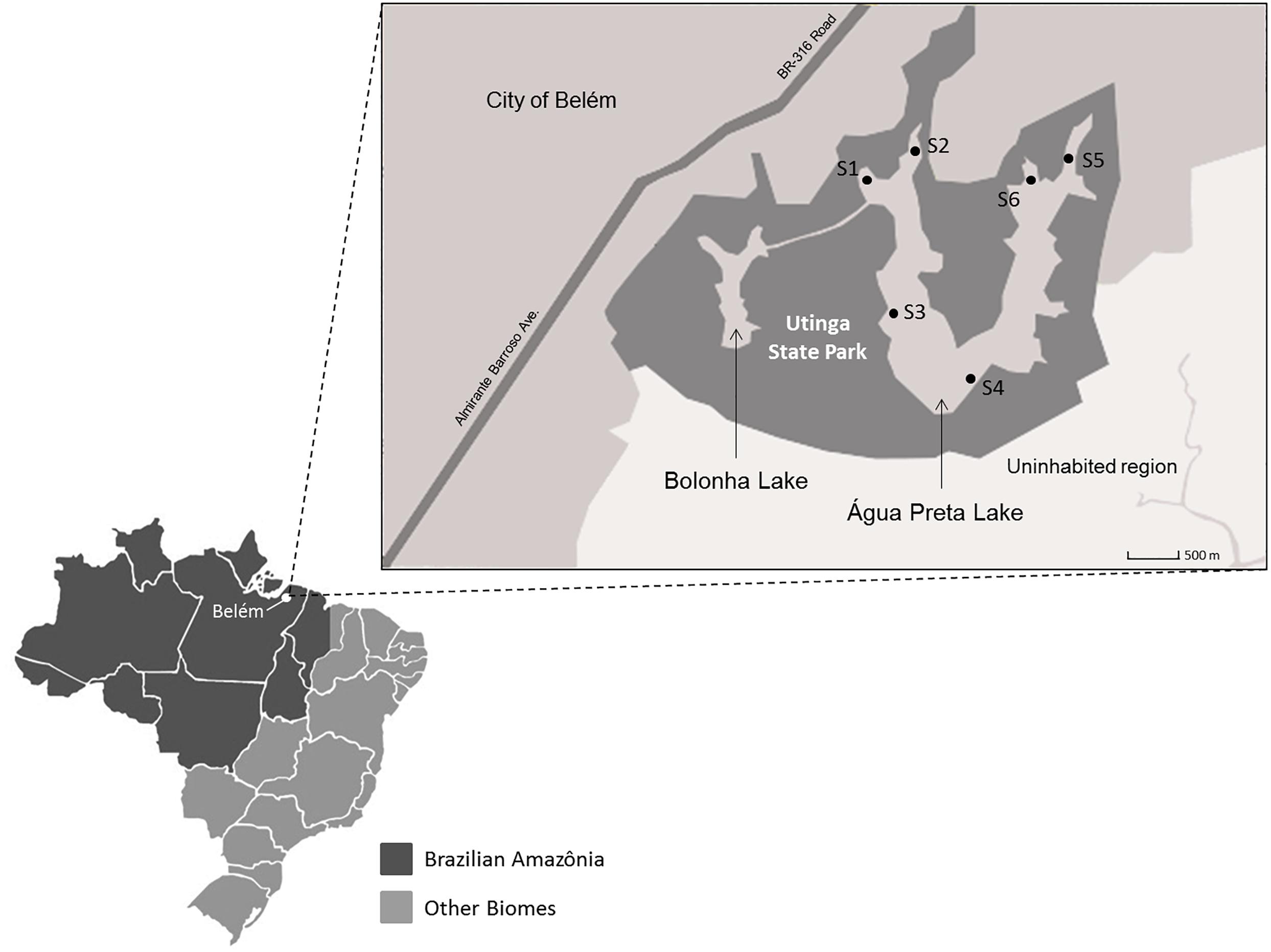
Figure 1. Map of the Utinga State Park (dark gray area). Sampling points are identified as S1, S2, S3, S4, S5, and S6. The urban area of Belém is represented by the gray area in the upper left half of the map. Therefore, the city is closer to the sampling points S1, S2, S5, and S6.
Results were evaluated according to the resolution no. 357/2005 of the Environment National Council of Brazil (CONAMA, 2005).
Bacteria Growth Conditions and Isolation
Water samples (1, 10, and 50 mL) were filtered through 0.45-μm-pore-size cellulose ester filters (Millipore). Membranes were placed onto MacConkey agar medium supplemented with cefotaxime (8 μg mL−1) (Sigma-Aldrich) and incubated at 37°C for 16 h. Individual colonies were purified in the same medium and stored in 20% glycerol at −80°C.
DNA Extraction and Identification of the Isolates
For DNA extraction, the bacterial isolates were inoculated in Tryptic Soy Broth medium (Himedia) supplemented with cefotaxime (8 μg mL−1) and cultivated at 37°C overnight with aeration. An aliquot of 5 ml of the culture was centrifuged at 6,000 g at 4°C for 10 min. The cell pellet was subjected to DNA extraction using the DNeasy Blood and Tissue kit (Qiagen), according to the manufacturer’s protocol. The integrity of the DNA was visualized on 1% agarose gel. DNA was stored in TE buffer (Tris 10 mM, EDTA 1mM, pH 8.0) at −20°C.
To determine the phylogenetic affiliation of the isolates, the 16S rRNA gene was amplified using the universal primers 8F (5′-AGAGTTTGATCCTGGCTCAG-3′) and 1492R (5′-TACGGYTACCTTGTTACGACTT-3′). PCR was carried out in 50 μL reaction mixtures containing buffer 1×, 1.5 mM of MgCl2, 0.2 mM of dNTP, 0.2 pmol of each primer, 1 U of Taq DNA polymerase (Invitrogen) and 50–100 ng of DNA. Cycling conditions were as follows: an initial denaturation at 95°C for 5 min, followed by 35 cycles of 95°C for 1 min, 55°C for 1 min and 72°C for 1 min, and a final extension step of 72°C for 10 min. Amplicons were sequenced using the ABI 3730 DNA Analyzer platform (Thermo Fisher Scientific). Reverse and forward sequences were assembled with BioEdit v. 7.2.6.1 (Hall, 1999) and the consensus sequences (∼1.5 kb) were compared to the GenBank database using BLASTn1.
Antibiotic Susceptibility Testing
To estimate the level of resistance of the isolates, the disk-diffusion method was used (Bauer et al., 1966). E. coli ATCC 25922 was used as quality control strain. Sixteen antibiotics were tested including amoxicillin (10 μg), amoxicillin + clavulanic acid (20–10 μg), ampicillin (10 μg), cephalotin (30 μg), cefotaxime (30 μg), ceftazidime (30 μg), cefepime (30 μg), imipenem (10 μg), aztreonam (30 μg), kanamycin (30 μg), gentamicin (10 μg), nalidixic acid (30 μg), ciprofloxacin (5 μg), chloramphenicol (30 μg), tetracycline (30 μg) and the combination of sulfamethoxazole + trimethoprim (25 μg). CLSI (2017) breakpoints were used to classify strains as susceptible, intermediate or resistant. Antibiotics were selected based on the CLSI guidelines, which specify the antibiotics that should be considered when characterizing Gram-negative non-fastidious organisms (e.g., Enterobacteriaceae, Acinetobacter spp. and Pseudomonas aeruginosa). Minimal inhibitory concentrations (MIC) were determined for cefotaxime and ceftazidime, following CLSI guidelines.
PCR Amplification of Resistance Genes and Mobile Genetic Elements
Isolates were screened by PCR to determine the presence of genes conferring resistance to beta-lactams (blaTEM, blaSHV, blaCTX–M, blaIMP, blaV IM, blaKPC). We also analyzed the isolates for the presence of genes encoding integrases of class 1 (intI1) and 2 (intI2). The PCR reactions were performed in a GeneAmp PCR System 9700 (Applied Biosystem) using DNA purified as described above. PCR was carried out using buffer 1×, 1.5 mM of MgCl2, 0.2 mM of dNTP, 0.2 pmol of each primer and 1 U of Taq DNA polymerase (Invitrogen) with sufficient water for 25 μl of reaction. Primers used and PCR conditions were as previously described (Dallenne et al., 2010; Alves et al., 2014). The genomic context of blaCTX–M was characterized by PCR-targeting ISEcp1, IS26, orf477 and IS903, as previously described (Tacão et al., 2012). A negative and a positive control were included in each PCR experiment. The negative control differed from the reaction mixture by substituting DNA for the same volume of sterile dH2O. The amplicons were visualized on 1% agarose gels using the 1 kb Plus DNA ladder (Invitrogen) to assist in the identification of the PCR products.
Mating Assays
Mating assays were performed for blaCTX–M-positive strains, as previously described (Moura et al., 2012). In short, donor strains and the rifampicin-resistant E. coli CV601 (recipient strain) were grown overnight in Luria–Bertani broth (LB) at 37°C, 180 rpm. Donor and recipient strains were mixed at a 1:1 ratio and centrifuged (5 min, 7,000 g) to precipitate cells. After discarding the supernatant, 1 mL of fresh LB was added and left overnight at 37°C, without shaking. Then, cells were centrifuged (5 min, 7,000 g) and resuspended in a 0.9% NaCl solution. Putative transconjugants were selected by plating 100 μL of this suspension in plate count agar (PCA) supplemented with rifampicin (100 μg/mL), and cefotaxime (8 μg/mL). To confirm the identity of the transconjugants we used BOX-PCR typing (Versalovic et al., 1994) and blaCTX–M PCR amplification as described above.
Genome Sequencing, Assembly and Analysis
Two multi-drug resistant isolates were selected randomly to represent phylogenetic groups with high clinical relevance (i.e., Acinetobacter baumanii and E. coli) and their genome was sequenced. Genomic DNA, extracted as described in Section “DNA Extraction and Identification of the Isolates,” and sequenced in the Ion Torrent Personal Genome Machine (Thermo Fisher Scientific) using chip 318 v.2 according to the manufacturer’s protocol. The quality of the reads was visualized using FastQC2. The reads were trimmed, discarding bases with Phred values below 20, and filtered, discarding reads with less than 100 nucleotides. The reads were assembled in contigs using the software MIRA 4 (Chevreux et al., 2004). Redundant contigs were removed using the SeqMan Pro tool of the Lasergene software (DNASTAR). The sequenced genomes were submitted to the GenBank database under the accession numbers PKCA01000000 (E. coli APC43A) and PYSX01000000 (A. baumannii APC25).
The contigs were ordered in scaffolds with MAUVE (Darling et al., 2004). Automatic genome annotation was performed in RAST (Rapid Annotation using System Technology) (Aziz et al., 2008). The RAST SEED subsystems (Overbeek et al., 2014), CARD (Comprehensive Antibiotic Resistance Database) (McArthur et al., 2013) and Resfinder v.2.1 (Zankari et al., 2012) were used to search for resistance genes in the sequenced genomes.
An in silico analysis of Plasmid Multilocus Sequence Typing (MLST) was performed using the web tool pMLST v.1.8 (Larsen et al., 2012) available at the site of the Center for Genomic Epidemiology3. PlasmidFinder v.1.3 (Carattoli et al., 2014) was used for detection of plasmid sequences, PathogenFinder v.1.1 (Cosentino et al., 2013) was used to determine the strains’ pathogenicity level, SerotypeFinder v.1.1 (Joensen et al., 2015) was used for serotyping, and VirulenceFinder v.1.5 (Joensen et al., 2014) was used to detect virulence determinants.
Pathogenicity Islands (PAIs) and Resistance Islands (RIs) were predicted using the software GIPSy v.1.1.2 (Soares et al., 2016). E. coli K-12 substr. MG1655 (NC_000913.3) and Acinetobacter calcoaceticus CA16 (NZ_CP020000.1) were used as reference strains. The nucleotide sequence of each PAI and RI were recovered using the genome browser Artemis v.14.0.0 (Rutherford et al., 2000). In order to determine the location of PAIs and RIs, we designed a circular map using BLASTn in the software BRIG (Blast Ring Image Generator) (Alikhan et al., 2011).
A phylogenomic approach was used to determine the isolates species affiliation. Genomes used for comparison were obtained from GenBank. Four phylogenetic markers: 16S rRNA, rpoB, gyrB, and dnaJ were used to calculate a distance matrix based on a BLASTn comparison all-against-all in the software Gegenees v.2.2.1 (Ågren et al., 2012).
Results and Discussion
Water Quality and Characterization of Cultivable Antibiotic-Resistant Bacteria
The majority of physical, chemical and microbiological parameters were within the limits established by the Brazilian law for freshwater environments intended for human consumption after appropriate treatment (Supplementary Table S1). However, BOD in sampling points 1, 4, 5, and 6 was above the recommended values. Additionally, dissolved oxygen (DO) concentration was below the limit in all sampling points analyzed. These two results suggest high oxygen consumption by the microbial community in Lake Água Preta during the sampling period.
For this study, sampling was performed only in October, and seasonal variation was not assessed. The temperature in this geographic region, immediately below the equator, is high throughout the year, though there are significant differences in terms of rainfall. The decision to sample in the dry season (July to November) was due to logistics issues related to lake access. However, in future studies it would be interesting to evaluate seasonal factors that may affect water quality and antibiotic resistance in Lake Água Preta.
Thirty-three isolates were obtained in this study (Table 1). Isolates affiliated mostly to genus Escherichia (7 isolates), followed by genera Acinetobacter, Enterobacter, and Klebsiella (5 isolates each), Pseudomonas (4 isolates), Shigella (3 isolates), and Chromobacterium, Citrobacter, Leclercia and Phytobacter (1 isolate each).
Most isolates were classified as multi-drug resistant (29/33–88%), meaning resistant to at least three classes of antibiotics. All isolates showed resistance to penicillins such as amoxicillin, ampicillin or both (Table 1), and 79% were also resistant when the penicillin (amoxicillin) was combined with a beta-lactamase inhibitor (clavulanic acid). Twenty-one of the 33 isolates showed resistance to cefotaxime (63.6%) and six showed intermediate resistance (18.2%). Resistance to carbapenems was detected only in the Chromobacterium isolate (Table 1). This genus has been commonly isolated from aquatic ecosystems and presents intrinsic resistance to these last-resort antibiotics (Lima-Bittencourt et al., 2011). The importance of Chromobacterium as progenitor of KPC carbapenemases has been recently discussed (Gudeta et al., 2016). For non-beta-lactam antibiotics, high levels of resistance or intermediate resistance were observed against aminoglycosides (76% of resistant isolates), tetracycline (64%), ciprofloxacin (58%) and the combination trimethoprim/sulfamethoxazole (55%). These results are in accordance with previous studies, which reported high levels of multi-drug resistance among strains resistant to third generation cephalosporins (Tacão et al., 2014). The presence of multi-drug resistant bacteria in natural aquatic systems may result from several anthropogenic pressures (Taylor et al., 2011; Tacão et al., 2012). The values of BOD and DO within Lake Água Preta are consistent with an impacted environment. An important cause may be the disposal of untreated sewage, resulting from an increasing number of illegal houses constructed along the margins. As in other geographic locations (e.g., Alves et al., 2014), wild life may also contribute to antibiotic resistance spread in this region. Finally, the presence of sub-lethal concentrations of antibiotics in aquatic systems has been reported to select for antibiotic resistant bacteria. In Brazil, until recently, antibiotics were among the most consumed medical drugs, and sold without medical prescription (Mattos et al., 2017). This situation might have contributed to the contamination of aquatic systems. These systems have been reported to act as reservoirs and to promote the transfer of antibiotic resistance genes among bacteria, thus contributing to multi-drug resistance spread.
The most frequently detected beta-lactamase gene was blaCTX–M (n = 12 positive isolates), followed by blaTEM (n = 5) and blaSHV (n = 4) (Table 1). As in our study, CTX-M is the most frequently reported ESBL worldwide (Tacão et al., 2012; Bevan et al., 2017). Carbapenemase genes blaIMP, blaV IM, and blaKPC were not detected among the isolates. Of the 22 isolates resistant to third generation cephalosporins, the gene blaCTX–M was not detected in 10. These isolates affiliated to the genera Acinetobacter (n = 3), Pseudomonas (n = 2), Citrobacter (n = 1), Enterobacter n = 1), Phytobacter (n = 1), Chromobacterium (n = 1) and Klebsiella (n = 1). The blaSHV is known to be intrinsic to Klebsiella pneumoniae (Babini and Livermore, 2000). Although we have used two sets of primers targeting this gene, under the conditions tested it was not detected in two of the isolates that affiliated with this species, including isolate API34 which showed resistance to cefotaxime. This result may be related to primer-template mismatches or to the affiliation of these isolates to a different Klebsiella species. Resistance to cefotaxime in Klebsiella spp. may be related with overproduction of other chromosomal beta-lactamases (e.g., blaOXY, blaLEN, blaOKP) due to mutations in the gene promoter regions (Hæggman et al., 2004). Overexpression of chromosomal beta-lactamases may also be the mechanism responsible for resistance to third-generation cephalosporins in isolates affiliated to other bacterial genera such as Enterobacter, Citrobacter, Chromobacterium, and Pseudomonas (intrinsic blaAmpC; Jacoby, 2009), or Acinetobacter (e.g., blaADC genes; Zhong et al., 2008). The blaCTX–M–15 gene was found in 5 isolates (affiliated with genera Klebsiella, Escherichia and Shigella), the blaCTX–M–14 gene was found in 1 isolate (affiliated with Escherichia), and the blaCTX–M–2 gene was detected in only 1 isolate (affiliated with Shigella). These variants have previously been reported in Brazil in both clinics and environmental settings (Dropa et al., 2016; Rocha et al., 2016; Nascimento et al., 2017; Sellera et al., 2017). For the remaining blaCTX–M-positive isolates, it was only possible to sequence a portion of the gene, insufficient to accurately determine its variant. For these isolates, PCR products were not obtained with the primers used to characterize the genomic context of blaCTX–M. ISEcp1 was found in the upstream region of all blaCTX–M–15 and blaCTX–M–14 genes. Downstream, all blaCTX–M–15 genes presented Orf477 and blaCTX–M–14 presented the insertion sequence IS903. The same contexts were previously reported for these genes in clinical and environmental isolates worldwide (Eckert et al., 2006; Tacão et al., 2012). Particularly, the association of ISEcp1 element with ESBL genes seems to be one of the reasons for the successful spread of these genes, being a major concern in clinical settings (Tian et al., 2011). The genetic context of blaCTX–M–2 carried by Shigella sp. APC22 was identical to that previously described (Eckert et al., 2006): an upstream region with a sul1 gene (encoding resistance to sulfonamides) followed by an ISCR1 element; and downstream an open reading frame designated Orf3, followed by qacEdelta1 (encoding for a quaternary ammonium compound resistance protein) and a sul1 gene. These CR-like elements are usually associated to complex class 1 integrons, usually identified between duplications of 3’conserved sequence (CS) regions, along with antibiotic resistance genes like blaCTX–M–2 (Toleman et al., 2006).
Conjugations assays were performed for nine out of twelve blaCTX–M-positive isolates. Three isolates were able to grow on rifampicin and were excluded from these experiments. Under the used conjugation conditions, 4 out of 9 donor strains generated transconjugants carrying blaCTX–M. In contrast with the recipient strain E. coli CV601, all transconjugants showed MIC for cefotaxime from 32 to >256 μg/mL, while for ceftazidime MICs varied from 2 to 8 μg/mL (Supplementary Table S2). Overall, the association of blaCTX–M genes to conjugative plasmids in these isolates was confirmed indicating that their mobilization to different hosts may be facilitated.
Previous studies highlighted the important contribution of integrons to multi-drug resistance profiles among ESBL-producers (Tacão et al., 2014). In this study, the integrase genes intI1 and intI2 were detected in 4 and 5 isolates, respectively (Table 1). All but one of these isolates were positive for the blaCTX–M gene.
Genomic Analysis of Two Multi-Drug Resistant Isolates
To obtain an in-depth characterization of the resistome of selected isolates, as well as insights into their mobilome and virulence potential, two isolates (i.e., E. coli APC43A and Acinetobacter baumannii APC25) were selected for whole genome sequencing. These isolates were randomly selected among isolates that: (1) belong to bacterial groups of public health concern, (2) presented multi-drug resistance profiles.
Identification at species level was confirmed using a phylogenomic approach as described in Material and Methods. Both strains were resistant to all beta-lactams except to imipenem (APC43A) or to imipenem and cefepime (APC25). Additionally, strains showed resistance to ciprofloxacin, nalidixic acid, and an intermediate susceptibility to kanamycin. Summary of both strains genomic features is presented in Table 2.
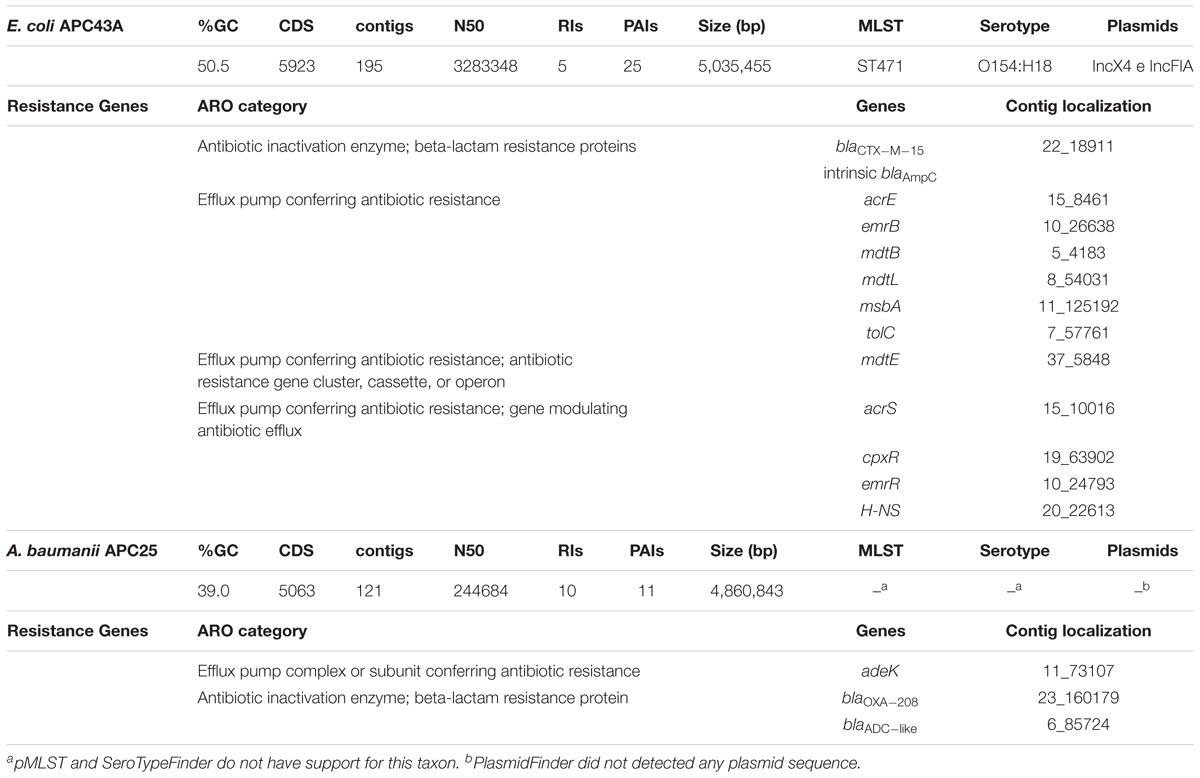
Table 2. Major genomic features of two isolates from Lake Água Preta and resistance genes annotated by CARD and/or ResFinder.
Escherichia coli APC43A Genomic Analysis
For E. coli APC43A the RAST server classified 162 CDSs in the subsystem of Virulence, Disease and Defense (3.2% of total genes) (Supplementary Table S3). Among them, 122 were genes related to antibiotic resistance and toxic compounds. Two beta-lactamase genes were predicted in the genome. As described above, blaCTX–M–15 gene was located between ISEcp1 and orf477. Genomic analysis revealed that a transposase gene followed orf477 and that two fragments of a truncated gene encoding a MATE efflux family protein flanked this entire region (Figure 2). This region showed identity values higher than 99% and coverage higher than 93% with the genomes of K. pneumoniae AR 0138 (CP021757.1) and E. coli K-15KW01 (CP016358.1) (Zurfluh et al., 2016). In E. coli K-15KW01 the blaCTX–M–15 gene was embedded at the right-hand extremity of an ISEcp1 element (Figure 2). In our strain APC43A, the inverted repeat sequence (IRR) (ACGTGGAATTTAGG), and the −35 (TTGAAA) and −10 (TACAAT) sites of the ISEcp1 element were conserved 48 base pairs upstream of the ATG start codon of blaCTX–M–15 (Figure 2). The annotation of the other identified beta-lactamase gene was evaluated by comparing its nucleotide sequence to the Uniprot database through BLASTn. The gene showed high identity with an intrinsic AmpC beta-lactamase encoding gene (above 99%), emphasizing its correct annotation. Mutations previously related to enzyme overexpression (Jacoby, 2009) were not detected in the blaAmpC gene promoter. Besides beta-lactamase genes, genes encoding resistance to other classes of antibiotics were detected in the genome of strain APC43A, mostly related with efflux pumps (Table 2).
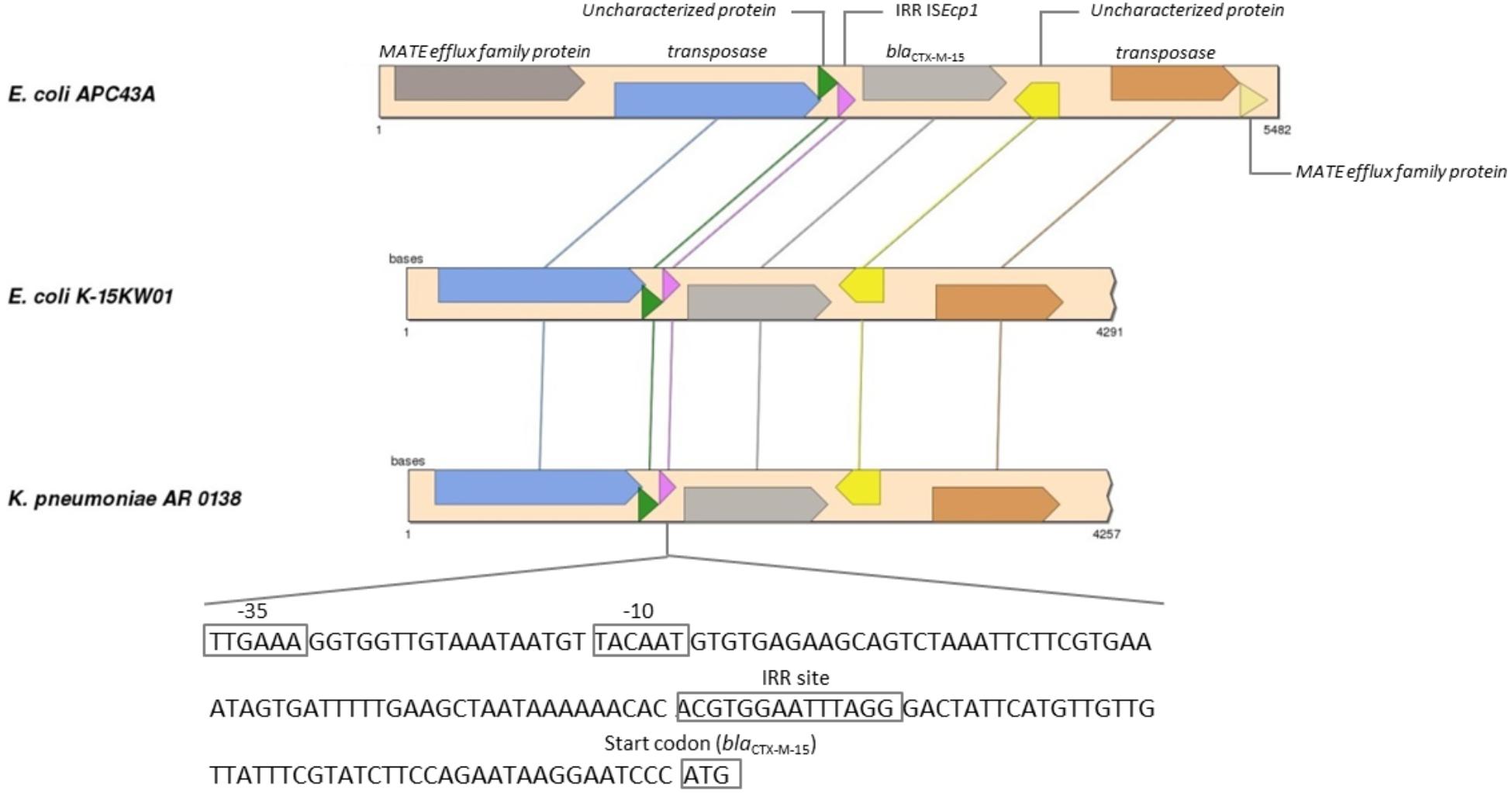
Figure 2. Synteny analysis of ISEcp1 element carrying blaCTX–M–15 gene in Escherichia coli APC43A. Conserved genes are connected by lines. The pink arrow represents the ISEcp1 element that is described in detail at the bottom of the figure. E. coli APC43A contains two CDSs identified as MATE efflux family proteins flanking the IS element. These CDSs are complementary and probably are part of the same gene that was fragmented during the insertion of the IS element.
Sequences representing two plasmids, assigned to the incompatibility groups IncX4 and IncFIA, were detected in the genome of E. coli APC43A (Table 2). The contig corresponding to replicon IncX4 has a size of 30,306 bp, which is very similar to the size of E. coli IncX4 plasmids in the GenBank database (e.g., accession number JX981514.1). This plasmid was detected in the porcine enterotoxigenic strain E. coli UMNF18 carrying genes for type II secretion system (Shepard et al., 2012). IncFIA is a fertility plasmid of E. coli and part of this plasmid was detected in a 9,933 bp contig. No resistance genes were found within plasmids.
PathogenFinder analysis showed that E. coli APC43A is a human pathogen and the SerotypeFinder tool classified this strain in the O154:H18 serotype. Six virulence factors (gad, lpfA, ltcA, astA, cba e cma) normally found in pathogenic E. coli were detected in the genome of E. coli APC43A. These virulence genes are involved in host-pathogen interaction during gastrointestinal infections caused by ingestion of contaminated food or water (Joensen et al., 2014). The strain was assigned to ST471, a high-risk clone previously reported in clinical settings and commonly associated with ESBL genes and genes encoding carbapenemases (Kapmaz et al., 2016; Yi et al., 2017). In Brazil, this sequence type was described in clinical isolates from Rio de Janeiro (Peirano et al., 2011).
Acinetobacter baumannii APC25 Genomic Analysis
High levels of intrinsic resistance to a number of antibiotics have been reported for A. baumanii, seriously compromising the treatment of patients infected with this pathogen. Intrinsic resistance mechanisms in members of this species include the production of chromosomal beta-lactamases and aminoglycoside-modifying enzymes, expression of efflux pumps and permeability defects. Nevertheless, A. baumanii is also known for its ability to acquire genes encoding resistance determinants.
For the genome of A. baumannii APC25 the RAST server classified 109 CDSs in the subsystem of Virulence, Disease and Defense (2.6% of total genes) (Supplementary Table S4). Eighty-three of these 109 CDSs are related to resistance to antibiotics and toxic compounds. The beta-lactamase genes blaOXA–208 and blaADC–like (98% similar to blaADC–25) were detected (Table 2). Both genes were previously reported as intrinsic genetic determinants in the chromosome of A. baumannii (Zhao and Hu, 2012). blaOXA–208 encodes an OXA-51-like chromosomally encoded beta-lactamase (Evans and Amyes, 2014). Clinically relevant oxacillinases have been reported in clinical isolates from sixteen states in Brazil, mostly OXA-23 and OXA-143 (Medeiros and Lincopan, 2013). The blaADC–25 encodes a cephalosporinase recently described to confer resistance to second and third generation cephalosporins (Zhong et al., 2008; Lee et al., 2012), a result that is in line with the antibiotic susceptibility profile of strain APC25.
Plasmids were not detected in A. baumannii APC25 and the isolate was not predicted as a human pathogen by the PathogenFinder tool (Supplementary Table S5). MLST sequences were uploaded to the Acinetobacter-MLST Pasteur database and since an unreported allele combination was observed, a new sequence type (ST1278) was assigned.
Resistance to Metals and Genomic Islands Prediction
Operons related to resistance to metals were determined in the sequenced strains. E. coli APC43A possesses incomplete mercury resistance operons (Supplementary Figure S1). In addition, the two-component system cusR-cusS and the efflux pump cusCFBA, described as responsible for copper and silver resistance in other strains of E. coli (Gudipaty and McEvoy, 2014), were annotated in the genome. In A. baumannii APC25, the zinc, cadmium, and cobalt resistance may be mediated by the operon czcABC, which was found duplicated in its genome (Supplementary Figure S1). Both genomes showed operons for resistance to arsenic. A. baumannii APC25 has an operon composed by an arsenical resistance-3 (ACR3) family protein, while E. coli APC43A has an arsRBC type operon (Supplementary Figure S1). Several studies have showed that some pollutants such as metals could co-select for antibiotic resistance (Wright et al., 2008; Rosewarne et al., 2010; Henriques et al., 2016). However, the level of aluminum, manganese, nickel, cadmium, copper and zinc in Lake Água Preta was in accordance to the standard values for mesotrophic lakes (Supplementary Table S1).
Twenty-five PAIs and five RIs were identified in the genome of E. coli APC43A (Figure 3). The location of the islands is shown in the comparative ring of Figure 4. It is worth noting that these islands are almost completely absent in the genome of the non-pathogenic E. coli K-12 (Figure 3). Interestingly, among the detected resistance genes only the gene mdtB was within a GEI (EcPAI16), suggesting that these resistance islands may encode resistance to other classes of compounds. In some cases, the program identified PAIs and RIs in the same genome region, e.g., EcPAI5 and EcRI1, which means that these regions may encode both resistance and virulence factors.
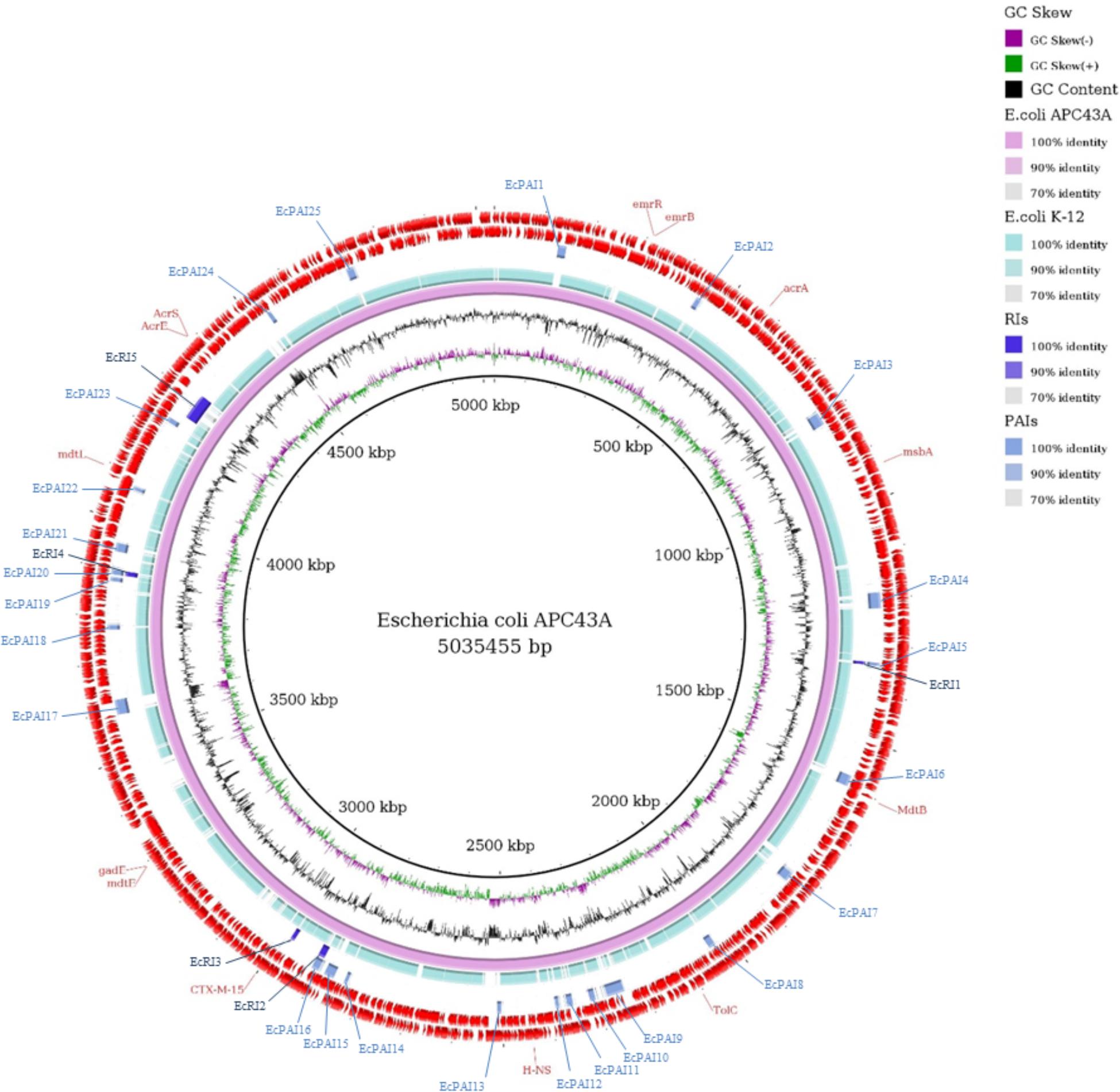
Figure 3. Comparative genomic ring designed in Gegenees program. The innermost ring to the outermost is presented in this figure, as follows: GC skew and the GC content of E. coli APC43A; the genome sequence of E. coli APC43A and E. coli K-12; the Resistance Islands (RIs) detected by GIPSy; the Pathogenicity Islands (PAIs) detected by GIPSy; CDSs identified in the genome of E. coli APC43A. The location of the resistance genes detected by CARD and ResFinder are shown and identified by their respective names in red.
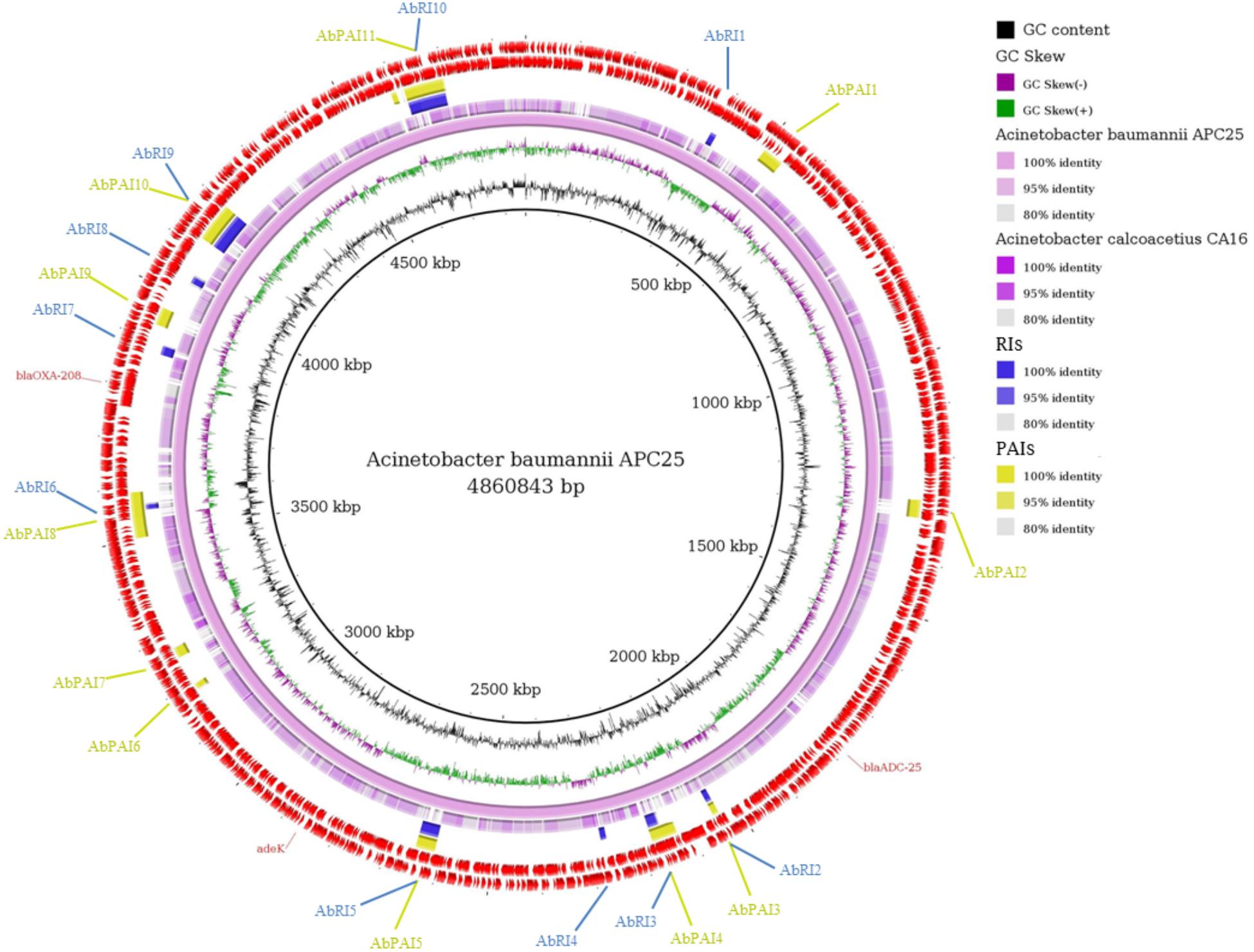
Figure 4. Comparative genomic ring designed in Gegenees program. The innermost ring to the outermost is presented in this figure, as follows: GC skew and the GC content of Acinetobacter baumannii APC25; the genome sequence of A. baumannii APC25 and A. calcoacetius CA16; the Resistance Islands (RIs) detected by GIPSy; the Pathogenicity Islands (PAIs) detected by GIPSy; CDSs identified in the genome of A. baumannii APC25. The location of the resistance genes detected by CARD and ResFinder are shown and identified by their respective names in red.
The genome of A. baumannii APC25 has 11 PAIs and 10 RIs (Figure 4). The low number of PAIs is in accordance with the prediction of PathogenFinder that classified the isolate as a non-pathogenic strain. The majority of PAIs and RIs were found in the same location of the genome similar to that observed for E. coli (Figure 4). No resistance genes predicted by CARD or ResFinder were located within GEI.
Conclusion
Lake Água Preta is an Amazonian mesotrophic lake located near a densely populated area that presented physical, chemical and microbiological parameters in accordance to the Brazilian environmental laws, with some exceptions. The majority of bacterial strains (29 out of 31; 88%) isolated from the lake, in media supplemented with cefotaxime, were multi-drug resistant, classified in the Enterobacteriaceae family, and carried ESBL genes, primarily blaCTX–M. In some cases the transfer potential of these genes were confirmed in conjugation assays. These results suggest a high dissemination of ESBL genes in Gram-negative bacteria of Lake Água Preta, which although not presenting characteristics of a highly impacted environment, contains multi-drug resistant pathogenic strains such as E. coli APC43A (ST471).
Author Contributions
AS, IH, AF, and RB conceived and designed the experiments. DF, SA, JA, and MT performed the experiments. DF, RR, and RB were involved in genome analysis. DF, SA, MT, RB, and IH prepared the manuscript.
Funding
This work was funded by the international cooperation project “ARTEMan: Antibiotic resistance transfer between environmental and human settings.” financed by the Brazilian agency Coordenação de Aperfeioamento de Pessoal de Nível Superior (CAPES) and the Portuguese agency Fundação para a Ciência e Tecnologia (FCT). Thanks are due for the financial support to CESAM (UID/AMB/50017/2019), to FCT/MEC through national funds, and the co-funding by the FEDER, within the PT2020 Partnership Agreement and Compete 2020. The authors acknowledge FCT financing to IH (FCT Investigator Program – IF/00492/2013), MT (SFRH/114855/2016), and SA (SFRH/BD/52573/2014). RB would like to thank the financial support of the Fundao Amaznia de Amparo a Estudos e Pesquisas (FAPESPA) (grant number 2155/2017).
Conflict of Interest Statement
The authors declare that the research was conducted in the absence of any commercial or financial relationships that could be construed as a potential conflict of interest.
Supplementary Material
The Supplementary Material for this article can be found online at: https://www.frontiersin.org/articles/10.3389/fmicb.2019.00364/full#supplementary-material
Footnotes
- ^ http://www.ncbi.nlm.nih.gov/
- ^ http://www.bioinformatics.babraham.ac.uk/projects/fastqc/
- ^ http://www.genomicepidemiology.org/
References
Ågren, J., Sundström, A., Håfström, T., and Segerman, B. (2012). Gegenees: fragmented alignment of multiple genomes for determining phylogenomic distances and genetic signatures unique for specified target groups. PLoS One 7:e39107. doi: 10.1371/journal.pone.0039107
Alikhan, N. F., Petty, N. K., Bem Zakour, N. L., and Beatson, S. A. (2011). BLAST Ring Image Generator (BRIG): simple prokaryote genome comparisons. BMC Genomics 12:402. doi: 10.1186/1471-2164-12-402
Alves, M. S., Pereira, A., Araujo, S. M., Castro, B. B., Correia, A. C. M., and Henriques, I. (2014). Seawater is a reservoir of multi-resistant Escherichia coli, including strains hosting plasmid-mediated quinolones resistance and extended-spectrum beta-lactamases genes. Front. Microbiol. 5:426. doi: 10.3389/fmicb.2014.00426
Ambler, R. P. (1980). Structure of β-lactamases. Philos. Trans. Royal Soc. London B Biol. Sci. 289, 321–331.
Aziz, R. K., Bartels, D., Best, A. A., DeJongh, M., Disz, T., Edwards, R. A., et al. (2008). The RAST Server: rapid annotations using subsystems technology. BMC Genomics 9:75. doi: 10.1186/1471-2164-9-75
Babini, G. S., and Livermore, D. (2000). Are SHV β-Lactamases universal in Klebsiella pneumoniae? Antimicrob. Agents Chemother. 44:2230.
Baquero, F., Martinez, J. L., and Cantón, R. (2008). Antibiotics and antibiotic resistance in water environments. Curr. Opin. Biotechnol. 19, 260–265. doi: 10.1016/j.copbio.2008.05.006
Bauer, A. W., Kirby, W. M., Sherris, J. C., and Turck, M. (1966). Antibiotic susceptibility testing by a standardized single disk method. Tech. Bull. Regist. Med. Technol. 36, 49–52.
Bevan, E. R., Jones, A. M., and Hawkey, P. M. (2017). Global epidemiology of CTX-M beta-lactamases: temporal and geographical shifts in genotype. J. Antimicrob. Chemother. 72, 2145–2155. doi: 10.1093/jac/dkx146
Carattoli, A., Zankari, E., García-Fernández, A., Voldby Larsen, M., Lund, O., Villa, L., et al. (2014). In silico detection and typing of plasmids using Plasmid Finder and plasmid multilocus sequence typing. Antimicrob. Agents Chemother. 58, 3895–3903. doi: 10.1128/AAC.02412-14
Chevreux, B., Pfisterer, T., Drescher, B., Driesel, A. J., Muller, W. E., Wetter, T., et al. (2004). Using the miraEST assembler for reliable and automated mRNA transcript assembly and SNP detection in sequenced ESTs. Genome Res. 14, 1147–1159.
CLSI (2017). Performance Standard for Antimicrobial Susceptibility Testing - Document Approved Standard M100-S27. Wayne, PA: CLSI.
CONAMA (2005). Ministério do Meio Ambiente Conselho Nacional do Meio Ambiente. Resolução CONAMA n° 357/2005. Brasília: Diário Oficial da República Federativa do Brasil.
Cosentino, S., Voldby Larsen, M., Møller Aarestrup, F., and Lund, O. (2013). PathogenFinder - distinguishing friend from foe using bacterial whole genome sequence data. PLoS One 8:e77302. doi: 10.1371/journal.pone.0077302
Dallenne, C., Da Costa, A., Decré, D., Favier, C., and Arlet, G. (2010). Development of a set of multiplex PCR assays for the detection of genes encoding important beta-lactamases in Enterobacteriaceae. J. Antimicrob. Chemother. 65, 490–495. doi: 10.1093/jac/dkp498
Darling, A. C. E., Mau, B., Blattner, F. R., and Perna, N. T. (2004). Mauve: multiple alignment of conserved genomic sequence with rearrangements. Genome Res. 14, 1394–1403.
de Oliveira, D. V., Nunes, L. S., Barth, A. L., and Van Der Sand, S. T. (2017). Genetic background of beta-lactamases in Enterobacteriaceae isolates from environmental samples. Microbial Ecol. 74, 599–607. doi: 10.1007/s00248-017-0970-6
Dropa, M., Lincopan, N., Balsalobre, L. C., Oliveira, D. E., Moura, R. A., Fernandes, M. R., et al. (2016). Genetic background of novel sequence types of CTX-M-8- and CTX-M-15-producing Escherichia coli and Klebsiella pneumoniae from public wastewater treatment plants in São Paulo, Brazil. Environ. Sci. Pollut. Res. Int. 23, 4953–4958. doi: 10.1007/s11356-016-6079-5
ECDC (2017). European Centre for Disease Prevention and Control. Antimicrobial resistance surveillance in Europe 2016. Annual Report of the European Antimicrobial Resistance Surveillance Network (EARS-Net). Stockholm: ECDC.
Eckert, C., Gautier, V., and Arlet, G. (2006). DNA sequence analysis of the genetic environment of various blaCTX-M genes. J. Antimicrob. Chemother. 57, 14–23.
Evans, B. A., and Amyes, S. G. B. (2014). OXA beta-Lactamases. Clin. Microbiol. Rev. 27, 241–263. doi: 10.1128/CMR.00117-13
Gudeta, D. D., Bortolaia, V., Jayol, A., Poirel, L., Nordmann, P., and Guardabassi, L. (2016). Chromobacterium spp. harbour Ambler class A beta-lactamases showing high identity with KPC. J. Antimicrob. Chemother. 71, 1493–1496. doi: 10.1093/jac/dkw020
Gudipaty, S. A., and McEvoy, M. M. (2014). The histidine kinase CusS senses silver ions through direct binding by its sensor domain. Biochim. Biophys. Acta 1844, 1656–1661. doi: 10.1016/j.bbapap.2014.06.001
Hall, T. (1999). Bioedit: a user-friendly biological sequence alignment editor and analysis program for Windows 95/96/NT. Nucleic Acids Ser. 41, 95–98.
Henriques, I., Tacão, M., Leite, L., Fidalgo, C., Araújo, S., Oliveira, C., et al. (2016). Co-selection of antibiotic and metal(loid) resistance in gram-negative epiphytic bacteria from contaminated salt marshes. Mar. Pollut. Bull. 109, 427–434. doi: 10.1016/j.marpolbul.2016.05.031
Hæggman, S., Löfdahl, S., Paauw, A., Verhoef, J., and Brisse, S. (2004). Diversity and evolution of the class A chromosomal beta-lactamase gene in Klebsiella pneumoniae. Antimicrob. Agents Chemother. 48, 2400–2408. doi: 10.1128/AAC.48.7.2400-2408.2004
Jacoby, G. A. (2009). AmpC -Lactamases. Clin. Microbiol. Rev. 22, 161–182. doi: 10.1128/CMR.00036-08
Jiao, Y. N., Chen, H., Gao, R. X., Zhu, Y. G., and Rensing, C. (2017). Organic compounds stimulate horizontal transfer of antibiotic resistance genes in mixed wastewater treatment systems. Chemosphere 184, 53–61. doi: 10.1016/j.chemosphere.2017.05.149
Joensen, K. G., Scheutz, F., Lund, O., Hasman, H., Kaas, R. S., Nielsen, E. M., et al. (2014). Real-time whole-genome sequencing for routine typing, surveillance, and outbreak detection of verotoxigenic Escherichia coli. J. Clin. Microbiol. 52, 1501–1510. doi: 10.1128/JCM.03617-13
Joensen, K. G., Tetzschner, A. M., Iguchi, A., Aarestrup, F. M., and Scheutz, F. (2015). Rapid and easy in silico serotyping of Escherichia coli isolates by use of whole-genome sequencing data. J. Clin. Microbiol. 53, 2410–2426. doi: 10.1128/JCM.00008-15
Kapmaz, M., Erdem, F., Abulaila, A., Yeniaras, E., Oncul, O., and Aktas, Z. (2016). First detection of NDM-1 with CTX-M-9, TEM, SHV and rmtC in Escherichia coli ST471 carrying IncI2, A/C and Y plasmids from clinical isolates in Turkey. J. Glob. Antimicrob. Resist. 7, 152–153. doi: 10.1016/j.jgar.2016.10.001
Larsen, M. V., Cosentino, S., Rasmussen, S., Friis, C., Hasman, H., Marvig, R. L., et al. (2012). Multilocus Sequence Typing of total genome sequenced bacteria. J. Clin. Micobiol. 50, 1355–1361. doi: 10.1128/JCM.06094-11
Lee, H. Y., Chang, R. C., Su, L. H., Liu, S. Y., Wu, S. R., Chuang, C. H., et al. (2012). Wide spread of Tn2006 in an AbaR4-type resistance island among carbapenem-resistant Acinetobacter baumannii clinical isolates in Taiwan. Int. J. Antimicrob. Agents 40, 163–167. doi: 10.1016/j.ijantimicag.2012.04.018
Lima-Bittencourt, C. I., Costa, P. S., Barbosa, F. A., Chartone-Souza, E., and Nascimento, A. M. (2011). Characterization of a Chromobacterium haemolyticum population from a natural tropical lake. Lett. Appl. Microbiol. 52, 642–650. doi: 10.1111/j.1472-765X.2011.03052.x
Marti, E., Variatza, E., and Balcazar, J. L. (2014). The role of aquatic ecosystems as reservoirs of antibiotic resistance. Trends Microbiol. 22, 36–41. doi: 10.1016/j.tim.2013.11.001
Mattos, K. P. H., Visacri, M. B., Quintanilha, J. C. F., Lloret, G. R., Cursino, M. A., Levin, A. S., et al. (2017). Brazil’s resolutions to regulate the sale of antibiotics: impact on consumption and Escherichia coli resistance rates. J. Glob. Antimicrob. Resist. 10, 195–199. doi: 10.1016/j.jgar.2017.05.023
McArthur, A. G., Waglechner, N., Nizam, F., Yan, A., Azad, M. A., Baylay, A. J., et al. (2013). The comprehensive antibiotic resistance database. Antimicrob. Agents Chemother. 57, 3348–3357. doi: 10.1128/AAC.00419-13
Medeiros, M., and Lincopan, N. (2013). Oxacillinase (OXA)-producing Acinetobacter baumannii in Brazil: clinical and environmental impact and therapeutic options. J. Bras. Patol. Med. Lab. 49, 391–405. doi: 10.1590/S1676-24442013000600003
Moura, A., Oliveira, C., Henriques, I., Smalla, K., and Correia, A. (2012). Broad diversity of conjugative plasmids in integron-carrying bacteria from wastewater environments. FEMS Microbiol. Lett. 330, 157–164. doi: 10.1111/j.1574-6968.2012.02544.x
Nascimento, T., Cantamessa, R., Melo, L., Lincopan, N., Fernandes, M. R., Cerdeira, L., et al. (2017). International high-risk clones of Klebsiella pneumoniae KPC-2/CC258 and Escherichia coli CTX-M-15/CC10 in urban lake waters. Sci. Total Environ. 598, 910–915. doi: 10.1016/j.scitotenv.2017.03.207
Overbeek, R., Olson, R., Pusch, G. D., Olsen, G. J., Davis, J. J., Disz, T., et al. (2014). The SEED and the Rapid annotation of microbial genomes using subsystems technology (RAST). Nucleic Acids Res. 42, D206–D214. doi: 10.1093/nar/gkt1226
Pei, R., and Gunsch, C. K. (2009). Plasmid conjugation in an activated sludge microbial community. Environ. Eng. Sci. 26, 825–831. doi: 10.1089/ees.2008.0236
Peirano, G., Asensi, M. D., Pitondo-Silva, A., and Pitout, J. D. D. (2011). Molecular characteristics of extended-spectrum beta-lactamase-producing Escherichia coli from Rio de Janeiro. Brazil. Clin. Microbiol. Infect. 17, 1039–1043. doi: 10.1111/j.1469-0691.2010.03440.x
Poirel, L., Kampfer, P., and Nordmann, P. (2002). Chromosome-encoded Ambler class A beta-lactamase of Kluyvera georgiana a probable progenitor of a subgroup of CTX-M extended-spectrum beta-lactamases. Antimicrob. Agents Chemother. 46:4038. doi: 10.1128/AAC.46.12.4038-4040.2002
Rice, E. W., Baird, R. B., Eaton, A. D., and Clesceri, L. S. (2012). Standard Methods for the Examination of Water and Wastewater. Washington, DC: APHA.
Rocha, F. R., Pinto, V. P. T., and Barbosa, F. C. B. (2016). The spread of CTX-M-type Extended-Spectrum beta-Lactamases in Brazil: a systematic review. Microb. Drug Resist. 22, 301–311. doi: 10.1089/mdr.2015.0180
Rosewarne, C. P., Pettigrove, V., Stokes, H. W., and Parsons, Y. M. (2010). Class 1 integrons in benthic bacterial communities: abundance, association with Tn402-like transposition modules and evidence for coselection with heavy metal resistance. FEMS Microbiol. Ecol. 72, 35–46. doi: 10.1111/j.1574-6941.2009.00823.x
Rutherford, K., Parkhill, J., Crook, J., Horsnell, T., Rice, P., Rajandream, M. A., et al. (2000). Artemis: sequence visualization and annotation. Bioinformatics 16, 944–945.
Santos, M. L. S., Saraiva, A. L. L., Pereira, J. A. R., Nogueira, P. F. S. M., and Silva, A. C. (2015). Hydrodynamic modelling of a reservoir used to supply water to Belém (Lake Agua Preta. Para, Brazil). Acta Sci. Technol. 37, 353–359. doi: 10.4025/actascitechnol.v37i3.25839
Sellera, F. P., Fernandes, M. R., Moura, Q., Souza, T. A., Cerdeira, L., and Lincopan, N. (2017). Draft genome sequence of Enterobacter cloacae ST520 harbouring blaKPC-2, blaCTX-M-15 and blaOXA-17 isolated from coastal waters of the South Atlantic Ocean. J. Glob. Antimicrob. Resist. 10, 279–280. doi: 10.1016/j.jgar.2017.07.017
Shepard, S. M., Danzeisen, J. L., Isaacson, R. E., Seemann, T., Achtman, M., and Johnson, T. J. (2012). Genome sequences and phylogenetic analysis of K88- and F18-positive porcine enterotoxigenic Escherichia coli. J. Bacteriol. 194, 395–405. doi: 10.1128/JB.06225-11
Soares, S. C., Geyik, H., Ramos, R. T., de Sá, P. H., Barbosa, E. G., Baumbach, J., et al. (2016). GIPSy: genomic island prediction software. J. Biotechnol. 232, 2–11. doi: 10.1016/j.jbiotec.2015.09.008
Tacão, M., Araújo, S., Vendas, M., Alves, A., and Henriques, I. (2018). Shewanella species as the origin of blaOXA-48 genes: insights into gene diversity, associated phenotypes and possible transfer mechanisms. Int. J. Antimicrob. Agents. 51, 340–348. doi: 10.1016/j.ijantimicag.2017.05.014
Tacão, M., Correia, A., and Henriques, I. (2012). Resistance to broad-spectrum antibiotics in aquatic systems: anthropogenic activities modulate the dissemination of blaCTX-M-like genes. App. Environ. Microbiol. 78, 4134–4140. doi: 10.1128/AEM.00359-12
Tacão, M., Moura, A., Correia, A., and Henriques, I. (2014). Co-resistance to different classes of antibiotics among ESBL-producers from aquatic systems. Water Res. 48, 100–107. doi: 10.1016/j.watres.2013.09.021
Taylor, N. G., Verner-Jeffreys, D. W., and Baker-Austin, C. (2011). Aquatic systems: maintaining, mixing and mobilising antimicrobial resistance? Trends Ecol. Evol. 26, 278–284. doi: 10.1016/j.tree.2011.03.004
Tian, S. F., Chu, Y. Z., Chen, B., Nian, H., and Shang, H. (2011). ISEcp1 element in association with blaCTX-M genes of E. coli that produce extended-spectrum beta-lactamase among the elderly in community settings. Enferm. Infecc. Microbiol. Clin. 29, 731–734. doi: 10.1016/j.eimc.2011.07.011
Toleman, M. A., Bennett, P. M., and Walsh, T. R. (2006). ISCR elements: novel gene-capturing systems of the 21st century? Microbiol. Mol. Biol. Rev. 70, 296–316.
Versalovic, J., Schneider, M., De Bruijn, F. J., and Lupski, J. R. (1994). Genomic fingerprinting of bacteria using repetitive sequence-based polymerase chain reaction. Methods Mol. Cell. Biol. 5, 25–40.
Wright, M. S., Baker-Austin, C., Lindell, A. H., Stepanauskas, R., Stokes, H. W., and McArthur, J. V. (2008). Influence of industrial contamination on mobile genetic elements: class 1 integron abundance and gene cassette structure in aquatic bacterial communities. ISME J. 2, 417–428. doi: 10.1038/ismej.2008.8
Yi, J., Kim, N., Ko, M. K., Kim, H., Kim, S. R., Hong, S. H., et al. (2017). Epidemiological and molecular characteristics of carbapenemase-producing Enterobacteriaceae in a tertiary hospital in korea: possible emergence of KPC-producing Escherichia coli ST471 strain. Open Forum Infect. Dis. 4:S599. doi: 10.1093/ofid/ofx163.1574
Zankari, E., Hasman, H., Cosentino, S., Vestergaard, M., Rasmussen, S., Lund, O., et al. (2012). Identification of acquired antimicrobial resistance genes. J. Antimicrob. Chemother. 67, 2640–2644. doi: 10.1093/jac/dks261
Zhao, W. H., and Hu, Z. Q. (2012). Acinetobacter: a potential reservoir and dispenser for beta-lactamases. Crit. Rev. Microbiol. 38, 30–51. doi: 10.3109/1040841X.2011.621064
Zhong, Z., Lu, X., Valenzuela, J. K., Partridge, S. R., and Iredell, J. (2008). An outbreak of carbapenem-resistant Acinetobacter baumannii producing OXA-23 carbapenemase in western China. Int. J. Antimicrob. Agents 31, 50–54.
Zurfluh, K., Hächler, H., Nüesch-Inderbinen, M., and Stephan, R. (2013). Characteristics of extended-spectrum β-lactamase- and carbapenemase-producing Enterobacteriaceae Isolates from rivers and lakes in Switzerland. Appl. Environ. Microbiol. 79, 3021–3026. doi: 10.1128/AEM.00054-13
Keywords: antibiotic resistance, Escherichia coli, Acinetobacter baumannii, blaCTX–M, whole genome analysis
Citation: Freitas DY, Araújo S, Folador ARC, Ramos RTJ, Azevedo JSN, Tacão M, Silva A, Henriques I and Baraúna RA (2019) Extended Spectrum Beta-Lactamase-Producing Gram-Negative Bacteria Recovered From an Amazonian Lake Near the City of Belém, Brazil. Front. Microbiol. 10:364. doi: 10.3389/fmicb.2019.00364
Received: 20 December 2017; Accepted: 12 February 2019;
Published: 28 February 2019.
Edited by:
Patrícia Poeta, University of Trás-os-Montes and Alto Douro, PortugalReviewed by:
Michael P. Ryan, University of Limerick, IrelandYvonne Pfeifer, Robert Koch Institute, Germany
Copyright © 2019 Freitas, Araújo, Folador, Ramos, Azevedo, Tacão, Silva, Henriques and Baraúna. This is an open-access article distributed under the terms of the Creative Commons Attribution License (CC BY). The use, distribution or reproduction in other forums is permitted, provided the original author(s) and the copyright owner(s) are credited and that the original publication in this journal is cited, in accordance with accepted academic practice. No use, distribution or reproduction is permitted which does not comply with these terms.
*Correspondence: Isabel Henriques, ihenriques@ua.pt