- 1Investigación y el Desarrollo, Biocultivos S.A., Bogotá, Colombia
- 2Instituto de Biotecnología, Universidad Nacional de Colombia, Bogotá, Colombia
- 3Departamento de Química. Universidad Nacional de Colombia, Bogotá, Colombia
- 4Biotechnology Development Unit, International Centre for Genetic Engineering and Biotechnology, Trieste, Italy
- 5Bacteriology and Plant Bacteriology Group, International Centre for Genetic Engineering and Biotechnology, Trieste, Italy
Bacterial Panicle Blight caused by Burkholderia glumae is a major disease of rice, which has dramatically affected rice production around the world in the last years. In this study we describe the assessment of three Streptomyces isolates as biocontrol agents for B. glumae. Additionally, the presence of other plant-growth promoting abilities and their possible beneficial effects upon their inoculation on rice plants was evaluated as an ecological analysis for their future inoculation in rice crops. Two isolates (A20 and 5.1) inhibited growth of virulent B. glumae strains, as well as a wide range of bacterial and fungal species, while a third strain (7.1) showed only antifungal activity. In vitro tests demonstrated the ability of these strains to produce siderophores, Indoleacetic acid (IAA), extracellular enzymes and solubilizing phosphate. Greenhouse experiments with two rice cultivars indicated that Streptomyces A20 is able to colonize rice plants and promote plant growth in both cultivars. Furthermore, an egfp tagged mutant was generated and colonization experiments were performed, indicating that Streptomyces A20 –GFP was strongly associated with root hairs, which may be related to the plant growth promotion observed in the gnotobiotic experiments. In order to characterize the antimicrobial compounds produced by strain A20 bacteria, mass spectrometry analyses were performed. This technique indicated that A20 produced several antimicrobial compounds with sizes below 3 kDa and three of these molecules were identified as Streptotricins D, E and F. These findings indicate the potential of Streptomyces A20 as a biocontrol inoculant to protect rice plants against bacterial diseases.
Introduction
Bacterial Panicle Blight (BPB) caused by Burkholderia glumae is a bacterial disease of rice with an increasing occurrence in South America since its first detection in 2007 (Correa et al., 2007; Castillo et al., 2011; Ham et al., 2011). This bacterial pathogen was first detected in Japan (Goto and Ohata, 1956; Tanii et al., 1976) and is currently widespread around the world. In the last 20 years, B. glumae has shown a significant occurrence in rice-growing countries in Latin America and United States causing grain rot and seedling rot of rice (Zeigler and Alvarez, 1987; Correa et al., 2007; Nandakumar et al., 2007; Diago et al., 2009; Nandakumar et al., 2009). B. glumae causes bacterial wilt in a wide variety of plant hosts, but disease in rice is the most studied due to the dramatic economic effects of BPB in rice yields (Jeong et al., 2003). Major symptoms of this BPB include panicle blight, seedling blight, and sheath rot, with a linear lesion extending downward from the leaf blade collar forms on the flag leaf. Affected panicles may have one or all of their florets blighted with grains not filling or aborting, which causes typical upright brown panicles due to the failure of grain filling (Goto and Ohata, 1956; Kurita and Tabei, 1967; Goto et al., 1987; Zeigler and Alvarez, 1987).
In Latin America and the United States, rice yield reductions due to BPB have reached 75% in severely infested fields as a result of a reduction in grain weight, sterility of florets and inhibition of seed germination (Correa et al., 2007; Nandakumar et al., 2007, 2009; Diago et al., 2009). In addition to the problems due to BPB, losses due to SBR caused by the phytopathogen Pseudomonas fuscovaginae have increased dramatically in rice-producing countries like Indonesia, Malaysia, and Colombia, with losses up to 76% (Razak et al., 2009). The occurrence of both pathogens has been favored by changes in climatic conditions and it is believed that these diseases may occur more frequently in tropical and semi-tropical countries.
The main current approaches to control B. glumae include rice breeding to obtain tolerant rice varieties (Mizobuchi et al., 2013) and isolation and identification of virulent strains, which have been analyzed at genomic level to identify putative virulence genes differing among them (Francis et al., 2013; Fory et al., 2014). Also, improvement of diagnostic tools for achieving detection at early stages of rice development, and intense diffusion and training of farmers in symptoms-detection in the fields have been used as an approach to diminish the incidence of B. glumae (Sayler et al., 2006; Kawanishi et al., 2011). Furthermore, recently transcriptome studies for the pathosystem rice-B. glumae have contributed to an understanding of the gene expression of this pathogen along rice development (Kim et al., 2014; Magbanua et al., 2014). Nonetheless, these approaches are not always efficient, considering that virulent strains are polymorphic and have developed resistance to chemical treatments (Hikichi et al., 2001; Karki et al., 2012).
In spite of the dramatic losses due to P. fuscovaginae and B. glumae, no biological or chemical approach has proven to be successful for controlling them in Latin America, and consequently, sustainable alternatives for reducing these two bacterial diseases of rice in the region are urgently needed. A possible alternative is a biocontrol approach, which involves the use of disease-suppressive microorganisms to control pathogens and improve plant health (Handelsman and Stabb, 1996). Major aspects of biocontrol are bioprospecting for new active isolates as well as understanding the mechanisms of pathogen antagonism for their future improvement and broader use.
Many Streptomyces strains are considered biocontrol agents, since they produce a wide range of antimicrobials, can persist in harsh environments, and efficiently colonize the rhizosphere of different plant species including rice (Qin et al., 2011; Kinkel et al., 2012). Furthermore, Streptomyces are able to elicit induced resistance, as it has been described before (Conn et al., 2008; Kurth et al., 2014). Because of these features, it is not surprising that diverse Streptomyces strains had been studied to control fungal and bacterial diseases of rice like Bacterial Leaf Blight caused by Xanthomonas oryzae, however very few Streptomyces are currently being developed as biocontrol products.
Research and development of biocontrol products commonly focuses on four major points: (1) studying the biocontrol traits for microbial agents, (2) assessment of the plant–microorganism interaction, (3) monitoring the beneficial and ecological effects of inoculation of each agent in the rhizosphere, and finally (4) verifying proper release of the microbial agents upon inoculation of crops with formulated products (Herrmann and Lesueur, 2013; Miransari, 2013). In this study, we describe the isolation and characterization of three Streptomyces isolates and assess their potential as biocontrol agents in order to explore their interaction and possible beneficial effects upon inoculation of rice plants. Colonization and plant growth promotion experiments were performed in sterile and non-sterile conditions as an approach to evaluate the ecological effects of their use in rice fields, and the colonization patterns were visualized microscopically for one isolate.
Materials and Methods
Isolation and Identification of Streptomyces-Like Isolates
Forty-five samples of rhizospheric soils were collected from rice-cultivated fields in Tolima (Colombia). In order to conduct this research, the ANLA (Autoridad Nacional de Licencias Ambientales) and the Ministry of Environment (Ministerio de Ambiente y Desarrollo Sostenible) were requested for permission to collect samples and study the recovered bacteria.
Briefly, plants roots were removed from the soil and subsequently handshaked for 10 min to remove bulk soil. The remaining adhering soil was considered as rhizospheric soil, and was collected by handshaking roots for 10 min in a sterile plastic bag. Rhizospheric soil samples (4–5 g for each) were then mixed with 1 g of CaCO3. Samples were further dried at 45°C for 1 h, as described previously (Gurung et al., 2009). Actinobacteria were subsequently isolated by spread plate technique following the serial dilution of soil samples on starch casein agar (Starch 10 g/L, Casein 1 g/L, K2HPO4 0.5 g/L, Agar 13 g/L) and incubated at 30°C for a week. In order to select Streptomyces-like bacteria, microscopic and macroscopic characteristics of the obtained colonies were assessed resulting in a total of 60 isolates, which were further purified and sub-cultured. Single colonies were characterized by their colony morphology in International Streptomyces Project media (ISP2, ISP3, ISP4), Nutrient agar and Mueller Hinton Agar (Supplementary Figure S1). Catalase, oxidase and their carbon source utilization were tested, as suggested for Streptomyces-like bacteria (Shirling and Gottlieb, 1968; Goodfellow, 2012).
Antimicrobial Activity Assays
Actinobacteria strains were grown for 5 days in M3.7 liquid media (glucose 5 g/L, Yeast Extract 5 g/L, (NH4)2SO4 5 g/L, Corn gluten 5 g/L, CaCO3 2 g/L, NaCl 2 g/L, FeSO4 1 mg/L, starch 10 g/L pH 7.2). Five-day cultures were used for antimicrobial tests. Antibacterial activities against 21 strains from 15 species (Supplementary Table S1) were determined by using the Kirby-Bauer agar well-diffusion method, modified from the CLSI 2011 guidelines (Clinical Laboratory Standards Institute, 2011). Briefly, each strain to be tested was grown overnight in LB media, and its OD600 was determined. Bacterial absorbance was then adjusted to 0.25, and a cotton swab was fully immersed in each bacterial dilution. The inoculated swab was used to spread the entire surface of a Mueller Hinton Agar plate, containing 25 mL of agar five times. Seven mm (diameter) wells were perforated in the agar, and 50 μL of each actinobacterial culture were poured into the well. Plates were subsequently incubated at 30 or 37°C (according to the best temperature for each bacteria to be tested). Inhibition zones were measured after 24 h of incubation. Antibacterial assays were also performed against a collection of 48 B. glumae isolates recovered from rice plants exhibiting symptoms of BPB (kindly provided by FEDEARROZ-Colombia).
Antifungal activities against 9 fungal plant pathogens (Supplementary Table S1) were measured as described previously (Kanini et al., 2013). For this assay, fungal strains were grown in Potato Dextrose Agar (PDA) plates for 3 days at 25°C, and two 6 mm disks of mycelium from each phytopathogenic fungi were then placed in opposites edges of a new PDA plate. Following fungal inoculation, two 6 mm wells were open in opposite sides of the PDA plate, containing 50 μL of a 5-day culture for each Actinobacteria strain tested. The plates were incubated at 28°C for 5 days and antagonistic activity was estimated by measuring the growth inhibition zone.
Taxonomical Identification and Multilocus Sequence Analysis (MLST)
Three strains were selected based on their wide range of antimicrobial activity. Genomic DNA was isolated by using the Salting-out procedure described previously (Pospiech and Neumann, 1995), with few modifications. Briefly, single colonies of each isolate were grown in 30 mL Tryptic Soy Broth, with shaking at 30°C for 48 h. Cells were harvested by centrifugation and washed with 10% sucrose, and suspended in 5 ml of STE Buffer (75 mM NaCl, 25 mM EDTA pH 8.0, 20 mM Tris-HCl pH 7.5), containing heat-treated pancreatic RNase A at 10 mg/mL. Hundred microliters of lysozyme (50 mg/mL) were added to the mixture and incubated for 1 h at 37°C, with gentle tapping at intervals. This was followed by the addition of 140 μL of proteinase K (20 mg/ml) and 600 μL of 10% SDS, with further incubation at 55°C for 2 h. Two mL of 5M NaCl were added and mixed by inversion until the temperature of the suspension reached the 37°C. The samples were cooled to room temperature and extracted twice with 5 ml of chloroform. Aqueous fractions were separated by centrifuging at 10000 g for 10 min at 4°C, and genomic DNA was precipitated by adding one volume of isopropanol, mixed by inversion and centrifuged 15 min at 15000 rpm. The obtained DNA pellet was rinsed with 70% ethanol, air-dried and suspended in 100 μL of DNAse-free sterile water.
Taxonomical identification was first approached by PCR amplification, sequencing, and analysis of the entire 16S rRNA locus by using primers 16S_A and 16S_B described by Cui et al. (2001), and the cleaned PCR products were directly sequenced using universal primers 27F, 500F, 818R and 1492R (Supplementary Table S1). Closely related sequences were obtained from RDP (Ribosomal Database Project) (Cole et al., 2014) and EZTaxon (Kim et al., 2012). Obtained sequences were imported into MEGA6 software and aligned with ClustalW for phylogenetic analysis (Thompson et al., 1994).
Multilocus Sequence Typing was used to elucidate a further taxonomical affiliation (Guo et al., 2008; Rong et al., 2009). Briefly, five loci (gyrB, atpD, recA trpB, and rpoB) were PCR amplified and purified by using the EuroGOLD Gel extraction Kit (EuroCLONE, Italy). Each fragment was then sequenced by using primers described previously (Guo et al., 2008), and listed in Supplementary Table S1. The sequences for all loci for each strain were concatenated head to tail in frame and exported in FASTA format. Sequences were aligned using CLCbio Mainworkbench 6.9.1 (CLC-Bio Qiagen) and MEGA 6.0 (Tamura et al., 2011). A phylogenetic tree was constructed from the concatenated sequences of all six loci, and representative Streptomyces strains available in the Streptomyces MLST database (Jolley and Maiden, 2010)1 Maximum likelihood algorithm trees were generated with MEGA based on the Tamura-Nei model (Tamura and Nei, 1993), and a tree with the highest log likelihood was generated calculating the percentage of trees in which the associated taxa clustered together. Initial trees for the heuristic search were obtained automatically by applying Neighbor-Join and BioNJ algorithms (Gascuel, 1997) to a matrix of pairwise distances estimated using the Maximum Composite Likelihood (MCL) approach, and then selecting the topology with superior log-likelihood value.
In vitro Assessment of Plant Growth Promotion (PGP) Traits
Biological nitrogen fixation, phosphate solubilization, indoleacetic acid production (IAA), ACC deaminase and extracellular enzyme production were evaluated. For this purpose, each isolate was grown in 100 mL M3.7 medium for 5 days, and each assay was performed with three independent cultures for each strain.
Biological Nitrogen Fixation
To test biological nitrogen fixation, Acetylene Reduction Assay (ARA) was performed as described elsewhere (Hardy et al., 1968). To this purpose, 100 μL of washed cells were inoculated into 17 mL of semisolid NFb medium in 25 mL serum bottles with a cotton cap, and cultures were maintained at 30°C, without agitation (Dobereiner, 1995). After 5 days, 2 mL of acetylene were injected into the flask and a rubber stopper was used to replace the cotton cap. The acetylene and ethylene concentrations of each sample were determined by gas chromatography, using a chromatograph Varian 3400-G-crom, equipped with a flame ionization detector and a capillary column (Hayesep Porapak N 80/100 column; 6′ X 1/8″). Ethylene and acetylene estimation was done by integrating the area under the curve for each compound, at retention times of 1.417 and 2.40 min, respectively.
Phosphate Solubilization
Phosphate solubilizing abilities were qualitatively determined by inoculating single colonies of each strain in National Botanical Research Institute’s Phosphate growth medium (NBRIP) (Nautiyal, 1999) and SRS media (Sundara-Rao and Sinha, 1963). Plates were incubated at 30°C for 5 days, and colonies with a clear orange halo in NBRIP media and/or a purple color in SRS media were marked positive for phosphate solubilization. A quantitative determination was performed by growing each strain in 100 ml of NBRIP liquid media supplemented with 5 g/L CaCO3, for 5 days. Subsequently, the solubilized phosphate present in the supernatant was measured with the Spectroquant Phosphor Kit (Merck).
Siderophore Production
Siderophore production was determined in Chrome Azurol Blue agar (Schwyn and Neilands, 1987), modified to use KOH to adjust the pH, as suggested previously (Mahmoud and Abdallah, 2001).
ACC Deaminase Production
1-aminocyclopropane-1-carboxylate (ACC) deaminase production was determined by checking the ability of each strain to use ACC as sole nitrogen source. For this purpose, single colonies were inoculated in Dorwin-Foster media (DF) supplemented with 3 mM ACC as sole nitrogen source, or with 2 g/L (NH4)2SO4 as growth control (Belimov et al., 2001; El-tarabily, 2008). In each case, 2 μL of a 106 CFU/mL culture, were spotted on the surface of a DF plate with or without N source, and growth was evaluated after 5 days at 30°C, and Burkholderia unamae MT1 641T was included as a positive control. An absence of phenotypic changes in growth or sporulation of the isolates in the medium supplemented with ACC in comparison with an (NH4)2SO4 supplemented medium were considered as indicators of the capacity of the microorganisms to use ACC as a source of nitrogen mediated by the production of ACC-deaminase.
Indoleacetic Acid
For the quantitative determination of Indoleacetic acid (IAA) and IAA-like molecules, the colorimetric Salkowski’s assay was performed (Tang and Bonner, 1948). For this purpose, each Streptomyces strain was grown in 20 mL Tryptic Soy Broth (TBS) supplemented with 60 mM tryptophan. After 4 days, 1 ml of Salkowski reagent (Glickmann and Dessaux, 1995) was pipetted into test tubes containing 1 ml of culture spent supernatant. The tubes containing the mixture were gently vortexed and left for 30 min for development of color at room temperature (26 ± 1°C). The intensity of the color was spectrophotometrically determined at 535 nm.
Extracellular Enzyme Production
Extracellular enzyme production was evaluated in vitro. Cellulolytic activity was evaluated by spotting 2 μL of 5-day old cultures on the surface of CMC-media plates, in which CMC was the sole carbon source. Spots were incubated at 30°C for 4 days, and then strains were assayed for their ability to degrade CMC by flooding each plate with a 0.2% Congo red solution for 15 min, followed by washing with 1 M NaCl. Activity halos dimensions were then measured from the border of the colony to the outer edge of the halo. Similarly, ligninolytic activities were determined in guaiacol containing media and positive activity was scored when a red halo was formed around colonies, due to the oxidation of the substrate to tetraguaiacol, as described previously (Nagadesi and Arya, 2012).
Plant-Growth Promotion Experiments
Gnotobiotic Experiments
Gnotobiotic inoculation experiments were performed in rice cultivars FEDEARROZ 733 (F733) and FEDEARROZ 60 (F60). For this purpose, rice seeds were surface sterilized and pre-germinated in 1% agar, for 5 days in complete darkness (Mattos et al., 2008). Each seed was then transferred into glass flasks (15 cm × 4 cm) with 40 mL of semisolid Hoagland media previously inoculated with 103 CFU/mL of each strain. To this end, each strain was grown in M3.7 media, and cells were suspended in sterile saline solution and added to the semi-solid medium to reach a final concentration of 103CFU/mL, before solidification of the medium.
Plantlets were then transferred to a greenhouse (Relative humidity 43% ± 8.6, Average Temperature 24°C ± 5.2, natural light). Azotobacter chroococcum (APFN010) and Azospirillum brasilense (AZPP010) strains (105 CFU/mL) were used as positive controls, and sterile water was used as negative control. Each treatment included two biological replicates (two independent colonies for each bacterial strain), and 10 plants were inoculated with each inoculum. The entire experiment was performed twice, and biometric properties (root and shoot fresh weight, plant dry weight, and length) were measured 15 days post-inoculation.
Soil Inoculation Experiments
Inoculation experiments were also performed in sterile and non-sterile soil using cultivars F733 and F60 with pregerminated seeds, as described above. For these assays, 10 mL from a 108 CFU/mL inoculum were mixed with 250 g of sterile soil, to reach a concentration of 105 CFU/g. Subsequently, pre-germinated seeds were planted inside the pot and plants were kept in the greenhouse at 25°C for 31 days post-inoculation. A. chroococcum (APFN010) and A. brasilense (AZPP010) strains were used as positive controls, and sterile water was used as negative control. Each treatment included two biological replicates (two independent colonies for each bacterial strain), and 10 plants were inoculated with each inoculum. Biometric properties (root and shoot fresh weight, plant dry weight and length) were measured 31 days post-inoculation. Dry weight for each plant was determined after drying the plant material at 50°C, until obtaining a constant weight.
Plant Colonization Experiments
To assess the ability of the three Streptomyces for rice plant colonization, two independent assays were performed under gnotobiotic and soil conditions as described before, using in each case three biological replicates for each strain, with five plants per replicate. Bacterial strains were re-isolated from the rhizoplane by cutting and washing 2 cm segments of each plant root and placing the excised root segments on the surface of ISP3 plates. Roots of the soil experiment were placed on ISP3 medium supplemented with nalidixic acid (20 μg/ml) and nitrofurantoin (10 μg/ml) to inhibit the growth of other soil microorganisms. The identity of colonies recovered from the roots was verified from their phenotype and their antimicrobial profile.
To verify endophytic colonization, inoculated plants were surface sterilized with 1% sodium hypochlorite and 70% ethanol for 4 min each, followed by several washes with sterile water. Each plant was then excised in the root, plant shoot and leaves, and each part was macerated with a sterile pestle. Each suspension was then serially diluted and plated in ISP3 plates. Surface sterilization was regarded as optimum when no growth was observed: (1) after placing the processed plants on ISP3 plates, and (2) after plating 100 μL from the last wash in ISP3 (see more details from the experimental set-up in Supplementary Figure S2).
Finally, to test the persistence and viability of isolates in the soil, rhizospheric soil portions were sampled from each treatment. 1 g of each sample was diluted in Tween 80 (0.1% v/v) and plated in ISP3 medium supplemented with nalidixic acid and nitrofurantoin. Results were evaluated after 4 days incubation at 30°C and reported as CFU/g soil rhizosphere.
Fluorescence Microscopy
An egfp tagged mutant from Streptomyces sp. A20 was generated by intergeneric conjugation with Escherichia coli S17-1 λ pir harboring pIJ8641 plasmid, (kindly provided by M. Bibb from the John Innes Centre-Norwich, United Kingdom) (Sun et al., 1999; Kieser et al., 2000; Willey et al., 2007), and transconjugants were selected into Apramycin ISP3 plates (100 μg/ml). Fluorescent transconjugants were further selected for gnotobiotic inoculation experiments as described before, using either semisolid Hoagland or sterile vermiculite supplemented with Apramycin (100 μg/ml). For these experiments, 15 plants were inoculated with A20pIJ8641, non-tagged Streptomyces A20, and a non-inoculated set of plants was included as a control. To assess the colonization process three roots were evaluated for each treatment at days 1, 2, 3, 10, and 15 days post inoculation. In each evaluation day, the roots were excised from the matrix, washed three times with sterile water and cut in 3 mm × 3 mm fragments. Observations were performed in an Olympus BX41 Microscope with a CCD Infinity 3 Camera (dichroic mirror DM500; excitation filter BP460-490 and barrier filter BA520IF).
Purification and Characterization of Antimicrobial Compounds
Streptomyces A20 was grown in 80 L M3.7 media in a 100 L stirrer tank bioreactor with constant shaking (40 rpm) at 25°C. After 4 days, 1 L of culture was centrifuged and spent supernatants were filter-sterilized using a 0.22 μm membrane. Bioassay-guided fractionation of A20 fermentation sterile supernatants was then performed by a sequential partition with ethyl acetate (2 × 500 mL), followed by butanol (4 × 500 mL) extraction (Supplementary Figure S3), to yield organic (EtOAc), butanolic and residual aqueous fraction (ABP fractions). Each fraction was evaporated to dryness and suspended into sterile water to be subjected to antibacterial assays. Active fractions were first purified by ultra-filtration by using 1, 3, and 10 kDa Amicon filters (Millipore).
Several chromatographic techniques were used to purify antimicrobial compounds produced by strain Streptomyces A20. Briefly, residual aqueous fractions from butanol extraction were dried and suspended into buffer A (sodium acetate 0.05 M pH 5.5) to be loaded into a strong anion exchanger column, Q Sepharose Fast Flow, previously equilibrated and washed with buffer A, using a BioRad Biologic Duoflow chromatography system. Compounds bound to the column were eluted with a linear gradient of increasing NaCl concentration (0–1M). Antimicrobial compounds present in the flowthrough, were purified by loading it into an S SepharoseTM Fast Flow column (cation exchange chromatography), and antimicrobial compounds were eluted again with a gradient of increasing NaCl concentration. Antimicrobial fractions were then suspended into 20 mM ammonium acetate, containing 0.1% heptafluorobutyric acid (HFBA), and injected onto a semipreparative C18 reversed phase column (1x 25 cm). Three fractions were recovered due to their antibacterial activity and were further analyzed by LC-MS.
Samples for LC-MS were dissolved in 90% methanol and 10 μl injected onto a Gemini C18 column (2 × 150 mm, 5 μm; Phenomenex, Torrance, CA, United States) and separated using a gradient from water to 90% acetronitrile mobile phases containing 0.1% formic acid at 0.2 ml min-1. The column effluent was delivered to an ESI ion trap mass spectrometer (Amazon SL, Bruker Daltonics, Bremen, Germany) and mass spectra collected in positive ion mode in the range 200–2000 m/z.
Statistical Analysis
Most experiments were performed at least three times and means are given. Statistical analyses included unpaired t-tests and ANOVA with Dunnett’s post-test and were performed with PRISM 4.0 software (GraphPad Software, San Diego CA, United States). A P-value of < 0.05 was considered significant.
DNA Sequencing and Nucleotide Sequence Accession Numbers
16S rRNA nucleotide sequences for strains Streptomyces A20, 5.1, 7.1 and Streptomyces racemochromogenes DSM 40194 were deposited in GenBank/EMBL/DDBJ, under the accession numbers KP082880, KP082881, KP082882, and KP082883.
Results
Isolation and Identification of Streptomyces Strains With Anti-bacterial Activity From Rice Cultivated Soils
Sixty actinobacterial-like isolates were obtained from Colombian soils upon enrichment with CaCO3 however, only three isolates denoted as A20, 5.1 and 7.1 showed antimicrobial activity against either two plant pathogenic bacterial strains of B. glumae and P. fuscovaginae, or fungal phytopathogens. Strains A.20 and 5.1 showed both antibacterial and antifungal activity, whereas strain 7.1 showed only antifungal activity (Table 1).
Biochemical tests indicated that these three strains were catalase positive; oxidase negative, and their carbon source analysis indicated that all strains were able to metabolize glucose, but were unable to use rhamnose, arabinose, xylose or mannitol as carbon source (Table 2). Taxonomical analyses derived from 16S rRNA sequencing confirmed that all three strains belong to the genus Streptomyces and similarity calculations of the 16S rRNA locus, based on Maximum Likelihood and Neighbor Joining analyses indicated that the closest relatives for strain A20 were S. racemochromogenes/S. polychromogenes and S. flavotricini with similarity values of 99.93 and 99.8 % respectively, placing this strain within the previously described clade 38 (Labeda et al., 2012). A similar analysis located strain 7.1 within clades 2 and 71 with similarity values of 99.89 and 99.79% to S. chrestomyceticus, S. corchorusii/S. canarius. Strain 5.1 was related to the S. roseoverticillatus clade 65, having 98.9% similarity to Streptomyces kashimirensis and S. salmonis (Figure 1A).
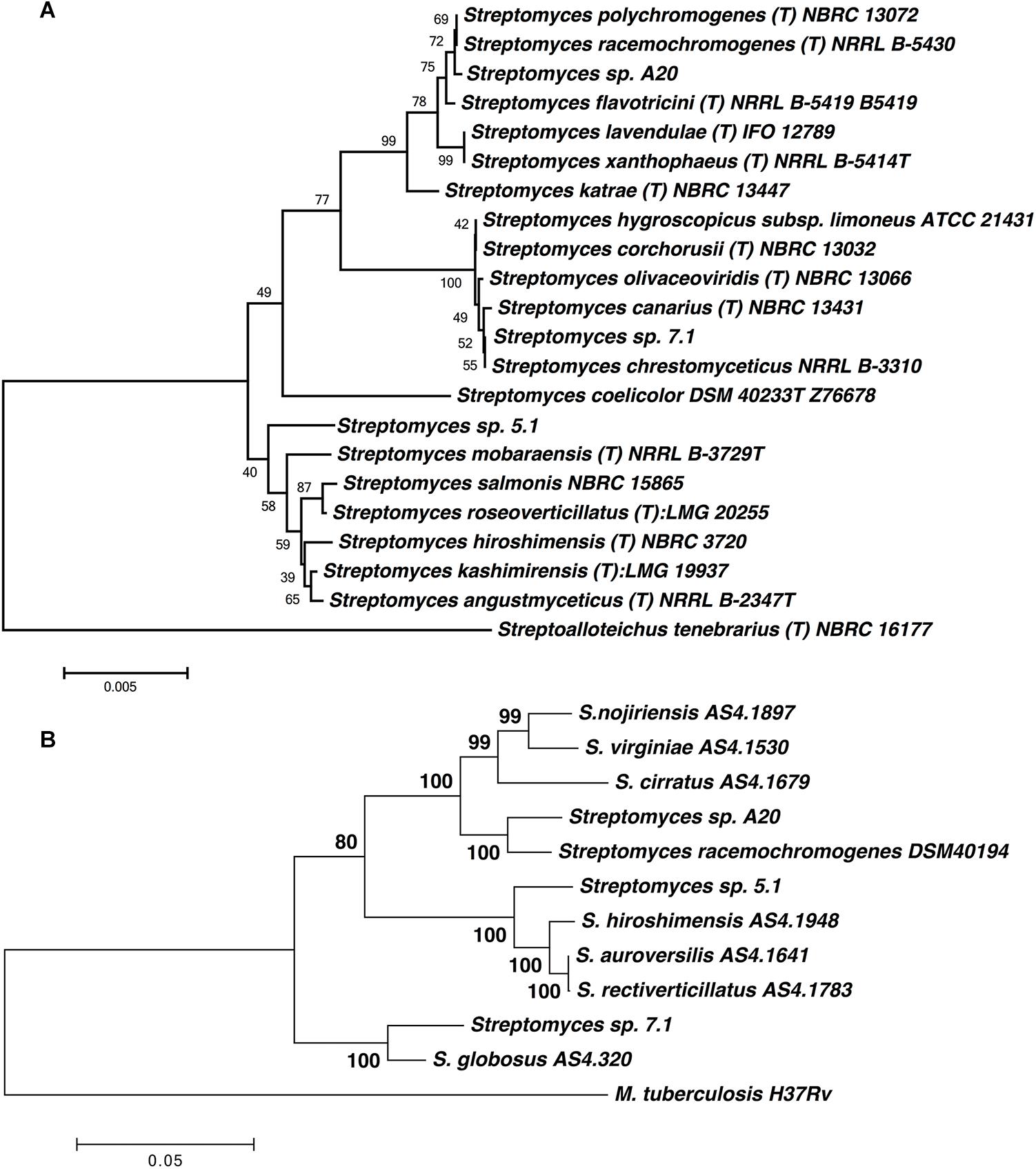
Figure 1. (A) Phylogenetic tree based on 16S rRNA sequences of the Streptomyces strains. The evolutionary history was inferred using the Neighbor-Joining method (Saitou and Nei, 1987). The tree is drawn to scale, with branch lengths in the same units as those of the evolutionary distances used to infer the phylogenetic tree. Numbers at branch nodes are bootstrap values. Sequence from Streptoalloteichus tenebrarius NBRC 16177 was included as outgroup. (B) Phylogenetic tree based on MLST analyses from five loci (gyrB, atpD, recA trpB, and rpoB). The tree was drawn to scale using Maximum Likelihood method and Tamura-Nei model, with branch lengths measured in the number of substitutions per site. The analysis involved 12 nucleotide sequences. All positions containing gaps and missing data were eliminated. There were a total of 2896 positions in the final dataset.
In order to improve the taxonomical identification, MLST was performed for all three strains, and type strain S. racemochromogenes DSM 40194. MLST analyses for 12 strains, including the closest neighbors for each isolate revealed that all six loci have unique sequences compared to all available allele types in the MLST Streptomyces database. MLST profiles for strains 5.1 and 7.1 defined these two isolates as independent entities, and MLST profiles for close neighbors these two should be obtained to improve their taxonomical identification.
All loci obtained for strain A20 were highly similar to those obtained for strain S. racemochromogenes DSM 40194, and concatenated loci for both strains shared 97.2% identity at sequence level, however evolutionary distance calculated with Kimura 2 parameters, was above the species-definitive MLSA distance of 0.007 (0.028) (Figure 1B) (Rong and Huang, 2012; Labeda et al., 2014). Further studies may be required to generate MLST profiles for other type strains from this cluster in order to verify these results and define if strain A20 corresponds to a known species or may be described as a new species.
Streptomyces A20, 5.1, and 7.1 Exhibit a Wide Range of Antimicrobial Activity
In order to study the strength and range of antimicrobial activity, all three selected strains were tested by Kirby-Bauer well-diffusion assays, which indicated that Streptomyces strains A20 and 5.1 could inhibit the growth of a wide range of bacterial and fungal phytopathogens to varying degrees, whereas strain 7.1 was able to inhibit mainly fungal phytopathogens (Table 1). Strain A20 showed the wider range of activity, with antimicrobial effects against 20 out of 21 bacterial species tested, and against all fungal pathogens evaluated (Figure 2). All three Streptomyces strains were also tested against a collection of 48 pathogenic B. glumae isolates isolated from Colombian rice-growing areas. In these assays, Streptomyces strains A20 and 5.1 exhibited antimicrobial activities against 91.48 and 78.72% of pathogens tested, respectively. Remarkably most of the pathogenic B. glumae isolates were inhibited by Streptomyces A20.
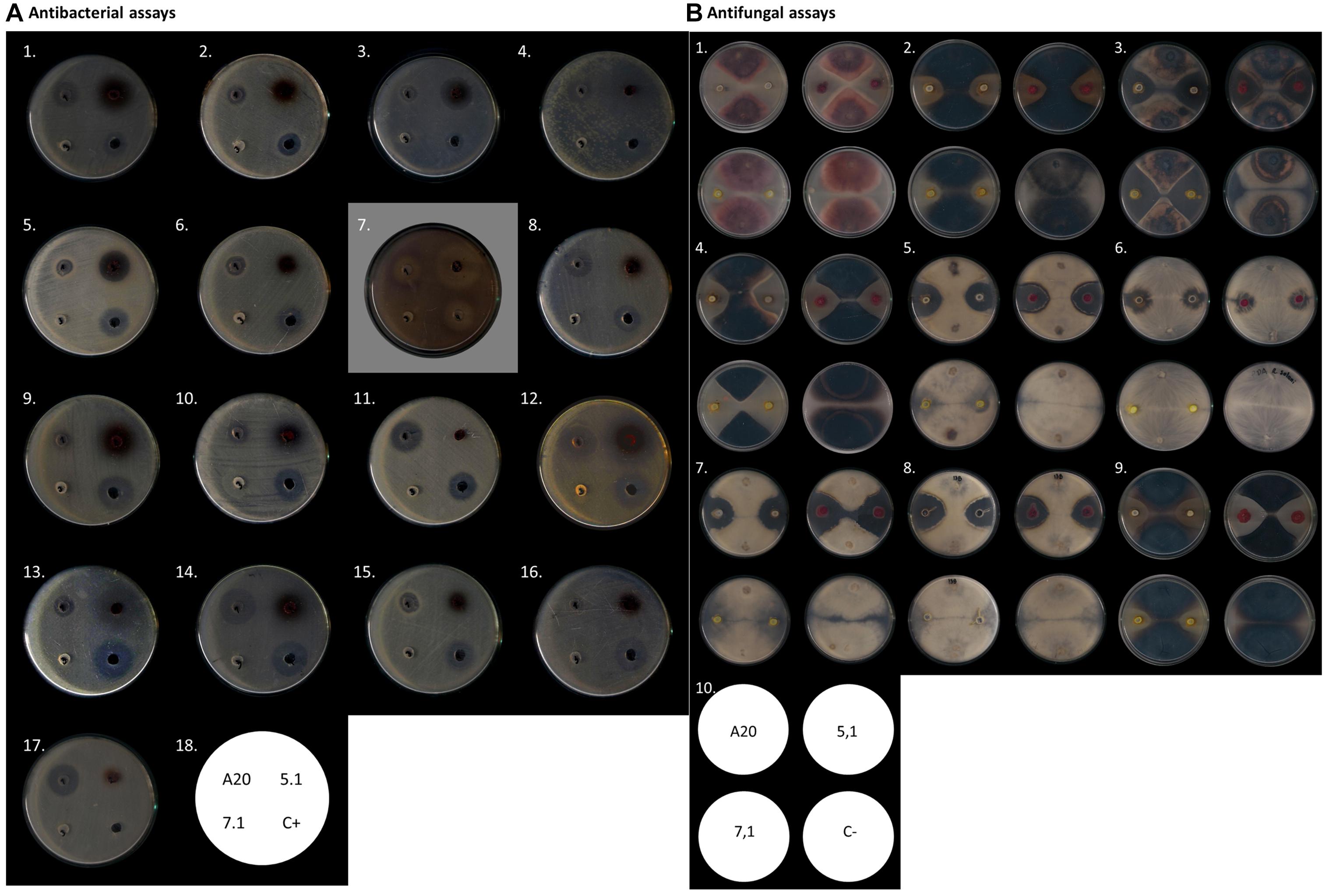
Figure 2. Antimicrobial activity of Streptomyces A20, 5.1, and 7.1. (A) Antibacterial assays by Kirby- Bauer well diffusion test. In the figure from left to right: (1) A. avenae CIAT 4008-2, (2) A. baumannii ATCC19606, (3) S. aureus ATCC 25923, (4) B. glumae CIAT 4026, (5) B. subtillis ATCC 21556, (6) Bacillus C636, (7) C. violaceum ATCC 31532, (8) E. coli ATCC12167, (9) B. gladioli CIAT 3962, (10) B. gladioli CIAT 3704-1, (11) E. coli ATCC23724, (12) B. glumae 320012, (13) P. fuscovaginae CIAT 3638-19, (14) E. coli DH5α, (15) K. pneumoniae ATCC 700603, (16) P. aeruginosa ATCC 27853, (17) P. putida F117. (18) Assay scheme. (B). Antifungal assay as described by Kanini et al. (2013). In the figure from left to right: (1) Fusarium oxysporum, (2) Gaeumannomyces sp., (3) Colletotrichum sp. 24C, (4) Colletotrichum sp. 26B, (5) Phomosis sp. DC1B, (6) Rhizoctonia solani, (7) Fusarium sp. DC9, (8) Fusarium sp. DC13B, (9) Ulocladium sp., (10) Assay scheme.
Streptomyces Strains Isolated From Colombian Soils Are Able to Promote Rice Growth in Several Rice Cultivars
In vitro tests suggested that all three strains were able to produce siderophores, as well as proteolytic enzymes. All three strains also showed an ability to produce IAA and ACC deaminase, but none of the Streptomyces isolates could fix nitrogen. Streptomyces strain 7.1 was able to solubilize inorganic phosphate and showed a remarkable cellulolytic ability (Table 3 and Figure 3).
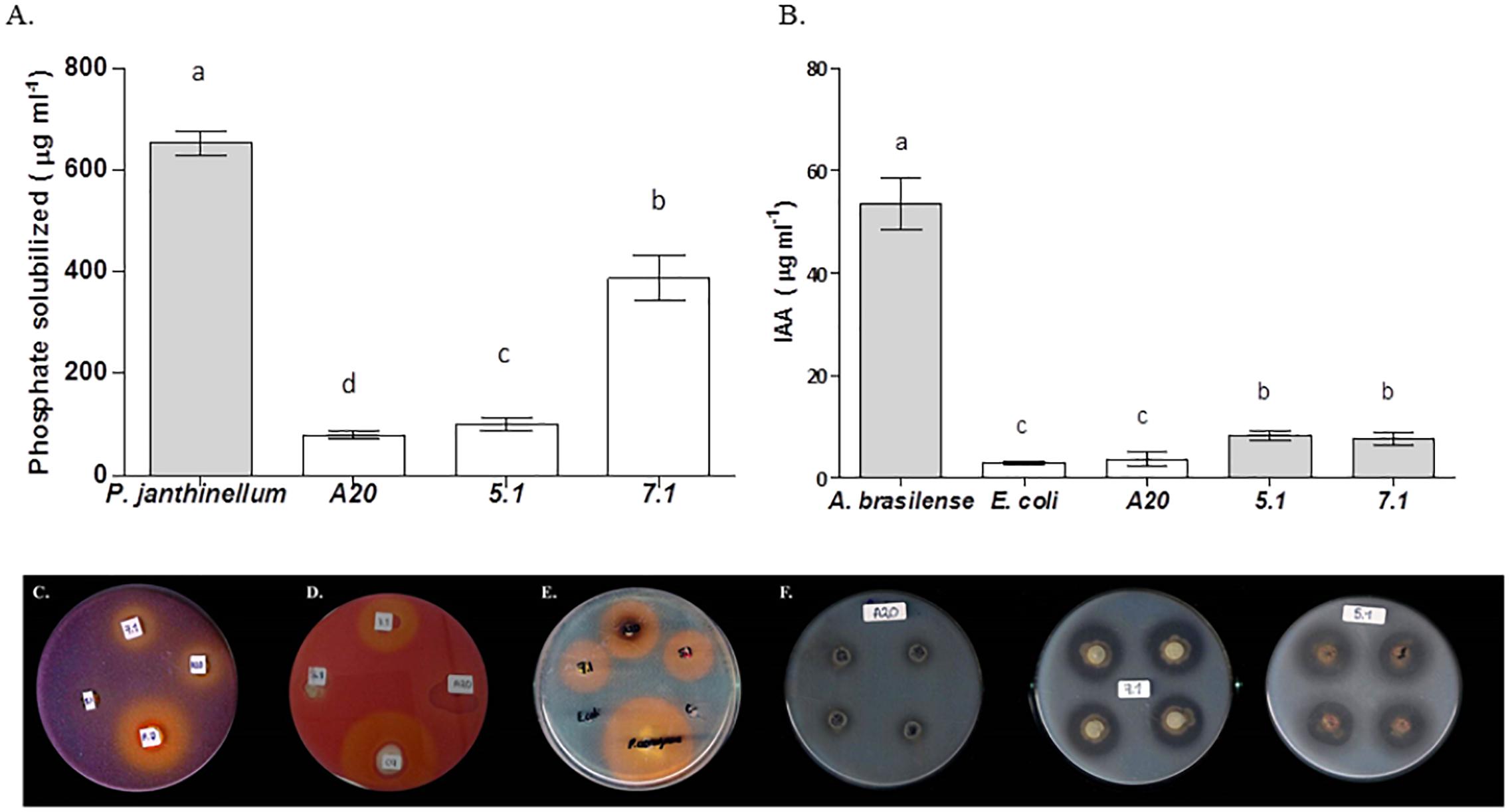
Figure 3. Evaluation of Streptomyces strains for key traits related to direct plant growth promotion. Bars correspond to (A) Phosphate solubilization assay or (B) Indoleacetic Acid production. The results are means of three values ± SD of three independent biological replicates. Means were compared with ANOVA analysis in combination with Tukey post-test. Means were considered statistically different when p < 0.05, and bars sharing a letter are not statistically different. (C) Qualitative phosphate solubilization assay, (D) Cellulase qualitative activity assay, (E) Siderophores qualitative production assay, (F) Protease qualitative production assay.
Gnotobiotic inoculation experiments suggested that strain A20 was able to promote rice growth in comparison to non-inoculated plants, since it generated increases in shoot length and fresh weight in roots and plants of two different cultivars being however statistically significant only for cultivar F60 (Figure 4A). Streptomyces 7.1 was able to increase root and shoot fresh weight in the cultivar F733 and did not have any effect on rice cultivar F60. Streptomyces 5.1 did not show any increase in any of the evaluated parameters, in any of the cultivars tested. In soil experiments, Streptomyces 5.1 was able to improve the fresh weight of roots and shoots in cultivar 733, and strains A20 and 7.1 displayed the ability to significantly increase all the biometric parameters evaluated in cultivar F733 in comparison to the non-inoculated control (Figure 4B and Supplementary Figure S4).
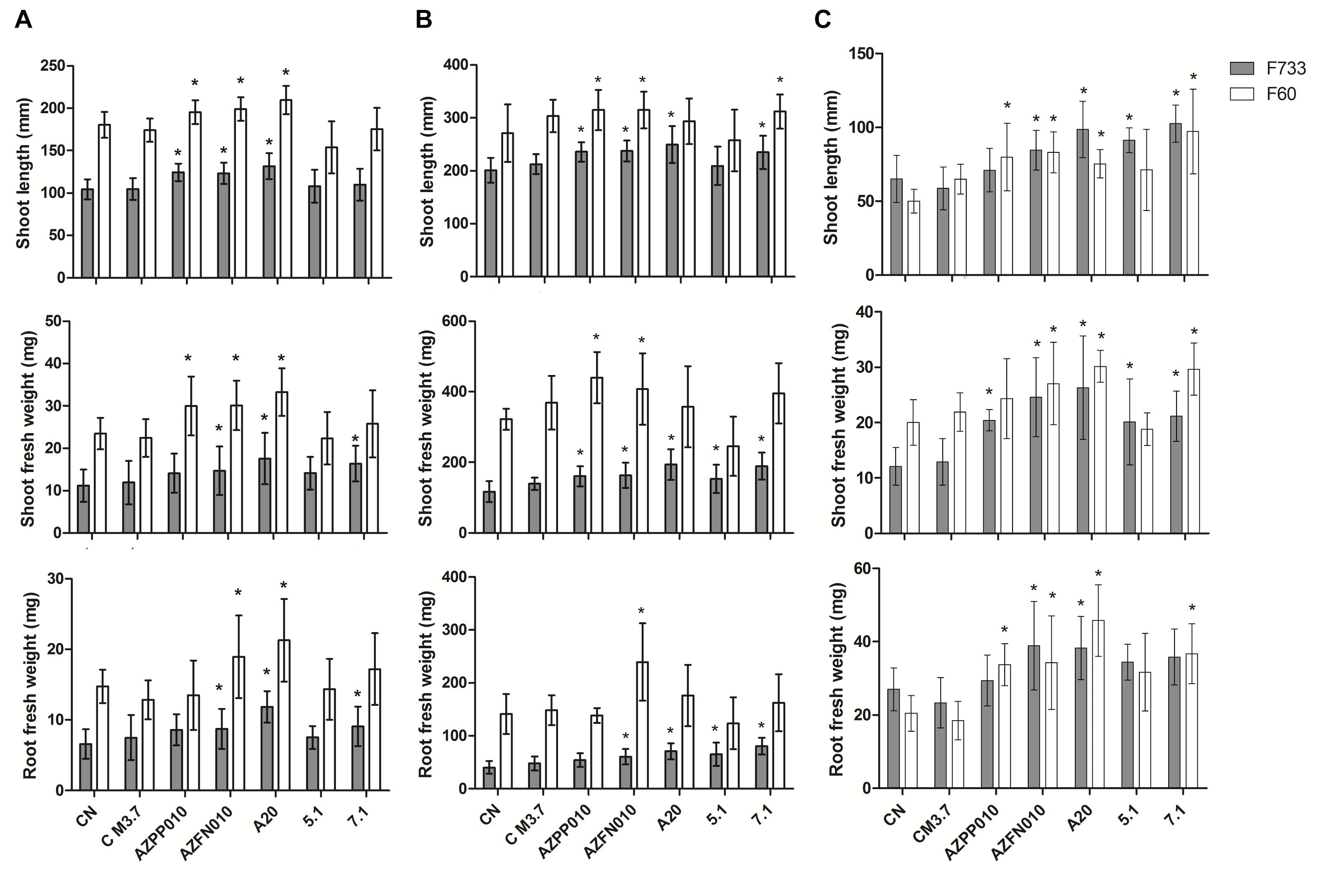
Figure 4. Plant-growth promotion experiments. Bars correspond to (A) Gnotobiotic assay, (B) Sterile Soil assay, and (C) Non-sterile soil assay. The results are means of twenty values ± SD, from two independent biological replicates. Means were compared with ANOVA analysis in combination with Tukey post-test, means were considered statistically different when p < 0.05. Bars with (∗) above are statistically different from the negative control. CN, Negative control. C M3.7, Medium control. AZPP010, A. brasilense. AZFN010, A. chroococcum.
Plant growth promotion experiments were performed both with sterile and non-sterile conditions in order to compare the performance of the three strains in such environments and foresee their behavior under natural conditions. In non-sterile soil, plants inoculated with Streptomyces A20 and Streptomyces 7.1 showed increases in their biometric parameters, with the same trend they showed in the gnotobiotic experiments. In contrast, strain Streptomyces 5.1 was able to increase plant length and fresh weight in plantlets from cultivar 733 (Figure 4C). Importantly, plantlets from non-sterile soils were smaller due to the presence of fungal strains, observed along the process (data not shown), which were most likely controlled by antifungal metabolites produced by isolates A20 and 5.1.
Streptomyces A20, 5.1, and 7.1 Are Able to Colonize Two Different Rice Cultivars Under Gnotobiotic and Soil Conditions
Colonization experiments indicated that all Streptomyces strains tested were able to colonize the roots, since they were recovered from the rhizoplane in gnotobiotic experiments with roots from cultivars F733 and F60, even after 31 days post-inoculation (dpi) (Table 4 and Supplementary Material S2). Streptomyces A20 and Streptomyces 7.1 were also able to colonize the root endosphere, as they were recovered from a percentage of surface sterilized plants (80 and 20 %, respectively) grown in a gnotobiotic system.
An egfp tagged mutant was generated for strain A20 and colonization experiments were performed. Our results indicated that egfp expressing strain strongly associated with root hairs (Figure 5). Also, our experiments suggested that Streptomyces A20 first established itself in the main root and on the surface of the rice seeds (1 dpi, Figure 5), followed by the formation of micro-colonies between 2 and 3 dpi, and the colonization of secondary roots (Figure 5). After 10 days of growth, Streptomyces A20 had colonized the points of emergence for secondary roots and the surface of the main and secondary roots. After 15 days an overall colonization from the surface of the root is observed both in vermiculite and semisolid Hoagland media.
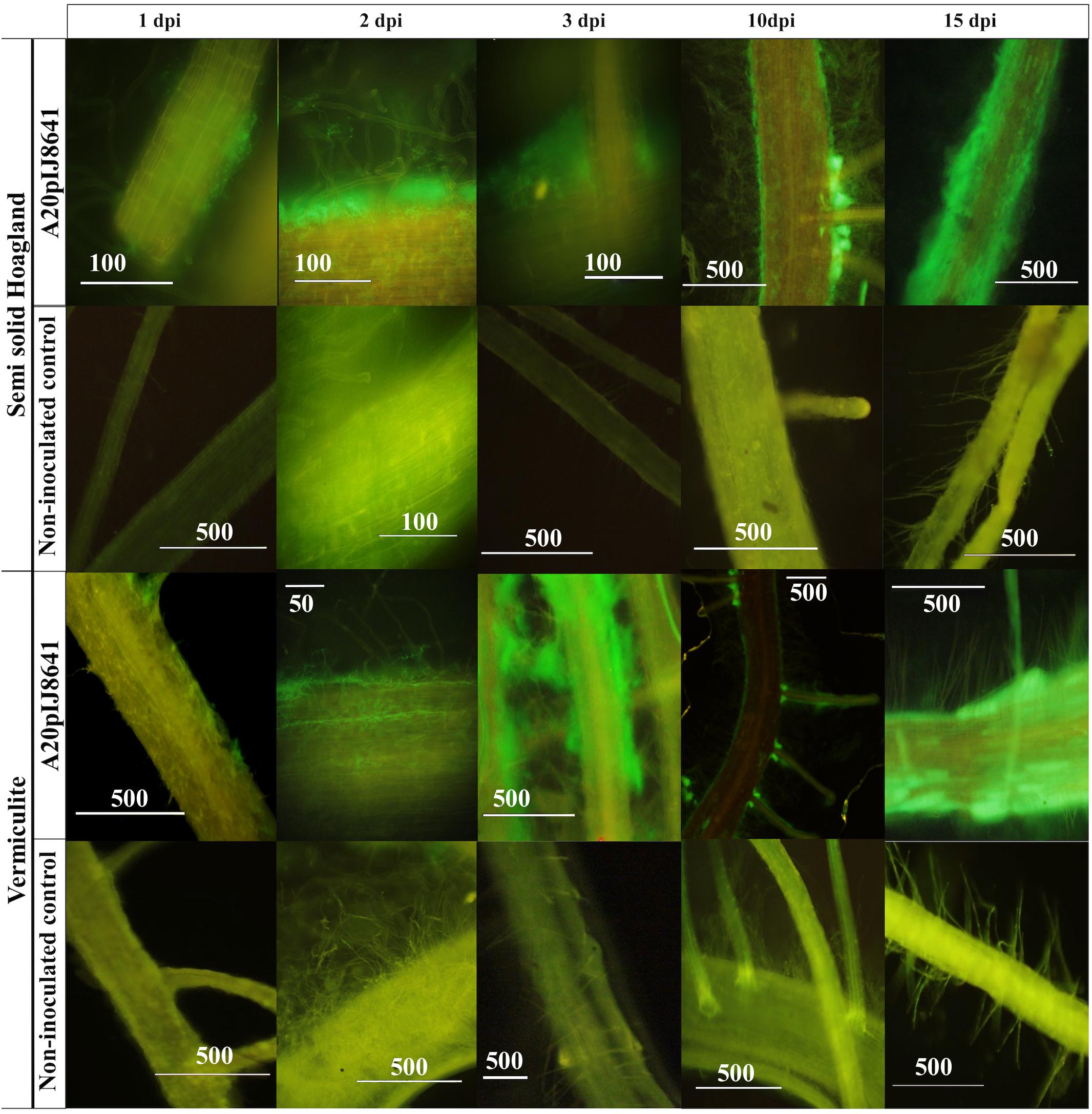
Figure 5. Microscopic visualization of rice root plants (cultivar F733) colonized with Streptomyces A20 EGFP mutant. This experiment was performed under gnotobiotic conditions with a non-inoculated control and an EGFP tagged strain (A20 pIJ8641), both in Semisolid Hoagland media and Vermiculite. Numbers above indicate days post inoculation (dpi), and numbers on bars correspond to the scale in micrometers.
Streptomyces A20 Produces Several Hydrophilic Charged Compounds
Strain A20 was chosen for further characterization of antimicrobial metabolites. Bioassay-guided extraction of A20 spent supernatants with solvents of increasing-polarity suggested that the antimicrobial compounds produced by this strain are hydrophilic ionic compounds since they remain in the aqueous fraction after the butanol extraction (Supplementary Figure S3). Ultrafiltration assays followed by antimicrobial activity assays indicated that the antimicrobial compounds present in residual aqueous fractions have sizes below 3 kDa (Table 5). Cation-exchange chromatography generated three more fractions with antimicrobial activity (namely fractions 21, 32, and 33), which were further submitted to ion-pairing chromatography in order to obtain defined peaks with antimicrobial activity. This technique allowed the resolution of 2 identical peaks in fractions 32 and 33, and one single peak in fraction 21, which possessed antibacterial activity. All the fractions were subsequently subjected to mass spectrometry analysis. Results have indicated that strain A20 produces three compounds with same spectra patterns and m/z values (503.3, 631.4, and 759.4), to those reported for Streptothricin F, D and E, as suggested by comparison of their mass spectra (Figure 6) (Ji et al., 2007; Maruyama and Hamano, 2009). Characterization of the streptothricin D was confirmed as follows: the MS/MS spectra for the parent ion at m/z 759.4 [M+H]+, proposed as the pseudomolecular ion for Streptothricine D, presented daughter ions at m/z 589 [M-L3]+, 571[M-L3-H2O], 554[M-L3-H2O-NH3]+, 553 [M-L3-H2O-H2O]+, and 510 [M-L3-H2O-H2O-CONH2]+ where the L3 represents the chain of three lysine residues of Streptothricin D, as suggested previously (Ji et al., 2007, 2008, 2009). These analyses were also applied for Streptothricin E and F, indicating the presence of an ion m/z 171, a signature peak characteristic of streptolidine lactam for all Streptothricins (Figure 6) (Ji et al., 2009).
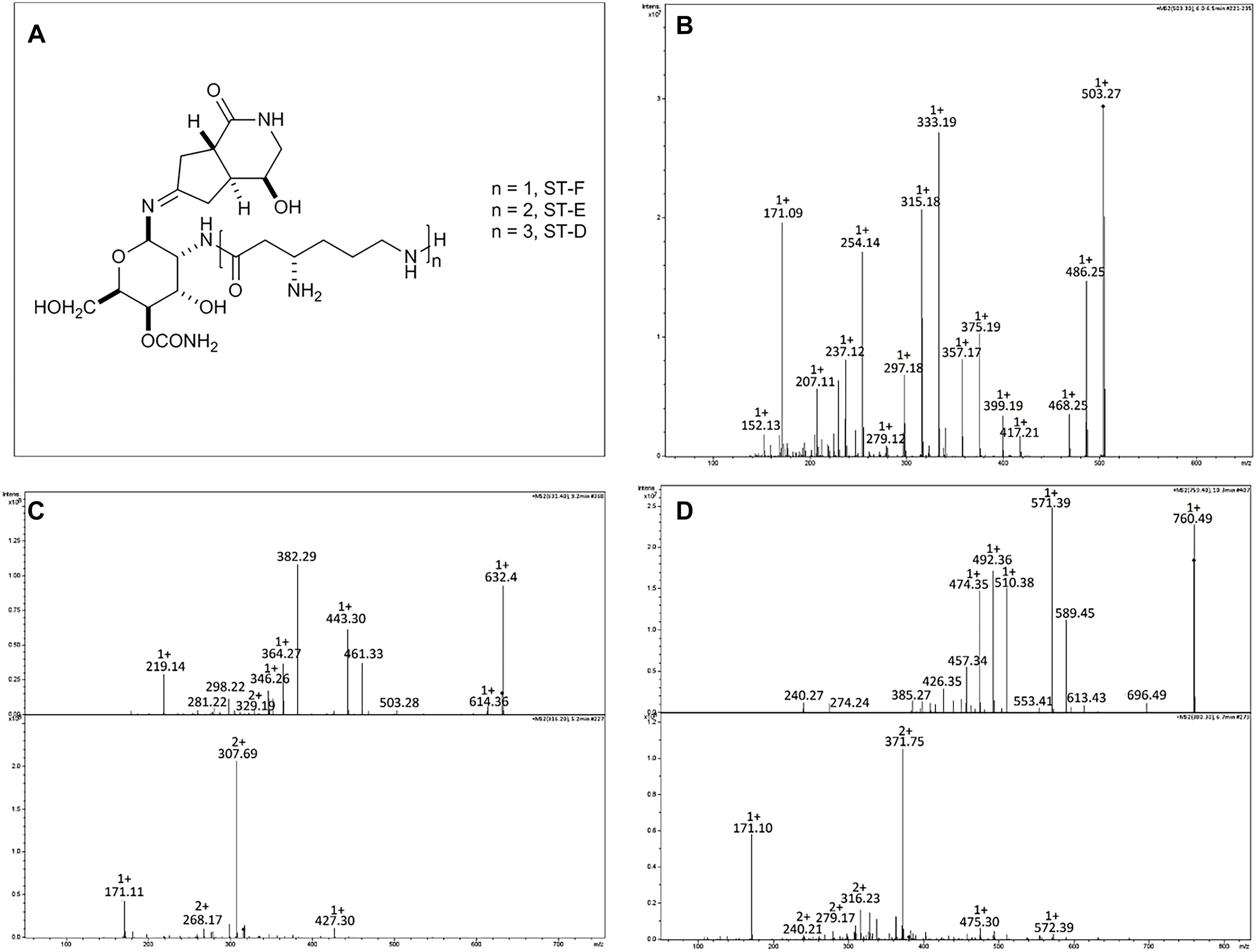
Figure 6. Structure and HPLC/ESI-MS analysis of the metabolites produced by strain Streptomyces A20. (A) Structures of the streptothricin-class antibiotics. MS/MS Spectra for (B) Streptothricin F (m/z 503.3), (C) Streptothricin D (m/z 631.4), and (D) Streptothricin E (m/z 759.5). For Streptothricins D and E lower panel corresponds to the MS/MS analysis of the doubly charged ion species for each compound. Analyzed compounds were obtained from fraction 16 collected in the RP-HPLC from the original fraction 32.
Discussion
High densities of antagonistic Streptomyces sp. have been associated with plant disease suppression in many soils, and thus biocontrol via bioinoculants is today regarded as a promising alternative for the management of bacterial diseases. These potential biocontrol bacteria can combine several mechanisms to inhibit pathogens in soil; which include nutrient competition, production of degradative enzymes, nitrous oxide production and quorum quenching (Kinkel et al., 2012). Streptomyces spp. have been widely recognized for their potential for controlling fungal pathogens, including Fusarium moniliforme, Fusarium oxysporum f. sp. ciceri, Rhizoctonia solani, R. bataticola, Magnaporthe grisea, and Magnaporthe oryzae (Emmert and Handelsman, 1999; Samac and Kinkel, 2001; Taechowisan et al., 2003; Tian et al., 2007; Zarandi et al., 2009; Gopalakrishnan et al., 2011; Kanini et al., 2013), as well as rice bacterial pathogen Xanthomonas oryzae pv. oryzae (Hastuti et al., 2012). Furthermore, diversity of secondary metabolite production among Streptomyces has supported the formulation of inoculants for biocontrol like Mycostop® (Verdera, Finland) and Actinovate (Natural Industries, Incorporated, United States), based on S. griseoviridis K61 and S. lidicus WYEC 108, respectively.
Biocontrol agent selection is not a simple task due to the diversity of agents and interactions with the host plant (Mota et al., 2017). Efficient selection strategies have been proposed in order to increase the possibility of identifying organisms that can be produced in a large scale and maintain their viability and efficiency for extended periods of time (Schisler et al., 1997; Köhl et al., 2011). This study presents a systematic assessment of Streptomyces strains for development of bioinoculants able to promote plant growth and control rice phytopathogens like B. glumae and P. fuscovaginae. Our approach included: (1) Screening for actinobacterial isolates from the rice rhizosphere, favoring selection of bacteria from the same ecological niche; (2) Screening for PGPR and biocontrol properties in vitro, (3) Taxonomical identification for isolates with broader antimicrobial activity, (4) Assessment of plant growth promotion and colonization, in vivo, and (4) Antimicrobial compounds identification.
Sixty actinobacterial-like isolates were obtained from Colombian soils upon enrichment with CaCO3, but only three of them (A20, 5.1 and 7.1) showed antibacterial activity against pathogenic bacterial strains of both B. glumae and P. fuscovaginae. Taxonomical analyses based on 16S rRNA confirmed that all three strains belong to the genus Streptomyces, and MLST results suggested that these isolates might represent new species within the genus. Evolutionary distance analyses between concatenated loci from isolate A20 and type strain S. racemochromogenes DSM 40194 suggested that Streptomyces A20 is likely to be a new member of this species clade.
Those selected isolates were further studied, regarding their antimicrobial potential, plant growth promotion and plant colonization. Despite their common origin (rice rhizosphere), all strains showed a different spectrum of action, being Streptomyces A20 able to inhibit growth for a broader range of bacterial and fungal phytopathogens. When tested against 48 B. glumae pathogenic isolates, most of them were also inhibited by Streptomyces A20, which indicated that this isolate could be potentially used, alone or in combination with other strains to control BPB.
Production of broad-spectrum antimicrobials has been reported for plant-associated Streptomyces species, supporting their use to control fungal and nematode phytopathogens (Emmert and Handelsman, 1999; Samac and Kinkel, 2001; Taechowisan et al., 2003; Tian et al., 2004; Zarandi et al., 2009). Furthermore, biogeographic studies about the ecology of Streptomyces have hypothesized that Streptomyces with broad and highly potent inhibitory phenotypes might be competitive ‘hot spots,’ which have selected for populations that are useful inhibitors of resource competitors, which may be the case for rice rhizosphere (Schlatter and Kinkel, 2014; Behie et al., 2016).
The three selected strains were tested in vitro for properties that are known to be important for plant growth promoting activities of bacteria, such as the production of siderophores, solubilization of inorganic phosphate, phytohormone levels etc. Overall, these suggested that all three strains can solubilize phosphate and produce siderophores, proteases, and IAA, which are also typical of plant-associated Actinobacteria, and have been related to plant-growth promotion (Nimnoi et al., 2010; Bouizgarne and Aouamar, 2014; Carvalho et al., 2017).
Many biocontrol bacteria are only capable of preventing or limiting the establishment of soilborne pathogens after colonizing plant roots, as root colonization is necessary for the suppression of root pathogens (Mota et al., 2017). As a result, several studies have been directed at identifying the different bacterial traits that may contribute to their rhizospheric competence (De Weert and Bloemberg, 2006). Since in vitro experiments revealed that Streptomyces A20, 5.1 and 7.1 strains carry several features important for biocontrol and plant growth promotion, we were interested in assessing plant growth promotion and colonization in vivo, using two cultivars of rice. Plant growth promotion experiments were performed both with sterile and non-sterile conditions in order to compare the performance of these strains in such environments and foresee their behavior under natural conditions. Our results indicated that in non-sterile soil, plants inoculated with Streptomyces A20 and Streptomyces 7.1 showed significant increases in their biometric parameters, with the same trend they showed in the gnotobiotic experiments. Furthermore, colonization experiments indicated that all Streptomyces strains tested were able to colonize the roots, since they were able to prevail in close association with roots, in two different cultivars, even after 31 days post-inoculation (dpi).
Interestingly, Streptomyces A20 and Streptomyces 7.1 were also able to colonize the root endosphere, as they were recovered from a percentage of surface sterilized plants (80 and 20%, respectively), grown in a gnotobiotic system. This last trend was not observed for plants grown in soil indicating that it might be due to the growth conditions, as well as possible competition against the other microbiota present in the soil and associated to the plant. These results are consistent with similar findings reported for other Actinobacteria, including Streptomyces strains (S. cyaneus, S. lanatus, and S. galilaeus), which were also recovered from the endosphere of the roots (Tian et al., 2004, 2007; Knief et al., 2012). Field experiments with more extended growth periods may be required to determine if these strains can colonize the endosphere under natural conditions.
In order to monitor the rice colonization process by strain Streptomyces A20, colonization experiments were performed with an egfp tagged mutant. Our results indicated that egfp expressing strain strongly associated with root hairs and experiments in inoculated and non-inoculated plants showed an increase in root-hair formation, which may be related to the plant growth promotion observed in the gnotobiotic experiments.
Antimicrobial compound identification is convenient when developing biocontrol products. In this study, several chromatographic techniques were used to purify antimicrobial compounds produced by strain A20, that exhibited antibacterial activity against B. glumae AU6208 and P. fuscovaginae UPB0736. Results indicated that strain A20 produces three close related compounds, according to their similar spectra patterns (m/z values at 503.3, 631.4, and 759.4). The mass spectra and their MS/MS study allowed identifying those compounds as Streptothricin F, D and E. A typical streptothricin structure consists of a streptolidine, a carbamoyl-D-gulosamine and a β-lysine chain, which varies from one to six units in streptothricins F to A, respectively (Khokhlov and Shutova, 1972; Telesnina et al., 1973; Khokhlov, 1983). Streptothricins (STs) were the earliest reported antibiotics with a broad antimicrobial spectrum from actinomycetes and are found in approximately 10% of randomly collected soil actinomycetes. These compounds are also known as nourseothricins, racemomycins, and yazumycins, and the first member of this group, designated ST-F, was isolated from Streptomyces lavendulae in 1943 (Waksman, 1943; Maruyama and Hamano, 2009).
STs are known to inhibit protein biosynthesis in prokaryotic cells and strongly inhibit the growth of eukaryotes like fungi and have been suggested as a selection marker for a wide range of organisms including bacteria, yeast, filamentous fungi, and plant cells. A known example is Nourseothricin (CloNat), an effective selective agent for molecular cloning technologies in fungi and plants (Goldstein and McCusker, 1999; Kochupurakkal and Iglehart, 2013). Despite their wide range of antimicrobial activity, STs are not currently indicated for clinical or veterinary uses because of their inherent toxicity, including nephrotoxicity (Hartl et al., 1986). On the other hand, streptothricins are currently being tested as fungistatic agents in agriculture for the treatment of blast and other plant diseases (Goo et al., 1996). Lastly, STs have been registered as agrochemical fungicides in China (Kusumoto et al., 1982).
As indicated in the Streptomyces chemical database StreptomeDB (Lucas et al., 2013), streptothricins production has been reported for Streptomyces ginlingensis, S. rochei, S. flavus, S. lavendulae, S. griseus, S. roseochromogenes, and S. noursei. Maruyama and Hamano reported an extended study describing the genetic cluster responsible of the streptothricin synthesis in S. rochei NBRC12908. Interestingly, this cluster includes a self-resistance gene encoding a ST acetyltransferase (SAT). In addition degenerate primers were proposed as a tool to identify SAT-related immunity genes using highly conserved amino acid sequences of SATs identified in ST-resistant microorganisms (Maruyama et al., 2012). We did not obtain amplification for a potential SAT locus using genomic DNA from Streptomyces A20 (data not shown); this may indicate that this locus in strain A20 differs at sequence level from those reported so far, although it is also possible that strain A20 possesses a different mechanism for ST tolerance.
Plant growth promotion, colonization abilities and antimicrobial activities shown by strain Streptomyces A20 suggest that this strain could be used for plant growth promotion and biocontrol in rice. One significant advantage for this isolate is that it was initially recovered from rice sown soils, and thus it fulfills the premise that microorganisms chosen for inoculation should ideally be selected from local ecological niches and re-inoculated into the same environments, to ensure the desired benefits. Field experiments are necessary to further verify the effects on rice plants under natural growth conditions. Further studies will allow establishing the relationship between application of strain Streptomyces A20 and plant disease suppression, as well as the spatial-temporal dynamics of the pathogens and antagonists in soil (Johnson and Dileone, 1999).
Author Contributions
ZS-M, DV-V, and DV-M performed the experiments for bacterial isolation, identification and antimicrobial screening and plant experiments. LC and FR designed chemical fractionation schemes for the elucidation of bioactive compounds and data analyses for all chromatographic techniques. CG and GD developed ultrafiltration studies, chromatography and mass spectrometry experiments for compound elucidation and provided advice about data analyses. ZS-M, NM-S, LC, FR, GD, and VV contributed to the conception and design of the study. NM-S was the leader of the project. All authors contributed to the manuscript revision, read and approved the submitted version.
Funding
ZS-M was supported by a fellowship from the program “Inserción Laboral de Doctores en Empresas Colombianas-535” from COLCIENCIAS (Contract No. 0185-2012). ZS-M and DV-M were funded by an ICGEB Short-term fellowship to perform collaborative research at ICGEB-Trieste.
Conflict of Interest Statement
The authors declare that the research was conducted in the absence of any commercial or financial relationships that could be construed as a potential conflict of interest.
Acknowledgments
ZS-M thanks the Program “Inserción Laboral de Doctores en Empresas Colombianas” (535) for the granted scholarship. ZS-M and DV-M thank ICGEB-Trieste for the granted fellowships to perform research in Italy. Strains of Burkholderia spp and fungal strains were kindly provided by the Biotechnology Institute of the Universidad Nacional de Colombia. E. coli S17-1 λ pir harboring pIJ8641 plasmid was kindly provided by M. Bibb from the John Innes Centre, Norwich, United Kingdom. Chromobacterium violaceum ATCC31532 was kindly provided by Prof. Catalina Arévalo Ferro, Departamento de Biología, Universidad Nacional de Colombia.
Supplementary Material
The Supplementary Material for this article can be found online at: https://www.frontiersin.org/articles/10.3389/fmicb.2019.00290/full#supplementary-material
FIGURE S1 | The figure shows the plate count technique used for the determination of Colony-Forming Units of Streptomyces isolates (A) and the macroscopic and microscopic characterization of the selected Streptomyces strains (B).
FIGURE S2 | Rice colonization assays. Two independent assays were performed under gnotobiotic and soil conditions, using three biological replicates for each strain, with five plants per replicate in two rice cultivars (F60 and F733). Bacterial strains were re-isolated from the rhizoplane by cutting and washing 2 cm segments of each plant root and placing the excised root segments on the surface of ISP3 plates. To assess the ability of each strain to colonize the rhizoplane, samples were macerated and serially diluted and plated on ISP3 plates. Note that strain Streptomyces A20 was able to colonize both cultivars. To verify endophytic colonization, inoculated plants were surface sterilized and each plant was then excised in the root, plant shoot and leaves, and each part was macerated with a sterile pestle. Each suspension was then serially diluted and plated in ISP3 plates, and surface sterilization was regarded as optimum when no growth was observed after placing the processed plants on nutrient agar plates. This experiment suggests that strains A20 and 7.1 are able to colonize the endosphere of both cultivars. Furthermore, microscopy of the treated roots, stained with Lactophenol blue suggests the presence of Streptomyces A20 mycelium on and around the surface of and adventitious root (see arrows).
FIGURE S3 | Bioassay-guided fractionation of A20 antibacterial compounds. (A) Fractionation Scheme. (B) Antimicrobial assays in B. glumae AU6208 and P. fuscovaginae UPB0736. The red numbers in the fractionating scheme (A) correspond to the fractions tested in the Kirby-Bauer test shown in (B). Control plates include Gentamycin (Gm) as positive control for antimicrobial activity, and Water (W) as negative control. To exclude any effect from the media, media, M3.7 was processed in the same way, and fractions 3, 4, 5, and 6 were tested in control plates.
FIGURE S4 | Picture of inoculated rice plants (Cultivar 733) with Streptomyces spp. strains vs. controls. Streptomyces 5.1 was able to improve the fresh weight of roots and shoots in cultivar 733, and strains A20 and 7.1 displayed the ability to significantly increase all the biometric parameters evaluated in cultivar F733 in comparison to the non-inoculated control. Azotobacter chroococcum was used as positive control for plant growth promotion (AC).
TABLE S1 | Microbial strains and primers used in this study.
Abbreviations
ACC, 1-aminocyclopropane-1-carboxylate; BPB, Bacterial panicle Blight; CMC, carboxymethylcelulose; IAA, Indoleacetic Acid; LC-MS, liquid chromatography–mass spectrometry; MLST, Multilocus Sequence Typing; PGPB, Plant-Growth Promoting Bacteria; SBR, Sheath Brown Rot.
Footnotes
References
Behie, S. W., Bonet, B., Zacharia, V. M., McClung, D. J., and Traxler, M. F. (2016). Molecules to ecosystems: actinomycete natural products in situ. Front. Microbiol. 7:2149. doi: 10.3389/fmicb.2016.02149
Belimov, A., Safronova, V. I., and Sergeyeva, T. A. (2001). Characterization of plant growth promoting rhizobacteria isolated from polluted soils and containing 11-aminocyclopropane-1-carboxylate deaminase. Can. J. Microbiol. 47, 642–652. doi: 10.1139/w01-062
Bouizgarne, B., and Aouamar, A. A. B. (2014). “Diversity of Plant Associated Actinobacteria,” in Bacterial Diversity in Sustainable Agriculture, ed. D. K. Maheshwari (Cham: Springer International Publishing), 41–99.
Carvalho, M. F., Ma, Y., Oliveira, R. S., and Freitas, H. (2017). “Endophytic Actinobacteria for Sustainable Agricultural Applications,” in Endophytes: Crop Productivity and Protection, Vol. 2, eds D. K. Maheshwari and K. Annapurna (Cham: Springer International Publishing), 163–189. doi: 10.1007/978-3-319-66544-3_8
Castillo, L. A., Pineda, D., Ospina, J., Echeverry, J., Perafan, R., and Garcés, G. (2011). Cambio climático y producción de Arroz. Revista Arroz 58, 5–12.
Clinical Laboratory Standards Institute (2011). Performance Standards for Antimicrobial Susceptibility Testing; Twenty-first Informational Supplement CLSI document M100-S21. Wayne, PA: CLSI.
Cole, J. R., Wang, Q., Fish, J. A., Chai, B., McGarrell, D. M., Sun, Y., et al. (2014). Ribosomal database project: data and tools for high throughput rRNA analysis. Nucleic Acids Res. 42, D633–D642. doi: 10.1093/nar/gkt1244
Conn, V. M., Walker, A. R., and Franco, C. M. (2008). Endophytic actinobacteria induce defense pathways in Arabidopsis thaliana. Mol. Plant Microbe Interact. 21, 208–218. doi: 10.1094/MPMI-21-2-0208
Correa, F., Perez, C. R., and Saavedra, E. (2007). Añublo bacterial de la panícula del arroz. Revista Arroz 468, 26–31.
Cui, X., Mao, P. H., Zeng, M., Li, W. J., Zhang, L. P., Xu, L. H., et al. (2001). Streptimonospora salina gen. nov., sp. nov., a new member of the family Nocardiopsaceae. Int. J. Syst. Evol. Microbiol. 51, 357–363. doi: 10.1099/00207713-51-2-357
De Weert, S., and Bloemberg, G. V. (2006). “Rhizosphere competence and the role of root colonization in biocontrol,” in Plant-Associated Bacteria, ed. S. S. Gnanamanickam (Dordrecht: Springer Netherlands), 317–333.
Diago, M., Ospina, J., Peìrez, C. R., Saavedra, J., Echeverri, J., Cuevas, J., et al. (2009). Un buen manejo del cultivo, verdadera barrera contra el añϸublo bacterial. Revista Arroz 57, 30–38.
Dobereiner, J. (1995). “Isolation and identification of aerobic nitrogen fixing bacteria form soil and plants,” in Methods in Applied Soil Microbiology and Biochemistry, eds K. Alef and P. Nampieri (London: Academic Press), 161–174.
El-tarabily, K. A. (2008). Promotion of tomato (Lycopersicon esculentum Mill.) plant growth by rhizosphere competent 1-aminocyclopropane-1-carboxylic acid deaminase - producing streptomycete actinomycetes. Plant Soil 308, 161–174. doi: 10.1007/s11104-008-9616-2
Emmert, E. A., and Handelsman, J. (1999). Biocontrol of plant disease: a gram positive perspective. FEMS Microbiol. Lett. 171, 1–9. doi: 10.1111/j.1574-6968.1999.tb13405.x
Fory, P. A., Triplett, L. R., Ballen, C., Abello, J. F., Duitama, J., Aricapa, G., et al. (2014). Comparative analysis of two emerging rice seed bacterial pathogens. Phytopathology 104, 436–444. doi: 10.1094/PHYTO-07-13-0186-R
Francis, F., Kim, J., Ramajaj, T., Farmer, A., Rush, M. C., and Ham, J. H. (2013). Comparative genomic analysis of two Burkholderia glumae strains from different geographic origins reveals a high degree of plasticity in genome structure associated with genomic islands. Mol. Genet. Genomics 288, 195–203. doi: 10.1007/s00438-013-0744-x
Gascuel, O. (1997). BIONJ: an improved version of the NJ algorithm based on a simple model of sequence data. Mol. Biol. Evol. 14, 685–695. doi: 10.1093/oxfordjournals.molbev.a025808
Glickmann, E., and Dessaux, Y. (1995). A critical examination of the specificity of the salkowski reagent for indolic compounds produced by phytopathogenic bacteria. Appl. Environ. Microbiol. 61, 793–796.
Goldstein, A. L., and McCusker, J. H. (1999). Three new dominant drug resistance cassettes for gene disruption in Saccharomyces cerevisiae. Yeast 15, 1541–1553. doi: 10.1002/(SICI)1097-0061(199910)
Goo, Y. M., Kim, O. Y., Joe, Y. A., Lee, Y. B., Ju, J., Kim, B. T., et al. (1996). A new streptothricin family antibiotic producing Streptomyces spp. SNUS 8810-111; characterization of the producing organisms, fermentation, isolation, and structure elucidation of antibioitics. Arch. Pharm. Res. 19, 153–159. doi: 10.1007/BF02976852
Goodfellow, M. (2012). “Phylum XXVI. Actinobacteria phyl. nov,” in Bergey’s Manual of Systematic Bacteriology, eds W. B. Whitman, M. Goodfellow, P. Kämpfer, H. J. Busse, M. E. Trujillo, W. Ludwig, et al. (New York, NY: Springer Science Business Media), 34–2028. doi: 10.1007/978-0-387-68233-4
Gopalakrishnan, S., Pande, S., Sharma, M., Humayun, P., Kiran, B. K., Sandeep, D., et al. (2011). Evaluation of actinomycete isolates obtained from herbal vermicompost for the biological control of Fusarium wilt of chickpea. Crop Prot. 30, 1070–1078. doi: 10.1016/j.cropro.2011.03.006
Goto, K., Nishiyama, K., and Ohata, K. (1987). Bacteria causing grain rot of rice. Ann. Phytopathol. Soc. Jpn. 53, 141–149. doi: 10.3186/jjphytopath.53.141
Goto, K., and Ohata, K. (1956). New bacterial diseases on rice (brown stripe and grain rot). Ann. Phytopathol. Soc. Jpn. 21, 46–47.
Guo, Y., Zheng, W., Rong, X., and Huang, H. Y. (2008). A multilocus phylogeny of the Streptomyces griseus 16S rRNA gene clade: use of multilocus sequence analysis for streptomycete systematics. Int. J. Syst. Evol. Microbiol. 2008, 149–159. doi: 10.1099/ijs.0.65224-0
Gurung, T. D., Sherpa, C., Agrawal, V. P., and Lekhak, B. (2009). Isolation and characterization of antibacterial actinomycetes from soil samples of kalapatthar, mount everest region. Nepal J. Sci. Technol. 10, 173–182. doi: 10.3126/njst.v10i0.2957
Ham, J. H., Melanson, R. A., and Rush, M. C. (2011). Burkholderia glumae: next major pathogen of rice? Mol. Plant Pathol. 12, 329–339. doi: 10.1111/j.1364-3703.2010.00676.x
Handelsman, J., and Stabb, E. V. (1996). Biocontrol of soilborne plant pathogens. Plant Cell 8, 1855–1869. doi: 10.1105/tpc.8.10.1855
Hardy, R. W., Holsten, R. D., Jackson, E. K., and Burns, R. C. (1968). The acetylene-ethylene assay for N(2) fixation: laboratory and field evaluation. Plant Physiol. 43, 1185–1207. doi: 10.1104/pp.43.8.1185
Hartl, A., Guttner, J., Stockel, U., and Hoffmann, H. (1986). Acute and subchronic toxicity of nourseothricin in laboratory animals. Arch. Exp. Veterinarmed. 40, 727–735.
Hastuti, R. D., Lestari, Y., Saraswati, R., and Suwanto, A. (2012). Capability of Streptomyces spp. in controlling bacterial leaf blight disease in rice plants. Am. J. Agric. Biol. Sci. 7, 217–223. doi: 10.3844/ajabssp.2012.217.223
Herrmann, L., and Lesueur, D. (2013). Challenges of formulation and quality of biofertilizers for successful inoculation. Appl. Microbiol. Biotechnol. 97, 8859–8873. doi: 10.1007/s00253-013-5228-8
Hikichi, Y., Tsujiguchi, K., Maeda, Y., and Okuno, T. (2001). Development of increased oxolinic acid-resistance in Burkholderia glumae. J. Gen. Plant Pathol. 67, 57–62. doi: 10.1007/PL00012988
Jeong, Y., Kim, J., Kim, S., Kang, Y., Nagamatsu, T., and Hwang, I. (2003). Toxoflavin produced by Burkholderia glumae causing rice grain rot is responsible for inducing bacterial wilt in many field crops. Plant Dis. 87, 890–895. doi: 10.1094/PDIS.2003.87.8.890
Ji, Z., Wang, M., Wei, S., Zhang, J., and Wu, W. (2009). Isolation, structure elucidation and antibacterial activities of streptothricin acids. J. Antibiot. 62, 233–237. doi: 10.1038/ja.2009.16
Ji, Z., Wang, M., Zhang, J., Wei, S., and Wu, W. (2007). Two new members of streptothricin class antibiotics from Streptomyces qinlingensis sp. nov. J. Antibiot. 60, 739–744. doi: 10.1038/ja.2007.96
Ji, Z., Wei, S., Zhang, J., Wu, W., and Wang, M. (2008). Identification of streptothricin class antibiotics in the early-stage of antibiotics screening by electrospray ionization mass spectrometry. J. Antibiot. 61, 660–667. doi: 10.1038/ja.2008.93
Johnson, K. B., and Dileone, J. A. (1999). Effect of antibiosis on antagonist dose-plant disease response relationships for the biological control of crown gall of tomato and cherry. Phytopathology 89, 974–980. doi: 10.1094/PHYTO.1999.89.10.974
Jolley, K. A., and Maiden, M. C. (2010). BIGSdb: scalable analysis of bacterial genome variation at the population level. BMC Bioinformatics 11:595. doi: 10.1186/1471-2105-11-595
Kanini, G. S., Katsifas, E. A., Savvides, A. L., Hatzinikolaou, D. G., and Karagouni, A. D. (2013). Greek indigenous streptomycetes as biocontrol agents against the soil-borne fungal plant pathogen Rhizoctonia solani. J. Appl. Microbiol. 114, 1468–1479. doi: 10.1111/jam.12138
Karki, H. S., Shrestha, B. K., Han, J. W., Groth, D. E., Barphagha, I. K., Rush, M. C., et al. (2012). Diversities in virulence, antifungal activity, pigmentation and DNA fingerprint among strains of Burkholderia glumae. PLoS One 7:e45376. doi: 10.1371/journal.pone.0045376
Kawanishi, T., Shiraishi, T., Okano, Y., Sugawara, K., Hashimoto, M., Maejima, K., et al. (2011). New detection systems of bacteria using highly selective media designed by SMART: selective medium-design algorithm restricted by two constraints. PLoS One 6:e16512. doi: 10.1371/journal.pone.0016512
Khokhlov, A. S. (1983). Achievements in the study of streptothricin antibiotics. Antibiotiki 28, 613–622.
Khokhlov, A. S., and Shutova, K. I. (1972). Chemical structure of streptothricins. J. Antibiot. 25, 501–508. doi: 10.7164/antibiotics.25.501
Kieser, T., Bibb, M. J., Buttner, K. F., Chater, K. F., and Hopwood, D. A. (2000). Practical Streptomyces Genetics. Norwich: The John Innes Foundation.
Kim, O. S., Cho, Y. J., Lee, K., Yoon, S. H., Kim, M., Na, H., et al. (2012). Introducing EzTaxon-e: a prokaryotic 16S rRNA gene sequence database with phylotypes that represent uncultured species. Int. J. Syst. Evol. Microbiol. 62(Pt 3), 716–721. doi: 10.1099/ijs.0.038075-0
Kim, S., Park, J., Lee, J., Shin, D., Park, D. S., Lim, J. S., et al. (2014). Understanding pathogenic Burkholderia glumae metabolic and signaling pathways within rice tissues through in vivo transcriptome analyses. Gene 547, 77–85. doi: 10.1016/j.gene.2014.06.029
Kinkel, L. L., Schlatter, D. C., Bakker, M. G., and Arenz, B. E. (2012). Streptomyces competition and co-evolution in relation to plant disease suppression. Res. Microbiol. 163, 490–499. doi: 10.1016/j.resmic.2012.07.005
Knief, C., Delmotte, N., Chaffron, S., Stark, M., Innerebner, G., Wassmann, R., et al. (2012). Metaproteogenomic analysis of microbial communities in the phyllosphere and rhizosphere of rice. ISME J. 6, 1378–1390. doi: 10.1038/ismej.2011.192
Kochupurakkal, B. S., and Iglehart, J. D. (2013). Nourseothricin N-acetyl transferase: a positive selection marker for mammalian cells. PLoS One 8:e68509. doi: 10.1371/journal.pone.0068509
Köhl, J., Postma, J., Nicot, P., Ruocco, M., and Blum, B. (2011). Stepwise screening of microorganisms for commercial use in biological control of plant-pathogenic fungi and bacteria. Biol. Control 57, 1–12. doi: 10.1016/j.biocontrol.2010.12.004
Kurita, T., and Tabei, H. (1967). On the pathogenic bacterium of bacterial grain rot of rice. Ann. Phytopathol. Soc. Jpn. 33:111.
Kurth, F., Mailander, S., Bonn, M., Feldhahn, L., Herrmann, S., Grosse, I., et al. (2014). Streptomyces-induced resistance against oak powdery mildew involves host plant responses in defense, photosynthesis, and secondary metabolism pathways. Mol. Plant Microbe Interact. 27, 891–900. doi: 10.1094/MPMI-10-13-0296-R
Kusumoto, S., Kambayashi, Y., Imaoka, S., Shima, K., and Shiba, T. (1982). Total chemical structure of streptothricin. J. Antibiot. 35, 925–927. doi: 10.7164/antibiotics.35.925
Labeda, D. P., Doroghazi, J. R., Ju, K. S., and Metcalf, W. W. (2014). Taxonomic evaluation of Streptomyces albus and related species using multilocus sequence analysis and proposals to emend the description of Streptomyces albus and describe Streptomyces pathocidini sp. nov. Int. J. Syst. Evol. Microbiol. 64(Pt 3), 894–900. doi: 10.1099/ijs.0.058107-0
Labeda, D. P., Goodfellow, M., Brown, R., Ward, A. C., Lanoot, B., Vanncanneyt, M., et al. (2012). Phylogenetic study of the species within the family Streptomycetaceae. Antonie Van Leeuwenhoek 101, 73–104. doi: 10.1007/s10482-011-9656-0
Lucas, X., Senger, C., Erxleben, A., Gruning, B. A., Doring, K., Mosch, J., et al. (2013). StreptomeDB: a resource for natural compounds isolated from Streptomyces species. Nucleic Acids Res. 41, D1130–D1136. doi: 10.1093/nar/gks1253
Magbanua, Z. V., Arick, M. II, Buza, T., Hsu, C. Y., Showmaker, K. C., Chouvarine, P., et al. (2014). Transcriptomic dissection of the rice-Burkholderia glumae interaction. BMC Genomics 15:755. doi: 10.1186/1471-2164-15-755
Mahmoud, A. L. E., and Abdallah, M. A. (2001). Siderophores production by some microorganisms and their effect on Bradyrhizobium-mungbean symbiosis. Int. J. Agric. Biol. 3, 157–162.
Maruyama, C., and Hamano, Y. (2009). The biological function of the bacterial isochorismatase-like hydrolase SttH. Biosci. Biotechnol. Biochem. 73, 2494–2500. doi: 10.1271/bbb.90499
Maruyama, C., Toyoda, J., Kato, Y., Izumikawa, M., Takagi, M., Shin-ya, K., et al. (2012). A stand-alone adenylation domain forms amide bonds in streptothricin biosynthesis. Nat. Chem. Biol. 8, 791–797. doi: 10.1038/nchembio.1040
Mattiuzzo, M., Bertani, I., Ferluga, S., Cabrio, L., Bigirimana, J., Guarnaccia, C., et al. (2010). The plant pathogen Pseudomonas fuscovaginae contains two conserved quorum sensing systems involved in virulence and negatively regulated by RsaL and the novel regulator RsaM. Environ. Microbiol. 13, 145–162. doi: 10.1111/j.1462-2920.2010.02316.x
Mattos, K. A., Padua, V. L., Romeiro, A., Hallack, L. F., Neves, B. C., Ulisses, T. M., et al. (2008). Endophytic colonization of rice (Oryza sativa L.) by the diazotrophic bacterium Burkholderia kururiensis and its ability to enhance plant growth. An. Acad. Bras. Cienc. 80, 477–493. doi: 10.1590/S0001-37652008000300009
Miransari, M. (2013). Soil microbes and the availability of soil nutrients. Acta Physiol. Plant 35, 3075–3084. doi: 10.1007/s11738-013-1338-2
Mizobuchi, R., Sato, H., Fukuoka, S., Tanabata, T., Tsushima, S., Imbe, T., et al. (2013). Mapping a quantitative trait locus for resistance to bacterial grain rot in rice. Rice 6:13. doi: 10.1186/1939-8433-6-13
Moreno, N. (2011). Optimising carbon and nitrogen sources for Azotobacter chrococcum growth. Afr. J. Biotechnol. 10, 2951–2958. doi: 10.5897/AJB10.1484
Moreno, N., and Rojas, J. (2008). Producción y formulación de prototipos de un biofertilizante a partir de bacterias nativas asociadas al cultivo de arroz (Oryza sativa). Rev. Colomb. Biotecnol. 10, 50–62.
Mota, M. S., Gomes, C. B., Souza Junior, I. T., and Moura, A. B. (2017). Bacterial selection for biological control of plant disease: criterion determination and validation. Braz. J. Microbiol. 48, 62–70. doi: 10.1016/j.bjm.2016.09.003
Nagadesi, P. K., and Arya, A. (2012). Lignocellulolytic activity of wood-inhabiting fungi from ratanmahal wildlife sanctuary gujarat, india. Biotechnol. Adv. 12, 30–36.
Nandakumar, R., Rush, M. C., and Correa, F. (2007). Association of Burkholderia glumae and Burkholderia gladioli with panicle blight symptoms on rice in panama. Plant Dis. 91:767. doi: 10.1094/PDIS-91-6-0767C
Nandakumar, R., Shahjahan, A. K. M., Yuan, X. L., Dickstein, E. R., Groth, D. E., Clarck, C. A., et al. (2009). Burkholderia glumae and B. gladioli cause bacterial panicle blight in rice in the Southern United States. Plant Dis. 93, 896–905. doi: 10.1094/PDIS-93-9-0896
Nautiyal, C. S. (1999). An efficient microbiological growth medium for screening phosphate solubilizing microorganisms. FEMS Microbiol. Lett. 170, 265–270. doi: 10.1111/j.1574-6968.1999.tb13383.x
Nimnoi, P., Pongsilp, N., and Lumyong, S. (2010). Endophytic actinomycetes isolated from Aquilaria crassna pierre ex Lec and screening of plant growth promoters production. World J. Microbiol. Biotechnol. 26, 193–203. doi: 10.1007/s11274-009-0159-3
Pospiech, A., and Neumann, B. (1995). A versatile quick-prep of genomic DNA from Gram-positive bacteria. Trends Genet. 11, 217–218. doi: 10.1016/S0168-9525(00)89052-6
Qin, S., Xing, K., Jiang, J. H., Xu, L. H., and Li, W. J. (2011). Biodiversity, bioactive natural products and biotechnological potential of plant-associated endophytic actinobacteria. Appl. Microbiol. Biotechnol. 89, 457–473. doi: 10.1007/s00253-010-2923-2926
Razak, A. A., Zainudin, N. A., Sidiqe, S., Ismail, N. A., Mohamad, N. I., and Baharuddin, S. (2009). Sheat brown rot disease of rice caused by Pseudomonas fuscovaginae in the peninsular Malasya. J. Plant Prot. Res. 49, 244–249. doi: 10.2478/v10045-009-0037-x
Riedel, K., Hentzer, M., Geisenberger, O., Huber, B., Steidle, A., Wu, H., et al. (2001). N-acylhomoserine-lactone-mediated communication between Pseudomonas aeruginosa and Burkholderia cepacia in mixed biofilms. Microbiology 147(Pt 12), 3249–3262. doi: 10.1099/00221287-147-12-3249
Rong, X., Guo, Y., and Huang, H. Y. (2009). Proposal to reclassify the Streptomyces albidoflavus clade on the basis of multilocus sequence analysis and DNA–DNA hybridization, and taxonomic elucidation of Streptomyces griseus subsp. solvifaciens. Syst. Appl. Microbiol. 32, 314–322. doi: 10.1016/j.syapm.2009.05.003
Rong, X., and Huang, Y. (2012). Taxonomic evaluation of the Streptomyces hygroscopicus clade using multilocus sequence analysis and DNA-DNA hybridization, validating the MLSA scheme for systematics of the whole genus. Syst. Appl. Microbiol. 35, 7–18. doi: 10.1016/j.syapm.2011.10.004
Saitou, N., and Nei, M. (1987). The neighbor-joining method: a new method for reconstructing phylogenetic trees. Mol. Biol. Evol. 4, 406–425. doi: 10.1093/oxfordjournals.molbev.a040454
Samac, D. A., and Kinkel, L. L. (2001). Suppression of the root-lesion nematode (Pratylenchus penetrans) in alfalfa (Medicago sativa) by Streptomyces spp. Plant Soil 235, 35–44. doi: 10.1023/a:1011820002779
Sayler, R. J., Cartwright, R. D., and Yang, K. Y. (2006). Genetic characterizacion and real-time PCR detection of Burkholderia glumae, a newly emerging bacterial pathogen of rice in the United States. Plant Dis. 90, 603–610. doi: 10.1094/PD-90-0603
Scervino, J. M., Prieto, M., Della Mónica, I., Recchi, M., Moreno, N., and Godeas, A. (2010). Soil fungal isolates produce different organic acid patterns involved in phosphate salts solubilization. Biol. Fertil. Soils 46, 755–763. doi: 10.1007/s00374-010-0482-8
Schisler, D. A., Slininger, P. J., and Bothast, R. J. (1997). Effects of antagonist cell concentration and two-strain mixtures on biological control of fusarium dry rot of potatoes. Phytopathology 87, 177–183. doi: 10.1094/PHYTO.1997.87.2.177
Schlatter, D. C., and Kinkel, L. L. (2014). Global biogeography of Streptomyces antibiotic inhibition, resistance, and resource use. FEMS Microbiol. Ecol. 88, 386–397. doi: 10.1111/1574-6941.12307
Schwyn, B., and Neilands, J. B. (1987). Universal chemical assay for the detection and determination of siderophores. Anal. Biochem. 160, 47–56. doi: 10.1016/0003-2697(87)90612-9
Shirling, E., and Gottlieb, D. (1968). Cooperative descriptions of type cultures of Streptomyces III. Additional species descriptions from first and second studies. Int. J. Syst. Bacteriol. 18, 279–392. doi: 10.1099/00207713-18-4-27
Sun, J., Kelemen, G. H., Fernandez-Abalos, J. M., and Bibb, M. J. (1999). Green fluorescent protein as a reporter for spatial and temporal gene expression in Streptomyces coelicolor A3(2). Microbiology 145(Pt 9), 2221–2227. doi: 10.1099/00221287-145-9-2221
Sundara-Rao, W. V. B., and Sinha, M. K. (1963). Phosphate disolving microorganisms in the soil and rhizosphere. Indian J. Agric. Sci. 33, 272–278.
Taechowisan, T., Peberdy, J. F., and Lumyong, S. (2003). Chitinase production by endophytic Streptomyces aureofaciens CMUAc130 and its antagonism against phytopathogenic fungi. Ann. Microbiol. 53, 447–461.
Tamura, K., and Nei, M. (1993). Estimation of the number of nucleotide substitutions in the control region of mitochondrial DNA in humans and chimpanzees. Mol. Biol. Evol. 10, 512–526. doi: 10.1093/oxfordjournals.molbev.a040023
Tamura, K., Peterson, D., Peterson, N., Stecher, G., Nei, M., and Kumar, S. (2011). MEGA5: molecular evolutionary genetics analysis using maximum likelihood, evolutionary distance, and maximum parsimony methods. Mol. Biol. Evol. 28, 2731–2739. doi: 10.1093/molbev/msr121
Tang, Y. W., and Bonner, J. (1948). The enzymatic inactivation of indole acetic acid; the physiology of the enzyme. Am. J. Bot. 35, 570–578. doi: 10.1002/j.1537-2197.1948.tb08123.x
Tanii, A., Miyajima, K., and Akita, T. (1976). The sheath brown rot disease of rice plant and its causal bacterium. Ann. Phytopathol. Soc. Jpn. 42, 540–548. doi: 10.3186/jjphytopath.42.540
Telesnina, G. N., Riabova, I. D., Shutova, K. I., and Khokhlov, A. S. (1973). Mechanism of action of streptothricins. Dokl. Akad. Nauk SSSR 213, 743–745.
Thompson, J. D., Higgins, D. G., and Gibson, T. J. (1994). CLUSTAL W: improving the sensitivity of progressive multiple sequence alignment through sequence weighting, position-specific gap penalties and weight matrix choice. Nucleic Acids Res. 22, 4673–4680. doi: 10.1093/nar/22.22.4673
Tian, X., Cao, L., Tan, H., Han, W., Chen, M., Liu, Y., et al. (2007). Diversity of cultivated and uncultivated actinobacterial endophytes in the stems and roots of rice. Microb. Ecol. 53, 700–707. doi: 10.1007/s00248-006-9163-9164
Tian, X. L., Cao, L. X., Tan, H. M., Zeng, Q. G., Jia, Y. Y., Han, W. Q., et al. (2004). Study on the communities of endophytic fungi and endophytic actinomycetes from rice and their antipathogenic activities in vitro. World J. Microbiol. Biotechnol. 20, 303–309. doi: 10.1023/B:WIBI.0000023843.83692.3f
Weinberg, J. B., Alexander, B. D., Majure, J. M., Williams, L. W., Kim, J. Y., Vandamme, P., et al. (2007). Burkholderia glumae infection in an infant with chronic granulomatous disease. J. Clin. Microbiol. 45, 662–665. doi: 10.1128/JCM.02058-06
Willey, J. M., Sherwood, L. M., and Woolverton, C. J. (2007). Prescott, Harley, and Klein′s Microbiology. New York, NY: McGraw Hill.
Zarandi, M. E., Bonjar, G. H. S., Dehkaei, F. P., Moosavi, S. A. A., Farokhi, P. R., and Aghighi, S. A. (2009). Biological control of rice blast (Magnaporthe oryzae) by use of Streptomyces sindeneusis isolate 263 in greenhouse. Am. J. Appl. Sci. 6, 194–199. doi: 10.3844/ajassp.2009.194.199
Keywords: Streptomyces, biological control, plant growth promotion, Burkholderia glumae, streptothricins
Citation: Suárez-Moreno ZR, Vinchira-Villarraga DM, Vergara-Morales DI, Castellanos L, Ramos FA, Guarnaccia C, Degrassi G, Venturi V and Moreno-Sarmiento N (2019) Plant-Growth Promotion and Biocontrol Properties of Three Streptomyces spp. Isolates to Control Bacterial Rice Pathogens. Front. Microbiol. 10:290. doi: 10.3389/fmicb.2019.00290
Received: 31 August 2018; Accepted: 04 February 2019;
Published: 25 February 2019.
Edited by:
Dirk Tischler, Ruhr-Universität Bochum, GermanyReviewed by:
Collin M. Timm, Johns Hopkins University, United StatesJan Bobek, Charles University, Czechia
Matouš Čihák, Contipro Inc., Czechia, in collaboration with reviewer JB
Copyright © 2019 Suárez-Moreno, Vinchira-Villarraga, Vergara-Morales, Castellanos, Ramos, Guarnaccia, Degrassi, Venturi and Moreno-Sarmiento. This is an open-access article distributed under the terms of the Creative Commons Attribution License (CC BY). The use, distribution or reproduction in other forums is permitted, provided the original author(s) and the copyright owner(s) are credited and that the original publication in this journal is cited, in accordance with accepted academic practice. No use, distribution or reproduction is permitted which does not comply with these terms.
*Correspondence: Zulma Rocío Suárez-Moreno, enJzdWFyZXptQGdtYWlsLmNvbQ==; enVsbWEuc3VhcmV6QHZlY29sLmNvbS5jbw== Nubia Moreno-Sarmiento, bmNtb3Jlbm9zQHVuYWwuZWR1LmNv