- 1Department of Microbiology, Faculty of Life Sciences, University of Central Punjab, Lahore, Pakistan
- 2Department of Microbiology and Molecular Genetics, University of the Punjab, Lahore, Pakistan
- 3Department of Pharmaceutical Biology and Biotechnology, Institute of Pharmaceutical Sciences, University of Freiburg, Freiburg im Breisgau, Germany
- 4School of Food and Agriculture, The University of Maine, Orono, ME, United States
Potato common scab (PCS) is an economically important disease worldwide. In this study we demonstrated the possible role of Streptomyces violaceusniger AC12AB in controlling PCS. Isolates of Streptomyces scabies were obtained from CS infected tubers collected from Maine United States, which were confirmed by morphological and molecular analysis including 16S rRNA sequencing and RFLP analysis of amplified 16S-23S ITS. Pathogenicity assays related genes including txtAB, nec1, and tomA were also identified in all S. scabies strains through PCR reaction. An antagonistic bacterial strain was isolated from soil in Punjab and identified as S. violaceusniger AC12AB based on 16S rRNA sequencing analysis. Methanolic extract of S. violaceusniger AC12AB contained azalomycin RS-22A which was confirmed by 1H and 13C-NMR, 1H/1H-COSY, HMBC and HMQC techniques. S. violaceusniger AC12AB exhibited plant growth promotion attributes including Indole-3-acetic acid production with 17 μgmL-1 titers, siderophores production, nitrogen fixation and phosphates solubilization potential. When tubers were inoculated with S. violaceusniger AC12AB, significant (P < 0.05) PCS disease reduction up to 90% was observed in greenhouse and field trials, respectively. Likewise, S. violaceusniger AC12AB significantly (P < 0.05) increased potato crop up to 26.8% in field trial. Therefore, plant growth promoting S. violaceusniger AC12AB could provide a dual benefit by decreasing PCS disease severity and increasing potato yield as an effective and inexpensive alternative strategy to manage this disease.
Introduction
Potato common scab (PCS) is considered among top five diseases by potato farmers in United States (Slack, 1991). PCS is caused by Gram positive, filamentous bacteria in the genus Streptomyces. Streptomyces are soilborne saprophytic bacteria, mostly famous for the production of antibiotics (Kemung et al., 2018). However, only few of them are plant pathogens. Although, several species of Streptomyces can cause common scab (CS), Streptomyces scabies was considered as predominant plant pathogen (Lambert and Loria, 1989). PCS infection is characterized by superficial, raised or pitted scab lesions on the surface of the tubers. The occurrence of PCS infection is, generally not hazardous to human health. However, they may deteriorate the quality of tubers and effects the market value. For instance, potato industry in Canada reported to experience $1.2 million loss every year due to this disease (Al-Mughrabi et al., 2016).
Scab lesions on the surface of the tubers develop due to a phytotoxin called thaxtomin. In 1898, thaxtomin was firstly described (King et al., 1989; Lawrence et al., 1990) as toxin responsible to produce CS on immature tubers. All PCS causing Streptomyces spp. produce thaxtomin A or another member of thaxtomin family (Loria et al., 2008). Biosynthesis of this phytotoxin encompasses non-ribosomal peptide synthetases encoded by txtA and txtB genes (Loria et al., 2008). The genes responsible for pathogenicity like txtAB, nec1 and tomA are clustered together and termed as pathogenicity island (PAI) (Kers et al., 2005). PAI consists of thaxtomin genes including txtAB (Healy et al., 2000), txtH, txtC (Healy et al., 2002), txtR, txtE (Joshi et al., 2007), nos/txtD (Kers et al., 2004), and genes for pathogenicity factors like Tomatinase (tomA) and Necrotic protein (nec1) (Kers et al., 2005; Barry et al., 2012). Secreted Nec1 protein helps to enhance the virulence by weakening the plant defense mechanism (Bukhalid et al., 1998). TomA gene encodes for a virulent protein having high similarity with phytopathogenic fungi tomatinase (Kers et al., 2005).
Although thaxtomin A, which is encoded by txtAB gene, is considered as a major player toward plant pathogenicity, other genes including nec1 and tomA are also somehow required for the virulence (Loria et al., 2006). Many unknown factors can play a supportive role in pathogenicity. However, the prevalence of CS pathogens without txtAB genes are either very rare or confined to geographic locations (Park et al., 2003; Wanner, 2004).
Over the decades, PCS management remained a serious problem among potato growers. The control strategies are challenging due to limited understanding of genetic diversity of S. scabies and genetic differences in various potato cultivars (Dees and Wanner, 2012). Several physiochemical approaches like reducing soil pH, crop rotation, and soil fumigation agents like chloropicrin (trichloronitromethane) have conventionally been used with harmful effects to the environment (Larkin et al., 2011; Xue et al., 2018). In contrast, research in biological control as an alternative strategy is emerging. Several antagonistic bacteria including Bacillus spp. (Meng et al., 2013), Pseudomonas spp. (Arseneault et al., 2015) and Streptomyces spp. (Sarwar et al., 2018) have been used as biocontrol agent against PCS.
The present study was designed to evaluate the pathogens responsible for PCS incidences and to assess the antagonistic ability of S. violaceusniger. It was hypothesized that S. violaceusniger AC12AB could be used as effective biological control agent due to its ability to promote plant growth and suppress PCS.
Materials and Methods
Sample Collection, Bacterial Isolation, and Identification
Potatoes having visible CS symptoms were collected from Presque Isle, ME, United States. All collected samples were carefully transferred to the laboratory at the University of Maine, United States. Samples were stored at 4°C prior to use.
Tubers with CS symptoms were washed and surface sterilized with 5% sodium hypochlorite (NaOCl) for 1 min. Surface sterilized tubers were rinsed with sterile distilled water and air dried. The infected portion from CS tubers were carefully excised with sterile scalpel and triturated to form a homogenized paste by adding 1 mL Tris-HCl. The homogenized suspension was poured into 2 mL Eppendorf tubes, separately. The Eppendorf tubers were placed at 55°C for 2 h to remove unwanted microorganisms. This suspension was ten-fold diluted with sterile distilled water before pouring onto yeast malt extract (YME) agar plates (Shirling and Gottlieb, 1966). An aliquot of 100 μL from diluted suspension was used to spread on YME agar plates and placed in an incubator for 5–7 days at 28°C. After incubation, YME agar plates were checked for the white cottony Streptomyces like colonies.
Antagonistic bacterial isolates were collected from agriculture field located at Lahore, Pakistan having no visible CS symptoms over the period of past 5 years. Suppressive soil samples were used to isolate Actinomycetes by serial dilution method (Wang et al., 2015). Colonies were further purified on YME agar plates (Shirling and Gottlieb, 1966). Microorganisms particularly antibiotic producing actinomycetes were targeted as promising candidate as PCS antagonistic bacteria (Kharel et al., 2010).
DNA Extraction and PCR Amplification
Selected bacterial spores were inoculated into YME broth and incubated for 3 days in shaking incubator with 180 rpm at 28°C temperature. After incubation, supernatant was separated from cell pellet by centrifugation. The cell pellet was used for genomic DNA extraction by using the FastDNA® kit (MP Biomedicals, Santa Ana, CA, United States). PCR amplification of DNA samples were performed with 16S rRNA primers (Edwards et al., 1989). PCR reaction was performed in PCR tubes with 25 μL reaction volume which included 1 μL (50ng) DNA (A260/A280 ratio was 1.9) template, 5 μL 5X PCR buffer, 0.50 μL 10 mM dNTPs, 0.50 μL 10 μM forward and reverse primers each, 0.10 5 u/μL Taq polymerase and 17.90 μL H2O. PCR reaction was programmed as, initial denaturation for 5 min at 95°C followed by 30 cycles of denaturation for 30 s at 95°C, annealing for 40 s at 60°C, extension for 40 s at 72°C and final extension was performed for 5 min at 72°C.
Identification of PCS Pathogens
Streptomyces species specific primers were used for the identification of PCS causing pathogens. Species specific primers for Streptomyces pathogens including S. scabies (Lehtonen et al., 2004), S. europaeiscabiei, S. bottropensis, S. stelliscabiei (Wanner, 2006), S. acidiscabiei, and S. turgidiscabiei (Tagawa et al., 2008) were used for the identification by PCR amplification. Amplification of 16S-23S internal transcribed spacer (ITS) sequence was performed with ITS forward and reverse primers (Song et al., 2004). PCR amplified product was digested with Hpy99I restriction enzyme which expurgated the amplicon at 1629–1633 nucleotide position.
DNA fragments were visualized under gel electrophoresis. PCR amplified product was sent to DNA sequencing facility, University of Maine for sequencing. 16S rRNA sequences were submitted to NCBI to obtain accession numbers.
Polymerase chain reaction was used to amplify PAI related genes including txtAB, nec1, and tomA (Bukhalid et al., 1998; Wanner, 2006). PCR reaction conditions were same as above except the annealing temperature was adjusted at 60°C, 55°C and 48°C for nec1, tomA, and txtAB genes, respectively.
Disk Diffusion Assay
Antagonistic Streptomyces spp. were checked against PCS pathogens by disk diffusion assay (Clinical and Laboratory Standards Institute, 2015). Pure cultures of twelve antagonistic Streptomyces spp. were prepared by inoculating few spores into 100 mL YME broth and incubated at 28°C in shaking incubator at 180 rpm for 5–7 days. The broth culture was centrifuged at 9,000 ×g and supernatant were used for preparation of methanol extract. Meanwhile, YME broth cultures of PCS pathogenic Streptomyces strains were spread on YME agar plates with RattlerTM plating beads (Zymo Research Cooperation, United States), separately. 25 μL methanolic extract of antagonistic Streptomyces were poured on filter paper disks and placed on YME- agar plates previously spread with pathogenic PCS suspension. The plates were incubated for 48–72 h at 28°C. After incubation, clear zone around filter paper disks were checked and results were recorded in mm.
Plant Growth Promotion
Twelve antagonistic Streptomyces spp. were evaluated for plant growth attributes including indole-3-acetic acid (IAA) production, phosphate solubilization, siderophores production and in vitro nitrogen fixation. IAA production was estimated by colorimetric method (Gordon and Weber, 1951; Amin and Latif, 2017) and confirmed by HPLC-DAD-MS as mentioned by Sarwar et al. (2018). The IAA production titer from antagonistic Streptomyces spp. was performed by observing optical density (O.D530nm) against standard curve of IAA and recorded in μg mL-1 (Bric et al., 1991). Phosphate solubilization was assessed by the method previously described by Sylvester-Bradley et al. (1982). Glucose yeast medium (GY) along with two solutions; one containing 10% 50 mL K2HPO4 and second solution containing 10% 100 mL CaCl2 were prepared and added in 1 L GY medium (Ambrosini et al., 2012). The medium was autoclaved and poured into petri plates after cooling. The addition of two solutions made an opaque insoluble layer of CaCl2. The plates were inoculated with antagonistic Streptomyces isolates, separately and incubated for 7 days at 28°C. After incubation, inhibition zone was observed and recorded.
Siderophores production was checked by inoculating bacterial spores on chrome azurol S (CAS) agar plates as mentioned by Schwyn and Neilands (1987). After the incubation of 5–7 days at 28°C, development of yellow to orange color was observed.
Nitrogen fixation potential of antagonistic Streptomyces spp. was examined by acetylene reduction assay (ARA) as described by Rice and Paul (1971). Nitrogen free mannitol (NFM) medium (Doty et al., 2009) slants were prepared in glass tubes and inoculated with antagonistic Streptomyces spp., separately. The tubes were sealed with a stopper and head space was filled with 2% oxygen. About 10% head space was exchanged with equal amount of acetylene. The tubes were placed in an incubator at 28°C for 2 weeks. Reduction of acetylene to ethylene was measured by gas chromatography (Agilent technologies 7890A GC system), which was equipped with flame ionizing detector and Agilent CP7348 column (25m × 0.25mm). As a positive control, two bacterial strains belonged Bacillus amyloliquefaciens (ZM2; accession number JX185642) and Pseudomonas aerouginosa (ZS24; accession number JQ990311) were used (kindly provided as positive control by Dr. ZL, University of the Punjab, Pakistan).
Extraction and Analysis of Bioactive Compounds by HPLC-DAD-MS
Antagonistic Streptomyces strains were inoculated in 150 mL YME broth in a 500 mL shaking flask. The flasks were incubated for 3 days at 28°C in an incubator shaker with 180 rpm. After incubation, the culture was centrifuged, pellet was discarded, and supernatant was undergone twice extraction with equal amount of ethyl-acetate. The extract was concentrated in-vacuo and re-suspended in methanol. For the HPLC-DAD-MS analysis, Agilent 1100 system was used equipped with a XBridge C-18 (3.5 mm, 100 mm × 4.6 mm) reverse phase column, a diode array detector and a quadrupole mass detector. An aliquot of 20 μL diluted crude extract was injected into the HPLC system and eluted isocratically with 95:5 methanol/water at a 0.5 mL min-1 flow rate.
Purification and Structural Elucidation of Azalomycin
Culture of S. violaceusniger strain AC12AB (100 mL) was used to inoculate in 10 L YME broth at 28°C for 5 days in an incubator shaker with 150 rpm. After incubation, the culture was sonicated for half an hour. The culture was then centrifuged at 11,200 ×g, supernatant was used for extraction with equal amount of ethyl acetate. The ethyl acetate extract was concentrated in-vacuo and powdered extract was re-suspended in methanol. The methanolic extract was used for thin layer chromatography (TLC) and silica gel column chromatography with 5:1 dichloromethane and methanol buffer system. All the fractions were analyzed for their biological activity against S. scabies and most active fraction was further purified by Sephadex LH-20 column chromatography system with methanol as mobile phase. The fraction was analyzed by HPLC-DAD-MS system and further purification was performed by SPE Oasis® HLB20 35 cc cartridge (6 g). Fractions were eluted in SPE column with step gradient (20–100%) of methanol. Purified fraction was obtained after final purification with semi-preparative HPLC.
Final purification was achieved with help of semi-preparative HPLC system (Agilent 1100 Series). In HPLC system, as a stationary phase Zorbax B-C C18 (9.4 mm × 20 mm) main column and Zorbax B-C18 (9.4 mm × 150 mm) pre-column was used. The compound was eluted with buffer A (acetonitrile/acetic acid 0.5%) and buffer B (water/acetic acid 0.5%) with 2 mL min-1. Methanolic extract purified from SPE Oasis® HLB20 35 cc cartridge was spiked on the column. 6mg purified azalomycin obtained from semi-preparative HPLC system, was dissolved in CD3OD and analyzed for one dimensional NMR including 1H (400 MHZ) and 13C-NMR (100 MHZ) and 2-D NMR including HMQC, 1H/1H-COSY and HMBC on a Bruker DRX-500 NMR spectroscopy (Bruker, Karlsruhe, Germany).
Plant Growth Promotion and Pathogenicity Assay on Potato Tubers
Greenhouse Assay
Pathogenicity assay on tubers was performed in greenhouse assay (Wanner, 2006). From eighteen isolates of pathogenic Streptomyces spp., two isolates namely S. scabies strain AJ-7 (Accession number MG725948.1) and AJ-10 were selected whereas S. violaceusniger strain AC12AB (Accession number MH388022.1) was used as antagonistic and plant growth promoter strain. As a positive control PCS pathogenic S. scabies strain AC-46 (Accession number KU560917.1) was also used. To observe normal growth pattern, tubers were also inoculated without any bacterial inoculation. The greenhouse assay was performed twice at greenhouse facility, University of Maine, Orono, United States during 2016-2017. Pathogenic S. scabies AJ-7, AJ-10, AC-46, and antagonistic S. violaceusniger AC12AB were cultivated separately in YME broth for 3–5 days at 28°C in incubator with shaking at 150 rpm, until they attained 106 CFU mL-1 conc. After incubation, cultures were separately centrifuged at 9,000 ×g. Supernatant was discarded, and bacterial cell mass was re-suspended in sterile distilled water to prepare inocula with 106 CFU mL-1 conc. Pots were filled with compo Sana Universal® (Munster, Germany). Tubers were surface sterilized with 5% NaOCl for 5–10 min and washed with sterilized water. Washed tubers were sown into respective five pots as replicates. After 2–3 weeks of sowing, pots were inoculated by drenching with 100 mL bacterial suspension. The average temperature was maintained between 25 and 28°C. The plants were kept hydrated and continuously monitored for the increase in shoot, root length, tuber weight and decrease in PCS symptoms for 3 months. After harvesting, potato tubers were evaluated against growth and disease parameters. The results were recorded and pathogenic Streptomyces spp. was re-isolated from CS infected tubers to confirm the source of CS infection.
Field Trial
Field trial was conducted to determine disease suppression and plant growth promotion of antagonistic S. violaceusniger AC12AB. Field trial was conducted in a field available at University of the Punjab, Lahore Pakistan. Indigenous CS pathogen S. scabies AC-46 and antagonistic S. violaceusniger AC12AB were used. Inoculum of pathogenic and antagonistic strains were prepared as mentioned above. Disease free tubers (cv. Berna; Purchased from Punjab Seed Corporation, Pakistan) were disinfected with 5% NaOCl and washed with sterile water. Tubers were sown in a randomized complete block design in duplicates.
Each block (62 square feet) contained four rows; the length of each row was 1.5 m with 2.5 m distance between each block. Six potato seeds were implanted into each row. After 2–3 weeks of plantation, bacterial spore suspension (prepared as described above) was drenched 20–30 cm deep into the plant’s roots. Plants were watered as required under natural light and temperature. Plants were monitored for growth in shoots and roots length. After harvesting, tubers were evaluated for decrease in CS symptoms, increase in tuber weight and yield acre-1 were recorded.
Statistical Analysis
All the experiments were performed in triplicates and P < 0.05 was considered as statistically significant. The results were subject to one-way analysis of variance (ANOVA) and compared means were separated by Tukey’s test. Statistical analysis was performed by using SPSS software (IBM SPSS Statistics, version 21).
Results
Identification and Molecular Characterization of Streptomyces Causing PCS
Eighteen bacterial isolates were analyzed by PCR amplification of 16S rRNA gene, out of which, all isolates were confirmed as Streptomyces. Streptomyces isolates were further identified by PCR amplification with using species specific primers, PCR amplification of 16-23S ITS region with ITS primers and digesting the amplicon with Hpy99I enzyme. After RFLP pattern analysis, all eighteen Streptomyces isolates were found to belong S. scabies (Flores-González et al., 2008).
PCR was also performed to identify the pathogenicity-related genes in Streptomyces isolates, which were subject to PCR amplification of txtAB, nec1 and tomA genes. All Streptomyces isolates were found to contain txtAB, nec1, and tomA genes. Antagonistic bacterial isolates were also screened for txtAB, nec1 and tomA genes; but, did not test positive for those genes which showed that antagonistic bacterial isolates do not produce thaxtomin A and are non-pathogenic. Among antagonistic bacterial isolates, one bacterial strain AC12AB was identified as S. violaceusniger after 16S rRNA analysis with MH388022.1 accession number.
Antagonistic Potential and Isolation of Azalomycin From Streptomyces violaceusniger AC12AB
Disk diffusion assay was performed to determine the antibacterial potential of antagonistic Streptomyces isolates. The bacterial extract from S. violaceusniger AC12AB had high inhibitory activity (18 mm) against S. scabies (AJ-7) (Figure 1 and Supplementary Table S1).
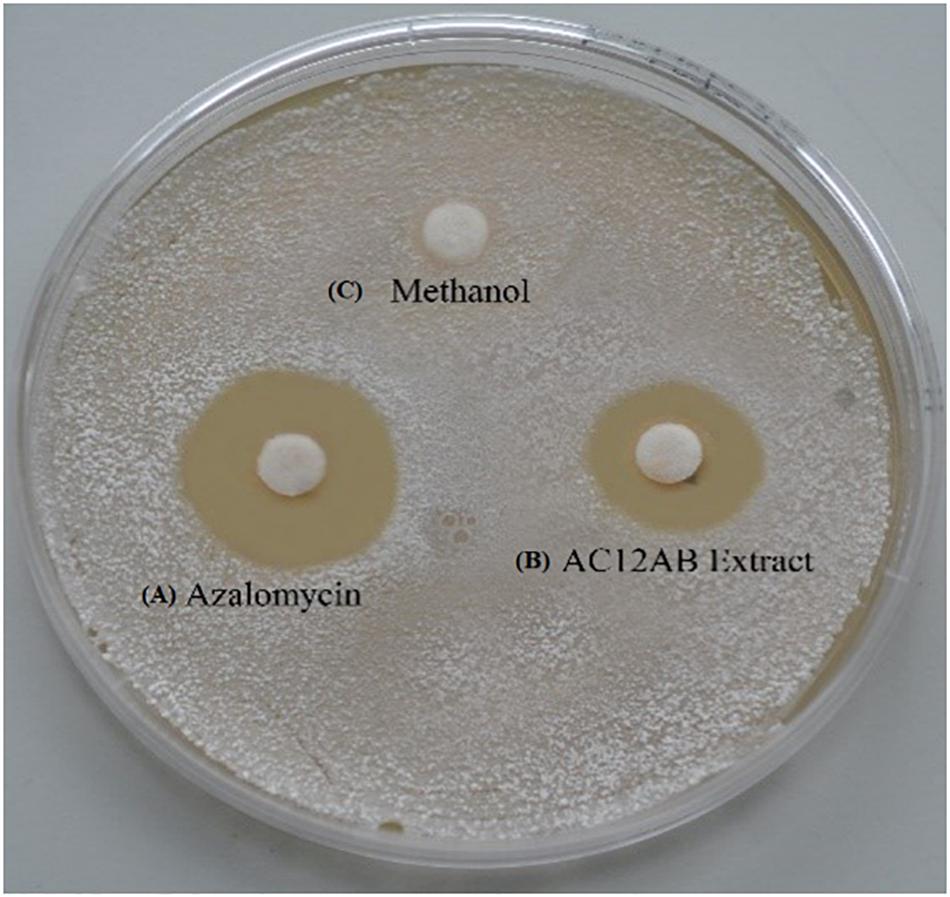
Figure 1. Inhibition of S. scabies (AJ-7) by disk diffusion assay. Streptomyces scabies (AJ-7) was grown on YME agar plates with filter paper disks containing (A) purified azalomycin dissolved in methanol; (B) Streptomyces violaceusniger AC12AB crude extract; (C) methanol only.
Plant Growth Promoting Potential of Streptomyces violaceusniger AC12AB
Streptomyces violaceusniger AC12AB was analyzed to produce plant growth promoting attributes including IAA, phosphate solubilization, siderophores production and in vitro nitrogen fixation. With colorimetric method, the highest potential of IAA production was estimated in case of S. violaceusniger AC12AB as 17 μgmL-1 after 4 days of incubation at 28°C. Production of IAA was also confirmed by HPLC analysis (Figure 2). From twelve antagonistic bacterial strains, three antagonistic Streptomyces isolates (Streptomyces A1RT, S. violaceusniger AC12AB and Streptomyces A-1; data not shown except S. violaceusniger AC12AB) were positive for siderophores production by producing blue color around bacterial cultures in NFM medium (Supplementary Figure S1). Phosphate solubilization test was performed with antagonistic bacterial strains and only S. violaceusniger AC12AB and Streptomyces A1RT exhibited clear zone around bacterial colonies (Supplementary Figure S2). ARA was performed against antagonistic bacterial isolates. The maximum value for ARA 4351.0 nMole/24h was recorded from S. violaceusniger AC12AB. However, 2278 and 1549 nMole/24h ethylene production were estimated from Streptomyces A1RT and Streptomyces A-1 strains, respectively (Supplementary Table S2).
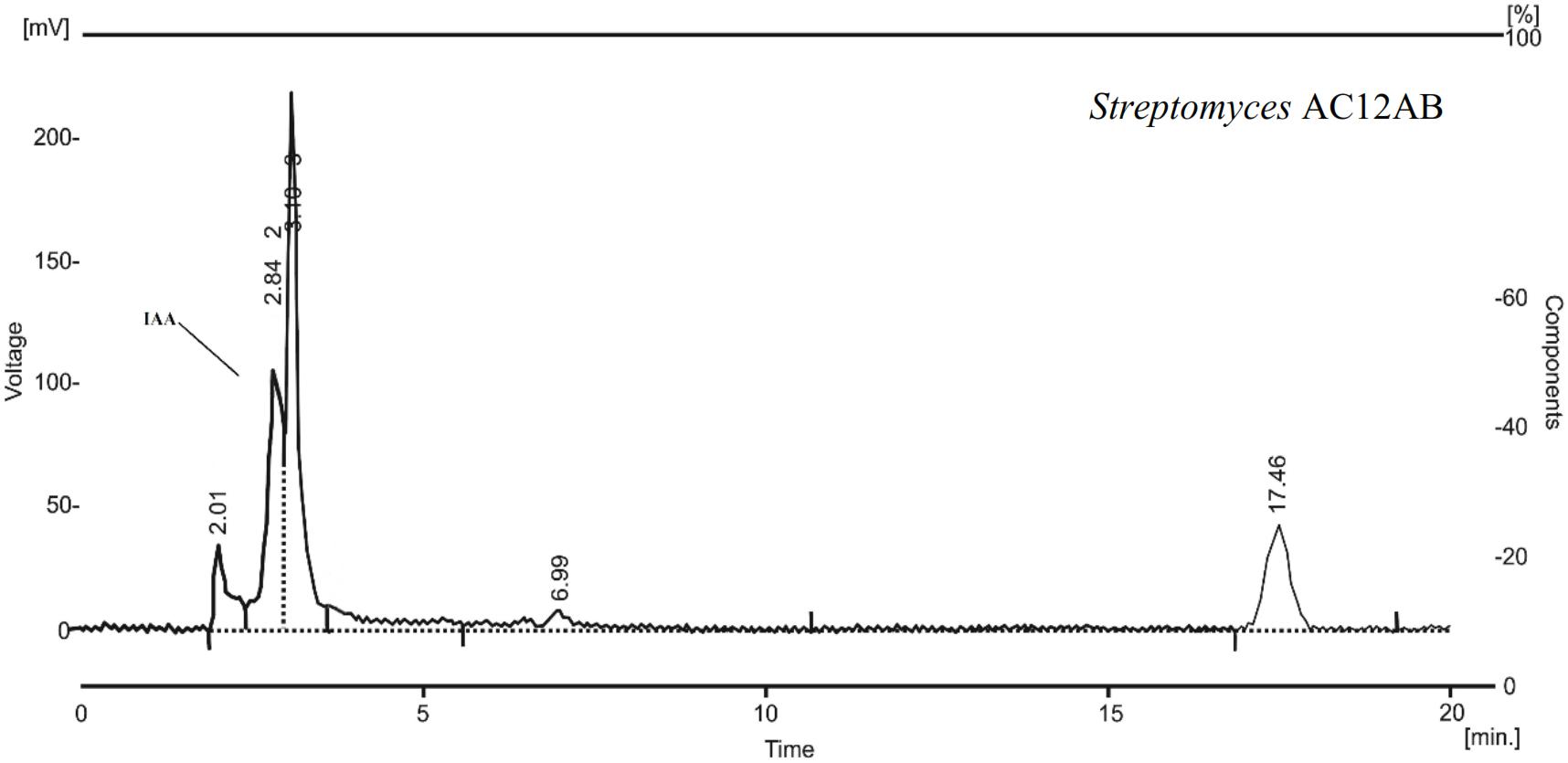
Figure 2. HPLC chromatogram of S. violaceusniger AC12AB showing indole-3-acetic acid (IAA) peak at 2.84 min retention time.
Purification and Structural Elucidation of Bioactive Compound
Through a targeted mass fractionation, a white colored amorphous powder was purified having strong antibacterial activity against S. scabies. The molecular mass of the compound was predicted to be C54H91N3O17 based on observed molecular ion [M-H]-; m/z 1054.5 (Supplementary Figure S3). Analysis of one-dimensional NMR (1H and 13C NMR spectra) indicated ten olefinic carbons, twelve oxy-methine and one quaternary hemiacetal carbon (Supplementary Figures S4, S5). Analysis of 2D-NMR revealed the characteristic guanidine carbon (Supplementary Figures S6–S8 and Supplementary Table S3). The absorbance spectrum of compound (Supplementary Figure S9) exhibited distinct maxima 250–300 nm closely related to azalomycin (Figure 3) analog RS-22A (Ubukata et al., 1995).
Plant Growth Promotion and PCS Disease Suppression Under Greenhouse and Field Conditions
In greenhouse assay, pathogenic S. scabies isolates (AJ-7, AJ-10, and AC46) caused CS lesions on potato tubers (Figures 4A,B and Table 1). The inoculation with antagonistic S. violaceusniger AC12AB significantly reduced DS index (P < 0.05) (Table 1). There were 47, 24.6, and 41% increases in shoot length, root length and tuber weight, respectively, when S. scabies AJ10 was used in combination with S. violaceusniger AC12AB (P < 0.05) (Figures 5, 6). Field trial using S. violaceusniger AC12AB revealed 83% disease reduction (Figures 4C,D and Table 1), 26.8% yield increase (Table 1), and significant (P < 0.05) increase in plant growth attributes (including increase in shoot/root length, number of tubers and tuber weight) (Figures 5, 6).
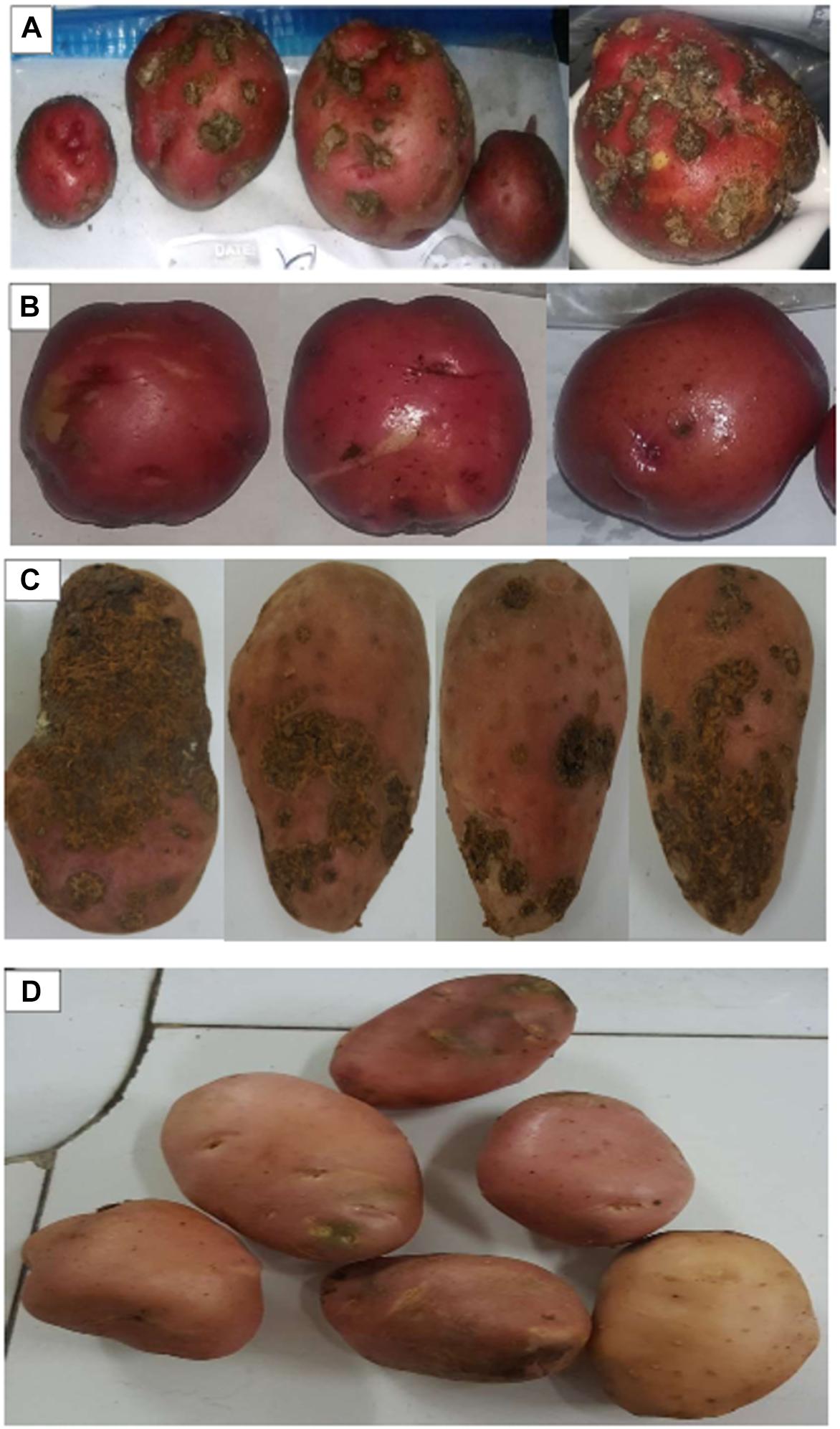
Figure 4. Tubers harvested from greenhouse assay and field trial. (A) Tubers harvested from after inoculation with S. scabies AJ-7 in greenhouse assay. (B) Tubers harvested after inoculation with S. scabies AJ-7 + S. violaceusniger AC12AB in greenhouse assay. (C) Tubers harvested after inoculation with S. scabies AC-46 in field trial. (D) Tubers harvested after inoculation with S. scabies AC-46 + S. violaceusniger AC12AB in field trial.
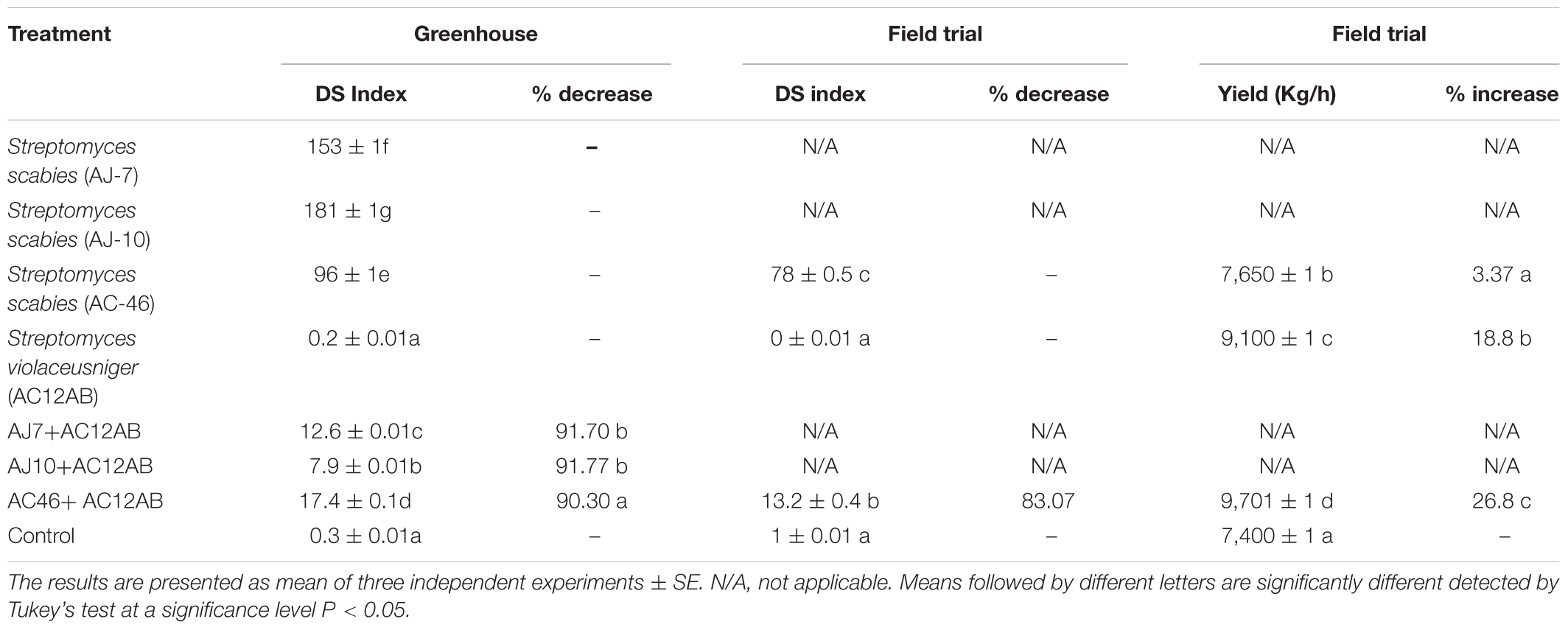
Table 1. Effect of growth promoting Streptomyces on potato (Solanum tuberosum) grown under greenhouse at the University of Maine, Maine, United States and field conditions in University of the Punjab, Lahore, Pakistan.
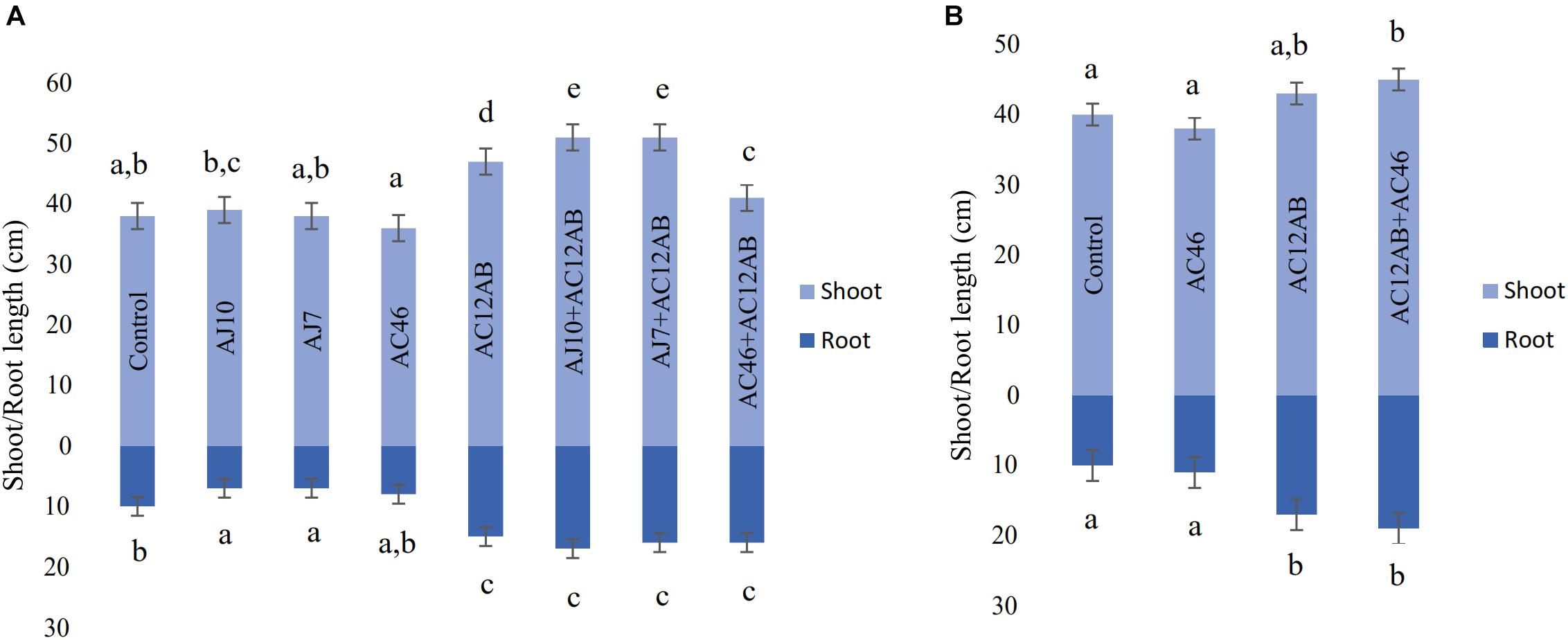
Figure 5. Effect of S. violaceusniger AC12AB on potato growth. (A) Root and shoot growth in greenhouse trial. (B) Root and shoot growth in field trial. Error bars representing ± SE. Measurement was represented by mean ± SE of triplicates. Means followed by different letters show significant differences detected by Tukey’s test at a significance level P < 0.05.
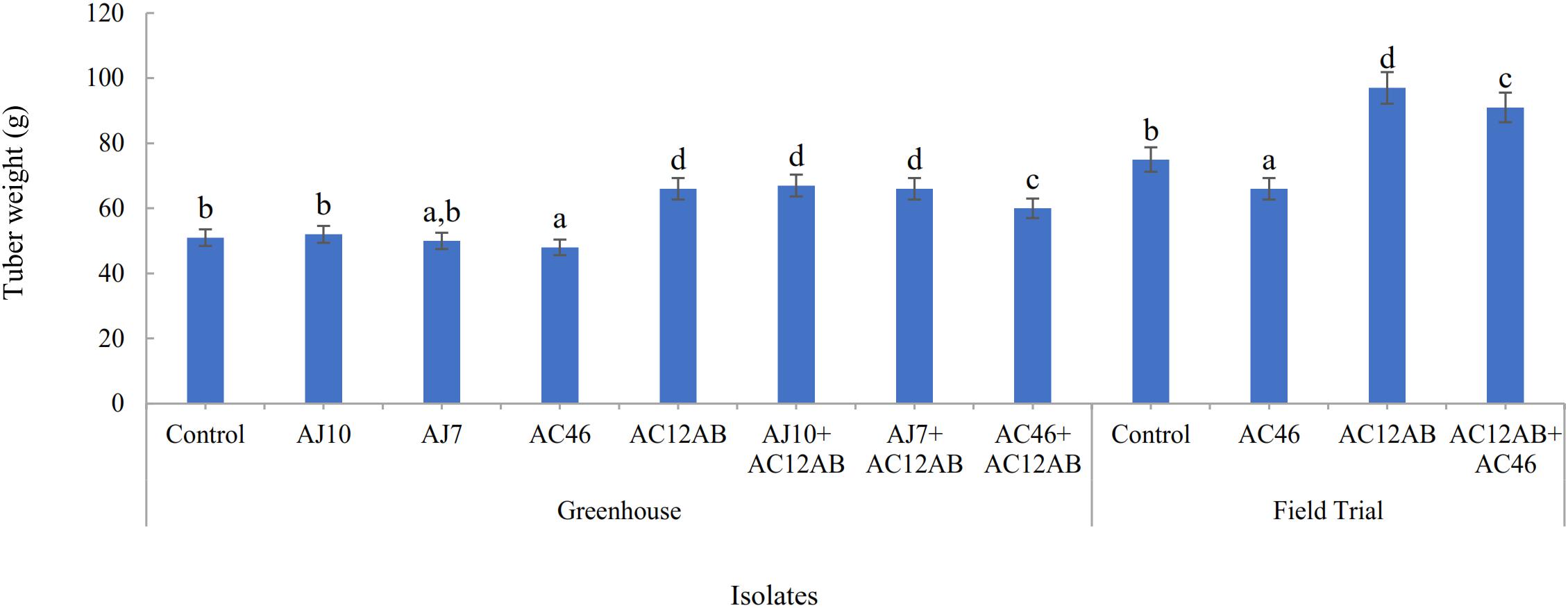
Figure 6. Effect of S. violaceusniger AC12AB on potato tuber, which were inoculated with one of S. scabies isolates (AJ10, AJ7, and AC46) in greenhouse and field trials. Statistical analysis for greenhouse and field trial was calculated separately. Tuber weight was represented by mean ± SE of triplicates. Means followed by different letters show significant differences detected by Tukey’s test at a significance level P < 0.05.
Discussion
In this research, S. violaceusniger AC12AB was assessed for its efficacy in suppressing CS disease and plant growth promotion in potato crop. The results indicated that although S. violaceusniger AC12AB application reduced the PCS disease up to 83%, their efficacy of plant growth promotion in field trial varied as compared to greenhouse assay.
Field soil is a complex environment that contains multiple factors which are difficult to control. CS development is not only dependent upon bacterial inoculum, but also by other physical and biological factors, including soil condition, irrigation strategy, plant variety, and weather conditions (Lazarovits et al., 2007). In the current study, field trial was conducted in the soil, and the average disease severity index was recorded as 1.1 (in the control). Moreover, dry and hot weather conditions of Pakistan may favor the development of CS infections in the tubers. All these factors could affect the disease outcome and may hinder the disease management under natural conditions. Previously, disease management remained dependent upon the use of chemical pesticides (Hvězdová et al., 2018), maintaining high soil moisture level (Powelson and Rowe, 2008), use of resistant cultivars (Dees and Wanner, 2012) and crop rotation (Larkin et al., 2011). Biological control agents have been extensively studied to control plant pathogens and simultaneously reducing environmental pollution and ecological distribution due to the irrational use of pesticides in fumigation.
Eckwall and Schottel (1997) used Streptomyces diastatochromogenes PonSSII as biocontrol agent against PCS by demonstrating antibiosis and competition mechanism. Similarly, Han et al. (2005) and Singhai et al. (2011) used Bacillus sp. sunhua and Pseudomonas spp. to control CS infections, respectively. Moreover, antimicrobial agents from bacterial spp. such as macrolactin A, iturin A, surfactin, bacillaene, fengycin, isatropolone C and difficidin (Schneider et al., 2007; Chowdhury et al., 2015; Lin et al., 2018; Sarwar et al., 2018) have been used against plant pathogens. In this study, we have identified a novel plant growth promoting S. violaceusniger AC12AB, which was confirmed to be an effective and inexpensive method to control PCS and simultaneously enhance the crop yield. PCS management remains unsolved as there is lack of chemical products. In this study, S. violaceusniger AC12AB exhibited strong antibacterial activity against S. scabies. Further analysis by NMR revealed that the main bioactive compound produced by S. violaceusniger AC12AB was azalomycin RS-22A, which has been previously used as a broad-spectrum antibiotic, antifungal and also as a moderate antitumor agent (Cheng et al., 2010; Yuan et al., 2013). To our knowledge, this is the first report of using S. violaceusniger AC12AB producing azalomycin as biological control agent in an agriculture system.
For a successful biocontrol agent, it is important to acquire root colonization ability so that the secondary metabolites produced by microorganisms would be available to the plant roots system (Johnston-Monje and Raizada, 2011; Larkin et al., 2011). Plant growth promoting Streptomyces (PGPS) are important microorganisms to develop a successful beneficial interaction between plants and microbes in a rhizoplane. PGPS are preferred over other plant growth promoting bacteria due to their enhanced colonization ability, their effect as biofertilization, biostimulation, and bioprotection (Saharan and Nehra, 2011; Rajput et al., 2013; Jog et al., 2014; Qiao et al., 2014). In current study, we demonstrated that S. violaceusniger AC12AB had the ability to produce plant growth hormone IAA along with its ability to produce siderophores and solubilize phosphates. Therefore, potato tubers treated with S. violaceusniger AC12AB developed in terms of root and shoot growth, tuber weight and better yield.
Results of greenhouse and field trials showed up to 90 and 80%, respectively, decrease in CS disease severity was observed when potato tubers inoculated with S. violaceusniger AC12AB. These results coupled with agar plate assay may explain the role of azalomycin as an antagonistic agent against PCS pathogens. Moreover, more than 25% increased yield was observed which could be attributed to the enhanced colonization ability of Streptomyces, production of plant growth hormones, siderophores, nitrogen fixation, and phosphate solubilization potential. Application of this type of bacteria will greatly enhance the production of potato and profit, which is especially important in developing countries.
Author Contributions
AS conducted all experimental work. ZL assisted with project development and data analysis. SZ and AB assisted in performing NMR analysis. JH assisted in manuscript writing.
Funding
This research was supported by the Higher Education Commission, Government of Pakistan (IRSIP-1-8/HEC/HRD/2016/5915) and USDA National Institute of Food and Agriculture Hatch/Multi-state Projects ME031906 and ME021508. We gratefully acknowledge the Robert S. McNamara World Bank fellowship for providing visiting and research opportunity to United States.
Conflict of Interest Statement
The authors declare that the research was conducted in the absence of any commercial or financial relationships that could be construed as a potential conflict of interest.
Acknowledgments
We gratefully acknowledge University of Maine Orono, ME, United States, University of the Punjab, and University of Central Punjab, Lahore, Pakistan for providing greenhouse and field trial facilities. Thanks to University of Freiburg, Germany for providing access to NMR facility.
Supplementary Material
The Supplementary Material for this article can be found online at: https://www.frontiersin.org/articles/10.3389/fmicb.2019.00202/full#supplementary-material
References
Al-Mughrabi, K. I., Vikram, A., Poirier, R., Jayasuriya, K., and Moreau, G. (2016). Management of common scab of potato in the field using biopesticides, fungicides, soil additives, or soil fumigants. Biocontrol Sci. Technol. 26, 125–135. doi: 10.1080/09583157.2015.1079809
Ambrosini, A., Beneduzi, A., Stefanski, T., Pinheiro, F. G., Vargas, L. K., and Passaglia, L. M. P. (2012). Screening of plant growth promoting rhizobacteria isolated from sunflower (Helianthus annuus L.). Plant Soil 356, 245–264. doi: 10.1007/s11104-011-1079-1
Amin, A., and Latif, Z. (2017). Screening of mercury-resistant and indole-3-acetic acid producing bacterial-consortium for growth promotion of Cicer arietinum L. J. Basic Microbiol. 57, 204–217. doi: 10.1002/jobm.201600352
Arseneault, T., Goyer, C., and Filion, M. (2015). Pseudomonas fluorescens lbum223 increases potato yield and reduces common scab symptoms in the field. Phytopathology 105, 1311–1317. doi: 10.1094/Phyto-12-14-0358-R
Barry, S. M., Kers, J. A., Johnson, E. G., Song, L., Aston, P. R., Patel, B., et al. (2012). Cytochrome P450-catalyzed L-tryptophan nitration in thaxtomin phytotoxin biosynthesis. Nat. Chem. Biol. 8, 814–816. doi: 10.1038/nchembio.1048
Bric, J. M., Bostock, R. M., and Silverstone, S. E. (1991). Rapid in situ assay for indoleacetic acid production by bacteria immobilized on a nitrocellulose membrane. Appl. Environ. Microbiol. 57, 535–538.
Bukhalid, R. A., Chung, S. Y., and Loria, R. (1998). nec1, a gene conferring a necrogenic phenotype, is conserved in plant-pathogenic Streptomyces spp. and linked to a transposase pseudogene. Mol. Plant Microbe Interact. 11, 960–967. doi: 10.1094/mpmi.1998.11.10.960
Cheng, J., Yang, S. H., Palaniyandi, S. A., Han, J. S., Yoon, T.-M., Kim, T.-J., et al. (2010). Azalomycin F complex is an antifungal substance produced by Streptomyces malaysiensis MJM1968 isolated from agricultural soil. J. Korean Soc. Appl. Biol. Chem. 53, 545–552. doi: 10.3839/jksabc.2010.084
Chowdhury, S. P., Hartmann, A., Gao, X., and Borriss, R. (2015). Biocontrol mechanism by root-associated Bacillus amyloliquefaciens FZB42 - a review. Front. Microbiol. 6:780. doi: 10.3389/fmicb.2015.00780
Clinical and Laboratory Standards Institute (2015). Performance Standards for Antimicrobial Susceptibility Testing: Twenty-Fifth Information Supplement M100-S25 (Wayne, PA: Clinical and Laboratory Standards Institute).
Dees, M., and Wanner, L. (2012). In search of better management of potato common scab. Potato Res. 55, 249–268. doi: 10.1007/s11540-012-9206-9
Doty, S. L., Oakley, B., Xin, G., Kang, J. W., Singleton, G., Khan, Z., et al. (2009). Diazotrophic endophytes of native black cottonwood and willow. Symbiosis 47, 23–33. doi: 10.1007/BF03179967
Eckwall, E. C., and Schottel, J. L. (1997). Isolation and characterization of an antibiotic produced by the scab disease-suppressive Streptomyces diastatochromogenes strain PonSSII. J. Ind. Microbiol. Biotechnol. 19, 220–225. doi: 10.1038/sj.jim.2900455
Edwards, U., Rogall, T., Blocker, H., Emde, M., and Bottger, E. C. (1989). Isolation and direct complete nucleotide determination of entire genes. Characterization of a gene coding for 16S ribosomal RNA. Nucleic Acids Res. 17, 7843–7853. doi: 10.1093/nar/17.19.7843
Flores-González, R., Velasco, I., and Montes, F. (2008). Detection and characterization of Streptomyces causing potato common scab in Western Europe. Plant Pathol. 57, 162–169. doi: 10.1111/j.1365-3059.2007.01734.x
Gordon, S. A., and Weber, R. P. (1951). Colorimetric estimation of indoleacetic acid. Plant Physiol. 26, 192–195. doi: 10.1104/pp.26.1.192
Han, J. S., Cheng, J. H., Yoon, T. M., Song, J., Rajkarnikar, A., Kim, W. G., et al. (2005). Biological control agent of common scab disease by antagonistic strain Bacillus sp. sunhua. J. Appl. Microbiol. 99, 213–221. doi: 10.1111/j.1365-2672.2005.02614.x
Healy, F. G., Krasnoff, S. B., Wach, M., Gibson, D. M., and Loria, R. (2002). Involvement of a cytochrome P450 monooxygenase in thaxtomin A biosynthesis by Streptomyces acidiscabies. J. Bacteriol. 184, 2019–2029. doi: 10.1128/jb.184.7.2019-2029.2002
Healy, F. G., Wach, M., Krasnoff, S. B., Gibson, D. M., and Loria, R. (2000). The txtAB genes of the plant pathogen Streptomyces acidiscabies encode a peptide synthetase required for phytotoxin thaxtomin A production and pathogenicity. Mol. Microbiol. 38, 794–804. doi: 10.1046/j.1365-2958.2000.02170.x
Hvězdová, M., Kosubová, P., Košíková, M., Scherr, K. E., Šimek, Z., Brodský, L., et al. (2018). Currently and recently used pesticides in Central European arable soils. Sci. Total Environ. 613, 361–370. doi: 10.1016/j.scitotenv.2017.09.049
Jog, R., Pandya, M., Nareshkumar, G., and Rajkumar, S. J. M. (2014). Mechanism of phosphate solubilization and antifungal activity of Streptomyces spp. Isolated from wheat roots and rhizosphere and their application in improving plant growth. Microbiology 160, 778–788. doi: 10.1099/mic.0.074146-0
Johnston-Monje, D., and Raizada, M. J. C. B. (2011). Plant and Endophyte Relationships: Nutrient Management, 2nd Edn. Burlington, NJ: Academic,713–727.
Joshi, M. V., Bignell, D. R., Johnson, E. G., Sparks, J. P., Gibson, D. M., and Loria, R. (2007). The AraC/XylS regulator TxtR modulates thaxtomin biosynthesis and virulence in Streptomyces scabies. Mol. Microbiol. 66, 633–642. doi: 10.1111/j.1365-2958.2007.05942.x
Kemung, H. M., Tan, L. T.-H., Khan, T. M., Chan, K.-G., Pusparajah, P., Goh, B.-H., et al. (2018). Streptomyces as a prominent resource of future Anti-MRSA Drugs. Front. Microbiol. 9:2221. doi: 10.3389/fmicb.2018.02221
Kers, J. A., Cameron, K. D., Joshi, M. V., Bukhalid, R. A., Morello, J. E., Wach, M. J., et al. (2005). A large, mobile pathogenicity island confers plant pathogenicity on Streptomyces species. Mol. Microbiol. 55, 1025–1033. doi: 10.1111/j.1365-2958.2004.04461.x
Kers, J. A., Wach, M. J., Krasnoff, S. B., Widom, J., Cameron, K. D., Bukhalid, R. A., et al. (2004). Nitration of a peptide phytotoxin by bacterial nitric oxide synthase. Nature 429, 79–82. doi: 10.1038/nature02504
Kharel, M. K., Shepherd, M. D., Nybo, S. E., Smith, M. L., Bosserman, M. A., and Rohr, J. (2010). Isolation of Streptomyces Species from Soil. Curr. Protoc. Microbiol. 19, 10E.4.1–10E.4.5. doi: 10.1002/9780471729259.mc10e04s19
King, R. R., Lawrence, C. H., Clark, M. C., and Calhoun, L. A. (1989). Isolation and characterization of phytotoxins associated with Streptomyces scabies. J. Chem. Soc., Chem. Commun. 13, 849–850. doi: 10.1094/MPMI-09-14-0255-R
Lambert, D. H., and Loria, R. (1989). Streptomyces scabies sp. nov., nom. rev.†. Int. J. Syst. Evol. Microbiol. 39, 387–392. doi: 10.1099/00207713-39-4-387
Larkin, R. P., Honeycutt, C. W., Griffin, T. S., Olanya, O. M., Halloran, J. M., and He, Z. J. P. (2011). Effects of different potato cropping system approaches and water management on soilborne diseases and soil microbial communities. Phytopathology 101, 58–67. doi: 10.1094/PHYTO-04-10-0100
Lawrence, C., Clark, M., and King, R. (1990). Induction of common scab symptoms in aseptically cultured potato tubers by the vivotoxin, thaxtomin. Phytopathology 80, 606–608. doi: 10.1094/Phyto-80-606
Lazarovits, G., Hill, J., Patterson, G., Conn, K. L., and Crump, N. S. (2007). Edaphic soil levels of mineral nutrients, pH, organic matter, and cationic exchange capacity in the geocaulosphere associated with potato common scab. Phytopathology 97, 1071–1082. doi: 10.1094/phyto-97-9-1071
Lehtonen, M. J., Rantala, H., Kreuze, J. F., Bång, H., Kuisma, L., Koski, P., et al. (2004). Occurrence and survival of potato scab pathogens (Streptomyces species) on tuber lesions: quick diagnosis based on a PCR-based assay. Plant Pathol. 53, 280–287. doi: 10.1111/j.0032-0862.2004.01009.x
Lin, C., Tsai, C.-H., Chen, P.-Y., Wu, C.-Y., Chang, Y.-L., Yang, Y.-L., et al. (2018). Biological control of potato common scab by Bacillus amyloliquefaciens Ba01. PLoS One 13:e0196520. doi: 10.1371/journal.pone.0196520
Loria, R., Bignell, D. R., Moll, S., Huguet-Tapia, J. C., Joshi, M. V., Johnson, E. G., et al. (2008). Thaxtomin biosynthesis: the path to plant pathogenicity in the genus Streptomyces. Antonie Van Leeuwenhoek 94, 3–10. doi: 10.1007/s10482-008-9240-4
Loria, R., Kers, J., and Joshi, M. (2006). Evolution of plant pathogenicity in Streptomyces. Ann. Rev. Phytopathol. 44, 469–487. doi: 10.1146/annurev.phyto.44.032905.091147
Meng, Q., Hanson, L. E., Douches, D., and Hao, J. J. (2013). Managing scab diseases of potato and radish caused by Streptomyces spp. using Bacillus amyloliquefaciens BAC03 and other biomaterials. Biol. Control 67, 373–379. doi: 10.1016/j.biocontrol.2013.09.009
Park, D. H., Yu, Y. M., Kim, J. S., Cho, J. M., Hur, J. H., and Lim, C. K. (2003). Characterization of streptomycetes causing potato common scab in Korea. Plant Dis. 87, 1290–1296. doi: 10.1094/PDIS.2003.87.11.1290
Powelson, M., and Rowe, R. (2008). “Managing diseases caused by seedborne and soilborne fungi and fungus-like pathogens,” in Potato Health Management, ed. D. A. Johnson (St. Paul, MN: APS Press), 183–195.
Qiao, J.-Q., Wu, H.-J., Huo, R., Gao, X.-W., Borriss, R. J. C., and Agriculture, B. T. I. (2014). Stimulation of plant growth and biocontrol by Bacillus amyloliquefaciens subsp. Plantarum FZB42 engineered for improved action. Chem. Biol. Technol. Agric. 1:12. doi: 10.1186/s40538-014-0012-2
Rajput, M. S., Kumar, G. N., and Rajkumar, S. (2013). Repression of oxalic acid-mediated mineral phosphate solubilization in rhizospheric isolates of Klebsiella pneumoniae by succinate. Arch. Microbiol. 195, 81–88. doi: 10.1007/s00203-012-0850-x
Rice, W., and Paul, E. (1971). The acetylene reduction assay for measuring nitrogen fixation in waterlogged soil. Can. J. Microbiol. 17, 1049–1056. doi: 10.1139/m71-166
Saharan, B., and Nehra, V. (2011). Plant growth promoting rhizobacteria: a critical review. Life Sci. Med. Res. 2011:LSMR–21.
Sarwar, A., Latif, Z., Zhang, S., Zhu, J., Zechel, D. L., and Bechthold, A. (2018). Biological control of potato common scab with rare isatropolone C compound produced by plant growth promoting Streptomyces A1RT. Front. Microbiol. 9:1126. doi: 10.3389/fmicb.2018.01126
Schneider, K., Chen, X. H., Vater, J., Franke, P., Nicholson, G., Borriss, R., et al. (2007). Macrolactin is the polyketide biosynthesis product of the pks2 cluster of Bacillus amyloliquefaciens FZB42. J. Nat. Prod. 70, 1417–1423. doi: 10.1021/np070070k
Schwyn, B., and Neilands, J. (1987). Universal chemical assay for the detection and determination of siderophores. Anal. Biochem. 160, 47–56. doi: 10.1016/0003-2697(87)90612-9
Shirling, E. B., and Gottlieb, D. (1966). Methods for characterization of Streptomyces species. Int. J. Syst. Evol. Microbiol. 16, 313–340. doi: 10.1099/00207713-16-3-313
Singhai, P. K., Sarma, B. K., and Srivastava, J. S. (2011). Biological management of common scab of potato through Pseudomonas species and vermicompost. Biol. Control 57, 150–157. doi: 10.1016/j.biocontrol.2011.02.008
Slack, S. A. (1991). A look at potato leafroll virus and potato virus Y: past, present, future. Badger Common Tater 43, 16–21.
Song, J., Lee, S.-C., Kang, J.-W., Baek, H.-J., and Suh, J.-W. (2004). Phylogenetic analysis of Streptomyces spp. Isolated from potato scab lesions in Korea on the basis of 16S rRNA gene and 16S–23S rDNA internally transcribed spacer sequences. Int. J. Syst. Evol. Microbiol. 54, 203–209. doi: 10.1099/ijs.0.02624-0
Sylvester-Bradley, R., Asakawa, N., Torraca, S. L., Magalhães, F., Oliveira, L., and Pereira, R. (1982). Levantamento quantitativo de microrganismos solubilizadores de fosfatos na rizosfera de gramíneas e leguminosas forrageiras na Amazônia. Acta Amazonica 12, 15–22. doi: 10.1590/1809-43921982121015
Tagawa, M., Tamaki, H., Manome, A., Koyama, O., and Kamagata, Y. (2008). Development of a genotyping method for potato scab pathogens based on multiplex PCR. Biosci., Biotechnol. Biochem. 72, 2324–2334. doi: 10.1271/bbb.80234
Ubukata, M., Morita, T.-I., and Osada, H. (1995). RS-22A, B and C: new macrolide antibiotics from Streptomyces violaceusniger. J. Antibiot. 48, 293–299. doi: 10.7164/antibiotics.48.293
Wang, L. Y., Xing, M. Y., Di, R., and Luo, Y. P. (2015). Isolation, identification and antifungal activities of Streptomyces aureoverticillatus HN6. J. Plant Pathol. Microb. 6:281. doi: 10.4172/2157-7471.1000281
Wanner, L. A. (2004). Field isolates of Streptomyces differ in pathogenicity and virulence on radish. Plant Dis. 88, 785–796. doi: 10.1094/PDIS.2004.88.8.785
Wanner, L. A. (2006). A survey of genetic variation in Streptomyces isolates causing potato common scab in the United States. Phytopathology 96, 1363–1371. doi: 10.1094/phyto-96-1363
Xue, D., Christenson, R., Genger, R., Gevens, A., and Lankau, R. (2018). Soil microbial communities reflect both inherent soil properties and management practices in wisconsin potato fields. Am. J. Potato Res. 95, 696–708. doi: 10.1007/s12230-018-9677-6
Keywords: Streptomyces scabies, biological control, plant growth promoting Streptomyces, antagonistic Streptomyces, potato common scab
Citation: Sarwar A, Latif Z, Zhang S, Hao J and Bechthold A (2019) A Potential Biocontrol Agent Streptomyces violaceusniger AC12AB for Managing Potato Common Scab. Front. Microbiol. 10:202. doi: 10.3389/fmicb.2019.00202
Received: 23 November 2018; Accepted: 24 January 2019;
Published: 08 February 2019.
Edited by:
Rossana Coda, University of Helsinki, FinlandReviewed by:
Raul Antonio Sperotto, University of Taquari Valley, BrazilMaria Carolina Quecine, University of São Paulo, Brazil
Copyright © 2019 Sarwar, Latif, Zhang, Hao and Bechthold. This is an open-access article distributed under the terms of the Creative Commons Attribution License (CC BY). The use, distribution or reproduction in other forums is permitted, provided the original author(s) and the copyright owner(s) are credited and that the original publication in this journal is cited, in accordance with accepted academic practice. No use, distribution or reproduction is permitted which does not comply with these terms.
*Correspondence: Andreas Bechthold, YW5kcmVhcy5iZWNodGhvbGRAcGhhcm1hemllLnVuaS1mcmVpYnVyZy5kZQ==