- 1Niehoff School of Nursing, Stritch School of Medicine, Loyola University Chicago, Maywood, IL, United States
- 2Bioinformatics Program, Loyola University Chicago, Chicago, IL, United States
- 3Department of Biology, Loyola University Chicago, Chicago, IL, United States
- 4Department of Computer Science, Loyola University Chicago, Chicago, IL, United States
- 5Department of Microbiology and Immunology, Stritch School of Medicine, Loyola University Chicago, Maywood, IL, United States
Freshwater lakes are home to bacterial communities with 1000s of interdependent species. Numerous high-throughput 16S rRNA gene sequence surveys have provided insight into the microbial taxa found within these waters. Prior surveys of Lake Michigan waters have identified bacterial species common to freshwater lakes as well as species likely introduced from the urban environment. We cultured bacterial isolates from samples taken from the Chicago nearshore waters of Lake Michigan in an effort to look more closely at the genetic diversity of species found there within. The most abundant genus detected was Pseudomonas, whose presence in freshwaters is often attributed to storm water or runoff. Whole genome sequencing was conducted for 15 Lake Michigan Pseudomonas strains, representative of eight species and three isolates that could not be resolved with named species. These genomes were examined specifically for genes encoding functionality which may be advantageous in their urban environment. Antibiotic resistance, amidst other known virulence factors and defense mechanisms, were identified in the genome annotations and verified in the lab. We also tested the Lake Michigan Pseudomonas strains for siderophore production and resistance to the heavy metals mercury and copper. As the study presented here shows, a variety of pseudomonads have inhabited the urban coastal waters of Lake Michigan.
Introduction
Bacteria plays a critical role in the ecosystems of freshwater lakes driving global biogeochemical cycles. As such, numerous surveys of bacterial communities within freshwaters have been conducted, including those examined by Newton et al. (2011) and more recent studies (e.g., Oh et al., 2011; Jones et al., 2012; Han M. et al., 2016; Hayden and Beman, 2016; Lee et al., 2016; Llorens-Marès et al., 2016; Yang et al., 2016; Linz et al., 2017; Ren et al., 2017; Salmaso et al., 2018). Differences in the bacterial communities of these lakes can vary significantly (Newton et al., 2011) as local environmental factors often play a role in structuring these communities (Hayden and Beman, 2016). The community structure of a single lake can vary over time, over the course of a single day (Jones et al., 2012) as well as over years (Linz et al., 2017). Nevertheless, many bacterial groups within freshwaters have a global distribution: Proteobacteria, Actinobacteria, Bacteroidetes, Cyanobacteria, and Verrucomicrobia (Zwart et al., 1998; Newton et al., 2011). Dominant members of these phyla include the Proteobacteria genus Acinetobacter (Lee et al., 2016), the acI cluster of Actinobacteria (Newton et al., 2007; Neuenschwander et al., 2018), and the Bacteroidetes genera Sphingobacteriales, Sediminibacterium, Fluviicola, and Flavobacterium (Salmaso et al., 2018). These communities, however, can be impacted by disruptions, such as cyanobacterial blooms (Eiler and Bertilsson, 2004; Scherer et al., 2017). Furthermore, bacteria can be introduced into a community via effluent from water treatment plants (e.g., Escherichia coli and Enterococcus) and stormwater runoff (e.g., Pseudomonas and Sphingomonas). Such introductions can include species harboring antibiotic resistance genes into the lake ecosystem (Drury et al., 2013; Marti et al., 2013; Fisher et al., 2015; Saarenheimo et al., 2017; Chu et al., 2018; Lorenzo et al., 2018).
The Great Lakes are one of the planet’s largest freshwater ecosystems (Fuller et al., 1995) and as dynamic as coastal oceans (Rao and Schwab, 2007). Not unsurprisingly, microbial surveys of the Great Lakes have found that they share common bacterial diversity with other freshwater habitats (Mueller-Spitz et al., 2009; Mou et al., 2013; Wilhelm et al., 2014; Fisher et al., 2015; Malki et al., 2015a; Newton and McLellan, 2015; Sible et al., 2015). As the Great Lakes are the source of drinking water for millions of North Americans, protection is of critical concern in the face of the many known effects of urbanization on water quality (Foley et al., 2005). This concern is perhaps most evident within Chicago, whose city and surrounding suburbs sprawl along the Lake Michigan shoreline. Lake Michigan waters are routinely monitored (Whitman and Nevers, 2008; Wong et al., 2009; Haack et al., 2013; Oster et al., 2014; Wijesinghe et al., 2015), and the incidence of illness attributed to these waters has been extensively studied (Dorevitch et al., 2011; DeFlorio-Barker et al., 2018). Aside from prompt and thorough testing, contamination via stormwater and fecal pollution has been a consistent concern for all cities along the Lake Michigan shore (Bower et al., 2005; Whitman and Nevers, 2008; Wong et al., 2009; Fisher et al., 2015; Templar et al., 2016). Despite these concerns, the study of Newton and McLellan (2015) found that, at least in the Milwaukee area, the dispersal of bacteria introduced from the urban environment was limited.
Freshwater pseudomonads may be persistent or ephemeral members of the community. Dispersal of pseudomonads from agricultural to non-agricultural environments may be the result of the water cycle (Morris et al., 2008, 2014). As a whole, pseudomonads are often not abundant within freshwater environments (Newton et al., 2011). When pseudomonads are abundant within freshwaters, their source is typically associated with storm waters (Hoadley and McCoy, 1966; Oliveri et al., 1977; Selvakumar and Borst, 2006; Fisher et al., 2015). Culture-independent, 16S rRNA gene amplification surveys of freshwaters, rarely detect pseudomonads indicative of their absence/low abundance. In our prior surveys of the Lake Michigan nearshore waters, Pseudomonas was rarely detected via 16S rRNA sequencing (Malki et al., 2015a; Sible et al., 2015). Nevertheless, diverse species of Pseudomonas have been isolated from freshwaters (Drucker and Panasyuk, 2006; Morris et al., 2008; Pietsch et al., 2017), including the Great Lakes Erie, Ontario and Superior (Bennett, 1969; Chatterjee et al., 2017). Furthermore, bacteriophage (phage) infectious of Pseudomonas species have been routinely found within Lake Michigan nearshore waters (Malki et al., 2015b; Watkins et al., 2018), thus suggesting the presence of the bacterial host. This motivated the study presented here in which Pseudomonas species found within Chicago’s nearshore waters of Lake Michigan were sequenced and characterized.
Materials and Methods
Sample Collection
Three Chicago public beaches, Loyola Beach, Montrose Beach, and 57th Street Beach, were selected for sampling of the Lake Michigan nearshore waters. These three sites are located 8.9 miles north, 6.1 miles north, and 7.5 miles south of Chicago’s city center, respectively, and include areas for swimming. In addition, Montrose Beach is home to one of Chicago’s boat marinas and a dog beach. Water samples were collected from the swimming areas of the waters at these beaches twice during the summer of 2009 – June 24 and July 14. Water samples were collected near the shore in a depth of 0.5 m using sterile 50 ml centrifuge tubes. Additionally, the Loyola Beach was revisited and sampled on June 3, 2016 after a heavy rain. In addition to collecting samples from the lake (at the same depth sampled in 2009), water samples from two shallow puddles on the beach front were collected. A soil sample was also collected from the beach (a mixed sand and soil beach) by boring a sterile 15 ml centrifuge tube. The soil sample was diluted with 500 mL phosphate buffered saline (PBS) and agitated in a shaking incubator overnight.
Sample Culture and Strain Isolation
Six different medias were used to plate the water samples and diluted soil sample collected: LB, EMB, Sulfate API, Eugonics (HiMedia Laboratories), Centrimide (HiMedia Laboratories), and Streptococcus (HiMedia Laboratories). For each collection site and date, four plates per media were prepared as follows: 100 μL of water was aliquoted and spread on 1.7% agar plate. Two plates were grown overnight at 25°C and two at 37°C. Seventy-two individual colonies were picked and resuspended in 1 mL of the same media as the plate it was isolated. The resuspended colony was grown overnight in a shaking incubator at the temperature it was previously grown (either 25 or 37°C). Using an inoculation loop, the culture was streaked on an agar plate of the same media from which it was originally isolated and incubated overnight. This process was repeated additional times until a consistent colony morphology was observed. A single colony was selected and grown in liquid culture overnight, shaking, at either 25 or 37°C. This culture was used both for producing a freezer stock [1 mL culture and 1 mL 50/50 (%v/v) glycerol stored at -80°C] and DNA extraction.
16S rRNA Gene Sequencing
DNA was extracted from liquid culture using the Mo Bio UltraClean Microbial Kit following the manufacturer’s protocol. DNA concentration was quantified using the Quibit fluorometer. To ascertain the genus and species of the environmental isolate, the 16S rRNA gene sequence was amplified using the 63f and 1387r primers (Marchesi et al., 1998). Amplicons were visualized on a 1.2% agarose gel and purified using the E.Z.N.A.® Cycle-Pure Kit. Sixty-one of the 72 colonies isolated were successfully sequenced; isolates that did not lead to successful DNA extraction and/or PCR were repeated three times before being removed from further investigation. Each amplicon was sequenced by Genewiz (New Brunswick, NJ, United States), using both primers for a 2x coverage; sequences were manually trimmed and paired in Geneious v. 11.0.5 (Biomatters, Ltd., Auckland, New Zealand). The complete amplicon was used to query the 16S ribosomal RNA sequences database via BLASTn. 16S rRNA gene sequences are listed in Supplementary Data Sheet 1.
Pseudomonas Genome Sequencing
Isolates confirmed as belonging to the genus Pseudomonas and selected for whole genome sequencing were processed as follows. For each sample, a 1.7% agar LB plate was streaked with a loop (sampling the freezer stock) and grown overnight at 37°C. A single colony was selected from this plate and grown in 1.5 mL of LB overnight, shaking, at 37°C. DNA was extracted using the Qiagen DNeasy UltraClean Microbial Kit following the manufacturer’s protocol. DNA concentration was quantified using the Quibit fluorometer. Sequencing libraries were constructed using the Nextera XT DNA Library preparation kit. The library was sequenced on the Illumina MiSeq platform using the MiSeq Reagent Kit v2 (500-cycles) at Loyola University Chicago’s Genomics Facility (Maywood, IL United States).
Genome Assembly and Annotation
Raw reads were first trimmed for quality using the tool sickle1 and then assembled by SPAdes (v3.10.1) (Bankevich et al., 2012). Contigs less than 500 nucleotides in length were removed from further consideration. Assembled contigs were then manually inspected and individually queried against the nr/nt database via megaBLAST (Supplementary Table 1). Details regarding the number of raw reads, number of contigs, and the assembly N50 scores are listed in Supplementary Table 2. The genome coverage of each assembly was calculated using BBMap’s bbwrap and pileup scripts2. Coverage values are also listed in Supplementary Table 2. Each assembly was annotated using the RAST web service with the “Classic RAST” option (Aziz et al., 2008). CRISPR/Cas detection was performed using CRISPRCasFinder using the parameters “general” and “unordered,” which allow a permissive search for CAS genes within contigs respectively (Couvin et al., 2018).
Prophage Detection and Analysis
Identification of prophage sequences within the genome assemblies was conducted using PHASTER (Arndt et al., 2016). Predicted prophage sequences were queried against the NCBI nr/nt database and compared to each other using progressiveMauve (Darling et al., 2004). Prophage sequences were annotated using the RAST web service with the “Classic RAST” option (Aziz et al., 2008). PCR primers were designed for each of the PHASTER predicted intact prophages using Primer33 and obtained from Eurofins Genomics (Louisville, KY, United States). DNA from each sample was then amplified using the primers to confirm the integration of the predicted prophages.
Phylogenetic Analyses
All of the Lake Michigan isolate 16S rRNA gene amplicon sequences were aligned using MUSCLE (Edgar, 2004). The 16S rRNA gene, gyrB, rpoB, and rpoD nucleotide sequences were retrieved from the GenBank annotations for the following strains: P. aeruginosa PAO1 (NC_002516), P. brassicacearum NFM421 (NC_015379), P. denitrificans ATCC 13867 (NC_020829), P. entomophila str. L48 (NC_008027), P. fluorescens Pf0-1 (NC_007492), P. fulva 12-X (NC_015556), P. mendocina ymp (NC_009439), P. poae RE∗1-1-14 (NC_020209), P. protogens Pf-5 (NC_004129), P. putida KT2440 (NC_002947), P. savastanoi pv. phaseolicola (NC_005773), Pseudomonas sp. UW4 (NC_019670), P. stutzeri A1501 (NC_009434), P. syringae pv. tomato str. DC3000 (NC_004578), and Cellvibrio japonicus Ueda107 (NC_010995). These gene sequences were concatenated through Geneious and aligned using Clustal Omega (Sievers et al., 2011). All phylogenetic trees were derived using FastTree (Price et al., 2010) through Geneious and visualized in iTOL (Letunic and Bork, 2016).
Average nucleotide identity (ANI) was computed for the 15 sequenced Pseudomonas genomes, the aforementioned Pseudomonas RefSeq genomes, and the outgroup C. japonicus Ueda107 using FastANI (Jain et al., 2018) with the minFrag option set to 30 to accommodate the distance between the Pseudomonas strains and the outgroup. The lower triangular matrix generated by the software was then converted to a distance matrix, clustered using UPGMA through the PHYLIP neighbor executable4, and visualized in iTOL (Letunic and Bork, 2016). The ANI Calculator (Goris et al., 2007) was also used to confirm results.
Siderophore Production Assays
To test siderophore production within the Lake Michigan Pseudomonas strains, we used the universal siderophore assay with chrome azurol S (CAS) and hexadecyltrimethylammonium bromide (HDTMA) as indicators. Media was prepared as detailed in Louden et al. (2011). In the presence of siderophores, the media will change color from blue to orange. 7 mL of 1.7% agar media was aliquoted into each well of a 6-well plate. Once solidified, 4 μL of the turbid, overnight Pseudomonas culture was spotted onto the media and placed in the incubator at 37°C. After ∼48 h, the plates were removed from the incubator and evaluated.
Heavy Metal Susceptibility Testing
To test the resistance of Pseudomonas strains to mercury, the methods of Sone et al. (2013) were employed. Briefly, Pseudomonas strains were grown overnight in 30 mL LB, shaking, at 37°C. 200 μL of the overnight culture was suspended in 3 mL LB medium containing HgCl2 (Acros Organics; Geel, Belgium) at varied concentrations. Each concentration/strain was cultured in triplicate. Following incubation for 16 h at 37°C, each culture’s absorbance at A600 was measured. The same approach was taken for testing the resistance of Pseudomonas strains to copper: 200 μL of the overnight culture was suspended in 3 mL LB medium containing CuSO4 5H2O (Acros Organics; Geel, Belgium) at varied concentrations. Each concentration/strain was cultured in triplicate for 16 h at 37°C and each culture’s absorbance at A600 was measured.
Antibiotic Susceptibility Testing
The Kirby-Bauer (KB) disk diffusion susceptibility method was used to test the Pseudomonas strains’ sensitivity to three antibiotics: Fosfomycin (BD Diagnostics; Sparks, MD, United States), Ciprofloxacin (BD Diagnostics; Sparks, MD, United States), and Vancomycin (BD Diagnostics; Sparks, MD, United States). 200 μL of the bacterial overnight culture was spread on a 1.7% agar LB plate and dried for 5 min. On each plate the three antibiotic disks were placed on the lawn with sterile forceps and incubated overnight at 37°C. Plates were then removed and the zone of inhibition was measured. Susceptibility was determined based upon the BD Sensi-Disk protocol for each chemical. These tests were conducted in duplicate. Three isolates were excluded from the results due to inconsistent growth and subsequent inconclusive test results.
Results
Water collected from the Chicago nearshore waters of Lake Michigan in the summer of 2009 and 2016 were plated on six different medias. The plated samples were monitored over 5 days in which the number of bacterial colonies was counted. As the medias used selected for different species of culturable bacteria, variation between the growth rates and total growth between media was expected. The “less-selective” medias – LB, Eugonics and EMB – always exhibited more colonization than the “more-selective” Streptococcus, API, and Centrimide medias; this is true across collection days and sampling sites (Supplementary Data Sheet 2). Distinct colonies were selected from the plates and identified by 16S rRNA gene sequencing (Figure 1). The genera identified were representative of species which are known to be present within freshwaters and more critically are amenable to the conditions of culture used in this study. The most abundant genus detected was Pseudomonas with several different species identified. Given the number of the pseudomonads recovered, we sequenced the genomes of 15 representatives of the diversity detected, each with a unique 16S rRNA gene sequence. Twelve of the isolates came from samples taken directly from the water, two from puddles on the beach, and one from the soil of the beach. Sequencing statistics, including the number of reads generated, the number of contigs, N50 scores, and coverage can be found in Supplementary Table 2. Table 1 summarizes the 15 genomes sequenced, including their GenBank accession numbers.
As prior studies have noted that the 16S rRNA gene sequence can be insufficient in distinguishing species within the genus, we employed two methods to determine the species present. First, Pseudomonas species can be differentiated based upon the sequence homology of four housekeeping genes: the 16S rRNA gene sequence, gyrB, rpoB, and rpoD (Gomila et al., 2015). Figure 2A shows the concatenated sequence phylogenetic tree for the 15 genomes sequenced here and complete RefSeq Pseudomonas genomes, with Cellvibrio japonicus as the outgroup. Second, the ANI was calculated for the 15 genomes sequenced here, RefSeq Pseudomonas genomes, and the C. japonicus outgroup (Figure 2B). ANI values ranged between 78.5711% (RefSeq genomes P. savastanoi pv. phaeseolicola 1448A and P. aeruginosa PAO1) and 99.9975% (P. fulva str. LB-090714 and P. fulva str. 57B-090714). Eight Pseudomonas species were identified as well as three isolates for which a species designation could not be made. Two of these species, Pseudomonas sp. MB-090624 and Pseudomonas sp. LB-090624, clade with P. putida (Figure 2A). These two species have a pairwise ANI of 88.3160% and are more similar to each other than any of the other Lake Michigan isolates (Figure 2B). Of the RefSeq sequences included in this analysis, they are most similar to P. putida KT2440 (87.8185–90.0128% ANI); this is nevertheless below the 95% threshold expected of strains belonging to the same species. The concatenated sequence phylogenetic tree and ANI analysis, coupled with BLAST results for the individual contigs (Supplementary Table 1), informed the species classifications made. In the case of P. jessenii str. LBp-160603, further investigation was required; the gene annotations for this strain were compared to those previously identified for differentiating P. jessenii from other close relatives (Verhille et al., 1999) (Supplementary Table 3). Furthermore, upon submission of the 15 genome sequences, NCBI confirmed species designation via ANI against the type strains and proxytype strains that are already in GenBank (Federhen et al., 2016).
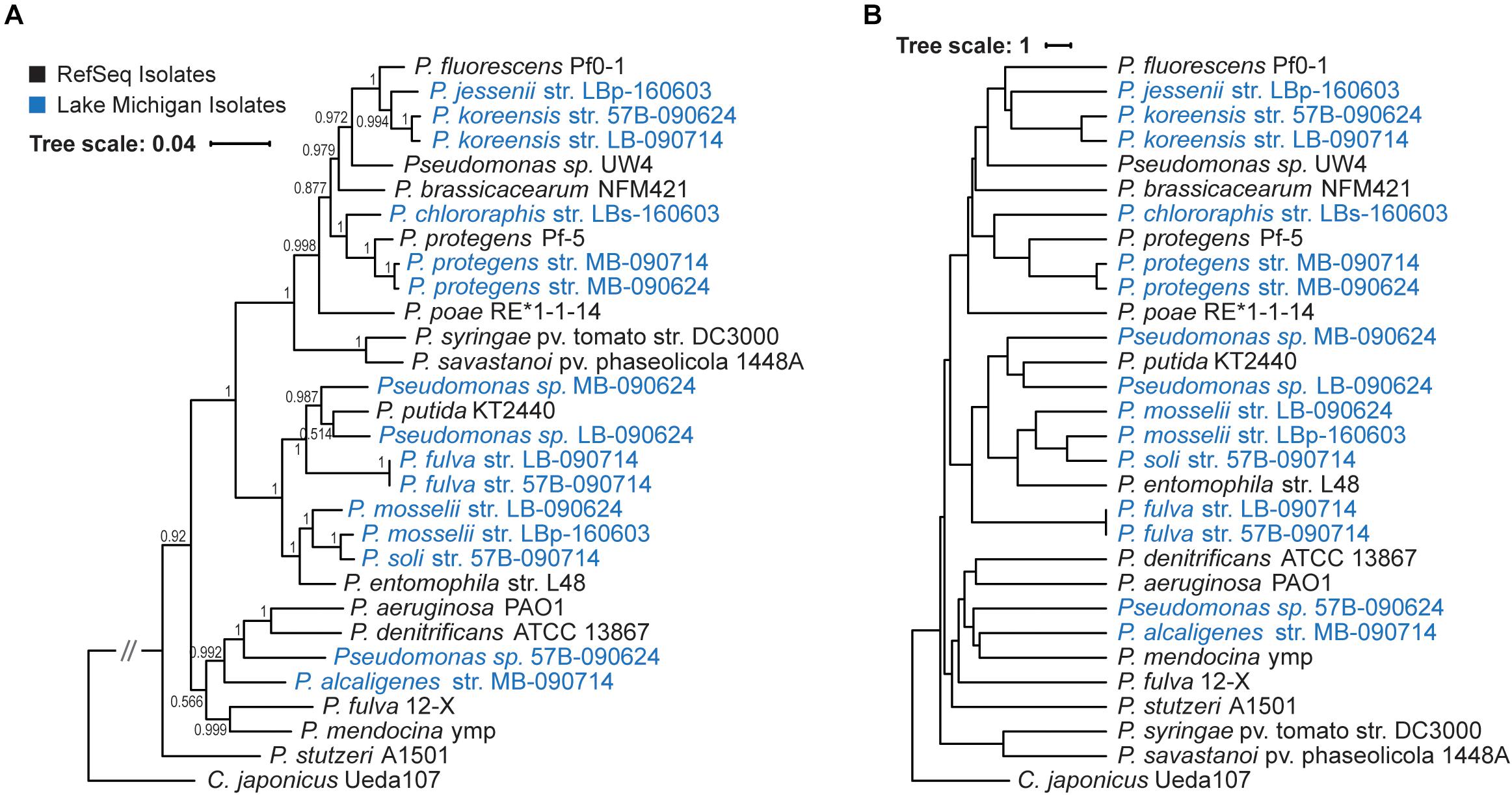
Figure 2. Phylogenetic diversity of 15 Lake Michigan pseudomonads. (A) Phylogenetic tree of concatenated 16S rRNA, gyrB, rpoB, and rpoD gene sequence. Branch support values are shown. (B) Hierarchical clustering based upon reciprocal ANI values. RefSeqs are indicated in black font and genomes generated in this study in blue font.
The 15 Pseudomonas genomes sequenced here vary in size from 4.01 to 7.08 Mbp, encoding 3812 to 6342 protein sequences (Table 1). Genome annotations can be found in Supplementary Table 4 and in GenBank (see Table 1 for accession numbers). No evidence of the CRISPR-Cas system was found. Annotations were compared identifying core subsystems present within the genomes as well as strain specific pathways predicted by the RAST web service (Aziz et al., 2008) (Supplementary Table 5). Comparative analysis revealed 175 subsystems that varied in their presence/absence amongst the 15 strains (Supplementary Table 6). From this list we probed deeper to identify strain- and group-specific attributes distinguishing the Lake Michigan Pseudomonas strains. While all genomes contained genes associated with ammonia assimilation, strains varied in the presence of functionality associated with other roles of nitrogen fixation (Supplementary Table 5). Variation between the strains was also observed with respect to sources for sulfur and carbon (Supplementary Table 5). Several of the strains also included gene annotations for the synthesis of achromobactin, aerobactin, and pyoverdine siderophores (Table 2). Evidence for the synthesis of all three of these types of siderophores was found within the genome of Pseudomonas sp. MB-090624. In contrast, the genome of P. mosselii LBp-160603 does not include any genes associated with siderophore synthesis. Siderophore production was evaluated in the lab using a universal siderophore assay (see section “Materials and Methods”). Siderophore production was prominent within P. koreensis str. 57B-090624, P. fulva str. LB-090714, P. fulva str. 57B-090714, and P. alcaligenes str. MB-090714 (Supplementary Data Sheet 3).
Genome annotations were further mined for virulence factors and defense mechanisms. All 15 strains encoded for genes associated with bacteriocin (colicin E2 and V) resistance, multidrug efflux pumps, and resistance to fluoroquinolones; resistance to Fosfomycin, Vancomycin, Spectinomycin, and Streptothricin was also detected in several of the strains (Supplementary Table 6). Sensitivity was tested for three of the antibiotics, Ciprofloxacin (a fluoroquinolone), Fosfomycin, and Vancomycin, via the Kirby-Bauer (KB) disk diffusion susceptibility method. As shown in Table 3, all of the strains tested exhibited sensitivity to Ciprofloxacin. However, all of the Lake Michigan Pseudomonas genome annotations included gene(s) associated with resistance to fluoroquinolones. While resistance to Fosfomycin was predicted for several strains, resistance was only observed for one of the strains predicted: the P. protogens str. MB-090624. Vancomycin resistance was observed for all of the strains tested (Table 3), although this resistance was only identified in three of the strains: P. jessenii str. LBp-160603, P. protogens str. MB-090624, and P. protogens str. MB-090714 (Supplementary Table 6).
Given prior evidence of co-selection of antibiotic and heavy metal resistance in freshwater bacteria (see review, Di Cesare et al., 2016), we next turned our attention to heavy metal resistance genes within the strains. All 15 strains encoded genes conferring resistance to arsenic, cobalt-zinc-cadmium, and copper. Resistance to chromium was also identified for the Pseudomonas strains, with the exception of. the P. fulva and P. mosselii strains, and the P. chlororaphis LBs-160603 strain encoded genes associated with resistance to zinc. Genes associated with resistances to mercury were only annotated for the genome of Pseudomonas sp. MB-090624. To test resistance to mercury, this strain as well as one of the P. fulva and one of the P. mosselii strains were grown in the presence of Hg(II). Even in the presence of 100 μM of mercury, no effect was detected on bacterial fitness within the Pseudomonas sp. MB-090624 strain (Figure 3A). Resistance to copper was also tested for nine of the Pseudomonas strains, all of which included a coding region annotated for copper tolerance (Figure 3B). Four of the strains, P. koreensis str. 57B-090624, Pseudomonas sp. LB-090624, P. mosselii str. LB-090624, and Pseudomonas sp. 57B-090624, saw only minimal reduction in fitness in the presence of high levels of copper (4 mM).
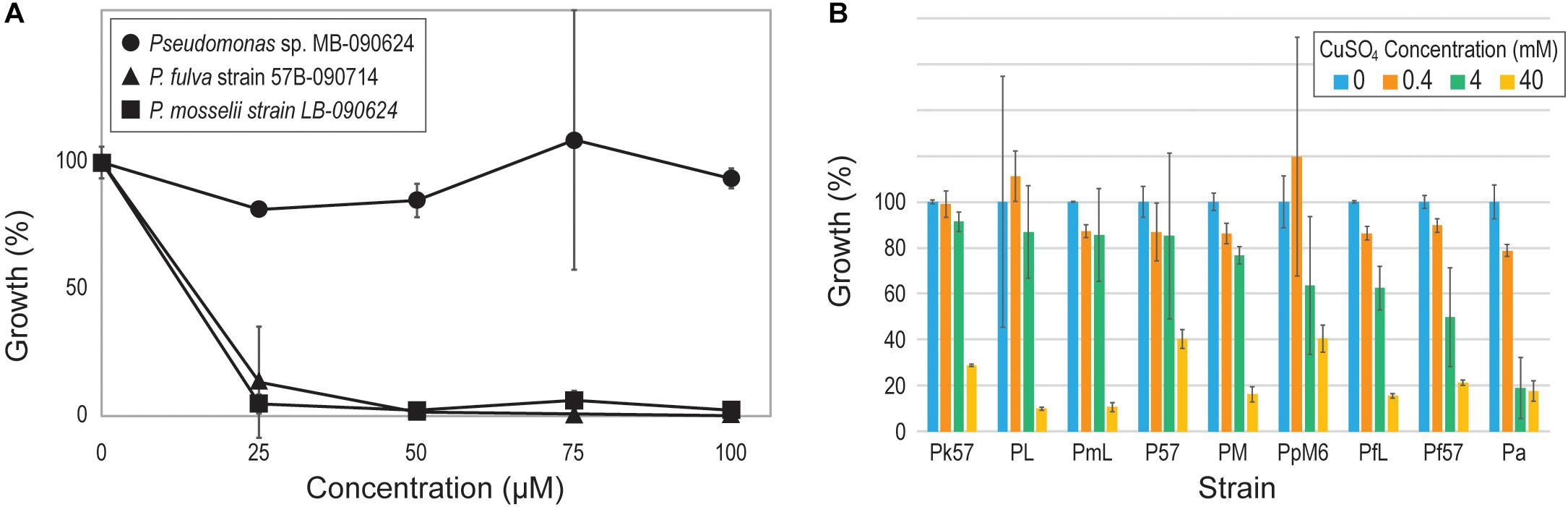
Figure 3. Pseudomonad resistance to (A) mercury and (B) copper. [Strain abbreviations in (B) are those listed in Table 2].
All 15 of the genome annotations produced by RAST included phage-related genes. To investigate further, each genome was examined using PHASTER (Arndt et al., 2016). Twenty-two intact, 11 incomplete, and one questionable phage sequence (prophage) was identified amongst the 15 genomes. Only Pseudomonas sp. LB-090624 did not contain an intact prophage, containing a single incomplete prophage. The prophages identified here exhibited homology to prophages of Pseudomonas genomes in GenBank. The one exception being the prophage identified for the P. alcaligenes str. MB-090714 sequence; it had only modest sequence homology to an uncultured Caudovirales phage (Supplemental Table 7). Focusing on the intact phages identified, 19 were unique. The intact prophage sequences are provided in Supplementary Data Sheet 4. Two of the integrated prophages identified within the P. koreensis strains, 35.2 and 37.3 kbp in length, were similar (>95%) to each other and a prophage identified within the sequence of Pseudomonas moraviensis BS3668 (GenBank: LT629788) (Figure 4A). Two prophages 34.5 kbp (Figure 4B) and 53.7 kbp (Figure 4C) in length (subsequently referred to as Pfulva1 and Pfulva2, respectively) were identified in both P. fulva strains. While their sequences were identical within the two P. fulva strains isolated here, they were distinct from sequence within the nr/nt database (Supplementary Table 7). Moreover, no sequence homology was identified between the Pfulva1 and Pfulva2 prophages. The annotated Pfulva1 sequence includes an integrase, C-5 cytosine-specific DNA methylase, and terminase, amongst structural phage genes, lysis proteins (holin and spanin), and hypothetical proteins. Pfulva2 encodes several virulence factors, including a Clp protease, DNA methyltransferase, and antitoxin (Supplementary Tables 8, 9). The presence of these two prophages were further confirmed via PCR amplification.
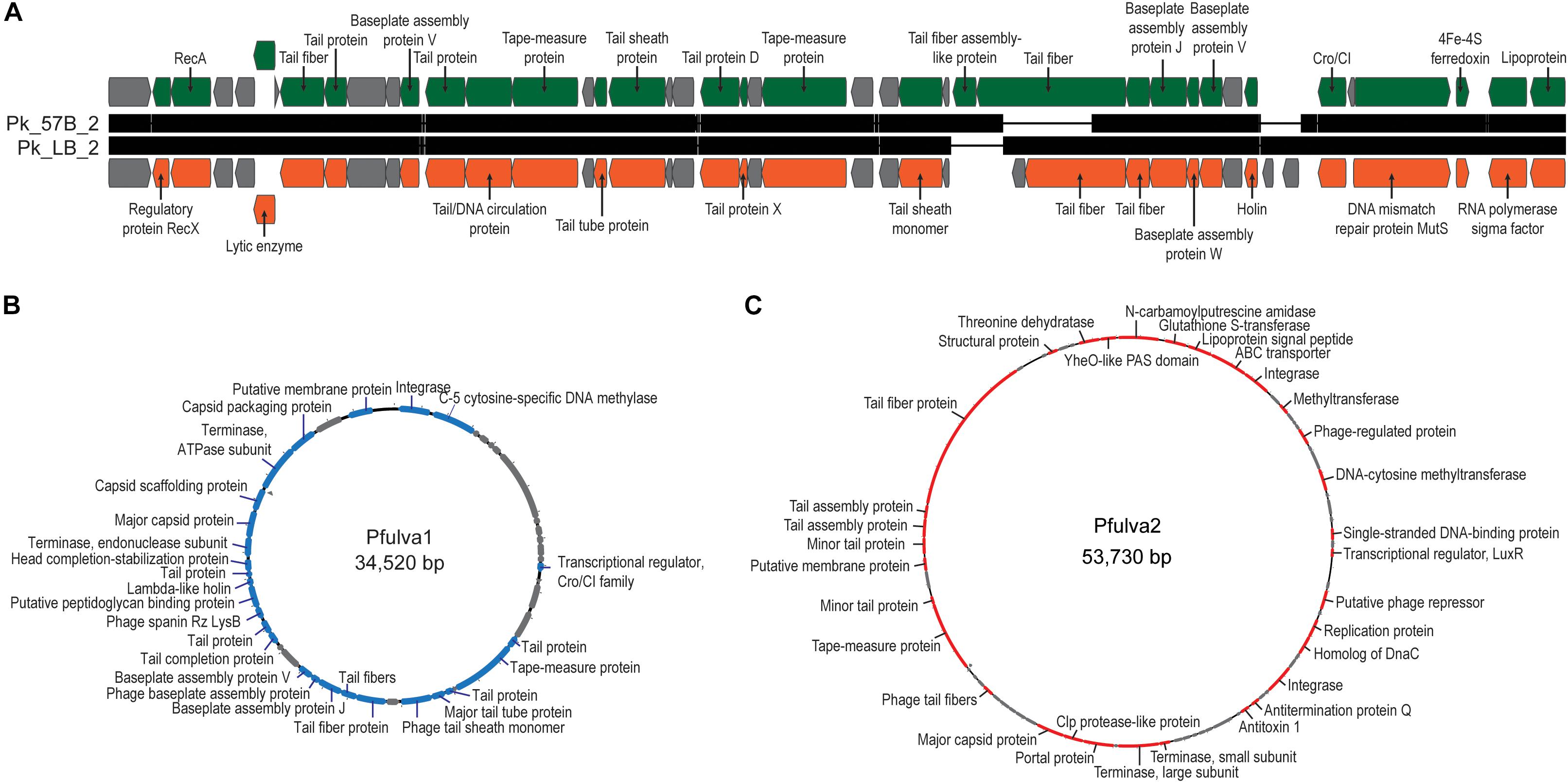
Figure 4. Genome maps of the prophages identified within the Lake Michigan (A) P. koreensis and (B,C) P. fulva strains. Hypothetical proteins are indicated in gray.
Discussion
In 2014 and 2015 we conducted high-throughput 16S rRNA sequencing surveys of the nearshore waters of Lake Michigan, which included the Montrose and 57th Street Beaches, and detected little or no pseudomonads (Malki et al., 2015a; Sible et al., 2015). Thus, Pseudomonas is likely not a dominant constituent of these waters. Even so, several different species of Pseudomonas have been isolated from other Great Lakes waters via targeted gene sequencing (Chatterjee et al., 2017). Here we have begun a comprehensive catalog of this diversity via whole genome sequencing. Through culture-dependent methods we were able to isolate 11 different Pseudomonas species from the Chicago nearshore waters. P. chlororaphis, P. fulva, P. jessenii, P. koreensis, P. mosselii, and P. protegens, often found in soil, can protect associated plants from fungal and bacterial pathogens (Xie et al., 2003; Deora et al., 2010; Ramette et al., 2011; Liu et al., 2014; Deng et al., 2015; Wu et al., 2018) through the production of diverse molecules (see review, Haas and Défago, 2005). The Pseudomonas species isolated here do not commonly cause disease in humans. P. alcaligenes, P. fulva, and P. mosselii, also commonly found in soil, are rare opportunistic human pathogens (Valenstein et al., 1983; Almuzara et al., 2010; Leneveu-Jenvrin et al., 2013; Suzuki et al., 2013). We did not isolate any strains of the well-studied human pathogen P. aeruginosa. This was initially surprising given that P. aeruginosa has been detected and/or isolated from stream, river, and lake freshwaters (Pellett et al., 1983; Römling et al., 1994; Pirnay et al., 2005; Khan et al., 2007; Selezska et al., 2012; Magalhães et al., 2016), is prevalent within urban wastewaters (Slekovec et al., 2012), and is the host for phages we previously isolated from these waters (Malki et al., 2015b). However, prior sampling of Lake Erie waters similarly did not find P. aeruginosa either (Chatterjee et al., 2017).
Whole genome sequencing of the Lake Michigan Pseudomonas isolates provides insight into their evolutionary relationships. As Figure 2 shows, these strains represent a diversity of species within the Pseudomonas tree. As prior studies have found that 16S rRNA sequences alone cannot distinguish between Pseudomonas species (Mulet et al., 2012; Gomila et al., 2015), two methods were employed here: the concatenated housekeeping genes and whole genome ANI. Only the two strains identified as P. protegens, the two strains identified as P. koreensis, and the two strains identified as P. fulva have a pairwise ANI value exceeding the threshold demarking species delineation (Richter and Rosselló-Móra, 2009). While phylogenetic and ANI analyses both identified the P. fulva strains here within the P. putida group, they do not clade with the RefSeq P. fulva 12-X strain. Prior studies evaluating the ANI (e.g., Han N. et al., 2016; Peña et al., 2016) and phylogeny for this genus (Gomila et al., 2015) have likewise found the P. fulva 12-X genome distant from the P. putida group. Until quite recently, this was the only complete genome for the species available. Earlier this year, another complete genome was produced: P. fulva FDAARGOS_167 (NZ_CP014025), isolated from sputum of a Cystic Fibrosis patient (per the genome’s GenBank record metadata). We found that this new P. fulva strain also has an ANI value less than that expected for two representatives of the same species (Supplementary Data Sheet 5), suggesting that P. fulva 12-X is a distinct species (i.e., not fulva). The three isolates for which a strain determination could not be made – Pseudomonas sp. MB-090624, Pseudomonas sp. LB-090624, and Pseudomonas sp. 57B-090624 – may be representatives of new species.
Investigation of the annotations generated for the 15 Lake Michigan genomes identified unique features including metabolic and nutrient utilization pathways (Supplementary Table 6). Prevalent amongst the Lake Michigan Pseudomonas strains is the presence of gene annotations for the synthesis of siderophores (Table 2). We were able to verify siderophore production within four of the 14 strains containing annotations for siderophores using the universal siderophore assay (Supplementary Data Sheet 3). It is important to note that siderophore production within the other 10 strains is likely; however, we were unable to cultivate these strains on the universal siderophore assay media. Although these strains were cultivated routinely under the same laboratory conditions, the universal siderophore media may exclude a vital nutrient/resource for growth. Moreover, it is well-known that species-specific siderophore systems exist amongst the pseudomonads (Meyer et al., 2002). Siderophore production within most of the pseudomonads from Lake Michigan suggests a possible role in enhancing iron abundance within the water column, similar to speculations for siderophore-producing bacteria within marine surface waters (see review, Ahmed and Holmström, 2014). Furthermore, recent evidence found that siderophores play a role in the interactions between co-occurring Pseudomonas strains and likely drive diversification in nature (Butaitė et al., 2017).
The Lake Michigan pseudomonads encode for several antibiotic resistance genes (Supplementary Table 6). Fluoroquinolone resistance was identified within the annotations of all 15 Lake Michigan Pseudomonas strains. Prior studies have noted its prevalence amongst clinical Pseudomonas samples (Horii et al., 2005; López-Causapé et al., 2018) as well as aquatic isolates (Adachi et al., 2013; Johnning et al., 2015; Chu et al., 2018). KB tests performed here using the fluoroquinolone Ciprofloxacin, however, revealed that all of the strains were actually sensitive to the antibiotic (Table 3). The annotated fluoroquinolone resistance clearly does not confer resistance to Ciprofloxacin. The Lake Michigan pseudomonads may be resistant to other fluoroquinolones not tested here, as a prior study found P. aeruginosa strains vary in their sensitivity to the fluoroquinolones Ciprofloxacin, Levofloxacin, and Moxifloxacin (Grillon et al., 2016). While the genome annotations also indicated that some strains encode for resistance to Fosfomycin and Vancomycin, KB diffusion assays were often found to contradict these predictions (Table 3). KB assays found that all 15 of the strains are resistant to Vancomycin, nine exhibiting no inhibition of growth. Therefore, the annotations alone cannot definitively characterize a strain’s sensitivities to antibiotics. Correlating mutations within these coding regions and confirmed sensitive and resistant strains is necessary to improve annotations and antibiotic resistance predictions.
As previous research has found, heavy metal resistance genes can cause positive selection of antibiotic resistance genes (Baker-Austin et al., 2006; Pal et al., 2015; see review Di Cesare et al., 2016). The Lake Michigan Pseudomonas strains encode for resistance of several heavy metals. In the bioinformatic study of Pal et al. (2015) investigating co-occurrence patterns of resistance genes, the only metal resistance genes commonly co-occurring with antibiotic resistance genes were for mercury resistance. Only one of the Lake Michigan Pseudomonas strains, Pseudomonas sp. MB-090624, was shown to tolerant of high levels of mercury (Figure 3A). Mercury emissions remain a concern in the Chicago area (Sullivan and Mason, 1998; Gratz et al., 2013); mercury emissions within the area did not see strong regulation until 2006 with the Illinois EPA mercury and Clean Air Interstate Rule. Recently, an increased level of mercury in Lake Michigan fish has been observed (Blukacz-Richards et al., 2017). The multiple heavy metal resistance genes identified within the pseudomonads may prove beneficial within these urban waters in response to potential contaminations.
As our group’s prior work in these waters has focused on isolation and characterization of phages, and in particular Pseudomonas infecting phages (Malki et al., 2015b; Watkins et al., 2016, 2018), we were not surprised to find prophages within the 15 Lake Michigan Pseudomonas genomes. The P. koreensis prophage, identified in isolates from sampling sites 17 mi apart on two separate collection dates, may be (or have been at that time) a frequent member of the Lake Michigan nearshore community. We may hypothesize this to be true for the Pfulva1 and Pfulva2 prophages as well (Figures 4B,C). The P. fulva prophages most closely resemble Pseudomonas phage φCTX and the unclassified Cvm10 virus Enterobacter phage Arya, respectively. It is important to note that these similarities are tenuous; only ∼50% of these prophages exhibit sequence homology to a GenBank record. Novel prophages with < 50% sequence homology to any annotated phage or prophage sequence were also identified within the P. alcaligenes str. MB-090714, P. koreensis str. 57B-090624, P. protogens str. MB-090624, Pseudomonas sp. 57B-090624, and Pseudomonas sp. MB-090624 genomes (Supplementary Table 7). This hints at a large reservoir of phages within freshwaters that have yet to be discovered. Recent efforts have begun to isolate and characterize freshwater phages, in particular Pseudomonas infecting phages (e.g., Mäntynen et al., 2015; Lu et al., 2017).
Pseudomonas is a cosmopolitan genus, and while not commonly abundant within freshwater environments (Newton et al., 2011), it has been isolated from numerous freshwater lakes including the Great Lakes (Bennett, 1969; Chatterjee et al., 2017). Here we have presented the first whole genome sequences of pseudomonads from the Great Lakes. Given the anthropogenic impact on the Chicago nearshore waters, it is not possible to determine if these isolates are constituents of the microbial community or ephemeral members recently introduced. Nevertheless, pseudomonads’ ability to breakdown and utilize a wide variety of organic compounds serves them well, especially in varied conditions such as Chicago winters when the nearshore waters often freeze. As the study presented here shows, a variety of pseudomonads have inhabited the coastal waters of Lake Michigan.
Author Contributions
MB extracted and prepared isolates for sequencing. RS, BH, MA, AA, MKh, PP, TR, SSi, SSa, EX, TH, and CP performed genome assemblies and annotations. MKo and AC collected samples. MB and LM performed phenotype assays. MB, LM, and CP conducted genome sequence analyses and drafted the manuscript. All authors contributed to the editing of the manuscript and approve of the final manuscript.
Funding
This work was supported by the National Science Foundation (award # 1149387) to CP.
Conflict of Interest Statement
The authors declare that the research was conducted in the absence of any commercial or financial relationships that could be construed as a potential conflict of interest.
Acknowledgments
The authors would like to thank Salma Javeed, Naureen Shakir, Vinicio Reynoso, Martin Pugliese, and April Williams for their assistance in collecting samples during 2009. Thanks also to Mohammed Abourahma, Ola Al-Bayati, Mohammed Ismail, Waleed Omar, and Eyas Zorub for their contributions in preliminary genome analysis.
Supplementary Material
The Supplementary Material for this article can be found online at: https://www.frontiersin.org/articles/10.3389/fmicb.2019.00195/full#supplementary-material
Footnotes
- ^ https://github.com/najoshi/sickle
- ^ https://sourceforge.net/projects/bbmap/
- ^ http://bioinfo.ut.ee/primer3-0.4.0/
- ^ http://evolution.genetics.washington.edu/phylip.html
References
Adachi, F., Yamamoto, A., Takakura, K.-I., and Kawahara, R. (2013). Occurrence of fluoroquinolones and fluoroquinolone-resistance genes in the aquatic environment. Sci. Total Environ. 444, 508–514. doi: 10.1016/j.scitotenv.2012.11.077
Ahmed, E., and Holmström, S. J. M. (2014). Siderophores in environmental research: roles and applications. Microb Biotechnol. 7, 196–208. doi: 10.1111/1751-7915.12117
Almuzara, M. N., Vazquez, M., Tanaka, N., Turco, M., Ramirez, M. S., Lopez, E. L., et al. (2010). First case of human infection due to Pseudomonas fulva, an environmental bacterium isolated from cerebrospinal fluid. J. Clin. Microbiol. 48, 660–664. doi: 10.1128/JCM.01849-09
Arndt, D., Grant, J. R., Marcu, A., Sajed, T., Pon, A., Liang, Y., et al. (2016). PHASTER: a better, faster version of the PHAST phage search tool. Nucleic Acids Res. 44, W16–W21. doi: 10.1093/nar/gkw387
Aziz, R. K., Bartels, D., Best, A. A., DeJongh, M., Disz, T., Edwards, R. A., et al. (2008). The RAST Server: rapid annotations using subsystems technology. BMC Genomics 9:75. doi: 10.1186/1471-2164-9-75
Baker-Austin, C., Wright, M. S., Stepanauskas, R., and McArthur, J. V. (2006). Co-selection of antibiotic and metal resistance. Trends Microbiol. 14, 176–182. doi: 10.1016/j.tim.2006.02.006
Bankevich, A., Nurk, S., Antipov, D., Gurevich, A. A., Dvorkin, M., Kulikov, A. S., et al. (2012). SPAdes: a new genome assembly algorithm and its applications to single-cell sequencing. J. Comput. Biol. 19, 455–477. doi: 10.1089/cmb.2012.0021
Bennett, E. A. (1969). A Study of the Distribution of Heterotrophic Bacteria in the Great Lakes. I. The Heterotrophs in Lake Water. Ontario: Ontario Water Resources Commission, Laboratory Report.
Blukacz-Richards, E. A., Visha, A., Graham, M. L., McGoldrick, D. L., de Solla, S. R., Moore, D. J., et al. (2017). Mercury levels in herring gulls and fish: 42 years of spatio-temporal trends in the Great Lakes. Chemosphere 172, 476–487. doi: 10.1016/j.chemosphere.2016.12.148
Bower, P. A., Scopel, C. O., Jensen, E. T., Depas, M. M., and McLellan, S. L. (2005). Detection of genetic markers of fecal indicator bacteria in Lake Michigan and determination of their relationship to Escherichia coli densities using standard microbiological methods. Appl. Environ. Microbiol. 71, 8305–8313. doi: 10.1128/AEM.71.12.8305-8313.2005
Butaitė, E., Baumgartner, M., Wyder, S., and Kümmerli, R. (2017). Siderophore cheating and cheating resistance shape competition for iron in soil and freshwater Pseudomonas communities. Nat. Commun. 8:414. doi: 10.1038/s41467-017-00509-4
Chatterjee, P., Davis, E., Yu, F., James, S., Wildschutte, J. H., Wiegmann, D. D., et al. (2017). Environmental Pseudomonads inhibit cystic fibrosis patient-derived Pseudomonas aeruginosa. Appl. Environ. Microbiol. 83:e2701-16. doi: 10.1128/AEM.02701-16
Chu, B. T. T., Petrovich, M. L., Chaudhary, A., Wright, D., Murphy, B., Wells, G., et al. (2018). Metagenomics reveals the impact of wastewater treatment plants on the dispersal of microorganisms and genes in aquatic sediments. Appl. Environ. Microbiol. 84:e2168-17. doi: 10.1128/AEM.02168-17
Couvin, D., Bernheim, A., Toffano-Nioche, C., Touchon, M., Michalik, J., Néron, B., et al. (2018). CRISPRCasFinder, an update of CRISRFinder, includes a portable version, enhanced performance and integrates search for Cas proteins. Nucleic Acids Res. 46, W246–W251. doi: 10.1093/nar/gky425
Darling, A. C. E., Mau, B., Blattner, F. R., and Perna, N. T. (2004). Mauve: multiple alignment of conserved genomic sequence with rearrangements. Genome Res. 14, 1394–1403. doi: 10.1101/gr.2289704
DeFlorio-Barker, S., Wing, C., Jones, R. M., and Dorevitch, S. (2018). Estimate of incidence and cost of recreational waterborne illness on United States surface waters. Environ. Health 17:3. doi: 10.1186/s12940-017-0347-9
Deng, P., Wang, X., Baird, S. M., and Lu, S.-E. (2015). Complete genome of Pseudomonas chlororaphis strain UFB2, a soil bacterium with antibacterial activity against bacterial canker pathogen of tomato. Stand. Genomic Sci. 10:117. doi: 10.1186/s40793-015-0106-x
Deora, A., Hatano, E., Tahara, S., and Hashidoko, Y. (2010). Inhibitory effects of furanone metabolites of a rhizobacterium, Pseudomonas jessenii, on phytopathogenic Aphanomyces cochlioides and Pythium aphanidermatum. Plant Pathol. 59, 84–99. doi: 10.1111/j.1365-3059.2009.02177.x
Di Cesare, A., Eckert, E., and Corno, G. (2016). Co-selection of antibiotic and heavy metal resistance in freshwater bacteria. J. Limnol. 75, 59–66. doi: 10.4081/jlimnol.2016.1198
Dorevitch, S., Pratap, P., Wroblewski, M., Hryhorczuk, D. O., Li, H., Liu, L. C., et al. (2011). Health risks of limited-contact water recreation. Environ. Health Perspect. 120, 192–197. doi: 10.1289/ehp.1103934
Drucker, V. V., and Panasyuk, E. Y. (2006). Potentially pathogenic bacteria in a microbial community of Lake Baikal. Hydrobiologia 568, 267–271. doi: 10.1007/s10750-006-0304-z
Drury, B., Rosi-Marshall, E., and Kelly, J. J. (2013). Wastewater treatment effluent reduces the abundance and diversity of benthic bacterial communities in urban and suburban rivers. Appl. Environ. Microbiol. 79, 1897–1905. doi: 10.1128/AEM.03527-12
Edgar, R. C. (2004). MUSCLE: multiple sequence alignment with high accuracy and high throughput. Nucleic Acids Res. 32, 1792–1797. doi: 10.1093/nar/gkh340
Eiler, A., and Bertilsson, S. (2004). Composition of freshwater bacterial communities associated with cyanobacterial blooms in four Swedish lakes. Environ. Microbiol. 6, 1228–1243. doi: 10.1111/j.1462-2920.2004.00657.x
Federhen, S., Rossello-Mora, R., Klenk, H.-P., Tindall, B. J., Konstantinidis, K. T., Whitman, W. B., et al. (2016). Meeting report: GenBank microbial genomic taxonomy workshop (12–13 May, 2015). Stand. Genomic Sci. 11:15. doi: 10.1186/s40793-016-0134-1
Fisher, J. C., Newton, R. J., Dila, D. K., and McLellan, S. L. (2015). Urban microbial ecology of a freshwater estuary of Lake Michigan. Elementa 3:000064. doi: 10.12952/journal.elementa.000064
Foley, J. A., Defries, R., Asner, G. P., Barford, C., Bonan, G., Carpenter, S. R., et al. (2005). Global consequences of land use. Science 309, 570–574. doi: 10.1126/science.1111772
Fuller, K., Shear, H., and Wittig, J. (1995). The Great Lakes: an Environmental Atlas and Resource Book. Toronto: United States Environmental Protection Agency.
Gomila, M., Peña, A., Mulet, M., Lalucat, J., and García-Valdés, E. (2015). Phylogenomics and systematics in Pseudomonas. Front. Microbiol. 6:214. doi: 10.3389/fmicb.2015.00214
Goris, J., Konstantinidis, K. T., Klappenbach, J. A., Coenye, T., Vandamme, P., and Tiedje, J. M. (2007). DNA-DNA hybridization values and their relationship to whole-genome sequence similarities. Int. J. Syst. Evol. Microbiol. 57, 81–91. doi: 10.1099/ijs.0.64483-0
Gratz, L. E., Keeler, G. J., Marsik, F. J., Barres, J. A., and Dvonch, J. T. (2013). Atmospheric transport of speciated mercury across southern Lake Michigan: Influence from emission sources in the Chicago/Gary urban area. Sci. Total Environ. 448, 84–95. doi: 10.1016/j.scitotenv.2012.08.076
Grillon, A., Schramm, F., Kleinberg, M., and Jehl, F. (2016). Comparative activity of Ciprofloxacin, Levofloxacin and Moxifloxacin against Klebsiella pneumoniae, Pseudomonas aeruginosa and Stenotrophomonas maltophilia assessed by minimum inhibitory concentrations and time-kill studies. PLoS One 11:e0156690. doi: 10.1371/journal.pone.0156690
Haack, S. K., Fogarty, L. R., Stelzer, E. A., Fuller, L. M., Brennan, A. K., Isaacs, N. M., et al. (2013). Geographic setting influences Great Lakes beach microbiological water quality. Environ. Sci. Technol. 47, 12054–12063. doi: 10.1021/es402299a
Haas, D., and Défago, G. (2005). Biological control of soil-borne pathogens by fluorescent pseudomonads. Nat. Rev. Microbiol. 3, 307–319. doi: 10.1038/nrmicro1129
Han, M., Gong, Y., Zhou, C., Zhang, J., Wang, Z., and Ning, K. (2016). Comparison and interpretation of taxonomical structure of bacterial communities in two types of lakes on Yun-Gui plateau of China. Sci. Rep. 6:30616. doi: 10.1038/srep30616
Han, N., Qiang, Y., and Zhang, W. (2016). ANItools web: a web tool for fast genome comparison within multiple bacterial strains. Database 2016:baw084. doi: 10.1093/database/baw084
Hayden, C. J., and Beman, J. M. (2016). Microbial diversity and community structure along a lake elevation gradient in yosemite national park, California, USA. Environ. Microbiol. 18, 1782–1791. doi: 10.1111/1462-2920.12938
Hoadley, A. W., and McCoy, E. (1966). Studies of certain Gram-negative bacteria from surface waters. Health Lab. Sci. 3, 20–32.
Horii, T., Muramatsu, H., and Iinuma, Y. (2005). Mechanisms of resistance to fluoroquinolones and carbapenems in Pseudomonas putida. J. Antimicrob. Chemother. 56, 643–647. doi: 10.1093/jac/dki254
Jain, C., Rodriguez-R, L. M., Phillippy, A. M., Konstantinidis, K. T., and Aluru, S. (2018). High throughput ANI analysis of 90K prokaryotic genomes reveals clear species boundaries. Nat. Commun. 9:5114. doi: 10.1038/s41467-018-07641-9
Johnning, A., Kristiansson, E., Fick, J., Weijdegård, B., and Larsson, D. G. J. (2015). Resistance mutations in gyrA and parC are common in Escherichia communities of both fluoroquinolone-polluted and uncontaminated aquatic environments. Front. Microbiol. 6:1355. doi: 10.3389/fmicb.2015.01355
Jones, S. E., Cadkin, T. A., Newton, R. J., and McMahon, K. D. (2012). Spatial and temporal scales of aquatic bacterial beta diversity. Front. Microbiol. 3:318. doi: 10.3389/fmicb.2012.00318
Khan, N. H., Ishii, Y., Kimata-Kino, N., Esaki, H., Nishino, T., Nishimura, M., et al. (2007). Isolation of Pseudomonas aeruginosa from open ocean and comparison with freshwater, clinical, and animal isolates. Microb. Ecol. 53, 173–186. doi: 10.1007/s00248-006-9059-3
Lee, C. S., Kim, M., Lee, C., Yu, Z., and Lee, J. (2016). The microbiota of recreational freshwaters and the implications for environmental and public health. Front. Microbiol. 7:1826. doi: 10.3389/fmicb.2016.01826
Leneveu-Jenvrin, C., Madi, A., Bouffartigues, E., Biaggini, K., Feuilloley, M., Chevalier, S., et al. (2013). Cytotoxicity and inflammatory potential of two Pseudomonas mosselii strains isolated from clinical samples of hospitalized patients. BMC Microbiol. 13:123. doi: 10.1186/1471-2180-13-123
Letunic, I., and Bork, P. (2016). Interactive tree of life (iTOL) v3: an online tool for the display and annotation of phylogenetic and other trees. Nucleic Acids Res. 44, W242–W245. doi: 10.1093/nar/gkw290
Linz, A. M., Crary, B. C., Shade, A., Owens, S., Gilbert, J. A., Knight, R., et al. (2017). Bacterial community composition and dynamics spanning five years in freshwater bog lakes. mSphere 2:e169-17. doi: 10.1128/mSphere.00169-17
Liu, H., Yang, C.-L., Ge, M.-Y., Ibrahim, M., Li, B., Zhao, W.-J., et al. (2014). Regulatory role of tetR gene in a novel gene cluster of Acidovorax avenae subsp. avenae RS-1 under oxidative stress. Front. Microbiol. 5:547. doi: 10.3389/fmicb.2014.00547
Llorens-Marès, T., Triadó-Margarit, X., Borrego, C. M., Dupont, C. L., and Casamayor, E. O. (2016). High bacterial diversity and phylogenetic novelty in dark euxinic freshwaters analyzed by 16S tag community profiling. Microb. Ecol. 71, 566–574. doi: 10.1007/s00248-015-0696-2
López-Causapé, C., Cabot, G., del Barrio-Tofiño, E., and Oliver, A. (2018). The versatile mutational resistome of Pseudomonas aeruginosa. Front. Microbiol. 9:685. doi: 10.3389/fmicb.2018.00685
Lorenzo, P., Adriana, A., Jessica, S., Carles, B., Marinella, F., Marta, L., et al. (2018). Antibiotic resistance in urban and hospital wastewaters and their impact on a receiving freshwater ecosystem. Chemosphere 206, 70–82. doi: 10.1016/j.chemosphere.2018.04.163
Louden, B. C., Haarmann, D., and Lynne, A. M. (2011). Use of blue agar CAS assay for siderophore detection. J. Microbiol. Biol. Educ. 12, 51–53. doi: 10.1128/jmbe.v12i1.249
Lu, G., Luhr, J., Stoecklein, A., Warner, P., and Tapprich, W. (2017). Complete genome sequences of Pseudomonas fluorescens bacteriophages isolated from freshwater samples in Omaha, Nebraska. Genome Announc. 5:e1501-16. doi: 10.1128/genomeA.01501-16
Magalhães, M. J. T. L., Pontes, G., Serra, P. T., Balieiro, A., Castro, D., Pieri, F. A., et al. (2016). Multidrug resistant Pseudomonas aeruginosa survey in a stream receiving effluents from ineffective wastewater hospital plants. BMC Microbiol. 16:193. doi: 10.1186/s12866-016-0798-0
Malki, K., Bruder, K., and Putonti, C. (2015a). Survey of microbial populations within Lake Michigan nearshore waters at two Chicago public beaches. Data Brief 5, 556–559. doi: 10.1016/j.dib.2015.10.005
Malki, K., Kula, A., Bruder, K., Sible, E., Hatzopoulos, T., Steidel, S., et al. (2015b). Bacteriophages isolated from Lake Michigan demonstrate broad host-range across several bacterial phyla. Virol. J. 12:164. doi: 10.1186/s12985-015-0395-0
Mäntynen, S., Laanto, E., Kohvakka, A., Poranen, M. M., Bamford, J. K. H., and Ravantti, J. J. (2015). New enveloped dsRNA phage from freshwater habitat. J. Gen. Virol. 96, 1180–1189. doi: 10.1099/vir.0.000063
Marchesi, J. R., Sato, T., Weightman, A. J., Martin, T. A., Fry, J. C., Hiom, S. J., et al. (1998). Design and evaluation of useful bacterium-specific PCR primers that amplify genes coding for bacterial 16S rRNA. Appl. Environ. Microbiol. 64, 795–799.
Marti, E., Jofre, J., and Balcazar, J. L. (2013). Prevalence of antibiotic resistance genes and bacterial community composition in a river influenced by a wastewater treatment plant. PLoS One 8:e78906. doi: 10.1371/journal.pone.0078906
Meyer, J.-M., Geoffroy, V. A., Baida, N., Gardan, L., Izard, D., Lemanceau, P., et al. (2002). Siderophore typing, a powerful tool for the identification of fluorescent and nonfluorescent pseudomonads. Appl. Environ. Microbiol. 68, 2745–2753. doi: 10.1128/AEM.68.6.2745-2753.2002
Morris, C. E., Conen, F., Alex Huffman, J., Phillips, V., Pöschl, U., and Sands, D. C. (2014). Bioprecipitation: a feedback cycle linking Earth history, ecosystem dynamics and land use through biological ice nucleators in the atmosphere. Glob. Change Biol. 20, 341–351. doi: 10.1111/gcb.12447
Morris, C. E., Sands, D. C., Vinatzer, B. A., Glaux, C., Guilbaud, C., Buffière, A., et al. (2008). The life history of the plant pathogen Pseudomonas syringae is linked to the water cycle. ISME J. 2, 321–334. doi: 10.1038/ismej.2007.113
Mou, X., Jacob, J., Lu, X., Robbins, S., Sun, S., and Ortiz, J. D. (2013). Diversity and distribution of free-living and particle-associated bacterioplankton in Sandusky Bay and adjacent waters of Lake Erie Western Basin. J. Great Lakes Res. 39, 352–357. doi: 10.1016/j.jglr.2013.03.014
Mueller-Spitz, S. R., Goetz, G. W., and McLellan, S. L. (2009). Temporal and spatial variability in nearshore bacterioplankton communities of Lake Michigan. FEMS Microbiol. Ecol. 67, 511–522. doi: 10.1111/j.1574-6941.2008.00639.x
Mulet, M., Gomila, M., Scotta, C., Sánchez, D., Lalucat, J., and García-Valdés, E. (2012). Concordance between whole-cell matrix-assisted laser-desorption/ionization time-of-flight mass spectrometry and multilocus sequence analysis approaches in species discrimination within the genus Pseudomonas. Syst. Appl. Microbiol. 35, 455–464. doi: 10.1016/j.syapm.2012.08.007
Neuenschwander, S. M., Ghai, R., Pernthaler, J., and Salcher, M. M. (2018). Microdiversification in genome-streamlined ubiquitous freshwater Actinobacteria. ISME J. 12, 185–198. doi: 10.1038/ismej.2017.156
Newton, R. J., Jones, S. E., Eiler, A., McMahon, K. D., and Bertilsson, S. (2011). A guide to the natural history of freshwater lake bacteria. Microbiol. Mol. Biol. Rev. 75, 14–49. doi: 10.1128/MMBR.00028-10
Newton, R. J., Jones, S. E., Helmus, M. R., and McMahon, K. D. (2007). Phylogenetic ecology of the freshwater actinobacteria acI lineage. Appl. Environ. Microbiol. 73, 7169–7176. doi: 10.1128/AEM.00794-07
Newton, R. J., and McLellan, S. L. (2015). A unique assemblage of cosmopolitan freshwater bacteria and higher community diversity differentiate an urbanized estuary from oligotrophic Lake Michigan. Front. Microbiol. 6:1028. doi: 10.3389/fmicb.2015.01028
Oh, S., Caro-Quintero, A., Tsementzi, D., DeLeon-Rodriguez, N., Luo, C., Poretsky, R., et al. (2011). Metagenomic insights into the evolution, function, and complexity of the planktonic microbial community of Lake Lanier, a temperate freshwater ecosystem. Appl. Environ. Microbiol. 77, 6000–6011. doi: 10.1128/AEM.00107-11
Oliveri, V. P., Kruse, C. W., Kawata, K., and Smith, J. E. (1977). Microorganisms in Urban Stormwater. Cincinnati, OH: Office of Research and Development.
Oster, R. J., Wijesinghe, R. U., Haack, S. K., Fogarty, L. R., Tucker, T. R., and Riley, S. C. (2014). Bacterial pathogen gene abundance and relation to recreational water quality at seven Great Lakes beaches. Environ. Sci. Technol. 48, 14148–14157. doi: 10.1021/es5038657
Pal, C., Bengtsson-Palme, J., Kristiansson, E., and Larsson, D. G. J. (2015). Co-occurrence of resistance genes to antibiotics, biocides and metals reveals novel insights into their co-selection potential. BMC Genomics 16:964. doi: 10.1186/s12864-015-2153-5
Pellett, S., Bigley, D. V., and Grimes, D. J. (1983). Distribution of Pseudomonas aeruginosa in a riverine ecosystem. Appl. Environ. Microbiol. 45, 328–332.
Peña, A., Busquets, A., Gomila, M., Mulet, M., Gomila, R. M., Reddy, T. B. K., et al. (2016). High quality draft genome sequences of Pseudomonas fulva DSM 17717(T), Pseudomonas parafulva DSM 17004(T) and Pseudomonas cremoricolorata DSM 17059(T) type strains. Stand. Genomic Sci. 11:55. doi: 10.1186/s40793-016-0178-2
Pietsch, R. B., Vinatzer, B. A., and Schmale, D. G. (2017). Diversity and abundance of ice nucleating strains of Pseudomonas syringae in a freshwater lake in Virginia, USA. Front. Microbiol. 8:318. doi: 10.3389/fmicb.2017.00318
Pirnay, J.-P., Matthijs, S., Colak, H., Chablain, P., Bilocq, F., Van Eldere, J., et al. (2005). Global Pseudomonas aeruginosa biodiversity as reflected in a Belgian river. Environ. Microbiol. 7, 969–980. doi: 10.1111/j.1462-2920.2005.00776.x
Price, M. N., Dehal, P. S., and Arkin, A. P. (2010). FastTree 2 – approximately maximum-likelihood trees for large alignments. PLoS One 5:e9490. doi: 10.1371/journal.pone.0009490
Ramette, A., Frapolli, M., Saux, M. F.-L., Gruffaz, C., Meyer, J.-M., Défago, G., et al. (2011). Pseudomonas protegens sp. nov., widespread plant-protecting bacteria producing the biocontrol compounds 2,4-diacetylphloroglucinol and pyoluteorin. Syst. Appl. Microbiol. 34, 180–188. doi: 10.1016/j.syapm.2010.10.005
Rao, Y. R., and Schwab, D. J. (2007). Transport and mixing between the coastal and offshore waters in the great lakes: a review. J. Great Lakes Res. 33, 202–218. doi: 10.3394/0380-1330(2007)33[202:TAMBTC]2.0.CO;2
Ren, Z., Wang, F., Qu, X., Elser, J. J., Liu, Y., and Chu, L. (2017). Taxonomic and functional differences between microbial communities in qinghai lake and its input streams. Front. Microbiol. 8:2319. doi: 10.3389/fmicb.2017.02319
Richter, M., and Rosselló-Móra, R. (2009). Shifting the genomic gold standard for the prokaryotic species definition. Proc. Natl. Acad. Sci. 106, 19126–19131. doi: 10.1073/pnas.0906412106
Römling, U., Wingender, J., Müller, H., and Tümmler, B. (1994). A major Pseudomonas aeruginosa clone common to patients and aquatic habitats. Appl. Environ. Microbiol. 60, 1734–1738.
Saarenheimo, J., Aalto, S. L., Rissanen, A. J., and Tiirola, M. (2017). Microbial community response on wastewater discharge in Boreal Lake sediments. Front. Microbiol. 8:750. doi: 10.3389/fmicb.2017.00750
Salmaso, N., Albanese, D., Capelli, C., Boscaini, A., Pindo, M., and Donati, C. (2018). Diversity and cyclical seasonal transitions in the bacterial community in a large and deep perialpine lake. Microb. Ecol. 76, 125–143. doi: 10.1007/s00248-017-1120-x
Scherer, P. I., Millard, A. D., Miller, A., Schoen, R., Raeder, U., Geist, J., et al. (2017). Temporal dynamics of the microbial community composition with a focus on toxic cyanobacteria and toxin presence during harmful algal blooms in two South German Lakes. Front. Microbiol. 8:2387. doi: 10.3389/fmicb.2017.02387
Selezska, K., Kazmierczak, M., Müsken, M., Garbe, J., Schobert, M., Häussler, S., et al. (2012). Pseudomonas aeruginosa population structure revisited under environmental focus: impact of water quality and phage pressure. Environ. Microbiol. 14, 1952–1967. doi: 10.1111/j.1462-2920.2012.02719.x
Selvakumar, A., and Borst, M. (2006). Variation of microorganism concentrations in urban stormwater runoff with land use and seasons. J. Water Health 4, 109–124. doi: 10.2166/wh.2006.0009
Sible, E., Cooper, A., Malki, K., Bruder, K., Hatzopoulos, T., Watkins, S. C., et al. (2015). Assessment of microbial populations within Chicago area nearshore waters and interfaces with river systems. Data Brief 5, 305–308. doi: 10.1016/j.dib.2015.09.004
Sievers, F., Wilm, A., Dineen, D., Gibson, T. J., Karplus, K., Li, W., et al. (2011). Fast, scalable generation of high-quality protein multiple sequence alignments using Clustal Omega. Mol. Syst. Biol. 7:539. doi: 10.1038/msb.2011.75
Slekovec, C., Plantin, J., Cholley, P., Thouverez, M., Talon, D., Bertrand, X., et al. (2012). Tracking down antibiotic-resistant Pseudomonas aeruginosa isolates in a wastewater network. PLoS One 7:e49300. doi: 10.1371/journal.pone.0049300
Sone, Y., Mochizuki, Y., Koizawa, K., Nakamura, R., Pan-Hou, H., Itoh, T., et al. (2013). Mercurial-resistance determinants in pseudomonas strain K-62 plasmid pMR68. AMB Express 3:41. doi: 10.1186/2191-0855-3-41
Sullivan, K. A., and Mason, R. P. (1998). The concentration and distribution of mercury in Lake Michigan. Sci. Total Environ. 213, 213–228. doi: 10.1016/S0048-9697(98)00094-1
Suzuki, M., Suzuki, S., Matsui, M., Hiraki, Y., Kawano, F., and Shibayama, K. (2013). Genome sequence of a strain of the human pathogenic bacterium Pseudomonas alcaligenes that caused bloodstream infection. Genome Announc. 1:e919-13. doi: 10.1128/genomeA.00919-13
Templar, H. A., Dila, D. K., Bootsma, M. J., Corsi, S. R., and McLellan, S. L. (2016). Quantification of human-associated fecal indicators reveal sewage from urban watersheds as a source of pollution to Lake Michigan. Water Res. 100, 556–567. doi: 10.1016/j.watres.2016.05.056
Valenstein, P., Bardy, G. H., Cox, C. C., and Zwadyk, P. (1983). Pseudomonas alcaligenes endocarditis. Am. J. Clin. Pathol. 79, 245–247. doi: 10.1093/ajcp/79.2.245
Verhille, S., Baida, N., Dabboussi, F., Izard, D., and Leclerc, H. (1999). Taxonomic study of bacteria isolated from natural mineral waters: proposal of Pseudomonas jessenii sp. nov. and Pseudomonas mandelii sp. nov. Syst. Appl. Microbiol. 22, 45–58. doi: 10.1016/S0723-2020(99)80027-7
Watkins, S. C., Kuehnle, N., Ruggeri, C. A., Malki, K., Bruder, K., Elayyan, J., et al. (2016). Assessment of a metaviromic dataset generated from nearshore Lake Michigan. Mar. Freshwater Res. 67:1700. doi: 10.1071/MF15172
Watkins, S. C., Sible, E., and Putonti, C. (2018). Pseudomonas PB1-like phages: whole genomes from metagenomes offer insight into an abundant group of bacteriophages. Viruses 10:E331. doi: 10.3390/v10060331
Whitman, R. L., and Nevers, M. B. (2008). Summer E. coli patterns and responses along 23 Chicago beaches. Environ. Sci. Technol. 42, 9217–9224. doi: 10.1021/es8019758
Wijesinghe, R. U., Oster, R. J., Haack, S. K., Fogarty, L. R., Tucker, T. R., and Riley, S. C. (2015). Spatial, temporal, and matrix variability of Clostridium botulinum type E toxin gene distribution at Great Lakes beaches. Appl. Environ. Microbiol. 81, 4306–4315. doi: 10.1128/AEM.00098-15
Wilhelm, S. W., LeCleir, G. R., Bullerjahn, G. S., McKay, R. M., Saxton, M. A., Twiss, M. R., et al. (2014). Seasonal changes in microbial community structure and activity imply winter production is linked to summer hypoxia in a large lake. FEMS Microbiol. Ecol. 87, 475–485. doi: 10.1111/1574-6941.12238
Wong, M., Kumar, L., Jenkins, T. M., Xagoraraki, I., Phanikumar, M. S., and Rose, J. B. (2009). Evaluation of public health risks at recreational beaches in Lake Michigan via detection of enteric viruses and a human-specific bacteriological marker. Water Res. 43, 1137–1149. doi: 10.1016/j.watres.2008.11.051
Wu, L., Xiao, W., Chen, G., Song, D., Khaskheli, M. A., Li, P., et al. (2018). Identification of Pseudomonas mosselii BS011 gene clusters required for suppression of rice blast fungus Magnaporthe oryzae. J. Biotechnol. 282, 1–9. doi: 10.1016/j.jbiotec.2018.04.016
Xie, G.-L., Soad, A., Swings, J., and Mew, T. W. (2003). Diversity of Gram negative bacteria antagonistic against major pathogens of rice from rice seed in the tropic environment. J. Zhejiang Univ. Sci. 4, 463–468. doi: 10.1631/jzus.2003.0463
Yang, J., Jiang, H., Wu, G., Liu, W., and Zhang, G. (2016). Distinct factors shape aquatic and sedimentary microbial community structures in the lakes of Western China. Front. Microbiol. 7:1782. doi: 10.3389/fmicb.2016.01782
Zwart, G., Hiorns, W. D., Methé, B. A., van Agterveld, M. P., Huismans, R., Nold, S. C., et al. (1998). Nearly identical 16S rRNA sequences recovered from lakes in North America and Europe indicate the existence of clades of globally distributed freshwater bacteria. Syst. Appl. Microbiol. 21, 546–556. doi: 10.1016/S0723-2020(98)80067-2
Keywords: Pseudomonas, freshwater, Lake Michigan, heavy metal resistance bacteria, genomics
Citation: Batrich M, Maskeri L, Schubert R, Ho B, Kohout M, Abdeljaber M, Abuhasna A, Kholoki M, Psihogios P, Razzaq T, Sawhney S, Siddiqui S, Xoubi E, Cooper A, Hatzopoulos T and Putonti C (2019) Pseudomonas Diversity Within Urban Freshwaters. Front. Microbiol. 10:195. doi: 10.3389/fmicb.2019.00195
Received: 04 September 2018; Accepted: 23 January 2019;
Published: 15 February 2019.
Edited by:
George S. Bullerjahn, Bowling Green State University, United StatesReviewed by:
Hans Wildschutte, Bowling Green State University, United StatesMartin W. Hahn, University of Innsbruck, Austria
Copyright © 2019 Batrich, Maskeri, Schubert, Ho, Kohout, Abdeljaber, Abuhasna, Kholoki, Psihogios, Razzaq, Sawhney, Siddiqui, Xoubi, Cooper, Hatzopoulos and Putonti. This is an open-access article distributed under the terms of the Creative Commons Attribution License (CC BY). The use, distribution or reproduction in other forums is permitted, provided the original author(s) and the copyright owner(s) are credited and that the original publication in this journal is cited, in accordance with accepted academic practice. No use, distribution or reproduction is permitted which does not comply with these terms.
*Correspondence: Catherine Putonti, cputonti@luc.edu