- 1School of Life Sciences, Jawaharlal Nehru University, New Delhi, India
- 2School of Environmental Sciences, Jawaharlal Nehru University, New Delhi, India
Magnesium (Mg) is a crucial macronutrient required for the regular growth of plants. Here we report the identification, isolation and functional characterization of Mg-transporter PiMgT1 in root endophytic fungus Piriformospora indica. We also report the role of P. indica in the improvement of the Mg nutrition of the plant particularly under Mg deficiency condition. Protein BLAST (BLASTp) for conserved domains analysis showed that PiMgT1 belong to CorA like protein family of bacteria. We have also observed the presence of conserved ‘GMN’ signature sequence which suggests that PiMgT1 belongs to Mg transporter family. Phylogenetic analysis revealed that PiMgT1 clustered among fungal CorA family members nearer to basidiomycetes. Functionality of PiMgT1 was confirmed by complementation of a yeast magnesium transporter mutant CM66. We have observed that PiMgT1 restored the growth of mutant and showed comparable growth with that of WT. We found statistically significant (p < 0.05) two fold increase in the total intracellular Mg content of mutant complemented with PiMgT1 as compared to the mutant. These observations suggest that PiMgT1 is actively involved in Mg uptake by the fungus and may be helping in the nutritional status of the host plant.
Introduction
Piriformospora indica is a root endophyte isolated from the Thar desert of Rajasthan, India (Verma et al., 1998). Unlike arbuscular mycorrhizal fungi (AMF), P. indica can be cultured axenically and now a stable transformation system is available to know the gene functions in this fungus (Pham et al., 2008; Yadav et al., 2010). Further, P. indica has numerous beneficial effects on their host plant and help them to develop resistance against biotic as well as abiotic stresses (Waller et al., 2005; Baltruschat et al., 2008; Sherameti et al., 2008; Kumar et al., 2009; Vadassery et al., 2009; Sun et al., 2010; Yadav et al., 2010; Jogawat et al., 2013; Narayan et al., 2017).
It has been established that nutrient availability in the soil has major impact on the plant productivity. Metal ions are very important for various cellular and biochemical process as they play a vital role in various important biological processes. Mg is one of the most abundant elements on the earth and is an essential element which plays an important role in wide variety of biological processes. Being, central atom of the chlorophyll molecule, Mg plays a huge role in photosynthesis in plants. Additionally, Mg also acts as a cofactor in various biological enzymatic activities like RNA polymerase, DNA polymerase, ATPase, protein kinases, phosphatases and other cellular processes (Williams, 1993; Elin, 1994; Cowan, 1995; Shaul, 2002; Gardner, 2003).
Mg deficiency is an unfavorable plant disorder that occurs most often in strongly acidic, light, sandy soils, where it can easily leak away. It is a crucial macronutrient having dry mass of 0.2–0.4% and is compulsory for regular growth of plant. Due to deficiency of Mg, plants start damaging the chlorophyll in the old leaves which results in the yellowing of leaves. This condition is known as chlorosis which imparts the mottled appearance of leaves. In older leaves due to prolonged Mg deficiencies necrosis and drooping takes place.
Arbuscular mycorrhizal fungi has been reported to play important role in the uptake of the Mg from the soil and helps the plant grow healthy under Mg deficiency conditions (Wu et al., 2006; Doubková et al., 2012; Zhang et al., 2015). However, by taking a closer look of these reports, we found that no information is available on the functional characterization of the Mg transporter which is involved in the Mg transporter activity of the AMF. In the present work we have identified and functionally characterized a Mg transporter of P. indica. We recommend that utilization of P. indica and its Mg transporter not only complement crop-growing approaches, but may also be used as a model system to study the molecular mechanism of symbiotic plant–microbe interaction.
Materials and Methods
Maintenance of Bacterial, Fungal, and Plant Culture
Luria Bertani agar plates containing adequate amount of ampicillin (antibiotic) were used to maintain the bacterial strains. P. indica was cultured regularly on solidified Aspergillus modified medium (AMM) (Hill and Kafer, 2001) and incubated at 30 ± 2°C for 8–10 days. P. indica was also cultured in AMM broth in 250 ml culture flasks at 30 ± 2°C, and 110 rpm in shaking incubator (Multitron Incubator Shaker, HT-Infors, Switzerland). The yeast strains were maintained on YPD and SD medium at 30 + 2°C for 72 h. A. thaliana plants were maintained in controlled glasshouse conditions with a 10-h light (1000 Lux)/14-h dark period at temperature of 25 ± 2°C with a relative humidity 60–70%.
Interaction of A. thaliana and P. indica
Arabidopsis thaliana WT (ecotype Columbia-0) seeds were surface sterilized for 2 min in 0.1% mercuric chloride (HgCl2) and ethanol followed by 10 min in NaClO solution (0.75% Cl) and finally washed six times with sterile water and placed on Petri dishes containing MS medium (Murashige and Skoog, 1962). After cold treatment for 48 h at 4°C, plates were incubated for 10 days at 22°C under illumination (100 μmol m-2 s-1). P. indica was cultured as mentioned previously on AMM (Yadav et al., 2010). Co-cultivation of A. thaliana with the fungus P. indica was performed under in vitro culture conditions on solidified PNM media (Johnson et al., 2011). To do this, one P. indica disk was cut with the help of inverted portion of 1 ml tip and placed at the center on each plate and were allowed to grow for 10 days in dark. In case of plant, seedlings were first given cold treatment (4°C) for 48 h. After this treatment seedlings were allowed to grow for 10 days. Further, equal size seedlings were used for the co-cultivation assay. In order to study impact of Mg on plant growth and development during colonization with P. indica, plants colonized with P. indica were grown on PNM media and treated with five different Mg concentrations, i.e., 0, 2, 4, 10, and 25 mM. Seedlings were allowed to grow for 15 days at 22°C and 70–80% humidity in a 16-h light/8-h dark cycle. Plants were harvested after 15 dpi and total biomass, root length, number of leaves and chlorophyll content were measured. Kaefer media (KF) disk was used for mock treatment and mock-treated seedlings were used as control.
Root Colonization
Roots of A. thaliana cultivated with and without P. indica, were harvested after 15th dpi, washed intensively with double distilled water. In order to check the presence of chlamydospore in the roots, histochemical analysis was performed. To study colonization ten root samples from A. thaliana plant were randomly selected. Softening of the samples was done using 10% KOH solution for 15 min. Further samples were acidified with 1N HCl for 15 min. Finally, staining was performed using 0.02% Trypan blue overnight (Phillips and Hayman, 1970; Dickson and Smith, 1998; Kumar et al., 2009). The samples were de-stained with 50% lacto-phenol for 1–2 h prior to observation under the light microscope (Leica Microscope, Type 020-518.500, Germany and Nikon Eclipse Ti). Distribution of intracellular chlamydospores within the cortex region of root was taken as a symptom of colonization (McGonigle et al., 1990; Kumar et al., 2009).
Determination of Total Biomass, Root Length and Leaves Number
To study the impact of different concentration of Mg in the presence and in absence of P. indica the plant total biomass, root length, and number of leaves of the inoculated and non-inoculated plant after 15 days was measured as described (Jogawat et al., 2013). To calculate the total plant biomass, plants were harvested from co-cultivation plate and were washed properly with ddH2O and plants surface moisture was removed by keeping them on blotting paper and then the weight was recorded.
Measurement of Chlorophyll Content
To determine chlorophyll contents, leaves were harvested, weighed and was grounded in 90% ammonical acetone (acetone: water: 0.1 N ammonia, ratio of 90: 9: 1) at 4°C. This mixture was centrifuged at 12000 rpm at 4°C for 10 min. After centrifugation, supernatant obtained was used for the pigments measurement. Pigments contents were measured at 663 and 645 nm for Chl a, Chl b, respectively. Chlorophyll contents were calculated (as nmol/ml.), as follows, Chl a = (14.21 × OD663 - 3.01 × OD645), Chl b = (25.23 × OD645 - 5.16 × OD663), and total Chl (a+b) = (9.05 × OD663 + 22.2 × OD645). The obtained values were divided by leaf fresh weight to obtain values in nmol/ml/mg of leaf fresh weight as described (Porra et al., 1989).
Identification of Putative Mg Transporter
To identify Mg transporter in P. indica, genome database of P. indica (Zuccaro et al., 2011) was investigated. For this purpose, ALR1p nucleotides sequence of Saccharomyces cerevisiae was used as query. For BLASTP, we have fixed a cut off value of E = 10-2. Further, PiMgT1 and PiMgT2 were identified based on the amino acid similarity and domain analysis. Nucleotide sequence was studied using bioinformatics approaches such as GeneScan1, Translate tool2, nucleotide BLAST (BLASTn) and BLASTx3.
Isolation and Cloning of Putative Magnesium Transporter (PiMgT1 and PiMgT2)
Isolation of RNA and cDNA Synthesis
To do this, P. indica was grown in Kaefer media (Hill and Kafer, 2001) and MN media (Bécard and Fortin, 1988). Further, P. indica was washed twice with autoclaved ddH2O and transferred to MN media supplemented with 10 μM MgCl2 as a sole source of Mg. Ten days old culture of P. indica was harvested, freeze in liquid Nitrogen and stored at -80°C.
Total RNA was isolated with TRIzol reagent following the protocol provided by the manufacturer (Invitrogen, United States). Approximately 0.8 g fungal tissue was grounded to fine powder. To this, 1 ml TRIzol reagent was added and this was mixed vigorously. The homogenized samples were incubated further for 15 min at RT. Further, 200 μl chloroform was added (per ml of TRIzol reagent used) and this mixture was vigorously vortexed for 30 s and incubated at RT for 10 min. Following centrifugation (13,000 rpm; 15′; 4°C), upper aqueous phase was transferred into fresh RNase free tube (pre-cooled). The RNA from the aqueous layer was precipitated by mixing with 0.7 volumes of isopropyl alcohol. This mixture was incubated for 1 h at 4°C and centrifuged (12,000 rpm; 10′; 4°C). RNA pellet obtained was washed twice with 75% ethanol, centrifuged (10,000 rpm for 5 min at 4°C). Finally, RNA pellet obtained was dried for 10 min and dissolved in adequate volume of DEPC-treated water and quantified (NanoDrop 1000 spectrophotometer, Thermo Scientific).
Amplification of Putative P. indica PiMgT1 and PiMgT2
Predicted putative PiMgT1 and PiMgT2 were PCR amplified by using gene specific primers (Supplementary Table S1). For PCR reaction, P. indica cDNA was used as a template. PCR reactions were carried out in a final volume of 50 μl, containing 10 mM Tris-HCl (pH 8.3); 50 mM KCl; 1.5 mM MgCl2; 200 μM of dNTPs; 3 μM of each primer; 3 units of DNA Pfu-polymerase and 60–100 ng of cDNA as template. PCR program was used as follows: 94°C for 2 min (1 cycle), 94°C for 45 s, 59°C for 1 min 15 s, 72°C for 2.5 min (35 cycles) 72°C for 5 min (1 cycle), 4°C to hold the reaction. Amplified DNA was digested with specific restriction enzymes. PiMgT1 was digested with KPNI-XBAI and PiMgT2 with BamHI-XBAI. Digested DNA was electrophoresed and eluted using MinElute gel extraction kit (Qiagen, Germany). Eluted DNA was stored at -20°C. For cloning, ligation was performed at 4°C, overnight and for this purpose 1:3 of the vector-insert molar ratio was used. For transformation, Escherichia coli DH5-α bacterial strains were made competent as described by Inoue et al. (1990). The ligated product or plasmid was directly added to 200 μl competent cell suspension, mixed and was kept on ice for 30 min. The cells were then given a heat shock at 42°C for 90 s and quickly chilled on ice for 5 min. This was followed by addition of 0.8 ml of LB, and cells were incubated at 37°C for 45 min. The transformed competent cells were plated on LB plate containing ampicillin (100 μg/ml) and were incubated at 37°C overnight. Individual colonies were picked from overnight grown culture and mixed in 10 μl sterile dH2O in a 0.5 ml microcentrifuge tube. The lysis of cells was done by boiling for 2 min. After centrifugation, supernatant (5 μl) was used as a template for PCR. Colony PCR was carried out to confirm the presence of the insert in the transformed clone by using gene-specific primers (Supplementary Table S1). Further cloning was confirmed by restriction digestion and sequencing (GCC Biotech, West Bengal, India).
Phylogenetic and Homology Analysis
The functional sites and their pattern in putative PiMgT1 and PiMgT2 protein were determined using PROSITE data bank4. For identification purposes, BLASTn and BLASTx algorithm5 was used. Multalign analysis was done by using CLUSTALW software6. Phylogenetic and molecular evolutionary analyses of P. indica both putative PiMgT1 and PiMgT2 were conducted using MEGA7 with neighbor-joining (N-J) method7 (Tamura et al., 2011). Phylogenetic tree was constructed, with homologs protein of PiMgT1 and PiMgT2 from other fungi and other different groups like plant, bacteria and mammals (Supplementary Table S2).
Complementation Assay
The yeast CM66 mutant (MATa alr1::HIS3alr2::TRP1 his3-Δ200, ura3-52, leu2-Δ1, lys2-Δ202 trp1-Δ63) was used for the complementation assay to check the functionality of the of putative PiMgT1 and PiMgT2 protein. CM66 mutant of yeast S. cerevisiae lacks the plasma membrane-localized Mg2+ transporter genes ALR1 and ALR2 (Li et al., 2001). Both the genes PiMgT1 and PiMgT2 was subcloned into pYES2 yeast expression vector and positive clones were transformed into CM66 mutant.
Yeast mutant was used for transformation as described (Gietz et al., 1995). A single colony was inoculated into 20 ml YPD and incubated at 30°C at 220 rpm until OD650 reaches 1–1.5. One ml of freshly grown culture was transferred to fresh 100 ml YPD medium to produce an initial OD600 of 0.5. After reaching the OD600 of 0.5, cells were pelleted down by centrifugation at 4000 rpm for 5 min at RT. Supernatant was discarded and cell pellet was re-suspended in 50 ml H2O. Cells were again centrifuged (4000 rpm, 5 min, RT), supernatant was discarded and pellet obtained was re-suspended in 0.5 ml sterile LiAc solution [1 ml 10 × LiAc (1 M LiAc pH 7.5), 1 ml 10 × TE (0.1 M Tris-HCl pH 7.5, 10 mM EDTA), 8 ml H2O]. 100 μl of cell suspension was dispended in 1.5 ml micro centrifuge tubes, and 0.1 μg of each type of plasmid was added together with 100 μg of salmon sperm DNA followed by addition of 0.6 ml sterile PEG/LiAc solution (8 ml 50% PEG, 1 ml 10 × TE, 1 ml 10 × LiAc) to each tube and vortexed. Micro centrifuge tubes were incubated at 30°C for 30 min at 250 rpm. Post incubation period, 70 μl of 100% DMSO was added (10% final conc.) and this mixture was mixed gently. Further, heat shock treatment was given for 15 min at 42°C, followed by chilling on ice for 5 min and later was centrifuged for 1 min at 13,000 rpm. After centrifugation supernatant was discarded and cell pellet obtained was re-suspended in 0.5 mL of TE buffer. Finally 100 μl of this mixture was placed on Petri plates containing the appropriate selection medium (SD-URA-). Plates were incubated for 3–4 days until colonies appear. Positive clones were confirmed by performing plasmid PCR and by sequencing.
Functional Characterization
Growth Analysis
To study the growth pattern of S. cerevisiae WT, mutant transformed with vector only, and mutant transformed with PiMgT1 and PiMgT2 were inoculated separately in SD media supplemented with 250 mM MgCl2. Culture was allowed to grow till 0.6 OD600. This culture was harvested by centrifugation at 500 g for 2 min at 4°C. This culture was washed with 0.1 mM EDTA followed by washing with the autoclaved ddH2O. Equal number of cells (0.1 OD600) was taken for each strain and inoculated separately in SD media supplemented with different concentration of Mg, i.e., 0.1, 1, 4, 10, 25, and 100 mM. Culture was incubated at 30°C and OD600 were recorded at four hrs regular time intervals to observe comparison between the WT, mutant transformed with vector only and complemented mutants.
Spot Assay
To perform the spot assay S. cerevisiae WT, mutant transformed with vector only, mutant transformed with PiMgT1 and PiMgT2 were grown into YNB+URA- medium containing 250 mM MgCl2. Culture pellet was harvested by centrifugation at 500 g for 2 min at 4°C. Each culture was washed with 0.9% sodium chloride solution followed by washing with autoclaved ddH2O. Each cultures was serially diluted starting with fixed 0.1 OD600 (10-1, 10-2, 10-3, and 10-4) and was spotted in a row on YNB deficient in URA- agar plates supplemented with different concentration of MgCl2 (10 μM, 0.1 mM, 1, 4, 10, and 100 mM). The plates were incubated at 30°C for 5–10 days to observe comparison between the WT, complemented mutants and mutant transformed with vector only.
Cobalt Resistance Assay
To perform the cobalt resistance assay, S. cerevisiae WT, mutant transformed with vector, mutant transformed with PiMgT1 and PiMgT2 were cultured in YNB+URA- medium containing 250 mM MgCl2. Culture was harvested by centrifugation at 500 g for 2 min at 4°C. Each culture was washed with 0.9% sodium chloride solution followed by washing with autoclaved ddH2O. Each culture was serially diluted starting with fixed 0.1 OD600 (10-1, 10-2, 10-3, and 10-4) and was spotted in a row on YNB deficient in URA- agar plates supplemented with different concentration of CoCl2 (100 and 500 μM) and fixed concentration of MgCl2 (100 mM) in the medium. The plates were incubated at 30°C for 5–10 days to observe comparison between WT, complemented mutants and mutant transformed with vector only.
Uptake of Mg
To study uptake Mg, S. cerevisiae WT, mutant transformed with vector, mutant transformed with PiMgT1 were grown upto log phase (OD600 = 0.6 to 0.8) in LPM (low phosphate medium) medium containing 250 mM MgCl2. Cells were harvested by centrifugation at 500 g for 5 min at RT. Further, cells were washed with milli-Q water containing 1 mM EDTA and twice with autoclaved ddH2O and were incubated in LPM media without MgCl2 for 24 h. After starvation, culture was harvested by centrifugation at 500 g for 2 min. To energize, equal number of cells were re-suspended in 5 mM Tris-succinate buffer, at pH 4, supplemented with 1% galactose and incubated for 30 min at 25°C. To perform the uptake, yeast culture was centrifuged at 500 g, pellet obtained was re-suspended in uptake buffer (5 mM Tris-succinate buffer, pH 4, supplemented containing 4 mM MgCl2). Samples of 250 mL was withdrawn at different time points (0, 10, 20, 40, 60, and 120 min). To remove excess salts adhered with the cell surface, culture was washed three times with pre-chilled 30 mL of the washing solution (Milli-Q water, 1 mM EDTA). Further, pellet was collected by centrifugation at 500 g for 5 min. Pellet was air dried for 24–48 h and crushed with the help of mortar and pestle and fine powder was analyzed by EDXRF (Perring et al., 2017). An ED-XRF Epsilon 5XLE from PANalytical (Almelo, Netherlands) equipped with silver anode tube (maximum voltage = 50 kV) was used. Each sample was mixed with the 1 ml polymer binder to provide smooth surface. Further, settings for accelerating voltage and current were optimized in order to get the best compromise of sensitivity for the investigating Mg content regarding raw peak heights and ratios between raw peak and background heights. Mg content was estimated in the sample for 180 s. Zone of low energy with high overlapping emission lines, high resolution mode was preferred to better distinguish the energies of element Mg. Mg was analyzed at high resolution by setting background at region of interest (1.200) and region of maximum (1.280). Per pellet 8 min was devoted for total time of analysis, corresponding to the sum of measurement times and adjustment of flow of current, more time for atmosphere flushing, changing of filter, and holder rotation of sample holders. Three pellets were analyzed for each sample. Variability of investing yeast samples lead to matrix effects in calibration curves. Therefore, the background due to scattered primary radiation, was used to systematically correct all element ED-XRF signals. Calibration curves were established using the supplier software according to following formula:
Concentration = a × (Peak Count Rate = Background Count Rate) + b - overlapping factor × Peak count rate over lapping rate.
All pellets were analyzed and was mixed with the same polymer with similar ED-XRF conditions.
Statistical Analyses
The significance difference between colonized with P. indica and non-colonized plants was determined by unpaired t testing. P-values of <0.05 were considered significant. These statistical analyses were performed with Instat (GraphPad, San Diego, CA, United States).
Results
Growth Promotion Activity by P. indica
We have determined the growth of A. thaliana in the presence of different concentrations of Mg, i.e., 0, 2, 4, 10, and 25 mM during colonization with P. indica and non-colonized stage (Figure 1). Plants were found to be healthy when colonized with the P. indica as compared to the non-colonized plants at all the Mg concentrations (Figure 1). In order to test the impact of P. indica on plant growth and development, growth parameters like, total biomass, root length, number of leaves and chlorophyll contents were measured. Total plant biomass was found to be significantly high at 4 mM Mg when colonized with P. indica as compared to non-colonized plants (P = 0.03) (Figure 2A). Interestingly, biomass of the plants was found to be severely affected at 25 mM of Mg in both colonized and non-colonized state (Figure 2A). Root length of the non-colonized plants were found to be longer in comparison to P. indica colonized plants from 0 to 10 mM Mg concentration (Figures 1, 2B). However, in case of plants colonized with the P. indica and non-colonized plants treated with the high concentration of Mg, i.e., 25 mM, root length was found to be short in both the cases (Figures 1, 2B). The root of the non-colonized plants grown at a different concentrations of Mg (0–10 mM) were found very long along with numerous lateral roots, however, plants colonized with P. indica developed shorter roots as well as a lesser number of lateral roots (Figures 1, 2B). In case of colonized plants, leaves numbers were found to be high at all Mg concentrations as compared to the non-colonized plants (Figures 1, 2C). We have observed increase contents of chlorophyll a, b and total chlorophyll contents in plants colonized with the P. indica in comparison to the non-colonized plants at all Mg concentrations (Figures 3A–C). Additionally, chlorophyll contents was found to be less in the non-colonized and also in case of colonized plants at 0 mM Mg as compared to the colonized plant (Figures 3A–C). We have observed that level of colonization in the roots increased from 8 to 70% as the number of days progressed. A maximum percent of colonization, i.e., 70% was observed after 15 days of post inoculation of P. indica (Table 1).
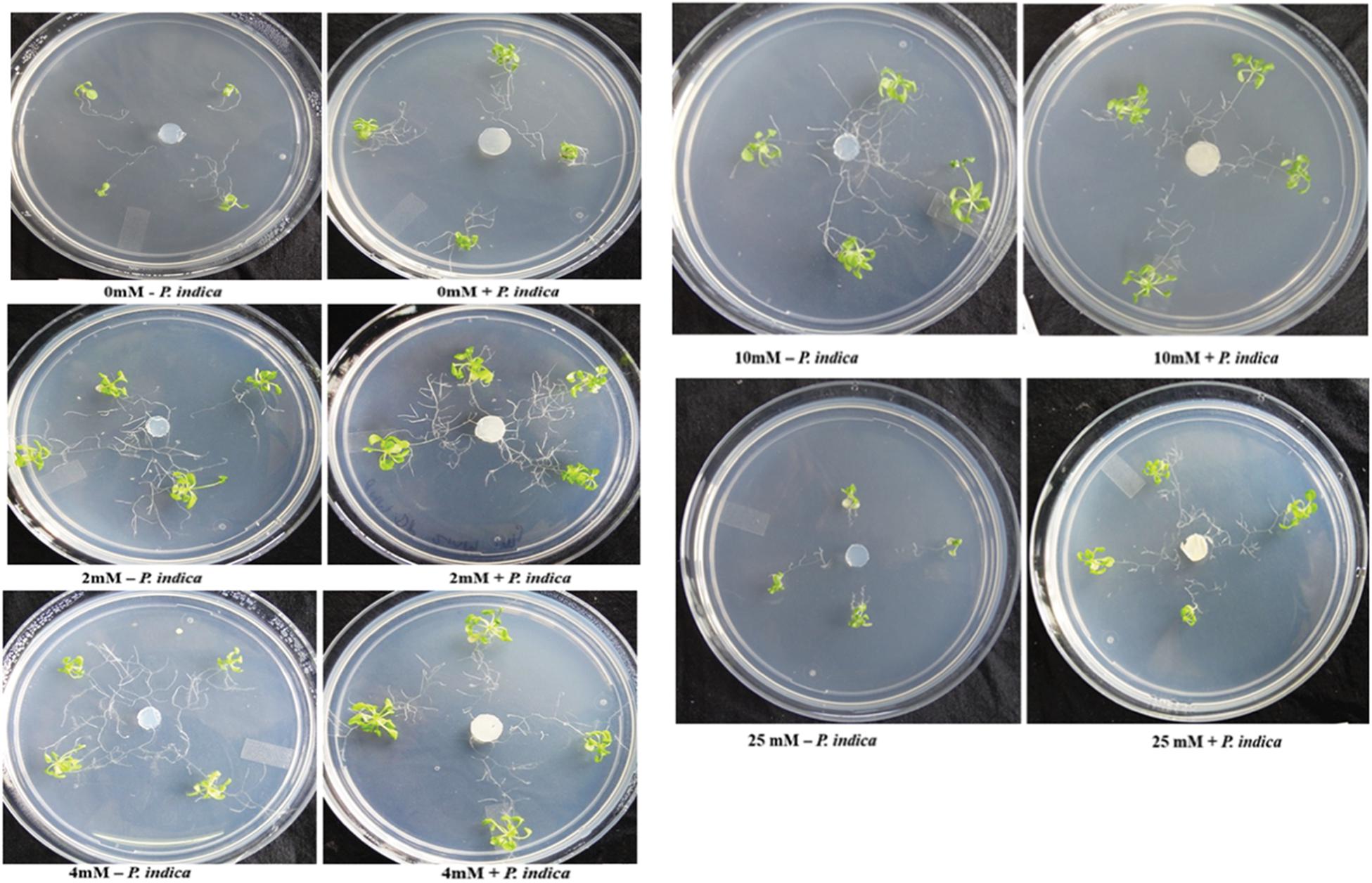
Figure 1. Interaction of plant with Piriformospora indica. Plants were cultivated with or without P. indica in PNM media supplemented with different concentration of Mg for 15 days under long day light conditions.
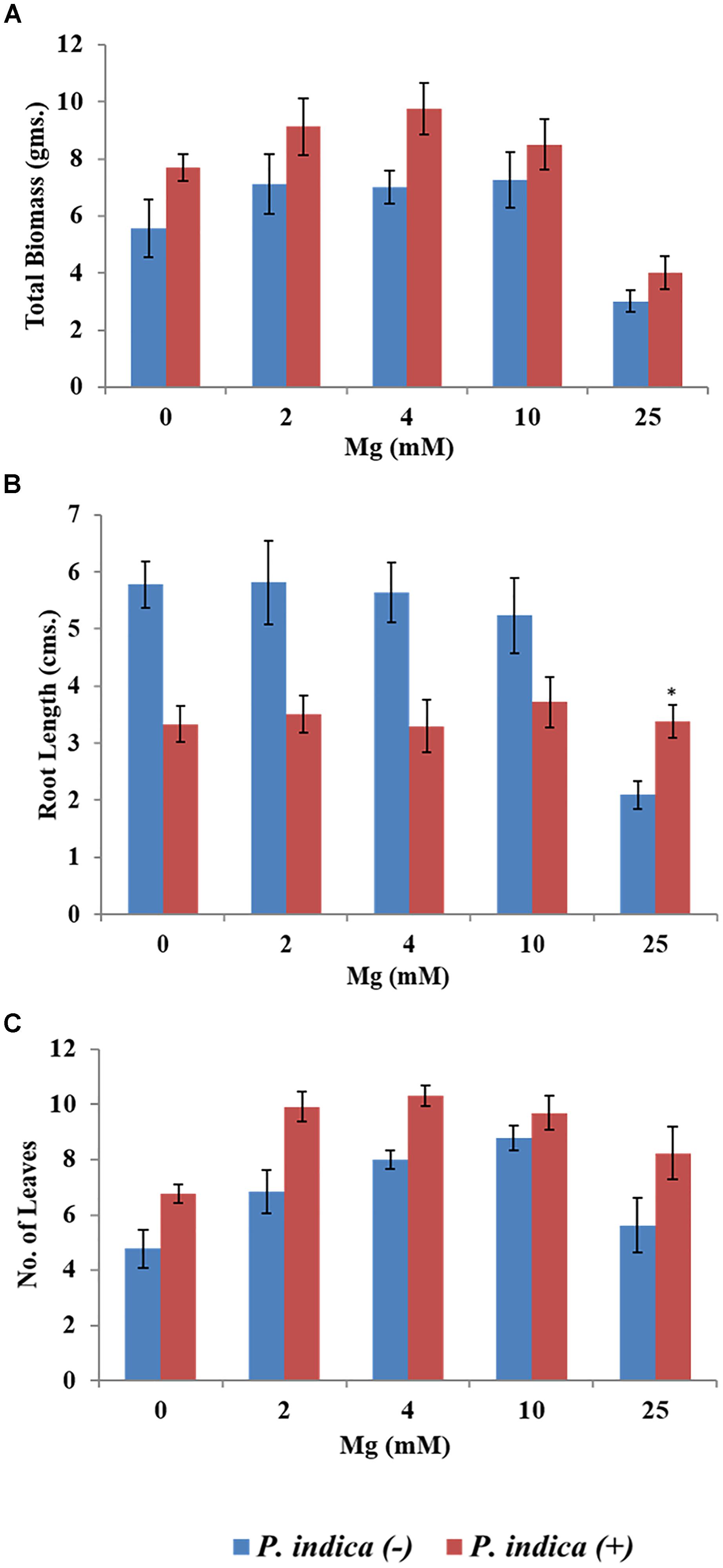
Figure 2. Impact of P. indica colonization on the growth of the plant. Growth parameters of Arabidopsis seedlings after 15 days of co-cultivation under different Mg concentration (A) Biomass (B) Root length (C) Number of leaves. Each data set represents the means of 3 independent measurements ± SE ∗indicates not significant as compared with the control (non-colonized); all other data are found significant at P < 0.05.
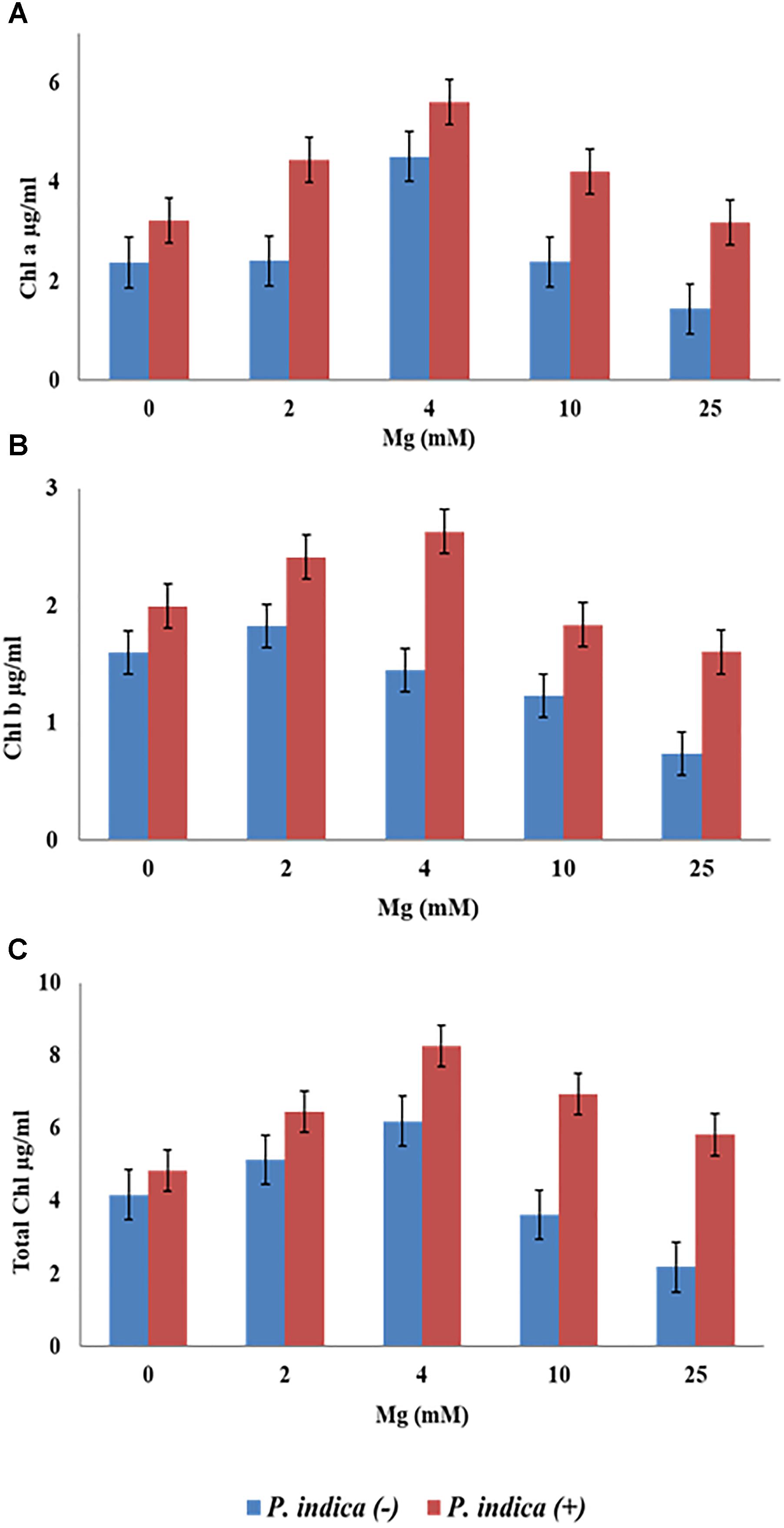
Figure 3. Measurement of chlorophyll contents of the plant during colonization with P. indica and during non-colonized stage. A. thaliana seedlings after 15 days of co-cultivation under different Mg concentration (A) chlorophyll a (B) chlorophyll b (C) total chlorophyll. Each data set represents the means of three independent measurements; all data are found significant at P < 0.05.
Identification and Isolation of Mg Transporter
In order to identify putative Mg transporter, BLASTn analysis was performed, by using the local alignment of the whole genome sequence of P. indica with the S. cerevisiae Mg transporter (ALR1p-1) as a query. Our analysis showed the presence of two homolog of ALR1p-1 named PiMgT1 and PiMgT2 in the genome of P. indica. We found that PiMgT1 and PiMgT2 belong to PIRI_contig_0025. Both the proteins PiMgT1 and PiMgT2 (accession number CCA67920.1 and CCA67912.1) shares 20 and 16% of query coverage as well as identity 31 and 25%, respectively, with the ALR1 protein of S. cerevisiae (Table 2). Protein BLAST (BLASTp) for conserved domains analysis showed that both putative transporters belong to CorA like protein family of bacteria.
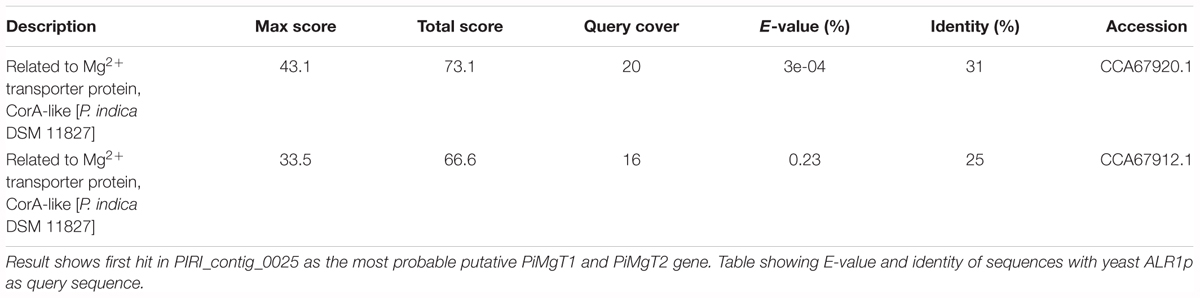
Table 2. BLASTn analysis for putative P. indica PiMgT1 and PiMgT2 gene sequence of P. indica genome.
Cloning of Putative Mg Transporter PiMgT1 and PiMgT2
After identification, both putative genes PiMgT1 and PiMgT2 ORF were cloned into a pGEMT-easy vector, sequenced and subsequently sub-cloned in pYES2 yeast shuttle vector. Positive clones were confirmed by colony PCR, restriction digestion and by sequencing. We found that putative PiMgT1 and PiMgT2 sequence was 1938 and 1923 bp long, respectively, with ATG as a start, TGA and TAG as stop codon, respectively (Supplementary Figures S1, S2).
Phylogenetic and Homology Analysis
It was found that both the putative PiMgT1 and PiMgT2 has all important motif, domains, and sites which are important for a protein to be defined as a member of CorA family. Both the putative PiMgT1 (361–598) and PiMgT2 (293–546) bears CorA domain. In case of PiMgT1 CorA domain was found to be conserved between 351 amino acid to 597 amino acid whereas, in case of PiMgT2 CorA was found to be between 301 amino acid and 539 amino acid. Both Mg transporters were found to be clustered among fungal CorA family members nearer to basidiomycetes (Figure 4). Multiple alignments analysis of PiMgT1 and PiMgT2 was performed which revealed that both of them have conserved ‘GMN’ signature sequence which is required to be defined as a Mg transporter (Figure 5).
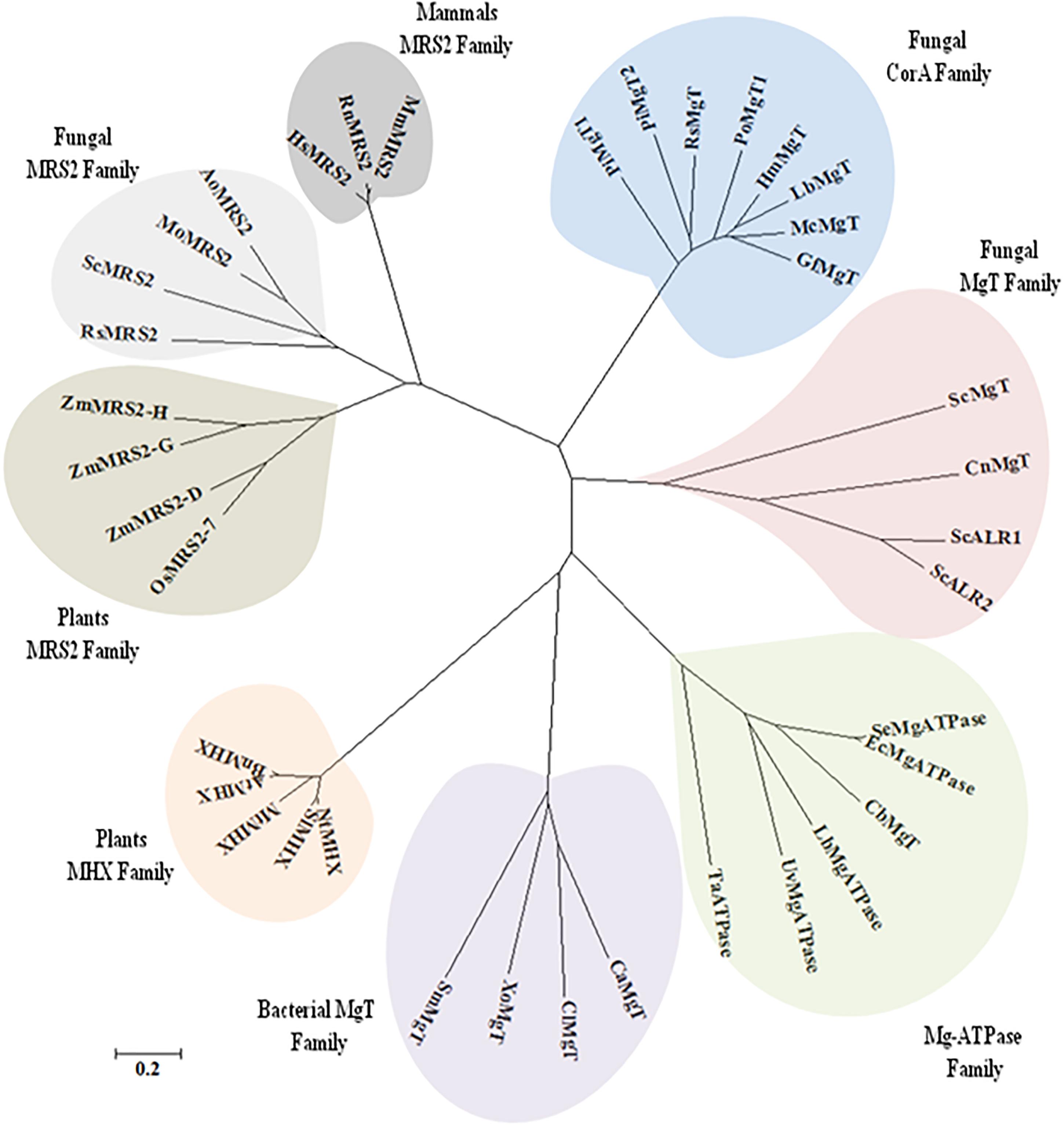
Figure 4. The phylogeny tree was inferred by using the maximum likelihood method based on the JTT matrix-based model. The tree with the highest log likelihood (–6494.5969) is shown. Boot strap value of 500 was taken in the tree construction. Initial tree(s) for the heuristic search were obtained automatically by applying Neighbor-Join and BioNJ algorithms to a matrix of pair wise distances estimated using a JTT model, and then selecting the topology with superior log likelihood value. The tree is drawn to scale, with branch lengths measured in the number of substitutions per site. The analysis involved 44 amino acid sequences. All positions containing gaps and missing data were eliminated. There were a total of 99 positions in the final dataset. Evolutionary analyses were conducted using MEGA7.
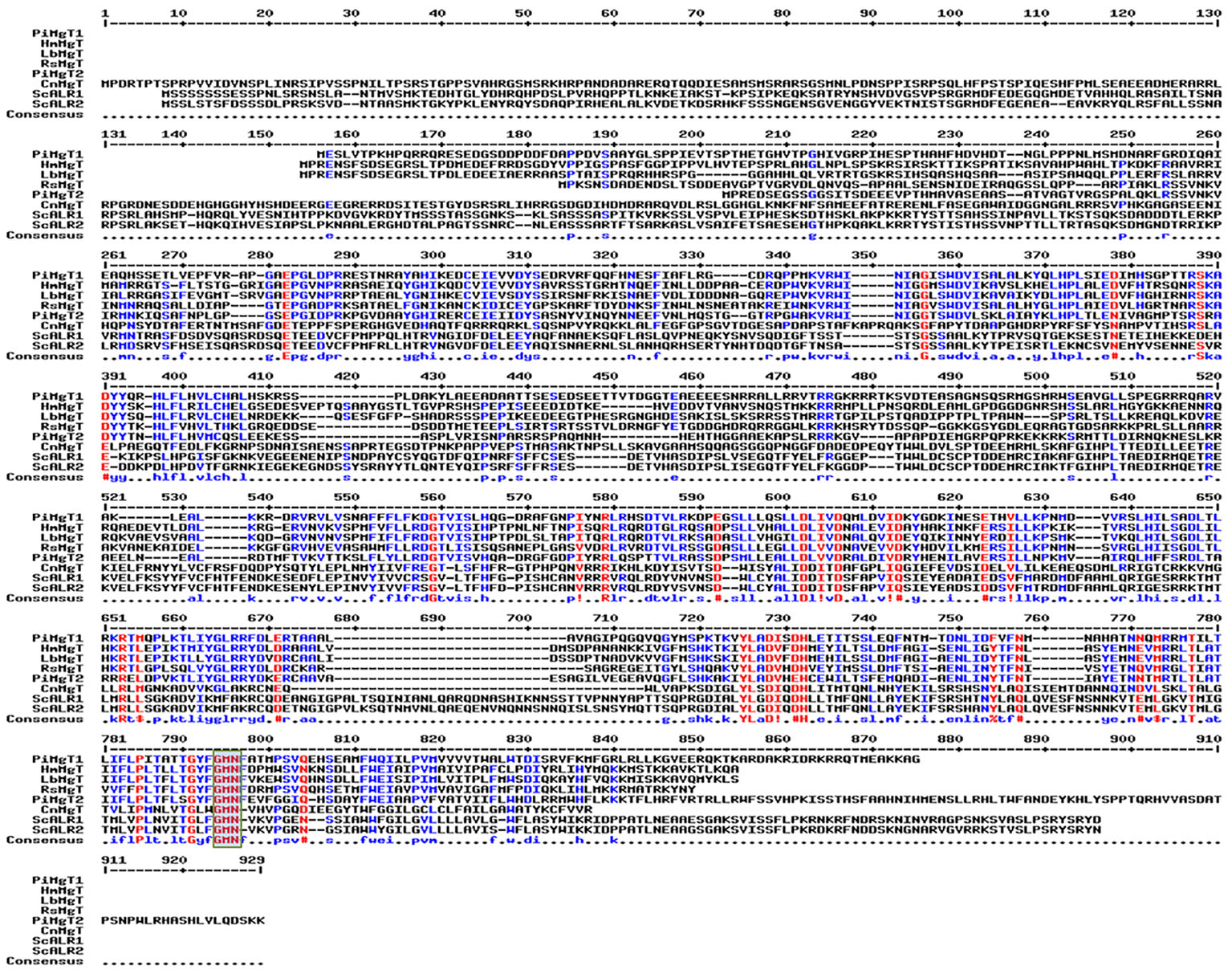
Figure 5. Multiple alignment analysis. Multiple alignments analysis of PiMgT1 and PiMgT2 was performed using MultAlign tool with six homologs from closely related fungi. The analysis revealed conserved ‘GMN’ signature sequence (highlighted in rectangular blue color box) which is required to be designated as an Mg transporter.
Our BLASTX analysis of putative PiMgT1 demonstrated that putative Mg transporter has approximately 44% identity with Mg transporter of Laccaria bicolor from the division of basidiomycete. Whereas, it has 38% similarity with the plant A. thaliana. Further, we found that, this putative Mg transporter has 36% and 28% similarity with Cyanobacterium aponinum (bacteria) and Homo sapiens, respectively (Table 3).
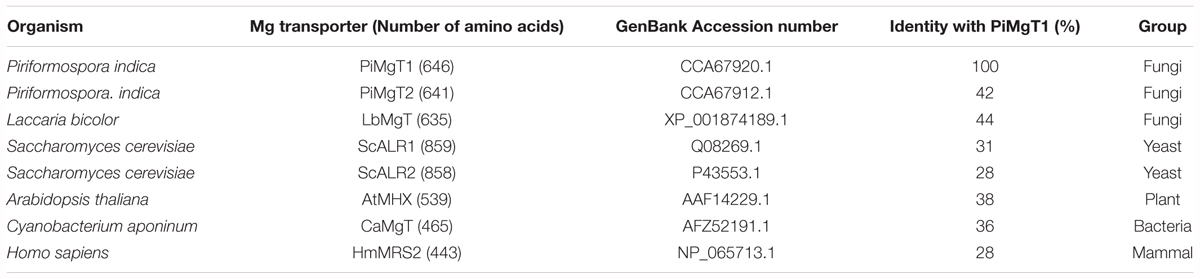
Table 3. Summary of amino acid identity (%) between P. indica Mg transporter PiMgT1 and other fungal, plant and animal magnesium transporters.
Complementation Assay
PiMgT1 Functionally Complement Mg Transporter Mutant
We have analyzed functional expression of putative PiMgT1 and PiMgT2 in Mg transporter mutant. Complementation assay was performed using the yeast mutant CM66, which lacks ALR1 and ALR2 gene responsible for Mg transportation, therefore, cannot grow on standard medium containing less than 4 mM Mg2+. We have found that complemented mutant has restored good growth in case of PiMgT1 as compared to the PiMgT2. We have observed comparable growth of mutant transformed with PiMgT1 with the WT when grown on 10 μM, 100 μM, 1 mM, 4 mM, and 10 mM Mg. Importantly, mutant transformed with empty vector and mutant transformed with PiMgT2 did not show growth on these Mg concentration (Figures 6A–E). However, a growth was observed in case of all the four strains when the concentration was increased upto 100 mM Mg (Figure 6F). We have also observed growth in case of all the strains when grown on YPD containing 4 mM Mg which was used as a control (Figure 6G).
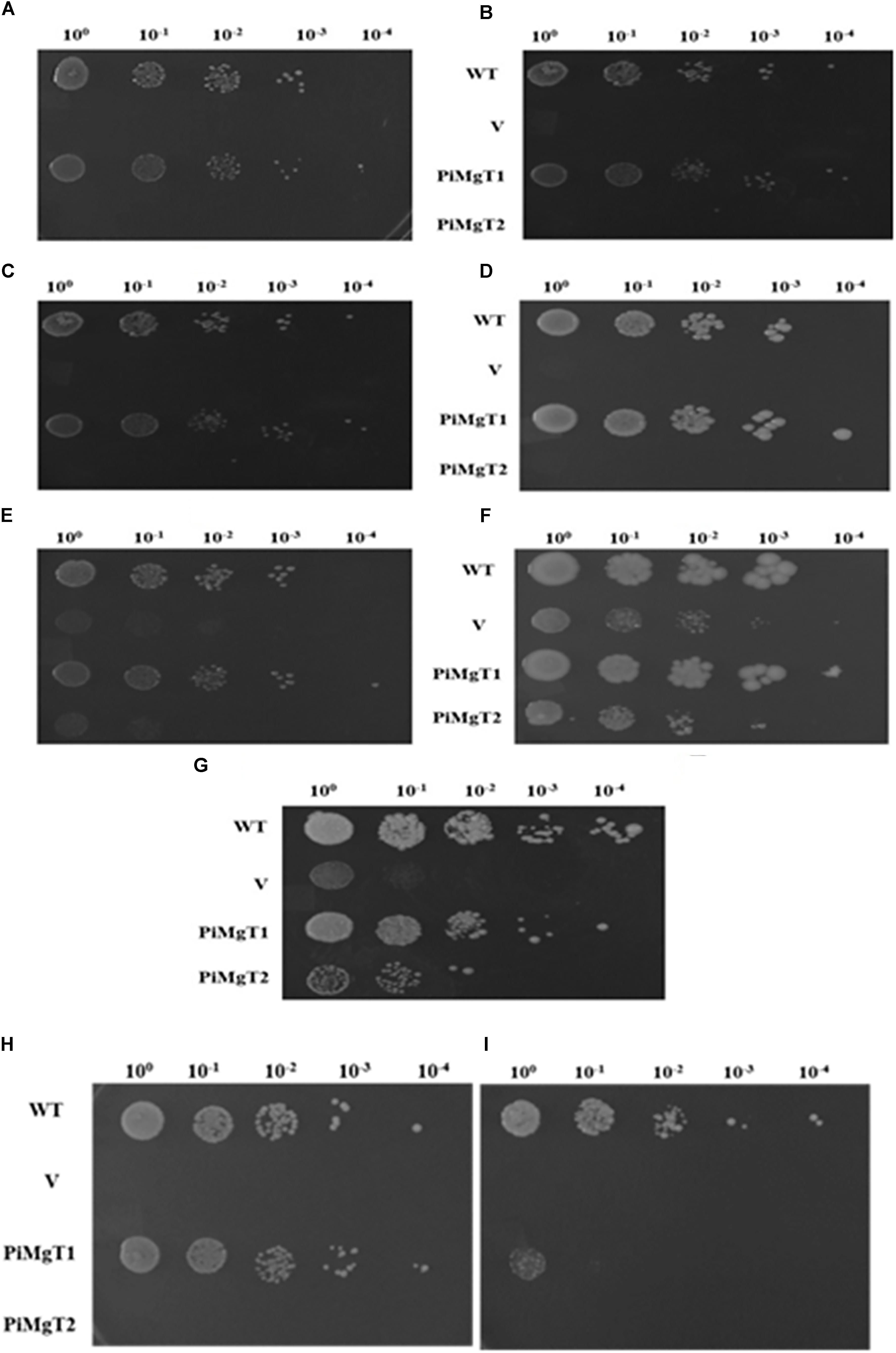
Figure 6. Complementation assay. Growth complementation assay in comparison of WT of the mutant S. cerevisiae strain CM66 transformed individually with plasmid vector only (V), PiMgT1 and PiMgT2 indicated and serially dilution of each strain was spotted on SC-URA medium containing different concentration of Mg (A) 10 μM (B) 100 μM (C) 1 mM (D) 4 mM (E) 10 mM (F) 100 mM (G) Control on YPD. Cobalt resistance assay: cobalt resistance assay in comparison of WT of the mutants S. cerevisiae strain CM66 which lacks Mg transport systems was transformed with plasmids vector only (V) PiMgT1 and PiMgT2 indicated and serially dilution of each strain was spotted on SC-URA medium containing different concentration of CoCl2 (H) 100 μM CoCl2 (I) 500 μM CoCl2 and fixed amount of 100 mM MgCl2.
Cobalt Resistance Assay
It has been reported that CorA family genes helps the cells in Mg uptake but also provides resistance against heavy metal like cobalt. Hence, to confirm the cobalt resistance capacity of both the genes, cobalt resistance assay was performed. Each complemented strain were grown on two different concentration of cobalt i.e., 100 and 500 μM and fixed concentration of MgCl2 (100 mM). We found that both WT and mutant transformed with PiMgT1 were able to grow on 100 and 500 μM CoCl2, whereas, mutant transformed with the vector only and with PiMgT2 did not show any growth on any of the concentration of CoCl2 (Figures 6H,I).
Growth Assay and Functional Characterization
We have observed that PiMgT1 restored the growth of mutant and showed comparable growth with that of WT when grown at low concentration of Mg (0.1, 1, 4, 10, and 100 mM) (Figures 7A,B). We have also observed a slow growth in case of mutant transformed with the PiMgT2 as compared to the WT and mutant transformed with the PiMgT1 (Figure 7C). Our result indicated that mutant was not able to grow at low Mg concentration (0.1, 1, 4, and 10 mM) but showed very slow growth when standard media was supplemented with 100 mM Mg (Figure 7D).
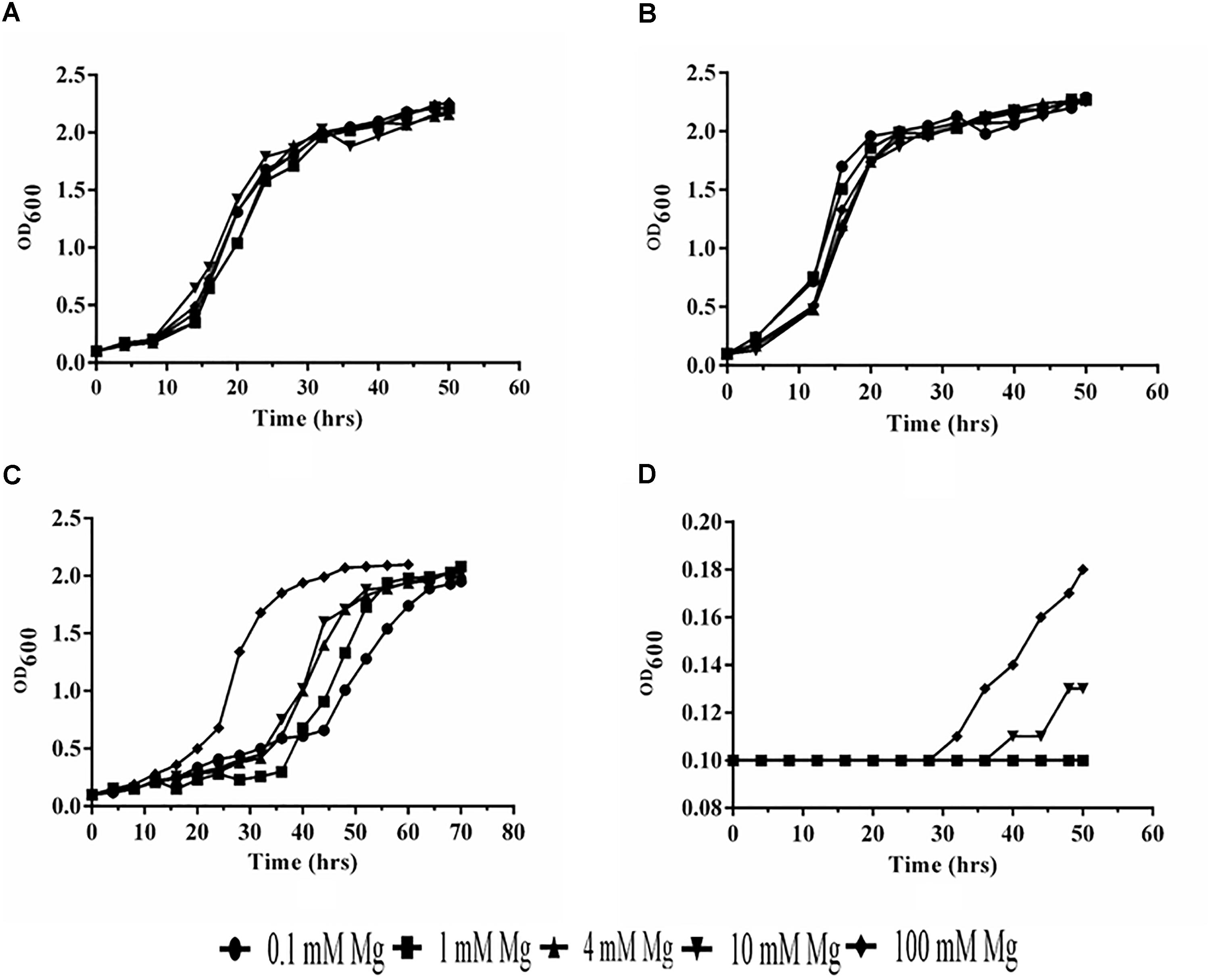
Figure 7. Analysis of growth. (A) Mutant transformed with PiMgT1 (B) WT (C) mutant transformed with the PiMgT2 (D) Mutant only.
In case of mutant complemented with the PiMgT1, total intracellular Mg content was found to be two fold after 20 min as compared to the mutant and this difference was found to be statistically significant (p ≤ 0.05). We have also found more than four fold increase in the Mg contents of complemented mutant after 1 h as compared to the mutant (Figure 8).
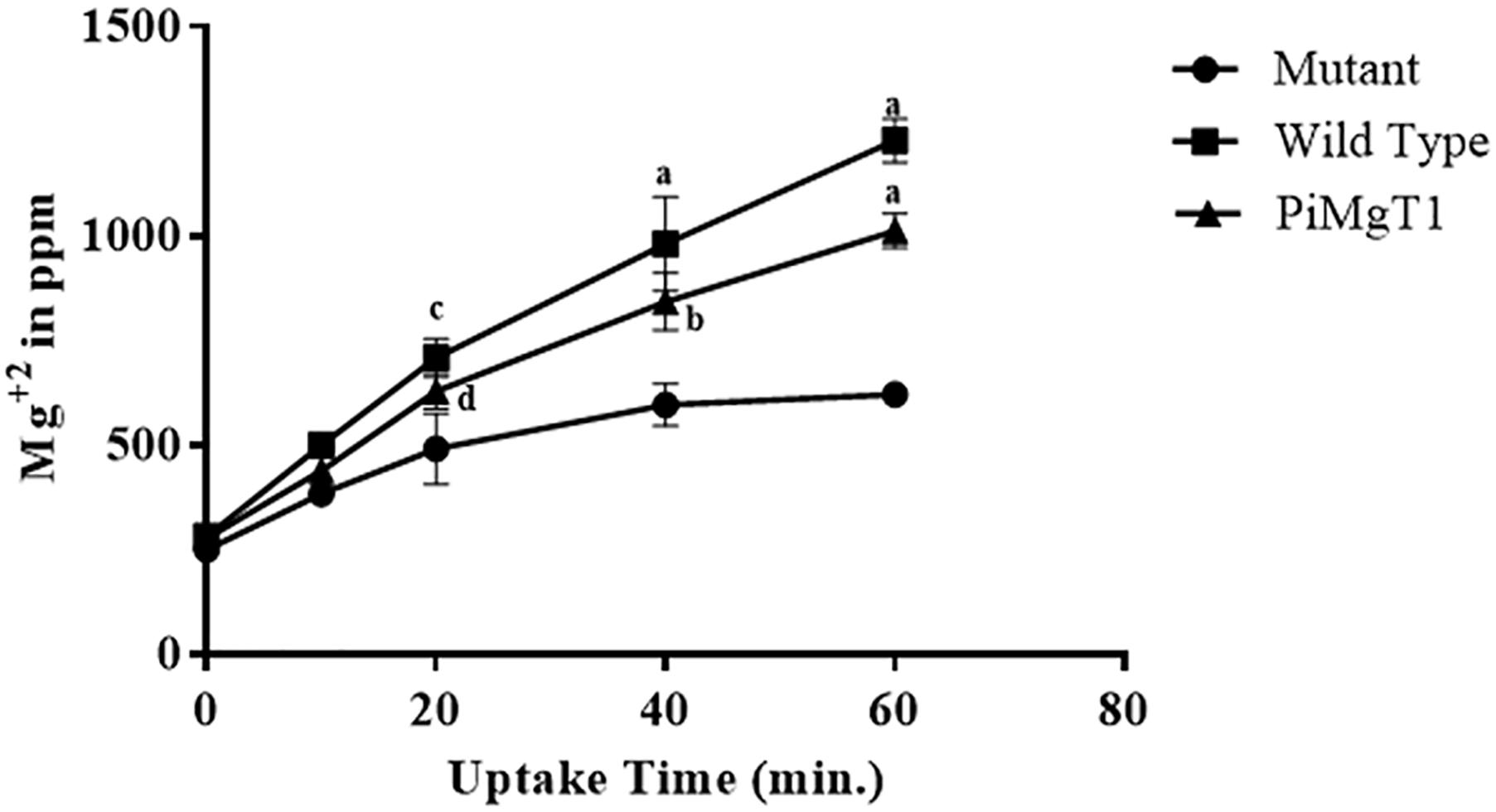
Figure 8. Uptake of Mg. Uptake of Mg by mutant, WT, and mutant complemented with PiMgT1. Significant differences (p-value) were calculated with respect to mutant using two way ANOVA dp ≤ 0.05, cp ≤ 0.01, bp ≤ 0.005, ap ≤ 0.001.
Discussion
Endophytic association of P. indica has shown as beneficial tool for the host plant to survive under abiotic and biotic stresses (Trivedi et al., 2013; Jogawat et al., 2016; Narayan et al., 2017). Additionally, P. indica can grow axenically, can colonized dicots, monocots and other medicinal plants which cannot be colonized by the AMF, therefore this fungus has been termed as plant probiotic (Aschheim et al., 2005).
Though reports are available which shows that AMF benefited the host plant during colonization under Mg limited conditions (Wu et al., 2006; Doubková et al., 2012; Zhang et al., 2015). However, no information is available related to the functional characterization of the Mg transporter of the AMF. In this study, Mg transporter from P. indica has been identified, isolated, and functionally characterized and also the role of P. indica in the plant growth and development has been investigated. When plants colonized with the P. indica and grown under Mg-limited condition, were found to be healthy and greener as compared to the non-colonized plants which showed retarded growth. Zhang et al. (2015) have reported that high concentration of Mg results in the low colonization of the AMF, in contrast to this report, we have found 70% colonization at 15 dpi. at all concentrations of Mg including 25 mM Mg, indicating that colonization of P. indica is independent of concentration of Mg. In order to check the impact of P. indica on plants, grown under Mg-limited condition, growth parameters were measured. We found that P. indica colonized plants have higher biomass, more number of leaves as compared to the non- colonized plants when grown at 4 mM Mg. Similarly, P. indica colonized plants found to have high amount of chlorophyll a, b and total chlorophyll at 4 mM Mg concentration as compared non- colonized plants. Similar observations were made by Zhang et al. (2015). They have found that when citrus plant colonized with the AMF Funneliformis mosseae under Mg-limited condition, AMF inoculation results in the increased biomass and chlorophyll contents of the inoculated plants as compared to the non-inoculated plants. Thus this study support our data. We proposed that, the long roots of non-colonized plants were due to the inaccessibility of Mg to the plants. Whereas, under the presence of P. indica the root length was found to be shorter which indicates that fungus is helping plants in the uptake of Mg from media. In case of colonized plants, leaves numbers were found to be high at 4 mM Mg as compared to the non-colonized plants. This indicates that the presence of an optimal amount i.e., 4 mM Mg in the medium enhances the leaves number. However, 25 mM of Mg found to be toxic to the plants as they found to be short, less number of leaves, chlorophyll contents with less biomass as compared to the plants grown at 4 mM Mg.
Further, to identify Mg transporter of P. indica, we have performed BLAST analysis by using Mg transporter of S. cerevisiae (ALR1-p) as a template against the P. indica genome. Our BLASTP analysis revealed that P. indica genome contains two putative Mg transporter showing significant degree of identity with the S. cerevisiae Mg transporter. Similarly, several studies proved that CorA-like proteins may represent the main transport systems in other organisms including eukaryotes like yeast, animals, as well as plants (Bui et al., 1999; Schock et al., 2000; Graschopf et al., 2001; Li et al., 2001; Kolisek et al., 2003). Our bioinformatics analysis revealed that both the putative Mg transporter of P. indica belongs to PIRI_contig_0025 (Accession no CCA67920.1 and CCA67912.1) and it falls under the range of MIT superfamily (Knoop et al., 2005). Further, we observed significant similarities in homology and phylogeny of deduced amino acid sequence of both putative Mg transporters (PiMgT1 and PiMgT2) of P. indica with known Mg transporter of prokaryotes, fungi, higher plants and animals. Phylogenetic analysis with diverse group of organisms indicates that both the putative Mg transporters are more closely related to high-affinity Mg transporter of fungi and is far distinct from plants, animals and prokaryotes. Both the Mg transporters of P. indica were clustered among fungal CorA family members nearer to basidiomycetes. We observed that PiMgT1 have homology with other fungi, animals, plants and bacteria. When CLUSTALW analysis with the other group of fungus was performed, we found the presence of signature motif GMN (Glycine, Methionine, and Asparagine, responsible for the Mg transportation) in Mg transporter of P. indica, similarly, presence of GMN motif was also reported in case of ALR proteins of S. cerevisiae, therefore, this study support our data (MacDiarmid and Gardner, 1998).
For complementation assay, we have used Mg double mutant of S. cerevisiae lacking Mg transporter gene (Li et al., 2001). CM66 mutant was individually transformed with both transporters PiMgT1 and PiMgT2. Spot assay confirmed that PiMgT1 complemented the mutant the most than that of PiMgT2. Further, WT and mutant transformed with PiMgT1 have shown typical diauxic growth in comparison to mutant transformed with the PiMgT2. Interestingly, only PiMgT1 complemented strain showed similar growth pattern like WT whereas, mutant transformed with PiMgT2 showed retarded growth. Hence, these results signify that PiMgT1 restores growth defects. Further, we observed that only complemented PiMgT1 and WT were able to grow on the media containing 10 μM Mg whereas, complemented PiMgT2 and mutant was not be able to grow at the same concentration. When concentration of Mg in the media increased from 10 to 100 mM Mg, both PiMgT2 and mutant showed good growth. A similar kind of study was reported in case of A. thaliana Mg transporter, MRS2/MGT family proteins and transformed in CM66 mutant, it showed similar kind of growth pattern and thus support our data (Saito et al., 2013).
Our bioinformatics data also showed that both putative Mg transporters belongs to CorA family, CorA family members are responsible for providing resistance against cobalt to the cells as well as for transportation of Mg across the membrane. Hence, we also performed cobalt resistance assay and found very interesting result that upto 500 μM cobalt concentration only WT and PiMgT1 was able to grow whereas, mutant and mutant transformed with PiMgT2 did not grow on the same concentration. Hence, it was confirmed that PiMgT1 belongs to the CorA family.
We have performed Mg uptake assay using WT, complemented mutant with PiMgT1 and mutant. Our ED-XRF analysis showed that amount of Mg content in case of WT and complemented mutant, increased with the time and both the strains showed the same pattern of Mg uptake whereas, mutant showed the negligible amount of Mg content as compared to WT and complemented strains. Hence, our ED-XRF analysis confirmed that PiMgT1 is helping the mutant to restore the Mg uptake ability. Similarly, Li et al. (2001) found an increase in the cellular Mg content after 60-min of uptake period in case of mutant transformed with plant Mg transporter AtMGT10, thus supporting our data.
Our study confirmed that P. indica has plant growth promoting activities and facilitating the plants to attain higher biomass, number of leaves as well as increased chlorophyll contents during Mg deficiency. The current study highlights the importance of P. indica Mg transporter (PiMgT1) for the improvement of Mg nutrition to the host plants when grown under Mg deprived condition. As P. indica has been termed as a “Plant Probiotic,” therefore, we propose that P. indica could be a better candidate for sustainable agriculture system to improve crop production in Mg-deficient agriculture field. Our present study will open new vista in order to apply P. indica as bio-fertilizer in the Mg deprived field to improve crop yield.
Author Contributions
DP designed and performed the experiments. MB, NV, AS, and ON performed the experiments. AJ and MD provided chemicals. DP, AJ, and MD wrote the manuscript. MD and AJ initiated and supervised the project.
Conflict of Interest Statement
The authors declare that the research was conducted in the absence of any commercial or financial relationships that could be construed as a potential conflict of interest.
Acknowledgments
We are very thankful to Prof. Keitaro Tanoi, University of Tokyo, Japan for providing mutant CM66 and Wild Type CM52. AS and ON are thankful to Council of Scientific and Industrial Research, and Indian Council of Medical Research, Government of India, respectively, for providing research fellowships. NV and DP are thankful to Jawaharlal Nehru University for providing the research fellowships. AJ and MD are also acknowledging JNU for providing DST-PURSE-II grant.
Supplementary Material
The Supplementary Material for this article can be found online at: https://www.frontiersin.org/articles/10.3389/fmicb.2018.03231/full#supplementary-material
Footnotes
- ^ http://genes.mit.edu/GENSCAN.html
- ^ http://web.expasy.org/translate/
- ^ http://www.ncbi.nlm.nih.gov/BLAST/
- ^ http://www.expasy.ch/prosite/
- ^ http://www.ncbi.nlm.nih.gov
- ^ https://www.ebi.ac.uk/Tools/msa/clustalo/
- ^ http://www.megasoftware.net/
References
Aschheim, K., Cervoni, N., DeFrancesco, L., Hare, P., and Taroncher-Oldenburg, G. (2005). Plant probiotic (news & views). Nat. Biotech 23:1241. doi: 10.1038/nbt1005-1241
Baltruschat, H., Fodor, J., Harrach, B. D., Niemczyk, E., Barna, B., Gullner, G., et al. (2008). Salt tolerance of barley induced by the root endophyte Piriformospora indica is associated with a strong increase in antioxidants. New Phytol. 180, 501–510. doi: 10.1111/j.1469-8137.2008.02583.x
Bécard, G., and Fortin, J. A. (1988). Early events of vesicular–arbuscular mycorrhiza formation on Ri T-DNA transformed roots. New Phytol. 108, 211–218. doi: 10.1111/j.1469-8137.1988.tb03698.x
Bui, D. M., Gregan, J., Jarosch, E., Ragnini, A., and Schweyen, R. J. (1999). The bacterial magnesium transporter CorA can functionally substitute for its putative homologue Mrs2p in the yeast inner mitochondrial membrane. J. Biol. Chem. 274, 20438–20443. doi: 10.1074/jbc.274.29.20438
Cowan, J. A. (1995). “Introduction to the biological chemistry of the magnesium ion,” in The Biological Chemistry of Magnesium, ed. J. A. Cowan (New York, NY: VCH Publishers), 1–23.
Dickson, S., and Smith, S. E. (1998). “Evaluation of vesicular-arbuscular mycorrhizal colonisation by staining,” in Mycorrhiza Manual (Heidelberg: Springer), 77–83. doi: 10.1007/978-3-642-60268-9_5
Doubková, P., Suda, J., and Sudová, R. (2012). The symbiosis with arbuscular mycorrhizal fungi contributes to plant tolerance to serpentine edaphic stress. Soil Biol. Biochem. 44, 56–64. doi: 10.1016/j.soilbio.2011.09.011
Elin, R. J. (1994). Magnesium: the fifth but forgotten electrolyte. Am. J. Clin. Pathol. 102, 616–622. doi: 10.1093/ajcp/102.5.616
Gardner, R. C. (2003). Genes for magnesium transport. Curr. Opin. Plant Biol. 6, 263–267. doi: 10.1016/S1369-5266(03)00032-3
Gietz, R. D., Schiestl, R. H., Willems, A. R., and Woods, R. A. (1995). Studies on the transformation of intact yeast cells by the LiAc/SS-DNA/PEG procedure. Yeast 11, 355–360. doi: 10.1002/yea.320070307
Graschopf, A., Stadler, J. A., Hoellerer, M. K., Eder, S., Sieghardt, M., Kohlwein, S. D., et al. (2001). The yeast plasma membrane protein Alr1 controls Mg+2 homeostasis and is subject to Mg+2-dependent control of its synthesis and degradation. J. Biol. Chem. 276, 16216–16222. doi: 10.1074/jbc.M101504200
Hill, T. W., and Kafer, E. (2001). Improved protocols for Aspergillus minimal medium: trace element and minimal medium salt stock solutions. Fungal Genet. Newslett. 48, 20–21. doi: 10.4148/1941-4765.1173
Inoue, H., Nojima, H., and Okayama, H. (1990). High efficiency transformation of Escherichia coli with plasmids. Gene 96, 23–28. doi: 10.1016/0378-1119(90)90336-P
Jogawat, A., Saha, S., Bakshi, M., Dayaman, V., Kumar, M., Dua, M., et al. (2013). Piriformospora indica rescues growth diminution of rice seedlings during high salt stress. Plant Signal. Behav. 8:e26891. doi: 10.4161/psb.26891
Jogawat, A., Vadassery, J., Verma, N., Oelmüller, R., Dua, M., Nevo, E., et al. (2016). PiHOG1, a stress regulator MAP kinase from the root endophyte fungus Piriformospora indica, confers salinity stress tolerance in rice plants. Sci. Rep. 6:36765. doi: 10.1038/srep36765
Johnson, J. M., Sherameti, I., Ludwig, A., Nongbri, P. L., Sun, C., Lou, B., et al. (2011). Protocols for Arabidopsis thaliana and Piriformospora indica co-cultivation–a model system to study plant beneficial traits. Endocytobiosis Cell Res. 21, 101–113.
Knoop, V., Groth-Malonek, M., Gebert, M., Eifler, K., and Weyand, K. (2005). Transport of magnesium and other divalent cations: evolution of the 2-TM-GxN proteins in the MIT superfamily. Mol. Genet. Genom. 274, 205–216. doi: 10.1007/s00438-005-0011-x
Kolisek, M., Zsurka, G., Samaj, J., Weghuber, J., Schweyen, R. J., and Schweigel, M. (2003). Mrs2p is an essential component of the major electrophoretic Mg2+ influx system in mitochondria. EMBO J. 22, 1235–1244. doi: 10.1093/emboj/cdg122
Kumar, M., Yadav, V., Tuteja, N., and Johri, A. K. (2009). Antioxidant enzyme activities in maize plants colonized with Piriformospora indica. Microbiology 155, 780–790. doi: 10.1099/mic.0.019869-0
Li, L., Tutone, A. F., Drummond, R. S., Gardner, R. C., and Luan, S. (2001). A novel family of magnesium transport genes in Arabidopsis. Plant Cell 13, 2761–2775. doi: 10.1105/tpc.13.12.2761
MacDiarmid, C. W., and Gardner, R. C. (1998). Over expression of the Saccharomyces cerevisiae magnesium transport system confers resistance to aluminum ion. J. Biol. Chem. 273, 1727–1732. doi: 10.1074/jbc.273.3.1727
McGonigle, T. P., Miller, M. H., Evans, D. G., Fairchild, G. L., and Swan, J. A. (1990). A new method which gives an objective measure of colonization of roots by vesicular—arbuscular mycorrhizal fungi. New Phytol. 115, 495–501. doi: 10.1111/j.1469-8137.1990.tb00476.x
Murashige, T., and Skoog, F. (1962). A revised medium for rapid growth and bio assays with tobacco tissue cultures. Physiol. Plant 15, 473–497. doi: 10.1111/j.1399-3054.1962.tb08052.x
Narayan, O. P., Verma, N., Singh, A. K., Oelmüller, R., Kumar, M., Prasad, D., et al. (2017). Antioxidant enzymes in chickpea colonized by Piriformospora indica participate in defense against the pathogen Botrytis cinerea. Sci. Rep. 7:13553. doi: 10.1038/s41598-017-12944-w
Perring, L., Nicolas, M., Andrey, D., Rime, C. F., Richoz-Payot, J., Dubascoux, S., et al. (2017). Development and validation of an ED-XRF method for the fast quantification of mineral elements in dry pet food samples. Food Anal. Methods 10, 1469–1478. doi: 10.1007/s12161-016-0695-z
Pham, G. H., Singh, A., Malla, R., Kumari, R., Prasad, R., Sachdev, M., et al. (2008). “Interaction of Piriformospora indica with diverse microorganisms and plants,” in Plant Surface Microbiology. Heidelberg: Springer, 237–265.
Phillips, J. M., and Hayman, D. S. (1970). Improved procedures for clearing roots and staining parasitic and vesicular-arbuscular mycorrhizal fungi for rapid assessment of infection. Trans. Br. Mycol. Soc. 55, 158–161. doi: 10.1016/S0007-1536(70)80110-3
Porra, R. J., Thompson, W. A., and Kriedemann, P. E. (1989). Determination of accurate extinction coefficients and simultaneous equations for assaying chlorophylls a and b extracted with four different solvents: verification of the concentration of chlorophyll standards by atomic absorption spectroscopy. Biochim. Biophys. Acta Bioenerg. 975, 384–394. doi: 10.1016/S0005-2728(89)80347-0
Saito, T., Kobayashi, N. I., Tanoi, K., Iwata, N., Suzuki, H., Iwata, R., et al. (2013). Expression and functional analysis of the CorA-MRS2-ALR-type magnesium transporter family in rice. Plant Cell Physiol. 54, 1673–1683. doi: 10.1093/pcp/pct112
Schock, I., Gregan, J., Steinhauser, S., Schweyen, R., Brennicke, A., and Knoop, V. (2000). A member of a novel Arabidopsis thaliana gene family of candidate Mg2+ ion transporters complements a yeast mitochondrial group II intron splicing mutant. Plant J. 24, 489–501. doi: 10.1111/j.1365-313X.2000.00895.x
Shaul, O. (2002). Magnesium transport and function in plants: the tip of the iceberg. Biometals 15, 307–321. doi: 10.1023/A:1016091118585
Sherameti, I., Tripathi, S., Varma, A., and Oelmüller, R. (2008). The root-colonizing endophyte Pirifomospora indica confers drought tolerance in Arabidopsis by stimulating the expression of drought stress–related genes in leaves. Mol. Plant Microbe Interact. 21, 799–807. doi: 10.1094/MPMI-21-6-0799
Sun, C., Johnson, J. M., Cai, D., Sherameti, I., Oelmüller, R., and Lou, B. (2010). Piriformospora indica confers drought tolerance in Chinese cabbage leaves by stimulating antioxidant enzymes, the expression of drought-related genes and the plastid-localized CAS protein. J. Plant Physiol. 167, 1009–1017. doi: 10.1016/j.jplph.2010.02.013
Tamura, K., Peterson, D., Peterson, N., Stecher, G., Nei, M., and Kumar, S. (2011). MEGA5: molecular evolutionary genetics analysis using maximum likelihood, evolutionary distance, and maximum parsimony methods. Mol. Biol. Evol. 28, 2731–2739. doi: 10.1093/molbev/msr121
Trivedi, D. K., Bhatt, H., Pal, R. K., Tuteja, R., Garg, B., Johri, A. K., et al. (2013). Structure of RNA-interacting cyclophilin a-like protein from Piriformospora indica that provides salinity-stress tolerance in plants. Sci. Rep. 3:3001. doi: 10.1038/srep03001
Vadassery, J., Tripathi, S., Prasad, R., Varma, A., and Oelmüller, R. (2009). Monodehydroascorbate reductase 2 and dehydroascorbate reductase 5 are crucial for a mutualistic interaction between Piriformospora indica and Arabidopsis. J. Plant Physiol. 166, 1263–1274. doi: 10.1016/j.jplph.2008.12.016
Verma, S., Varma, A., Rexer, K.-H., Hassel, A., Kost, G., Sarbhoy, A., et al. (1998). Piriformospora indica, gen. et sp. nov., a new root-colonizing fungus. Mycologia 60, 896–903. doi: 10.2307/3761331
Waller, F., Achatz, B., Baltruschat, H., Fodor, J., Becker, K., Fischer, M., et al. (2005). The endophytic fungus Piriformospora indica reprograms barley to salt-stress tolerance, disease resistance, and higher yield. Proc. Natl. Acad. Sci. U.S.A. 102, 13386–13391. doi: 10.1073/pnas.0504423102
Williams, R. J. P. (1993). “Magnesium: an introduction to its biochemistry,” in Magnesium and the Cell, ed. N. J. Birch (Boston, MA: Academic Press), 16–30.
Wu, Q. S., Xia, R. X., and Zou, Y. N. (2006). Reactive oxygen metabolism in mycorrhizal and non-mycorrhizal citrus (Poncirus trifoliata) seedlings subjected to water stress. J. Plant Physiol. 163, 1101–1110. doi: 10.1016/j.jplph.2005.09.001
Yadav, V., Kumar, M., Deep, D. K., Kumar, H., Sharma, R., Tripathi, T., et al. (2010). A phosphate transporter from the root endophytic fungus Piriformospora indica plays a role in phosphate transport to the host plant. J. Biol. Chem. 285, 26532–26544. doi: 10.1074/jbc.M110.111021
Zhang, F., Du, P., Song, C. X., and Wu, Q. S. (2015). Alleviation of mycorrhiza to magnesium deficiency in trifoliate orange: changes in physiological activity. Emir. J. Food Agric. 27, 763–769. doi: 10.9755/ejfa.2015.05.240
Keywords: Piriformospora indica, magnesium transporter, Arabidopsis thaliana, high affinity transporter, plant growth activity
Citation: Prasad D, Verma N, Bakshi M, Narayan OP, Singh AK, Dua M and Johri AK (2019) Functional Characterization of a Magnesium Transporter of Root Endophytic Fungus Piriformospora indica. Front. Microbiol. 9:3231. doi: 10.3389/fmicb.2018.03231
Received: 01 October 2018; Accepted: 12 December 2018;
Published: 09 January 2019.
Edited by:
Pankaj Kumar Arora, Babasaheb Bhimrao Ambedkar University, IndiaReviewed by:
Ravindra Nath Kharwar, Banaras Hindu University, IndiaMariusz Cycoń, Medical University of Silesia, Poland
Copyright © 2019 Prasad, Verma, Bakshi, Narayan, Singh, Dua and Johri. This is an open-access article distributed under the terms of the Creative Commons Attribution License (CC BY). The use, distribution or reproduction in other forums is permitted, provided the original author(s) and the copyright owner(s) are credited and that the original publication in this journal is cited, in accordance with accepted academic practice. No use, distribution or reproduction is permitted which does not comply with these terms.
*Correspondence: Meenakshi Dua, bWVlbmFrc2hpNzJAaG90bWFpbC5jb20= Atul Kumar Johri, YWtqb2hyaTE0QHlhaG9vLmNvbQ==