- 1Department of Food Science, University of Arkansas, Fayetteville, AR, United States
- 2Center for Food Safety, University of Arkansas, Fayetteville, AR, United States
- 3TetraClean Systems LLC, Omaha, NE, United States
Currently, the most utilized antimicrobial in processing facilities is peracetic acid, PAA; however, this chemical is increasingly recognized as a hazard to human health. Preliminary evidence suggests that ozone, when introduced in a specific manner, can reduce the noxious nature of PAA. Therefore, the objective of the current study was to evaluate the efficacy of TetraClean Systems aqueous ozone, O3, in combination with PAA as an antimicrobial spray on whole chicken carcasses. This trial used 70 whole hen carcasses (7 treatments; 10 replications) that were inoculated in a 400 mL cocktail containing Salmonella, Escherichia coli, and Campylobacter (107 CFU/mL) and allowed to adhere for 60 min at 4°C for a final concentration of 105 to 106 CFU/g. The experimental 5 s (4×) spray treatments included: a no treatment negative control, TW; TW + O3 (10 ppm), TW + PAA (50 ppm), TW + PAA (500 ppm), TW + O3 + PAA (50 ppm), and TW + O3 + PAA (500 ppm). During treatment application, ambient PAA vapor was measured with a ChemDAQ Safecide PAA vapor sensor. After treatment, carcasses were immediately rinsed in 400 mL of nBPW for 2 min. Following rinsing, the dot method was utilizing for enumeration with 10 μL of rinsate being serially diluted, plated on XLD and mCCDA agar, and incubated aerobically at 37°C for 24 h or microaerophilically at 42°C for 48 h. Log-transformed counts were analyzed using ANOVA in JMP 14.0. Means were separated using Tukey’s HSD when P ≤ 0.05. There was a significant treatment effect among Salmonella, E. coli, and Campylobacter counts, and a significant treatment effect among ambient PAA (P < 0.05). TW + O3 + PAA (500 ppm), reduced Salmonella significantly compared to TW (5.71 and 6.30 log CFU/g). Furthermore, TW + PAA (500 ppm), reduced the presence of E. coli significantly compared to TW or no treated control (5.57 and 6.18 log CFU/g). Also, TW + PAA (50 ppm), TW + PAA (500 ppm), and TW + O3 + PAA (500 ppm) significantly reduced Campylobacter compared to carcasses not treated (4.80, 4.81, and 4.86 log CFU/g). Lastly, the addition of ozone significantly reduced the ambient PAA when O3 was added to 500 ppm of PAA, as TW + O3 + PAA (500 ppm) produced less ambient PAA than TW + PAA (500 ppm) (0.052 and 0.565 ppm). In conclusion, the addition of ozone to PAA may demonstrated the ability to effectively reduce ambient PAA, thus increasing employee safety.
Introduction
Currently, the United States poultry industry utilizes peroxyacetic acid, also known as peracetic acid (PAA), to decontaminate poultry within poultry processing facilities. The disinfectant, PAA, is a product of the reaction between acetic acid and hydrogen peroxide. It is a colorless acid with a strong odor. The bactericidal effect of PAA is due to it being a strong oxidizing agent of the cell membrane and other cell components (Oyarzabal, 2005). However, this chemical is corrosive and unstable. PAA is one of the most common antimicrobials used in poultry processing facilities, as it is applied in the chillers (pre-chiller, chiller, and post-chiller), part dips, spray cabinets, in and out bird washes at concentrations typically ranging from 200 to 2,000 ppm; however, it has been known to be a hazard to human health (National Academies of Science [NAS], 2010). PAA is reported to be an irritant to the upper respiratory tract, eye, and skin (Merka and Urban, 1978; Fraser and Thorbinson, 1986; Janssen, 1989; Janssen and van Doorn, 1994). Direct contact in the eye and skin can be avoided if the proper personal protective equipment (PPE) is worn, but there are limited approaches to protect the upper respiratory tract from the vapors emitted from PAA (American Thoracic Society, 1996).
Currently, there is no OSHA (Occupational Safety and Health Administration) limit on the acute or long-term exposure limit of PAA during shifts for employees. However, other governing bodies have set limits and guidelines for the exposure of PAA vapor. In 2014, the American Conference Governmental Hygienists (ACGIH) set a threshold limit of 0.04 ppm as the 15-minute Short Term Exposure Limit (STEL; American Conference of Governmental Industrial Hygienists [ACGIH], 2016). Furthermore, the National Advisory Committee for Acute Exposure Guideline Levels for Hazardous Substances (NAC/AEGLL Committee), during an 8 h exposure time, set AEGL-1, 2, and 3 limits to 0.17, 0.51, and 1.3 ppm of PAA vapor, respectively (National Academies of Science [NAS], 2010), with firm limits of total exposure time for AEGL-1 and 2 limits at 0.17, and 0.51 ppm (National Academies of Science [NAS], 2010). An exposure at AEGL-1 produces noticeable discomfort, and irritation, with reversible effects upon removal from exposure site. AEGL-2 exposure produces irreversible or other long-lasting serious health conditions and may impair one’s ability to escape. Lastly, an AEGL-3 exposure results in life-threatening health conditions and can result in death. As of 2015, NIOSH published a draft Immediately Dangerous to Life or Health (IDLH) value for 0.64 ppm (National Institute for Occupational Safety and Health [NIOSH], 2015).
As there are no current strategies employed to reduce the ambient PAA in a processing facility, there is a significant need to develop and easily implement measures to prevent PAA vapor exposure. One novel approach is to utilize the commercial aqueous ozone product (ViriditecTM, TetraClean Systems LLC, Omaha, NE) to distribute aqueous ozone directly to PAA, as preliminary evidence suggests, the addition of ozone can reduce the noxious nature of PAA (data not shown).
Previously, chlorine was utilized as the primary sanitizer in processing facilities but has been replaced in the last decade with PAA. Studies have demonstrated that 85 ppm of PAA has the capability to reduce the incidence of Salmonella and Campylobacter by 92 and 43% on poultry carcasses when applied in a commercial poultry chiller (Bauermeister et al., 2008b). Whereas, 30 ppm of chlorine was only capable of reducing Salmonella and Campylobacter by 43 and 13%, when used in a poultry chiller (Bauermeister et al., 2008b). Furthermore, PAA has been shown to mitigate Staphylococcus spp., Listeria spp., and generic Escherichia coli more than 5-log CFU regardless of the food source being evaluated (Brinez et al., 2006). Bauermeister et al. (2008a) reported the reduction of Salmonella and Campylobacter to be greater in carcasses chilled in solutions containing 200 ppm of PAA compared to those chilled in 30 ppm chlorine, ≈1 log reduction. Therefore, it is imperative to mitigate the noxious nature of PAA without reducing the bactericidal effects of PAA in poultry processing facilities. Thus, it was the objective of the current experiment to evaluate the efficacy of a commercial aqueous ozone (O3) alone or in combination with PAA on reducing ambient PAA and poultry pathogens when applied as an antimicrobial spray on whole chicken carcasses.
Materials and Methods
ViriditecTM Aqueous Ozone Generation
TetraClean’s ViriditecTM aqueous ozone system has been characterized as a patented technology that utilizes Nanobubble Technology to combine water and ozone to yield aqueous ozone. In the current study, the system produced ozone gas which was injected into a water stream and further infused through the systems patented configuration and mixing technology. A Q46H/64 Dissolved Ozone Monitor (Analytical Technologies Industries, Collegeville, PA, United States) was utilized to measure the specific ozone levels generated from the ViriditecTM aqueous ozone system. The result was an aqueous ozone solution that contained 10 ppm of dissolved ozone in solution.
Carcass Procurement and Indigenous Pathogen Screening
A total of 70 whole hen carcasses (7 treatments; 10 replicates) with an average weight of 1749.87 g were obtained from a free-range poultry facility immediately after processing and were void of any antimicrobial treatments prior to the onset of the current experiment. A review by the institutional animal care and use committee (IACUC) was exempted because the birds were raised in an off-campus commercial farm operation and the current study was restricted to microbiological evaluation of bird carcasses selected for study. Immediately following evisceration, on the same day all 70 carcasses were shipped on ice and upon arrival at the University of Arkansas Center for Food Safety one carcass was screened for the background indigenous presence of Salmonella, E. coli, and Campylobacter. The remaining carcasses were stored at 4°C refrigeration until the onset of the study which began within 24 h post-slaughter.
Inocula Preparation and Inoculation
Prior to the study, a frozen stock of Salmonella Typhimurium (UK-1), E. coli (J53), and Campylobacter jejuni were streaked to isolation on respective media and incubated either aerobically at 37°C for 24 h or microaerophilically at 42°C for 48 h. Subsequently, one isolated colony from the incubated plates were streaked onto fresh medium and incubated under the previously mentioned conditions. Simultaneously, an isolated colony was streaked onto Xylose Lysine Deoxycholate (XLD; HiMedia, West Chester, PA, United States) and modified Charcoal-Cefoperazone-Deoxycholate agar (mCCDA; HiMedia, West Chester, PA, United States) for confirmation and incubated either aerobically at 37°C for 24 h or microaerophilically at 42°C for 48 h. Following confirmation, isolated colonies from the incubated media were then transferred to 40 mL of fresh Luria-Bertani Broth and Mueller Hinton Broth (Hardy Diagnostics, Irving, TX, United States) and incubated under previously mentioned conditions in a shaking incubator at 200 rpm for 12 to 16 h. The resulting cultures of 3 × 107 CFU/mL of Salmonella Typhimurium (UK-1), E. coli (J53), and C. jejuni, respectively.
Directly following the overnight (12 to 16 h) incubation of the cultures, the cultures were spun down at 18,000 g for 5 min, decanted, and then washed twice in 1 × Phosphate Buffered Saline (PBS; 8 g of NaCl, 0.2 g of KCl, 1.44 g of Na2HPO4, and 0.24 g of KH2PO4 per 1 L, with the pH adjusted to 7.4 with HCl). After the final wash, the pellet was re-suspended in 400 mL of sterile PBS.
The carcasses were inoculated in a 400 mL cocktail containing 3 × 107 CFU/mL of Salmonella Typhimurium (UK-1), E. coli (J53), and C. jejuni. Salmonella, E. coli, and Campylobacter inocula were allowed to adhere at 4°C for 60 min for a final attachment of 106, 106 and 105 CFU/g. Following the attachment period, the whole carcass weights were recorded, and the treatments were administered. The carcasses were independently placed into a spray cabinet constructed from a modified refrigerator (Model No. FFTR1814LW2, Fridgaire, Miami, FL, United States) with four pressure nozzles that administered 500 mL of treatments via a high-pressurized spray (15 psi). The treatment was applied 4 × with 5 s on 5 s off for a total duration of 20 s treatment application. The treatments utilized in the current study were: a no-treatment negative control, tap water (TW); TW + O3 (10 ppm), TW + PAA (50 ppm), TW + PAA (500 ppm), TW + O3 + PAA (50 ppm), and TW + O3 + PAA (500 ppm). The commercial PAA utilized in the current study was Spectrum (FMC, Philadelphia, PA, United States). To reduce cross contamination, treated carcasses were placed into individual sterile poultry rinse bags (Nasco, Fort Atkinson, WI, United States) and allowed to rest for 2 min.
Microbial Analysis
After the appropriated resting period, 400 mL of neutralizing Buffered Peptone Water (nBPW; 20.0 g of buffered peptone, 7 g of refined soy lecithin or equivalent, 1.0 g of sodium thiosulfate, 12.5 g of sodium bicarbonate, per 1 L of DI water; USDA Food Safety and Inspection Service, 2016) was poured directly on top and inside the carcasses. The carcasses were then manually agitated for 2 min in an 180° arcing motion. The carcasses were aseptically removed, discarded, and the subsequent rinsate was utilized for downstream analysis.
Salmonella, E. coli, and Campylobacter Enumeration
Rinsates were aliquoted to 15 mL conical tubes (VWR, Radnor, PA, United States) and subsequently 20 μL of rinsate was serially diluted to 10-6 in 180 μL of 1 × PBS via a flat bottom 96 well plate. The dot method was utilized in the current study where 10 μL of the rinsate was plated on XLD and mCCDA, allowed to dry completely, inverted, and incubated aerobically at 37°C for 24 h or microaerophilically at 42 °C for 48 h, respectively. On XLD, only colonies with black centers were considered as Salmonella and yellow colonies with surrounding yellow color change were considered as E. coli. On mCCDA, colony forming units with a silver metallic sheen were considered as C. jejuni.
Ambient PAA
To measure the ambient PAA vapor emitted from the treatment solution application, a SafeCide ChemDAQ sensor and meter was utilized (ChemDAQ Inc., Pittsburgh, PA, United States). The sensor was located directly outside the modified spray cabinet and measurements were recorded in real-time for each treatment application (n = 10; N = 70).
Statistical Analysis
Each carcass was randomly assigned to a treatment prior to the onset of the study. The CFU of Salmonella, E. coli, and Campylobacter were log transformed and reported on a CFU of bacteria per gram of chicken basis (CFU/g). The data were analyzed using One-Way ANOVA in JMP 14.0 (SAS Institute Inc., Cary, NC, United States). Means were separated using Tukey’s Protected HSD with a significant level of P ≤ 0.05.
Results
Quantification of Salmonella, E. coli, and Campylobacter Recovered From Treated Carcasses
In the current experiment, there was a treatment effect for Salmonella, E. coli, and Campylobacter recovered from the treated inoculated carcasses (P < 0.05). No treatments significantly reduced the concentration of Salmonella Typhimurium (UK-1) on whole carcasses compared to untreated carcasses (6.10 log CFU/g of Salmonella, Figure 1, P = 0.0476). However, those treated with TW + 500 ppm PAA + O3 (5.71 log CFU/g of Salmonella) had significantly lower log CFU per gram of Salmonella than those treated with TW alone (6.30 log CFU/g of Salmonella). Carcasses treated with TW + 500 ppm PAA + O3 (5.71 log CFU/g of Salmonella) did numerically possess the lowest log CFU/g of Salmonella compared to all other treatments. However, the treatment of both TW + PAA and TW + PAA + O3 did not differ significantly in recovered Salmonella (6.05, 5.86, 5.96, and 5.71 log CFU/g of Salmonella).
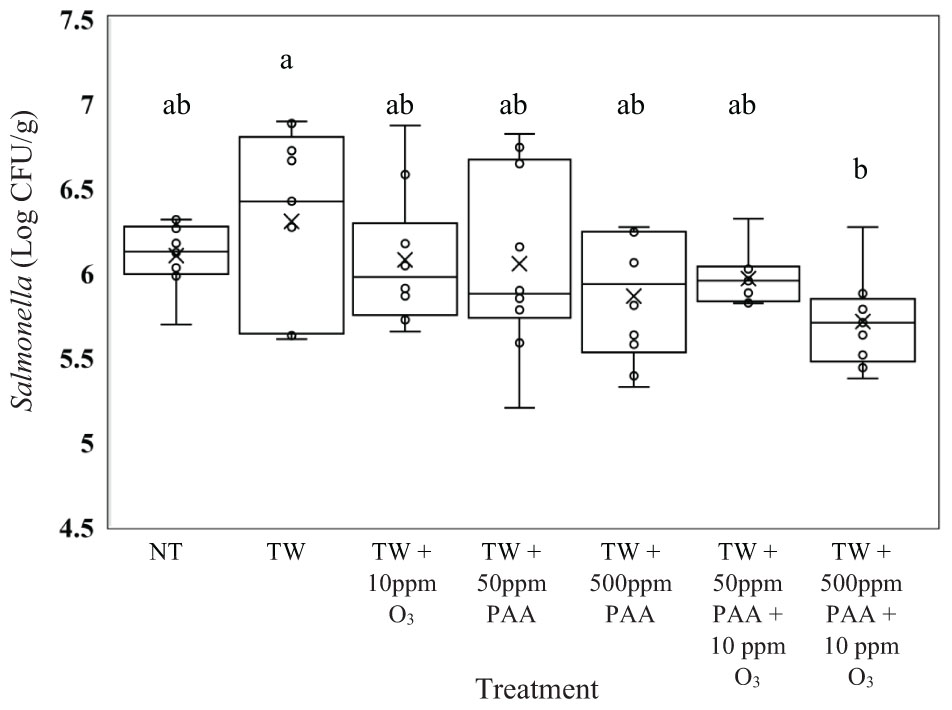
Figure 1. The effect of applying peracetic acid (PAA) alone and in combination with aqueous ozone at 10 ppm on the mean log CFU/g of Salmonella Typhimurium UK-1 on whole hen carcasses1,2. Carcasses were inoculated with 107 CFU/mL of Salmonella for a final attachment of 106 CFU/g of Salmonella Typhimurium UK-1. Birds were then placed in a modified spray cabinet to be treated for 5 s (4×) via a low pressurized spray. Immediately after, birds were rinsed in 400 mL of neutralizing buffered peptone water (nBPW) and subsequently plated to determine load of Salmonella. 1N = 67, n = 10, P = 0.0476. 2Means with different superscripts are considered significantly different (a,b).
Unlike the recovered Salmonella, E. coli (J53) recovered from the rinsates of carcasses treated with TW did not exhibit significantly higher counts compared to any of the other treated carcasses (Figure 2, P = 0.0126). Carcasses treated with TW + 500 ppm of PAA (5.57 log CFU/g of E. coli) yielded a lower load of E. coli than those not treated (6.18 log CFU/g of E. coli). Similar to Salmonella, the recovery of E. coli did not differ from carcasses treated with TW + PAA and TW + PAA + O3, regardless of PAA concentration.
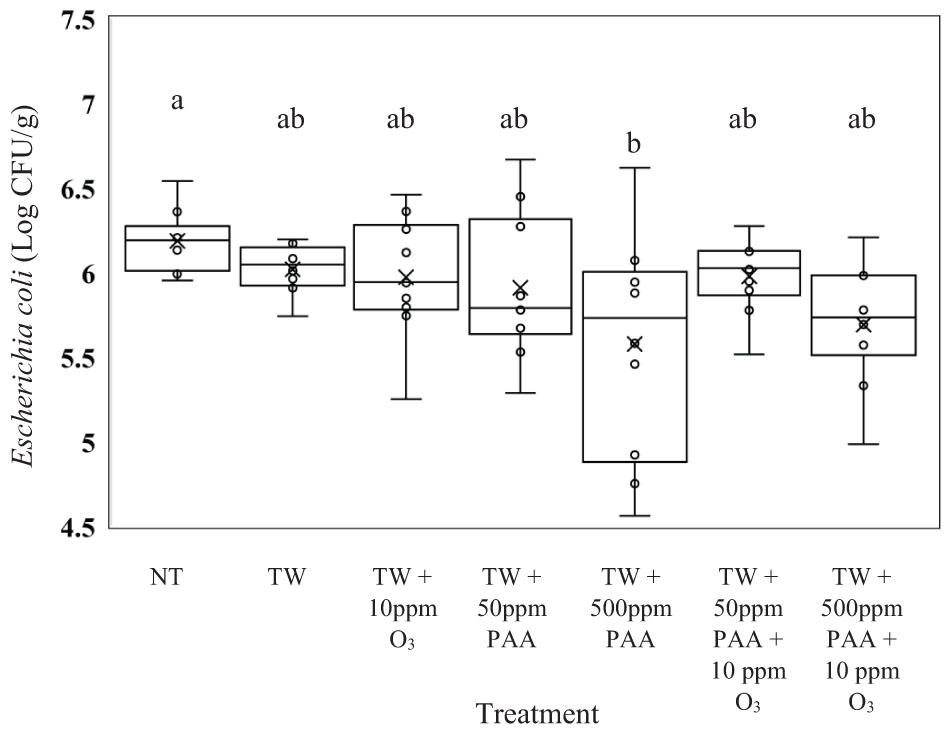
Figure 2. The effect of applying PAA alone and in combination with aqueous ozone at 10 ppm on the mean log CFU/g of Escherichia coli J53 on whole hen carcasses1,2. Carcasses were inoculated with 107 CFU/mL of E. coli for a final attachment of 106 CFU/g of E. coli J53. Birds were then placed in a modified spray cabinet to be treated for 5 s (4×) via a low pressurized spray. Immediately after, birds were rinsed in 400 mL of nBPW and subsequently plated to determine load of E. coli. 1N = 68, n = 10, P = 0.0126. 2Means with different superscripts are considered significantly different (a,b).
The recovered load of C. jejuni (log CFU/g of Campylobacter) was greatest in carcasses not treated (Figure 3; P = 0.0006). The C. jejuni recovered from carcasses not treated (5.20 log CFU/g of C. jejuni) did not differ from carcasses treated with TW, TW + O3 and TW + 50 ppm PAA + O3 (4.97, 5.00, and 4.96 log CFU/g of C. jejuni). Further, the lowest load of C. jejuni was recovered from carcasses treated with TW + 50 ppm PAA, TW + 500 ppm PAA, and TW + 500 ppm PAA + O3 (4.80, 4.81, and 4.86 log CFU/g of C. jejuni) which were significantly different from the untreated control.
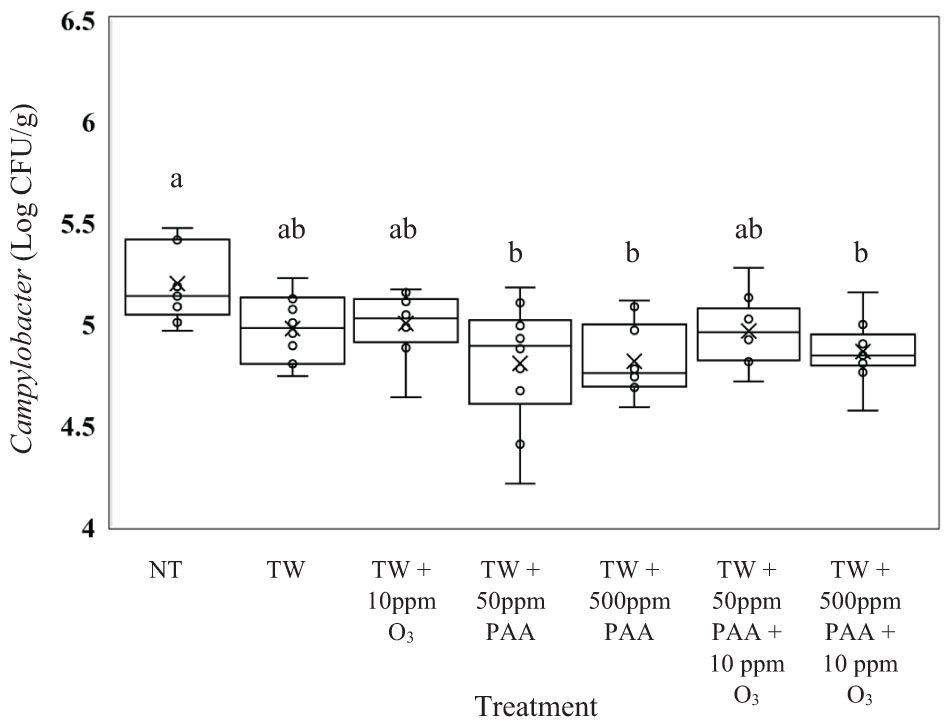
Figure 3. The effect of applying PAA alone and in combination with aqueous ozone at 10 ppm on the mean log CFU/g of Campylobacter jejuni on whole hen carcasses1,2. Carcasses were inoculated with 106 CFU/mL of C. jejuni for a final attachment of 105 CFU/g of C. jejuni. Birds were then placed in a modified spray cabinet to be treated for 5 s (4×) via a low pressurized spray. Immediately after, birds were rinsed in 400 mL of nBPW and subsequently plated to determine load of Campylobacter. 1N = 69, n = 10, P = 0.0006. 2Means with different superscripts are considered significantly different (a,b).
Quantification of PAA Vapor From Treated Carcasses
From the current experiment, it was determined that there was significant treatment effect on the production of ambient PAA (ppm) (Figure 4; P < 0.0001). Further, it was demonstrated that the greatest production of ambient PAA was derived from the treatment solution TW + 500 ppm PAA (0.565 ppm of Ambient PAA). The treatment solutions consisting of, NT, TW, TW + O3, and TW + 50 ppm PPA + O3 did not produce any ambient PAA; however, the ambient PAA produced from those treatments was not different that the ambient PAA produced off of the treatments: TW + 50 ppm PAA and TW + 500 ppm PAA + O3 (0.011 and 0.008 ppm of ambient PAA).
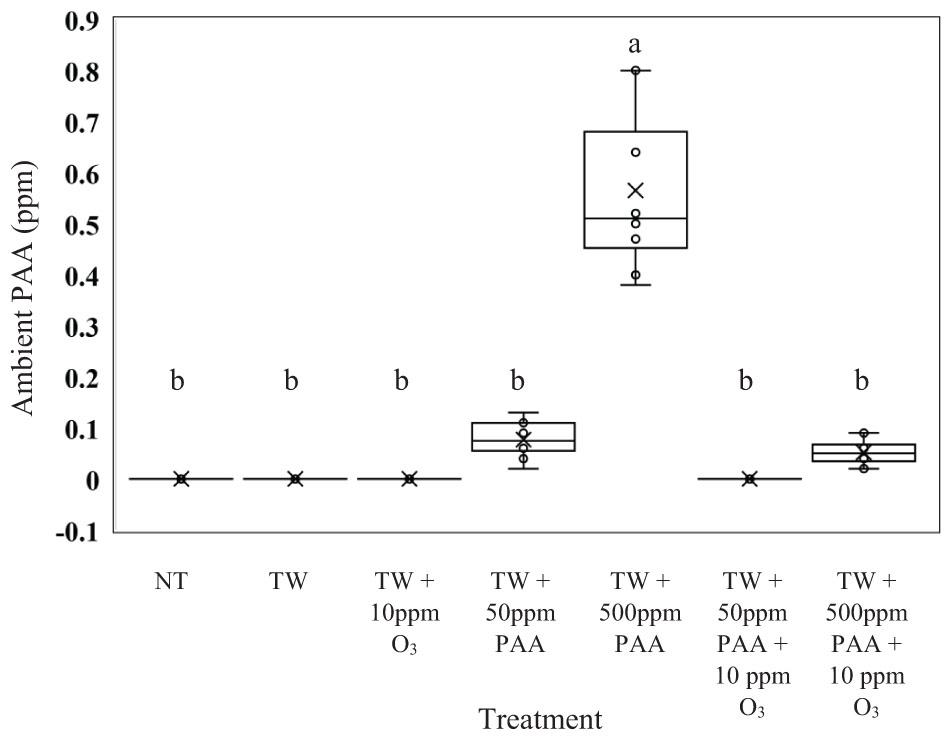
Figure 4. The effect of applying PAA alone and in combination with aqueous ozone at 10 ppm on whole hen carcasses on the mean ppm of the surrounding ambient PAA1,2. Carcasses were inoculated with Salmonella Typhimurium UK-1, E. coli J53, and C. jejuni. Birds were then placed in a modified spray cabinet to be treated for 5 s (4×) via a low pressurized spray. While the treatments were being applied, a ChemDaq SafeCide ambient PAA monitor and sensor, located outside the modified spray cabinet, was utilized to determine ppm of ambient PAA. 1N = 70, n = 10, P < 0.0001. 2Means with different superscripts are considered significantly different (a,b).
Discussion
Impact of Sanitizer Treatments on Salmonella, E. coli, and Campylobacter Inocula
In the current study, the addition of ViriditecTM aqueous ozone to a commercial PAA was utilized to determine if the addition of aqueous ozone possessed synergistic affects in mitigating three Gram-negative bacteria, commonly associated with poultry. Previously, it has been suggested that Gram-negative bacteria may be more sensitive to ozone than Gram-positive bacteria due to the greater presence of peptidoglycan in the cell wall of Gram-positive bacteria. Rey et al. (1995) demonstrated the resistance to aqueous ozone was enhanced when N-acetyl glucosamine, a constituent of the peptidoglycan of bacterial cell walls, was present (pH 3 to 7).
In addition, the utilization of ozone has been demonstrated to possess the ability to disrupt the cell membrane and induce membrane permeability in Salmonella and E. coli spp., respectively, thus weakening the bacterial cell wall and ultimately leading to cell death (Komanapalli and Lau, 1996; Dave, 1999). However, in the current study, there was no difference on the reduction of any of the Gram-negative bacteria, Salmonella, E. coli, or Campylobacter, between the use of tap water or aqueous ozone (10 ppm) when sprayed for 5 s (4×) on whole hen carcasses.
The lack of effect of aqueous ozone, alone, may have been in part due to the short duration of the treatment application (5 s; 4×) utilized in the current study. Previous research has generally utilized aqueous ozone for a longer duration of exposure, when evaluating bactericidal effects of food matrices. Gertzou et al. (2016) determined the addition of gaseous ozone for 1 h at 10 ppm to fresh chicken legs extended their shelf life 4 days more than the control when packaged in polyamide/polyethylene (PA/PE) packaging. Others have seen various levels of gaseous ozone (1, 0.1, and 33 ppm) applied for 5, 20, and 9 min, respectively, to be effective at reducing L. monocytogenes in water, fish, and poultry samples (Fisher et al., 2000; Vaz-Vehlo et al., 2001; Muthukumar and Muthuchamy, 2013). Previously, aqueous ozone (4.5 ppm) in poultry chillers has demonstrated the potential to significantly mitigate total aerobes, psychrotrophs, coliforms, fecal coliforms, and Salmonella (78, 37, 91, 91, and 81%, respectively) on chicken broiler carcasses that had been chilled for 45 min compared to those not chilled (Sheldon and Brown, 1986). When aqueous ozone (0.5 to 6.5 ppm) was applied to poultry meat, in a separate study, it reduced the load of Salmonella Enteritidis by 0.6 to 4 log CFU (Dave, 1999).
However, ozone has demonstrated to be more effective in reducing bacteria when suspended in pure water than in food products (Khadre et al., 2001). In agreement with the current study, where aqueous ozone did not have an effect on mitigating pathogen load when utilized alone at 10 ppm, Fabrizio et al. (2002) determined that the spray application of distilled water, 10 ppm aqueous ozone, 10% trisodium phosphate (TSP), 2% acetic acid (AA), 20 ppm sodium hypochlorite, electrolyzed oxidizing water (pH 2.4 to 2.7, 1,150 mV ORP, 50 ppm free CL) on chicken whole carcasses did not have an effect on Salmonella Typhimurium load on day 0. However, Fabrizio et al. (2002) did report that the submersion of electrolyzed oxidizing water, TSP, AA, and aqueous ozone reduced Salmonella Typhimurium to levels of detection only after selective enrichment on whole chicken carcasses. The submersion of whole carcasses in aqueous ozone has also demonstrated the potential to reduce total aerobic bacteria on d 0 and reduce E. coli and total coliforms on d 7 compared to whole carcasses not treated (Fabrizio et al., 2002). Thus, the complete submersion of carcasses may prove to have a greater bactericidal effect than sprays may have, as observed in the current study.
In the current study when PAA was utilized alone, both concentrations of PAA, 50 and 500 ppm, reduced C. jejuni load, but only 500 ppm reduced E. coli, and no concentration of PAA reduced Salmonella compared to the control. Although PAA has been demonstrated to be an effective antimicrobial in previous research, mitigating pathogens by 2 logs or greater (Bauermeister et al., 2008a), the current research did not demonstrate the same efficacy. In fact, the current study demonstrated no treatments were capable of exhibiting practical reductions of pathogen load of 1 log or greater. Unlike the current research, Bauermeister et al. (2008a) demonstrated that when PAA is applied in the chiller at 200 ppm Salmonella and Campylobacter load are reduced roughly below 2 and 2.5 log CFU when artificially inoculated with 106 CFU/mL of Salmonella and Campylobacter, respectively. However, as with aqueous ozone, the application method may play a part in the differences in pathogen reduction.
Other short duration antimicrobial treatments of poultry meat with PAA have demonstrated little consistency. Del Río et al. (2007) demonstrated that when chicken legs were dipped in solutions containing 220 ppm PAA (Inspexx 100, Ecolab, St. Paul, MN, United States) for 15 s, Enterobacteriaceae and coliforms were reduced 0.24 ± 0.19 and 0.28 ± 0.84, respectively, on d 0; however, the reduction of bacteria on legs dipped in PAA was not significantly different than the legs treated with water. In contrast, Nagel et al. (2013)found that the post-chill application of PAA at concentration 400 and 1,000 ppm for 20 s had the potential to reduce the load of Salmonella Typhimurium and C. jejuni on artificially contaminated chicken breasts by 2 log CFU/mL.
Although, aqueous ozone and PAA, alone, did not mitigate pathogens as previous studies have shown, the combination of aqueous and PAA demonstrated an additive effect. This additive effect may be in part due to the byproducts of PAA and O3. As PAA is the equilibrium product of acetic acid and hydrogen peroxide, when it dissociates acetic acid and hydrogen peroxide molecules are released. Hydrogen peroxide in aqueous solution is then capable of partially dissociating to hydroperoxide anion (HO2-) which is very reactive to ozone (Taube and Bray, 1940). Further, as acetic acid directly affects the pH, ozone is stabilized as ozone is more stable at a low pH (Khadre et al., 2001).
Overall, the current study demonstrated that the reduction of pathogens while utilizing the modified spray cabinet, was significant, it was not extensive. Previously, it has been demonstrated that bacteria reside not only on the exposed muscle surfaces, but within the feather follicles (Barnes and Impey, 1968). Thus, creating difficulties for antimicrobial treatments to properly disinfect poultry carcasses. This may explain the small reductions seen in the current study. This was also seen in a study performed by Sheldon and Brown (1986), who demonstrated less than a 1 log reduction of total aerobic bacteria, psychrotrophs, coliforms and fecal coliforms, and Salmonella when broiler carcasses were chilled in aqueous ozone for 45 min compared to those not chilled.
Decomposition of PAA Vapor
In the current study, the addition of aqueous ozone to PAA reduced the ambient PAA emitted when carcasses were treated in a modified spray cabinet. There is limited research on the proposed mechanism behind the reduction of PAA vapor, however, the authors have two proposed hypotheses to describe reduction in ambient PAA vapor. First, PAA (CH3COOOH) is formed from the equilibrium of hydrogen peroxide (H2O2 or OH) and acetic acid (CH3COOH). From the reaction of the acetic acid radical (Reaction 1) and ozone (O3, Reaction 2) result in the formation of the peroxyacetic acid radical which disproportionates (Reaction 3) to produce 70% hydrogen peroxide (H2O2) and other products: formaldehyde, glyoxylic acid, glycolic acid, and organic peroxides (Sehested et al., 1992) as seen in the following reactions:
The second explanation for the loss of ambient PAA vapor is that the ozone is being “robbed” an oxygen from the PAA to reduce it to acetic acid in the gas state, preventing the OH radical formation as seen in the following reaction:
Conclusion
In conclusion, the combination of 10 ppm of aqueous ozone, ViriditecTM, and 500 ppm of PAA has the potential to mitigate the presence of Salmonella Typhimurium (UK-1), E. coli J53, and C. jejuni. Furthermore, the combination of 10 ppm of aqueous ozone with 500 ppm of PAA demonstrated the ability of ozone to reduce the ambient PAA vapor by 90%, when compared to 500 ppm of PAA alone. Thus, the application of TetraClean’s product ViriditecTM has the ability to enhance the safety for poultry processing employees. Although the current study demonstrated the promising capabilities of aqueous ozone and PAA, in combination, future research is necessary to develop an understanding of the impact the combination of aqueous ozone and PAA has on the shelf life of processed poultry and the subsequent changes in the microbiome.
Author Contributions
All authors significantly contributed to the work of the current study. KF, DD, and SR designed and prepared the current study with the assistance from DW, BP, and MD. DD and DW conducted the experiments. DD analyzed the data and constructed the manuscript with the assistance from KF, DW, BP, MD, and SR.
Conflict of Interest Statement
BP, DW, and MD are employed by the company TetraClean Systems LLC, Omaha, NE, United States.
The remaining authors declare that the research was conducted in the absence of any commercial or financial relationships that could be construed as a potential conflict of interest.
Acknowledgments
DD would like to knowledge the Graduate College at the University of Arkansas for its support through the Distinguished Academy Fellowship and the continued support from the Cell and Molecular Biology Program and Department of Food Science at the University of Arkansas. Furthermore, the authors would like to acknowledge TetraClean Systems LLC for its support and assistance in completing this research.
References
American Conference of Governmental Industrial Hygienists [ACGIH] (2016). Annual TLVs® (Threshold Limit 4 Values) and BEIs® (Biological Exposure Indices) Booklet. Cincinnati, OH: ACGIH® SignaturePublications.
American Thoracic Society (1996). Respiratory Protection Guidelines. American Thoracic Society: Medical Section of the American Lung Association. Available at: https://www.thoracic.org/statements/resources/eoh/resp1-13.pdf [accessed October 16, 2018]
Barnes, E. M., and Impey, C. (1968). Psychrophilic spoilage bacteria of poultry. J. Appl. Bacteriol. 31, 97–107. doi: 10.1111/j.1365-2672.1968.tb00345.x
Bauermeister, L. J., Bowers, J. W. J., Townsend, J. C., and McKee, S. R. (2008b). Validating the efficacy of peracetic acid mixture as an antimicrobial in poultry chillers. J. Food Prot. 71, 1119–1122. doi: 10.4315/0362-028X-71.6.1119
Bauermeister, L. J., Bowers, J. W. J., Townsend, J. C., and McKee, S. R. (2008a). The microbial and quality properties of poultry carcasses treated with peracetic acid as an antimicrobial treatment. Poult. Sci. 87, 2390–2398. doi: 10.3382/ps.2008-00087
Brinez, W. J., Roig-Sagues, A. X., Hernandez Herrero, M. M., Lopez-Pedemonte, T., and Guamis, B. (2006). Bactericidal efficacy of peracetic acid in combination with hydrogen peroxide against pathogenic and non pathogenic strains of Staphylococcus spp., Listeria spp. and Escherichia coli. Food Contr. 17, 516–521. doi: 10.1016/j.foodcont.2005.02.014
Dave, S. A. (1999). Efficacy of Ozone Against Salmonella Enteritidis in Aqueous Suspensions on Poultry Meat. MSc. thesis, The Ohio State University, Columbus, OH.
Del Río, E., Muriente, R., Prieto, M., Alonso-Calleja, C., and Cpita, R. (2007). Effectiveness of trisodium phosphate, acidified sodium chlorite, citric acid, and peroxyacids against pathogenic bacteria on poultry during refrigerated storage. J. Food Prot. 70, 2063–2071. doi: 10.4315/0362-028X-70.9.2063
Fabrizio, K. A., Sharma, R. R., Dermirci, A., and Cutter, C. N. (2002). Comparison of electrolyzed oxidizing water with various antimicrobial interventions to reduce Salmonella species on poultry. Poult. Sci. 81, 1598–1605. doi: 10.1093/ps/81.10.1598
Fisher, C. W., Lee, D., Dodge, B.-A., Hamman, K. M., Robbins, J. B., and Martin, S. E. (2000). Influence of catalase and superoxide dismutase on ozone inactivation of Listeria monocytogenes. Appl. Environ. Microbiol. 66, 1405–1409. doi: 10.1128/AEM.66.4.1405-1409.2000
Fraser, J. A. L., and Thorbinson, A. (1986). Fogging Trials with Tenneco Organics Limited (30th June, 1986) at Collards Farm. Warrington: Solvay Interox.
Gertzou, I. N., Drosos, P. E., Karabagias, I. K., and Riganakos, K. A. (2016). Combined effect of ozonation and packaging on shelf life extension of fresh chicken legs during storage under refrigeration. J. Food Sci. Technol. 53, 4270–4277. doi: 10.1007/s13197-016-2421-7
Janssen, P. J. M. (1989). Acute Inhalation Toxicity Studies of Proxitane 1507 in Male Rats (I). Report No. S. 8906, Int. Doc. No. 56645/25/89. Weesp: Duphar B.V.
Janssen, P. J. M., and van Doorn, W. M. (1994). Acute Inhalation Toxicity Study with Proxitane AHC in Male and Female Rats. Report No. S. 9408, Int. Doc. No. 56345/48/94. Brussels: Solvay.
Khadre, M. A., Yousef, A. E., and Kim, J.-G. (2001). Microbiological aspects of ozone applications in food: a review. J. Food Sci. 66, 1242–1252. doi: 10.1111/j.1365-2621.2001.tb15196.x
Komanapalli, I. R., and Lau, B. H. S. (1996). Ozone-induced damage of Escherichia coli K-12. Appl. Environ. Biotechnol. 46, 610–614. doi: 10.1007/s002530050869
Merka, V., and Urban, R. (1978). Study of inhalation toxicity of performic, peracetic and perpropionic acid in mice. J. Hyg. Epidemiol. Microbiol. Immunol. 20, 54–60.
Muthukumar, A., and Muthuchamy, M. (2013). Optimization of ozone in gaseous phase to inactivate Listeria monocytogenes on raw chicken samples. Food Res. Int. 54, 1128–1130. doi: 10.1016/j.foodres.2012.12.016
Nagel, G. M., Bauermeister, L. J., Bratcher, C. L., Singh, M., and McKee, S. R. (2013). Salmonella and Campylobacter reduction and quality characteristics of poultry carcasses treated with various antimicrobials in a post-chill immersion tank. Int. J. Food Microbiol. 165, 281–286. doi: 10.1016/j.ijfoodmicro.2013.05.016
National Academies of Science [NAS] (2010). Chapter 7: Peracetic Acid-Acute Exposure Guideline Levels. In: Acute Exposure Guideline Levels for Selected Airborne Chemicals, Vol. 8. Available at: http://www.epa.gov/opptintr/aegl/pubs/peracetic_acid_final_volume8_2010.pdf
National Institute for Occupational Safety and Health [NIOSH] (2015). Immediately Dangerous to Life or Health (IDLH) Value Profile for Peracetic Acid. External Review Draft. Available at: https://www.cdc.gov/niosh/docket/review/docket156a/pdfs/g1-013-peracetic-acid-cas-79-21-0.pdf
Oyarzabal, O. A. (2005). Reduction of Campylobacter spp. by commercial antimicrobials applied during the processing of broiler chickens: a review from the United States perspective. J. Food Prot. 68, 1752–1760. doi: 10.4315/0362-028X-68.8.1752
Rey, R. P., Sellés, A. N., Baluja, C., and Otero, M. L. (1995). Ozonation kinetics of glucosamine and N-acetyl glucosamine in aqueous medium. Ozone Sci. Eng. 17, 463–467. doi: 10.1080/01919519508547349
Sehested, K., Corfitzen, H., Holcman, J., and Hart, E. J. (1992). Decomposition of ozone in aqueous acetic acid solutions (pH 0-4). J. Phys. Chem. 96, 1005–1009. doi: 10.1021/j100181a084
Sheldon, B. W., and Brown, A. L. (1986). Efficacy of ozone as a disinfectant for poultry carcasses. J. Food Sci. 51, 305–309. doi: 10.1111/j.1365-2621.1986.tb11116.x
Taube, H., and Bray, W. C. (1940). Chain reactions in aqueous solutions containing ozone, hydrogen peroxide, and acid. J. Am. Chem. Soc. 62, 3357–3373. doi: 10.1021/ja01869a027
USDA Food Safety and Inspection Service (2016). FSIS Notice 41-16. June 8, 2016. Washington D.C: United States Department Of Agriculture.
Keywords: poultry, spray cabinet, aqueous ozone, peracetic acid, pathogenic reduction, ambient peracetic acid vapor
Citation: Dittoe DK, Feye KM, Peyton B, Worlie D, Draper MJ and Ricke SC (2019) The Addition of ViriditecTM Aqueous Ozone to Peracetic Acid as an Antimicrobial Spray Increases Air Quality While Maintaining Salmonella Typhimurium, Non-pathogenic Escherichia coli, and Campylobacter jejuni Reduction on Whole Carcasses. Front. Microbiol. 9:3180. doi: 10.3389/fmicb.2018.03180
Received: 18 October 2018; Accepted: 07 December 2018;
Published: 08 January 2019.
Edited by:
Jean-Christophe Augustin, École Nationale Vétérinaire d’Alfort, FranceReviewed by:
Xiang Yang, University of California, Davis, United StatesVasileios Englezos, Università degli Studi di Torino, Italy
Copyright © 2019 Dittoe, Feye, Peyton, Worlie, Draper and Ricke. This is an open-access article distributed under the terms of the Creative Commons Attribution License (CC BY). The use, distribution or reproduction in other forums is permitted, provided the original author(s) and the copyright owner(s) are credited and that the original publication in this journal is cited, in accordance with accepted academic practice. No use, distribution or reproduction is permitted which does not comply with these terms.
*Correspondence: Steven C. Ricke, sricke@uark.edu