- Key Laboratory of Zoonosis, College of Animal Science and Veterinary Medicine, Shenyang Agricultural University, Shenyang, China
Toxoplasma gondii is an obligatory intracellular parasite that causes a common infection in many warm-blooded animals. During infection, the host’s immune system plays an important role in confining the dissemination of the parasites in the hosts. T cell immunoglobulin- and mucin domain–containing molecule 3 (Tim-3) has been characterized as an important regulator in cell-mediated immune responses in various infections. Here, we compared Tim-3 expression on splenic and circulatory T, B cells and a few cytokines in the sera of mice infected with the more virulent type I (RH) vs. the low virulent type II (ME49) strain. Tim-3 expression on the splenic and circulatory T cells of mice infected with T. gondii (RH strain) was higher than that in mice infected with T. gondii (ME49 strain). T. gondii infection reduced the proportion of splenic helper T cells (Th) and cytotoxic T cells (Tc) and increased Tim-3 expression. Further, serum levels of interleukin (IL)-2, interferon γ, tumor necrosis factor (TNF)-α, IL-12p70, IL-22, IL-17A, and IL-5 increased significantly after infection. Mice infected with T. gondii (ME49 strain) showed higher levels of TNF-α, IL-17A, IL-12p70, and IL-22 than that infected by the RH strain. Our study revealed that T. gondii strains may have their inherent ability in triggering different host immune responses, which may explain the clinical variation in diseases severity after infection.
Introduction
The parasite Toxoplasma gondii can infect almost all warm-blooded animals, including humans (Webster, 2010). It can cause widespread subclinical human infection (Mccabe and Remington, 1988) and severe disease in immunocompromised patients, especially people infected with HIV (Luft and Remington, 1988). Previous study (Howe and Sibley, 1995) has indicated that T. gondii strains fall into three distinct clonal lineages: type I (e.g., RH and GT-1), type II [e.g., ME49 and its derivatives (PDS, PLK, PTg)], and type III (e.g., CEP, CTg, VEG). The genetic difference of these strains is around 1% (Su et al., 2003). Different T. gondii genotypes might differ in their capacity for inducing pathology or occurrence in a particular animal species (Jia et al., 2013). A subpopulation of a type I strain exhibited faster migration in vitro compared with type II and type III strains (Antonio and David, 2002). Further, type I strains transmigrated across mouse intestinal epithelium and penetrated the vascular endothelium more quickly ex vivo (Antonio and David, 2002). Type II, but not type I or type III strains, mainly dominated in patients with AIDS and congenital infections (Honoré et al., 2000). Infection with type II strain parasites, and not type I or type III strains, stimulated the production of proinflammatory cytokines, and in macrophages, particularly high levels of the T helper 1 cell (Th1)-polarizing cytokine, IL-12, were highly dependent on the parasite genotype (Robben et al., 2004).
In T. gondii primary infection, both CD4+ and CD8+ T cells of the host participate in the immune response to the parasite (Purner et al., 1996). CD8+ T cells may determine cyst numbers in T. gondii–infected murine models (Brown and Mcleod, 1990). CD4+ T cells can control the growth of tachyzoites, and upon appropriate stimulation, all CD4+ T cells will produce interleukin (IL)-2 and interferon (IFN)-γ, which are central to resistance to T. gondii infection (Canessa et al., 1988). B cell–deficient mice vaccinated with an attenuated strain of Toxoplasma (ts-4) do not survive after challenge with T. gondii (RH strain) tachyzoites (Sayles et al., 2000). However, the immunized mice are protected after oral challenge with a mildly virulent T. gondii strain (Johnson et al., 2004).
In the immune response to various parasite infections and disease models, T cell immunoglobulin- and mucin domain–containing molecule 3 (Tim-3) plays a widespread and complex role in both adaptive and innate immunity (Freeman et al., 2010). Tim-3 is mainly expressed on activated Th1 cells (Monney et al., 2002). Tim-3 is also expressed on non–T cells, including dendritic cells, monocytes, macrophages, natural killer (NK) and NK T cells, mast cells, and at lower levels in Th17 cells (Nakayama et al., 2009; Gleason et al., 2012; Ndhlovu et al., 2012). In Plasmodium infection, increased Tim-3 expression results in lymphocyte exhaustion, while Tim-3 signaling blockade restores lymphocyte activity (Hou et al., 2016); blocking Tim-3 also improves the phagocytosis and parasitic mediator production of murine splenic macrophages (Hou et al., 2017). Further, differential Tim-3 expression of immune cells from the spleen, mesenteric lymph nodes (Berrocal Almanza et al., 2013), and brain (Wu et al., 2014) has been observed during T. gondii infection in mice from different backgrounds. However, there has been no further investigation on Tim-3 expression on the immune cells of hosts infected with different T. gondii genotypes.
In the present study, we investigated Tim-3 expression and its role in regulating cytokine production in mice infected with different T. gondii genotypes. The data indicates that differences in host’s Tim-3 expression and cytokine production triggered by T. gondii may contribute to the virulent characteristics of these parasite strains.
Materials and Methods
Ethics Statement
Female BALB/c mice (6–8 weeks old) were maintained in our animal facility under specific pathogen–free conditions. All animal procedures performed in the present study were conducted following the animal husbandry guidelines of Shenyang Agricultural University; the Ethical Committee of Shenyang Agricultural University approved the laboratory animal experiments (Permit No. SYXK < Liao > 2011-0001).
Infection Experiments
The tachyzoites of the T. gondii (RH strain) were obtained from the peritoneal fluid of BALB/c mice that had previously been inoculated with the parasite strain. The parasites of ME49 strain were, in first step, proliferated in monkey kidney adherent fibroblasts (Vero cells) at 37°C in a 5% CO2 incubator. Then nearly 108 parasites of ME49 strain were injected into the peritoneal cavity of mice. The peritoneal fluid of mice was repeatedly squeezed with a 5 ml curved syringe, then parasites were filtered through a 5.0 μm filter and checked under the microscope to ensure that they are all single bradyzoites. For the infection assays, mice (n = 20 per group) were each infected with 100 ME49 bradyzoites (ME49 group) or 100 RH tachyzoites (RH group) in 0.2 ml 1 × phosphate-buffered saline (pH 7.4).
Preparation of Splenic and Circulatory Immune Cells
The spleens of infected mice and healthy mice (Control group) were cut into pieces, minced, and pressed into a 70-μm cell strainer. Red blood cells (RBCs) were depleted with RBC lysis solution (155 mM NH4Cl, 10 mM KHCO3, 0.11 mM EDTA Na2, pH 7.2). Anticoagulated blood was obtained and the circulatory immune cells were isolated by depleting RBCs as described above. The mouse (n = 5 per group) splenic immune cells and circulatory immune cells were collected at day 3, 5, 7, and 9 post-infection (Supplementary Figure S1).
Flow Cytometry
Cells were stained with Zombie NIR Fixable Viability dye (catalog no. 423106; BioLegend, San Diego, CA, United States), which is non-permeant to live cells but is permeant to cells with compromised membranes, i.e., dead cells. The cells were then pre-incubated with purified anti-mouse CD16/32 antibody (catalog no. 101310; BioLegend) to block non-specific immunoglobulin binding to the Fc receptors. Finally, the cells were incubated with specific antibodies or isotype controls according to the manufacturers’ guidelines. The antibodies used were: Pacific Blue anti-mouse CD45; allophycocyanin-conjugated (APC) anti-mouse CD3; fluorescein isothiocyanate–conjugated (FITC) anti-mouse CD8a; peridinin chlorophyll protein complex–conjugated (PerCP) anti-mouse CD4; FITC anti-mouse CD19; phycoerythrin-conjugated (PE) anti-mouse CD366; Pacific Blue Rat immunoglobulin (Ig)G2b, κ Isotype Ctrl; APC Rat IgG2b, κ Isotype Ctrl; FITC Rat IgG2a, κ Isotype Ctrl; PerCP Rat IgG2b, κ Isotype Ctrl; and PE Rat IgG2a, κ Isotype Ctrl (all from BioLegend). The cells were detected and analyzed using a FACSAria III flow cytometer (BD Biosciences, San Jose, CA, United States); positive cells were defined using an isotype control or a fluorescence minus one control.
Cytokine Detection
IL-2, IL-4, IL-17A, tumor necrosis factor (TNF)-α, IL-5, IL-22, and IFN-γ levels in the mouse serum were determined using a LEGENDplex Mouse Th Cytokine Panel (catalog no. 740005; BioLegend). All serum samples were diluted 1:1 in assay buffer. Samples were treated according to the BioLegend standard protocol and were examined using FACSAria III (BD Biosciences) driven by FACSDiva software (BD Biosciences). IL-12p70 was assayed by ELISA in microplates (catalog no. 1211202; DAKEWE BIOTECH CO., LTD.) according to the standard protocol.
Statistical Analysis
All data were analyzed with GraphPad Prism 5.0 (GraphPad Software, Inc., La Jolla, CA, United States) and PASW Statistics 18 (IBM Co., Armonk, NY, United States). The results were analyzed using a two-tailed paired t-test. The mean and standard deviation (SD) were determined using 3–5 biological replicates. P < 0.05 was considered significant. Cytokine calculations were performed using the LEGENDplex 8.0 application (VigeneTech Inc., Carlisle, MA, United States) and ELISACalc data program. The heatmap was drawn using Heml 1.0.3.7 software (Huazhong University of Science and Technology, Hubei, Wuhan, China).
Results
The Splenic T and B Cells of T. gondii (RH Strain)–Infected Mice Showed Higher Tim-3 Expression Than That of Mice Infected With T. gondii (ME49 Strain)
Tim-3+ cells in the spleen and mesenteric lymph nodes are increased after T. gondii infection (Berrocal Almanza et al., 2013). Here, we investigated Tim-3 expression on splenic lymphocyte subpopulations in mice infected with different T. gondii genotypes. The splenic helper T (Th) cells and cytotoxic T (Tc) cells in the RH group had higher Tim-3 expression as compared to the ME49 group at most of the time points (Figures 1A–D). Although flow cytometry revealed nearly equal Tim-3 expression on the splenic CD3+CD4+ Th cells from the RH and ME49 groups at day 7, splenic Th cells in the RH group had higher Tim-3 expression at most of the time points compared to that of the ME49 group (Figures 1A,B, RH group vs. ME49 group: day 3, 3.50 ± 0.30 vs. 2.20 ± 0.52, P < 0.05; day 5, 4.43 ± 0.15 vs. 2.33 ± 0.15, P < 0.001; day 7, 3.70 ± 1.22 vs. 3.70 ± 0.46, P > 0.05; day 9, 5.23 ± 0.55 vs. 2.87 ± 0.21, P < 0.01).
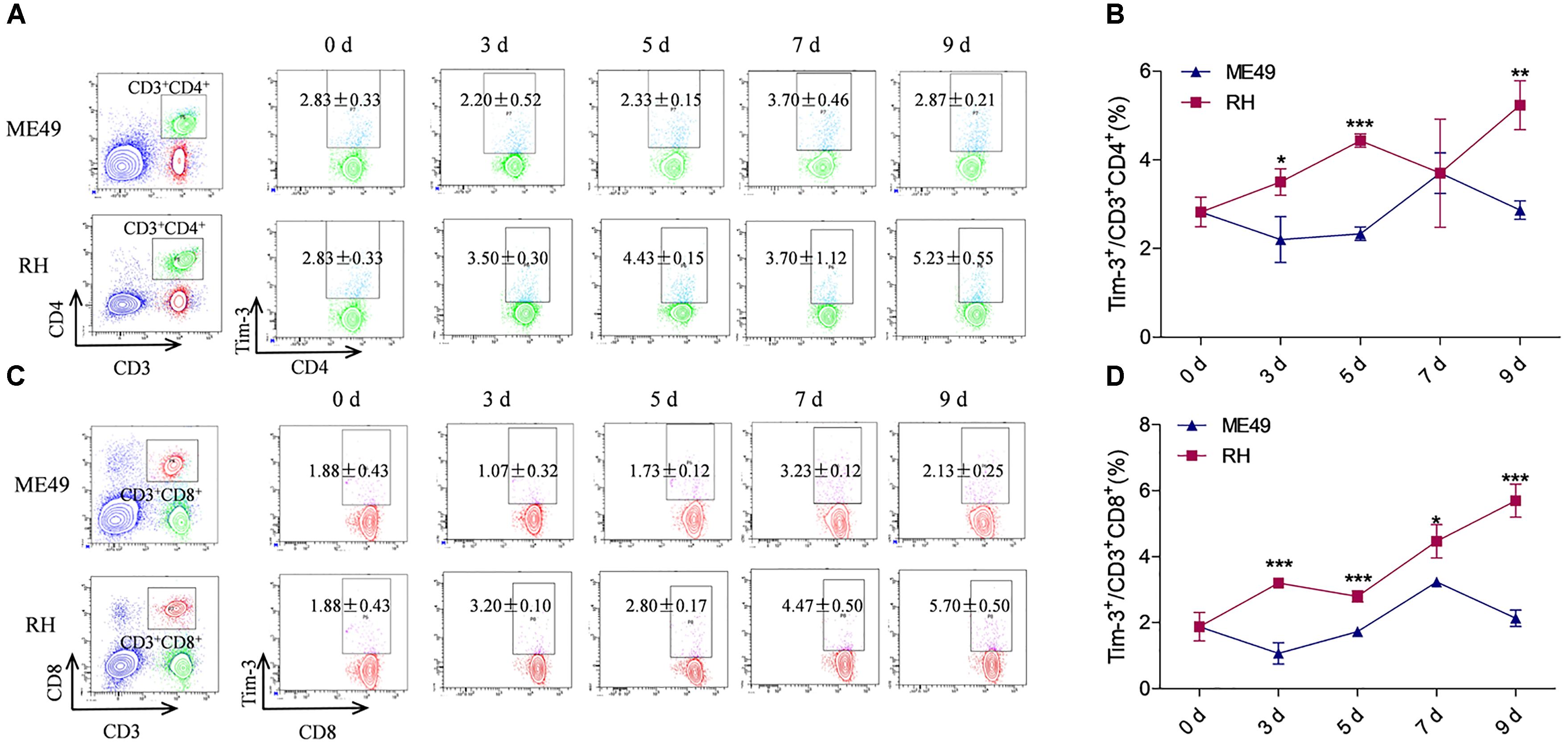
FIGURE 1. Tim-3 expression on splenic T cells in mice infected with T. gondii of the RH or ME49 strain. Tim-3 expression on splenic Th cells (A,B) and Tc cells (C,D) was detected by flow cytometry at day 3, 5, 7, and 9 post-infection. (A, left panel) The gating strategy of Th cells. (A, right panel) Representative dot plots of Tim-3 expression on CD3+CD4+ Th cells. (B) Comparisons of the proportions of Tim-3+ cells within the CD3+CD4+ cell population between the ME49 and RH groups. (C, left panel) The gating strategy of Tc cells. (C, right panel) Representative dot plots of Tim-3 expression on CD3+CD8+ Tc cells. (D) Comparisons of the proportions of Tim-3+ cells within the CD3+CD8+ cell population between ME49 group and RH group The results are representative of three independent experiments with 3–5 mice per group per experiment; data are the means ± SDs. ∗P < 0.05, ∗∗P < 0.01, ∗∗∗P < 0.001; ∗indicates comparisons to the ME49 group.
Splenic CD3+CD8+ Tc cells from the RH group had higher Tim-3 expression compared to that of the ME49 group post-infection (Figures 1C,D, RH group vs. ME49 group: day 3, 3.20 ± 0.10 vs. 1.07 ± 0.32, P < 0.001; day 5, 2.80 ± 0.17 vs. 1.73 ± 0.12, P < 0.001; day 7, 4.47 ± 0.50 vs. 3.23 ± 0.12, P < 0.05; day 9, 5.70 ± 0.50 vs. 2.13 ± 0.25, P < 0.001).
Tim-3 expression on splenic B cells after infection with different T. gondii genotypes was also observed: the RH group had significantly higher expression than the ME49 group at day 3, 5, and 7 post-infection (Supplementary Figure S2). Although Tim-3 expression on the splenic B cells in the RH group remained higher than that of the ME49 group at day 9, it was not significant (Supplementary Figure S2 and data not shown).
The Circulatory T Cells in T. gondii (RH Strain)–Infected Mice Had Higher Tim-3 Expression Than That of Mice Infected T. gondii (ME49 Strain)
Circulatory Th cells and Tc cells in the RH group had higher Tim-3 expression compared to that of the ME49 group at most of the time points (Figures 2A–D). The splenic Th cells in the RH group had almost identical Tim-3 expression nearly equal to that of the ME49 group at day 3 post-infection (Figures 2A,B, RH group vs. ME49 group: 5.83 ± 1.31 vs. 5.90 ± 0.2, P > 0.05). The circulatory Th cells in the RH group had significantly higher Tim-3 expression compared to that of the ME49 group at day 5 and 9 post-infection (Figures 2A,B, RH group vs. ME49 group: day 5, 4.77 ± 0.76 vs. 2.93 ± 0.76, P < 0.05; day 9, 3.67 ± 0.45 vs. 2.20 ± 0.17, P < 0.01). Although Tim-3 expression on splenic Th cells in the RH group remained higher than that of the ME49 group at day 7 post-infection, it was not significant (Figures 2A,B, RH group vs. ME49 group: 3.77 ± 0.81 vs. 3.23 ± 0.32, P > 0.05).
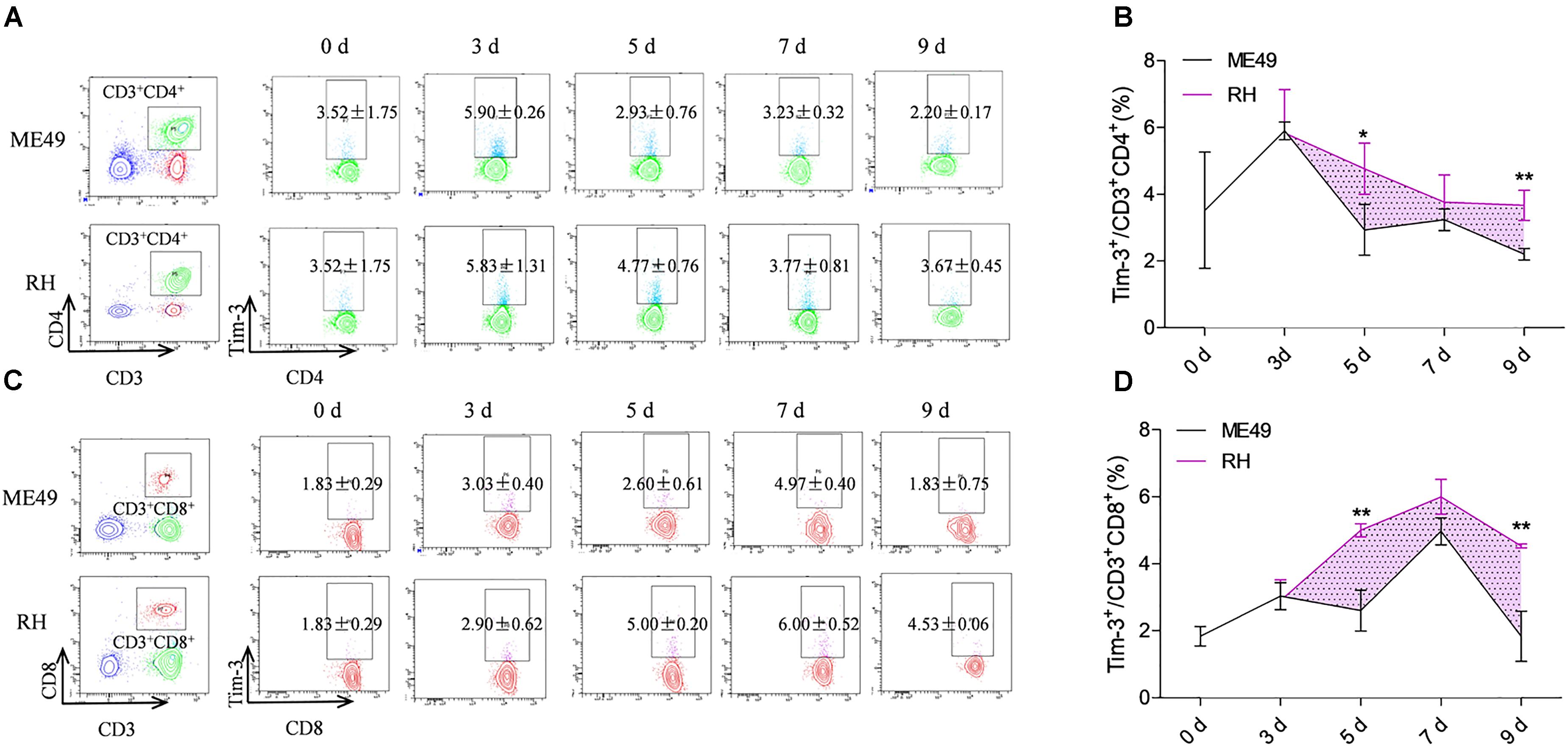
FIGURE 2. Tim-3 expression on circulatory T cells in mice infected with T. gondii of the RH or ME49 strain. Tim-3 expression on circulatory Th cells (A,B) and Tc cells (C,D) was detected by flow cytometry at day 3, 5, 7, and 9 post-infection. (A, left panel) The gating strategy of Th cells. (A, right panel) Representative dot plots of Tim-3 expression on CD3+CD4+ Th cells. (B) Comparisons of the proportions of Tim-3+ cells within the CD3+CD4+ cell population between ME49 group and RH group. (C, left panel) The gating strategy of Tc cells. (C, right panel) Representative dot plots of Tim-3 expression on CD3+CD8+ Tc cells. (D) Comparisons of the proportions of Tim-3+ cells within the CD3+CD8+ cell population between ME49 group and RH group. The results are representative of three independent experiments with 3–5 mice per group per experiment; data are the means ± SDs. ∗P < 0.05, ∗∗P < 0.01; ∗indicates comparisons to the ME49 group.
Tim-3 expression on splenic Tc cells in the RH group was almost identical to that of the ME49 group at day 3 post-infection (Figures 2C,D, RH group vs. ME49 group: 2.90 ± 0.62 vs. 3.03 ± 0.40, P > 0.05). The circulatory Tc cells in the RH group had significantly higher Tim-3 expression compared to that of the ME49 group at day 5 and 9 post-infection (Figures 2C,D, RH group vs. ME49 group: day 5, 5.00 ± 0.20 vs. 2.60 ± 0.61, P < 0.01; day 9, 4.53 ± 0.06 vs. 1.83 ± 0.75, P < 0.01). Although Tim-3 expression on the splenic Tc cells in the RH group remained higher than that of the ME49 group at day 7 post-infection, it was not significant (Figures 2C,D, RH group vs. ME49 group: 6.00 ± 0.52 vs. 4.97 ± 0.40, P > 0.05).
T. gondii Infection Induced Higher Tim-3 Expression and Reduced Proportion of Splenic T Lymphocytes
We observed increased Tim-3 expression on splenic CD3+CD4+ Th cells (Figure 3A, left) accompanied by a reduction in CD3+CD4+ Th cells (Figure 3B, left) from day 3–7 post-infection in the ME49 group. Although Tim-3 expression on CD3+CD4+ Th cells decreased at day 9 post-infection, it remained higher (but not significantly) than that on day 3 post-infection (Figure 3A, left, day 3 vs. day 9: 2.20 ± 0.52 vs. 2.87 ± 0.21, P > 0.05), and the proportion of splenic CD3+CD4+ Th cells at day 9 post-infection was significantly lower than that on day 3 post-infection (Figure 3B, left, day 3 vs. day 9: 39.00 ± 2.21 vs. 20.30 ± 2.16, P < 0.001) in the ME49 group. In the RH group, there was an increase in Tim-3 expression on the splenic CD3+CD4+ Th cells (Figure 3A, right) accompanied by a reduction of CD3+CD4+ Th cells (Figure 3B, right) on day 3–9 post-infection.
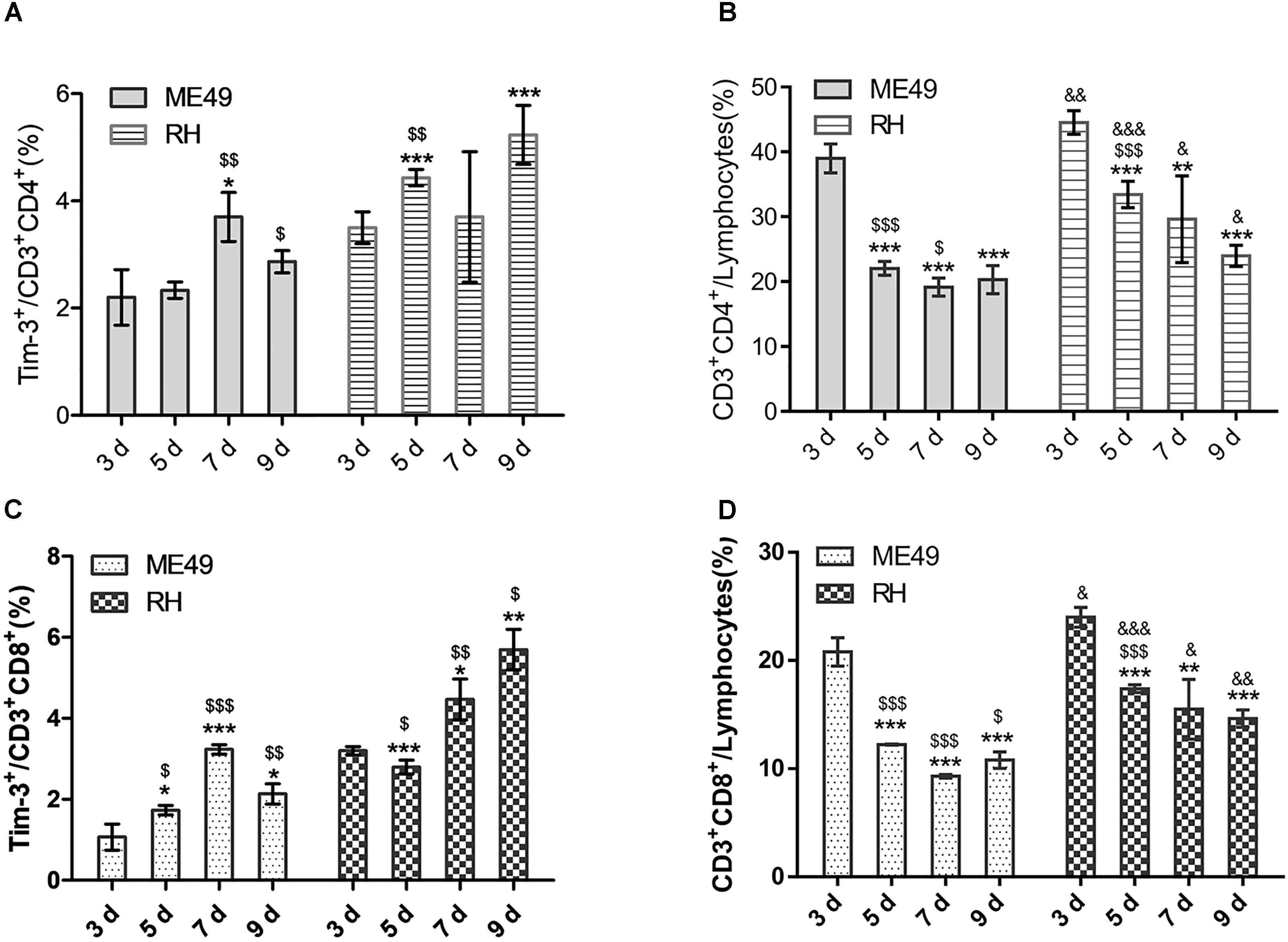
FIGURE 3. T. gondii infection reduced splenic T lymphocytes and increased Tim-3 expression in vivo. (A) Histograms showing the frequency of Tim-3+ cells in CD3+CD4+ T cells at day 3, 5, 7, and 9 post-infection. (B) Histograms showing the proportions of CD3+CD4+ Th cells in splenic lymphocytes at day 3, 5, 7, and 9 post-infection. (C) Histograms showing the frequency of Tim-3+ cells in CD3+CD8+ T cells at day 3, 5, 7, and 9 post-infection. (D) Histograms showing the proportions of CD3+CD8+ Tc cells in splenic lymphocytes at day 3, 5, 7, and 9 post-infection. The results are representative of three independent experiments with 3–5 mice per group per experiment; data are the means ± SDs. ∗,$,&P < 0.05, ∗∗,$$,&&P < 0.01, ∗∗∗,$$$,&&&P < 0.001; ∗indicates comparisons to day 3, $indicates comparisons to the previous time point. &indicates RH group comparisons to the ME49 group.
Further, there was increased Tim-3 expression on the splenic CD3+CD8+ Tc cells (Figure 3C, left) accompanied by a reduction of CD3+CD8+ Tc cells (Figure 3D, left) on day 3–7 post-infection in the ME49 group. Moreover, Tim-3 expression on CD3+CD8+ Tc cells decreased at day 9 post-infection, but remained higher than that on day 3 post-infection (Figure 3C, left, day 3 vs. day 9: 1.07 ± 0.32 vs. 2.13 ± 0.25, P < 0.05), and the proportion of splenic CD3+CD8+ Tc cells at day 9 post-infection remained lower than that on day 3 post-infection (Figure 3D, left, day 3 vs. day 9: 20.80 ± 1.31 vs. 10.80 ± 0.75, P < 0.05) in the ME49 group. In the RH group, there was increased Tim-3 expression on the splenic CD3+CD8+ Tc cells (Figure 3C, right) accompanied by a reduction of CD3+CD8+ Tc cells (Figure 3D, right) at day 7 and 9 post-infection.
Splenomegaly was observed post-infection (Figure 4A). The spleen indexes of the ME49 and RH groups both increased at day 7 and 9 post-infection (Figure 4B). Further, the ME49 group had a higher spleen index than the RH group at day 7 and 9 post-infection (Figure 4B, ME49 group vs. RH group: day 7, 84.48 ± 5.99 vs. 65.48 ± 5.75, P < 0.05; day 9, 89.37 ± 7.65 vs. 71.60 ± 4.98, P < 0.05).
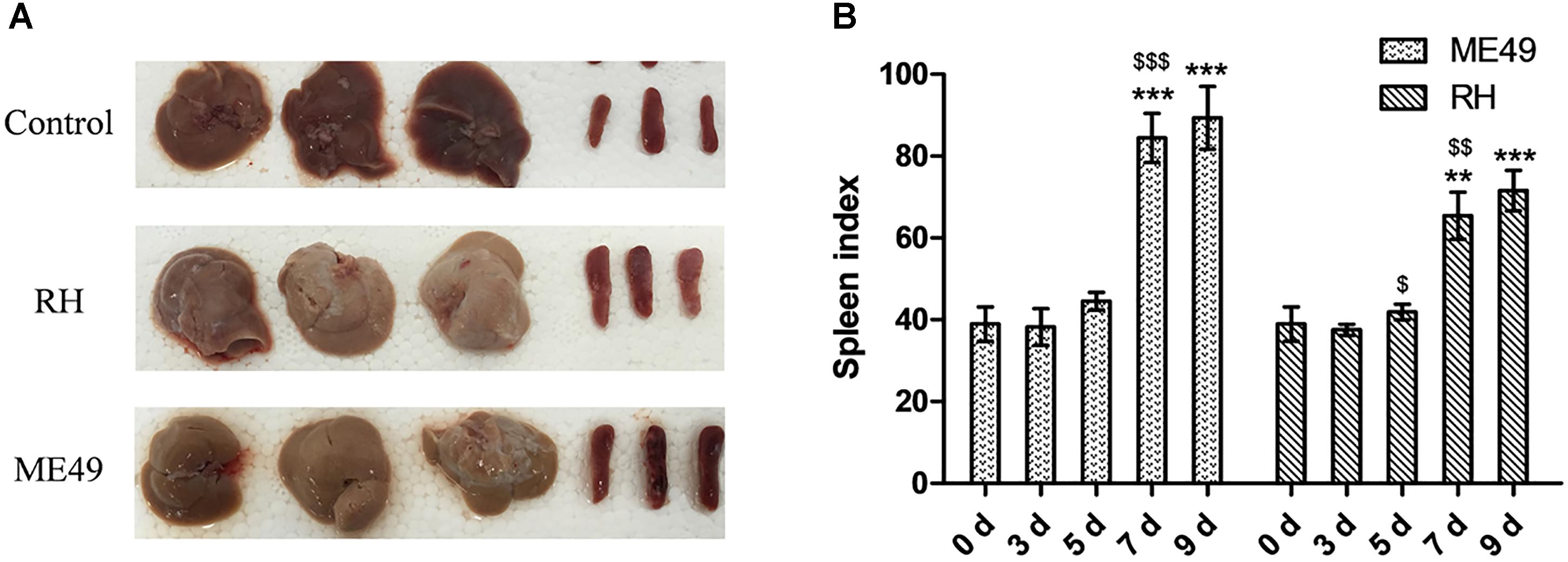
FIGURE 4. Splenomegaly post-infection. The spleen and liver were observed 7 days post-infection, and the spleen indexes [spleen weight (mg) × 10/body weight (g) × 100%] were calculated. (A) Representative images of spleen and liver from the Control, RH, and ME49 groups. (B) Spleen index of the ME49 and RH groups. The results are representative of three independent experiments with 3–5 mice per group per experiment; data are the means ± SDs. $P < 0.05, ∗∗,$$P < 0.01, ∗∗∗,$$$P < 0.001; ∗indicates comparisons to day 0, $indicates comparisons to the previous time point.
Serum Proinflammatory Cytokines Increased After Infection and the Cytokine Levels Was Associated With T. gondii Genotype
Cytokines play significant roles in T. gondii–resistant hosts (Mahmoudi et al., 2017). To investigate lymphocyte activity after infection, we quantitatively determined the presence of IL-2, IFN-γ, TNF-α, IL-4, IL-5, IL-17A, IL-12p70, and IL-22 (Figures 5A–H) in mouse sera at day 3, 5, 7, and 9 post-infection; the sera of healthy mice were used as controls. Type I cytokines such as IL-2 (Figures 5A,I), IFN-γ (Figures 5D,I), and TNF-α (Figures 5E,I) increased gradually in the sera of both the RH and ME49 groups, and there was an earlier significant increase of IFN-γ at day 3 post-infection in the RH group (Figure 5D, RH group vs. Control group: 12.74 ± 1.15 vs. 4.33 ± 0.88, P < 0.05). At day 9 post-infection, the RH group had higher IL-2 levels than the ME49 group (Figure 5A). However, the RH group had lower TNF-α levels than the ME49 group at day 3, 5, and 9 post-infection (Figure 5E). There were no significant changes in the secretion of the type II cytokine IL-4 (Figures 5B,I), but type II cytokine IL-5 (Figures 5C,I) was increased post-infection; the RH group had lower IL-5 levels than the ME49 group at day 5 and 9 post-infection (Figures 5C,I). There was a significant increase in the type 17 cytokines IL-17A (Figures 5F,I) and IL-22 (Figures 5H,I) in both the RH and ME49 group. The RH group had lower IL-17A levels than the ME49 group at day 3 and 7 post-infection (Figure 5F). An increase secretion of IL-12 was determined post infection and the production of IL-12 was significantly lower in RH group than ME49 group at day 5 (Figure 5G). The RH group had lower IL-22 levels than the ME49 group at day 3, 5, 7, and 9 post-infection (Figure 5H).
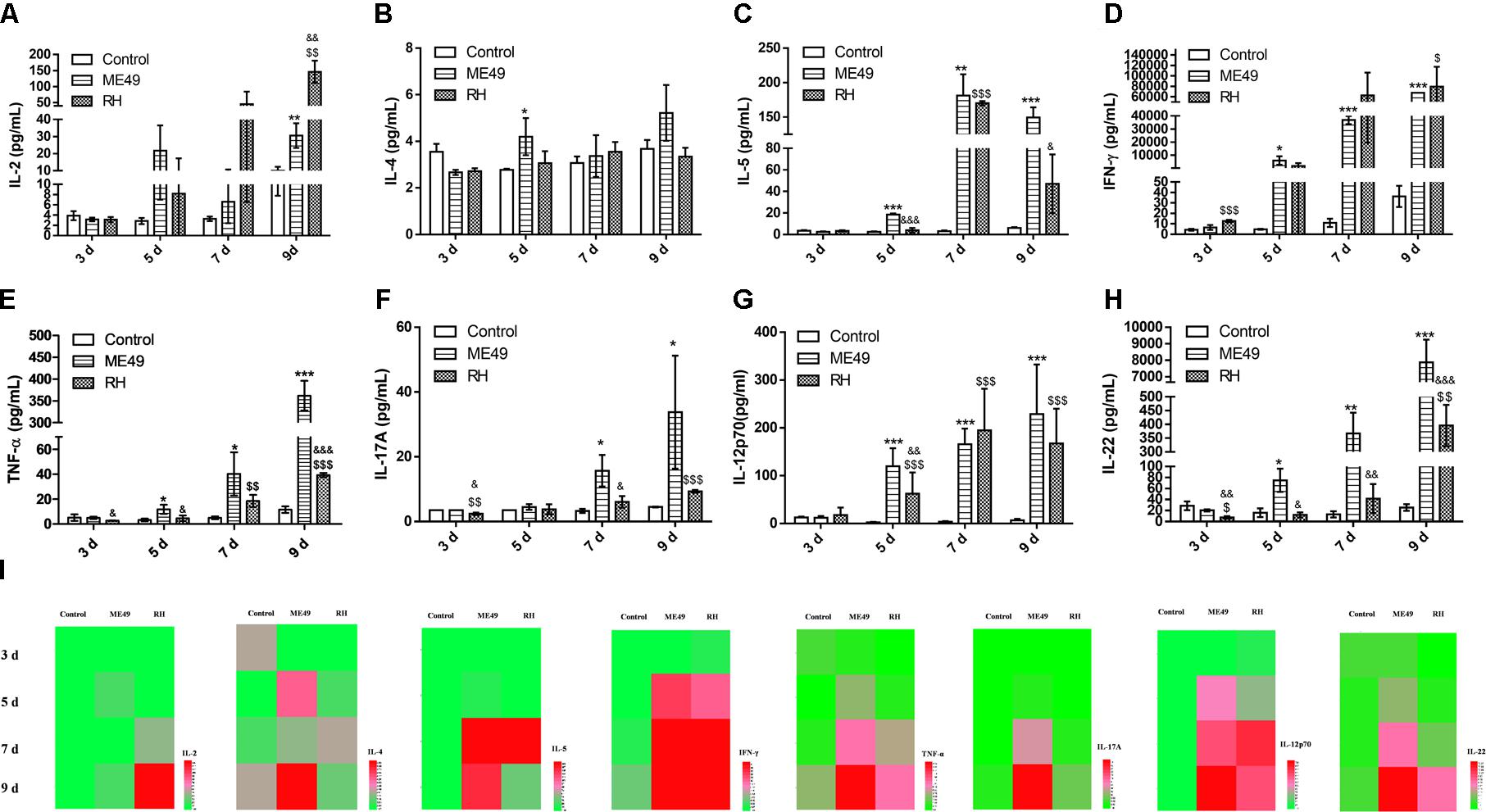
FIGURE 5. Cytokine production during infection with T. gondii of the RH or ME49 strain. (A–H) Comparisons of cytokine levels (IL-2, IL-4, IL-5, IFN-γ, TNF-α, IL-17A, IL-12p70, and IL-22) in sera from Control, ME49, and RH groups. (I) Heatmaps directly showing the difference in the cytokines detected. The results are representative of three independent experiments with 3–5 mice per group per experiment; data are the means ± SDs. ∗,$,&P < 0.05, ∗∗,$$,&&P < 0.01, ∗∗∗,$$$,&&&P < 0.001; ∗indicates ME49 group comparisons to the control group, $indicates RH group comparisons to the control group, &indicates RH group comparisons to the ME49 group.
Mice Infected With T. gondii (RH Strain) Had More Proportion of Splenic Th, Splenic Tc, Circulatory Tc Cells and Less Proportion of Splenic B Cells Than That Infected With T. gondii (ME49 Strain)
We compared the frequency of T and B cells in the splenic and circulatory lymphocytes of mice infected with different T. gondii genotypes. Mice infected with RH tachyzoites had weaker splenic B cell response at day 3 and 5 post-infection; at day 7 and 9 post-infection, the frequency of splenic B cells in the RH group remained lower than that of the ME49 group, but it was not significant (Supplementary Figure S3, RH group vs. ME49 group: day 3, 21.38 ± 4.00 vs. 40.44 ± 4.07, P < 0.001; day 5, 33.52 ± 2.66 vs. 52.44 ± 4.28, P < 0.001; day 7, 45.38 ± 12.58 vs. 57.28 ± 2.01, P > 0.05; day 9, 51.74 ± 4.32 vs. 58.50 ± 5.94, P > 0.05). The RH group had significantly more population of splenic Th and Tc cells than the ME49 group at day 3, 5, 7, and 9 post-infection (Figures 4B,D).
The RH group had significantly more proportion of circulatory B cells than the ME49 group at day 3, 5, and 9 post-infection, but had lower frequency of circulatory B cells at day 7 post-infection (Figure 6A, RH group vs. ME49 group: day 3, 27.07 ± 1.50 vs. 11.43 ± 2.32, P < 0.001; day 5, 24.67 ± 1.01 vs. 13.10 ± 2.81, P < 0.01; day 7, 5.07 ± 1.75 vs. 14.60 ± 1.30, P < 0.01; day 9, 14.87 ± 0.91 vs. 8.53 ± 0.76, P < 0.001). There was no significant difference in the population of circulatory Th cells (Figure 6B). The RH group had more proportion of circulatory Tc cells at day 5, 7, and 9 post-infection than the ME49 group (Figure 6C, RH group vs. ME49 group: day 5, 16.30 ± 0.75 vs. 13.73 ± 0.85, P < 0.05; day 7, 14.00 ± 2.98 vs. 7.50 ± 0.17, P < 0.05; day 9, 11.73 ± 0.38 vs. 8.60 ± 1.47, P < 0.05).
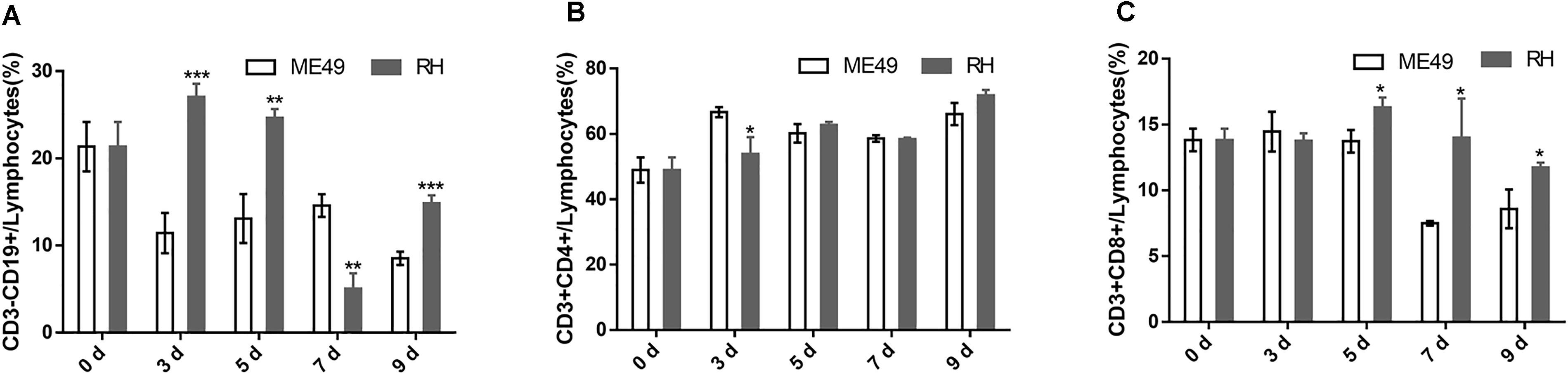
FIGURE 6. The proportion of circulatory T and B cells in mice after infection with T. gondii of the RH or ME49 strain. Circulatory immune cells (A–C) were collected at day 0, 3, 5, 7, and 9 post-infection. (A) The proportions of CD3-CD19+ B cells in circulatory lymphocytes. (B) The proportions of CD3+CD4+ Th cells in circulatory lymphocytes. (C) The proportions of CD3+CD8+ Tc cells in circulatory lymphocytes. The results are representative of three independent experiments with 3–5 mice per group per experiment; data are the means ± SDs. ∗P < 0.05, ∗∗P < 0.01, ∗∗∗P < 0.001; ∗indicates comparisons to the ME49 group.
Discussion
A type I membrane protein, Tim-3 is an important immune regulator that plays widespread and complex roles in the immune system. Tim-3 induces T cell apoptosis through interaction with galectin-9 (Tang and Lotze, 2012). Several studies have shown that Tim-3 can suppress certain aspects of cell function, including enforcing CD8+ T cell exhaustion (Fourcade et al., 2010), suppressing IL-12 secretion (Tomkowicz et al., 2015). During Plasmodium infection, blocking of Tim-3 signaling with an anti–Tim-3 antibody restored lymphocyte activity (Hou et al., 2016) and improves phagocytosis and parasitic mediator production of murine splenic macrophages (Hou et al., 2017). In T. gondii infection, both susceptible C57BL/6 and resistant BALB/c mice had increased frequencies of Tim-3+ cells in the spleen and mesenteric lymph nodes (Berrocal Almanza et al., 2013). Further, differential Tim-3 expression by immune cells from the spleen, mesenteric lymph nodes (Berrocal Almanza et al., 2013), and brain (Wu et al., 2014) has been observed in mice from different backgrounds during T. gondii infection. However, there has been no further investigation on Tim-3 expression on the immune cells of hosts infected with different T. gondii genotypes. Our results revealed the expression of Tim-3 on splenic and circulatory Th and Tc cells in mice was closely associated to the parasite pathogenicity, virulent parasites induced higher Tim-3 expression on splenic and circulatory T cells.
In natural oral infections, T. gondii initially crosses the intestinal epithelium, disseminates into the deep tissues, and traverses biological barriers to reach immunologically privileged sites. In protozoan pathogens, growth rate is a common virulence trait, and T. gondii virulent often correlates with its growth rate (Kaufman et al., 1959). In mice, the genotype influences strain pathogenicity: virulent type I strains consistently display greater migratory capacity compared to the nonvirulent type II and type III strains (Antonio and David, 2002). Tim-3 is often correlated with apoptosis (Fourcade et al., 2010), and virulent strains have superior migratory and invasion capacity (Antonio and David, 2002), which may lead to earlier and faster host cell lysis. Here, Tim-3 expression on critical populations of splenic and circulatory Th cells and Tc cells in mice infected with type I RH T. gondii, a virulent strain, was higher, and T. gondii infection reduced splenic T lymphocytes and increased Tim-3 expression in vivo.
Spleen enlargement may be the first sign of a serious disorder (Motyckova and Steensma, 2012). Monoclonal proliferation from malignant transformation of a lymphoid cell or polyclonal lymphocyte proliferation may enlarge the spleen. Spleen enlargement can also occur due to infiltration of the spleen by nonlymphoid cells, such as inflammatory reaction by neutrophils (Motyckova and Steensma, 2012). Here, we observed post-infection splenomegaly. Study has also demonstrated a direct correlation between the degree of splenomegaly and IgM level produced by B cells (Wells, 1968, 1970). Compared to the RH group, the ME49 group had a higher spleen index and a higher population of splenic B cells which suggests that host infected with low virulent T. gondii strain has a higher chance to increase B cell populations for better protection against the parasite infection.
After T. gondii infection, overstimulation of the immune system will lead to high levels of Th1 cytokines, increased apoptosis, and organ damage (Gavrilescu and Denkers, 2001; Mordue et al., 2001). IL-12 is a proinflammatory cytokine that drives Th1 cell differentiation and forms a link between innate and adaptive immunity, IL-12 also stimulates IFN-γ production (Trinchieri, 2003). The combined effects of IL-12 and IFN-γ are essential to resistance to T. gondii and eventually provoke a very strong Th1-biased CD4+ and CD8+ T cell–mediated immune response (Miller et al., 2009). In the present study, IL-12 in the mouse sera increased after infection. This result further emphasize that T. gondii will trigger a strong Th1 immune response. While the production of IL-12 was significantly lower in the group infected with the RH strain than that infected with the ME49 strain at day 5. IFN-γ release assays can be used as early detection methods of T. gondii infection (Mahmoudi et al., 2017). Here, the RH group had significantly increased IFN-γ secretion at day 3 post-infection. TNF-α is also important for controlling resistance to acute (Johnson, 1992) and chronic (Deckertschlüter et al., 1998) T. gondii infections. Following stimulation with ME49 or RH strain tachyzoite lysate antigens, the spleen cells of mice infected with T. gondii (ME49 strain) had increased IL-4 production (Rodgers et al., 2005). Here, serum IL-4 which is produced by type 2 immune cells was neither increased nor decreased, IL-4 secreted by the spleen cells was not detected in this study. A previous study showed that after infection with Babesia microti, which is also an apicomplexan parasite, Th1 cell–mediated immunity is equally important in clearance of this intracellular pathogen. Further, the secretion of serum cytokines such as IL-4 was not significantly changed (Djokic et al., 2018). Our data further explained the previous findings at molecular level.
Conclusively, our results indicated that Tim-3 expression on splenic and circulatory T Cells and various cytokine responses in infected mice was closely associated with the T. gondii virulence.
Author Contributions
The study was designed by QC. Experiments were performed by YZ, NJ, and TZ. Data were analyzed by QC, NJ, YZ, TZ, DW, YF, XS, and NY. Manuscript was written by QC, NJ, and YZ.
Funding
This research was supported by grants of the National Key Research and Development Program of China (2017YFD0500400 and 2017YFD0501200) and the National Natural Science Foundation of China (Grant Numbers 81420108023 and 81772219).
Conflict of Interest Statement
The authors declare that the research was conducted in the absence of any commercial or financial relationships that could be construed as a potential conflict of interest.
Supplementary Material
The Supplementary Material for this article can be found online at: https://www.frontiersin.org/articles/10.3389/fmicb.2018.02967/full#supplementary-material
References
Antonio, B., and David, S. L. (2002). Transepithelial migration of Toxoplasma gondii is linked to parasite motility and virulence. J. Exp. Med. 195, 1625–1633. doi: 10.1084/jem.20020258
Berrocal Almanza, L. C., Munoz, M., Kuhl, A. A., Kamradt, T., Heimesaat, M. M., and Liesenfeld, O. (2013). Tim-3 is differently expressed in genetically susceptible C57BL/6 and resistant BALB/c mice during oral infection with Toxoplasma gondii. Eur. J. Microbiol. Immunol. 3, 211–221. doi: 10.1556/EuJMI.3.2013.3.10
Brown, C. R., and Mcleod, R. (1990). Class I MHC genes and CD8+ T cells determine cyst number in Toxoplasma gondii infection. J. Immunol. 145, 3438–3441.
Canessa, A., Pistoia, V., Roncella, S., Merli, A., Melioli, G., Terragna, A., et al. (1988). An in vitro model for Toxoplasma infection in man. Interaction between CD4+ monoclonal T cells and macrophages results in killing of trophozoites. J. Immunol. 140, 3580–3588.
Deckertschlüter, M., Bluethmann, H., Rang, A., Hof, H., and Schlüter, D. (1998). Crucial role of TNF receptor type 1 (p55), but not of TNF receptor type 2 (p75), in murine toxoplasmosis. J. Immunol. 160, 3427–3436.
Djokic, V., Akoolo, L., and Parveen, N. (2018). Babesia microti infection changes host spleen architecture and is cleared by a th1 immune response. Front. Microbiol. 9:85. doi: 10.3389/fmicb.2018.00085
Fourcade, J., Sun, Z., Benallaoua, M., Guillaume, P., Luescher, I. F., and Sander, C. (2010). Upregulation of Tim-3 and PD-1 expression is associated with tumor antigen–specific CD8+ T cell dysfunction in melanoma patients. J. Exp. Med. 207, 2175–2186. doi: 10.1084/jem.20100637
Freeman, G. J., Casasnovas, J. M., Umetsu, D. T., and Dekruyff, R. H. (2010). TIM genes: a family of cell surface phosphatidylserine receptors that regulate innate and adaptive immunity. Immunol. Rev. 235, 172–189. doi: 10.1111/j.0105-2896.2010.00903.x
Gavrilescu, L. C., and Denkers, E. Y. (2001). IFN-γ overproduction and high level apoptosis are associated with high but not low virulence Toxoplasma gondii infection. J. Immunol. 167, 902–909. doi: 10.4049/jimmunol.167.2.902
Gleason, M. K., Lenvik, T. R., Mccullar, V., Felices, M., O’brien, M. S., Cooley, S. A., et al. (2012). Tim-3 is an inducible human natural killer cell receptor that enhances interferon gamma production in response to galectin-9. Blood 119, 3064–3072. doi: 10.1182/blood-2011-06-360321
Honoré, S., Couvelard, A., Garin, Y. J., Bedel, C., Hénin, D., Dardé, M. L., et al. (2000). Genotyping of Toxoplasma gondii strains from immunocompromised patients. Pathol. Biol. 48, 541–547.
Hou, N., Jiang, N., Zou, Y., Piao, X., Liu, S., Li, S., et al. (2017). Down-regulation of Tim-3 in monocytes and macrophages in plasmodium infection and its association with parasite clearance. Front. Microbiol. 8:1431. doi: 10.3389/fmicb.2017.01431
Hou, N., Zou, Y., Piao, X., Liu, S., Wang, L., Li, S., et al. (2016). T cell immunoglobulin- and mucin-domain-containing molecule-3 signaling blockade improves cell-mediated immunity against malaria. J. Infect. Dis. 214, 1547–1556. doi: 10.1093/infdis/jiw428
Howe, D. K., and Sibley, L. D. (1995). Toxoplasma gondii comprises three clonal lineages: correlation of parasite genotype with human disease. J. Infect. Dis. 172, 1561–1566. doi: 10.1093/infdis/172.6.1561
Jia, B., Lu, H., Quan, L., Yin, J., Ning, J., and Chen, Q. (2013). Genome-wide comparative analysis revealed significant transcriptome changes in mice after Toxoplasma gondii infection. Parasit. Vectors 6:161. doi: 10.1186/1756-3305-6-161
Johnson, L. L. (1992). A protective role for endogenous tumor necrosis factor in Toxoplasma gondii infection. Infect. Immun. 60, 1979–1983.
Johnson, L. L., Lanthier, P., Hoffman, J., and Chen, W. (2004). Vaccination protects B cell-deficient mice against an oral challenge with mildly virulent Toxoplasma gondii. Vaccine 22, 4054–4061. doi: 10.1016/j.vaccine.2004.03.056
Kaufman, H. E., Melton, M. L., Remington, J. S., and Jacobs, L. (1959). Strain Differences of Toxoplasma gondii. J. Parasitol. 45, 189–190. doi: 10.2307/3286527
Luft, B. J., and Remington, J. S. (1988). AIDS commentary. Toxoplasmic encephalitis. J. Infect. Dis. 157, 1–6. doi: 10.1093/infdis/157.1.1
Mahmoudi, S., Mamishi, S., Suo, X., and Keshavarz, H. (2017). Early detection of Toxoplasma gondii infection by using a interferon gamma release assay: a review. Exp. Parasitol. 172, 39–43. doi: 10.1016/j.exppara.2016.12.008
Mccabe, R., and Remington, J. S. (1988). Toxoplasmosis: the time has come. N. Engl. J. Med. 318, 313–315. doi: 10.1056/NEJM198802043180509
Miller, C. M., Boulter, N. R., Ikin, R. J., and Smith, N. C. (2009). The immunobiology of the innate response to Toxoplasma gondii. Int. J. Parasitol. 39, 23–39. doi: 10.1016/j.ijpara.2008.08.002
Monney, L., Sabatos, C. A., Gaglia, J. L., Ryu, A., Waldner, H., Chernova, T., et al. (2002). Th1-specific cell surface protein Tim-3 regulates macrophage activation and severity of an autoimmune disease. Nature 415, 536–541. doi: 10.1038/415536a
Mordue, D. G., Monroy, F., La, R. M., Dinarello, C. A., and Sibley, L. D. (2001). Acute toxoplasmosis leads to lethal overproduction of Th1 cytokines. J. Immunol. 167, 4574–4584. doi: 10.4049/jimmunol.167.8.4574
Motyckova, G., and Steensma, D. P. (2012). Why does my patient have lymphadenopathy or splenomegaly? Hematol. Oncol. Clin. North Am. 26, 395–408. doi: 10.1016/j.hoc.2012.02.005
Nakayama, M., Akiba, H., Takeda, K., Kojima, Y., Hashiguchi, M., Azuma, M., et al. (2009). Tim-3 mediates phagocytosis of apoptotic cells and cross-presentation. Blood 113, 3821–3830. doi: 10.1182/blood-2008-10-185884
Ndhlovu, L. C., Lopez-Vergès, S., Barbour, J. D., Jones, R. B., Jha, A. R., Long, B. R., et al. (2012). Tim-3 marks human natural killer cell maturation and suppresses cell-mediated cytotoxicity. Blood 119, 3734–3743. doi: 10.1182/blood-2011-11-392951
Purner, M. B., Berens, R. L., Nash, P. B., Van, L. A., Ross, E., Kruse, C., et al. (1996). CD4-mediated and CD8-mediated cytotoxic and proliferative immune responses to Toxoplasma gondii in seropositive humans. Infect. Immun. 64, 4330–4338.
Robben, P. M., Mordue, D. G., Truscott, S. M., Takeda, K., Akira, S., and Sibley, L. D. (2004). Production of IL-12 by macrophages infected with Toxoplasma gondii depends on the parasite genotype. J. Immunol. 172, 3686–3694. doi: 10.4049/jimmunol.172.6.3686
Rodgers, L., Wang, X., Wen, X., Dunford, B., Miller, R., and Suzuki, Y. (2005). Strains of Toxoplasma gondii used for tachyzoite antigens to stimulate spleen cells of infected mice in vitro affect cytokine responses of the cells in the culture. Parasitol. Res. 97, 332–335. doi: 10.1007/s00436-005-1416-5
Sayles, P. C., Gibson, G. W., and Johnson, L. L. (2000). B Cells are essential for vaccination-induced resistance to virulent Toxoplasma gondii. Infect. Immun. 68:1026. doi: 10.1128/IAI.68.3.1026-1033.2000
Su, C., Evans, D., Cole, R. H., Kissinger, J. C., Ajioka, J. W., and Sibley, L. D. (2003). Recent expansion of Toxoplasma through enhanced oral transmission. Science 299, 414–416. doi: 10.1126/science.1078035
Tang, D., and Lotze, M. T. (2012). Tumor immunity times out: TIM-3 and HMGB1. Nat. Immunol. 13, 808–810. doi: 10.1038/ni.2396
Tomkowicz, B., Walsh, E., Cotty, A., Verona, R., Sabins, N., Kaplan, F., et al. (2015). TIM-3 suppresses anti-CD3/CD28-induced TCR activation and IL-2 expression through the NFAT signaling pathway. PLoS One 10:e0140694. doi: 10.1371/journal.pone.0140694
Trinchieri, G. (2003). Interleukin-12 and the regulation of innate resistance and adaptive immunity. Nat. Rev. Immunol. 3, 133–146. doi: 10.1038/nri1001
Webster, J. P. (2010). Review of “toxoplasmosis of animals and humans (Second Edition)” by J.P. Dubey. Parasit. Vectors 3:112. doi: 10.1186/1756-3305-3-112
Wells, J. V. (1968). Serum immunoglobulin levels in tropical splenomegaly syndrome in New Guinea. Clin. Exp. Immunol. 3, 943–951.
Wells, J. V. (1970). Immunological studies in tropical splenomegaly syndrome. Trans. R. Soc. Trop. Med. Hyg. 64, 531–546. doi: 10.1016/0035-9203(70)90075-1
Keywords: Toxoplasma gondii, Tim-3, infection, immune cells, cytokine
Citation: Zhang Y, Jiang N, Zhang T, Wang D, Feng Y, Sang X, Yang N and Chen Q (2018) Toxoplasma gondii Genotype Determines Tim-3 Expression Levels in Splenic and Circulatory T Cells in Mice. Front. Microbiol. 9:2967. doi: 10.3389/fmicb.2018.02967
Received: 06 August 2018; Accepted: 18 November 2018;
Published: 04 December 2018.
Edited by:
Wanderley De Souza, Universidade Federal do Rio de Janeiro, BrazilReviewed by:
Renato Augusto DaMatta, Universidade Estadual do Norte Fluminense Darcy Ribeiro, BrazilJuan Li, The Rockefeller University, United States
Copyright © 2018 Zhang, Jiang, Zhang, Wang, Feng, Sang, Yang and Chen. This is an open-access article distributed under the terms of the Creative Commons Attribution License (CC BY). The use, distribution or reproduction in other forums is permitted, provided the original author(s) and the copyright owner(s) are credited and that the original publication in this journal is cited, in accordance with accepted academic practice. No use, distribution or reproduction is permitted which does not comply with these terms.
*Correspondence: Qijun Chen, cWlqdW5jaGVuNzU5QHN5YXUuZWR1LmNu
†These authors have contributed equally to this work