- 1Department of Microbiology, College of Basic Medical Sciences, Key Laboratory of Microbial Engineering Under the Educational Committee in Chongqing, Army Medical University (Third Military Medical University), Chongqing, China
- 2Institute of Modern Biopharmaceuticals, School of Life Sciences, Southwest University, Chongqing, China
- 3Department of Clinical Laboratory, The Fifth Affiliated Hospital of Guangzhou Medical University, Guangzhou, China
The emergence of vancomycin-intermediate Staphylococcus aureus (VISA) has raised healthcare concerns worldwide. VISA is often associated with multiple genetic changes. However, the relative contributions of these changes to VISA phenotypes are incompletely defined. We have characterized VISA XN108 with vancomycin MIC of 12 μg/ml. Genome comparison revealed that WalK(S221P), GraS(T136I), and RpoB(H481N) mutations possibly contributed to the VISA phenotype of XN108. In this study, the above mutations were stepwise cured, and the phenotypes between XN108 and its derivates were compared. We constructed four isogenic mutant strains, XN108-WalK(P221S) (termed as K65), XN108-GraS(I136T) (termed as S65), XN108-RpoB(N481H) (termed as B65), and XN108-WalK(P221S)/GraS(I136T) (termed as KS65), using the allelic replacement experiments with the native alleles derived from a vancomycin-susceptible S. aureus isolate DP65. Antimicrobial susceptibility test revealed K65 and S65 exhibited decreased vancomycin resistance, whereas B65 revealed negligibly differed when compared with the wild-type XN108. Sequentially introducing WalK(P221S) and GraS(I136T) completely converted XN108 into a VSSA phenotype. Transmission electronic microscopy and autolysis determination demonstrated that cell wall thickening and decreasing autolysis were associated with the change of vancomycin resistance levels. Compared with XN108, K65 exhibited 577 differentially expressed genes (DEGs), whereas KS65 presented 555 DEGs. Of those DEGs, 390 were common in K65 and KS65, including those upregulated genes responsible for citrate cycle and bacterial autolysis, and the downregulated genes involved in peptidoglycan biosynthesis and teichoic acid modification. In conclusion, a VSSA phenotype could be completely reconstituted from a VISA strain XN108. WalK(S221P) and GraS(T136I) mutations may work synergistically in conferring vancomycin resistance in XN108.
Introduction
Staphylococcus aureus is one of the most common pathogens causing hospital and community-associated infections worldwide (Boucher et al., 2010; Uhlemann et al., 2014; Kong et al., 2016). As one of the last-line antibiotics to treat S. aureus infections, vancomycin inhibits cell wall synthesis by binding to the D-alanyl–D-alanine carboxyl terminus of cell wall precursor molecules, thereby preventing the cross-linking of peptidoglycan (Walsh, 2000). However, its increased use in clinical practice has led to the emergence of VISA and hVISA strains, which are increasingly reported globally (Howden et al., 2010; Gardete and Tomasz, 2014; Hu et al., 2016; McGuinness et al., 2017).
VISA isolates usually exhibit several common features, such as thickened cell wall, reduced autolysis, impaired virulence, and vancomycin-intermediate resistance (Hu et al., 2016). However, the molecular mechanisms of VISA formation have not been fully elucidated. Diverse mutations that occur in genes encoding two-component systems (TCSs) play important roles in the formation of VISA, including VraSR (Mwangi et al., 2007), GraSR (Howden et al., 2008; Neoh et al., 2008), and WalKR (Howden et al., 2011; Shoji et al., 2011; McEvoy et al., 2013). WalKR regulates the genes involved in cell wall metabolism (Dubrac et al., 2008). The WalK(S221P), WalK(G223D) or WalR(K208R) mutations in vancomycin resistance have been verified experimentally using clinical or in vitro induced VISA isolates (Howden et al., 2011; Hu et al., 2015; Peng et al., 2017). Functionally, both WalK(S221P) and WalK(G223D) mutations exhibited a reduced autophosphorylation status of WalK, thereby decreasing the phosphorylation and transcriptional activity of WalR (Hu et al., 2015; Peng et al., 2017). GraSR confers vancomycin-intermediate resistance through upregulating dltABCD and mprF genes (Falord et al., 2011, 2012). GraR(N197S) is one of six mutations involved in VISA strain Mu50; GraS(T136I) mutation usually contributes to the formation of hVISA (Howden et al., 2008, 2011; Neoh et al., 2008). Other mutations in certain genes, including msrR, sle1, and rpoB, also play important roles in VISA formation. The RpoB(H481Y) mutation of the β subunit of bacterial RNA polymerase is often presented in VISA isolates to increase the vancomycin resistance level by 1–2 μg/ml (Cui et al., 2010; Matsuo et al., 2011; Watanabe et al., 2011).
A single mutation is usually not sufficient to fully convert a VSSA isolate to the VISA phenotype, and mutations in VISA may have cumulative effects in VISA formation (Hu et al., 2016). To determine the relative contribution of each mutation in VISA formation, the VISA phenotype of Mu50 was completely reconstituted by sequentially introducing mutations [VraS(S329L), MsrR(E146K), GraR(N197S), RpoB(H481Y), Fdh2(A297V), and Sle1(Δ67aa)] into six genes of the VSSA strain N315ΔIP (Katayama et al., 2016). However, genetic swapping is extensive work because several mutations usually exist in a VISA strain, and studies on the complete reconstitution or reversion of VISA are limited. We previously characterized an ST239 SCCmec-III VISA strain XN108 with 12 μg/ml vancomycin MIC (Zhang et al., 2013). Genome sequencing and comparisons showed that WalK(S221P), GraS(T136I), and RpoB(H481N) mutations might contribute to the VISA phenotype of XN108 (Peng et al., 2017). In this study, the above mutations were stepwise cured by introducing the WalK(P221S), GraS(I136T), and RpoB(N481H) alleles derived from a VSSA isolate DP65. The phenotypes, such as vancomycin susceptibilities, bacterial autolytic activities, and cell wall morphologies, between XN108 and its derivates were compared. The differentially expressed genes (DEGs) among XN108 and its derivates were analyzed after RNA-seq.
Materials and Methods
Bacterial Strains, Plasmids, and Growth Conditions
The S. aureus strains and plasmids used in this study are listed in Supplementary Table S1. The cloning and transformation of Escherichia coli DH5α were carried out by standard techniques (TianGen Biotech Co., Ltd., Beijing, China). All S. aureus strains were cultivated in brain heart infusion (BHI) broth or tryptic soy broth (TSB, Oxford) with aeration at 37°C, unless indicated otherwise. The antibiotic chloramphenicol (Sigma Chemical Co., St. Louis, MO, United States) 20 mg/ml was used for selection of the S. aureus transformants.
Antibiotic Susceptibility Tests
Antibiotic susceptibility was determined by E-test with a vancomycin strip according to instructions provided by the manufacturer (bioMérieux, France). The population analysis profile (PAP) method was conducted as previously described (Wootton et al., 2001). Briefly, the vancomycin PAP was determined by serial dilution of an overnight BHI culture and by inoculation of BHI agar (BHIA) containing 0–12 μg/ml of vancomycin. Colonies were counted after incubation for 48 h at 37°C and plotted as numbers of CFU/ml vs. the vancomycin concentration.
Construction of K65, S65, B65, and KS65 Mutants
All allelic replacement strains tested in this study are described in Supplementary Table S1. The candidate genes WalK(P221S), GraS(I136T), and RpoB(N481H) from a VSSA isolate DP65 which has identical molecular types (ST239 and SCCmec-III) to XN108 (Supplementary Table S1) were amplified using primers listed in Supplementary Table S2 and sequentially introduced into XN108 with an allelic replacement strategy as described (Peng et al., 2017). The resulting strains XN108-WalK(P221S), XN108-GraS(I136T), XN108-RpoB(N481H), and XN108-WalK(P221S)/GraS(I136T) were designated as K65, S65, B65, and KS65, respectively. The allelic replacement of each gene was confirmed by DNA sequencing.
Growth Curve
The wild-type VISA strain XN108 and its isogenic derivates were incubated overnight in 2 ml TSB at 37°C with shaking at 200 rpm. The overnight cultures were diluted in 100 ml fresh TSB to obtain the same starting optical density (OD) at 600 nm. The growth of each strain was added to the wells of a 96-well microtiter plate monitored by microplate reader (SpectraMax®M2/M2e, United States) at 1 h intervals for a total of 12 h.
Triton X-100 Stimulated Autolysis
Triton X-100 stimulated autolysis was performed as previously described with some modifications (Xue et al., 2013). Briefly, the strains were grown in TSB to early exponential phase (OD600 = 1.0) at 37°C with shaking. Bacterial cells were collected by centrifugation, washed twice with 0.05 M Tris–HCl buffer (pH 7.5), resuspended in an equal volume of Tris–HCl buffer containing 0.05% (v/v) Triton X-100, and incubated at 37°C with shaking. The decrease in the optical density at 600 nm (OD600) was measured every hour using a microplate reader. The experiment was repeated at least three times.
Transmission Electron Microscopy (TEM)
S. aureus strains were cultured in TSB for 24 h at 37°C with shaking. Samples were prepared as previously described (Yuan et al., 2013) and observed under a TECNAI10 transmission electron microscope (Philips, Netherlands). Cell wall thickness was determined in four separate quadrants of each cell, and 60 cells with nearly equatorial cut surfaces were chosen from each strain for determination. The results are expressed as means ± standard deviations (SD) and the data were evaluated using Student’s t-test, where a P-value < 0.05 was considered statistically significant. All statistical tests were two-tailed.
RNA-seq Analysis and RT-qPCR
For RNA-seq analysis, the total RNA was isolated from XN108, K65, and KS65 as previously described (Yuan et al., 2013), and three biological replicates were prepared for RNA sequencing. Library construction and Illumina sequencing was performed at Novogene Bioinformatics Technology Co., Ltd. (Beijing, China). RNA-seq analysis was performed according to the protocol recommended by the manufacturer (Illumina Inc.). Differentially expressed gene analysis was performed using the DESeq R package (1.10.1). For RT-qPCR detection, the first cDNA was synthesized using a RevertAid First Strand cDNA Synthesis Kit (Thermo Fisher Scientific, United States) from 500 ng of total RNA with random primers in a 20 μl reaction mixture. qPCR was performed with SsoAdvancedTM Universal SYBR®Green Supermix kit (Bio-Rad, United States) using the Bio-Rad CFX96TM Realtime detection system (Bio-Rad, United States). The primers used are listed in Supplementary Table S2. The 16S rRNA gene was selected as the reference gene for normalization. The assays were repeated with three independent biological samples. Statistical analyses were performed based on the Student’s t-test to determine the significance of the gene expression levels, where P < 0.05 was considered statistically significant.
Construction of LacZ Reporter
To construct the reporter plasmid pOS-dlt for detection of the effect of GraS(T136I) on dlt operon expression, a 442-bp fragment of the dlt operon promoter regions was amplified from S. aureus XN108 genomic DNA with the primers pOSdlt-lacZ-5 and pOSdlt-lacZ-3 (Supplementary Table S2). The fragment was digested with BamHI/EcoRI and then cloned into the shuttle vector pOS1 to generate reporter plasmid pOS-dlt. After transformed into S. aureus RN4220, the pOS-dlt was subsequently transformed into S. aureus K65 and KS65, respectively.
β-Galactosidase Activity Assay
The stationary-phase cultures of the K65 and KS65 carrying LacZ reporter plasmid pOS-dlt were diluted 1:100 into TSB with chloramphenical (Sigma Chemical Co., St. Louis, MO, United States), 20 mg/ml. Cells were collected at the early log phase (OD600 of 1.0) and lysed for 30 min at 37°C in 100 μl ABT LSA buffer (60 mM K2HPO4, 40 mM KH2PO4, 100 mM NaCl, 0.01% Triton X-100, 50 μg/ml lysostaphin). We then added 100 μl of ABT buffer and 100 μl of 4 μg/ml ONPG (onitrophenyl-β-D-galactopyranoside) to initiate the reaction. The samples were incubated at 37°C until a yellow color became apparent, and then 1 ml Na2CO3 was added to stop the reaction. The enzyme activities were expressed as Miller units. All samples were tested in triplicate.
Results
Sequentially Introducing WalK(P221S) and GraS(I136T) Sufficiently Convert XN108 to the VSSA Phenotype XN108 is an ST239 and SCCmec-III VISA isolate (vancomycin MIC = 12 μg/ml) characterized in our previous study (Zhang et al., 2013). Genome sequencing and comparison revealed that WalK(S221P), GraS(T136I), and RpoB(H481N) might contribute to the VISA phenotype of XN108 (Peng et al., 2017). Substitution of walK in the VSSA strain N315 with that from XN108 increased the vancomycin MIC of N315 from 1.5 μg/ml to 8 μg/ml, whereas replacement of walK in the XN108 with that from N315 decreased the vancomycin MIC of XN108 from 12 μg/ml to 4 μg/ml (Peng et al., 2017). However, walK from N315 has other mutations including WalK(R222K) and WalK(A468T), in addition to WalK(P221S), as compared with that of XN108 (Supplementary Figure S1). To avoid the effect of extra mutations on drug resistance, the walk, graS, and rpoB genes from the VSSA isolate DP65, which has identical molecular types (ST239 and SCCmec-III) to XN108 and no additional site mutations, were used as WalK(P221S), GraS(I136T), and RpoB(N481H) candidate genes and independently introduced into XN108 through an allelic replacement strategy. The allelic replacement of each gene was confirmed by DNA sequencing (Supplementary Figure S2). The resulting strains XN108-WalK(P221S), XN108-GraS(I136T), XN108-RpoB(N481H), and XN108-WalK(P221S)/GraS(I136T) were designated as K65, S65, B65, and KS65, respectively. Growth curve determination revealed that the growth rate of K65, S65, or KS65 increased slightly compared with that of XN108 (Supplementary Figure S3). Antimicrobial susceptibility test demonstrated that K65 had a vancomycin MIC of 4 μg/ml (Figure 1A), which was equal to that of XN108-WalK(P221S-R222K-A468T) constructed using S. aureus N315 walK (Peng et al., 2017), suggesting that the WalK(R222K) and WalK(A468T) mutations in N315 may not affect the vancomycin resistance. S65 had a vancomycin MIC of 8 μg/ml, while B65 had a vancomycin MIC of 12 μg/ml (Figure 1A), suggesting that the GraS(T136I) mutation also contributes to vancomycin resistance in XN108 whereas the RpoB(H481N) mutation has limited role. Both K65 and S65 presented a VISA phenotype in accordance with the standards of the CLSI (2016). Continuously restoring GraS(T136I) in K65 with GraS(I136T) from DP65 resulted in KS65 to be a VSSA strain with vancomycin MIC of 2 μg/ml (Figure 1A). Population analysis also showed that independent or sequential introduction of WalK(P221S), GraS(I136T) into VISA XN108 decreased vancomycin resistance gradually (Figure 1B).
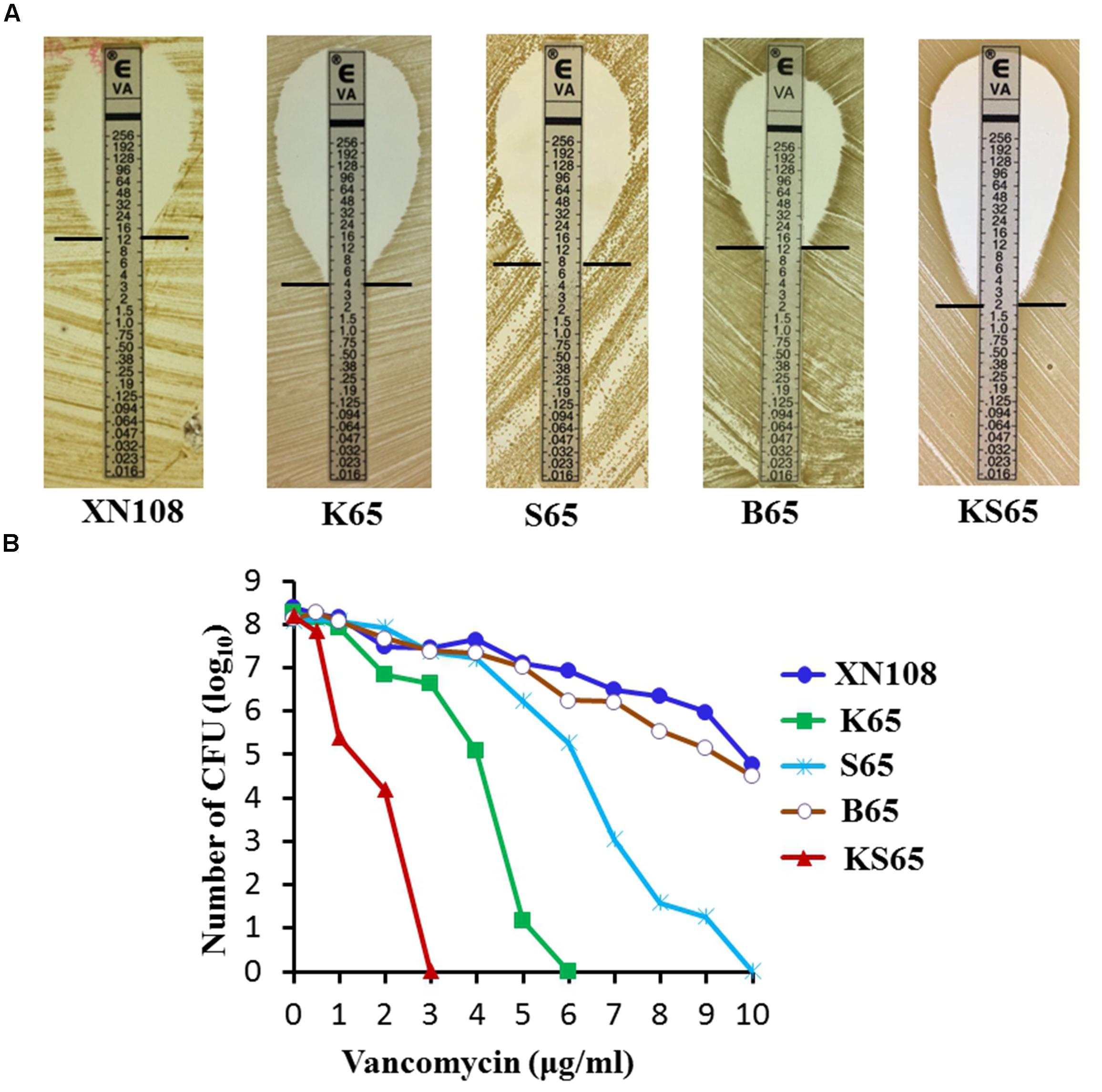
FIGURE 1. Vancomycin susceptibilities of VISA XN108 and its derivates. (A) E-test of vancomycin susceptibilities of XN108, K65, S65, B65, and KS65. (B) Population analysis of the vancomycin susceptibility profiles of XN108, K65, S65, B65, and KS65.
K65 and KS65 Exhibited Decreased Cell Wall Thickening and Increased Autolysis Compared With XN108
A VISA strain usually has a thickened cell wall, which is considered to be associated with the clogging mechanism of vancomycin resistance (Cui et al., 2003; Hu et al., 2016). VISA XN108 exhibited a thickened cell wall (Figure 2A; Zhang et al., 2013). We determined whether the reduced vancomycin resistance in the derivates of XN108 is associated with the decrease in cell wall thickness. Transmission electron microscopy revealed that K65 presented significantly decreased cell wall thickness (28.3 ± 2.9 nm) compared with XN108 (40.1 ± 3.5 nm; P < 0.0001). KS65 showed a normal S. aureus cell wall thickness (22.7 ± 1.4 nm), which is much thinner than that of K65 (Figures 2A,B). In addition, VISA strains often exhibit reduced autolysis (Hu et al., 2016). Autolytic analysis stimulated by Triton X-100 demonstrated that the autolytic activities of K65 and KS65 were gradually increased compared with that of XN108 (Figure 2C).
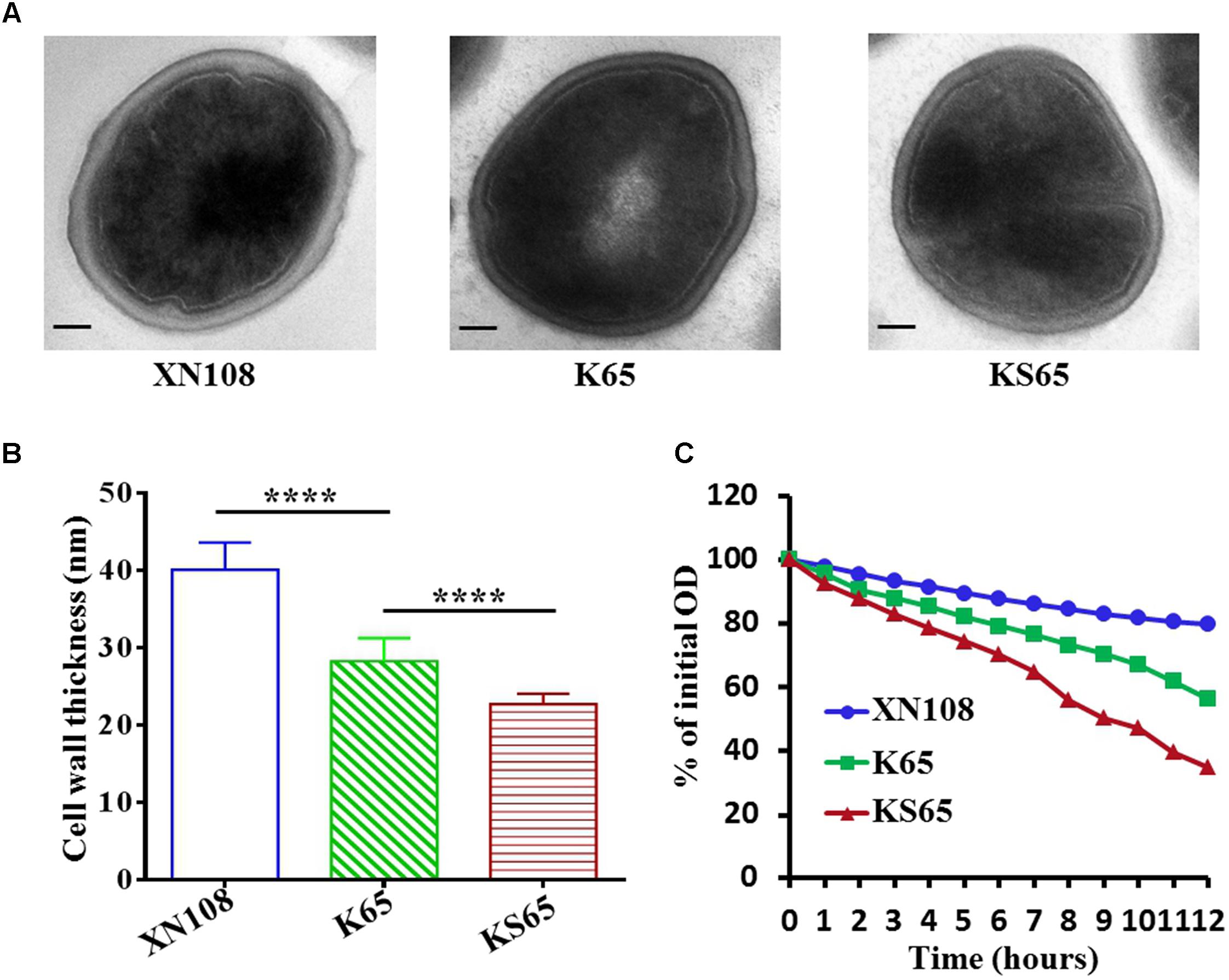
FIGURE 2. Transmission electron microscopy and autolytic analysis of the XN108 and its derivates. (A) Morphological features of VISA XN108 and its derivates K65 and KS65 observed under a transmission electron microscope (magnification, × 120,000; bar = 100 nm). (B) Comparison of cell wall thickness of XN108, K65 and KS65. Data are expressed as the mean ± SD for 60 cells of each strain. Differences were evaluated by Student’s t-test, and all statistical tests were two-tailed. ∗∗∗∗P < 0.0001. (C) Triton X-100 stimulated autolytic analysis. Bacterial cells were grown in BHI broth to mid-logarithmic phase, pelleted, washed twice with ice-cold water, and then analyzed as described in Methods. The percentage of the remaining optical density of each strain at each time point was plotted. The test was repeated 3 times, and 1 representative was indicated.
Comparison of Gene Expression Profiles Among XN108, K65, and KS65
Although numerous mutations in certain genes are associated with VISA phenotypes, their mechanisms on promoting cell wall biosynthesis and impairing bacterial autolysis are incompletely defined (Howden et al., 2010; Hu et al., 2016). The above results showed that the introduction of WalK(P221S) and GraS(I136T) into VISA XN108 completely restored the VSSA phenotype and prompted us to study how WalK(S221P) and GraS(T136I) mutations alter gene expression profiles in VISA strains. Thus, RNA-seq analysis was performed on XN108, K65, and KS65. The differentially expressed genes (DEGs) in the three independent biological repeats were illustrated by Euclidean distance calculation and showed global alteration in gene expression profiles between XN108 and its derivates (Figure 3A). Eight significantly upregulated (sel1, atlA, and isaA) and downregulated (dltA, mprF, femX, pbp2, and mraY) genes in the XN108 derivates, compared with those in XN108, were selected. Their expression levels in K65, KS65, and XN108 were verified by RT-qPCR. The RT-qPCR results were consistent with those obtained after RNA-seq (Figure 3B).
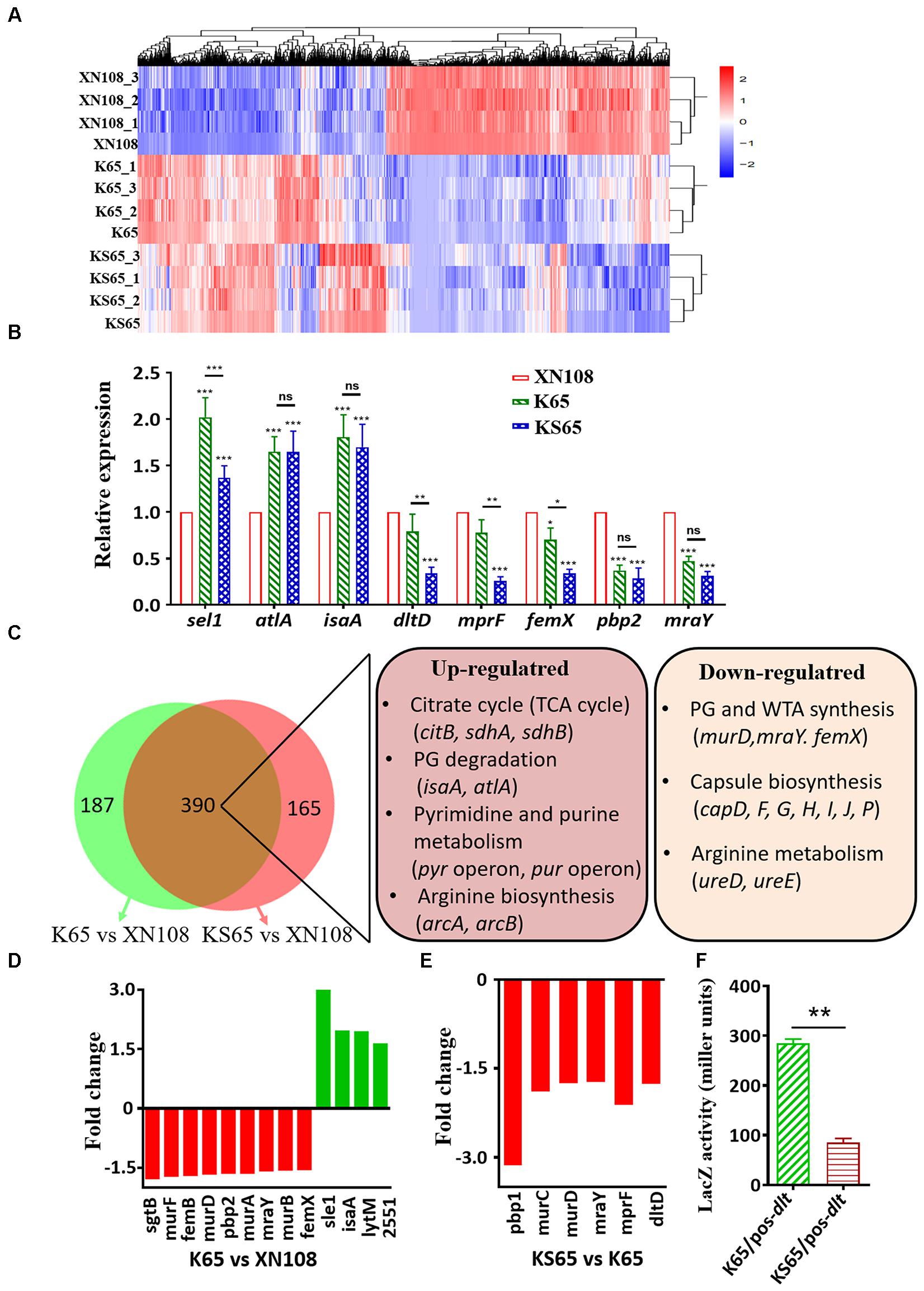
FIGURE 3. Transcriptional profiles of XN108, K65, and KS65. (A) Pairwise Euclidean distance with respect to the transcriptional profiles from each biological repeated sample. (B) RT-qPCR detection of the expression levels of indicated genes in XN108, K65, and KS65. Data from one experiment with three biological replicates were presented as mean ± SD. Expression of each gene of interest in XN108 was normalized to the 16S RNA gene expression and adjusted to 1.0, and their relative expressions in K65 and KS65 strains were indicated. ∗P < 0.05, ∗∗P < 0.01, and ∗∗∗P < 0.001, and ns represents no significance. (C) Schematic representation of the expression altered genes in K65 vs. XN108 (green) and KS65 vs. XN108 (pink). A total of 390 genes were commonly changed, and the major upregulated and downregulated genes are involved in certain biological pathways in KEGG analysis. (D)The fold change of the upregulated (red) and downregulated (green) genes responsible for cell wall biosynthesis and bacterial autolysis in K65 vs. XN108. (E) The fold change of the downregulated genes responsible for cell wall biosynthesis and modification in KS65 vs. XN108. (F) β-galactosidase assay. The pOS-dlt reporter plasmid was transformed into K65 and KS65, respectively. The LacZ activity was detected and represented as mean ± SD (n = 3). ∗∗P < 0.01.
A gene expression determined by RNA-seq and demonstrated a fold change ≥ 1.5 with an adjusted P < 0.05 was included in the analysis. Compared with XN108, K65 with reduced vancomycin resistance had 299 upregulated and 278 downregulated genes (Supplementary Table S3), whereas KS65 with a vancomycin-susceptible phenotype had 260 upregulated and 295 downregulated genes (Supplementary Table S4). However, KS65 had only 42 upregulated and 73 downregulated genes compared with K65 (Supplementary Table S5). WalK(S221P) mutation that plays crucial roles in conferring VISA phenotype in XN108 (Peng et al., 2017) altered more gene expressions than GraS(T136I) did. In total, 390 changed genes in K65 vs. XN108 (67.7%, 390/577) were identical to those in KS65 vs. XN108 (70.3%, 390/555), including those common upregulated genes responsible for citrate cycle, bacterial autolysis, pyrimidine and purine metabolisms, and arginine biosynthesis, and the downregulated genes involved in peptidoglycan, teichoic acid, and capsule biosynthesis, and arginine metabolism based on KEGG analysis (Figure 3C).
WalKR is a master regulon for cell wall metabolism in S. aureus (Dubrac et al., 2008). WalR regulates S. aureus by directly binding to the promoter regions of certain autolysis-associated genes, such as atlA, lytM, isaA, and ssaA (Dubrac and Msadek, 2004). RNA-seq revealed that the expression levels of atlA, isaA, SAXN108_2551, and lytM were upregulated in K65 compared with those in XN108 (Figure 3D and Supplementary Table S3). GraR binds to the promoter regions of dltABCD and mprF to initiate their transcription (Falord et al., 2011). RNA-seq also revealed that the expression levels of dltD and mprF were downregulated in KS65 compared to K65 (Figure 3E). When transformed with a LacZ fusion reporter plasmid pOS-dlt, the β-galactosidase activities in KS65 were significantly decreased compared with those in K65 (P < 0.01, Figure 3F). This result suggested that GraS(T136I) mutation promoted the promoter activity of dlt operon, whereas WalK(S221P) mutation decreased WalKR activity to regulate its downstream genes (Hu et al., 2016; Peng et al., 2017).
Discussion
With the increasing use of vancomycin, VISA infections pose a global threat (Walsh, 2000; Hu et al., 2016). VISA is often associated with multiple genetic changes, but a limited number of mutations in several genes contributing to VISA formation were evaluated, and the regulatory mechanism of vancomycin resistance in VISA strains has not been fully explored (Hu et al., 2016). Several mutations in VISA may work synergistically to promote its common phenotypes, and understanding the relative contribution of each mutation to VISA formation would help in determining the key molecular pathway linking the multiple genetic variations and the common downstream characteristics of VISA. In this study, we performed allelic exchange experiments to independently or stepwise revert the WalK(S221P), GraS(T136I), and RpoB(H481N) mutations in VISA XN108 and assessed the contributions of these mutations to vancomycin susceptibility and other VISA phenotypes. Our data revealed that WalK(S221P) and GraS(T136I) mutations played crucial roles in conferring the VISA phenotype of XN108, whereas RpoB(H481N) had negligible role in the VISA formation of XN108 (Figures 1, 2). RpoB(H481Y) mutation is one of the regulatory mutations increasing the level of resistance to vancomycin, daptomycin, and β-lactams (Katayama et al., 2016). The RpoB(H481Y) mutation exerts a stringent response in S. aureus whereas the role of RpoB(H481N) is not known (Gao et al., 2013). RpoB(H481N) mutation is usually associated with the fitness cost compensation mutation of RpoB(L466S) in the ST239-t030 linage (Shang et al., 2016). In XN108, RpoB(H481N) mutation is indeed associated with RpoB(L466S) mutation. Overall, we showed that RpoB(H481N) in XN108 was unlike RpoB(H481Y), which plays an important role in the VISA formation of Mu50. Thus, the exact function of RpoB(H481N) needs further investigation.
At the genetic level, the reversion of WalK(S221P) together with GraS(T136I) sufficiently convert XN108 to the VSSA phenotype. Mutations in both WalKR and GraSR TCSs are major evolutionary pathways to generate VISA, such as WalK(G223D) and GraS(T136I) in VISA JKD6008 compared with its VSSA parent strain JKD6009 (Howden et al., 2011). However, the mechanism of mutations in WalKR and GraSR that promote the common VISA phenotypes remains unclear. Our RNA-seq analysis revealed that the WalK(S221P) reverted strain K65 and the WalK(S221P)/GraS(T136I) reverted strain KS65 exhibited 390 common DEGs compared with XN108, including those upregulated genes responsible for citrate cycle, bacterial autolysis, pyrimidine and purine metabolisms, and arginine biosynthesis, and the downregulated genes involved in peptidoglycan synthesis, teichoic acid modification, capsule biosynthesis, and arginine metabolism (Figure 3C). Specific and reversible metabolic alterations are usually observed in genetically distinct VISA strains (Nuxoll et al., 2012; Alexander et al., 2014), suggesting that multiple genetic changes in VISA may alter certain metabolic pathways to contribute to common VISA phenotypes.
WalKR is the only essential TCS for viability in S. aureus, and it acts as an information conduit between extracellular structures and intracellular processes required for cell wall metabolism (Dubrac et al., 2008; Peng et al., 2017). Mutations in WalKR observed in VISA strains usually result in reduced WalKR function (Hu et al., 2015; Peng et al., 2017). RT-qPCR and RNA-seq analyses revealed that the downstream genes of WalKR, such as sel1 and atlA, which are responsible for autolysis, were significantly upregulated, whereas those for cell wall biosynthesis, such as femX and pbp2, were significantly downregulated in WalK(S221P) reverted K65 and KS65 compared with those in XN108 (Figures 3B,C). GraSR is a TCS that is involved in glycopeptide resistance by modulating the D-alanylation of teichoic acids and lysylination of phosphatidylglycerol to increase the surface positive charges (Falord et al., 2012). Mutations in the GraSR of VISA strains often increase GraSR function (Hu et al., 2016). LacZ reporter assay showed that the dlt promotor controlled β-galactosidase activities in GraS(T136I) cured strain KS65 were significantly decreased compared with those in K65 (Figure 3E). Simultaneously, the downstream genes of GraSR, such as pbp1, mprF, and dltD, were significantly downregulated in the GraS(T136I) reverted KS65 compared with those in XN108 (Figures 3B–D). The RT-qPCR data revealed that GraSR regulon could overlap with the WalKR regulon in mediating cell wall synthesis. The reversion of WalK(S221P) resulted in the significantly down-regulation of femX, and sequentially cured GraS(T136) further decreased femX expression in KS65 (Figure 3B). This phenomenon was also observed by other investigators (Falord et al., 2011). Taken together, mutations in both WalKR and GraSR could promote decreased autolysis and thickened cell wall, thereby mediating vancomycin resistance in VISA.
In conclusion, adopting the allelic replacement strategy, a VSSA phenotype of the VISA XN108 was completely reconstructed by sequential introduction of WalK(P221S) and GraS(I136T) to cure certain mutations. Here, we proposed a model for the interpretation of vancomycin resistance in VISA XN108 (Figure 4). On the one hand, the mutated and reduced activity of the WalKR system decreased the expression of autolysins and increased pbp2, thereby decreasing autolysis and increasing the cell wall synthesis of XN108. On the other hand, the mutated and activated GraSR system increased the transcription of genes for cell wall synthesis and modification, including pbp1, dltABCD, and mprF, thereby increasing the cell wall biosynthesis and modification. Both WalK(S221P) and GraS(T136I) work synergistically to activate cell wall synthesis, promote cell wall modification, and decrease bacterial autolysis, which are all common features of VISA.
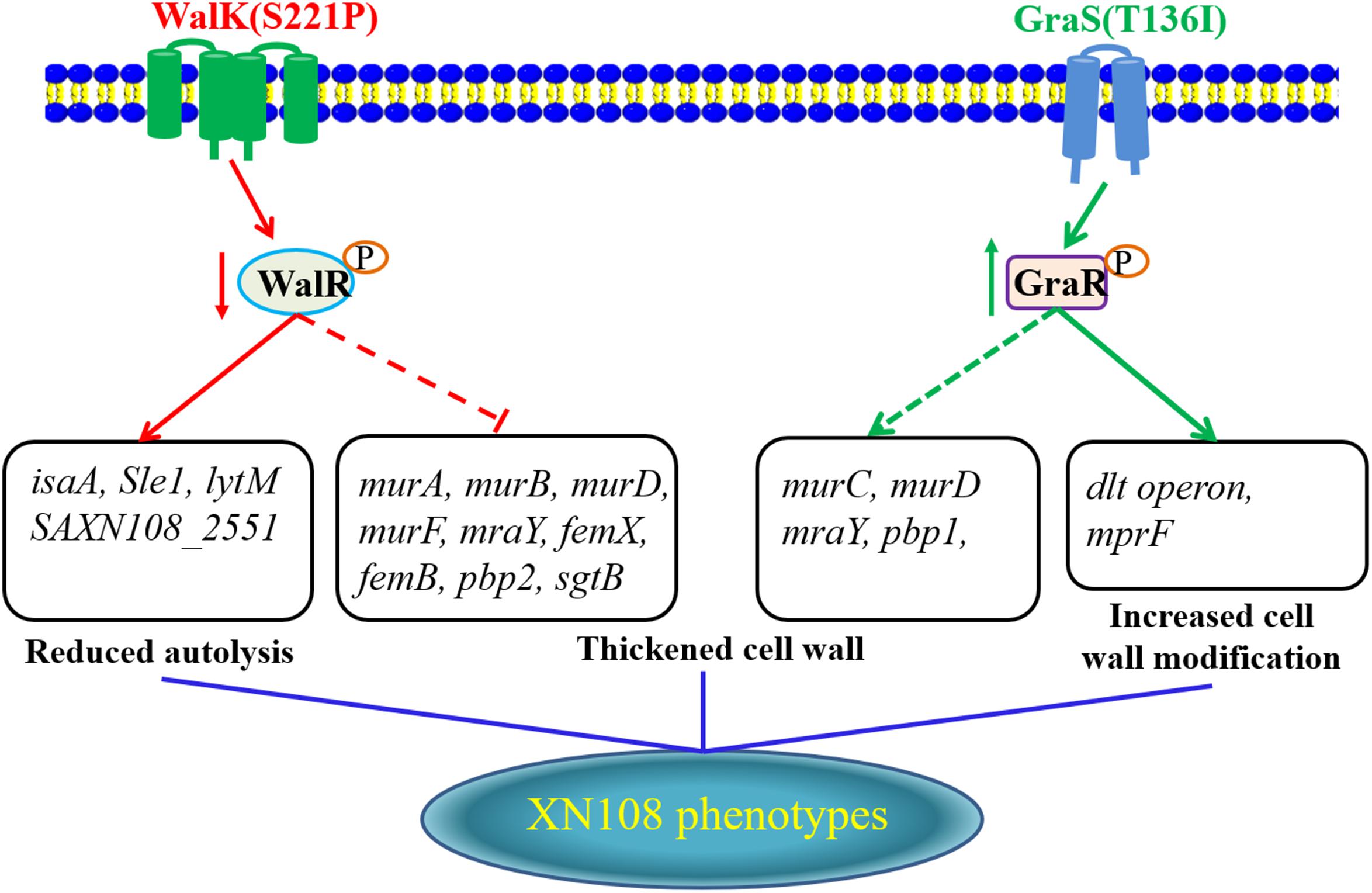
FIGURE 4. Proposed model for how the WalK(S221P) and GraS(T136I) mutations regulate vancomycin resistance in XN108.
Author Contributions
XR and QH conceived the study. HP, YR, WY, and YZ performed the experiments. HP, WS, YY, and QH analyzed the data. ZH, LT, KX, SL, and JZ contributed to reagents and materials. HP, XH, QH, and XR wrote the paper.
Funding
This work was supported by the National Natural Science Foundation of China (Award Nos. 81772237 and 81471993).
Conflict of Interest Statement
The authors declare that the research was conducted in the absence of any commercial or financial relationships that could be construed as a potential conflict of interest.
Supplementary Material
The Supplementary Material for this article can be found online at: https://www.frontiersin.org/articles/10.3389/fmicb.2018.02955/full#supplementary-material
Abbreviation
B65, XN108-RpoB(N481H); CLSI, clinical and laboratory standards institute; DEG, differential expressed gene; hVISA, heterogeneous VISA; K65, XN108-WalK(P221S); KS65, XN108-WalK(P221S)/GraS(I136T); MIC, minimum inhibitory concentration; S65, XN108-GraS(I136T); VISA, vancomycin-intermediate S. aureus; VSSA, vancomycin-susceptible S. aureus.
References
Alexander, E. L., Gardete, S., Bar, H. Y., Wells, M. T., Tomasz, A., and Rhee, K. Y. (2014). Intermediate-type vancomycin resistance (VISA) in genetically-distinct Staphylococcus aureus isolates is linked to specific, reversible metabolicalterations. PLoS One 9:e97137. doi: 10.1371/journal.pone.0097137
Boucher, H., Miller, L. G., and Razonable, R. R. (2010). Serious infections caused by methicillin-resistant Staphylococcus aureus. Clin. Infect. Dis. 51(Suppl. 2), S183–S197. doi: 10.1086/653519
CLSI (2016). Performance Standards for Antimicrobial Susceptibility Testing, Informational Supplement (M100-S26), 26th Edn. Wayne, PA: Clinical and Laboratory Standards Institute.
Cui, L., Isii, T., Fukuda, M., Ochiai, T., Neoh, H. M., Camargo, I. L., et al. (2010). An RpoB mutation confers dual heteroresistance to daptomycin and vancomycin in Staphylococcus aureus. Antimicrob. Agents Chemother. 54, 5222–5233. doi: 10.1128/AAC.00437-10
Cui, L., Ma, X., Sato, K., Okuma, K., Tenover, F. C., Mamizuka, E. M., et al. (2003). Cell wall thickening is a common feature of vancomycin resistance in Staphylococcus aureus. J. Clin. Microbiol. 41, 5–14. doi: 10.1128/JCM.41.1.5-14.2003
Dubrac, S., Bisicchia, P., Devine, K. M., and Msadek, T. (2008). A matter of life and death: cell wall homeostasis and the WalKR (YycGF) essential signal transduction pathway. Mol. Microbiol. 70, 1307–1322. doi: 10.1111/j.13652958.2008.06483.x
Dubrac, S., and Msadek, T. (2004). Identification of genes controlled by the essential YycG/YycF two-component system of Staphylococcus aureus. J. Bacteriol. 186, 1175–1181. doi: 10.1128/JB.186.4.1175-1181.2004
Falord, M., Karimova, G., Hiron, A., and Msadek, T. (2012). GraXSR proteins interact with the VraFG ABC transporter to form a five-component system required for cationic antimicrobial peptide sensing and resistance in Staphylococcus aureus. Antimicrob. Agents Chemother. 56, 1047–1058. doi: 10.1128/AAC.05054-11
Falord, M., Mader, U., Hiron, A., Debarbouille, M., and Msadek, T. (2011). Investigation of the Staphylococcus aureus GraSR regulon reveals novel links to virulence, stress response and cell wall signal transduction pathways. PLoS One 6:e21323. doi: 10.1371/journal.pone.0021323
Gao, W., Cameron, D. R., Davies, J. K., Kostoulias, X., Stepnell, J., Tuck, K. L., et al. (2013). The RpoB H481Y rifampicin resistance mutation and an active stringent response reduce virulence and increase resistance to innate immune responses in Staphylococcus aureus. J. Infect. Dis. 207, 929–939. doi: 10.1093/infdis/jis772
Gardete, S., and Tomasz, A. (2014). Mechanisms of vancomycin resistance in Staphylococcus aureus. J. Clin. Invest. 124, 2836–2840. doi: 10.1172/JCI68834
Howden, B. P., Davies, J. K., Johnson, P. D., Stinear, T. P., and Grayson, M. L. (2010). Reduced vancomycin susceptibility in Staphylococcus aureus, including vancomycin-intermediate and heterogeneous vancomycin-intermediate strains: resistance mechanisms, laboratory detection, and clinical implications. Clin. Microbiol. Rev. 23, 99–139. doi: 10.1128/CMR.00042-09
Howden, B. P., McEvoy, C. R., Allen, D. L., Chua, K., Gao, W., Harrison, P. F., et al. (2011). Evolution of multidrug resistance during Staphylococcus aureus infection involves mutation of the essential two component regulator WalKR. PLoS Pathog. 7:e1002359. doi: 10.1371/journal.ppat.1002359
Howden, B. P., Stinear, T. P., Allen, D. L., Johnson, P. D., Ward, P. B., and Davies, J. K. (2008). Genomic analysis reveals a point mutation in the two-component sensor gene graS that leads to intermediate vancomycin resistance in clinical Staphylococcus aureus. Antimicrob. Agents Chemother. 52, 3755–3762. doi: 10.1128/AAC.01613-07
Hu, J., Zhang, X., Liu, X., Chen, C., and Sun, B. (2015). Mechanism of reduced vancomycin susceptibility conferred by walK mutation in community-acquired methicillin-resistant Staphylococcus aureus strain MW2. Antimicrob. Agents Chemother. 59, 1352–1355. doi: 10.1128/AAC.04290-14
Hu, Q., Peng, H., and Rao, X. (2016). Molecular events for promotion of vancomycin resistance in vancomycin intermediate Staphylococcus aureus. Front. Microbiol. 7:1601. doi: 10.3389/fmicb.2016.01601
Katayama, Y., Sekine, M., Hishinuma, T., Aiba, Y., and Hiramatsu, K. (2016). Complete reconstitution of the vancomycin-intermediate Staphylococcus aureus phenotype of strain Mu50 in vancomycin-susceptible S. aureus. Antimicrob. Agents Chemother. 60, 3730–3742. doi: 10.1128/AAC.00420-16
Kong, E. F., Johnson, J. K., and Jabra-Rizk, M. A. (2016). Community-associated methicillin-resistant Staphylococcus aureus: an enemy amidst us. PLoS Pathog. 12:e1005837. doi: 10.1371/journal.ppat.1005837
Matsuo, M., Hishinuma, T., Katayama, Y., Cui, L., Kapi, M., and Hiramatsu, K. (2011). Mutation of RNA polymerase beta subunit (rpoB) promotes hVISA-to-VISA phenotypic conversion of strain Mu3. Antimicrob. Agents Chemother. 55, 4188–4195. doi: 10.1128/AAC.00398-11
McEvoy, C. R., Tsuji, B., Gao, W., Seemann, T., Porter, J. L., Doig, K., et al. (2013). Decreased vancomycin susceptibility in Staphylococcus aureus caused by IS256 tempering of WalKR expression. Antimicrob. Agents Chemother. 57, 3240–3249. doi: 10.1128/AAC.00279-13
McGuinness, W. A., Malachowa, N., and DeLeo, F. R. (2017). Vancomycin resistance in Staphylococcus aureus. Yale. J. Biol. Med. 90, 269–281.
Mwangi, M. M., Wu, S. W., Zhou, Y., Sieradzki, K., de Lencastre, H., Richardson, P., et al. (2007). Tracking the in vivo evolution of multidrug resistance in Staphylococcus aureus by whole-genome sequencing. Proc. Natl. Acad. Sci. U.S.A. 104, 9451–9456. doi: 10.1073/pnas.0609839104
Neoh, H. M., Cui, L., Yuzawa, H., Takeuchi, F., Matsuo, M., and Hiramatsu, K. (2008). Mutated response regulator graR is responsible for phenotypic conversion of Staphylococcus aureus from heterogeneous vancomycin-intermediate resistance to vancomycin-intermediate resistance. Antimicrob. Agents Chemother. 52, 45–53. doi: 10.1128/AAC.00534-07
Nuxoll, A. S., Halouska, S. M., Sadykov, M. R., Hanke, M. L., Bayles, K. W., Kielian, T., et al. (2012). CcpA regulates arginine biosynthesis in Staphylococcus aureus through repression of proline catabolism. PLoS Pathog. 8:e1003033. doi: 10.1371/journal.ppat.1003033
Peng, H., Hu, Q., Shang, W., Yuan, J., Zhang, X., Liu, H., et al. (2017). WalK(S221P), a naturally occurring mutation, confers vancomycin resistance in VISA strain XN108. J. Antimicrob. Chemother. 72, 1006–1013. doi: 10.1093/jac/dkw518
Shang, W., Hu, Q., Yuan, W., Cheng, H., Yang, J., Hu, Z., et al. (2016). Comparative fitness and determinants for the characteristic drug resistance of ST239-MRSA-III-t030 and ST239-MRSA-III-t037 strains isolated in China. Microb. Drug Resist. 22, 185–192. doi: 10.1089/mdr.2015.0226
Shoji, M., Cui, L., Iizuka, R., Komoto, A., Neoh, H. M., Watanabe, Y., et al. (2011). walK and clpP mutations confer reduced vancomycin susceptibility in Staphylococcus aureus. Antimicrob. Agents Chemother. 55, 3870–3881. doi: 10.1128/AAC.01563-10
Uhlemann, A. C., Otto, M., Lowy, F. D., and DeLeo, F. R. (2014). Evolution of community- and healthcare-associated methicillin-resistant Staphylococcus aureus. Infect. Genet. Evol. 21, 563–574. doi: 10.1016/j.meegid.2013.04.030
Walsh, C. (2000). Molecular mechanisms that confer antibacterial drug resistance. Nature 406, 775–781. doi: 10.1038/35021219
Watanabe, Y., Cui, L., Katayama, Y., Kozue, K., and Hiramatsu, K. (2011). Impact of rpoB mutations on reduced vancomycin susceptibility in Staphylococcus aureus. J. Clin. Microbiol. 49, 2680–2684. doi: 10.1128/JCM.02144-10
Wootton, M., Howe, R. A., Hillman, R., Walsh, T. R., Bennett, P. M., and MacGowan, A. P. (2001). A modified population analysis profile (PAP) method to detect hetero-resistance to vancomycin in Staphylococcus aureus in a UK hospital. J. Antimicrob. Chemother. 47, 399–403. doi: 10.1093/jac/47.4.399
Xue, T., Zhao, L., and Sun, B. (2013). LuxS/AI-2 system is involved in antibiotic susceptibility and autolysis in Staphylococcus aureus NCTC 8325. Int. J. Antimicrob. Agents 41, 85–89. doi: 10.1016/j.ijantimicag.2012.08.016
Yuan, W., Hu, Q., Cheng, H., Shang, W., Liu, N., Hua, Z., et al. (2013). Cell wall thickening is associated with adaptive resistance to amikacin in methicillin-resistant Staphylococcus aureus clinical isolates. J. Antimicrob. Chemother. 68, 1089–1096. doi: 10.1093/jac/dks522
Keywords: Vancomycin-intermediate Staphylococcus aureus, vancomycin resistance, WalKR, GraSR, RpoB
Citation: Peng H, Rao Y, Yuan W, Zheng Y, Shang W, Hu Z, Yang Y, Tan L, Xiong K, Li S, Zhu J, Hu X, Hu Q and Rao X (2018) Reconstruction of the Vancomycin-Susceptible Staphylococcus aureus Phenotype From a Vancomycin-Intermediate S. aureus XN108. Front. Microbiol. 9:2955. doi: 10.3389/fmicb.2018.02955
Received: 15 August 2018; Accepted: 16 November 2018;
Published: 28 November 2018.
Edited by:
Dongsheng Zhou, Beijing Institute of Microbiology and Epidemiology, ChinaReviewed by:
Min Li, Shanghai Jiao Tong University School of Medicine, ChinaHe Yan, South China University of Technology, China
Copyright © 2018 Peng, Rao, Yuan, Zheng, Shang, Hu, Yang, Tan, Xiong, Li, Zhu, Hu, Hu and Rao. This is an open-access article distributed under the terms of the Creative Commons Attribution License (CC BY). The use, distribution or reproduction in other forums is permitted, provided the original author(s) and the copyright owner(s) are credited and that the original publication in this journal is cited, in accordance with accepted academic practice. No use, distribution or reproduction is permitted which does not comply with these terms.
*Correspondence: Qiwen Hu, aHVxaXdlbjIwMDRAMTYzLmNvbQ== Xiancai Rao, cmFveGlhbmNhaUAxMjYuY29t