- 1IFAPA, Centro Las Torres-Tomejil, Seville, Spain
- 2Departamento de Microbiología, Facultad de Biología, Universidad de Sevilla, Avenida Reina Mercedes, Seville, Spain
- 3Department of Botany and Plant Biology, University of Geneva, Geneva, Switzerland
- 4Institute of Microbiology, Heilongjiang Academy of Sciences, Harbin, China
- 5Departamento de Biología Celular, Facultad de Biología, Universidad de Sevilla, Seville, Spain
Sinorhizobium fredii indigenous populations are prevalent in provinces of Central China whereas Bradyrhizobium species (Bradyrhizobium japonicum, B. diazoefficiens, B. elkanii, and others) are more abundant in northern and southern provinces. The symbiotic properties of different soybean rhizobia have been investigated with 40 different wild soybean (Glycine soja) accessions from China, Japan, Russia, and South Korea. Bradyrhizobial strains nodulated all the wild soybeans tested, albeit efficiency of nitrogen fixation varied considerably among accessions. The symbiotic capacity of S. fredii HH103 with wild soybeans from Central China was clearly better than with the accessions found elsewhere. S. fredii NGR234, the rhizobial strain showing the broadest host range ever described, also formed nitrogen-fixing nodules with different G. soja accessions from Central China. To our knowledge, this is the first report describing an effective symbiosis between S. fredii NGR234 and G. soja. Mobilization of the S. fredii HH103 symbiotic plasmid to a NGR234 pSym-cured derivative (strain NGR234C) yielded transconjugants that formed ineffective nodules with G. max cv. Williams 82 and G. soja accession CH4. By contrast, transfer of the symbiotic plasmid pNGR234a to a pSym-cured derivative of S. fredii USDA193 generated transconjugants that effectively nodulated G. soja accession CH4 but failed to nodulate with G. max cv. Williams 82. These results indicate that intra-specific transference of the S. fredii symbiotic plasmids generates new strains with unpredictable symbiotic properties, probably due to the occurrence of new combinations of symbiotic signals.
Introduction
Rhizobia are α- and β-proteobacteria able to establish nitrogen-fixing symbioses with legumes (Sprent et al., 2017). The enormous ecological and economic importance of legume crops justifies the many extensive studies carried out these past four decades on rhizobia-legume symbioses. Different species belonging to the Sinorhizobium (=Ensifer) and Bradyrhizobium genera induce the formation of nitrogen-fixing nodules on soybean roots (Margaret et al., 2011; Yang et al., 2018). Glycine max [L.] Merr. (soybean) is the most important pulse legume in the world with its seeds as essential sources of proteins and oils. However, soybean is not only an important source of proteins and oils, but also a rich source of nutraceuticals compounds including bioflavonoids, lecithins, oligosaccharides, phytosterols, saponins, and tocopherols (Rosell et al., 2004; Hamilton-Reeves et al., 2007). Glycine soja (Siebold and Zucc.) is the wild ancestor of the domesticated soybean, Glycine max (recently reviewed by Kofsky et al., 2018). G. soja is native to East Asia and can be found in a broad geographic range that encompasses from East Russia to South China. Although wild and domesticated soybeans differ in many characteristics, the fact that they have the same number of chromosomes (2n = 40) and are cross-compatible make wild soybean very attractive as a potential genetic source of interesting traits that can have been partially or totally lost during domestication and improvement of G. max (Kofsky et al., 2018).
Soybeans are cropped on an estimated 6% of the world’s arable land and, in the last 40 years, areas under soybean production increased more than any other major crop (Hartman et al., 2011). Such continuous increase in soybean production is to match a growing demand for soybean-based meals and oils. Total world soybean production has increased from 17 million metric tons (MMT) in 1960 to 320 MMT in 2015, according to the USDA data (Westcott and Hansen, 2016). Soybean production is still expected to raise with improved yields and development of additional production areas. The increase in soybean cropping is concomitant with a burgeoning inoculant industry. In fact, the development of effective rhizobial inoculants for this crop has been the key to its spread into non-native regions of the world (Alves et al., 2003).
China is the center of origin and diversification of soybean plants, where it has been cultivated for more than 5,000 years (Mammadov et al., 2018; Yang et al., 2018). According to ancient texts and mass spectrometry analyses of carbonized soybean seeds, the origin of soybean could be delimited from the North-eastern Hebei province to South-eastern areas of Northeast China. China should also be the center of origin of soybean rhizobia and the place where both symbionts coevolved. Apparently, the presence of root nodules was documented by Chinese writers from the very beginning of soybean cultivation, as illustrated by the ancient Chinese character “Shu” (soybean) that includes two dots below a horizontal stroke, which symbolizes root nodules belowground (Qiu and Chang, 2010). Slow-growing rhizobia isolated from either soybean or wild soybean nodules collected in Chinese soils belonged to diverse bradyrhizobia species including those currently classified as Bradyrhizobium diazoefficiens, B. elkanii, B. japonicum, B. liaoningense, and B. yuanmingense, while fast-growing soybean rhizobia belong to Sinorhizobium fredii, S. sojae or Sinorhizobium spp. (Tian et al., 2012; Zhao et al., 2014). The distribution of slow- versus fast-growing soybean rhizobia in Chinese provinces varies along a North–South axis (Tian et al., 2012), with fast-growers absent in Northern acidic soils (Heilongjiang, Jilin, and Liaoning provinces) that are mainly colonized by Bradyrhizobium species (Tian et al., 2012). Similarly, nodules of soybean plants grown in the acidic soils of Hubei, Anhui, and Jiangsu in Central China and all those provinces situated in the South mostly contain Bradyrhizobium strains (Figure 1) with few occurrences of S. fredii isolates (Tian et al., 2012). By contrast, the alkaline soil from Central China defined by the Huang-Huai-Hai rivers (Hebei, Shanxi, Henan, and Shandong provinces) mainly contains fast-growing soybean rhizobia, with S. fredii being the most abundant, as well as occasionally a few strains of B. elkanii, B. liaoningense, B. yuanmingense, and Bradyrhizobium spp. (Tian et al., 2012). Additional studies further highlighted the predominance of slow-growers in acidic soils while fast-growers dominated rhizobia populations in alkaline soils (Yang et al., 2018; Zhang et al., 2018). Thus, soil pH appears to be a decisive criterion in determining whether fast- or slow-growing soybean rhizobia communities will populate fields, including soils from alkaline spots of otherwise acidic soils of Honghu county (Hubei province) where S. fredii populations were found to be predominant (Camacho et al., 2002). In fact, sinorhizobial strains are often associated with legumes that are native to alkaline soils, not only in China but also in other countries such as India (Sankhla et al., 2017; Rathi et al., 2018).
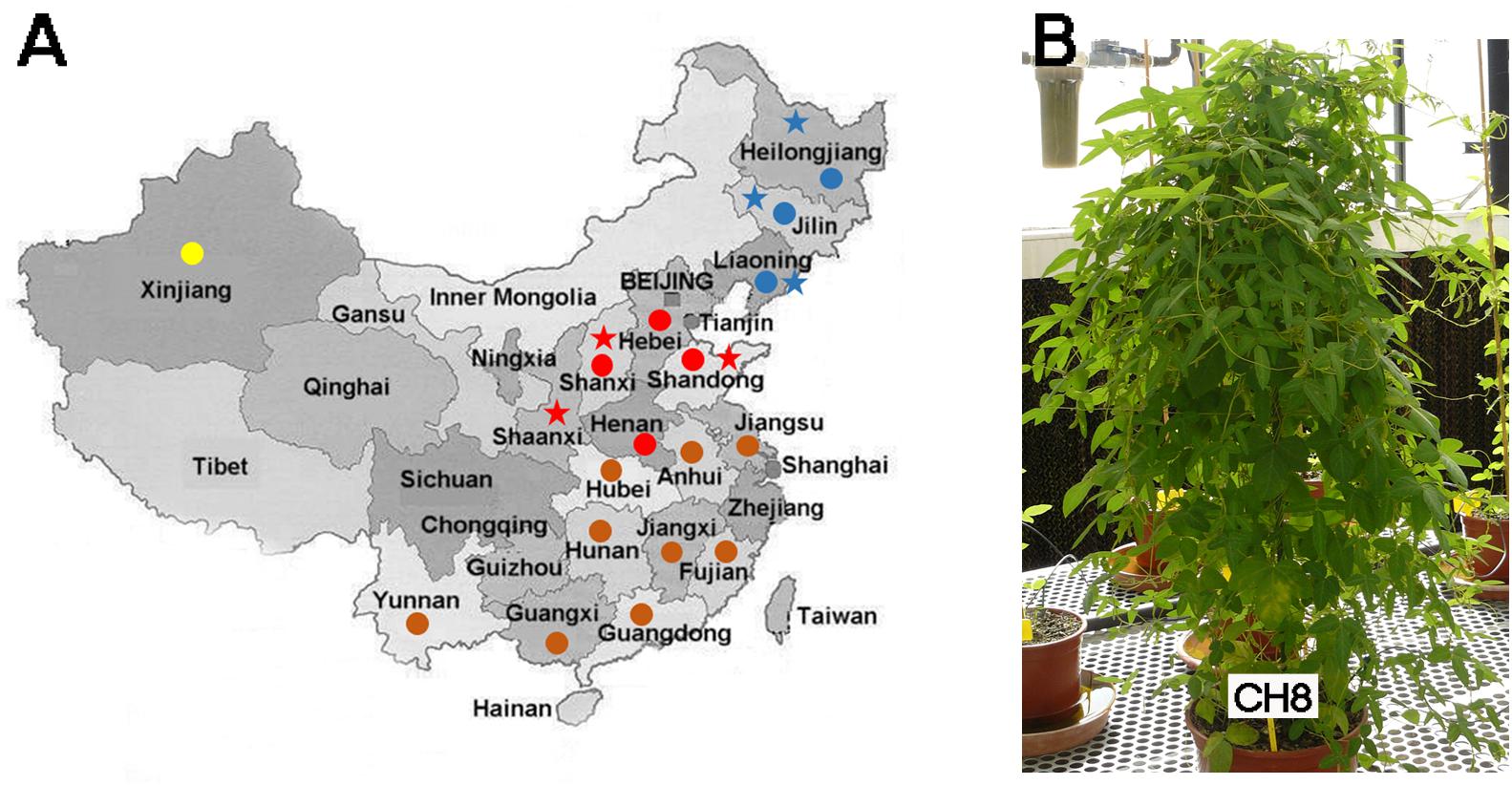
FIGURE 1. (A) Biogeography of Glycine soja cultivars analyzed in this work (stars) and soybean-nodulating rhizobia (circles) studied by Tian et al. (2012). Blue and red stars indicate Northern and Central Chinese provinces, respectively. Blue, red, brown, and yellow circles indicate Northern, Central, Southern, and Western Chinese provinces respectively. According to Tian et al. (2012), Sinorhizobium species are predominant in Central China whereas Bradyrhizobium species predominate in Northern and Southern China. In Xinjiang (Western China), the proportions of Sinorhizobium and Bradyrhizobium species are similar. (B) A wild G. soja cultivar (CH8, from Heilongjiang, Northern China) grown in greenhouse conditions in Seville, Spain.
The genetic differentiation and diversity from Glycine soja Sieb. & Zucc. to G. max [L.] Merr. has been studied at the DNA sequence level (Li et al., 2010, 2014; Zhou et al., 2015) and with proteomic analyses (Natarajan et al., 2007; Xu et al., 2007). Most of the reported results indicated that genetic diversity in G. soja was higher than in G. max (Qiu and Chang, 2010). The comparison of the genomes of seven G. soja accessions showed lineage-specific genes with variations in copy numbers and large-effect mutations, some of which may have contributed to the selection of important agronomic traits such as biotic resistance, composition of seeds, times of flowering and maturity, organ size and final biomass (Li et al., 2014).
Natural phenotypic changes affecting plant development, flowering time, seed size and color or dormancy have taken place during soybean domestication processes (Zhou et al., 2015; Mammadov et al., 2018). Wild soybeans (G. soja) can be a source of many elite traits, such as tolerance to salt (Luo et al., 2005), cold and dehydration stresses (Chen et al., 2006), or high lutein content (Kanamaru et al., 2006). Hence, wild soybeans can be used in breeding programs to obtain fertile hybrids of G. max and G. soja crosses (Singh, 2007; Li et al., 2010). In such G. soja/G. max hybrids, various important characteristics such as seed size, plant height and/or tolerance to waterlogging can be selected for, with some intermediate phenotypes occurring as well (Qiu and Chang, 2010).
In this work we compared the symbiotic capacity of 40 wild soybean accessions from Russia, South Korea, Japan, and China to associate with three different well-known soybean rhizobia strains: B. diazoefficiens USDA110T, B. elkanii USDA76T, and S. fredii HH103. Wild soybeans from North or Central China showed lower symbiotic efficiency with S. fredii HH103 than with bradyrhizobia strains USDA110T and USDA76T. By contrast, in association with G. soja accessions from Central China, strain HH103 was equally or more efficient than USDA110T and USDA76T strains.
In addition, several G. soja accessions from Central China inoculated with S. fredii NGR234 were found to form nitrogen-fixing nodules. Out of 30 rhizobia isolated from nodules of Lablab purpureus collected in a slightly alkaline soil (pH 8.5) of Papua New Guinea, NGR234 was the only fast-growing strain (soil pH 8.5) (Trinick, 1980). Because of its unsurpassed host-range (Pueppke and Broughton, 1999), NGR234 became a model to study the molecular basis of symbiotic promiscuity (Broughton et al., 2000; Perret et al., 2000), although this strain failed to nodulate all G. max or G. soja varieties tested so far (Pueppke and Broughton, 1999). Thus, to our knowledge, this is the first report of NGR234 forming proficient nitrogen-fixing nodules with G. soja accessions.
Materials and Methods
Bacterial Strains, Plasmids, Culture Conditions, and Media
Bacterial strains and plasmids used in this study are listed in Supplementary Table S1. S. fredii strains were cultivated at 28°C in/on TY (Beringer, 1974), RMS (Broughton et al., 1986), or YMA (Vincent, 1970) media. B. diazoefficiens USDA110T and B. elkanii USDA76T were grown in/on YMA medium. Escherichia coli strains were cultivated on LB medium (Sambrook and Russell, 2001) at 37°C. When necessary, TY or YMA media were supplemented with streptomycin (50 μg/ml), rifampicin (50 μg/mL), chloramphenicol (5 μg/ml), kanamycin (25 μg/ml), neomycin (50 μg/ml) tetracycline (10 μg/ml), or spectinomycin (50 μg/ml). The isoflavone genistein was dissolved in ethanol and used at a final concentration of 1 μg/ml (3.7 μM). Plasmids were transferred from E. coli to rhizobia by conjugation as described by Simon (1984). Plasmid pMUS262 was used to detect conjugational transfer of symbiotic plasmids as described by Vinardell et al. (1993).
Construction of Sinorhizobium fredii Hybrids by Intra-Specific Transfer of S. fredii Symbiotic Plasmids
To provide a selectable marker in the transfer of plasmids by conjugation, the S. fredii NGR234 symbiotic plasmid pSfNGR234a was tagged with transposon Tn5-Mob, which confers neomycin resistance (NmR). Random Tn5-Mob mutagenesis was carried out by crossing E. coli strain S17-1 carrying the suicide plasmid pSUP5011 with NGR234. A NmR derivative of NGR234, called NGR234-M, was identified by its capacity to transfer the NmR marker via a triparental cross between NGR234-M (as donor), E. coli HB101 (pRK2013) (as helper) and Agrobacterium tumefaciens GMI9023 CmR (pMUS262) (as recipient). Plasmid pMUS262 carries the tet gene from pBR322 [coding for tetracycline efflux MFS transporter Tet(C) protein, WP_010891057] under the control of a nodA promoter (pnodA). This construct only confers resistance to tetracycline when recipient cells are grown in presence of a flavonoid inducer, such as genistein, that activates the NodD1 transcriptional regulator for pnodA::tet expression (Vinardell et al., 1993). Thus, only the symbiotic plasmid pSfNGR234a that carries a functional copy of nodD1 could change the NmR A. tumefaciens strain GMI9023 CmR (pMUS262) into a transconjugant resistant to tetracycline when it was grown in the presence of genistein (Vinardell et al., 1993). Then, the symbiotic plasmid of NGR234-M carrying a Tn5-Mob insertion, now called pSfNGR234a::Tn5-Mob or pSymNGR234M, was mobilized into the pSym cured derivative strain called S. fredii USDA193C (Buendía-Clavería et al., 1989). Using another tri-parental mating, the symbiotic plasmid of S. fredii HH103 marked with Tn5-Mob (called pSfHH103d::Tn5-Mob or pSymHH103M; Romero, 1993) was transferred to ANU265 (here after called NGR234C), a derivative strain of NGR234 cured of pNGR234a (Morrison et al., 1983).
Plant Tests
Nodulation tests on G. max (L.) Merr. cultivar Williams 82 (American soybean cultivar) were carried out as previously described (Buendía-Clavería et al., 1989; Yang et al., 2001). Leonard jars were used to grow plants for 6 weeks in a plant-growth chamber with a 16 h photoperiod at 25°C in the light and 18°C in the dark. G. max [L] and G. soja Sieb. & Zucc. (wild soybean) accessions from Japan, Korea, Russia, and China (Figure 1, Table 1, and Supplementary Table S2) were tested in a plant-growth chamber or in a greenhouse using Leonard jars. Because many G. soja accessions have very hard seed coats, treatments with sulfuric acid for 20 min followed by three washing steps with sterilized water were carried out. After this scarification treatment, seeds were surface sterilized with sodium hypochlorite 5% (w/v) for 5 min followed by six washes with sterilized water. Surface sterilized seeds were germinated on 1% (w/v) agar-water at 28°C for 2–3 days. Three or four germinated seeds were transferred to each sterilized Leonard jar consisting of a lower vessel with 500 ml of a pH 7 N-free half-strength nutrient solution (Rigaud and Puppo, 1975) supplemented with trace elements (Turner and Gibson, 1980) and an upper vessel with 300 ml of a mixture of moistened 2/1 (v/v) vermiculite-perlite (Camacho et al., 2002). Each seed was inoculated with approximately 108 bacteria. Jars were placed in a greenhouse under natural light supplemented with light lamps to reach 14 h daylight with a daily minimum-maximum temperature of 18/32°C or in a plant-growth chamber with a photoperiod of 16 h light/day and a temperature range of 18–25°C (night–day). A week after emergence, seedlings were thinned to two per jar. Forty two or 60 days after inoculation, plant tops were dried at 80°C for 48 h and weighed. The relative efficiency index (REI), that measures the accumulation of N fixed in relation to the controls (Brockwell et al., 1966), was calculated for each inoculant/wild-soybean combination. REI was defined as (I-U/N-U) × 100, where (I), (U), and (N) correspond to dry-weight of shoots in each inoculation treatment (I) and in the corresponding uninoculated (U), and nitrogen-fertilized (N) controls. Each jar of the N-fertilized controls received 120 mg N, divided in three treatments of 30, 40, and 50 mg N that were applied at 15, 25, and 35 days of cultivation. Bacterial isolation from surface-sterilized nodules was carried out as previously described (Buendía-Clavería et al., 2003). These nodulation assays were carried out at IFAPA Research Center (Sevilla, Spain) and Harbin (China).
Additional nodulation tests on G. soja CH2 and CH4, and those on Leucaena leucocephala, Tephrosia vogelii, and Vigna radiata cv. King were carried out at the University of Geneva (Switzerland) using Magenta jars. Seeds were surface sterilized as previously described (Fumeaux et al., 2011) and incubated for 2 days, at 27°C and in the dark to germinate. Once germinated, seedlings were planted in Magenta jars (two seedlings per jar) containing sterile vermiculite (Lewin et al., 1990) and watered with nitrogen-free B&D nutritive solution (Broughton and Dilworth, 1971). After 2–4 days recovery, each seedling was inoculated with 2 × 108 freshly grown bacteria. Plants were grown in controlled conditions with a 12 h photoperiod, a day temperature of 27°C and a night temperature of 20°C. To confirm the identity of bacteria found inside nodules, the 5′-end of the 23S rRNA genes of nodule bacteria was amplified using total genomic DNA of nodules as templates (Fossou et al., 2016) together with the 23S-For1 (5′-ACGAACTAGTGTCAAAAGGGC-3′) and ITS-Rev2 (5′-TGGTCCGCGTTCGCTCGCC-3′) primers. The resulting amplicons of NGR234 (317 bp) and HH103 (380 bp) were clearly discriminated after migration on a 1% agarose gel.
Experiments of competition for nodulation using pairs of co-inoculants among S. fredii HH103 RifR, B. diazoefficiens USDA110T or B. elkanii USDA76T, were carried out on G. soja accessions CH2, CH3, and CH4. In accession CH2, only the USDA110T/USDA76T combination was used, since it does not nodulate with strain HH103 RifR. Bacteria were grown to mid-log phase and G. soja plants were inoculated with 1 ml of a mixture of bacterial competitors containing 108 (O.D. ∼ 0.1) bacteria at a 1:1 ratio. Plants were grown for 60 days under greenhouse conditions. Nodule occupancy was determined by the differential intrinsic antibiotic-resistance of the inoculants used: tetracycline (10 μg/ml) to positively differentiate bradyrhizobia (resistant) from HH103 RifR (sensitive) and streptomycin (50 μg/ml) to distinguish between USDA110T (sensitive) and USDA76T (resistant) bradyrhizobia strains. In addition, rifampicin (50 μg/ml) was also used to distinguish between USDA110T (sensitive) and HH103 (resistant). These wild soybean accessions were also used in competition experiments between HH103 and its mutant derivatives affected in the T3SS HH103-1 rhcJ::Tn5::lacZ (=SVQ288) and HH103 RifR ttsI::Ω (=SVQ533). Nodule occupancy by mutants SVQ288 or SVQ533 was determined by plating bacterial nodule-isolates on TY media supplemented with neomycin (50 μg/ml) or spectinomycin (100 μg/ml), respectively.
Microscopy Studies
Small fragments of nodules were fixed in 4% (v/v) glutaraldehyde prepared in 0.1 M cacodylate buffer, pH 7.2 for 1 h at 4°C. Samples were washed in 0.1 M cacodylate buffer, pH 7.2 for 12 h and post-fixed in 1% OsO4 for 1 h at 4°C. Then, samples were dehydrated in ethanol at progressively higher concentrations and embedded in Epon (epoxy embedding medium). Toluidine blue-stained semi-thin sections (0.5 μm thick) used as controls were viewed in a Leitz (Aristoplan) light microscope.
Thin sections (60-80 nm thick) were cut on a Reichert-Jung Ultracut E ultramicrotome, stained with uranyl acetate and lead citrate, and examined in a Libra 120 Plus transmission electron microscope (TEM) from Carl Zeiss (Germany) at an accelerating voltage of 80 kV.
Results
Symbiotic Efficacy of B. diazoefficiens USDA110T, B. elkanii USDA76T, and S. fredii HH103 RifR on Soybean Accessions From Russia, North Korea, Japan, and China
Twenty wild soybean (G. soja) accessions from Russia (R1 to R5), South Korea (K1 to K5), Japan (J1 to J5), and China (CH1 to CH5) from the USDA-Soybean Germplasm Collection were inoculated with three different soybean rhizobia strains: B. diazoefficiens USDA110T, B. elkanii USDA76T, and S. fredii HH103 RifR. Two independent nodulation tests showed that USDA110T and USDA76T formed nitrogen fixing nodules with all accessions tested.
Some particular accessions, such as K3, K4, J2, R2, R5, CH1, CH2, CH3, and CH5 inoculated with USDA110T produced REI values higher than 50%, and three accessions (K2, K5, and R3) gave REI values higher than 80% (Table 2 and Supplementary Table S3). The highest REI value in G. soja plants inoculated with USDA76T was obtained with accession R4 (57.7%). REI values higher than 40% were found with Korean (K2 and K3), Russian (R3), Northern (CH1 and CH2) and Central China (CH3, CH4, and CH5) accessions (Table 2 and Supplementary Table S4). Wild-soybean accessions from Russia, Korea, Japan, and Northern China nodulated with HH103 RifR but REI values were lower than 5% (Table 2 and Supplementary Table S5). Accession CH2 (Jilin province, Northern China) failed to nodulate with HH103 RifR. In contrast, one of the accessions tested from Central China (CH4, Shanxi province) gave a REI value of 54%, which was associated with healthy plant growth and absence of nitrogen deficiency symptoms (Supplementary Table S5).
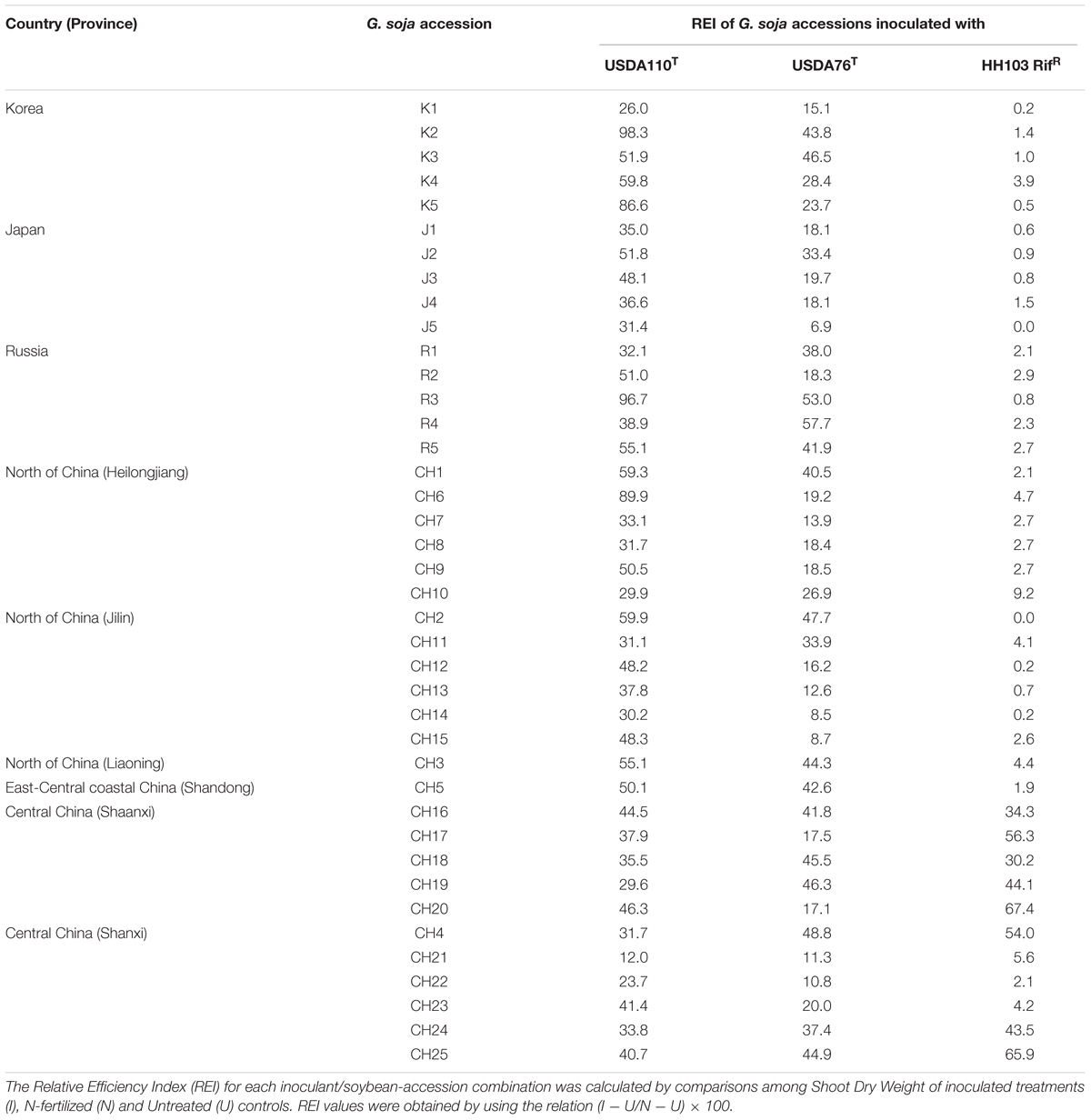
TABLE 2. Relative Efficiency Indexes (REI) of Glycine soja accessions from Korea, Japan, Russia, and China when grown in combination with B. diazoefficiens USDA110T, B. elkanii USDA76T or S. fredii HH103 RifR.
Indigenous S. fredii populations are predominant in soils of Central China while B. japonicum and other slow-growing soybean-rhizobial strains are more abundant in soils of Northern and Southern China (Figure 1; Tian et al., 2012). To investigate whether the relative abundance of fast- and slow-growing populations of indigenous soybean rhizobia in particular areas correlates with symbiotic preferences of local G. soja accessions to nodulate with slow- or fast-growing soybean rhizobia, 10 accessions from Shanxi and Shaanxi provinces (Central China) and 10 wild soybeans from Heilongjiang and Jilin provinces (Northern China) were tested in combination with USDA110T, USDA76T, or HH103 RifR. USDA110T formed nitrogen-fixing nodules with the 20 wild soybeans tested. REI values close or equal to 50% were found with accessions CH9, CH12, and CH15, all from Northern China (Table 2 and Supplementary Table S6). One accession (CH6) from Heilongjiang province gave a remarkably high REI value of 89.9%. USDA76T also nodulated all of the 20 accessions (Supplementary Table S7). None of the accessions from Northern or Central China gave REI values higher than 46.5%. Nine out of the 10 accessions from Northern China inoculated with HH103 RifR formed nodules, although REI values were below 9.5% (Table 2 and Supplementary Table S8). In contrast, all of the 10 accessions from Central China were nodulated by HH103 RifR and 5 of them gave REI values in the range of 43–67%.
Competition Experiments Between B. diazoefficiens USDA110T, B. elkanii USDA76T and S. fredii HH103 RifR to Nodulate Different Wild Soybean Accessions
HH103 RifR formed nitrogen-fixing nodules with G. soja accessions CH3 (REI 4.4%) and CH4 (REI 54%). Competition experiments for nodulation of accessions CH3 and CH4 were carried out between HH103 RifR and USDA110T or USDA76T. Both bradyrhizobial strains outcompeted HH103 RifR to nodulate CH3 and CH4. HH103 Rifr did not form nodules with accession CH3 when co-inoculated with both bradyrhizobial strains. On CH4, nodules occupied exclusively by HH103 RifR were less than 20% of the total number of nodules analyzed (Table 3). Similar experiments carried out between USDA110T and USDA76T showed that strain USDA110T was always more competitive with accession CH2 and CH3 from Northern China, but less competitive with accession CH4 from Central China (Table 3).
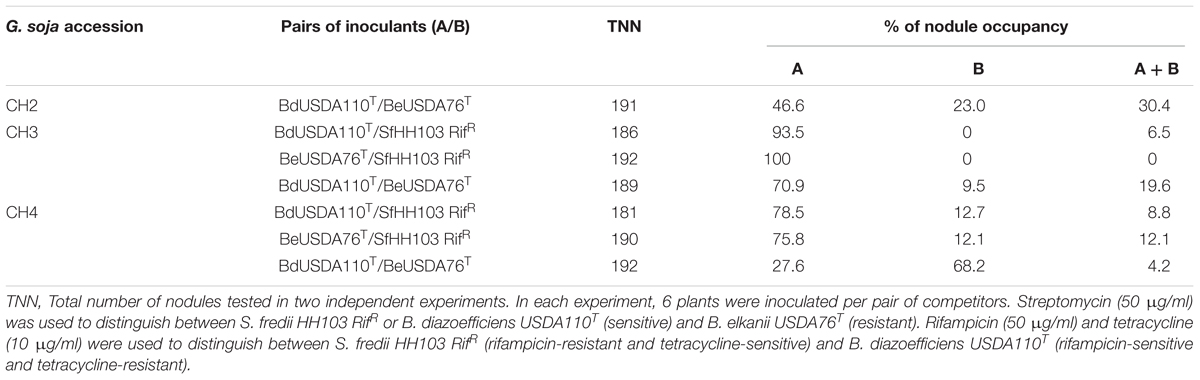
TABLE 3. Nodule occupancy of Glycine soja accessions CH2, CH3, and CH4 inoculated with pairs of competitors formed among B. diazoefficiens (Bd) USDA110T, B. elkanii (Be) USDA76T or S. fredii (Sf) HH103 RifR.
S. fredii NGR234 Forms Nitrogen-Fixing Nodules With Wild Soybeans From Central China
Since the G. soja accession CH4 (Central China) formed proficient symbiosis with HH103 RifR, we tested whether NGR234 was also capable of inducing the formation of nitrogen-fixing nodules on CH4 roots. Accession CH2 (Northern China), which was unable to nodulate with HH103, was also included in the nodulations tests. CH4 plants inoculated with NGR234 formed nitrogen-fixing nodules that effectively contributed to plant growth (Table 4 and Figure 2), whereas on CH2 accession NGR234 induced the formation of more nodules than on CH4 but plants were poorly developed. To our knowledge, this is the first report showing that S. fredii NGR234 can form proficient symbiosis with G. soja plants. Then, bacteria from nodules formed on CH2 and CH4 plants were isolated and identified. Isolates from well-developed nodules of CH2 and CH4 plants inoculated with NGR234 were resistant to rifampicin, a chromosomal antibiotic-resistance maker for this strain. To further confirm the presence of NGR234, a pair of PCR primers that specifically amplifies the 5′ end of the 23S rRNA gene was used. These primers can also be used to distinguish between NGR234 and HH103 strains, since the size of the predicted amplicons are of 317 and 380 bp, respectively. PCR using bacteria isolated from nodules formed on CH2 plants inoculated with NGR234 only amplified the DNA fragment corresponding to this strain (Figure 3).
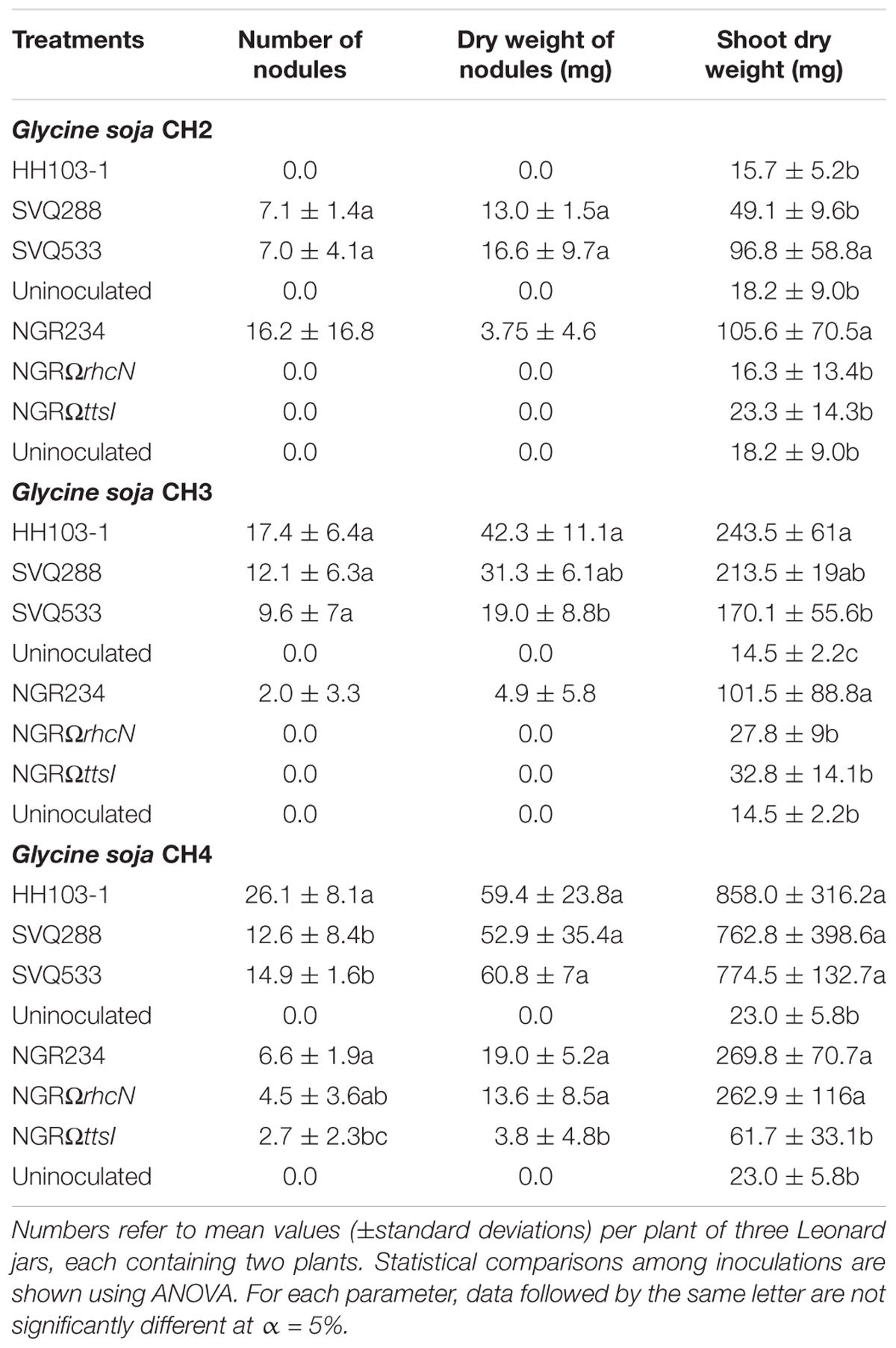
TABLE 4. Symbiotic responses of Glycine soja accessions CH2, CH3, and CH4 to inoculation with diverse S. fredii HH103 and NGR234 mutants affected in the Type Three Secretion System (T3SS).
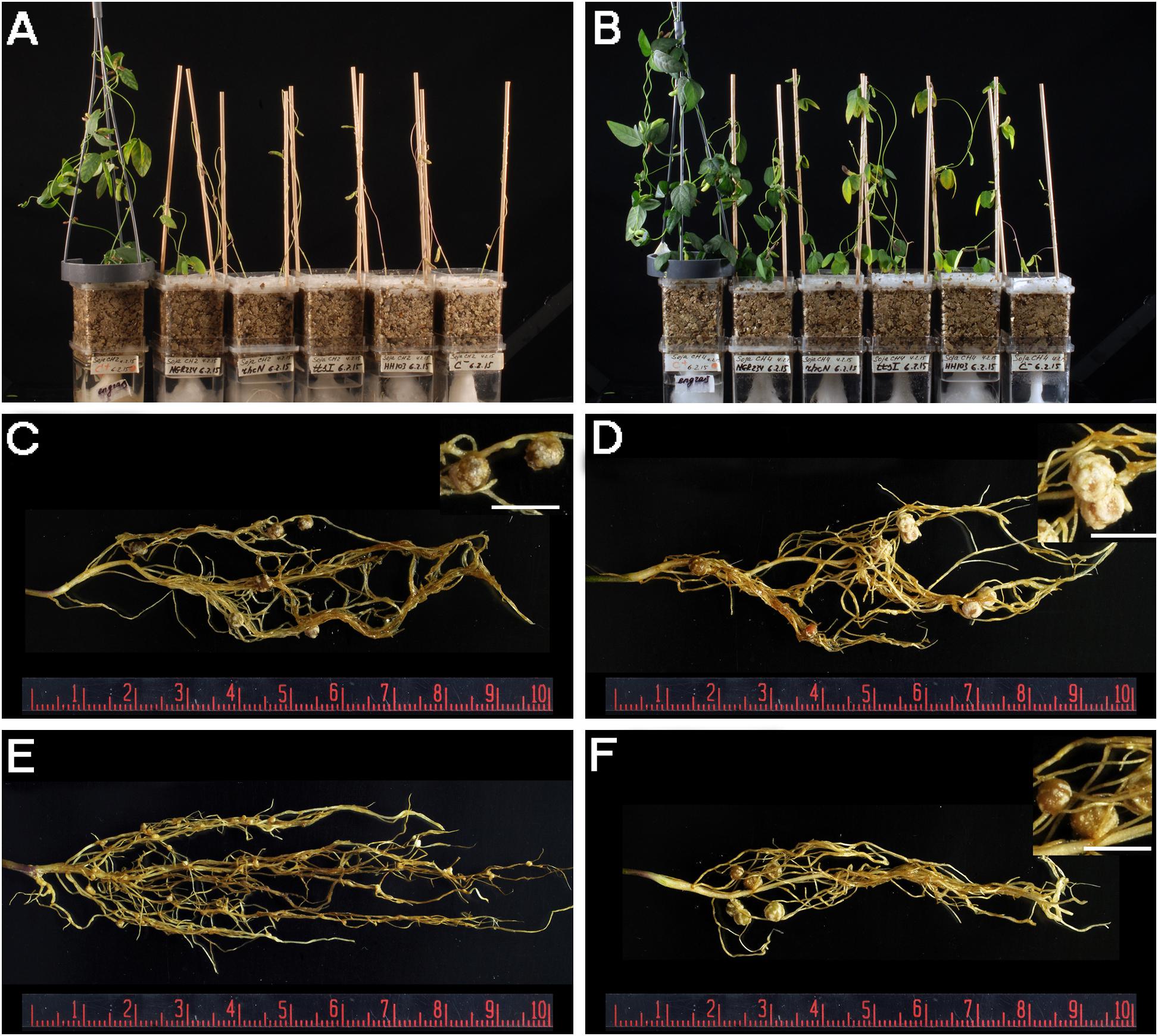
FIGURE 2. (A,B) Plant responses of G. soja accessions CH2 (A) and CH4 (B) inoculated with different S. fredii strains. From left to right, Magenta jars were treated as follows: N-fertilizer, NGR234, NGRΩrhcN, NGRΩttsI, HH103 RifR, and uninoculated control. (C,D) Nitrogen-fixing nodules induced by NGR234 on CH2 (C) and CH4 (D) roots. (E) Ineffective nodules induced by HH103 RifR on CH2 roots. (F) Nitrogen-fixing nodules induced by HH103 RifR on CH4 roots. White bars (C,D,F) correspond to 5 mm.
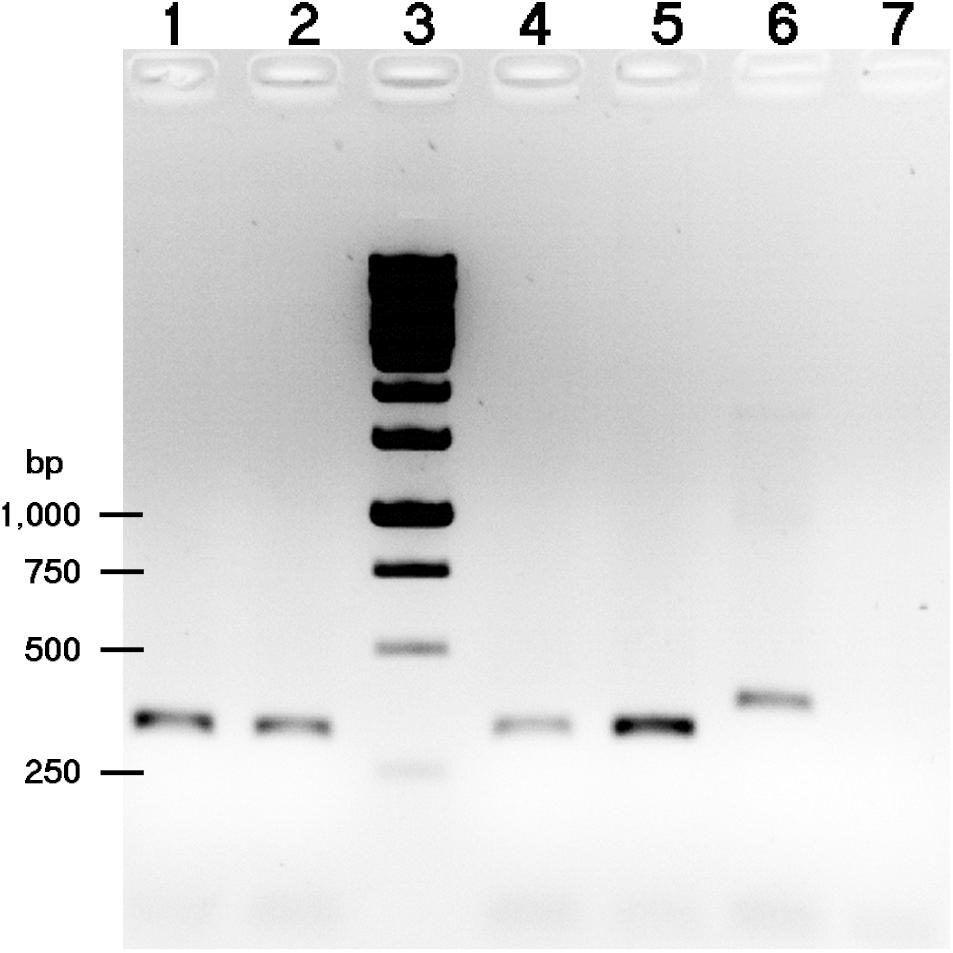
FIGURE 3. Separation of 23S-For1+ITS-Rev2 PCR amplified products on 1% agarose gel. The templates used were as follows: lanes 1, 2, and 4, gDNA from bacteria isolated from different nodules of G. soja CH2 inoculated with NGR234; lane 5, NGR234 gDNA; lane 6, HH103 RifR gDNA; lane 7, no DNA. Lane 3, DNA molecular weight marker (bands corresponding to 1,000, 750, 500, and 250-bp are indicated on the left).
To investigate whether NGR234 was also able to effectively nodulate other wild soybean accessions from Central China, six accessions from Shanxi or Shaanxi provinces were inoculated with this strain. HH103 RifR was used as positive control in these experiments, which showed that NGR234 formed nitrogen-fixing nodules on all of the six accessions tested, albeit associations were clearly less proficient than those formed by HH103 RifR (Table 5).
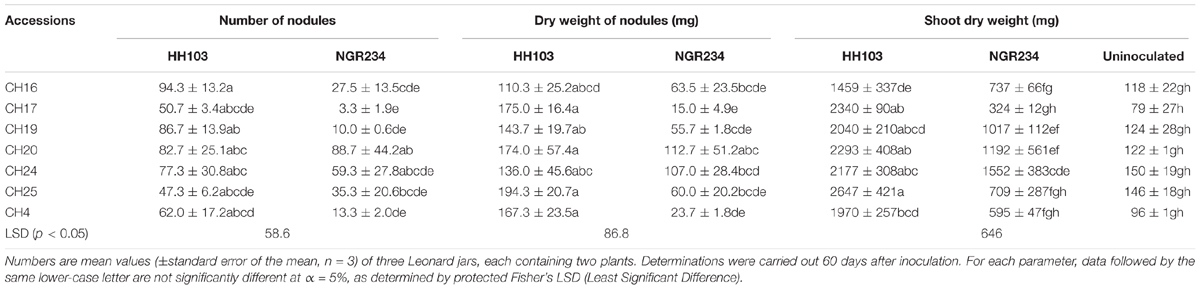
TABLE 5. Symbiotic responses of some Glycine soja accessions from Central China with S. fredii HH103 and NGR234 strains.
Type Three Secretion Systems (T3SS) of S. fredii HH103 and NGR234 Determine Symbiotic Compatibility With Some G. soja Accessions
It has been established that secretion of nodulation outer proteins (Nop) through type three secretion systems (T3SS) modulates symbiotic compatibility of S. fredii strains with different G. max cultivars and other legume species (for a review see López-Baena et al., 2016). T3SS deliver effector proteins into the cytoplasm of animal and plant cells. In rhizobia, genes encoding structural components of symbiotically active T3SS and effector proteins secreted by these systems are controlled by a transcriptional regulator called TtsI (for a recent review see Staehelin and Krishnan, 2015). Amongst the many proteins assembled into a functional secretion machinery, RhcJ (first annotated as NolT in S. fredii strain USDA257) is embedded in the protein ring that spans the inner membrane of bacteria. By contrast, the nodulation outer protein (Nop) NopJ is one of the several effectors that together contribute to define the host range of strain NGR234 (Kambara et al., 2009). To test whether Nop-secretion also contributed to symbioses with wild soybean accessions from different regions of China, the nodulation properties of parent (HH103 and NGR234) and T3SS-deficient (SVQ288, a mutant of HH103 in RhcJ, and NGRΩrhcN) strains were compared.
Neither a streptomycin-resistant derivative (S. fredii HH103-1) nor a rifampicin resistant mutant (S. fredii HH103 RifR) of HH103 nodulated G. soja CH2, an accession from Northern China (Table 4 and Supplementary Table S5). Interestingly, mutants of S. fredii HH103 in ttsI (strain SVQ533) or rhcJ (strain SVQ288), both of which cannot secrete Nops, induced the formation of nodules (Table 4). These nodules, however, poorly contributed to plant growth. Conversely, S. fredii NGR234 that induced the formation of nitrogen-fixing nodules with G. soja CH2, completely lost its nodulation capacity when T3SS was inactivated as in mutants NGRΩrhcN or NGRΩttsI (Table 4). Both S. fredii strains HH103-1 and NGR234 induced the formation of nodules with G. soja accession CH3 (Northern China), with a shoot dry weight of inoculated plants significantly higher than that of uninoculated CH3 plants. While SVQ288 and SVQ533 mutants retained the ability to nodulate CH3 accession, the NGRΩrhcN and NGRΩttsI could no longer induce the formation of nodules (Table 4). By contrast the less selective G. soja accession CH4 from Central China nodulated with all the HH103 and NGR234 mutants tested: plants inoculated with SVQ288 and SVQ533 strains formed fewer nodules but shoot dry weight was not significantly altered when compared to CH4 plants inoculated with HH103-1 at 60 days post-inoculation (dpi). G. soja CH4 responses to inoculation with NGRΩrhcN or NGR234 were similar, while nodulation and nitrogen-fixation of NGRΩttsI was significantly reduced on CH4 (Table 4).
As previously described, S. fredii strains HH103 RifR and HH103-1 failed to nodulate G. soja CH2. As SVQ288 and SVQ533 mutants deficient in T3SS-dependent secretion gained nodulation, it seems likely that secretion of HH103 Nop complement triggers defensive responses in CH2 plants. Therefore, we tested whether presence of HH103 RifR would block nodulation of CH2 accession by the proficient symbiont USDA110T. When co-inoculated at ratios of 1:1 and 1:10, USDA110T and HH103 RifR inocula formed at 25 dpi as many as 7.0 ± 1.8 and 7.1 ± 1.4 nodules/plant, respectively. Thus, the presence of HH103 RifR does not appear to block nodulation of CH2 accession by USDA110T.
Competition experiments for nodulation of G. soja accessions CH3 and CH4 were carried out between HH103 RifR and its T3SS-mutant derivatives SVQ533 and SVQ288. On both accessions, SVQ533 and SVQ288 were clearly outcompeted by HH103 RifR with mutants only occupying 10–15% of the nodules (Table 6).
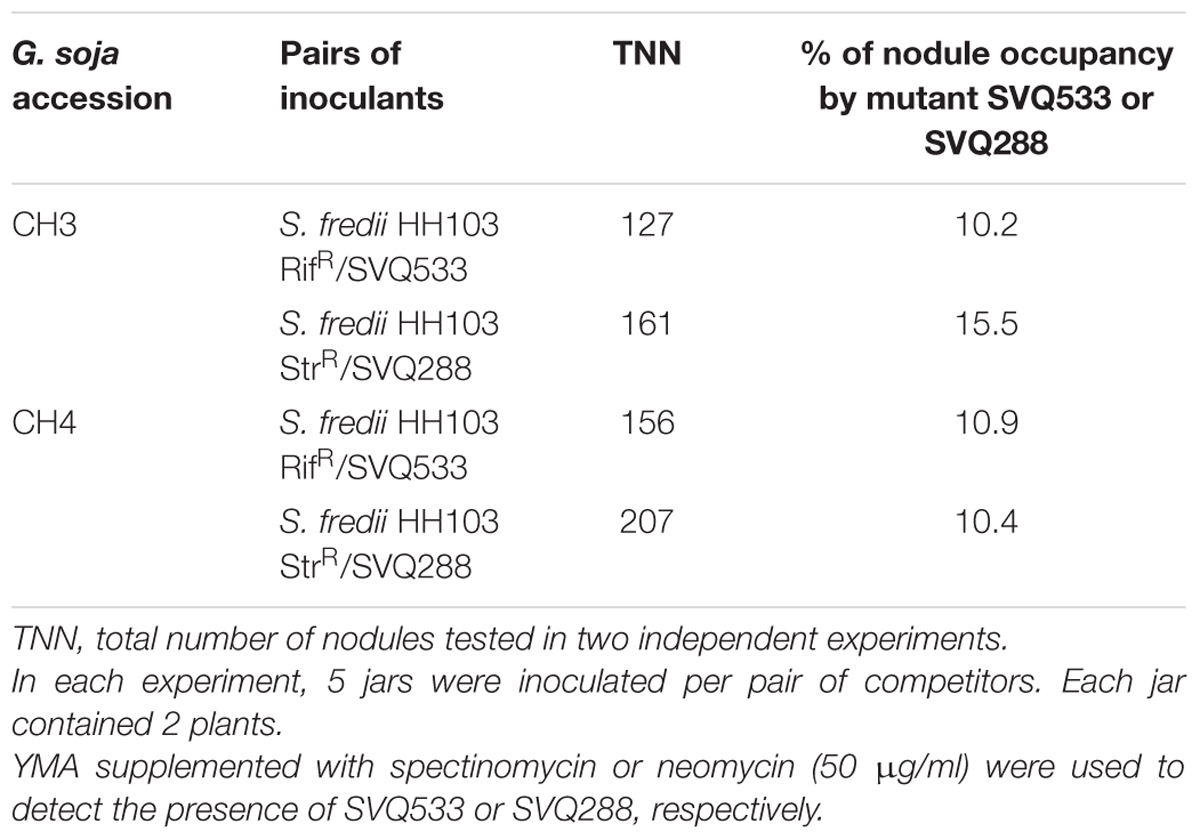
TABLE 6. Nodule occupancy of Glycine soja accessions CH3 and CH4 inoculated with S. fredii HH103 and its ttsI and rhcJ mutants.
Host-Range of S. fredii-Symbiotic Plasmid Hybrids on G. max, G. soja and Other Legumes Normally Nodulated by NGR234
USDA193C and NGR234C transconjugants were tested in nodulation assays with G. max cv. Williams 82 and the G. soja accession CH4 (Table 7 and Figures 4, 5). Whereas strains NGR234, NGR234-M, and NGR234C (=ANU265) failed to nodulate G. max cv. Williams (Table 7), the NGR234C transconjugants carrying plasmid pSfHH103d::Tn5-Mob (hereafter pSymHH103M) induced the formation of white ineffective nodules that did not contribute to plant growth. Similarly, USDA193C carrying the symbiotic plasmid pSfNGR234a::Tn5-Mob (hereafter pSymNGR234M) only induced the formation of ineffective root outgrowths on G. max cv. Williams 82 (Figure 5D). By contrast, G. soja CH4 inoculated with USDA193C carrying the symbiotic plasmid pSymNGR234M induced the formation of nitrogen-fixing nodules (Figures 4G,H) and plants did not show symptoms of nitrogen starvation. On the same CH4 host, plants inoculated with the NGR234C pSymHH103M hybrid formed inefficient nodules, however (Table 7).
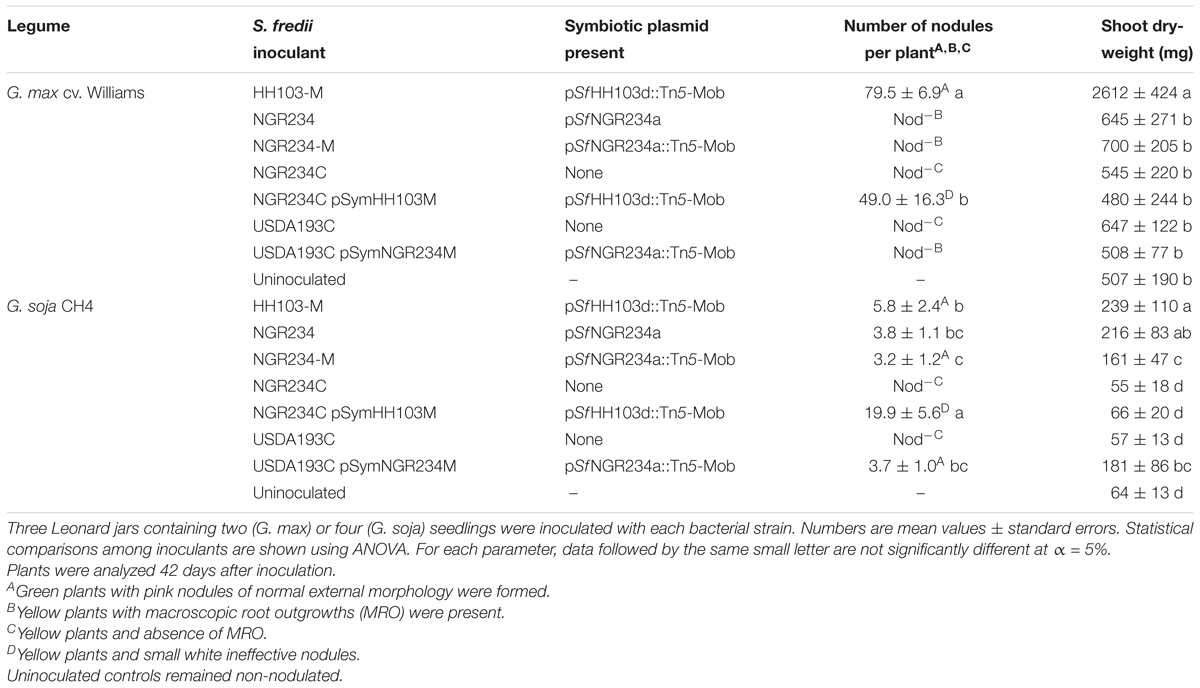
TABLE 7. Glycine max cv. Williams 82 and G. soja CH4 responses to inoculation with S. fredii pSym-cured derivatives carrying different S. fredii pSym plasmids.
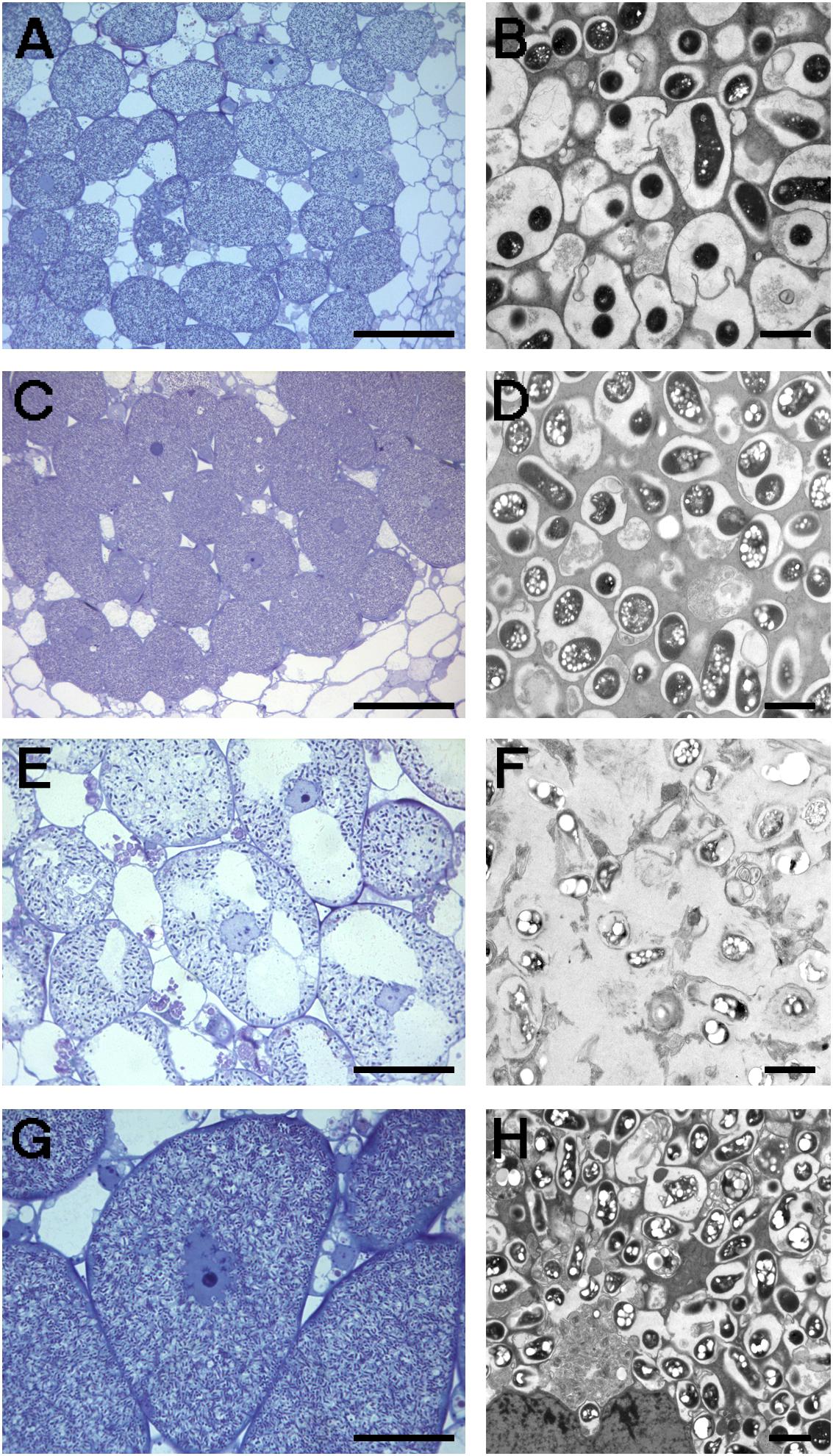
FIGURE 4. Optical (A,C,E,G) and electronic (B,D,F,H) microscopy of nodules induced by different S. fredii strains in G. soja CH4. (A,B) HH103 RifR; (C,D) NGR234; (E,F) NGR234C pSymHH103M; (G,H) USDA193C pSymNGR234M. Bars correspond to 100 μm in (A,C), 30 μm in (E,G), and 2 μm in (B,D,F,H).
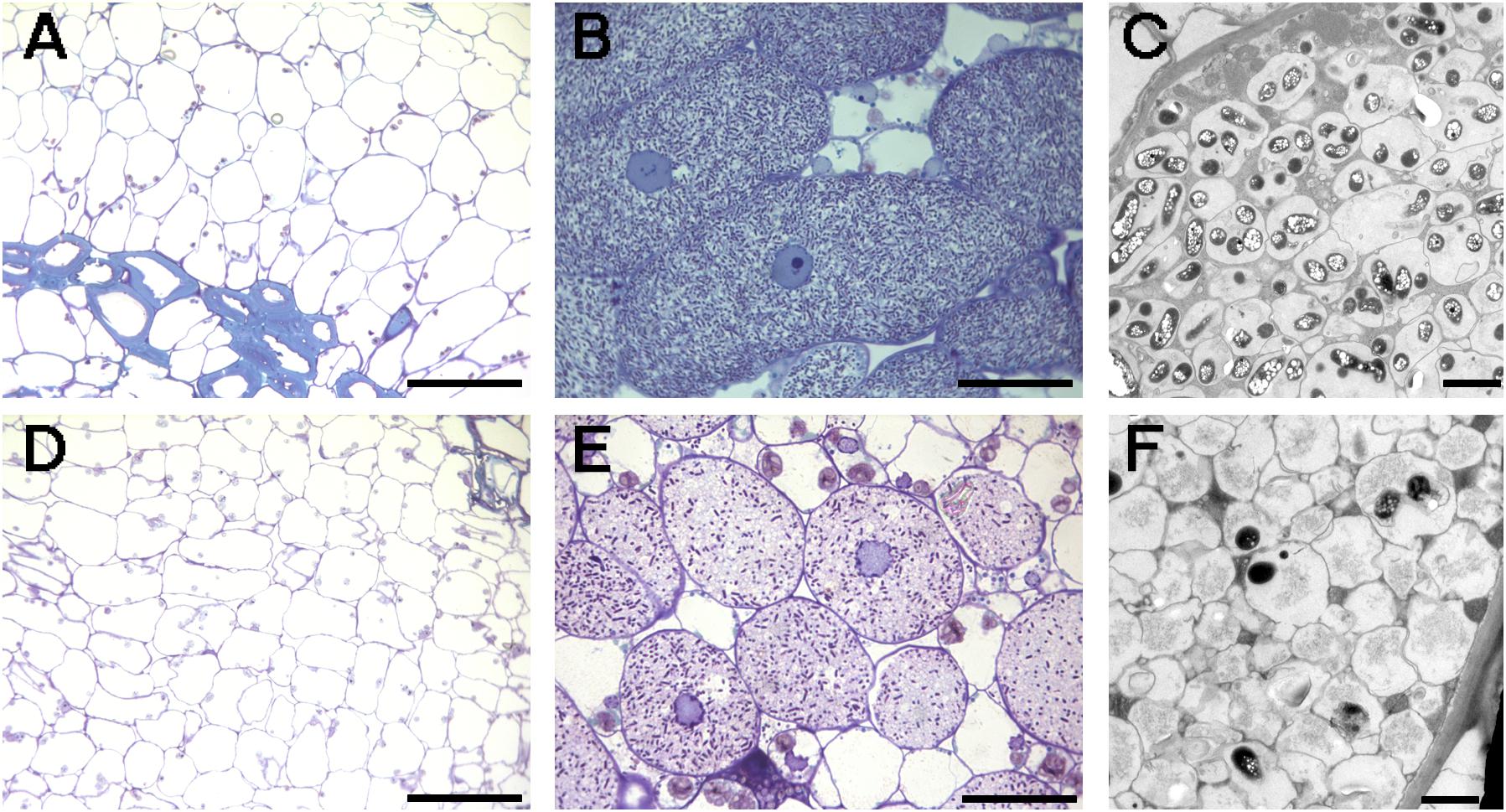
FIGURE 5. Optical (A,B,D,E) and electronic (C,F) microscopy of nodules induced by different S. fredii strains in soybean cv. Williams 82. (A) NGR234; (B,C) HH103 RifR; (D) USDA193C pSymNGR234M; (E,F) NGR234C pSymHH103M. Bars correspond to 100 μm in (A,D), 30 μm in (B,E), and 2 μm in (C,F).
Nodules formed by S. fredii inoculants were sectioned, stained with methylene blue, and observed by light microscopy. As expected, G. soja CH4 nodules induced by NGR234 contained a high density of symbiosomes (Figures 4C,D), similarly to those induced by HH103 RifR (Figures 4A,D). By contrast, macroscopic root outgrowths (MRO) formed in G. max cv. Williams 82 inoculated with NGR234 were devoid of bacteria inside the cells and in the intercellular spaces. Clusters of plant cells showing thick walls were observed (Figure 5A). Ineffective nodules formed by G. soja CH4 and G. max cv. Williams 82 inoculated with NGR234C carrying pSymHH103M contained many but poorly infected cells as in senescent nodules (Figures 4E, 5E). Electron micrographs confirmed that G. soja CH4 and G. max cv. Williams 82 nodules occupied by NGR234C carrying pSymHH103M were senescent, with nodule cells containing degraded bacteroids at 42 dpi (Figures 4F, 5F). At this same time point, G. soja nodules infected with NGR234 (Figures 4C,D) and G. max nodules occupied by HH103 RifR (Figures 5B,C) did not show visible symptoms of senescence.
Further nodulation assays were carried out to investigate the capacity of the symbiotic plasmid donor S. fredii HH103-M and the resulting NGR234C pSymHH103M hybrid strain to nodulate with three different legumes that form indeterminate (L. leucocephala and T. vogelii) or determinate (V. radiata) nodules with NGR234. V. radiata cv. King and T. vogelii plants inoculated with HH103-M formed nodules (Figures 6B,E), although both symbioses were less effective than those established with NGR234 (Table 8 and Figures 6A,D). L. leucocephala roots inoculated with HH103-M or NGR234C carrying pSymHH103M formed macroscopic root outgrowths (MRO) that did not contribute to plant growth (Table 8 and Figure 6K). Although V. radiata plants inoculated with NGR234 or HH103-M formed nitrogen-fixing nodules, the hybrid strain NGR234C carrying pSymHH103M only formed ineffective nodules (Figure 6C) and the color and development of plants were similar to those of the uninoculated control (Figure 6A). Despite the number of nodules formed by T. vogelii roots inoculated with NGR234 or HH103-M being similar (Table 8 and Figures 6E,F), the former was much more effective in supporting plant growth (Figure 6D). In contrast to the fully proficient indeterminate nodules induced by NGR234 on T. vogelii, (Figure 6I), those formed upon inoculation with HH103-M appeared mostly senescent with presence of leghemoglobin restricted to small sections of these nodules (Figure 6H). Hybrid strain NGR234C pSymHH103M induced the formation of ineffective nodules on T. vogelii roots (Table 8 and Figures 6G,J).
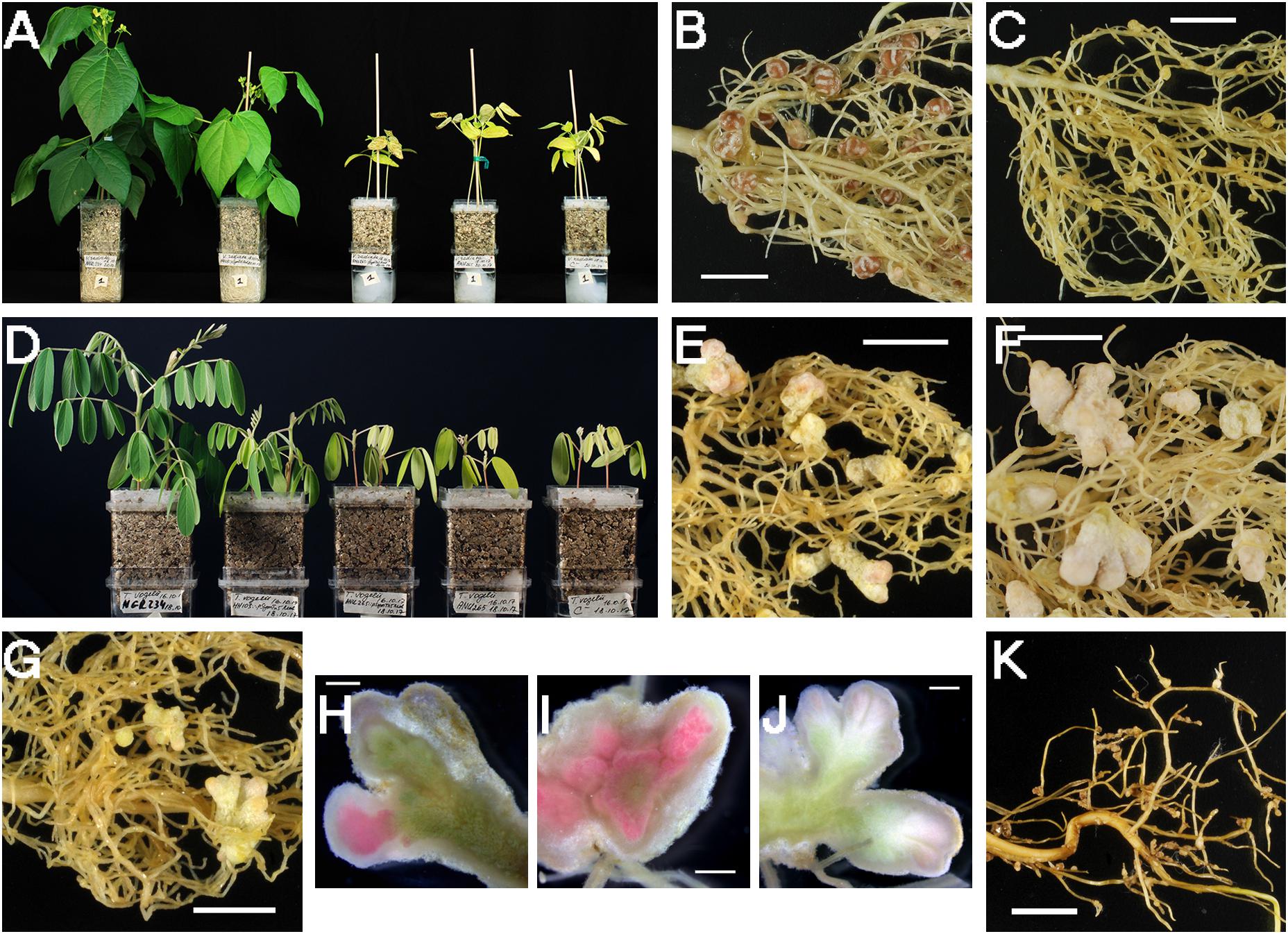
FIGURE 6. Plant responses of three host legumes of S. fredii NGR234, Vigna radiata (A–C), Tephrosia vogelii (D–J) and Leucaena leucocephala (K), with different S. fredii strains. (A) Phenotypes of strains on mung bean (V. radiata) at 42 dpi. From left to right, plants inoculated with NGR234, HH103-M, NGR234C pSymHH103M, NGR234C, and uninoculated control. (B) Nodules induced by HH103-M in V. radiata roots (42 dpi). (C) Pseudonodules induced by NGR234C pSymHH103M in V. radiata roots (42 dpi). (D) Phenotypes of strains on T. vogelii at 58 dpi. From left to right, plants inoculated with NGR234, HH103-M, NGR234C pSymHH103M, NGR234C, and uninoculated controls. (E–J) Nodules induced by HH103-M (E,H), NGR234 (F,I), and NGR234C pSymHH103M (G,J) in T. vogelii roots (58 dpi). (K) Abnormal root outgrowths on L. leucocephala 81 dpi with NGR234C pSymHH103M. Bars correspond to 1 cm in (B,C,E–G,K), and 1 mm in (H–J).
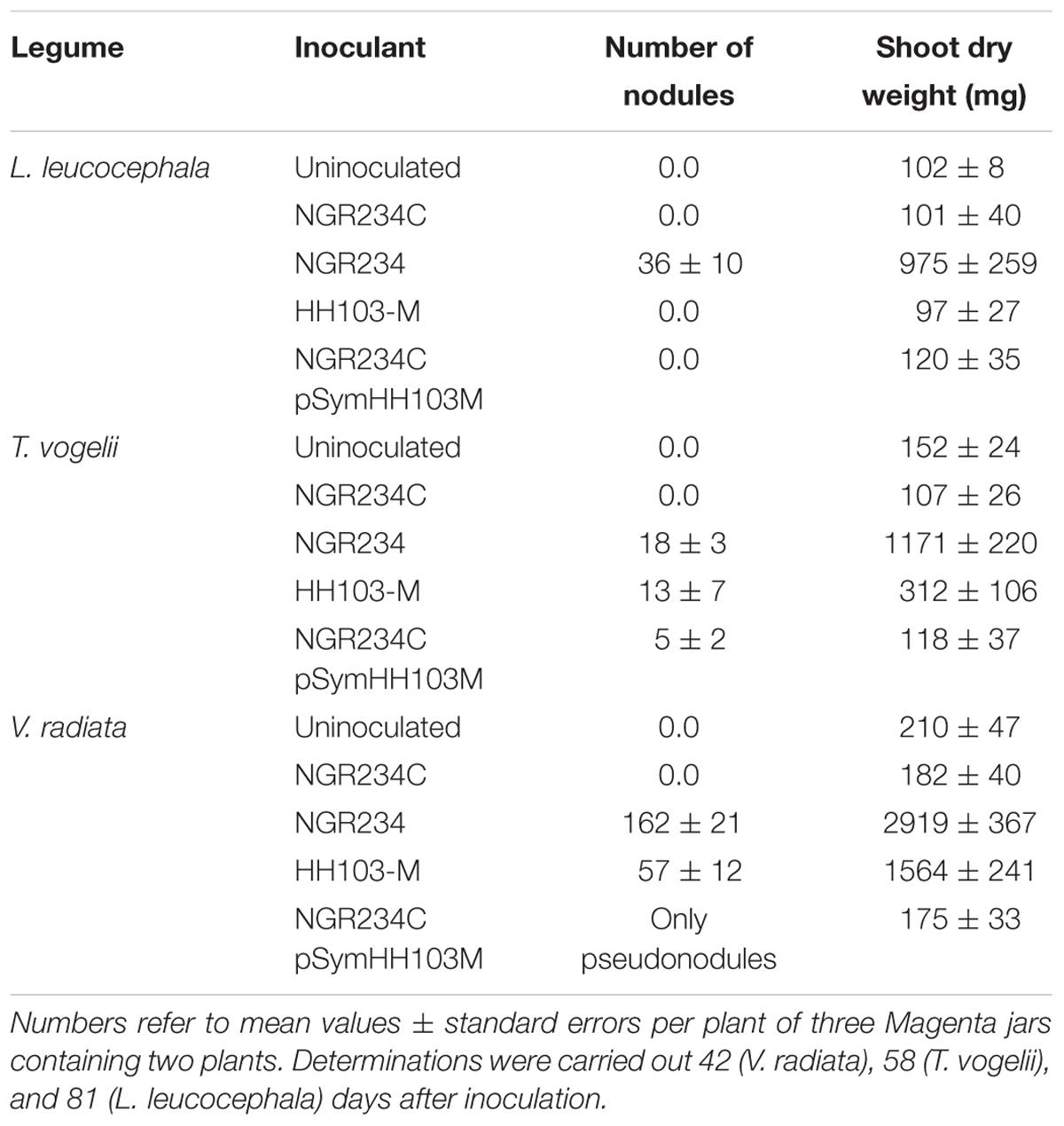
TABLE 8. Leucaena leucocephala, T. vogelii, and V. radiata responses to inoculation with a NGR234 pSym-cured derivative (ANU265) carrying the symbiotic plasmid of HH103 RifR tagged with Tn5-Mob.
Discussion
In the last two decades, different reports describing the geographical occurrence and distribution of slow- and fast-growing soybean rhizobia have been reported. A general conclusion from these studies is that soil pH appears to determine the prevalence of soybean bradyrhizobia (slow growers) or soybean sinorhizobia (fast growers). The most detailed report (Tian et al., 2012) confirmed that soybean bradyrhizobia populations are more abundant in acidic soils (Northern and Southern China), while soybean sinorhizobia are predominant in alkaline soils of Central China (Hebei, Shanxi, Henan, and Shandong provinces). The central province of Shaanxi was not included in this study. Recent work from India also suggests that Bradyrhizobium strains are very abundant as nodulators of a number of native legumes in acidic soils, whereas Sinorhizobium are more prevalent in neutral-alkaline soils (Ojha et al., 2017; Sankhla et al., 2017; Rathi et al., 2018).
In this work we have investigated the symbiotic capacity of wild soybeans from Northern and Central China to associate with B. diazoefficiens USDA110T, B. elkanii USDA76T, and S. fredii HH103 RifR, three well-known soybean-rhizobia strains whose genome have been fully sequenced and deposited in public databases. These soybean-rhizobia were also tested with wild soybeans from Russia, South Korea, and Japan, which are the only geographical areas outside China in which G. soja was so far reported.
Symbiotic nitrogen fixation of wild soybean accessions from Russia, South Korea, Japan, and Northern China inoculated with HH103 RifR was not effective enough to support appropriate plant growth in the absence of nitrogen fertilizers (Table 2 and Supplementary Tables S5, S8). These results indicate that wild soybeans from geographical areas in which fast-growing soybean-rhizobia populations are absent, or at very low levels, appear to have a low symbiotic affinity for HH103 RifR. Moreover, two accessions from the Jilin province, CH2 and CH12, even failed to nodulate with this strain, indicating that in these two accessions, symbiotic incompatibility was triggered by yet unknown molecular determinants. All the G. soja accessions from Russia, South Korea, Japan, and Northern China formed nitrogen-fixing nodules with USDA110T and USDA76T, the former generally being more effective than the latter (Table 2 and Supplementary Tables S3, S4, S6, S7). Interestingly, B. japonicum and B. diazoefficiens appear to be more abundant than B. elkanii in the Northern provinces of China (Figure 1; Tian et al., 2012). Nodules formed by HH103 RifR in six wild soybeans from the Shanxi province (Central China, alkaline soils) fixed enough nitrogen to support plant growth (Supplementary Tables S5, S8). All these results suggest that, at least in Northern and Central China, there is a correlation between symbiotic fitness of wild soybeans from a particular geographical area and the relative abundance of the different soybean rhizobia genera and species dwelling in soils of that specific zone. Concomitantly, G. soja accessions from Northern China are generally more effective with USDA110T than with USDA76T.
In Central China S. fredii indigenous populations are more abundant than bradyrhizobia populations (Tian et al., 2012). Some G. soja accessions from Shanxi (CH4, CH24, and CH25) were found to be more effective with HH103 RifR than with USDA110T, whereas the CH21 and CH23 accessions favored USDA110T. Thus, the possible correlation between the relative abundance of specific soybean-rhizobia and symbiotic fitness with local G. soja accessions from Central China is not as clear as in the case of plant-rhizobia combinations from the Northern provinces. To our knowledge, there are no reports describing the soybean indigenous populations of soils from Shaanxi province. Some accessions (e.g., CH17 and CH20) were more effective with HH103 RifR but others showed higher REI values with USDA110T and USDA76T (such as CH16 and CH18). Together these results indicate that Shanxi and Shaanxi provinces are promising places for finding wild soybeans showing high nitrogen-fixation capacities with S. fredii strains. Central China also appears to be promising for finding wild soybeans showing good symbiotic capacities with slow- and fast-growing soybean rhizobia, which is in contrast with G. soja accessions from Northern China, that appear to be only effective with bradyrhizobia.
Competition studies showed that USDA110T was more competitive than USDA76T to nodulate accessions CH2 and CH3 (Northern China) but less competitive with accession CH4 (Central China). These results fit well with the fact that soils from Northern China contain B. japonicum and B. diazoefficiens strains but are apparently devoid of B. elkanii populations, whereas soils of Central China are rather populated by B. elkanii instead of B. japonicum or B. diazoefficiens strains (Tian et al., 2012).
Sinorhizobium fredii NGR234 shows the broadest nodulation host-range ever described (Pueppke and Broughton, 1999). Strikingly, and unlike most S. fredii strains known, NGR234 is unable to nodulate soybean (G. max). The fact that some wild soybeans from Central China formed effective symbioses with HH103 RifR prompted us to investigate whether NGR234 could also form nitrogen-fixing nodules with any of these G. soja accessions. In fact, NGR234 formed nitrogen-fixing nodules with at least 6 different wild soybean accessions form Shaanxi or Shanxi provinces. Plant tests carried out in Spain, Switzerland, and China proved that NGR234 consistently nodulated several G. soja accessions, regardless of experimental settings and plant growth conditions used. To our knowledge, this is the first report describing the formation of nitrogen-fixing nodules in G. soja plants inoculated with S. fredii NGR234. Such a robust symbiotic phenotype on G. soja make attractive further searches for finding amongst the G. max varieties grown by Chinese farmers in Central China those capable of forming nitrogen-fixing nodules with NGR234.
It is well known that formation of symbiotic root nodules in soybean is controlled by several host genes referred to as Rj (rj) genes and it has been repeatedly reported that S. fredii T3SS plays important roles in determining symbiotic compatibility with G. max cultivars and other legume species (Hayashi et al., 2012; Staehelin and Krishnan, 2015; López-Baena et al., 2016; Yasuda et al., 2016). Here, we also tested whether the T3SS of HH103 RifR and NGR234 strains influenced nodulation of wild soybeans. Results confirmed that mutations blocking Nop-secretion significantly impacted symbiosis with a number of G. soja accessions (Table 4). For example, CH2 accession from Northern China formed nodules with NGR234 but not with HH103 RifR. Conversely, while mutants of NGR234 with deficient T3SS (strains NGRΩttsI and NGRΩrhcN) lost their capacity to nodulate CH2 the HH103 mutants in ttsI and rhcJ gained nodulation on this accession. These results indicate that one or more of the five Nop secreted by NGR234 promote(s) CH2 nodulation, while the combination of Nop secreted by HH103 RifR prevents CH2 nodulation. Although the sequences of the Nop shared by HH103 RifR and NGR234 are nearly identical, there are some differences in the repertoire of T3SS effectors secreted by both strains (López-Baena et al., 2016). For example, HH103 RifR carries nopI and nopD genes, which are absent from the NGR234 genome. By contrast, NGR234 genome includes a copy of the nopJ gene that is absent from HH103 RifR. These three genes are therefore good candidates for determining symbiotic specificity on CH2. On the CH3 (from Northern China) and CH4 (Central China) accessions, HH103 RifR and the ttsI (SVQ533) or rhcJ (SVQ288) derivative mutants retained nodulation. Shoot dry-weight of CH3 plants inoculated with SVQ533 was significantly lower than for plants inoculated with the parent strain (Table 4). Mutations in ttsI and rhcN of NGR234 totally abolished nodulation on both CH3 and CH2 accessions, however. These phenotypes suggest that NopJ (the only T3SS-effector not secreted by HH103 RifR) is a prime candidate for promoting nodulation of CH2 and CH3 by NGR234. Since CH4 plants responded in the same manner to inoculation with NGR234 or NGRΩrhcN, T3SS and its panel of effectors don’t play a significant role during nodulation of this accession. That a significant reduction in all phenotypic parameters monitored occurred when CH4 plants were inoculated with NGRΩttsI, indicate that a TtsI-regulated function other than T3SS plays an important role during the NGR234-CH4 interaction, however. Together these results confirm that T3SS play important roles (either positively or negatively) during symbioses between S. fredii strains and wild soybean accessions. Also, the different effect that the studied T3SS mutations have in the symbiotic behavior with the different G. soja accessions tested suggests that these G. soja accessions might have different Rj (rj) genotypes, although further research is necessary for clarifying this issue.
There is a theoretically alternative way to carry out screenings aimed to isolate new S. fredii strains showing altered symbiotic capacities with soybean and other legumes: the construction of S. fredii hybrid strains (chimeras) by intraspecific transfer of symbiotic plasmids. The simplest way for constructing such chimeras is by transferring S. fredii symbiotic plasmids to pSym-cured derivatives of other S. fredii strains. If the symbiotic properties of some of these combinations (S. fredii genome background/S. fredii pSym) appear to be interesting, the analysis of the hybrids will be easier if the genome sequences of the donor and recipient strains are already known. This would be the situation for hybrids constructed between S. fredii strains NGR234 and HH103 RifR. Following this approach, we transferred the symbiotic plasmid of HH103 RifR to strain NGR234C, a pSym-cured derivative of NGR234. The symbiotic plasmid of NGR234 was marked with transposon Tn5-Mob and transferred to S. fredii USDA193C, a pSym-cured derivative of S. fredii USDA193 (unfortunately, a pSym-cured derivative of HH103 RifR is not available). USDA193 forms nitrogen-fixing nodules with Asiatic soybean cultivars but root-swellings and/or ineffective nodules with American soybeans (Keyser et al., 1982).
NGR234 does not effectively nodulate the American soybean cultivar Williams 82. Although Trinick (1980) reported that NGR234 induce Fix+ nodules on G. max, the cultivar employed in that study was not specified. Balatti et al. (1995) showed that NGR234 only induces the formation of small, irregular swellings on the roots of 89 different varieties of soybean, including McCall (restriction genotype Rfg1) and Dunfield (restriction genotype Rj4). Pueppke and Broughton (1999) also found that NGR234 was ineffective with one Asiatic (Peking) and two American (McCall and Preston) varieties of soybean. So, it can be concluded that NGR234 is ineffective with the vast majority of soybean cultivars tested so far.
The Rj genotype of soybean Williams 82 is Rfg1, which restricts nodulation by S. fredii USDA257 (reviewed by Hayashi et al., 2012). This Rj genotype is shared by other American varieties such as McCall. This restriction is most probably due to the type three associated effectors secreted by USDA257, since mutants of this strain in this secretion system gain the ability to nodulate American soybeans (reviewed by Staehelin and Krishnan, 2015; López-Baena et al., 2016). Most probably, this is also the reason why other S. fredii strains such as USDA192 or USDA193 also fail in nodulating American soybeans. However, the story appears to be more complex since strain HH103, which also possesses a functional symbiotic type three secretion system (T3SS), naturally nodulates American soybeans (including Williams 82) and mutants negatively affected in its T3SS undergo a partial impairment in their interactions with these soybeans (de Lyra et al., 2006).
The chimeric strain NGR234C pSymHH103M formed nodules that did not fix nitrogen with soybean Williams 82, so that the shoot dry-weight of plants inoculated with the hybrid strain was equal to that of the uninoculated control (Table 7). Thus, the presence of the pSymHH103M, which is the symbiotic plasmid of a strain (HH103-M) that effectively nodulates Williams 82, is not enough to enable NGR234C to form nitrogen-fixing nodules with this G. max cultivar. Three possible explanations can be formulated: (i) some genes essential for effective soybean nodulation are neither present in NGR234C nor provided by pSymHH103M; (ii) some of the NGR234C genes block nodule development; and (iii) the combination of symbiotic signals produced by the resulting chimeric strain is not suitable for effective nodulation of G. max cultivar Williams 82. Although the NGR234C pSymHH103M transconjugant nodulated G. soja CH4, nodules that were formed did not contribute to plant growth (Table 7). Microscopy studies showed that peribacteroid membranes were disrupted or missing, as is often the case when nodules senesce (Figure 4F). This result, that was unexpected as both NGR234 and HH103 RifR strains were capable to form proficient nodules on this accession, suggest the combination of symbiotic signals produced by the hybrid strain might not be suitable for making nitrogen-fixing nodules on CH4 accession. For instance, the hybrid must produce the HH103 RifR complement of nodulation factors and secreted Nop since genes involved in these symbiotic functions are carried by the symbiotic plasmid. The hybrid, however, should produce the KPS synthesized by NGR234 because rkp genes are carried by pNGR234b (Schmeisser et al., 2009). Possibly, LPS of the NGR234C pSymHH103M hybrid should differ from those of NGR234 and HH103 RifR as most genes involved in LPS biosynthesis are chromosome born. However, some genes required for the biosynthesis of the flavonoid-inducible rhamnan modification of the NGR234 LPS (Marie et al., 2004) are encoded by pSfNGR234a and thus neither present in NGR234 nor provided by plasmid pSymHH103M (Vinardell et al., 2015).
The S. fredii strains NGR234 and HH103-M nodulated T. vogelii, the former being more effective (Table 8). T. vogelii plants inoculated with the hybrid NGR234C pSymHH103M showed reduced nodulation in comparison to the parental strains and the nodules formed did not support plant growth (Table 8). These results suggest again that the combination of symbiotic signals produced by the hybrid is less appropriate than that produced by each parental strains. The failure of hybrid NGR234C pSymHH103M to nodulate L. leucocephala (Table 8) could be due to the absence of a functional nodS gene, which is required for nodulation of this legume (Lewin et al., 1990). The nodS gene is located in the symbiotic plasmid of NGR234 (missing in NGR234C) and the nodS present in pSymHH103 is not functional (Vinardell et al., 2015).
The hybrid USDA193C carrying the symbiotic plasmid of NGR234 (USDA193C pSymNGR234M) failed to nodulate G. max cv. Williams 82 but formed nitrogen-fixing nodules with G. soja CH4. Thus, the symbiotic properties of the two hybrids studied (NGR234C pSymHH103M and USDA193C pSymNGR234M) are different. We could expect that new combinations would produce “new” S. fredii strains in which the symbiotic interaction with a particular legume could be positive or negatively affected or without any noticeable change. The genetic information already available about S. fredii HH103 RifR and NGR234 strains, together with the structural information of their Nodulation factors, surface polysaccharides and nodulation outer proteins, might allow the prediction of the symbiotic signals produced by the hybrid strains. Taking the advantage that S. fredii strains nodulate many different legumes, the study of S. fredii hybrids could provide valuable information about the relative importance of particular symbiotic signals, or combination of signals, for the bacterial interaction with a wide range of legumes.
Author Contributions
FT-V, XP, JR-S, and JV conceived and designed the experiments. All authors performed the experiments. FT-V, SA-J, XP, JR-S, FL-B, and JV analyzed the data. FT-V, XP, JR-S, and JV contributed with reagents, materials, and analysis tools. FT-V, XP, FL-B, JR-S, and JV wrote the paper. All authors read and approved the final manuscript.
Funding
This work was funded by grants P11-CVI-7500 from the Andalusian Government and BIO2016-78409-R from the Ministry of Economy and Competitiveness (Spanish Government). XP acknowledges the generous financial supports of the University of Geneva and the Swiss National Science Foundation (Grants 31003A-146548 and 31003A-173191).
Conflict of Interest Statement
The authors declare that the research was conducted in the absence of any commercial or financial relationships that could be construed as a potential conflict of interest.
Acknowledgments
XP would like to thank Natalia Giot for her help in many aspects of this work. SA-J is the recipient of a Ph.D. grant from the VPPI of the University of Seville. They also thank the Centro de Investigación, Tecnología e Innovación (CITIUS) of the University of Seville for light and electronic microscopy facilities.
Supplementary Material
The Supplementary Material for this article can be found online at: https://www.frontiersin.org/articles/10.3389/fmicb.2018.02843/full#supplementary-material
References
Alves, B. J. R., Boddey, R. M., and Urquiaga, S. (2003). The success of BNF in soybean in Brazil. Plant Soil 252, 1–9. doi: 10.1023/a:1024191913296
Balatti, P. A., Kovacs, L. G., Krishnan, H. B., and Pueppke, S. G. (1995). Rhizobium sp. NGR234 contains a functional copy of the soybean cultivar specificity locus, nolXWBTUV. Mol. Plant Microbe Interact. 8, 693–699. doi: 10.1094/MPMI-8-0693
Beringer, J. E. (1974). R factor transfer in Rhizobium leguminosarum. J. Gen. Microbiol. 84, 188–198. doi: 10.1099/00221287-84-1-188
Brockwell, J., Hely, F. W., and Neal-Smith, C. A. (1966). Some symbiotic characteristics of rhizobia responsible for spontaneous, effective field nodulation of Lotus hispidus. Austr. J. Exp. Agric. Anim. Husband. 6, 365–370. doi: 10.1071/EA9660365
Broughton, W. J., and Dilworth, M. J. (1971). Control of leghaemoglobin synthesis in snake beans. Biochem. J. 125, 1075–1080. doi: 10.1042/bj1251075
Broughton, W. J., Jabbouri, S., and Perret, X. (2000). Keys to symbiotic harmony. J. Bacteriol. 182, 5641–5652. doi: 10.1128/JB.182.20.5641-5652.2000
Broughton, W. J., Wong, C.-H., Lewin, A., Samrey, U., Myint, H., Meyer, H. z. A., et al. (1986). Identification of Rhizobium plasmid sequences involved in recognition of Psophocarpus, Vigna, and other legumes. J. Cell Biol. 102, 1173–1182. doi: 10.1083/jcb.102.4.1173
Buendía-Clavería, A. M., Moussaid, A., Ollero, F. J., Vinardell, J. M., Torres, A., Moreno, J., et al. (2003). A purL mutant of Sinorhizobium fredii HH103 is symbiotically defective and altered in its lipopolysaccharide. Microbiology 149, 1807–1818. doi: 10.1099/mic.0.26099-0
Buendía-Clavería, A. M., Romero, F., Cubo, T., Perez-Silva, J., and Ruiz-Sainz, J. E. (1989). Inter and intraspecific transfer of a Rhizobium fredii symbiotic plasmid: expression and incompatibility of symbiotic plasmids. Syst. Appl. Microbiol. 12, 210–215. doi: 10.1016/S0723-2020(89)80016-5
Camacho, M., Santamaría, C., Temprano, F., Rodriguez-Navarro, D. N., Daza, A., Espuny, R., et al. (2002). Soils of the Chinese Hubei province show a very high diversity of S. fredii strains. Syst. Appl. Microbiol. 25, 592–602. doi: 10.1078/07232020260517733
Chen, Y., Chen, P., and de los Reyes, B. G. (2006). Differential responses of the cultivated and wild species of soybean to dehydration stress. Crop Sci. 46, 2041–2046. doi: 10.2135/cropsci2005.12.0466
de Lyra, M. C. C. P., Lopez-Baena, F. J., Madinabeitia, N., Vinardell, J. M., Espuny, M. R., Cubo, M. T., et al. (2006). Inactivation of the Sinorhizobium fredii HH103 rhcJ gene abolishes nodulation outer proteins (Nops) secretion and decreases the symbiotic capacity with soybean. Int. Microbiol. 9, 125–133.
Fossou, R. K., Ziegler, D., Zézé, A., Barja, F., and Perret, X. (2016). Two major clades of bradyrhizobia dominate symbiotic interactions with pigeonpea in fields of Côte d’Ivoire. Front. Microbiol. 7:1793. doi: 10.3389/fmicb.2016.01793
Fumeaux, C., Bakkou, N., Kopcińska, J., Golinowski, W., Westenberg, D. J., Müller, P., et al. (2011). Functional analysis of the nifQdctA1y4vGHIJ operon of Sinorhizobium fredii strain NGR234 using a transposon with a NifA-dependent read-out promoter. Microbiology 157, 2745–2758. doi: 10.1099/mic.0.049999-0
Hamilton-Reeves, J. M., Rebello, S. A., Thomas, W., Slaton, J. W., and Kurzer, M. S. (2007). Soy protein isolate increases urinary estrogens and the ratio of 2:16 α-hydroxyestrone in men at high risk of prostate cancer. J. Nutr. 137, 2258–2263. doi: 10.1093/jn/137.10.2258
Hartman, G. L., West, E. D., and Herman, T. K. (2011). Crops that feed the World 2. Soybean-worldwide production, use, and constraints caused by pathogens and pests. Food Sec. 3, 5–17. doi: 10.1007/s12571-010-0108-x
Hayashi, M., Saeki, Y., Haga, M., Harada, K., Kouchi, H., and Umehara, Y. (2012). Rj (rj) genes involved in nitrogen-fixing root nodule formation in soybean. Breed. Sci. 61, 544–553. doi: 10.1270/jsbbs.61.544
Kambara, K., Ardissone, S., Kobayashi, H., Saad, M. M., Schumpp, O., Broughton, W. J., et al. (2009). Rhizobia utilize pathogen-like effector proteins during symbiosis. Mol. Microbiol. 71, 92–106. doi: 10.1111/j.1365-2958.2008.06507.x
Kanamaru, K., Wang, S. D., and Abe, J. (2006). Identification and characterization of wild soybean (Glycine soja sieb. et Zucc.) strains with high lutein content. Breed. Sci. 56, 3231–3234. doi: 10.1270/jsbbs.56.231
Keyser, H. H., Bohlool, B. B., Hu, T. S., and Weber, D. F. (1982). Fast-growing rhizobia isolated from root nodules of soybean. Science 215, 1631–1632. doi: 10.1126/science.215.4540.1631
Kofsky, J., Zhang, H., and Song, B. H. (2018). The untapped genetic reservoir: the past, current, and future applications of the wild soybean (Glycine soja). Front. Plant Sci. 9:949. doi: 10.3389/fpls.2018.00949
Lewin, A., Cervantes, E., Wong, C.-H., and Broughton, W. J. (1990). nodSU, two new nod genes of the broad host range Rhizobium strain NGR234 encode host-specific nodulation of the tropical tree Leucaena leucocephala. Mol. Plant Microbe Interact. 3, 317–326. doi: 10.1094/MPMI-3-317
Li, Y., Zhou, G., Ma, J., Jiang, W., Jin, L., Zhang, Z., et al. (2014). De novo assembly of soybean wild relatives for pan-genome analysis of diversity and agronomic traits. Nat. Biotechnol. 32, 1045–1052. doi: 10.1038/nbt.2979
Li, Y.-H., Li, W., Zhang, C. H., Yang, L., Chang, R.-Z., Gaut, B. S., et al. (2010). Genetic diversity in domesticated soybean (Glycine max) and its wild progenitor (Glycine soja) for simple sequence repeat and single-nucleotide polymorphism loci. New Phytol. 188, 242–253. doi: 10.1111/j.1469-8137.2010.03344.x
López-Baena, F. J., Ruiz-Sainz, J. E., Rodríguez-Carvajal, M. A., and Vinardell, J. M. (2016). Bacterial molecular signals in the Sinorhizobium fredii-soybean symbiosis. Int. J. Mol. Sci. 17:E755. doi: 10.3390/ijms17050755
Luo, Q., Yu, B., and Liu, Y. (2005). Differential, sensitivity to chloride and sodium ions in seedlings of Glycine max and G. soja under NaCl stress. J. Plant Physiol. 162, 1003–1012. doi: 10.1016/j.jplph.2004.11.008
Mammadov, J., Buyyarapu, R., Guttikonda, S. K., Parliament, K., Abdurakhmonov, I. Y., and Kumpatla, S. P. (2018). Wild relatives of maize, rice, cotton, and soybean: treasure troves for tolerance to biotic and abiotic stresses. Front. Plant Sci. 9:886. doi: 10.3389/fpls.2018.00886
Margaret, I., Becker, A., Blom, J., Bonilla, I., Goesmann, A., Göttfert, M., et al. (2011). Symbiotic properties and first analyses of the genomic sequence of the fast growing model strain Sinorhizobium fredii HH103 nodulating soybean. J. Biotechnol. 155, 11–19. doi: 10.1016/j.jbiotec.2011.03.016
Marie, C., Deakin, W. J., Ojanen-Reuhs, T., Diallo, E., Reuhs, B., Broughton, W. J., et al. (2004). TtsI, a key regulator of Rhizobium species NGR234 is required for Type III-dependent protein secretion and synthesis of rhamnose-rich polysaccharides. Mol. Plant Microbe Interact. 17, 958–966. doi: 10.1094/MPMI.2004.17.9.958
Morrison, N. A., Hau, C. Y., Trinick, M. J., Shine, J., and Rolfe, B. G. (1983). Heat curing of a Sym plasmid in a fast-growing Rhizobium sp. that is able to nodulate legumes and the nonlegume Parasponia sp. J. Bacteriol. 153, 527–531.
Natarajan, S., Xu, C. P., and Bae, H. (2007). Proteomic and genomic characterization of Kunitz trypsin inhibitors in wild and cultivated soybean genotypes. J. Plant Physiol. 164, 756–763. doi: 10.1016/j.jplph.2006.05.014
Ojha, A., Tak, N., Rathi, S., Chouhan, B., Rao, S. R., Barik, S. K., et al. (2017). Molecular characterization of novel Bradyrhizobium strains nodulating Eriosema chinense and Flemingia vestita, important unexplored native legumes of the sub-Himalayan region (Meghalaya) of India. Syst. Appl. Microbiol. 40, 334–344. doi: 10.1016/j.syapm.2017.06.003
Perret, X., Staehelin, C., and Broughton, W. J. (2000). Molecular basis of symbiotic promiscuity. Microbiol. Mol. Biol. Rev. 64, 180–201. doi: 10.1128/MMBR.64.1.180-201.2000
Pueppke, S. G., and Broughton, W. J. (1999). Rhizobium sp. strain NGR234 and R. fredii USDA257 share exceptionally broad, nested host ranges. Mol. Plant Microbe Interact. 12, 293–318. doi: 10.1094/MPMI.1999.12.4.293
Qiu, L. J., and Chang, R. Z. (2010). “The origin and history of soybean,” in The Soybean. Botany, Production and Uses, ed. G. Singh (Wallingford: CAB International), 4.
Rathi, S., Tak, N., Bissa, G., Chouhan, B., Ojha, A., Adhikari, D., et al. (2018). Selection of Bradyrhizobium or Ensifer symbionts by the native Indian caesalpinioid legume Chamaecrista pumila depends on soil pH and other edaphic and climatic factors. FEMS Microbiol. Ecol. 94:fiy180. doi: 10.1093/femsec/fiy180
Rigaud, J., and Puppo, A. (1975). Indole-3-acetic acid catabolism by soybean bacteroids. J. Gen. Microbiol. 88, 223–228. doi: 10.1099/00221287-88-2-223
Romero, F. (1993). Estudio Genetico de la Nodulacion de Rhizobium fredii. Ph.D. thesis, University of Seville, Seville.
Rosell, M. S., Appleby, P. N., Spencer, E. A., and Key, T. J. (2004). Soy intake and blood cholesterol considerations: a cross-sectional study of 1033 pre- and post-menopausal women in the Oxford arm of the European prospective investigation into cancer and nutrition. Am. J. Clin. Nutr. 80, 1391–1396. doi: 10.1093/ajcn/80.5.1391
Sambrook, J., and Russell, D. (2001). Molecular Cloning: A Laboratory Manual, 3rd Edn. Cold Spring Harbor, NY: Cold Spring Harbor Laboratory.
Sankhla, I. S., Tak, N., Meghwal, R. R., Choudhary, S., Tak, A., Rathi, S., et al. (2017). Molecular characterization of nitrogen fixing microsymbionts from root nodules of Vachellia (Acacia) jacquemontii, a native legume from the Thar Desert of India. Plant Soil 410, 21–40. doi: 10.1007/s11104-016-2838-9
Schmeisser, C., Liesegang, H., Krysciak, D., Bakkou, N., Le Quéré, A., Wollherr, A., et al. (2009). Rhizobium sp. NGR234 possesses a remarkable number of secretion systems. Appl. Environ. Microbiol. 75, 4035–4045. doi: 10.1128/AEM.00515-09
Simon, R. (1984). High frequency mobilization of gram-negative bacterial replicons by the in vivo constructed Tn5-Mob transposon. Mol. Gen. Genet. 196, 413–420. doi: 10.1007/BF00436188
Singh, R. J. (2007). Methods for Producing Fertile Crosses Between Wild and Domestic Soybean Species. US Patent. Application number 11/417,369. Washington, DC: U.S. Patent and Trademark Office.
Sprent, J. I., Ardley, J., and James, E. K. (2017). Biogeography of nodulated legumes and their nitrogen-fixing symbionts. New Phytol. 215, 40–56. doi: 10.1111/nph.14474
Staehelin, C., and Krishnan, H. B. (2015). Nodulation outer proteins: double-edged swords of symbiotic rhizobia. Biochem. J. 470, 263–274. doi: 10.1042/BJ20150518
Tian, C. F., Zhou, Y. J., Zhang, Y. M., Li, Q. Q., Zhang, Y. Z., and Li, D. F. (2012). Comparative genomics of rhizobia nodulating soybean suggest extensive recruitment of lineage specific genes in adaptations. Proc. Natl. Acad. Sci. U.S.A. 109, 8629–8634. doi: 10.1073/pnas.1120436109
Trinick, M. J. (1980). Relationships amongst the fast-growing Rhizobium of Lablab purpureus, Leucarena leucocephala, Mimosa sp., Acacia farnesiana, and Sesbania grandiflora and their affinities with other Rhizobium groups. J. Appl. Bacteriol. 49, 39–53. doi: 10.1111/j.1365-2672.1980.tb01042.x
Turner, G. L., and Gibson, A. H. (1980). “Measurement of nitrogen fixation by indirect means,” in Methods for Evaluating Biological Nitrogen Fixation, ed. F. J. Bergersen (New York, NY: Wiley), 111–138.
Vinardell, J. M., Acosta-Jurado, S., Zehner, S., Göttfert, M., Becker, A., Baena, I., et al. (2015). The Sinorhizobium fredii HH103 genome: a comparative analysis with S. fredii strains differing in their symbiotic behavior with soybean. Mol. Plant Microbe Interact. 28, 811–824. doi: 10.1094/MPMI-12-14-0397-FI
Vinardell, J. M., Buendía-Clavería, A. M., and Ruiz-Sainz, J. E. (1993). A method for the positive selection of spontaneous Rhizobium mutants showing transcriptional activation of nodulation genes in the absence of nod-inducers. Mol. Plant Microbe Interact. 6, 782–785. doi: 10.1094/MPMI-6-782
Vincent, J. M. (1970). A Manual for the Practical Study of Root-Nodule Bacteria. Oxford: Blackwell Scientific Publications.
Westcott, P., and Hansen, J. (2016). USDA Agricultural Projections to 2025. No. (OCE-2016-1). Office of the Chief Economist, World Agricultural Outlook Board, U.S. Department of Agriculture. Available at: https://www.ers.usda.gov/publications/pub-details/?pubid=37818
Xu, C. P., Capena, T. J., and Garret, W. M. (2007). Proteomic analysis of the distribution of the major seed allergens in wild, landrace, ancestral, and modern soybean genotypes. J. Sci. Food Agric. 87, 2511–2518. doi: 10.1002/jsfa.3017
Yang, S. H., Chen, W. H., Wang, E. T., Chen, W. F., Yan, J., Han, X. Z., et al. (2018). Rhizobial biogeography and inoculation application to soybean in four regions across China. J. Appl. Microbiol. 125, 853–866. doi: 10.1111/jam.13897
Yang, S. S., Bellogin, R. A., Buendía, A., Camacho, M., Chen, M., Cubo, T., et al. (2001). Effect of pH and soybean cultivars on the quantitative analyses of soybean rhizobia populations. J. Biotechnol. 91, 243–255. doi: 10.1016/S0168-1656(01)00340-6
Yasuda, M., Miwa, H., Masuda, S., Takebayashi, Y., Sakakibara, H., and Okazaki, S. (2016). Effector-triggered immunity determines host genotype-specific incompatibility in Legume–Rhizobium symbiosis. Plant Cell Physiol. 57, 1791–1800. doi: 10.1093/pcp/pcw104
Zhang, B., Du, N., Li, Y., Shi, P., and Wei, G. (2018). Distinct biogeographic patterns of rhizobia and non-rhizobial endophytes associated with soybean nodules across China. Sci. Total Environ. 643, 569–578. doi: 10.1016/j.scitotenv.2018.06.240
Zhao, L., Fan, M., Zhang, D., Yang, R., Zhang, F., Xu, L., et al. (2014). Distribution and diversity of rhizobia associated with wild soybean (Glycine soja Sieb. & Zucc.) in Northwest China. Syst. Appl. Microbiol. 37, 449–456. doi: 10.1016/j.syapm.2014.05.011
Keywords: Sinorhizobium, Bradyrhizobium, Glycine max, Glycine soja, rhizobia-legume symbiosis
Citation: Temprano-Vera F, Rodríguez-Navarro DN, Acosta-Jurado S, Perret X, Fossou RK, Navarro-Gómez P, Zhen T, Yu D, An Q, Buendía-Clavería AM, Moreno J, López-Baena FJ, Ruiz-Sainz JE and Vinardell JM (2018) Sinorhizobium fredii Strains HH103 and NGR234 Form Nitrogen Fixing Nodules With Diverse Wild Soybeans (Glycine soja) From Central China but Are Ineffective on Northern China Accessions. Front. Microbiol. 9:2843. doi: 10.3389/fmicb.2018.02843
Received: 09 August 2018; Accepted: 05 November 2018;
Published: 21 November 2018.
Edited by:
Suhelen Egan, University of New South Wales, AustraliaReviewed by:
Euan James, James Hutton Institute, United KingdomJulie Ardley, Murdoch University, Australia
Copyright © 2018 Temprano-Vera, Rodríguez-Navarro, Acosta-Jurado, Perret, Fossou, Navarro-Gómez, Zhen, Yu, An, Buendía-Clavería, Moreno, López-Baena, Ruiz-Sainz and Vinardell. This is an open-access article distributed under the terms of the Creative Commons Attribution License (CC BY). The use, distribution or reproduction in other forums is permitted, provided the original author(s) and the copyright owner(s) are credited and that the original publication in this journal is cited, in accordance with accepted academic practice. No use, distribution or reproduction is permitted which does not comply with these terms.
*Correspondence: Jose Maria Vinardell, anZpbmFyQHVzLmVz