- 1School of Earth Sciences and Environmental Engineering, Gwangju Institute of Science and Technology, Gwangju, South Korea
- 2Pohang Accelerator Laboratory, Pohang University of Science and Technology, Pohang, South Korea
- 3Department of Bioenvironmental Chemistry, Chonbuk National University, Jeonju, South Korea
Nanomaterials exhibit extraordinary properties based on their size, shape, chemical composition, and crystal structure. Owing to their unique properties nanomaterials are preferred over their bulk counterparts for a number of applications. Although conventional physical and chemical routes were established for the massive production of nanomaterials, there are some drawbacks such as environmental burden and high cost that cannot be disregarded. Recently, there has been great interest toward the green synthesis of inorganic nanomaterials. It has been reported that dissimilatory metal reduction by microorganisms is a cost-effective process to remediate toxic organic and inorganic compounds under anaerobic conditions. Particularly, members of the Shewanella genus have been utilized to produce various biogenic nanomaterials with unique micro/nanostructured morphologies through redox transformations as well as to remove harmful metals and metalloids in eco-efficient and environment-friendly methods under ambient conditions. In the present mini-review, we specifically address the active utilization of microbial respiration processes for the synthesis of novel functional biogenic nanomaterials by the members of the Shewanella genus. This biosynthetic method may provide alternative approaches to produce electrode materials for sustainable energy storage applications.
Introduction
Nanostructured materials are indispensable to modern life, and they are critical components of multiple industrial and consumer products, including daily necessities. For example, metallic nanoparticles (NPs) with tunable size, shape, and crystal structure have been applied in optical, electrical, and medical instruments. Over the years, researchers in academia as well as industries have investigated several methods for the synthesis of NPs, and various conventional physical and chemical methods have been established. However, most methods are not sustainable due to requirement of harmful or expensive chemicals, extreme temperatures and pressures during manufacturing processes, increased risk to environment and public health, and high energy consumption and waste generation (Cueva and Horsfall, 2017). Biotechnological routes such as the use of bacteria, fungi, and plant-extracts for synthesizing NPs are attractive alternative green approaches that utilize environment-friendly precursors and processes under mild conditions (Li et al., 2011; Pantidos and Horsfall, 2014; Prasad et al., 2018). In general, interdisciplinary studies between bionanotechnology and materials science are expected to accomplish major breakthroughs to resolve urgent environmental challenges such as industrial pollution and depletion of resources and achieve sustainable development goals.
Metal-reducing bacteria are considered eco-friendly catalysts for both bioremediation and materials synthesis. In particular, the members of the Shewanella genus can assist in the formation of diverse metal oxides and chalcogenides (materials containing S, Se, or Te elements) through microbial respiration processes. Notably, biogenic nanomaterials with unique morphologies and crystal structures produced by the members of the Shewanella genus can be used as Li-ion active electrode materials for lithium ion batteries (LIBs). The search for new chemistry or materials has received considerable attention for the production of high-performance LIBs, which are essential components in electric cars, portable gadgets, and several home appliances.
To the best of our knowledge, the biosynthesis of nanomaterials through the respiration process of metal-reducing bacteria and the application of these nanomaterials in LIBs has scarcely been reported. The current review provides a comprehensive overview on evidence from the two areas of research, namely the eco-efficient production of biogenic nanomaterials by the members of the Shewanella genus and their possible applications as Li-ion active electrodes for use as sustainable energy storage systems.
Dissimilatory Metal Reduction Process to Materials Synthesis
Microbial Respiration Process
Microbial dissimilatory anaerobic respiration can transfer electrons from reduced organic to oxidized inorganic compounds, thereby facilitating the cycling of carbon and metals as well as bioremediation processes. It is well-known that the members of the Shewanella genus are capable of coupling the oxidation of organic acids, as electron donors, to the reduction of inorganic metals, metalloids, and radionuclides, as electron acceptors, thereby changing the valence and oxidation state of the molecules through anaerobic respiration (Heidelberg et al., 2002; Tiedje, 2002; Harris et al., 2018). Bioreduction by the members of the Shewanella genus was discovered through the function of extracellularly excreted flavins and bacterial nanowires (Marsili et al., 2008; El-Naggar et al., 2010; Beblawy et al., 2018). This research, initiated for the immobilization of toxic metals and metalloids in aqueous environments, has since been utilized for the production of nano-sized inorganic compounds through microbial metal reduction processes.
Synthesis of Biogenic Nanomaterials by Shewanella Species
Anaerobic respiration processes provide facile synthetic strategies for the formation of unique minerals and nanomaterials under ambient conditions (Gadd, 2010). Recently, it was reported that the members of the Shewanella genus could produce various metal NPs such as silver (Ag), copper (Cu), palladium (Pd), iron (Fe), or manganese (Mn) oxides. Shewanella oneidensis MR-1 produced biogenic Ag-NPs that exhibited higher bactericidal activity compared with abiotic counterparts (Suresh et al., 2010). Kimber et al. (2018) showed that S. oneidensis MR-1 can synthesize Cu-NPs through bioreduction of Cu(II). The Pd-NPs produced by S. oneidensis MR-1 and S. loihica PV-4 showed significantly higher catalytic activities than the chemically produced equivalents (Wang et al., 2018; Xiong et al., 2018). In addition, the members of the Shewanella genus can produce iron or manganese oxides (Jiang et al., 2013; Wright et al., 2016).
The elements sulfur (S), selenium (Se), and tellurium (Te) belong to the oxygen family, also called chalcogens (meaning “copper producing”). Chalcogenide materials have been used widely in optical devices, phase change materials, and photovoltaic applications (Kovalenko et al., 2009; Sousa, 2011; Zhou et al., 2018). Production of diverse metal chalcogenides by a number of bacteria has been reported (Jacob et al., 2016; Vena et al., 2016; Boedicker et al., 2018). The chalcogens have a high affinity toward metals, making them powerful adsorbents for bioremediation process (Ralston, 2008). For example, toxic arsenate (As5+) can be reduced to arsenite (As3+) via microbial reduction process and precipitated into arsenic sulfide nanomaterials in the presence of thiosulfate (S2O32−) by using Shewanella sp. strain HN-41 (Figure 1A; Lee et al., 2007). The precipitation of the arsenic sulfide has also been observed using other members of the Shewanella genus (McFarlane et al., 2015).
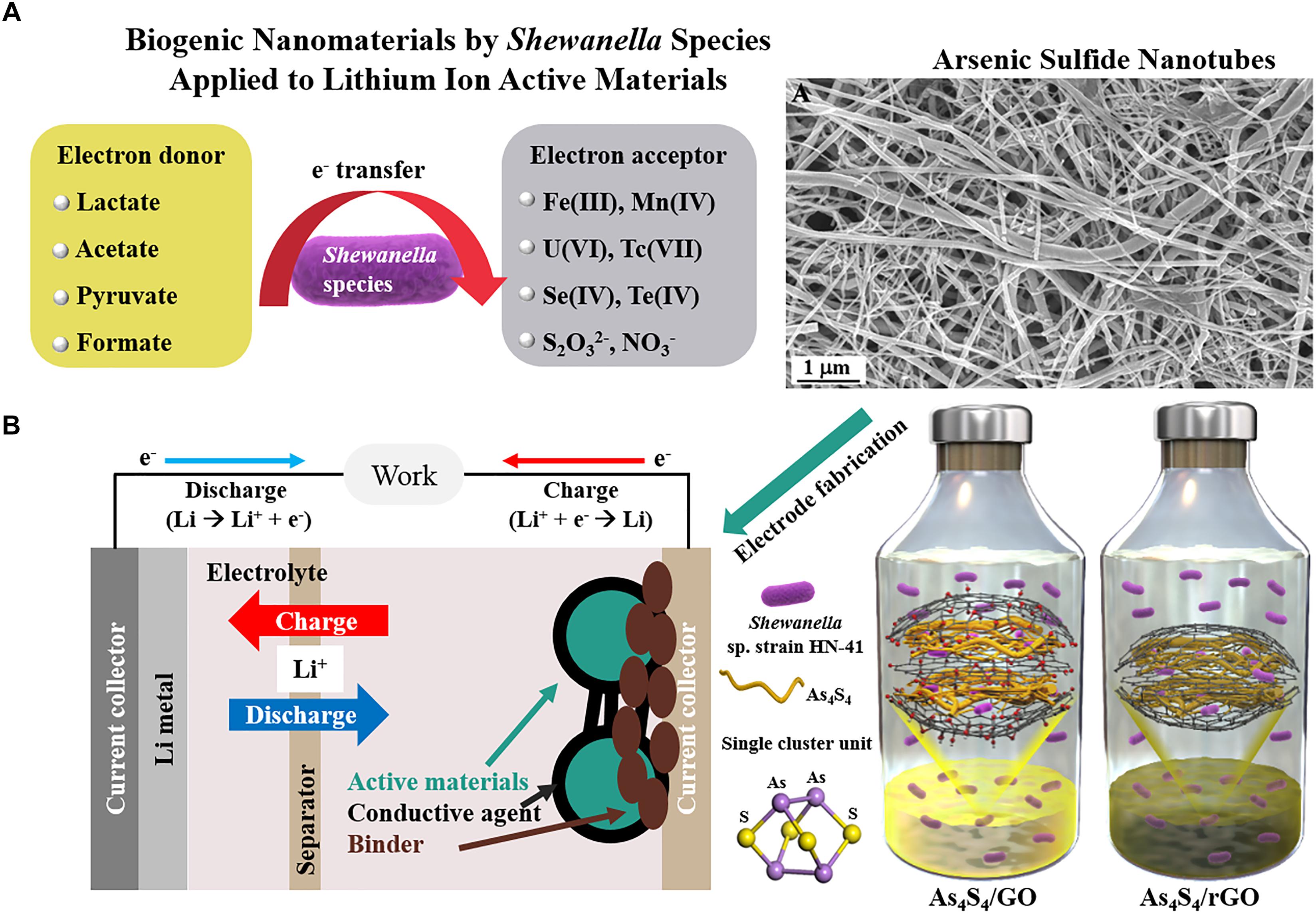
FIGURE 1. Application of biogenic nanomaterials produced by members of the Shewanella genus. (A) Microbiological respiration process and biogenic arsenic sulfide nanotubes (Reproduced with permission from PNAS 104 (51), 20410-20415. Copyright 2007 National Academy of Sciences, United States) and (B) biogenic As4S4/reduced graphene oxide applied as Li-ion active materials (Copyright 2017 Wiley. Used with permission from Adv. Sustain. Syst. 1(7), 1700056).
Another chalcogen element, Se, can be toxic in high doses and occurs in the form of oxyanions Se(IV) and Se(VI), which can be reduced by diverse Se-respiring bacteria to insoluble elemental Se (Pearce et al., 2011; Fernández-Llamosas et al., 2017; Shoeibi et al., 2017). It was reported that biogenic Se nanospheres could be applied to capture toxic mercury to remove both heavy metals without leading secondary pollutions (Jiang et al., 2012). Interestingly, biogenic amorphous Se nanospheres can be transformed into crystalline nanowires in dimethyl sulfoxide solvent at ambient conditions (Ho et al., 2010), suggesting that biological synthesis of nanomaterials could be more tunable and controllable with a combination of materials such as organic solvents and substrates.
The other chalcogen element, Te, has been exploited for numerous applications, leading to environmental issues due to high-toxicity of its oxyanions Te(IV) and Te(VI) (Ba et al., 2010). S. oneidensis MR-1 can utilize toxic soluble tellurite as electron acceptor, resulting in the formation of Te nanorods. It has been reported that needle-shaped crystalline Te nanorods were intracellularly or extracellularly formed depending on the reaction conditions (Kim et al., 2012, 2013). In short, the chalcogenide nanomaterials formed as the by-products from their bioremediation processes, with controllable morphologies, can be a valuable precursor for multiple applications.
Application of Biogenic Nanomaterials by Shewanella Species for Lithium Ion Batteries
LIBs are widely used as energy storage devices for portable electronics such as smartphones, home electronics, medical devices, and defense systems, mainly due to their light weight, high energy density, low memory effect, and low self-discharge rate. Interdisciplinary research can help with the current challenges of making sustainable LIBs with more eco-efficient processes (Larcher and Tarascon, 2015). Nanomaterials are expected to enhance the battery performance owing to short diffusion length for Li-ion traveling and high surface area (Aricò et al., 2005). Various nanostructured metal oxides and sulfides have gained attention as potential Li-ion active electrode material for LIBs (Wu et al., 2012; Xu et al., 2014). Furthermore, the use of nanomaterials synthesized by various nanobiotechnological routes as electrode materials in LIBs has been reviewed (Zhang et al., 2015). For example, the surfaces of modified viruses or bacteria have been utilized as biotemplates for LIB electrode materials. However, the low yield and complicated synthetic processes have hindered the industrial application of viral templates. In contrast, biomineralized iron oxides, produced by metal-oxidizing bacteria, have been used extensively as precursors for Li-ion active electrode materials (Miot et al., 2014). The LIB cell is composed of an anode, cathode, electrolyte, and a separator (Figure 1B). In a commercial cell, the cathode part is a lithium cobalt oxide and the anode is usually graphite. To test Li-ion storage capability of biogenic nanomaterials, they can be reacted with lithium metal. For the preparation of the LIB cell, biogenic nanomaterials as Li-ion active materials have to be physically mixed with conductive agent and binder to increase conductivity and mechanical stability between active materials, conductive agent, and current collector (Korthauer, 2018). Thus, nanostructured iron oxide and metal chalcogenide NPs produced by the members of the Shewanella genus have gained considerable interest as new materials or chemistry for LIBs because S, Se, or Te have already been extensively investigated as Li-ion storage materials (Bruce et al., 2012; Seo et al., 2015; Eftekhari, 2017).
Biogenic arsenic sulfide (As4S4) nanomaterials have been applied as photoconductive devices (Lee et al., 2007) or field-effect transistors (McFarlane et al., 2015). Moreover, the unique cage-like molecular structure of the biogenic As4S4 formed by Shewanella sp. strain HN-41 was adopted for Li-ion active electrode materials (Kim et al., 2017). Intrinsic low conductivity of the biogenic As4S4 was improved by in situ microbial reduction of graphene oxide (Salas et al., 2010; Wang et al., 2011), a usual tactic to increase electrical conductivity (Raccichini et al., 2014). As a second example, biogenic Te nanorods formed by S. oneidensis MR-1 have an integrated network of closely packed atomic wires. The empty volume space between the atomic wires of the Te nanorods was anticipated as a unique Li-ion storage material (Kim et al., 2015). Thermal carbonization of intracellular or extracellular biogenic Te nanorods with bacterial cells provided encapsulation of the Te nanorods in the carbon matrix, which increased electrical conductivity and enhanced battery performance. Interestingly, the intracellular Te nanorods exhibited different phase transition during the carbonization process compared with the extracellular Te material, revealed as distinct voltage profile characteristics. Distinct Li-ion storage mechanism of biogenic nanomaterials can be investigated during battery charge-discharge cycles via various in situ methods such as X-ray diffraction or X-ray absorption techniques (Figure 2A; Hapuarachchi et al., 2018). The structural changes of the biogenic As4S4 or Te nanomaterials during charge-discharge processes suggest the possible application of the biogenic nanomaterials for high-performance Li-ion active anode systems.
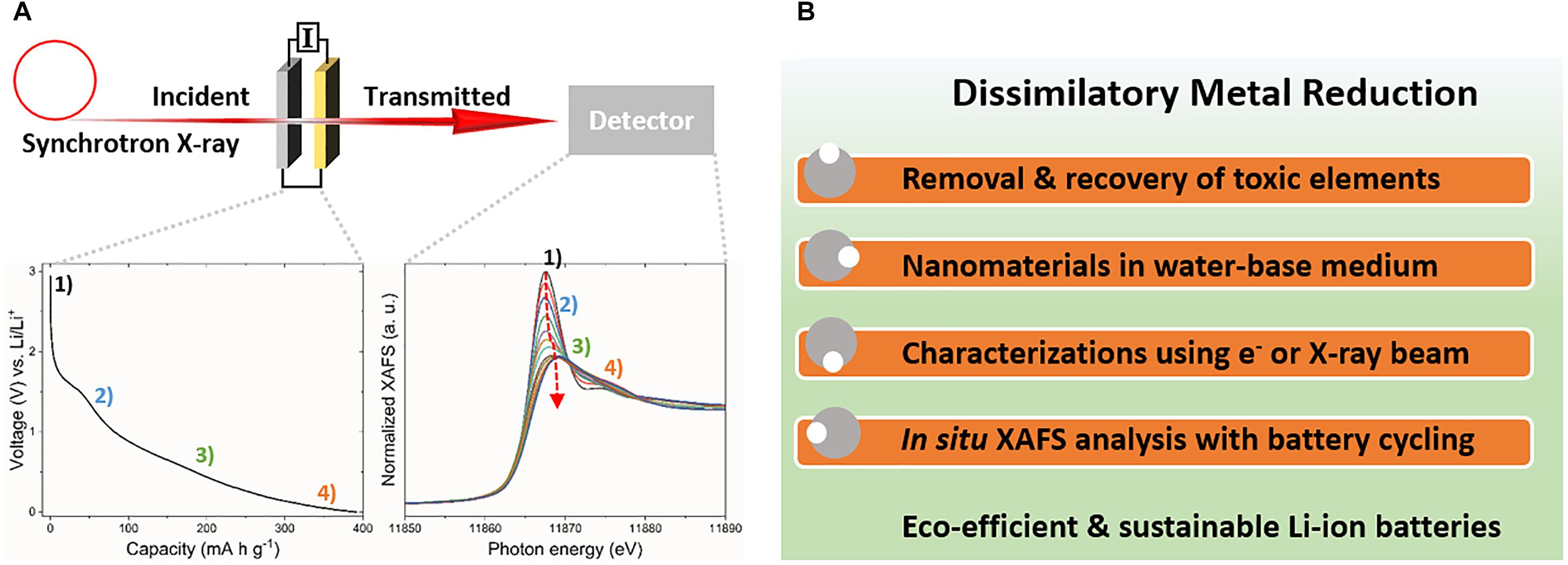
FIGURE 2. Methods for research on biogenic nanomaterials. (A) In situ X-ray absorption spectroscopy during charge-discharge processes and (B) collaborative research activities for microbiological synthesis and application of biogenic nanomaterials.
Thus, research has enabled the removal of the toxic water-soluble As or Te from aqueous environments through immobilization into insoluble As4S4 or Te precipitates that have lower bioavailability. Furthermore, these precipitated As4S4 or Te nanomaterials have been successfully revalorized in field-effect transistors (McFarlane et al., 2015) and LIBs (Kim et al., 2017). However, the issue of recycling these batteries and transistors still needs to be addressed. Compared with the well-established conventional recycling of lead-acid batteries, there are currently no procedures in place for the recycling of spent LIBs (Gaines, 2014). However, bioleaching appears to be an environment-friendly way to recover valuable compounds such as Co, Ni, or Au, as well as As or Te in spent LIBs (Biswal et al., 2018). Apart from the issue of recycling, it is desirable to synthesize valuable nanomaterials using non-toxic resources. Iron oxide nanomaterials biosynthesized by metal-oxidizing bacteria have been applied as electrode materials (Miot et al., 2014). Moreover, it was reported that iron phosphate nanomaterials produced by Shewanella species have been successfully applied as electrode materials in LIBs (Kageyama et al., 2015).
Future Perspectives
The synthesis of nanomaterials by dissimilatory metal-reducing bacteria and their application as Li-ion active electrode materials should involve interdisciplinary collaborative research with following aims (Figure 2B):
(1) Development of eco-efficient and environment-friendly bioremediation processes for the removal of harmful heavy metals, metalloids, and radionuclides.
(2) Green synthesis for low-cost and environment-friendly biogenic nanomaterials.
(3) Characterization of the size, morphology, and crystal structures of the prepared biogenic nanomaterials using electron and X-ray analysis.
(4) Application of biogenic nanomaterials as Li-ion active electrode materials and in situ synchrotron X-ray analysis during charge-discharge processes to reveal their Li-ion storage mechanism.
One of challenges for the application of biogenic nanomaterials is their inhomogeneous morphologies or chemical compositions compared with the chemically synthesized counterparts. In addition, the presence of large quantities of organic compounds such as bacterial cell debris or excreted proteins may reduce the electrical conductivity and hinder a direct application of biogenic nanomaterials. Thus, post-treatment might be a requirement to improve the applicability of biogenic nanomaterials or to enhance their physicochemical properties.
Concluding Remarks
The exploitation of microorganisms for bioremediation has garnered considerable research interest. The anaerobic respiration process can be used to transform toxic elements into sparingly soluble forms with low bioavailability and toxicity. This microbiological transformation process can also provide an alternative green chemistry approach for the biosynthesis of nanomaterials for use in sustainable Li-ion storage systems. The investigation and application of the biogenic nanomaterials produced by members of the Shewanella genus, can provide valuable insights into the ecological aspects of microbe–metal interaction and yield important milestones for the development of eco-efficient and sustainable Li-ion storage technologies, while simultaneously reducing environmental burden.
Author Contributions
T-YK wrote the draft of the manuscript as the first author. MGK and H-GH contributed to the conception and design of the work. J-HL revised the manuscript critically for important intellectual content. H-GH provided approval for the publication as the corresponding author.
Funding
This work was supported by the National Research Foundation of Korea (NRF) grant funded by the Korea government (MSIP) (NRF-2016R1A2B3013974) and the Ministry of Education (2016R1D1A3B01012231 and 2012R1A1A2043811). Synchrotron-based experiments performed at Pohang Light Source II (PLS-II) were supported in part by the Ministry of Education and POSTECH.
Conflict of Interest Statement
The authors declare that the research was conducted in the absence of any commercial or financial relationships that could be construed as a potential conflict of interest.
The reviewer VE-B and handling Editor declared their shared affiliation.
References
Aricò, A. S., Bruce, P., Scrosati, B., Tarascon, J.-M., and Van Schalkwijk, W. (2005). Nanostructured materials for advanced energy conversion and storage devices. Nat. Mater. 4, 366–377. doi: 10.1038/nmat1368
Ba, L. A., Döring, M., Jamier, V., and Jacob, C. (2010). Tellurium: an element with great biological potency and potential. Org. Biomol. Chem. 8, 4203–4216. doi: 10.1039/c0Ob00086h
Beblawy, S., Bursac, T., Paquete, C., Louro, R., Clarke, T. A., and Gescher, J. (2018). Extracellular reduction of solid electron acceptors by Shewanella oneidensis. Mol. Microbiol. 109, 571–583. doi: 10.1111/mmi.14067
Biswal, B. K., Jadhav, U. U., Madhaiyan, M., Ji, L., Yang, E.-H., and Cao, B. (2018). Biological leaching and chemical precipitation methods for recovery of Co and Li from spent lithium-ion batteries. ACS Sustain. Chem. Eng. 6, 12343–12352. doi: 10.1021/acssuschemeng.8b02810
Boedicker, J. Q., Chellamuthu, P., Tran, F., Silva, P., and El Naggar, M. (2018). Engineering bacteria for biogenic synthesis of chalcogenide nanomaterials. Microb. Biotech. (in press). doi: 10.1111/1751-7915.13320
Bruce, P. G., Freunberger, S. A., Hardwick, L. J., and Tarascon, J.-M. (2012). Li-O2 and Li-S batteries with high energy storage. Nat. Mater. 11, 19–29. doi: 10.1038/nmat3191
Cueva, M. E., and Horsfall, L. E. (2017). The contribution of microbially produced nanoparticles to sustainable development goals. Microb. Biotechnol. 10, 1212–1215. doi: 10.1111/1751-7915.12788
Eftekhari, A. (2017). The rise of lithium-selenium batteries. Sustain. Energy Fuels 1, 14–29. doi: 10.1039/C6SE00094K
El-Naggar, M. Y., Wanger, G., Leung, K. M., Yuzvinsky, T. D., Southam, G., Yang, J., et al. (2010). Electrical transport along bacterial nanowires from Shewanella oneidensis MR-1. Proc. Natl. Acad. Sci. U.S.A. 107, 18127–18131. doi: 10.1073/pnas.1004880107
Fernández-Llamosas, H., Castro, L., Blázquez, M. L., Díaz, E., and Carmona, M. (2017). Speeding up bioproduction of selenium nanoparticles by using Vibrio natriegens as microbial factory. Sci. Rep. 7:16046. doi: 10.1038/s41598-017-16252-1
Gadd, G. M. (2010). Metals, minerals and microbes: geomicrobiology and bioremediation. Microbiology 156, 609–643. doi: 10.1099/mic.0.037143-0
Gaines, L. (2014). The future of automotive lithium-ion battery recycling: charting a sustainable course. Sustain. Mater. Technol. 1–2, 2–7. doi: 10.1016/j.susmat.2014.10.001
Hapuarachchi, S. N. S., Sun, Z., and Yan, C. (2018). Advances in in situ techniques for characterization of failure mechanisms of li-ion battery anodes. Adv. Sustain. Syst. 2:1700182. doi: 10.1002/adsu.201700182
Harris, H. W., Sánchez-Andrea, I., Mclean, J. S., Salas, E. C., Tran, W., El-Naggar, M. Y., et al. (2018). Redox sensing within the genus Shewanella. Front. Microbiol. 8:2568. doi: 10.3389/fmicb.2017.02568
Heidelberg, J. F., Paulsen, I. T., Nelson, K. E., Gaidos, E. J., Nelson, W. C., Read, T. D., et al. (2002). Genome sequence of the dissimilatory metal ion-reducing bacterium Shewanella oneidensis. Nat. Biotechnol. 20, 1118–1123. doi: 10.1038/nbt749
Ho, C. T., Kim, J. W., Kim, W. B., Song, K., Kanaly, R. A., Sadowsky, M. J., et al. (2010). Shewanella-mediated synthesis of selenium nanowires and nanoribbons. J. Mater. Chem. 20, 5899–5905. doi: 10.1039/b923252d
Jacob, J. M., Lens, P. N. L., and Balakrishnan, R. M. (2016). Microbial synthesis of chalcogenide semiconductor nanoparticles: a review. Microb. Biotechnol. 9, 11–21. doi: 10.1111/1751-7915.12297
Jiang, S., Ho, C. T., Lee, J.-H., Van Duong, H., Han, S., and Hur, H.-G. (2012). Mercury capture into biogenic amorphous selenium nanospheres produced by mercury resistant Shewanella putrefaciens 200. Chemosphere 87, 621–624. doi: 10.1016/j.chemosphere.2011.12.083
Jiang, S., Kim, M.-G., Kim, I. Y., Hwang, S.-J., and Hur, H.-G. (2013). Biological synthesis of free-standing uniformed goethite nanowires by Shewanella sp. HN-41. J. Mater. Chem. A 1, 1646–1650. doi: 10.1039/C2TA00466F
Kageyama, H., Hashimoto, Y., Oaki, Y., Saito, S., Konishi, Y., and Imai, H. (2015). Application of biogenic iron phosphate for lithium-ion batteries. RSC Adv. 5, 68751–68757. doi: 10.1039/C5RA11090D
Kim, D.-H., Kanaly, R. A., and Hur, H.-G. (2012). Biological accumulation of tellurium nanorod structures via reduction of tellurite by Shewanella oneidensis MR-1. Bioresour. Technol. 125, 127–131. doi: 10.1016/j.biortech.2012.08.129
Kim, D.-H., Kim, M.-G., Jiang, S., Lee, J.-H., and Hur, H.-G. (2013). Promoted reduction of tellurite and formation of extracellular tellurium nanorods by concerted reaction between iron and Shewanella oneidensis MR-1. Environ. Sci. Technol. 47, 8709–8715. doi: 10.1021/es401302w
Kim, M. G., Kim, D.-H., Kim, T., Park, S., Kwon, G., Kim, M. S., et al. (2015). Unusual Li-ion storage through anionic redox processes of bacteria-driven tellurium nanorods. J. Mater. Chem. A 3, 16978–16987. doi: 10.1039/C5TA04038H
Kim, T. Y., Ahn, H., Jeon, J., Kim, M. S., Kim, M. G., and Hur, H. G. (2017). Biogenic realgar As4S4 molecular clusters formed by a one-pot microbial-driven process as a li-ion storage material. Adv. Sustain. Syst. 1:1700056. doi: 10.1002/adsu.201700056
Kimber, R. L., Lewis, E. A., Parmeggiani, F., Smith, K., Bagshaw, H., Starborg, T., et al. (2018). Biosynthesis and characterization of copper nanoparticles using Shewanella oneidensis: application for click chemistry. Small 14:1703145. doi: 10.1002/smll.201703145
Korthauer, R. (2018). Lithium-Ion Batteries: Basics and Applications. Berlin: Springer. doi: 10.1007/978-3-662-53071-9
Kovalenko, M. V., Scheele, M., and Talapin, D. V. (2009). Colloidal nanocrystals with molecular metal chalcogenide surface ligands. Science 324, 1417–1420. doi: 10.1126/science.1170524
Larcher, D., and Tarascon, J. M. (2015). Towards greener and more sustainable batteries for electrical energy storage. Nat. Chem. 7, 19–29. doi: 10.1038/nchem.2085
Lee, J.-H., Kim, M. G., Yoo, B., Myung, N. V., Maeng, J., Lee, T., et al. (2007). Biogenic formation of photoactive arsenic-sulfide nanotubes by Shewanella sp. strain HN-41. Proc. Natl. Acad. Sci. U.S.A. 104, 20410–20415. doi: 10.1073/pnas.0707595104
Li, X., Xu, H., Chen, Z.-S., and Chen, G. (2011). Biosynthesis of nanoparticles by microorganisms and their applications. J. Nanomater. 2011:270974. doi: 10.1155/2011/270974
Marsili, E., Baron, D. B., Shikhare, I. D., Coursolle, D., Gralnick, J. A., and Bond, D. R. (2008). Shewanella secretes flavins that mediate extracellular electron transfer. Proc. Natl. Acad. Sci. U.S.A. 105, 3968–3973. doi: 10.1073/pnas.0710525105
McFarlane, I. R., Lazzari-Dean, J. R., and El-Naggar, M. Y. (2015). Field effect transistors based on semiconductive microbially synthesized chalcogenide nanofibers. Acta Biomater. 13, 364–373. doi: 10.1016/j.actbio.2014.11.005
Miot, J., Recham, N., Larcher, D., Guyot, F., Brest, J., and Tarascon, J. M. (2014). Biomineralized α-Fe2O3: texture and electrochemical reaction with Li. Energy Environ. Sci. 7, 451–460. doi: 10.1039/C3EE41767K
Pantidos, N., and Horsfall, L. E. (2014). Biological synthesis of metallic nanoparticles by bacteria, fungi and plants. J. Nanomed. Nanotechnol. 5:233. doi: 10.4172/2157-7439.1000233
Pearce, C. I., Baesman, S. M., Blum, J. S., Fellowes, J. W., and Oremland, R. S. (2011). “Nanoparticles formed from microbial oxyanion reduction of toxic group 15 and group 16 metalloids,” in Microbial Metal and Metalloid Metabolism, eds J. F. Stolz and R. S. Oremland (Washington, DC: American Society for Microbiology), 297–319.
Prasad, R., Kumar, V., Kumar, M., and Wang, S. (2018). Fungal Nanobionics: Principles and Applications. Berlin: Springer. doi: 10.1007/978-981-10-8666-3
Raccichini, R., Varzi, A., Passerini, S., and Scrosati, B. (2014). The role of graphene for electrochemical energy storage. Nat. Mater. 14, 271–279. doi: 10.1038/nmat4170
Ralston, N. (2008). Nano-selenium captures mercury. Nat. Nanotechnol. 3, 527–528. doi: 10.1038/nnano.2008.251
Salas, E. C., Sun, Z., LüTtge, A., and Tour, J. M. (2010). Reduction of graphene oxide via bacterial respiration. ACS Nano 4, 4852–4856. doi: 10.1021/nn101081t
Seo, J.-U., Seong, G.-K., and Park, C.-M. (2015). Te/C nanocomposites for Li-Te secondary batteries. Sci. Rep. 5:7969. doi: 10.1038/srep07969
Shoeibi, S., Mozdziak, P., and Golkar-Narenji, A. (2017). Biogenesis of selenium nanoparticles using green chemistry. Top. Curr. Chem. 375:88. doi: 10.1007/s41061-017-0176-x
Sousa, V. (2011). Chalcogenide materials and their application to non-volatile memories. Microelectron. Eng. 88, 807–813. doi: 10.1016/j.mee.2010.06.042
Suresh, A. K., Pelletier, D. A., Wang, W., Moon, J.-W., Gu, B., Mortensen, N. P., et al. (2010). Silver nanocrystallites: biofabrication using Shewanella oneidensis, and an evaluation of their comparative toxicity on gram-negative and gram-positive bacteria. Environ. Sci. Technol. 44, 5210–5215. doi: 10.1021/es903684r
Tiedje, J. M. (2002). Shewanella-the environmentally versatile genome. Nat. Biotechnol. 20, 1093–1094. doi: 10.1038/nbt1102-1093
Vena, M. P., Jobbágy, M., and Bilmes, S. A. (2016). Microorganism mediated biosynthesis of metal chalcogenides; a powerful tool to transform toxic effluents into functional nanomaterials. Sci. Total Environ. 565, 804–810. doi: 10.1016/j.scitotenv.2016.04.019
Wang, G., Qian, F., Saltikov, C. W., Jiao, Y., and Li, Y. (2011). Microbial reduction of graphene oxide by Shewanella. Nano Res. 4, 563–570. doi: 10.1007/s12274-011-0112-2
Wang, W., Zhang, B., Liu, Q., Du, P., Liu, W., and He, Z. (2018). Biosynthesis of palladium nanoparticles using Shewanella loihica PV-4 for excellent catalytic reduction of chromium(VI). Environ. Sci. Nano 5, 730–739. doi: 10.1039/C7EN01167A
Wright, M. H., Farooqui, S. M., White, A. R., and Greene, A. C. (2016). Production of manganese oxide nanoparticles by Shewanella species. Appl. Environ. Microbiol. 82, 5402–5409. doi: 10.1128/AEM.00663-16
Wu, H. B., Chen, J. S., Hng, H. H., and Lou, X. W. D. (2012). Nanostructured metal oxide-based materials as advanced anodes for lithium-ion batteries. Nanoscale 4, 2526–2542. doi: 10.1039/c2nr11966h
Xiong, L., Zhang, X., Huang, Y.-X., Liu, W.-J., Chen, Y.-L., Yu, S.-S., et al. (2018). Biogenic synthesis of Pd-based nanoparticles with enhanced catalytic activity. ACS Appl. Nano Mater. 1, 1467–1475. doi: 10.1021/acsanm.7b00322
Xu, X., Liu, W., Kim, Y., and Cho, J. (2014). Nanostructured transition metal sulfides for lithium ion batteries: progress and challenges. Nano Today 9, 604–630. doi: 10.1016/j.nantod.2014.09.005
Zhang, X., Hou, Y., He, W., Yang, G., Cui, J., Liu, S., et al. (2015). Fabricating high performance lithium-ion batteries using bionanotechnology. Nanoscale 7, 3356–3372. doi: 10.1039/c4nr06815g
Keywords: Shewanella species, metal-reducing bacteria, biogenic nanomaterials, chalcogenides, lithium ion batteries, in situ XAFS analysis
Citation: Kim T-Y, Kim MG, Lee J-H and Hur H-G (2018) Biosynthesis of Nanomaterials by Shewanella Species for Application in Lithium Ion Batteries. Front. Microbiol. 9:2817. doi: 10.3389/fmicb.2018.02817
Received: 21 August 2018; Accepted: 02 November 2018;
Published: 21 November 2018.
Edited by:
Louise Elizabeth Horsfall, The University of Edinburgh, United KingdomReviewed by:
Virginia Echavarri-Bravo, The University of Edinburgh, United KingdomM. Oves, King Abdulaziz University, Saudi Arabia
Copyright © 2018 Kim, Kim, Lee and Hur. This is an open-access article distributed under the terms of the Creative Commons Attribution License (CC BY). The use, distribution or reproduction in other forums is permitted, provided the original author(s) and the copyright owner(s) are credited and that the original publication in this journal is cited, in accordance with accepted academic practice. No use, distribution or reproduction is permitted which does not comply with these terms.
*Correspondence: Hor-Gil Hur, hghur@gist.ac.kr