- 1Department of Hematology and Rheumatology, Faculty of Medicine, Kindai University, Osaka, Japan
- 2Division of Hematology, Department of Internal Medicine, School of Medicine, Aichi Medical University, Nagakute, Japan
Hematopoietic stem cell transplantation (HSCT) is a highly advanced technique that offers a potential cure for an increasing number of life-threatening diseases. Enormous progress achieved in the last decade, including the refinement of donor selection and advancements in patient supportive care, had significantly improved transplant outcomes; however, invasive infections, graft-vs.-host disease (GVHD) and other serious complications still represent a major source of morbidity and mortality in HSCT recipients. The damage of anatomical barriers due to pre-transplant conditioning, a severely damaged immune function and a profound disruption in the composition of gut microbial commensals (gut microbiota) are alterations inherent to the transplant procedure that are directly implicated in the development of invasive infections and other HSCT complications. Although HLA-matching represents the most important genetic predictor of transplant outcomes, genetic variants in non-HLA genes, especially single nucleotide polymorphisms (SNPs) of genes encoding proteins associated with the immune response to tissue injury and pathogen infection have also been proposed as additional risk factors implicated in the occurrence of HSCT complications. Furthermore, although the microbiota composition is affected by several factors, recent evidence suggests that certain host genetic variants are associated with an altered composition of the gut microbiome and may, therefore, predispose some individuals to invasive infectious complications. This article summarizes the current understanding of the influence that genetic variants in non-HLA genes have on the development of infectious complications in HSCT recipients.
Introduction
Hematopoietic stem cell transplantation (HSCTs) involves the intravenous infusion of hematopoietic stem and progenitor cells (HSPCs) to restore the bone marrow (BM) function after intensive cancer-eradicating treatments or to replace defective bone marrow with normal bone marrow in non-malignant hematologic disorders (Gratwohl et al., 2010; Passweg et al., 2016). Currently, HSCT is utilized with curative intent in a variety of blood malignant diseases, such as leukemias, lymphomas and myeloma, as well as some solid tumors, such as germ cell tumors, soft tissue sarcomas and neuroblastoma (Holtick et al., 2014; Passweg et al., 2016) and is a curative option for thalassemia, sickle cell anemia, severe combined immunodeficiency and for young patients with severe aplastic anemia (Bacigalupo and Sica, 2016).
HSPCs for HSCT are harvested from BM, peripheral blood (after the donor receives granulocyte colony-stimulating factor to mobilize HSPCs) or cord blood, being donor availability, patient's age and the underlying disease the main factors that determine the decision to use a certain source of HSPCs (Holtick et al., 2014; Niederwieser et al., 2016). Although BM was the main source of HSPCs for many years, currently HSCT is most frequently done with peripheral blood stem cells (PBSCT) (Gratwohl et al., 2010; Anasetti et al., 2012; Juric et al., 2016).
Depending on the donor source, HSCT can be autologous (auto-HSCT) or allogenic (allo-HSCT) (Anasetti et al., 2012; Juric et al., 2016). Auto-HSCT is essentially indicated in patients undergoing intensive or high-dose chemoradiotherapy (myeloablative) for the treatment of certain malignant diseases and involves the harvesting and cryopreservation of HSPCs from patients themselves and then reinfusing those HSPCs into the patients after myeloablative therapy in order to restore hematopoiesis and hasten the immune function (Niederwieser et al., 2016; Bogunia-Kubik and Łacina, 2017). Allo-HSCT is the transplantation of HSPCs from a histocompatible healthy donor that may be a fully matched or half matched (haploidentical) family relative to the patient or an unrelated donor (Juric et al., 2016; Niederwieser et al., 2016).
A critical component of HSCT is the conditioning regimen administered prior to the infusion of HSPCs and is aimed to eradicate the patient's disease and to prevent rejection of the transplanted graft. Conditioning can be myeloablative, in which the recipient BM is destroyed (ablated), usually using high doses of chemotherapy and whole-body irradiation, or non-myeloablative (Holtick et al., 2014; Juric et al., 2016). Graft-vs.-host disease (GVHD) and transplant-related mortality (TRM) constitute important limitations associated with HSCT (Gratwohl et al., 2010; Passweg et al., 2016). In addition, the exposure of HSCT recipients to multiple antibiotics, utilized either for curative or preventive purposes, eventually results in the disruption of the gut microbiota composition that further increases the patients' susceptibility to severe infection complications (Taur et al., 2014; Dandoy et al., 2017).
HLA-matching is the most important genetic determinant of transplant outcomes; however, single-nucleotide polymorphisms (SNPs) in non-HLA genes, especially in genes encoding immune response factors, appear to affect HSCT outcomes, including relapse, survival and GVHD, (Espinoza et al., 2009; Dickinson and Norden, 2015; Bogunia-Kubik and Łacina, 2017).
This article highlights potential associations between genetic variants in genes encoding non-classical HLA proteins and the development of infection-related complications in HSCT recipients. Recent studies that appear to link genetic variants in certain genes with specific changes in the gut microbiota composition are also discussed.
Infectious Complications in HSCT Recipients
Conditioning regimens required for HSCT incite mucosal injuries and destroys hematopoiesis leading to the damage of normal anatomical barriers and extensive immunodeficiency, especially in those receiving myeloablative regimens (Balletto and Mikulska, 2015; Zama et al., 2017). As a result, infection complications are major cause of morbidity and mortality in HSCT recipients and are often associated with commensal bacteria and fungi derived from the gastrointestinal tract and with opportunistic organisms colonizing the skin that entry the bloodstream via intravenous catheters. Engraftment, which occurs at a range: 6–84 days after HSCT (median 22 days), is defined by the capacity of HSCT recipients to maintain an absolute neutrophil count of > 500/mm3 and platelet count of >20,000, lasting >3 consecutive days without transfusions. The median time to engraftment is longer for bone marrow transplantation (BMT) recipients than in those receiving PBSCT, which may explain the relative higher risk of infections in BMT in comparison with PBSCT (Young et al., 2016).
Immune reconstitution after HSCT occurs in three phases: the pre-engraftment phase (<30 days after HSCT); early post-engraftment phase (30–100 days after HSCT); and late post-engraftment phase (>100 days after HSCT) (van den Brink et al., 2015; Ogonek et al., 2016). Each phase is characterized by specific host-defense defects with defined risk of infections (Table 1). During the pre-engraftment phase the presence of neutropenia, severe mucositis and central venous catheter are major risks of infection (Gudiol et al., 2014). The majority of infections in this period are due to bacteria, with Gram positive organisms predominating over Gram negatives (Gudiol et al., 2014; Kikuchi et al., 2015; Schuster et al., 2017; Mikulska et al., 2018). Clostridium difficile infection in HSCT recipients most often occurs during early pre-engraftment (Schuster et al., 2017) and fungal infections (Candida spp. and Aspergillus spp.) and herpes simplex virus (HSV) reactivation can be also seen in this phase (Balletto and Mikulska, 2015; Sahin et al., 2016; Alp and Akova, 2017; Cho et al., 2018).
During the post-engraftment phase, the weakened cell-mediated immunity, together with the development of GVHD and the use of corticosteroids and immunosuppressive constitute key risk factors of infection, especially pneumonia and gastrointestinal infections. In this phase, Gram positive organisms also predominating over Gram negatives (Gudiol et al., 2014; Sahin et al., 2016; Cho et al., 2018). In the late post-engraftment phase patients have increased risk for infections with encapsulated bacteria, due to hypogammaglobulinemia and The exposure to immunosuppressive treatment for controlling GVHD. In addition, Invasive fungal infections, cytomegalovirus (CMV), varicella-zoster virus (VZV), EBV-related post-transplant lymphoproliferative disease (PTLD) can be seen during this period.
SNPs of Immunoregulatory Genes and HSCT
The most frequent genetic variations of the human genome are SNPs, and the majority occur in non-coding regions, including intronic, intergenic, and untranslated regions (UTRs). Some are considered functional, as they can affect the gene expression or may alter the function of their coding protein (Dickinson and Norden, 2015; Bogunia-Kubik and Łacina, 2017). To identify non-HLA gene SNPs associated with transplant outcomes, two approaches have been utilized so far: candidate gene studies (CGSs), which directly test target gene variants selected on the basis of the biological potential reported in previous studies; and genome-wide association studies (GWASs), in which many gene polymorphisms are examined on a hypothesis-free basis. With both the CGS and GWAS approaches, it is important that the association found in a discovery cohort also be observed in an independent cohort in order to validate the association (Takami, 2013; Bogunia-Kubik and Łacina, 2017).
For over a decade, several groups, including our own, have reported that genetic variants outside of the HLA region, especially in genes encoding components of the immune system, are predictive of the outcome following HSCT (Espinoza et al., 2009; Takami, 2013; Bogunia-Kubik and Łacina, 2017). However, many of these gene-association studies were conducted in small series, and some included heterogeneous diseases with patients treated across multiple years with variable transplant regimens; furthermore, the data were often not adjusted to a standardized format (Karaesmen et al., 2017). In addition, the majority of the published studies did not include a confirmatory cohort, so few of the reported gene associations have been consistently validated. Nonetheless, a number of polymorphisms in genes encoding cytokines and chemokines, such as INF-γ, IL-2, IL-1, IL-6, and TNF-α, Granzyme B (GZMB), IL-17 and their receptors, have been reported in association with various transplant outcomes, including the overall survival, disease relapse, graft rejection and GVHD (Espinoza et al., 2009, 2011a,b; Nakata et al., 2013; Dickinson and Norden, 2015; Bogunia-Kubik and Łacina, 2017; Gam et al., 2017).
Non-HLA Variants and Infectious Complications After HSCT
Early studies showed that the donor and recipient genotypes in genes that regulate the host response to microorganisms, including myeloperoxidase (MPO), mannose binding lectin (MBL) and Fcγ receptors (FcγRIIa, IIIa, IIIb), were reportedly associated with infections after BMT (Rocha et al., 2002). In addition, the steroid hormone receptor supergene family includes the estrogen receptor and the vitamin D receptor (VDR), both of which have marked effects on the development of the immune system (Rocha et al., 2002; Middleton et al., 2003). The AA genotype of the VDR gene in recipients is associated with less severe GVHD, while its presence in the donor appears to lead to a reduced protective effect against infection and relapse, most notably in those treated with more intense immunosuppressive therapies (Middleton et al., 2002).
Mezger et al. reported an association between the recipient CXCL10 and the development of invasive aspergillosis in a European cohort of 81 allo-HSCT recipients (Mezger et al., 2008). The variant 642C/G, which is determined by the rs3921 SNP, has been associated with susceptibility to other infections (Pineda-Tenor et al., 2014; Hoh et al., 2015). In a larger cohort of 652 HSCT Japanese patients who underwent unrelated HLA-matched (BMT) for hematologic malignancies, our group found that a recipient C/G or G/G genotype associated with a significantly better 5-year overall survival (OS) rate, a lower TRM rate and a reduced incidence of death due to organ failure compared with the recipient C/C genotype (Nakata et al., 2013). CXCL10 is produced by innate immune cells and by several non-hematopoietic cells, including endothelial cells, vascular pericytes, keratinocytes, fibroblasts and astrocytes, and is considered a “key driver chemokine” that has been linked with the pathogenesis of autoimmune diseases, such as inflammatory bowel disease (IBD), multiple sclerosis and rheumatoid arthritis, and is involved in the immune response to infections (Karin and Razon, 2018). Elevated levels of CXCL10 have been consistently observed in bodily fluids and inflamed tissues of HSCT recipients with acute GVHD, so this chemokine has been proposed as a biomarker of this transplant complication (Ahmed et al., 2015).
Toll-like receptors (TLRs) are a family of transmembrane proteins on the surface of immune cells that detect microbe-associated molecular patterns (MAMP) from a variety of microorganisms. In response to their interaction with microbial motifs, TLRs activate intracellular signals, leading to the synthesis of cytokines and other inflammatory mediators. Although TLRs constitute the first line of defense against many pathogens, they may also promote excessive immune responses, leading to tissue damage. Previous studies have implicated TLR signals in cytokine storm and the inflammatory process leading to GVHD and other complications in HSCT recipients (Maeda, 2013; Heidegger et al., 2014), and several SNPs in TLR genes have been associated with susceptibility to infections (Skevaki et al., 2015; Dhangadamajhi et al., 2017). Bochud et al. reported that donor TLR4 haplotype S4, which is defined by the TLR4 variations rs4986790 [D299G] and rs4986791 [T399I], was associated with a risk of invasive aspergillosis after allo-HSCT (Bochud et al., 2008). This haplotype appears to influence the TLR4 function, but it is absent in Asian populations (Aki et al., 2015). In a cohort of 365 Japanese patients who underwent unrelated HLA-matched bone marrow transplantation (BMT) for hematologic malignancies, the SNP rs11536889 in TLR4 gene, whose minor allele frequencies in the Asian population are relatively high (>0.2) (Tahara et al., 2010; Sato et al., 2012), +3725G/G genotype in the donor side was significantly associated with a better 5-years progression-free survival and a lower 5-years TRM than other variations. Furthermore, the donor TLR4 genotype +3725G/G was associated with a significantly lower incidence of fatal infections than other variations and these results were confirmed in a validation cohort (Uchino et al., 2017).
Another component of the innate system involved in the response to pathogen products is the nucleotide-binding oligomerization domain-containing protein 2 (NOD2). This intracellular sensor recognizes conserved motifs in bacterial peptidoglycan and activates intracellular signals that drive antimicrobial immune responses cooperating with TLRs in the detection and elimination of invading microbes (Caruso et al., 2014). NOD2 is considered a major risk factor for the development of IBD (Caruso et al., 2014). SNPs within the NOD2 gene are associated with the susceptibility to various bacterial infections (van Well et al., 2013; Branković et al., 2015) and have been linked with the risk for allo-HSCT complications, including TRM, GVHD, and bronchiolitis obliterans (Holler et al., 2004; Hildebrandt et al., 2008). In a small cohort of allo-HSCT recipients, the donor NOD2-SNP13 was significantly associated with the incidence of septic shock (Grube et al., 2015). The association between the NOD2-SNP13 and the incidence of sepsis in the context of allo-HSCT was further replicated in a larger cohort (464 patients) of allo-HSCT recipients treated in five different transplantation units in Poland (Jaskula et al., 2014), thus substantiating the biological significance of this genetic variation in infectious complications.
Dectin-1 is a C-type lectin receptor member expressed on the surface granulocyte immune cells that plays an important role in the immune response to fungal pathogens by sensing the cell wall components of fungi, including Candida species and Aspergillus species (Hirose et al., 2017). In a cohort of 142 patients undergoing HSCT, recipients harboring the Y238X polymorphism in the DECTIN-1 gene were more likely to be colonized with Candida species than patients bearing WT DECTIN-1 and required the more frequent use of fluconazole to prevent systemic Candida infection (Plantinga et al., 2009). In a cohort of 205 patients receiving allo-HSCT for blood malignancies, the presence of the DECTIN1 Y238X polymorphism was found to be associated with an increased susceptibility to aspergillosis, with the risk being highest when Y238X was present simultaneously in both donors and recipients (adjusted hazard ratio = 3.9; P = 0.005) (Cunha et al., 2010). Functional studies showed a decreased cytokine production by immune cells bearing this polymorphism on fungal-specific stimulation (Plantinga et al., 2009; Cunha et al., 2010). Dectin-1 knockout (KO) mice showed a higher fungal burden than wild type mice indicating that Dectin-1 is crucial for clearing fungal infections (Cunha et al., 2010).
The Influence of Human Microbiota on the Outcomes of Transplantation
Antibiotics that destroy gut commensal microbiota communities deplete the host's commensal gut bacteria and ultimately allow the growth of pathogenic microbial species (Zama et al., 2017), and the outgrowth of opportunistic pathogens belonging to the phylum Proteobacteria and genus Enterococcus have also been linked to increased TRM, including GVHD, infections and organ failure after allo-HSCT (Staffas et al., 2017).
Compelling evidence suggest that microbiota composition before transplant may predict the risk of infection complications, including bloodstream infections (BSI) (Figure 1). For example, patients who develop BSI after HSCT, exhibit decreased microbiome diversity and decreased abundance of taxa including Barnesiellaceae, Coriobacteriaceae, Faecalibacterium, Dehalobacterium, and Sutterella (Montassier et al., 2016). In pediatric allo-HSCT recipients, acute-GVHD and non-acute-GVHD subjects also possess a different pre-HSCT gut microbiota composition. In particular, non- acute-GVHD patients showed a pre-HSCT gut microbiota characterized by a higher abundance of members of the Bacteroidetes phylum (Parabacteroides and Bacteroides) (Biagi et al., 2015).
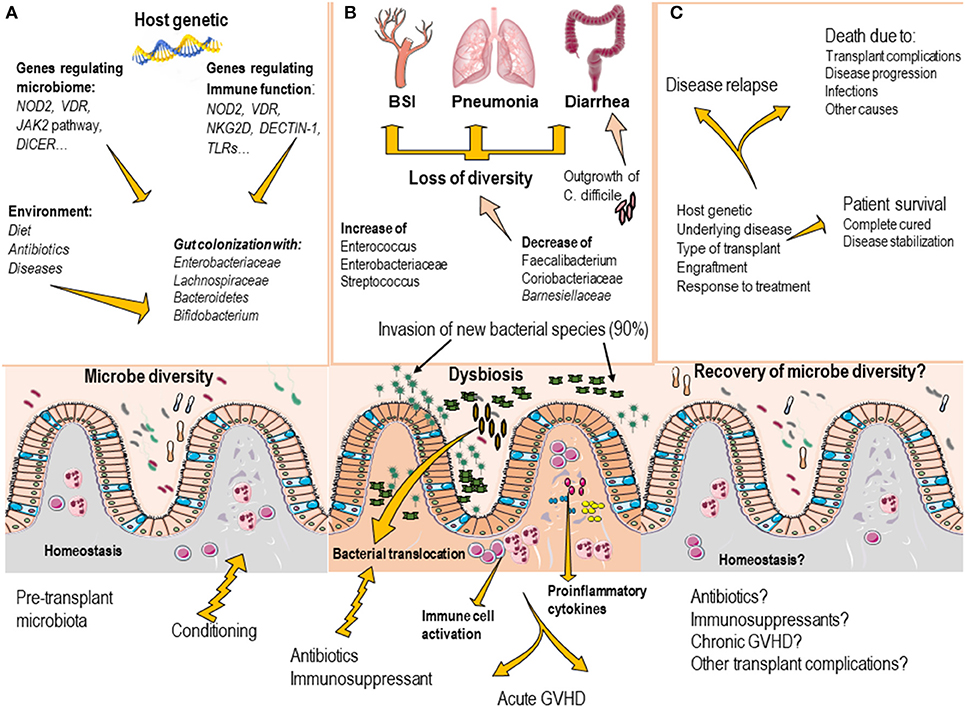
Figure 1. Commensal bacteria of the gut are important modulators of tissue and immune homeostasis (A). The gut microbiota composition is mainly regulated by environmental factors, including diet, use of antibiotics, and the disease status. Host genetics factors appears to contribute to regulate the gut microbiota composition as genetic variations in genes that regulate immune response and genes that directly influence microbiota has been identified. (B) In transplant recipients, the conditioning regimen and the use of antibiotics, induce profound disruption of gut microbiota composition (loss of bacterial diversity, decrease of protective species and outgrowth of pathogenic microorganism), which may result in the development of invasive infections (BSI, pneumonia and clostridium difficile–induced diarrhea). Dysbiosis also contributes to increase the risk to develop transplant complications, such as graft vs. host disease. (C) As the transplant progress, most patients recover the gut microbiota composition (usually by day 60 after transplant), however the presence of transplant complications, massive use of antibiotics may delay the gut microbiota recovery.
In other study, the presence of Enterobacteriaceae at the beginning of the pretransplant conditioning regimen in stool samples of allo-HSCT recipients was the only significant marker for the risk of microbiologically confirmed sepsis. An increased risk of overall mortality, including death from both infectious and non-infectious causes, was observed when Lachnospiraceae were ≤10% at the beginning of the pretransplant conditioning regimen (Mancini et al., 2017). In addition, the presence of 3 distinct bacterial taxa (Bacteroidetes, Lachnospiraceae, and Ruminococcaceae) in the gut microbiome of allo-HSCT recipients correlated with protection against C. difficile infection (Lee et al., 2017). These data strongly suggest that manipulating the gut microbiota may be useful to prevent BSI in high-risk patients and efforts aimed at maintaining protective commensals may help to minimize the risk of C. difficile infection in allo-HSCT recipients.
Genetic Variation and Microbiota Composition
Environmental factors play a central role in determining the composition of human microbiota; however, accumulating evidence suggest that host genetics can influence the microbiome composition. The host genetics regulate the immune system, nutrient availability and the secretion of immunoglobulin A (IgA) and thus can shape the chemical and physical composition of the environment inhabited by the gut microbiome.
Recent microbiome genome-wide association studies (mGWASs), which are cohort studies that combine human genotyping or GWASs with microbiome analyses, have identified numerous associations between the gut microbiome and specific human genetic variants (Table 2) (Goodrich et al., 2014, 2016; Knights et al., 2014; Wacklin et al., 2014; Davenport et al., 2015, 2016; Beaumont et al., 2016; Bonder et al., 2016; Turpin et al., 2016; Wang et al., 2016). Thus far, the most consistent evidence emerging from mGWAS that support a role for the host genetics in defining the composition of the gut microbiome is the link between the host genotype, the post-weaning consumption of milk and the colonization with Bifidobacterium, which has been consistently validated in various cohorts (Goodrich et al., 2017; Hall et al., 2017). In addition, several immunity-related risk alleles have been found to alter the microbiome composition. For example, Knights et al. reported that individuals carrying specific NOD2 variants show significant enrichment of Enterobacteriaceae bacteria (Knights et al., 2014). These findings may support the above discussed observations linking NOD2 variants with severe infectious complications in HSCT (Holler et al., 2004; Hildebrandt et al., 2008; Jaskula et al., 2014; Grube et al., 2015), as such infections in HSCT recipients are associated with gut microbiota dysbiosis (Zama et al., 2017). Of note, the study by Knights et al. also identified specific bacteria taxa in the gut microbiome associated with various IBD-related SNPs and involving genes that regulate the innate immune response and the JAK-STAT pathway (Knights et al., 2014).
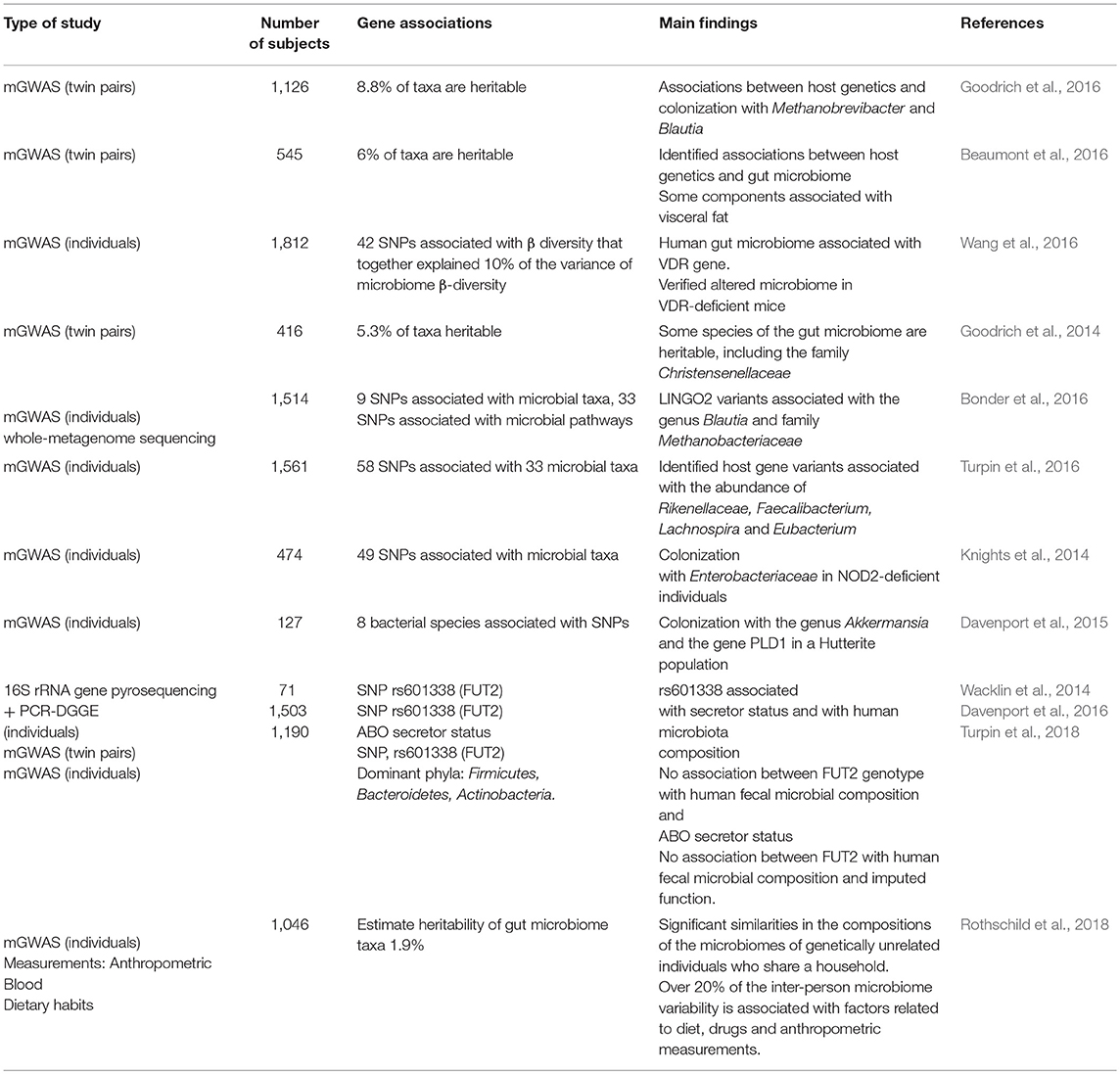
Table 2. Studies investigating potential associations between human genetics and microbiota composition.
A recent GWAS of the gut microbiota using two cohorts from Germany (1,812 individuals in total) revealed significant associations between the overall microbial variation and individual taxa at multiple genetic loci, including the VDR gene. In addition, the microbiota composition correlated with the serum levels of selected bile and fatty acids, including known ligands and downstream metabolites of VDR (Wang et al., 2016). Mouse experiments conducted by the same team revealed significant shifts in the microbiota of Vdr−/− mice relative to control mice (Wang et al., 2016), which is consistent with a previous study that observed the massive depletion of Lactobacillus with the concomitant enrichment of Clostridium and Bacteroides in the intestines of Vdr−/− mice (Jin et al., 2015).
FUT2 encodes fucosyltransferase 2, a major component of mucosal barrier that serves as energy source for some bacteria and a binding site for others. A common nonsense mutation (428G > A) in the FUT2 gene determines that individuals who have an AA genotype lack terminal fucose in the mucin layer; these people are considered nonsecretors (Hall et al., 2017). GWAS identified a FUT2 variant (AA genotype) associated with an altered gut microbiome, FUT2 nonsecretor-status and increased risk of Crohn's disease (McGovern et al., 2010). The AA genotype has been also linked with increased susceptibility to certain infections (Lindesmith et al., 2003; Folseraas et al., 2012) and with increased incidence of bacteremia and high risk of acute GVHD in allo-HSCT recipients (Rayes et al., 2016). Notably, no association was found between FUT2 genotypes, microbiota composition and secretor status in two large cohorts of healthy, highlighting the importance of replicating microbiome-associated traits in large cohorts (Davenport et al., 2016; Turpin et al., 2018).
Pathogenic bacteria are capable of modulating the expression of small non-coding micro RNAs (miRNAs) in the host, and given the crucial role of miRNA in the post-transcriptional regulation of several host genes, bacterial can thus manipulate host cell pathways that are directly implicated in the outcome of infection (Maudet et al., 2014). However, emerging evidence suggests that commensal microorganisms are also capable of regulating the host miRNA expression (Williams et al., 2017). Liu et al. recently reported that miRNAs are detectable in the gut lumen and feces of both mice and humans and that these RNAs are able to regulate the gut microbiota composition (Liu et al., 2016) and Moloney et al, proposed that the fecal miRNA composition can be used as an independent biomarker of microbial fluctuations along with the gut pathology in the intestine (Moloney et al., 2018). It must be noted that SNPs affecting the miRNA levels, such as those implicated in miRNA biogenesis or SNPs capable of affecting the miRNA/target interaction, can modulate the expression of cytokines, chemokines or immune receptors encoding genes and consequently may contribute to the risk of complications associated with HSCT (Gam et al., 2017; Stickel et al., 2017). For example, the SNP rs1049174 in the KLRK1 gene (which encodes the immune receptor NKG2D) resides in a motif that is targeted by miRNA1245, a negative regulator of the NKG2D expression. Notably, allo-HSCT recipients receiving grafts from donors with the LNK genotype of rs1049174 have worse transplant outcomes, including an impaired OS and increased risk of TRM, than recipients receiving graft from donors with the HNK genotype in rs1049174(Hayashi et al., 2006; Espinoza et al., 2009). Natural killer (NK) cells from individuals harboring the LNK genotype express lower levels of NKG2D on their surface and have lower NKG2D-mediated functions, including killing target cells expressing NKG2D ligands on their surface than NK cells from HNK due to the higher affinity of the LNK allele for miR1245 (Espinoza et al., 2012, 2016). Consequently, rs1049174 is implicated in HSCT outcomes via the regulation of the NKG2D expression by immune cells.
Concluding Remarks and Future Directions
HSCT recipients often suffer severe alterations in their microbiota composition, and it has become evident that dysbiosis, especially that of the gut microbiota, is involved in the development of transplant complications, including acute GVHD and invasive BSI.
Although the human microbiota is essentially determined by environmental factors, convincing evidence indicates that genetics help regulate the microbiota composition. Of particular interest is the finding that several immunity-related risk alleles, such as NOD2 variants, have been shown to alter the microbiome composition in association with Enterobacteriaceae family enrichment in the gut microbiota (Knights et al., 2014), which may explain the link between NOD2 variants and severe infectious complications in HSCT (Holler et al., 2004; Hildebrandt et al., 2008; Jaskula et al., 2014; Grube et al., 2015). However, most previously reported associations failed to reach statistically significance after multiple testing correction (Kurilshikov et al., 2017) and a recent study that evaluated a cohort of several different ancestral origins that share a relatively homogeneous environment demonstrate that gut microbiome composition is shaped predominantly by environmental factors (Rothschild et al., 2018).
Fecal microbiota transplantation (FMT), which consists of the infusion of fecal material or fecal bacterial preparations from a healthy donor into the gut of a recipient patient with altered microbiota, is safe and effective in controlling C. difficile infection after HSCT (Webb et al., 2016). This indicates that therapeutic interventions aimed at modifying the altered microbiota, such as FMT or via the use of probiotics, may have clinical utility in certain patients. Alternative approaches may include the use of more precise antibiotics as well as dietary changes, nutritional prebiotic supplements that support the growth of commensal organisms, probiotics and FMT. In addition, the use of “engineered microbiota,” has been shown in preclinical models to have great therapeutic potential. For example, a mixture of comprehensively selected strains of Clostridia was very effective in promoting the expansion and differentiation of Tregs in a mouse model of colitis (Atarashi et al., 2013), and the precision reconstitution of C. scindens, a commensal that confers resistance to C. difficile colonization via bile acid production, was very effective in preventing C. difficile infection (Buffie et al., 2015). Finally, the use of artificial intelligence tools capable of integrating clinical information with microbiome data represent a promising strategy for tackling infection complications in HSCT recipients (Espinoza, 2018).
Author Contributions
JE conceived the study, wrote the manuscript, and designed figures. YW collected references and wrote the manuscript. AT wrote the manuscript.
Conflict of Interest Statement
The authors declare that the research was conducted in the absence of any commercial or financial relationships that could be construed as a potential conflict of interest.
Acknowledgments
AT was supported by grants from the Ministry of Health, Labor and Welfare of Japan, and the Ministry of Education, Culture, Sports and Technology of Japan. The grant sources played no role in the study design, data collection and the decision to publish this manuscript.
References
Ahmed, S. S., Wang, X. N., Norden, J., Pearce, K., El-Gezawy, E., Atarod, S., et al. (2015). Identification and validation of biomarkers associated with acute and chronic graft versus host disease. Bone Marrow Transplant. 50, 1563–1571. doi: 10.1038/bmt.2015.191
Aki, K., Okubo, Y., Nanjo, H., Ishiwatari, T., Nihonyanagi, Y., Tochigi, N., et al. (2015). Genomic analysis of single nucleotide polymorphisms Asp299Gly and Thr399Ile in japanese patients with invasive aspergillosis. Jpn. J. Infect. Dis. 68, 330–332. doi: 10.7883/yoken.JJID.2014.420
Alp, S., and Akova, M. (2017). Antibacterial Resistance in Patients with Hematopoietic Stem Cell Transplantation. Mediterr. J. Hematol. Infect. Dis. 9:e2017002. doi: 10.4084/MJHID.2017.002
Anasetti, C., Logan, B. R., Lee, S. J., Waller, E. K., Weisdorf, D. J., Wingard, J. R., et al. (2012). Peripheral-blood stem cells versus bone marrow from unrelated donors. N. Engl. J. Med. 367, 1487–1496. doi: 10.1056/NEJMoa1203517
Atarashi, K., Tanoue, T., Oshima, K., Suda, W., Nagano, Y., Nishikawa, H., et al. (2013). Treg induction by a rationally selected mixture of Clostridia strains from the human microbiota. Nature 500, 232–236. doi: 10.1038/nature12331
Bacigalupo, A., and Sica, S. (2016). Alternative donor transplants for severe aplastic anemia: current experience. Semin. Hematol. 53, 115–119. doi: 10.1053/j.seminhematol.2016.01.002
Balletto, E., and Mikulska, M. (2015). Bacterial infections in hematopoietic stem cell transplant recipients. Mediterr. J. Hematol. Infect. Dis. 7:e2015045. doi: 10.4084/MJHID.2015.045
Beaumont, M., Andriamihaja, M., Lan, A., Khodorova, N., Audebert, M., Blouin, J. M., et al. (2016). Detrimental effects for colonocytes of an increased exposure to luminal hydrogen sulfide: the adaptive response. Free Radic. Biol. Med. 93, 155–64. doi: 10.1016/j.freeradbiomed.2016.01.028
Biagi, E., Zama, D., Nastasi, C., Consolandi, C., Fiori, J., Rampelli, S., et al. (2015). Gut microbiota trajectory in pediatric patients undergoing hematopoietic SCT. Bone Marrow Transplant. 50, 992–998. doi: 10.1038/bmt.2015.16
Bochud, P. Y., Chien, J. W., Marr, K. A., Leisenring, W. M., Upton, A., Janer, M., et al. (2008). Toll-like receptor 4 polymorphisms and aspergillosis in stem-cell transplantation. N. Engl. J. Med. 359, 1766–1777. doi: 10.1056/NEJMoa0802629
Bogunia-Kubik, K., and Łacina, P. (2017). From genetic single candidate gene studies to complex genomics of GvHD. Br. J. Haematol. 178, 661–675. doi: 10.1111/bjh.14704
Bonder, M. J., Kurilshikov, A., Tigchelaar, E. F., Mujagic, Z., Imhann, F., Vila, A. V., et al. (2016). The effect of host genetics on the gut microbiome. Nat. Genet. 48, 1407–1412. doi: 10.1038/ng.3663
Branković, I., van Ess, E. F., Noz, M. P., Wiericx, W. A., Spaargaren, J., Morré, S. A., et al. (2015). NOD1 in contrast to NOD2 functional polymorphism influence Chlamydia trachomatis infection and the risk of tubal factor infertility. Pathog. Dis. 73, 1–9. doi: 10.1093/femspd/ftu028
Buffie, C. G., Bucci, V., Stein, R. R., McKenney, P. T., Ling, L., Gobourne, A., et al. (2015). Precision microbiome reconstitution restores bile acid mediated resistance to Clostridium difficile. Nature 517, 205–208. doi: 10.1038/nature13828
Caruso, R., Warner, N., Inohara, N., and Núñez, G. (2014). NOD1 and NOD2: signaling, host defense, and inflammatory disease. Immunity 41, 898–908. doi: 10.1016/j.immuni.2014.12.010
Cho, S. Y., Lee, H. J., and Lee, D. G. (2018). Infectious complications after hematopoietic stem cell transplantation: current status and future perspectives in Korea. Korean J. Intern. Med. 33, 256–276. doi: 10.3904/kjim.2018.036
Cunha, C., Di Ianni, M., Bozza, S., Giovannini, G., Zagarella, S., Zelante, T., et al. (2010). Dectin-1 Y238X polymorphism associates with susceptibility to invasive aspergillosis in hematopoietic transplantation through impairment of both recipient- and donor-dependent mechanisms of antifungal immunity. Blood 116, 5394–5402. doi: 10.1182/blood-2010-04-279307
Dandoy, C. E., Ardura, M. I., Papanicolaou, G. A., and Auletta, J. J. (2017). Bacterial bloodstream infections in the allogeneic hematopoietic cell transplant patient: new considerations for a persistent nemesis. Bone Marrow Transplant. 52, 1091–1106. doi: 10.1038/bmt.2017.14
Davenport, E. R., Cusanovich, D. A., Michelini, K., Barreiro, L. B., Ober, C., and Gilad, Y. (2015). Genome-wide association studies of the human gut microbiota. PLoS ONE 10:e0140301. doi: 10.1371/journal.pone.0140301
Davenport, E. R., Goodrich, J. K., Bell, J. T., Spector, T. D., Ley, R. E., and Clark, A. G. (2016). ABO antigen and secretor statuses are not associated with gut microbiota composition in 1,500 twins. BMC Genomics. 17:941. doi: 10.1186/s12864-016-3290-1
Dhangadamajhi, G., Kar, A., Rout, R., and Dhangadamajhi, P. (2017). A meta-analysis of TLR4 and TLR9 SNPs implicated in severe malaria. Rev. Soc. Bras. Med. Trop. 50, 153–160. doi: 10.1590/0037-8682-0475-2016
Dickinson, A. M., and Norden, J. (2015). Non-HLA genomics: does it have a role in predicting haematopoietic stem cell transplantation outcome? Int. J. Immunogenet. 42, 229–238. doi: 10.1111/iji.12202
Espinoza, J. L. (2018). Machine learning for tackling microbiota data and infection complications in immunocompromised patients with cancer. J. Intern. Med. 284, 189–192. doi: 10.1111/joim.12746.
Espinoza, J. L., Nguyen, V. H., Ichimura, H., Pham, T. T., Nguyen, C. H., Pham, T. V., et al. (2016). A functional polymorphism in the NKG2D gene modulates NK-cell cytotoxicity and is associated with susceptibility to Human Papilloma Virus-related cancers. Sci. Rep. 6:39231. doi: 10.1038/srep39231
Espinoza, J. L., Takami, A., Nakata, K., Onizuka, M., Kawase, T., Akiyama, H., et al. (2011a). A genetic variant in the IL-17 promoter is functionally associated with acute graft-versus-host disease after unrelated bone marrow transplantation. PLoS ONE 6:e26229. doi: 10.1371/journal.pone.0026229
Espinoza, J. L., Takami, A., Onizuka, M., Sao, H., Akiyama, H., Miyamura, K., et al. (2009). NKG2D gene polymorphism has a significant impact on transplant outcomes after HLA-fully-matched unrelated bone marrow transplantation for standard risk hematologic malignancies. Haematologica. 94, 1427–1434. doi: 10.3324/haematol.2009.008318
Espinoza, J. L., Takami, A., Yoshioka, K., Nakata, K., Sato, T., Kasahara, Y., et al. (2012). Human microRNA-1245 down-regulates the NKG2D receptor in natural killer cells and impairs NKG2D-mediated functions. Haematologica97, 1295–1303. doi: 10.3324/haematol.2011.058529
Espinoza, L. J., Takami, A., Nakata, K., Yamada, K., Onizuka, M., Kawase, T., et al. (2011b). Genetic variants of human granzyme B predict transplant outcomes after HLA matched unrelated bone marrow transplantation for myeloid malignancies. PLoS ONE 6:e23827. doi: 10.1371/journal.pone.0023827
Fabiani, S., Fortunato, S., Petrini, M., and Bruschi, F. (2017). Allogeneic hematopoietic stem cell transplant recipients and parasitic diseases: A review of the literature of clinical cases and perspectives to screen and follow-up active and latent chronic infections. Transpl. Infect. Dis. 19:e12669. doi: 10.1111/tid.12669
Folseraas, T., Melum, E., Rausch, P., Juran, B. D., Ellinghaus, E., Shiryaev, A., et al. (2012). Extended analysis of a genome-wide association study in primary sclerosing cholangitis detects multiple novel risk loci. J. Hepatol. 57, 366–375. doi: 10.1016/j.jhep.2012.03.031
Gam, R., Shah, P., Crossland, R. E., Norden, J., Dickinson, A. M., and Dressel, R. (2017). Genetic association of hematopoietic stem cell transplantation outcome beyond histocompatibility genes. Front. Immunol. 8:380. doi: 10.3389/fimmu.2017.00380
Girmenia, C., Bertaina, A., Piciocchi, A., Perruccio, K., Algarotti, A., Busca, A., et al. (2017). Incidence, risk factors and outcome of pre-engraftment gram-negative bacteremia after allogeneic and autologous hematopoietic stem cell transplantation: an italian prospective multicenter survey. Clin. Infect. Dis. 65, 1884–1896. doi: 10.1093/cid/cix690
Goodrich, J. K., Davenport, E. R., Beaumont, M., Jackson, M. A., Knight, R., Ober, C., et al. (2016). Genetic determinants of the gut microbiome in UK twins. Cell Host Microbe. 19, 731–743. doi: 10.1016/j.chom.2016.04.017
Goodrich, J. K., Davenport, E. R., Clark, A. G., and Ley, R. E. (2017). the relationship between the human genome and microbiome comes into view. Annu. Rev. Genet. 51, 413–433. doi: 10.1146/annurev-genet-110711-155532
Goodrich, J. K., Waters, J. L., Poole, A. C., Sutter, J. L., Koren, O., Blekhman, R., et al. (2014). Human genetics shape the gut microbiome. Cell 159, 789–799. doi: 10.1016/j.cell.2014.09.053
Gratwohl, A., Baldomero, H., Aljurf, M., Pasquini, M. C., Bouzas, L. F., and Yoshimi, A. (2010). Hematopoietic stem cell transplantation: a global perspective. JAMA 303, 1617–1624. doi: 10.1001/jama.2010.491
Grube, M., Brenmoehl, J., Rogler, G., Hahn, J., Herr, W., and Holler, E. (2015). Donor nucleotide-binding oligomerization-containing protein 2 (NOD2) single nucleotide polymorphism 13 is associated with septic shock after allogeneic stem cell transplantation. Biol. Blood Marrow Transplant. 21, 1399–1404. doi: 10.1016/j.bbmt.2015.05.011
Gudiol, C., Garcia-Vidal, C., Arnan, M., Sánchez-Ortega, I., Patiño, B., Duarte, R., et al. (2014). Etiology, clinical features and outcomes of pre-engraftment and post-engraftment bloodstream infection in hematopoietic SCT recipients. Bone Marrow Transplant. 49, 824–830. doi: 10.1038/bmt.2014.37
Hall, A. B., Tolonen, A. C., and Xavier, R. J. (2017). Human genetic variation and the gut microbiome in disease. Nat. Rev. Genet. 18, 690–699. doi: 10.1038/nrg.2017.63
Hayashi, T., Imai, K., Morishita, Y., Hayashi, I., Kusunoki, Y., and Nakachi, K. (2006). Identification of the NKG2D haplotypes associated with natural cytotoxic activity of peripheral blood lymphocytes and cancer immunosurveillance. Cancer Res. 66, 563–70. doi: 10.1158/0008-5472.CAN-05-2776
Heidegger, S., van den Brink, M. R., Haas, T., and Poeck, H. (2014). The role of pattern-recognition receptors in graft-versus-host disease and graft-versus-leukemia after allogeneic stem cell transplantation. Front. Immunol. 5:337. doi: 10.3389/fimmu.2014.00337
Hildebrandt, G. C., Granell, M., Urbano-Ispizua, A., Wolff, D., Hertenstein, B., Greinix, H. T., et al. (2008). Recipient NOD2/CARD15 variants: a novel independent risk factor for the development of bronchiolitis obliterans after allogeneic stem cell transplantation. Biol. Blood Marrow Transplant. 14, 67–74. doi: 10.1016/j.bbmt.2007.09.009
Hirose, K., Ito, T., and Nakajima, H. (2017). Roles of dectin-1 in allergic airway inflammation. Crit. Rev. Immunol. 37, 15–21. doi: 10.1615/CritRevImmunol.2017024718
Hoh, B. P., Umi-Shakina, H., Zuraihan, Z., Zaiharina, M. Z., Rafidah-Hanim, S., Mahiran, M., et al. (2015). Common variants of chemokine receptor gene CXCR3 and its ligands CXCL10 and CXCL11 associated with vascular permeability of dengue infection in peninsular Malaysia. Hum. Immunol. 76, 421–426. doi: 10.1016/j.humimm.2015.03.019
Holler, E., Rogler, G., Herfarth, H., Brenmoehl, J., Wild, P. J., Hahn, J., et al. (2004). Both donor and recipient NOD2/CARD15 mutations associate with transplant-related mortality and GvHD following allogeneic stem cell transplantation. Blood 104, 889–894. doi: 10.1182/blood-2003-10-3543
Holtick, U., Albrecht, M., Chemnitz, J. M., Theurich, S., Skoetz, N., Scheid, C., et al. (2014). Bone marrow versus peripheral blood allogeneic haematopoietic stem cell transplantation for haematological malignancies in adults. Cochrane Database Syst. Rev. CD010189. doi: 10.1002/14651858.CD010189.pub2
Jarque, I., Salavert, M., and Pemán, J. (2016). Parasitic Infections in Hematopoietic Stem Cell Transplantation. Mediterr. J. Hematol. Infect. Dis. 8:e2016035. doi: 10.4084/MJHID.2016.035
Jaskula, E., Lange, A., Kyrcz-Krzemien, S., Markiewicz, M., Dzierzak-Mietla, M., Jedrzejczak, W. W., et al. (2014). NOD2/CARD15 single nucleotide polymorphism 13 (3020insC) is associated with risk of sepsis and single nucleotide polymorphism 8 (2104C>T) with herpes viruses reactivation in patients after allogeneic hematopoietic stem cell transplantation. Biol. Blood Marrow Transplant. 20, 409–414. doi: 10.1016/j.bbmt.2013.12.558
Jin, D., Wu, S., Zhang, Y. G., Lu, R., Xia, Y., and Dong, H. (2015). Lack of vitamin D receptor causes dysbiosis and changes the functions of the murine intestinal microbiome. Clin. Ther. 37, 996–1009.e7. doi: 10.1016/j.clinthera.2015.04.004
Juric, M. K., Ghimire, S., Ogonek, J., Weissinger, E. M., Holler, E., van Rood, J. J., et al. (2016). Milestones of hematopoietic stem cell transplantation - from first human studies to current developments. Front. Immunol. 7:470. doi: 10.3389/fimmu.2016.00470
Karaesmen, E., Rizvi, A. A., Preus, L. M., McCarthy, P. L., Pasquini, M. C., Onel, K., et al. (2017). Replication and validation of genetic polymorphisms associated with survival after allogeneic blood or marrow transplant. Blood 130, 1585–1596. doi: 10.1182/blood-2017-05-784637
Karin, N., and Razon, H. (2018). Chemokines beyond chemo-attraction: CXCL10 and its significant role in cancer and autoimmunity. Cytokine 109, 24–28. doi: 10.1016/j.cyto.2018.02.012
Kikuchi, M., Akahoshi, Y., Nakano, H., Ugai, T., Wada, H., Yamasaki, R., et al. (2015). Risk factors for pre- and post-engraftment bloodstream infections after allogeneic hematopoietic stem cell transplantation. Transpl. Infect. Dis. 17, 56–65. doi: 10.1111/tid.12345
Knights, D., Silverberg, M. S., Weersma, R. K., Gevers, D., Dijkstra, G., Huang, H., et al. (2014). Complex host genetics influence the microbiome in inflammatory bowel disease. Genome Med. 6:107. doi: 10.1186/s13073-014-0107-1
Kurilshikov, A., Wijmenga, C., Fu, J., and Zhernakova, A. (2017). Host genetics and gut microbiome: challenges and perspectives. Trends Immunol. 38, 633–647. doi: 10.1016/j.it.2017.06.003
Lee, Y. J., Arguello, E. S., Jenq, R. R., Littmann, E., Kim, G. J., Miller, L. C., et al. (2017). Protective factors in the intestinal microbiome against clostridium difficile infection in recipients of allogeneic hematopoietic stem cell transplantation. J. Infect. Dis. 215, 1117–1123. doi: 10.1093/infdis/jix011
Lin, R., and Liu, Q. (2013). Diagnosis and treatment of viral diseases in recipients of allogeneic hematopoietic stem cell transplantation. J. Hematol. Oncol. 6:94. doi: 10.1186/1756-8722-6-94
Lindesmith, L., Moe, C., Marionneau, S., Ruvoen, N., Jiang, X., Lindblad, L., et al. (2003). Human susceptibility and resistance to Norwalk virus infection. Nat. Med. 9, 548–553. doi: 10.1038/nm860
Liu, S., da Cunha, A. P., Rezende, R. M., Cialic, R., Wei, Z., Bry, L., et al. (2016). The Host Shapes the Gut Microbiota via Fecal MicroRNA. Cell Host Microbe 19, 32–43. doi: 10.1016/j.chom.2015.12.005
Maeda, Y. (2013). Pathogenesis of graft-versus-host disease: innate immunity amplifying acute alloimmune responses. Int. J. Hematol. 98, 293–299. doi: 10.1007/s12185-013-1421-x
Maertens, J. A., Girmenia, C., Brüggemann, R. J., Duarte, R. F., Kibbler, C. C., Ljungman, P., et al. (2018). European guidelines for primary antifungal prophylaxis in adult haematology patients: summary of the updated recommendations from the European Conference on Infections in Leukaemia. J. Antimicrob. Chemother. doi: 10.1093/jac/dky286. [Epub ahead of print].
Mancini, N., Greco, R., Pasciuta, R., Barbanti, M. C., Pini, G., Morrow, O. B., et al. (2017). Enteric microbiome markers as early predictors of clinical outcome in allogeneic hematopoietic stem cell transplant: results of a prospective study in adult patients. Open Forum Infect Dis. 4:ofx215. doi: 10.1093/ofid/ofx215
Maudet, C., Mano, M., and Eulalio, A. (2014). MicroRNAs in the interaction between host and bacterial pathogens. FEBS Lett. 588, 4140–4147. doi: 10.1016/j.febslet.2014.08.002
McGovern, D. P., Jones, M. R., Taylor, K. D., Marciante, K., Yan, X., Dubinsky, M., et al. (2010). Fucosyltransferase 2 (FUT2) non-secretor status is associated with Crohn's disease. Hum. Mol. Genet. 19, 3468–3476. doi: 10.1093/hmg/ddq248
Mezger, M., Steffens, M., Beyer, M., Manger, C., Eberle, J., Toliat, M. R., et al. (2008). Polymorphisms in the chemokine (C-X-C motif) ligand 10 are associated with invasive aspergillosis after allogeneic stem-cell transplantation and influence CXCL10 expression in monocyte-derived dendritic cells. Blood 111, 534–536. doi: 10.1182/blood-2007-05-090928
Middleton, P. G., Cullup, H., Dickinson, A. M., Norden, J., Jackson, G. H., Taylor, P. R., et al. (2002). Vitamin D receptor gene polymorphism associates with graft-versus-host disease and survival in HLA-matched sibling allogeneic bone marrow transplantation. Bone Marrow Transplant. 30, 223–228. doi: 10.1038/sj.bmt.1703629
Middleton, P. G., Norden, J., Cullup, H., Cavet, J., Jackson, G. H., Taylor, P. R., et al. (2003). Oestrogen receptor alpha gene polymorphism associates with occurrence of graft-versus-host disease and reduced survival in HLA-matched sib-allo BMT. Bone Marrow Transplant. 32, 41–47. doi: 10.1038/sj.bmt.1704090
Mikulska, M., Raiola, A. M., Galaverna, F., Balletto, E., Borghesi, M. L., Varaldo, R., et al. (2018). Pre-engraftment bloodstream infections after allogeneic hematopoietic cell transplantation: impact of T cell-replete transplantation from a haploidentical donor. Biol. Blood Marrow Transplant. 24, 109–118. doi: 10.1016/j.bbmt.2017.08.024
Moloney, G. M., Viola, M. F., Hoban, A. E., Dinan, T. G., and Cryan, J. F. (2018). Faecal microRNAs: indicators of imbalance at the host-microbe interface? Benef. Microbes 9, 175–183. doi: 10.3920/BM2017.0013
Montassier, E., Al-Ghalith, G. A., Ward, T., Corvec, S., Gastinne, T., Potel, G., et al. (2016). Pretreatment gut microbiome predicts chemotherapy-related bloodstream infection. Genome Med. 8:49. doi: 10.1186/s13073-016-0301-4
Nakata, K., Takami, A., Espinoza, J. L., Matsuo, K., Morishima, Y., Onizuka, M., et al. (2013). The recipient CXCL10 +1642C>G variation predicts survival outcomes after HLA fully matched unrelated bone marrow transplantation. Clin. Immunol. 146, 104–111. doi: 10.1016/j.clim.2012.11.009
Niederwieser, D., Baldomero, H., Szer, J., Gratwohl, M., Aljurf, M., Atsuta, Y., et al. (2016). Hematopoietic stem cell transplantation activity worldwide in 2012 and a SWOT analysis of the Worldwide Network for Blood and Marrow Transplantation Group including the global survey. Bone Marrow Transplant. 51, 778–785. doi: 10.1038/bmt.2016.18
Ogonek, J., Kralj Juric, M., Ghimire, S., Varanasi, P. R., Holler, E., Greinix, H., et al. (2016). Immune Reconstitution after Allogeneic Hematopoietic Stem Cell Transplantation. Front. Immunol. 7:507. doi: 10.3389/fimmu.2016.00507
Passweg, J. R., Baldomero, H., Bader, P., Bonini, C., Cesaro, S., Dreger, P., et al. (2016). Hematopoietic stem cell transplantation in Europe 2014: more than 40 000 transplants annually. Bone Marrow Transplant. 51, 786–792. doi: 10.1038/bmt.2016.20
Pineda-Tenor, D., Berenguer, J., Jiménez-Sousa, M. A., Guzmán-Fulgencio, M., Aldámiz-Echevarria, T., Carrero, A., et al. (2014). CXCL9, CXCL10 and CXCL11 polymorphisms are associated with sustained virologic response in HIV/HCV-coinfected patients. J. Clin. Virol. 61, 423–429. doi: 10.1016/j.jcv.2014.08.020
Plantinga, T. S., van der Velden, W. J., Ferwerda, B., van Spriel, A. B., Adema, G., Feuth, T., et al. (2009). Early stop polymorphism in human DECTIN-1 is associated with increased candida colonization in hematopoietic stem cell transplant recipients. Clin. Infect. Dis. 49, 724–732. doi: 10.1086/604714
Prestes, D. P., Mendes, C., Batista, M. V., Ramos, J. F., Junior, J. S., Okay, T. S., et al. (2018). A case-series of Toxoplasmosis in hematopoietic stem cell transplantation: still a concern for endemic countries. Bone Marrow Transplant. doi: 10.1038/s41409-018-0179-4. [Epub ahead of print].
Rayes, A., Morrow, A. L., Payton, L. R., Lake, K. E., Lane, A., and Davies, S. M. (2016). A genetic modifier of the gut microbiome influences the risk of graft-versus-host disease and bacteremia after hematopoietic stem cell transplantation. Biol. Blood Marrow Transplant. 22, 418–422. doi: 10.1016/j.bbmt.2015.11.017
Rocha, V., Franco, R. F., Porcher, R., Bittencourt, H., Silva, W. A., Latouche, A., et al. (2002). Host defense and inflammatory gene polymorphisms are associated with outcomes after HLA-identical sibling bone marrow transplantation. Blood 100, 3908–3918. doi: 10.1182/blood-2002-04-1033
Rothschild, D., Weissbrod, O., Barkan, E., Kurilshikov, A., Korem, T., Zeevi, D., et al. (2018). Environment dominates over host genetics in shaping human gut microbiota. Nature 555, 210–215. doi: 10.1038/nature25973
Sahin, U., Toprak, S. K., Atilla, P. A., Atilla, E., and Demirer, T. (2016). An overview of infectious complications after allogeneic hematopoietic stem cell transplantation. J. Infect. Chemother. 22, 505–514. doi: 10.1016/j.jiac.2016.05.006
Sato, K., Yoshimura, A., Kaneko, T., Ukai, T., Ozaki, Y., Nakamura, H., et al. (2012). A single nucleotide polymorphism in 3'-untranslated region contributes to the regulation of Toll-like receptor 4 translation. J. Biol. Chem. 287, 25163–25172. doi: 10.1074/jbc.M111.338426
Schuster, M. G., Cleveland, A. A., Dubberke, E. R., Kauffman, C. A., Avery, R. K., Husain, S., et al. (2017). Infections in hematopoietic cell transplant recipients: results from the organ transplant infection project, a multicenter, prospective, cohort study. Open Forum Infect. Dis. 4:ofx050. doi: 10.1093/ofid/ofx050
Skevaki, C., Pararas, M., Kostelidou, K., Tsakris, A., and Routsias, J. G. (2015). Single nucleotide polymorphisms of Toll-like receptors and susceptibility to infectious diseases. Clin. Exp. Immunol. 180, 165–177. doi: 10.1111/cei.12578
Staffas, A., Burgos da Silva, M., and van den Brink, M. R. M. (2017). The intestinal microbiota in allogeneic hematopoietic cell transplant and graft-versus-host disease. Blood. 129:2204. doi: 10.1182/blood-2017-03-771543
Stickel, N., Hanke, K., Marschner, D., Prinz, G., Köhler, M., Melchinger, W., et al. (2017). MicroRNA-146a reduces MHC-II expression via targeting JAK/STAT signaling in dendritic cells after stem cell transplantation. Leukemia 31, 2732–2741. doi: 10.1038/leu.2017.137
Tahara, T., Shibata, T., Wang, F., Yamashita, H., Hirata, I., and Arisawa, T. (2010). Genetic polymorphisms of molecules associated with innate immune responses, TRL2 and MBL2 genes in Japanese subjects with functional dyspepsia. J. Clin. Biochem. Nutr. 47, 217–223. doi: 10.3164/jcbn.10-40
Takami, A. (2013). Role of non-HLA gene polymorphisms in graft-versus-host disease. Int. J. Hematol. 98, 309–318. doi: 10.1007/s12185-013-1416-7
Taur, Y., Jenq, R. R., Perales, M. A., Littmann, E. R., Morjaria, S., Ling, L., et al. (2014). The effects of intestinal tract bacterial diversity on mortality following allogeneic hematopoietic stem cell transplantation. Blood 124, 1174–1182. doi: 10.1182/blood-2014-02-554725
Turpin, W., Bedrani, L., Espin-Garcia, O., Xu, W., Silverberg, M. S., Smith, M. I., et al. (2018). FUT2 genotype and secretory status are not associated with fecal microbial composition and inferred function in healthy subjects. Gut. Microbes. 1–12. doi: 10.1080/19490976.2018.1445956
Turpin, W., Espin-Garcia, O., Xu, W., Silverberg, M. S., Kevans, D., Smith, M. I., et al. (2016). Association of host genome with intestinal microbial composition in a large healthy cohort. Nat. Genet. 48, 1413–1417. doi: 10.1038/ng.3693
Uchino, K., Mizuno, S., Sato-Otsubo, A., Nannya, Y., Mizutani, M., Horio, T, et al. (2017). Toll-like receptor genetic variations in bone marrow transplantation. Oncotarget 8, 45670–45686. doi: 10.18632/oncotarget.17315
van den Brink, M. R., Velardi, E., and Perales, M. A. (2015). Immune reconstitution following stem cell transplantation. Hematology Am. Soc. Hematol. Educ. Program. 2015, 215–219. doi: 10.1182/asheducation-2015.1.215
van Well, G. T., Sanders, M. S., Ouburg, S., Kumar, V., van Furth, A. M., and Morré, S. A. (2013). Single nucleotide polymorphisms in pathogen recognition receptor genes are associated with susceptibility to meningococcal meningitis in a pediatric cohort. PLoS ONE 8:e64252. doi: 10.1371/journal.pone.0064252
Wacklin, P., Tuimala, J., Nikkilä, J., Sebastian Tims, Mäkivuokko, H., Alakulppi, N., et al. (2014). Faecal microbiota composition in adults is associated with the FUT2 gene determining the secretor status. PLoS ONE 9:e94863. doi: 10.1371/journal.pone.0094863
Wang, J., Thingholm, L. B., Skieceviciene, J., Rausch, P., Kummen, M., Hov, J. R., et al. (2016). Genome-wide association analysis identifies variation in vitamin D receptor and other host factors influencing the gut microbiota. Nat. Genet. 48, 1396–1406. doi: 10.1038/ng.3695
Webb, B. J., Brunner, A., Ford, C. D., Gazdik, M. A., Petersen, F. B., and Hoda, D. (2016). Fecal microbiota transplantation for recurrent Clostridium difficile infection in hematopoietic stem cell transplant recipients. Transpl. Infect. Dis. 18, 628–633. doi: 10.1111/tid.12550
Williams, M. R., Stedtfeld, R. D., Tiedje, J. M., and Hashsham, S. A. (2017). MicroRNAs-based inter-domain communication between the host and members of the gut microbiome. Front. Microbiol. 8:1896. doi: 10.3389/fmicb.2017.01896
Young, J. H., Logan, B. R., Wu, J., Wingard, J. R., Weisdorf, D. J., Mudrick, C., et al. (2016). Infections after transplantation of bone marrow or peripheral blood stem cells from unrelated donors. Biol Blood Marrow Transplant. 22, 359–370. doi: 10.1016/j.bbmt.2015.09.013
Keywords: stem cells transplantation, GVHD, SNP, cytokine storm, microbiota, invasive infection
Citation: Espinoza JL, Wadasaki Y and Takami A (2018) Infection Complications in Hematopoietic Stem Cells Transplant Recipients: Do Genetics Really Matter? Front. Microbiol. 9:2317. doi: 10.3389/fmicb.2018.02317
Received: 24 May 2018; Accepted: 11 September 2018;
Published: 02 October 2018.
Edited by:
John W. A. Rossen, University Medical Center Groningen, NetherlandsReviewed by:
Guido Moll, Charité Universitätsmedizin Berlin, GermanyBrian Duncan Tait, Australian Red Cross Blood Service, Australia
Copyright © 2018 Espinoza, Wadasaki and Takami. This is an open-access article distributed under the terms of the Creative Commons Attribution License (CC BY). The use, distribution or reproduction in other forums is permitted, provided the original author(s) and the copyright owner(s) are credited and that the original publication in this journal is cited, in accordance with accepted academic practice. No use, distribution or reproduction is permitted which does not comply with these terms.
*Correspondence: J. Luis Espinoza, luis@med.kindai.ac.jp