- Department of Plant Pathology, College of Agriculture and Natural Resources, National Chung-Hsing University, Taichung, Taiwan
Alternaria alternata relies on the ability to produce a host-selective toxin and to detoxify reactive oxygen species to successfully colonize the host. An A. alternata major facilitator superfamily transporter designated AaMFS54 was functionally characterized by analysis of loss- and gain-of-function mutations to better understand the factors required for fungal pathogenesis. AaMFS54 was originally identified from a wild-type expression library after being subtracted with that of a mutant impaired for the oxidative stress-responsive transcription regulator Yap1. AaMFS54 contains 14 transmembrane helixes. Fungal mutant lacking AaMFS54 produced fewer conidia and increased sensitivity to many potent oxidants (potassium superoxide and singlet-oxygen generating compounds), xenobiotics (2,3,5-triiodobenzoic acid and 2-chloro-5-hydroxypyridine), and fungicides (clotrimazole, fludioxonil, vinclozolin, and iprodione). AaMFS54 mutant induced necrotic lesion sizes similar to those induced by wild-type on leaves of susceptible citrus cultivars after point inoculation with spore suspensions. However, the mutant produced smaller colonies and less fluffy hyphae on the affected leaves. Virulence assays on citrus leaves inoculated by spraying with spores revealed that AaMFS54 mutant induced less severe lesions than wild-type, indicating the requirement of AaMFS54 in pathogenesis. All defective phenotypes were restored in a strain re-acquiring a functional copy of AaMFS54. Northern blotting analysis revealed that the expression of AaMFS54 was suppressed by xenobiotics. The current studies indicate that the Yap1-mediated transporter plays a role in resistance to toxic oxidants and fungicides in A. alternata. The relationships of MFS transporters with other regulatory components conferring stress resistance and A. alternata pathogenesis are also discussed.
Introduction
Alternaria species have been documented to cause diseases in more than 400 plant species, including many economically important crops: citrus, apple, rice, strawberry, pear, tomato, broccoli, cauliflower, carrot, potato, tobacco, as well as many ornamental and weed species (Thomma, 2003). A. alternata alone can infect nearly 100 plant species and many pathotypes display host specificity due to the ability to produce host selective toxins (HST). The production of HST by A. alternata is important for pathogenesis because HST-deficient mutants are unable to induce lesions on host plants (Hatta et al., 2002; Ito et al., 2004). There are two different A. alternata pathotypes affecting citrus. The rough lemon pathotype produces ACRL toxin, which is toxic only to lemon (C. jambhiri Lush) and Rangpur lime (C. x limonia Osbeck). The tangerine pathotype produces ACT toxin, which affects tangerines (C. reticulate Blanco), grapefruit (C. paradise Macfad.), and their hybrids (Akimitsu et al., 2003).
In addition to HST, A. alternata has to overcome toxic reactive oxygen species (ROS) produced by the host plant to successfully colonize and obtain nutrients from the affected tissue. Previous studies have demonstrated that the ability to detoxify ROS mediated by the stress-responsive transcription regulator Yap1, the mitogen-activated protein (MAP) kinase Hog1, and the stress response regulator Skn7 plays a critical role for pathogenesis of A. alternata on citrus (Lin et al., 2009; Yang et al., 2009; Lin and Chung, 2010; Chen et al., 2012). Yap1 is a transcriptional regulator containing the basic leucine zipper (bzip) domain and has been demonstrated to be required for resistance to ROS, multidrug, and heavy metals in fungi and yeasts (Alarco and Raymond, 1999; Toone and Jones, 1999; Toone et al., 2001). The tangerine pathotype of A. alternata impaired for Yap1, Hog1, or Skn7 increases sensitivity to ROS and multidrug and reduces virulence in citrus, confirming the importance of the ability to detoxify ROS in pathogenesis of A. alternata.
Many genes potentially regulated by Yap1 were identified from a cDNA library prepared from the tangerine pathotype after being subtracted with a Yap1 mutant cDNA. Of them, many genes encode putative transporters including major facilitator superfamily (MFS) and ATP-binding cassette (ABC) transporters (Lin et al., 2011). Fungal transporters play an important role in multidrug and fungicide resistance (Gulshan and Moye-Rowley, 2007; Coleman and Mylonakis, 2009; Wang et al., 2012; dos Santos et al., 2014; Wu et al., 2016). Analysis of loss- and gain-of-function mutations in an A. alternata MFS transporter designated AaMFS19 has allowed us to determine its functions to be associated with cellular resistance to oxidative stress and fungicides including clotrimazole, fludioxonil, and kocide (Chen et al., 2017). The expression of AaMFS19 is regulated by Yap1, Hog1, and Skn7.
To better understand the molecular mechanisms implicated in resistance to ROS and xenobiotics and fungal pathogenesis, the AaMFS54 encoding a 14-helix MFS transporter was functionally inactivated by targeted gene disruption in the tangerine pathotype of A. alternata. The results indicated that AaMFS19 and AaMFS54 transporters had shared and unique functions to minimize the toxicity of oxidative stress-generating chemicals and fungicides. Unlike AaMFS19, expression of the AaMFS54 gene was primarily regulated by Yap1 but not Hog1. The interplays among different regulators and proteins leading to stress resistance and A. alternata pathogenesis were also discussed.
Materials and Methods
Fungal Strains and Maintenance
The wild-type EV-MIL31 isolate of Alternaria alternata was isolated from a diseased Minneola tangelo (Lin et al., 2009) and used for genetic modifications. Fungal isolates mutated at Yap1 or Hog1 were generated in previous studies (Lin et al., 2009; Lin and Chung, 2010). The YCp strain was identified from a Yap1 null mutant after being transformed a functional copy of Yap1. Fungal isolates were cultured on potato dextrose agar (PDA) (Difco, Sparks, MD, United States) with a layer of sterile cellophane when DNA or RNA purification was needed. For preparation of fungal protoplasts, fungal isolates were cultured in 50 ml potato dextrose broth (PDB) for 4 to 5 days. For conidial formation, fungal isolates were cultured on PDA plates. The plates placed in a plastic box were incubated under fluorescent light for 4 to 5 days. After transformation, fungal strains were recovered from regeneration medium supplemented with 3.42 g/ml sucrose as an osmotic reagent (Chung et al., 2002).
Targeted Gene Replacement
A cDNA clone #54 (accession no. OWY53006.1) encoding an A. alternata major facilitator superfamily (AaMFS) was identified from a wild-type cDNA library after being subtracted with that of a Yap1 null mutant (Lin et al., 2011). AaMFS54 open reading frame (ORF) and its flanking sequence were obtained from the completed genome sequence of A. alternata (Wang et al., 2016). Fungal genomic DNA was isolated using a DNeasy Plant kit (Qiagen, Valencia, CA). A modified split marker approach (Chung and Lee, 2014) was used to delete genes in A. alternata by a bacterial phosphotransferase B gene (HYG) cassette under the control of the Aspergillus nidulans trpC gene promoter and terminator, conferring resistance to hygromycin as a selectable marker. To disrupt AaMFS54, shortened but partially overlapping HYG fragments M13R/hyg3 and hyg4/M13F were obtained by PCR and joined with 5′ and 3′ truncated AaMFS54 fragments (F2-M13R54 and M13F54-R2), respectively, by second-round PCR (Figure 1). DNA fragments were combined (1:1, v/v) and transformed into protoplasts prepared from wild-type using a CaCl2 and polyethylene glycol-mediated method as previously described (Chung et al., 2002). Fungal transformants were recovered from a regeneration medium amended with 250 μg/ml hygromycin (Roche Applied Science, Indianapolis, IN) and examined by PCR with hyg3/hyg4 primers and an AaMFS54-specific primer (R1) and hyg4 as indicated. The R1 primer whose sequence is not present in the split marker fragments was paired with the hyg4 primer to confirm for the occurrence of homologous integration within AaMFS54. This primer set would amplify a fragment only from fungal strains carrying the integration of HYG within AaMFS54. An expected 2.5-kb fragment was amplified with the primers hyg3 and hyg4 from genomic DNA prepared from six transformants, and no product was amplified from that of wild-type. An expected 3.3-kb fragment was amplified with the primers R1 and hyg4 from genomic DNA of three transformants (D18, D46, and D47), indicative of the successful deletion of AaMFS54. Disruption of AaMFS54 was further validated by Northern blot analysis (Figure 1). Oligonucleotide primers used for PCR amplification are listed in Supplementary Table S1.
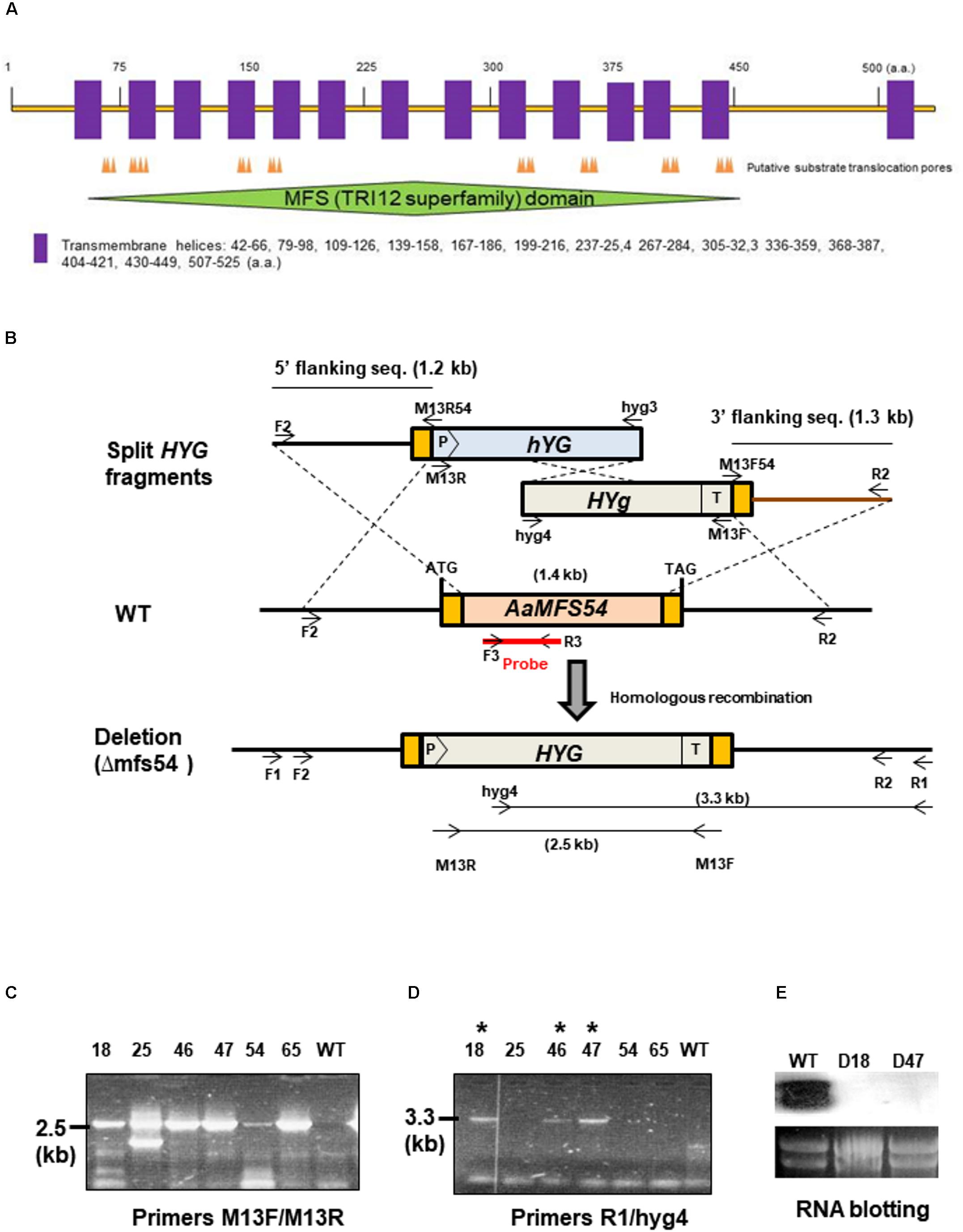
FIGURE 1. Targeted disruption of AaMFS54 in the tangerine pathotype of Alternaria alternata using a split marker approach. (A) Functional domains and transmembrane helices found in the AaMFS54. (B) Schematic illustration of generation of truncated but overlapping hygromycin phosphotransferase gene (HYG) under control by the Aspergillus nidulans trpC promoter (P) and terminator (T) within AaMFS54. Oligonucleotide primers used to amplify AaMFS54 fragments and to label the probe are indicated. (C) Amplification of DNA fragments from genomic DNA of wild type (WT) and transformants with the primer M13F and M13R. (D) Amplification of DNA fragments from genomic DNA of wild type (WT) and transformants with the primer R2 paired with hyg4, indicating that AaMFS54 is deleted in transformants D18, D46, and D47 (indicated by ∗). (E) Northern blot hybridization of fungal RNA with an AaMFS54-specific probe reveals the lack of the AaMFS54 gene transcripts in both D18 and D47 strains.
Genetic Complementation
Full-length AaMFS54 ORF and its promoter region were amplified with primers F1 and R1 and used for genetic complementation. The AaMFS54 fragment was co-transformed with the pBARKS1 carrying the BAR gene responsible for bialaphos resistance under control of the Aspergillus nidulans trpC promoter (Pall and Brunelli, 1993) into protoplasts prepared from the D18 mutant. Transformants were recovered from medium amended with 350 μg/ml bialaphos (Phytotechnology Lab., Lenexa, KS). Because fungal strains with AaMFS54 deficiency increased sensitivity to 0.5 μg/ml fludioxonil fungicide (see below for details), transformants were tested for restoration of cellular resistance to this fungicide.
Chemical Sensitivity Assays
Sensitivity assays to chemicals were carried out by transferring fungal mycelia as a toothpick point inoculation on PDA containing test compounds and incubated at 28°C. Colony radius was measured at 3 to 9 days. Each treatment contained four replicates, and all experiments were performed at least three times. The difference of radial growth of the disruption mutants was determined in relation to the wild-type grown on the same plate. Percentage change was calculated by dividing the relative difference of the growth by the wild-type growth and multiplying by 100.
Gene Expression
Northern-blot hybridization was conducted to examine the expression of AaMFS54 in the wild-type strain grown on PDA covered with a layer of cellophane for 3 days. Mycelium was harvested from medium treated with or without chemicals and used for RNA purification using a TriZol reagent (Invitrogen, Carlsbad, CA, United States). For Northern blot analysis, RNA was electrophoresed and denatured in a formaldehyde-containing agarose gel, blotted onto a nylon membrane, and hybridized to an AaMFS54 DNA probe according to the procedures described by Sambrook and Russell (2001). The probe was simultaneously amplified and labeled with a digoxigenin (DIG)-11-dUTP by PCR with the AaMFS54 gene-specific primers F3 and R3 (Supplementary Table S1). The probe (Figure 1) was identified by an immunological assay using disodium 3-(4-methoxyspiro [1,2-dioxetane-3,2′-(5′-chloro)tricyclo decan]-4-yl)phenyl phosphate (CSPD) as a chemofluorescent substrate for alkaline phosphatase according to the manufacturer’s recommendation (Roche Life Science, Penzberg, Germany). The first strand cDNA was synthesized by an M-MLV reverse transcriptase (Epicentre/Lucigen, Middleton, WI, United States) and used as a template for PCR amplification with gene-specific primers. PCR products were separated by a 1% agarose gel.
Virulence Assays
Fungal virulence was performed on excised calamondin (Citrus mitis Blanco) or Murcott (Citrus reticulata Blanco) leaves. Citrus leaves were inoculated by placing 5 μl of conidial suspension (104 conidia per ml) on each spot or sprayed to run-off with conidial suspensions using a min-sprayer. The inoculated leaves were kept in a plastic box for lesion formation. Each fungal strain was tested on at least five leaves, and experiments were repeated three times.
Statistical Analysis
The significance of treatments was determined by analysis of variance and means separated by student t-test (P < 0.05).
Results
Identification and Characterization of the MFS-Coding Gene
The A. alternata AaMFS54 gene was previously identified from the wild-type cDNA pool after being subtracted with that of a Yap1 mutant and was found to encode a MFS transporter. Sequence analysis revealed that AaMFS54 has a 1,614-bp open reading frame disrupted by four small introns that would encode a polypeptide of 538 amino acids after translation. AaMFS54 was found to contain several substrate translocation pores (Figure 1A). Hydropathy analysis revealed that AaMFS54 has 14 putative transmembrane domains. A MFS domain belonging to the fungal trichothecene efflux pump (TRI12) superfamily was also found in AaMFS54.
Targeted Gene Disruption
To investigate the functions of AaMFS54 transporter, a split marker approach was employed to delete AaMFS54 in the wild-type strain of A. alternata. Introduction of two truncated but overlapping HYG gene cassette fusing with 5′ or 3′ AaMFS54 fragment (Figure 1B) resulted in strains conferring resistance to hygromycin. Of six transformants recovered from hygromycin-containing medium, three had an expected deletion of AaMFS54 as evidenced by diagnostic PCR with a Hyg primer pairing with an AaMFS54 primer whose sequences were not present in the split marker fragments (Figures 1C,D). Only transformants carrying a successful integration of HYG within AaMFS54 would yield a product with the primer pairing with a HYG gene primer. Two mutant strains (designated Δmfs54 D18 and Δmfs54 D47) were used for further analyses. Northern blot hybridization (Figure 1E) of total RNA prepared from D18 and D47 with an AaMFGS54-specific probe failed to detect an expected AaMFS54 transcript as the wild-type, confirming that both D18 and D47 were AaMFS54 null mutants. Δmfs54 D18 and Δmfs54 D47 produced fewer conidia than wild-type (Figure 2A). Several transformants showing an increased resistance to 0.5 μg/ml fludioxonil were identified after transforming a copy of AaMFS54 into protoplasts prepared from Δmfs54-D18 (Figure 2B). PCR and RT-PCR analyses using AaMFS54-specific primers also confirmed successful complementation seen in recovered transformants (Figures 2C,D).
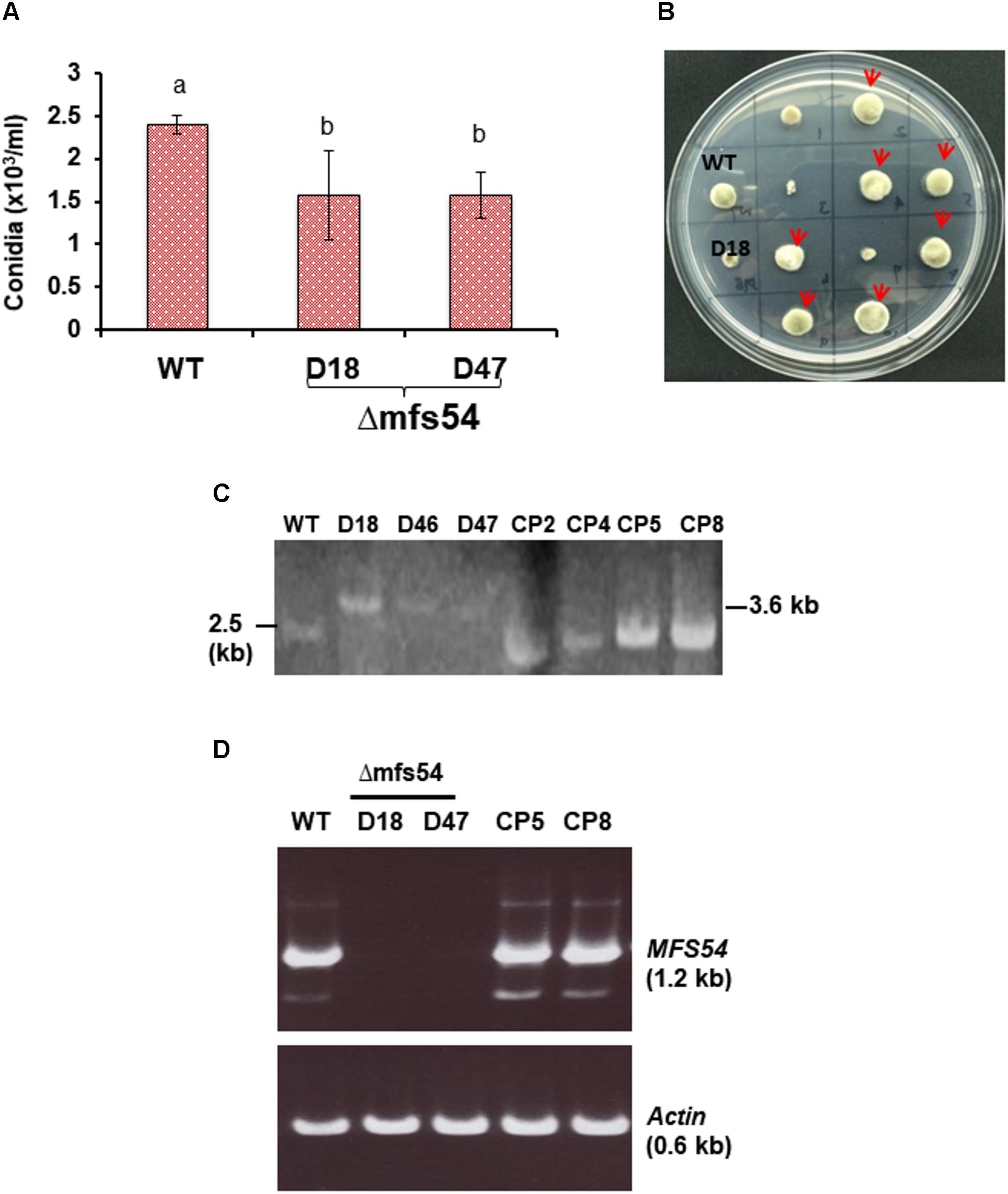
FIGURE 2. Phenotypic characterization of AaMFS54 strains. (A) Production of conidia by the wild-type (WT) and two AaMFS54 impaired mutants (Δmfs54-D18 and Δmfs54-D47) of A. alternata grown on PDA. Means followed by the same letters were not significantly different according to student t-test (P ≤ 0.05). (B) Growth of WT, D18 and fungal transformants from D18 protoplasts after being co-transformed with a copy of AaMFS54 and pBarks1 plasmid on PDA amended with 0.5 μg/ml fludioxonil 7 days. Several transformants (indicated by arrows) displayed WT growth, indicative of expression of AaMFS54. (C) Image of DNA fragments amplified from genomic DNA of WT, three Δmfs54 mutants (D18, D46, and D47) and four complementation strains (CP2, CP4, CP5, and CP8) with primers SSH54P and SSH54T. (D) Reverse transcriptase-PCR products from amplified from RNA samples isolated from A. alternata strains with SSH54QF1 and SSH54QR2 primers (top panel) and actin gene primers (bottom panel), indicating the expression of AaMFS54 in WT, CP5, and CP8. No product was amplified from RNA samples prepared from D18 and D47.
AaMFS54 Transporter Confers Resistance to Different Chemicals
Compared to wild-type, Δmfs54 reduced radial growth by ∼20% on potato dextrose agar plate. Δmfs54 increased sensitivity to xenobiotics [2,3,5-triiodobenzoic acid (TIBA) and 2-chloro-5-hydroxypyridine (CHP)], CuCl2 and several fungicides including clotrimazole, fludioxonil, vinclozolin, and iprodione (Figure 3). Although Δmfs54 colony diameter was not obviously different from untreated control on medium amended with CHP, the mutant strains apparently produced thinner colonies. Δmfs54 was more sensitive to potassium superoxide (KO2) and singlet oxygen-generating compound hematoporphyrin than wild-type. However, Δmfs54 showed no increased sensitivity on medium containing H2O2, singlet oxygen-generating compounds (rose Bengal and eosin Y), or a cell wall disturbing compound Congo red. Transformation of a functional copy of AaMFS54 into protoplasts prepared from Δmfs54-D18 yielded a Cp8 strain, which displayed wild-type resistance to all test chemicals.
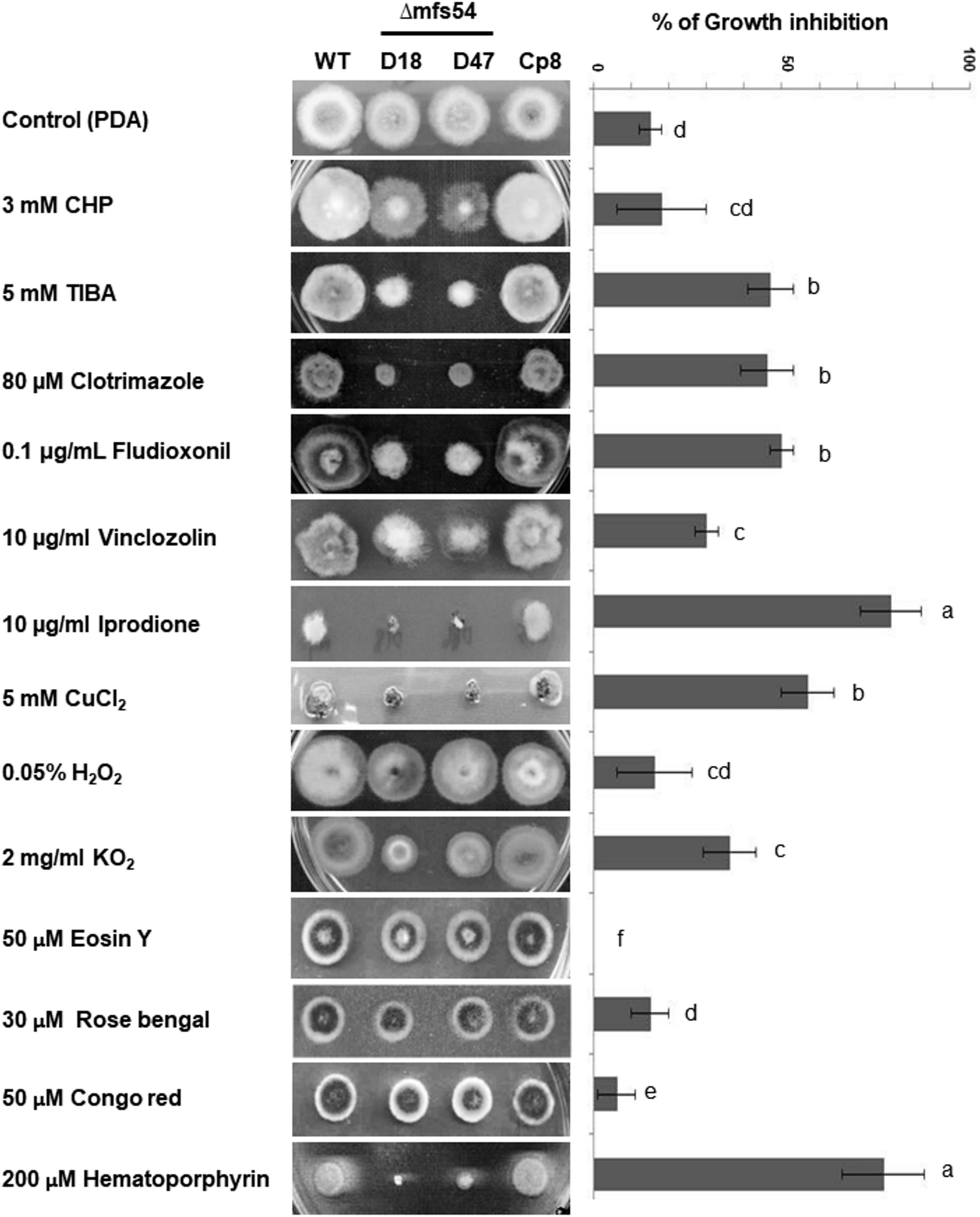
FIGURE 3. The Alternaria alternata major facilitator superfamily transporter (AaMFS54) is required for resistance to xenobiotics and fungicides. Image of the wild-type (WT), two Δmfs54 deletion mutants (D18 and D47), and the CP8 complementation strain grown on potato dextrose agar (PDA) amended with indicated chemicals (left panel). Only representatives are shown. Quantitative analysis of chemical sensitivity is shown on the right panel. Growth reduction was calculated as a cumulative percentage of growth of wild-type and Δmfs54 strains grown on the same plate. The data presented are the mean and standard deviation of two independent experiments with four replicates. Abbreviations: CHP, 2-chloro-5-hydroxypyridine; TIBA, 2,3,5-triiodobenzoic acid. Means followed by the same letters were not significantly different according to student t-test (P ≤ 0.05).
AaMFS54 Is Required for Fungal Virulence
Virulence assays performed on detached calamondin or Murcott leaves inoculated by placing conidial suspensions on the leaves revealed that Δmfs54 induced necrotic lesions comparable to wild-type (Figure 4A). However, both Δmfs54 strains produced much smaller colonies and less fluffy hyphae than wild-type and Cp8 strain on affected leaves 4 days post inoculation (Figure 4B). To mimic natural infection, virulence assays were also conducted on calamondin leaves inoculated by uniformly spraying with conidial suspensions. The results revealed that Δmfs54 induced less severe lesions than wild-type (Figure 4C).
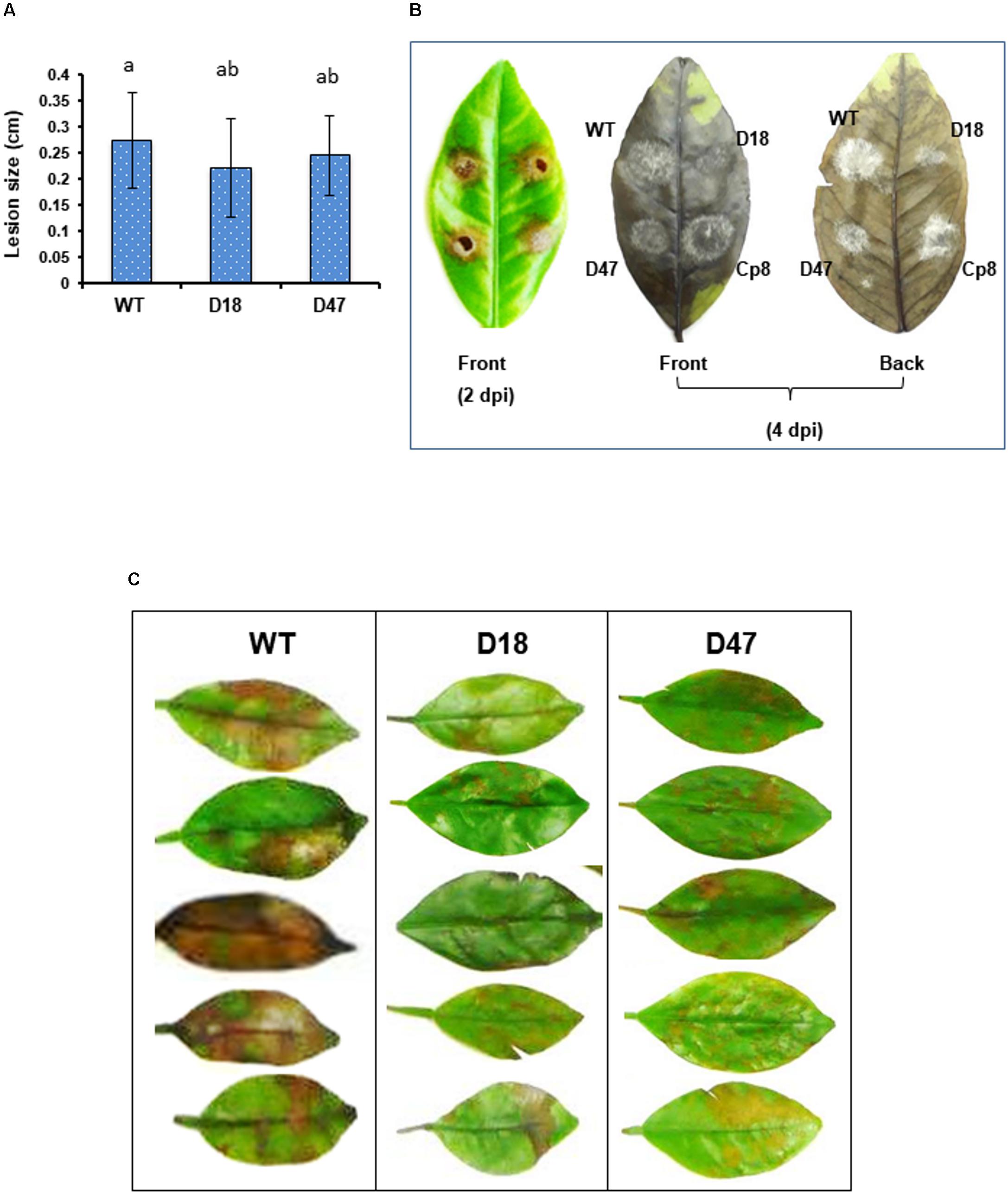
FIGURE 4. The A. alternata major facilitator superfamily transporter (AaMFS54) is a virulence determinant. (A) Conidial suspensions (104 conidia per ml) prepared from WT and Δmfs54 strains were inoculated by dropping 5 μl on detached calamondin leaves (n = 8). The diameter of lesions was measured 2 days post inoculation (dpi). Means followed by the same letters were not significantly different according to student t-test (P ≤ 0.05). (B) Formation of necrotic lesions (2 dpi) and fungal colonies and fluffy hyphae on necrotic lesions (4 dpi). (C) Development of necrotic lesions on detached calamondin leaves inoculated by spraying with conidial suspensions 2 dpi. The mock controls were treated with water only. The leaves were maintained in a moisture chamber for lesion development. Only representatives are shown.
Differential Expression of AaMFS54
Northern blotting revealed that the expression of AaMFS54 was down-regulated in the wild-type grown on a medium containing TIBA or CHP (Figure 5A). The expression of AaMFS54 was induced in the wild-type grown on medium amended with H2O2, clotrimazole, or vinclozolin. Northern blotting revealed further that the accumulation of the AaMFS54 transcript decreased in fungal strains defective for the stress-responsive transcription regulator Yap1 (Figure 5B). The level of the AaMFS54 transcript in the complementation strain YCp expressing a functional copy of Yap1 was similar to that of the wild-type. However, the expression of AaMFS54 was not affected in two fungal strains with the defective Hog1 MAP kinase.
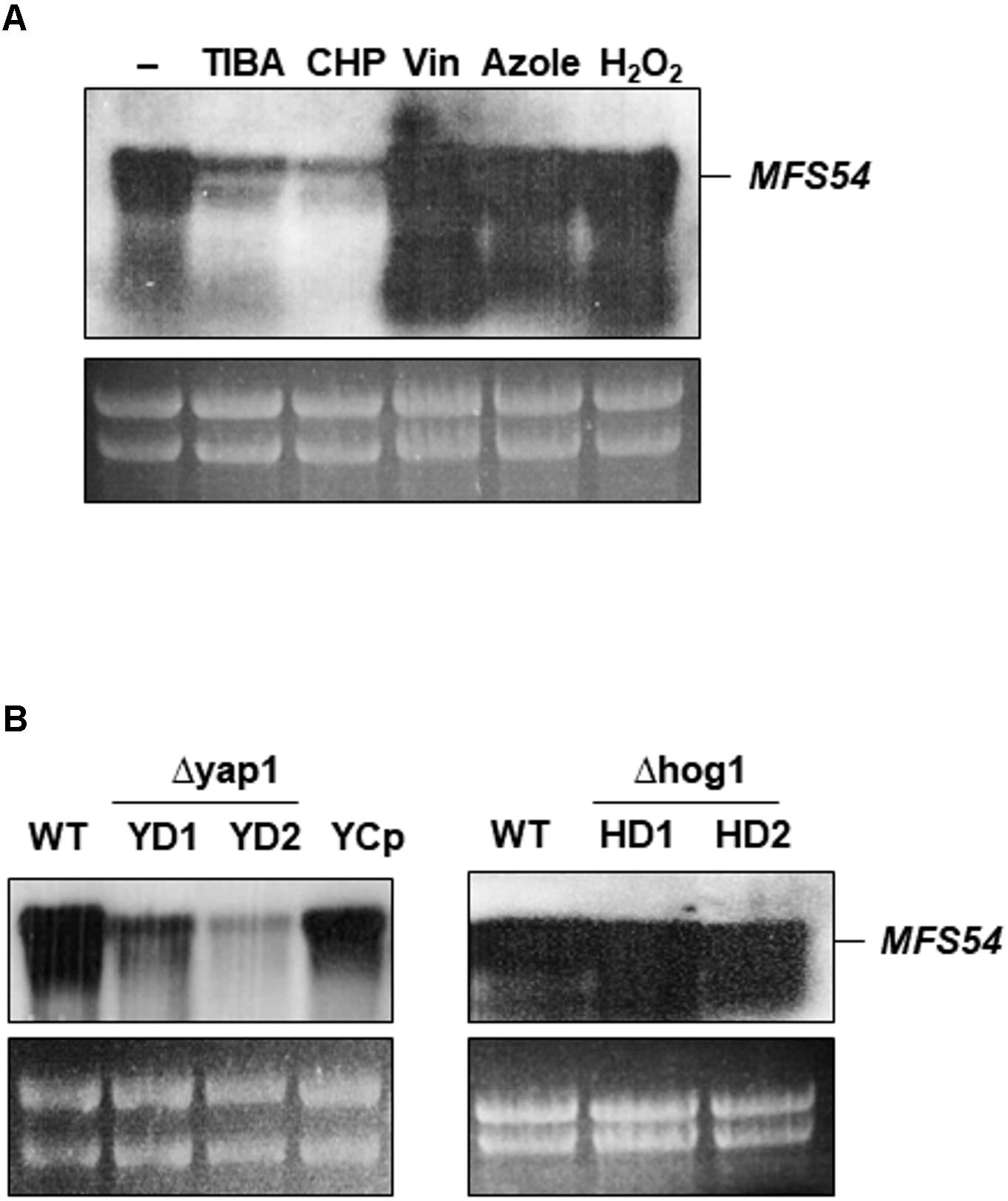
FIGURE 5. Expression of the AaMFS54 gene by Northern blot analysis. (A) RNA was purified from wild-type (WT) grown on PDA with or without chemicals. Abbreviations: TIBA, 2,3,5-triiodobenzoic acid; CHP, 2-chloro-5-hydroxypyridine; Vin, vinclozolin; and Azole, clotrimazole. (B) Detection of the AaMFS54 transcript in WT, two Δyap1 strains (YD1 and YD2) impaired at the Yap1 transcription regulator, the YCp strain re-acquiring a functional Yap1 gene, and two Δhog1 strains (HD1 and HD2) impaired at the Hog1 MAP kinase. RNA was electrophoresed on a formaldehyde-containing gel, blotted to membrane, and probed with an AaMFS54-specific probe (Figure 1B). Ribosomal RNA after being stained with ethidium bromide is used to indicate the loading of the samples.
Discussion
Fungal transporters are involved in cellular resistance to numerous chemicals, metal ions, molecules, and drugs. Investigation of A. alternata molecular determinants of H2O2 resistance has allowed us to identify several genes coding for membrane transporters (Lin et al., 2011). We have previously characterized an A. alternata major facilitator superfamily transporter 19 (AaMFS19) to be required for resistance to a wide range of oxidants, fungicides, and xenobiotics (Chen et al., 2017). AaMFS19, containing 12 transmembrane domains, also plays a role in fungal virulence. In the present study, we report functional characterization of second MFS transporter designated AaMFS54 to determine its role in cellular resistance to various chemicals, fungicides, and in fungal pathogenesis.
AaMFS54 protein contains 14 transmembrane domains. Phenotypic analysis of fungal mutants carrying an AaMFS54 deficiency reveals that AaMFS54 is required for resistance to a great diversity of chemicals, including 2,3,5-triiodobenzoic acid (TIBA) and 2-chloro-5-hydroxypyridine (CHP), clotrimazole, fludioxonil, vinclozolin, iprodione, CuCl2, KO2, and hematoporphyrin. Previous studies have shown that fungal mutant strains defective for AaMFS19 containing 12 transmembrane domains are also hypersensitive to those fungicides and chemicals (Chen et al., 2017). CHP is a potent oxidant because it could react with H2O2 to produce superoxide and hydroxyl radicals in the presence of Cu2+ (Watanabe et al., 1998; Nerud et al., 2001). Both CHP and TIBA have been demonstrated to be toxic to A. alternata, suppressing gene expression, causing the formation of shorter hyphal branches, reducing growth, and suppressing conidial formation and germination (Chen et al., 2017). Both AaMFS19 and AaMFS54 are involved in resistance to CHP and TIBA, even though expression of the two coding genes is down-regulated in A. alternata strain treated with CHP or TIBA. The A. alternata mutants carrying AaMFS19 or AaMFS54 deficiency display increased sensitivity to fludioxonil, vinclozolin, iprodione, and clotrimazole fungicides, confirming further the important role of MFS transporters in multidrug resistance and stress adaptation in fungi (Del Sorbo et al., 2000; Hayashi et al., 2002; Roohparvar et al., 2007, 2008; Costa et al., 2014; Paul and Moye-Rowley, 2014; Xu et al., 2014; Redhu et al., 2016).
Both AaMFS19 and AaMFS54 coding sequences were originally identified from a cDNA library prepared from the wild-type strain of A. alternata after subtraction with cDNA prepared from a Yap1 mutant (Lin et al., 2011). Northern blot hybridization confirms that accumulation of the AaMFS19 and AaMFS54 transcripts is coordinately regulated by the stress responsive transcription factor Yap1 (Chen et al., 2017 and this study). However, expression of AaMFS19 but not AaMFS54 is regulated by the stress-related regulators Hog1 and Skn7, supporting that AaMFS19 and AaMFS54 have divergent functions. Moreover, because Yap1, Hog1, and Skn7 regulators have been demonstrated to play a critical role in resistance to oxidative stress (Chung, 2014), it appears that ROS resistance in A. alternata is, at least in part, mediated by membrane-bound transporters. However, it is unlikely that MFS transporters are specifically functioning at ROS because functional analysis reveals that AaMFS19 and AaMFS54 are not required for resistance to H2O2 or many ROS-generating oxidants. For example: AaMFS54 mutant strains are hypersensitive to hematoporphyrin, but not eosin Y and rose Bengal, even though all could react with O2 to produce toxic singlet oxygen upon exposure to light (Daub et al., 2013). AaMFS54 mutant strains also display wild-type sensitivity to singlet oxygen-generating compounds, toluidine blue (200 μM), methylene blue (200 μM), cercosporin (50 μM), and elsinochromes (30 μM) (data not shown). In contrast, AaMFS19 mutant strains increased sensitivity to eosin Y, rose Bengal, hematoporphyrin, methylene blue, and cercosporin. The results clearly indicate that AaMFS19 and AaMFS54 transporters have shared and unique substrate preferences and functions to mitigate the toxicity of oxidative stress-generating chemicals. Expression of the MFS transporter coding genes in the budding yeast Saccharomyces cerevisiae is known to be regulated by Yap1 and other stress-related transcription factors (dos Santos et al., 2014). MFS transporters could function directly to avoid the toxicity of chemicals by pumping them out of the cell. MFS transporters could also act indirectly against toxic chemicals or oxidants by altering metabolic regulation or by changing the plasma membrane compositions (Coleman and Mylonakis, 2009; Dhaoui et al., 2011; Krüger et al., 2013; Rios et al., 2013).
Pathogenicity tests conducted on detached calamondin or Murcott leaves inoculated by placing conidial suspensions (5 μl, 104 conidia per ml) reveal no significant differences in lesion diameters induced by the wild-type and the AaMFS54 mutant. The AaMFS54 mutant reduced growth and produced much smaller colonies and less fluffy hyphae than wild-type. Pathogenicity assays on citrus leaves inoculated by spraying with conidial suspensions (mimicking natural infection) reveal that the AaMFS54 mutant induced less severe lesions than wild-type. Similar results were also observed in the fungal mutant impaired for AaMFS19 (Chen et al., 2017). The discrepancy between point and spray inoculations is likely due to the concentrations of conidia present in the infection court.
Current and previous studies have begun to shed a light on the interplays among different regulators and proteins required for stress resistance and fungal pathogenicity/virulence of A. alternata (Figure 6). A low level of H2O2 generated by the NADPH oxidase (Nox) likely activates Yap1, Hog1, and Skn7 at transcriptional and/or post-translational levels, which could subsequently enhance siderophore biosynthesis foriron uptake and storage and promote antioxidant activities including superoxide dismutase (SOD), catalase, and peroxidase,all leading to oxidative stress resistance (Chen et al., 2013, 2014). Yap1, Hog1, and Skn7 could also activate gluataredoxin and thioredoxin systems to cope with oxidative stress (Yang et al., 2016; Ma et al., 2018). Because H2O2 is toxic to cells, Yap1, Hog1, and Skn7 would suppress the expression of the Nox genes (transcriptional feedback inhibition) to avoid the unrestraint production of H2O2. In addition to oxidative stress, Yap1, Hog1, and Skn7 are involved in the adaptation to osmolytes, xenobiotics, or fungicides. These functions are in part mediated by the membrane-bound MFS transporters. All of these components would allow A. alternata to deal with environmental stress and enhance its ability to colonize the host plant.
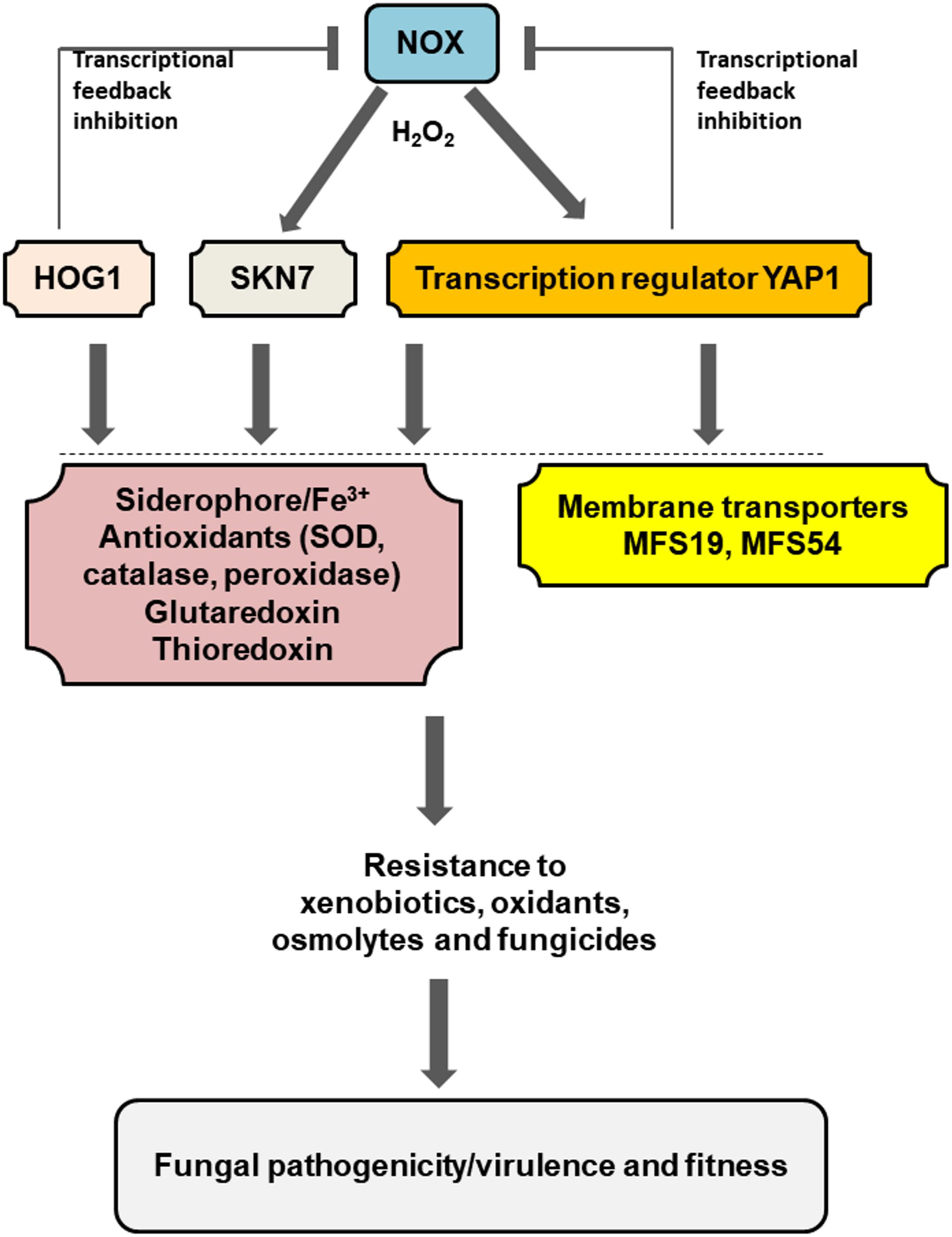
FIGURE 6. Schematic illustration of a regulation network associated with pathogenesis and fitness in the tangerine pathotype of A. alternata. Low level of H2O2 generated by the NADPH oxidases (NOX) plays a central role in the activation of Yap1, Hog1, and Skn7, which subsequently regulate the expression of numerous genes associated with iron uptake and resistance to different stress including oxidants xenobiotics, fungicides, and osmolytes. Two MFS transporters regulated by Yap1 and/or Hog1 play an important role in the process. Transcriptional feedback inhibition of the Nox-coding genes by Yap1 and Hog1 was also observed. Detailed description can be found in the text.
Author Contributions
K-RC designed the experiments and wrote the main manuscript text. H-CL, P-LY, L-HC, and H-CT performed the experiments and acquired the data. H-CL, P-LY, L-HC, H-CT, and K-RC analyzed and interpreted the data. All authors reviewed the manuscript.
Funding
This research was supported by grants from the Ministry of Science and Technology of Taiwan (Grant Nos. MOST105-2313-B-005-010-MY3 and MOST106-2313-B-005-025-MY3) to K-RC.
Conflict of Interest Statement
The authors declare that the research was conducted in the absence of any commercial or financial relationships that could be construed as a potential conflict of interest.
Supplementary Material
The Supplementary Material for this article can be found online at: https://www.frontiersin.org/articles/10.3389/fmicb.2018.02229/full#supplementary-material
References
Akimitsu, K., Peever, T. L., and Timmer, L. W. (2003). Molecular, ecological and evolutionary approaches to understanding Alternaria diseases of citrus. Mol. Plant Pathol. 4, 435–446. doi: 10.1046/j.1364-3703.2003.00189.x
Alarco, A., and Raymond, M. (1999). The bZip transcription factor Cap1p is involved in multidrug resistance and oxidative stress response. J. Bacteriol. 181, 700–708.
Chen, L. H., Lin, C. H., and Chung, K. R. (2012). Roles for SKN7 response regulator in stress resistance, conidiation and virulence in the citrus pathogen Alternaria alternata. Fungal Genet. Biol. 49, 802–813. doi: 10.1016/j.fgb.2012.07.006
Chen, L. H., Lin, C. H., and Chung, K. R. (2013). A nonribosomal peptide synthetase mediates siderophore production and virulence in the citrus fungal pathogen Alternaria alternata. Mol. Plant Pathol. 14, 497–505. doi: 10.1111/mpp.12021
Chen, L. H., Tsai, H. C., and Chung, K. R. (2017). A major facilitator superfamily transporter-mediated resistance to oxidative stress and fungicides requires Yap1, Skn7, and MAP kinases in the citrus fungal pathogen Alternaria alternata. PLoS One 12:e0169103. doi: 10.1371/journal.pone.0169103
Chen, L. H., Yang, S. L., and Chung, K. R. (2014). Resistance to oxidative stress via regulating siderophore-mediated iron-acquisition by the citrus fungal pathogen Alternaria alternata. Microbiology 160, 970–979. doi: 10.1099/mic.0.076182-0
Chung, K. R. (2014). Reactive oxygen species in the citrus fungal pathogen Alternaria alternata: the roles of NADPH oxidase. Physiol. Mol. Plant Pathol. 88, 10–17. doi: 10.1016/j.pmpp.2014.08.001
Chung, K. R., and Lee, M. H. (2014). “Split marker-mediated transformation and targeted gene disruption in filamentous fungi,” in Genetic Transformation Systems in Fungi, Vol. 2, eds M. A. van den Berg and K. Maruthachalam (Berlin: Springer International Publishing), 175–180.
Chung, K. R., Shilts, T., Li, W., and Timmer, L. W. (2002). Engineering a genetic transformation system for Colletotrichum acutatum, the causal fungus of lime anthracnose and postbloom fruit drop. FEMS Microbiol. Lett. 213, 33–39. doi: 10.1111/j.1574-6968.2002.tb11282.x
Coleman, J. J., and Mylonakis, E. (2009). Efflux in fungi: la Pièce de Résistance. PLoS Pathog. 5:e1000486. doi: 10.1371/journal.ppat.1000486
Costa, C., Dias, P. J., Sá-Correia, I., and Teixeira, M. C. (2014). MFS multidrug transporters in pathogenic fungi: do they have real clinical impact? Front. Physiol. 5:197. doi: 10.3389/fphys.2014.00197
Daub, M. E., Herrero, S., and Chung, K. R. (2013). Reactive oxygen species in plant pathogenesis: the role of perylenequinone photosensitizers. Antioxid. Redox Signal. 19, 970–989. doi: 10.1089/ars.2012.5080
Del Sorbo, G., Schoonbeek, H., and De Waard, M. A. (2000). Fungal transporters involved in efflux of natural toxic compounds and fungicides. Fungal Genet. Biol. 30, 1–15. doi: 10.1006/fgbi.2000.1206
Dhaoui, M., Auchere, F., Blaiseau, P. L., Lesuisse, E., Landoulsi, A., Camadro, J. M., et al. (2011). Gex1 is a yeast glutathione exchanger that interferes with pH and redox homeostasis. Mol. Biol. Cell 22, 2054–2067. doi: 10.1091/mbc.E10-11-0906
dos Santos, S. C., Teixeira, M. C., Dias, P. J., and Sá-Correia, I. (2014). MFS transporter required for multidrug/multixenobiotic (MD/MX) resistance in the model yeast: understanding their physiological function through post-genomic approaches. Front. Physiol. 5:180. doi: 10.3389/fphys.2014.00180
Gulshan, K., and Moye-Rowley, W. S. (2007). Multidrug resistance in fungi. Eukaryot. Cell 6, 1933–1942. doi: 10.1128/EC.00254-07
Hatta, R., Ito, K., Hosaki, Y., Tanaka, T., Tanaka, A., Yamamoto, M., et al. (2002). The conditionally dispensable chromosome controls host-specific pathogenicity in the fungal plant pathogen Alternaria alternata. Genetics 161, 59–70.
Hayashi, K., Schoonbeek, H., and De Waard, M. A. (2002). Bcmfs1, a novel major facilitator superfamily transporter from Botrytis cinerea, provides tolerance towards the natural toxic compounds camptothecin and cercosporin and towards fungicides. Appl. Environ. Microbiol. 68, 4996–5004. doi: 10.1128/AEM.68.10.4996-5004.2002
Ito, K., Tanaka, T., Hatta, R., Yamamoto, M., Akimitsu, K., and Tsuge, T. (2004). Dissection of the host range of the fungal plant pathogen Alternaria alternata by modification of secondary metabolism. Mol. Microbiol. 52, 399–411. doi: 10.1111/j.1365-2958.2004.04004.x
Krüger, A., Vowinckel, J., Mulleder, M., Grote, P., Capuano, F., Bluemlein, K., et al. (2013). Tpo1-mediated spermine and spermidine export controls cell cycle delay and times antioxidant protein expression during oxidative stress response. EMBO Rep. 14, 1113–1119. doi: 10.1038/embor.2013.165
Lin, C. H., and Chung, K. R. (2010). Specialized and shared functions of the histidine kinase- and HOG1 MAP kinase-mediated signaling pathways in Alternaria alternata, a filamentous fungal pathogen of citrus. Fungal Genet. Biol. 47, 818–827. doi: 10.1016/j.fgb.2010.06.009
Lin, C. H., Yang, S. L., and Chung, K. R. (2009). The YAP1 homolog-mediated oxidative stress tolerance is crucial for pathogenicity of the necrotrophic fungus Alternaria alternata in citrus. Mol. Plant Microbe Interact. 22, 942–952. doi: 10.1094/MPMI-22-8-0942
Lin, C. H., Yang, S. L., and Chung, K. R. (2011). Cellular responses required for oxidative stress tolerance, colonization and lesion formation by the necrotrophic fungus Alternaria alternata in citrus. Curr. Microbiol. 62, 807–815. doi: 10.1007/s00284-010-9795-y
Ma, H., Wang, M., Gai, Y., Fu, H., Ruan, R., Chung, K. R., et al. (2018). Thioredoxin and glutaredoxin systems required for oxidative stress resistance, fungicide sensitivity and virulence of Alternaria alternata. Appl. Environ. Microbiol. 84:e00086-18. doi: 10.1128/AEM.00086-18
Nerud, F., Baldrian, P., Gabriel, J., and Ogbeifun, D. (2001). Decolorization of synthetic dyes by the fenton reagent and the Cu/pyridine/H2O2 system. Chemosphere 44, 957–961. doi: 10.1016/S0045-6535(00)00482-3
Pall, M. L., and Brunelli, J. P. (1993). A series of six compact fungal transformation vectors containing polylinkers with multiple unique restriction sites. Fungal Genet. Newslett. 40, 59–62. doi: 10.4148/1941-4765.1413
Paul, S., and Moye-Rowley, W. S. (2014). Multidrug resistance in fungi: regulation of transporter-encoding gene expression. Front. Physiol. 5:143. doi: 10.3389/fphys.2014.00143
Redhu, A. K., Shah, A. H., and Prasad, R. (2016). MFS transporters of Candida species and their role in clinical drug resistance. FEMS Yeast Res. 16:fow043. doi: 10.1093/femsyr/fow043
Rios, G., Cabedo, M., Rull, B., Yenush, L., Serrano, R., and Mulet, J. M. (2013). Role of the yeast multidrug transporter Qdr2 in cation homeostasis and the oxidative stress response. FEMS Yeast Res. 13, 97–106. doi: 10.1111/1567-1364.12013
Roohparvar, R., De Waard, M. A., Kema, G. H., and Zwiers, L. H. (2007). MgMfs1, a major facilitator superfamily transporter from the fungal wheat pathogen Mycosphaerella graminicola, is a strong protectant against natural toxic compounds and fungicides. Fungal Genet. Biol. 44, 378–388. doi: 10.1016/j.fgb.2006.09.007
Roohparvar, R., Mehrabi, R., Van Nistelrooy, J. G., Zwiers, L. H., and De Waard, M. A. (2008). The drug transporter MgMfs1 can modulate sensitivity of field strains of the fungal wheat pathogen Mycosphaerella graminicola to the strobilurin fungicide trifloxystrobin. Pest Manag. Sci. 64, 685–693. doi: 10.1002/ps.1569
Sambrook, J., and Russell, D. W. (2001). Molecular Cloning: A Laboratory Manual, 3rd Edn. New York, NY: Cold Spring Harbor Press.
Thomma, B. P. (2003). Alternaria spp: from general saprophyte to specific parasite. Mol. Plant Pathol. 4, 225–236. doi: 10.1046/j.1364-3703.2003.00173.x
Toone, W. M., and Jones, N. (1999). AP-1 transcription factors in yeast. Curr. Opin. Genet. Dev. 9, 55–61. doi: 10.1016/S0959-437X(99)80008-2
Toone, W. M., Morgan, B. A., and Jones, N. (2001). Redox control of AP-1-like factors in yeast and beyond. Oncogene 20, 2336–2346. doi: 10.1038/sj.onc.1204384
Wang, J., Sun, X., Lin, L., Zhang, T., Ma, Z., and Li, H. (2012). PdMfs1, a major facilitator superfamily transporter from Penicillium digitatum, is partially involved in the imazalil-resistance and pathogenicity. Afr. J. Microbiol. Res. 6, 95–105.
Wang, M., Sun, X., Yu, D., Xu, J., Chung, K. R., and Li, H. (2016). Genomic and transcriptomic analyses of the tangerine pathotype of Alternaria alternata in response to oxidative stress. Sci. Rep. 6:32437. doi: 10.1038/srep32437
Watanabe, T., Koller, K., and Messner, K. (1998). Copper-dependent depolymerisation of lignin in the presence of fungal metabolite, pyridine. J. Biotechnol. 62, 221–230. doi: 10.1016/S0168-1656(98)00063-7
Wu, Z., Wang, S., Yuan, Y., Zhang, T., Liu, J., and Liu, D. (2016). A novel major facilitator superfamily transporter in Penicillium digitatum (PdMFS2) is required for prochloraz resistance, conidiation and full virulence. Biotechnol. Lett. 38, 1349–1357. doi: 10.1007/s10529-016-2113-4
Xu, X., Chen, J., Xu, H., and Li, D. (2014). Role of a major facilitator superfamily transporter in adaptation capacity of Penicillium funiculosum under extreme acidic stress. Fungal Genet. Biol. 69, 75–83. doi: 10.1016/j.fgb.2014.06.002
Yang, S. L., Lin, C. H., and Chung, K. R. (2009). Coordinate control of oxidative stress, vegetative growth and fungal pathogenicity via the AP1-mediated pathway in the rough lemon pathotype of Alternaria alternata. Physiol. Mol. Plant Pathol. 74, 100–110. doi: 10.1016/j.pmpp.2009.09.007
Keywords: citrus, efflux, reactive oxygen species, pathogen, virulence
Citation: Lin H-C, Yu P-L, Chen L-H, Tsai H-C and Chung K-R (2018) A Major Facilitator Superfamily Transporter Regulated by the Stress-Responsive Transcription Factor Yap1 Is Required for Resistance to Fungicides, Xenobiotics, and Oxidants and Full Virulence in Alternaria alternata. Front. Microbiol. 9:2229. doi: 10.3389/fmicb.2018.02229
Received: 14 April 2018; Accepted: 31 August 2018;
Published: 18 September 2018.
Edited by:
Jens Staal, Ghent University, BelgiumReviewed by:
Humberto Prieto, Instituto de Investigaciones Agropecuarias (INIA), ChileOswaldo Valdes-Lopez, Universidad Nacional Autónoma de México, Mexico
Copyright © 2018 Lin, Yu, Chen, Tsai and Chung. This is an open-access article distributed under the terms of the Creative Commons Attribution License (CC BY). The use, distribution or reproduction in other forums is permitted, provided the original author(s) and the copyright owner(s) are credited and that the original publication in this journal is cited, in accordance with accepted academic practice. No use, distribution or reproduction is permitted which does not comply with these terms.
*Correspondence: Kuang-Ren Chung, krchung@nchu.edu.tw
†Present address: Pei-Ling Yu, Department of Plant Pathology, University of Florida, Gainesville, FL, United States; Li-Hung Chen, Department of Plant Pathology, University of California, Davis, Davis, CA, United States; Hsieh-Chin Tsai, School of Food Science and Human Nutrition, Washington State University, Pullman, WA, United States