- State Key Laboratory of Urban Water Resources and Environment, School of Environment, Harbin Institute of Technology, Harbin, China
Quorum sensing (QS) is a central mechanism for regulating bacterial social networks in biofilm via the production of diffusible signal molecules (autoinducers). In this work, we assess the contribution of QS autoinducers to microbial extracellular electron transfer (EET) by Pseudomonas aeruginosa strain PAO1 and three mutants pure culture-inoculated in microbial electrolysis cells (MECs) and microbial fuel cells (MFCs). MECs inoculated with different P. aeruginosa strains showed a difference in current generation. All MFCs reached a reproducible cycle of current generation, and PQS-deficient pqsA mutant inoculated-MFCs obtained a much higher current generation than pqsL mutant inoculated-MFCs which overproduced PQS. lasIrhlI-inoculated MFCs produced a lower power output than others, as the strain was deficient in rhl and las. Exogenous N-butanoyl-l-homoserine lactone could remedy the electricity production by lasIrhlI mutants to a level similar to wild-type strains while signaling molecules had little effect on wild-type bacteria in MFCs. Meanwhile, experiments with the wild-type and pqsA, pqsL mutants indicated that the overexpression of PQS signaling molecules made no significant contribution to EET. QS signaling molecules therefore have dual-edged effects on microbial EET. These findings will provide favorable suggestions on the regulation of EET, but detailed QS regulatory mechanisms for extracellular electron transfer in pure- and mixed-cultures are yet to be elucidated.
Introduction
Microbial electrochemical systems (MESs) are a versatile group of technologies with the potential to achieve sustainable bioenergy generation, biosensing and bioelectrosynthesis using organic or inorganic carbon sources (Liu et al., 2016). Electroactive bacteria (EAB) function as biocatalysts and are able to exchange electrons between cells and electrodes via multiple processes of extracellular electron transfer (Bond and Lovley, 2003; Rabaey et al., 2004; Reguera et al., 2005). The optimal reactor configurations, operating conditions and electrode materials for increased electron transfer in MES have been described previously (Rinaldi et al., 2008; Karthikeyan et al., 2015; Logan et al., 2015; Mei et al., 2015; Xing et al., 2015; Zou et al., 2016; Bi et al., 2018). However, further improvement of electron transfer in MES is difficult due to inadequate understanding of electrode-biofilm formation in EAB. Low efficiency electron transfer at the anodic biofilm-electrode interface remains one of the major limitations for full-scale implementation of microbial fuel cells (MFCs) (Logan, 2009). Manipulating exoelectrogenic biofilms to improve the efficiency of the electron transfer pathway is therefore a feasible strategy to improve MES performance.
Quorum sensing (QS) is a cell-cell communication mechanism in which extracellular signal molecules called autoinducers are released by bacteria. The autoinducers pass on information about population density, and the bacteria population then collectively regulates the expression of related genes as a response (Swift et al., 1996). QS has been found to regulate many bacterial physiological activities, including biofilm formation, in several bacterial species (Davies et al., 1998; Zhu and Mekalanos, 2003; Boles and Horswill, 2008). In particular, relatively complex QS systems are found in Pseudomonas aeruginosa (Juhas et al., 2005), a species commonly found in the biofilms of MESs. The las system of QS in P. aeruginosa is comprised of the transcriptional activator LasR and the N-(3-oxododecanoyl)-L-homoserine lactone (3-oxo-C12-HSL) signal molecule synthase LasI (Gambello and Iglewski, 1991; Passador and Iglewski, 1993). Similarly, in the rhl system, the enzyme RhlI catalyzes the synthesis of the N-butyryl-L-homoserine lactone (C4-HSL) signal molecule, which is detected by the transcriptional activator protein RhlR (Ochsner et al., 1994; Parsek et al., 1997). In addition to the two N-Acyl homoserine lactone (AHL) type signal molecules, a third autoinducer, 2-heptyl-3-hydroxy-4-quinolone (designated as Pseudomonas quinolone signal, PQS), provides a link between the las and rhl quorum-sensing systems (Pesci et al., 1999; Mcknight et al., 2000). These QS systems constitute a hierarchical regulation network in P. aeruginosa (Williams and Camara, 2009; Yong et al., 2015).
Recent studies have shown that QS signaling molecules play crucial roles in electricity generation by MFCs (Yong et al., 2011, 2015; Chen et al., 2017). For example, genetic enhancement of the QS circuit was applied in MFCs to enhance electricity production (Yong et al., 2011). The overexpression of rhlI and rhlR genes in wild-type strain lead to a significant increase in phenazine production, which directly resulted in an increase of current output in the rhlI overexpressed strain inoculated-MFCs. A PQS defective strain also produced higher concentrations of phenazines and exhibited increased current production when used in MFCs compared to the parent strain (Wang et al., 2013). Electrochemical activity of the bio-anode was promoted by the addition of 3-oxo-hexanoyl-homoserine lactone and 3-oxo-dodecanoyl-homoserine lactone in microbial electrolysis cells (MECs), and a higher current was produced with the addition of short chain acyl-homoserine lactone (Liu et al., 2015). In particular, these studies substantiated the observation that QS regulatory networks are involved in microbial extracellular electron transfer, and most recent studies focused on genetically engineering QS to improve the electricity output in MFCs. However, it is still unclear which signal molecules could be applied to improve MFC performances, and whether or not they could remedy the electricity production of mutants. In this study, P. aeruginosa strain PAO1 and its three mutants were pure culture-inoculated in MFCs and MECs to investigate the effect of QS on extracellular electron transfer. We subsequently assess the ability of QS to enhance attachment of anodic bacteria and current generation of MFCs by the addition of exogenous QS signals such as PQS and AHLs.
Materials and Methods
Bacteria and Culture Conditions
Pseudomonas aeruginosa PAO1 (wild-type) and three mutants (pqsA, pqsL, and lasIrhlI) were kindly provided by Tim Tolker-Nielsen (University of Copenhagen). The pqsA mutant was deficient in the production of PQS signal molecule while pqsL mutant overproduced PQS. The lasIrhlI mutant was deficient in rhlI and lasI, and so produced fewer AHL autoinducers (Allesen-Holm et al., 2006). All strains were routinely cultivated in Luria–Bertani (LB) medium with shaking at 37°C. 0.5 mL of overnight cultures were inoculated in 50 mL fresh LB medium for subsequent inoculation in single-chamber MFCs and MECs. The optical density at 600 nm (OD600) of incubated cultures was measured and all cultures were adjusted to the same level before inoculation.
MFC and MEC Configuration and Operation
Eight single-chamber air-cathode MFCs (Figure 1) were constructed using glass bottles as described in previous studies (Logan et al., 2007). Two pieces of carbon paper anode (2.5 cm by 8 cm; TGP-H090, Toray, Japan) were connected to titanium wires and then tied together as a whole. The anode was fixed through a hole in the bottle lid, sealed with silicone (GE83, GE Toshiba Silicones, Japan) and suspended completely in the medium. The reactors were covered by aluminum foil prior to autoclave. Air-cathodes (7 cm2) were fabricated by rolling activated carbon with polytetrafluoroethylene (PTFE) to form a catalyst layer, according to a previous study (Dong et al., 2012). After assembly, the whole reactors were packed in sterilizing bags and autoclaved at 0.1 MPa, 121°C for 15 min and an air filter membrane (0.22 μm; FGLP04700, Merck Millipore, Germany) was added outside of the air cathodes. MFCs were fed with 300 mL of medium (after sterilization) containing (per liter) 1 g sodium acetate in 100 mM phosphate buffer solution (PBS), metal salt (12.5 mL) and vitamin solutions (5 mL). 5 mL of bacterial culture solution (OD600 = 0.5) was added into the reactors as inoculum. All reactors operated in fed-batch mode in a temperature-controlled room at 35°C.
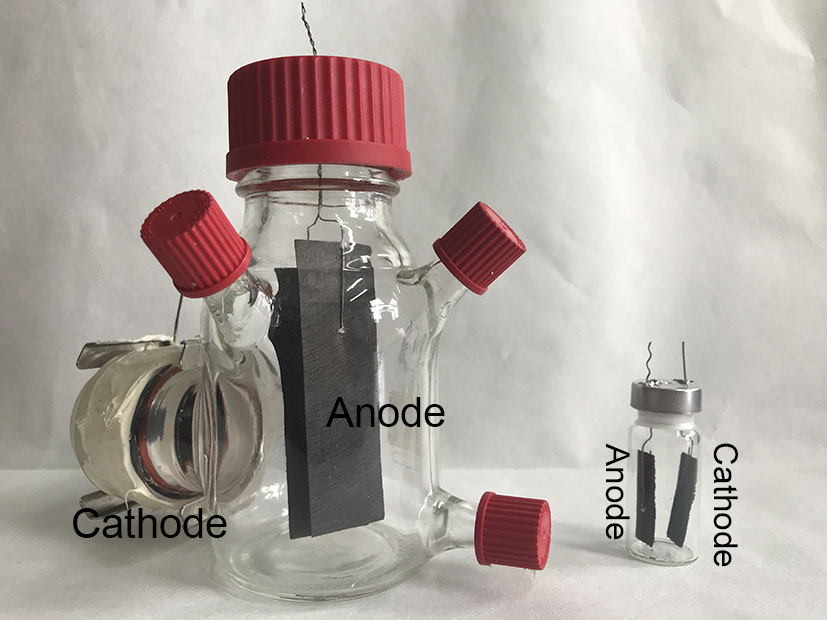
FIGURE 1. Photographs of air-cathode microbial fuel cell (left) and microbial electrolysis cell (right) containing carbon paper electrodes.
Eight single-chamber MECs (Figure 1) were constructed using 5 mL glass bottles as reported previously (Call and Logan, 2011). Carbon paper (2.5 cm by 1 cm) and rolling activated carbon (2.5 cm by 1 cm) were adopted as anode and cathode, respectively. After assembly, the whole reactors were autoclaved and fed with 5 mL of medium as described above. 0.5 mL of bacterial culture solution (OD600 = 0.5) was added into the reactors as inoculum. The fixed voltage of 0.8 V was applied to the whole cells by connecting the positive pole of a programmable power source (3645A, Array, Inc.) to the anodes, and the negative to the cathodes. All reactors were operated in a temperature-controlled room at 35°C.
The voltage across an external resistor of 1 kΩ (MFC) and 10 Ω (MEC) was automatically monitored through a data acquisition system (2700, Keithley Instrument, United States) connected to a personal computer. The current density was calculated as previously described (Logan et al., 2006).
Two AHLs type signal molecules of 3-oxo-C12-HSL, C4-HSL and PQS were purchased from Sigma-Aldrich (United States). Signal molecules were dissolved in dimethyl sulfoxide (DMSO) and sterilized with filter membrane (Millex-GP, 0.22 μm, Merck Millipore, Germany). These autoinducers were added with final concentration levels of 10 μM as previously described (Valle et al., 2004; Monzon et al., 2016).
Biomass Measurements
Anodic biofilms and cell suspension were collected from MFCs for protein extraction by ultrasound-assisted alkali pretreatment (Tian et al., 2015). Protein concentration was measured using a modified BCA Protein Assay Kit (Sangon Biotech, China). The effect of signal molecules on bacterial growth was assessed by pure culturing bacteria in 96-well plates (triplicates). 50 μL (OD600 = 0.5) bacterial culture and 350 μL sodium acetate (1 g/L) were added into the 96-well plates. Signal molecules were added separately to make a final concentration of 10 μM. An equivalent amount of DMSO was added to control cultures. The bacterial growth curve was acquired by using Bioscreen C automatic growth analyzer (Lab systems, Finland).
Results and Discussion
Electrochemical Performance of Pure-Culture MECs
The small-scale MECs could provide high throughput operation to maintain pure culture conditions. MECs inoculated with different P. aeruginosa strains showed a difference in current generation (Figure 2A). Two cycles of current generation indicated that the current output of the pqsA strain with deficient production of PQS was higher than that of pqsL with overproduced PQS. The overexpression of PQS signaling molecules unexpectedly limited extracellular electron transfer. In comparison to the wild-type, the AHL-deficient lasIrhlI mutant showed lower EET (extracellular electron transfer) rate based on the average of duplicate reactors.
Electrochemical Performance of Pure-Culture MFCs
Microbial fuel cells inoculated with four different strains of P. aeruginosa were operated simultaneously to further investigate the difference in electricity generation. MFC tests showed a similar and more obvious result compared to MECs. All MFCs attained reproducible cycles of current generation (Figure 2B). The maximum current density generated by wild-type P. aeruginosa PAO1 in MFC was 0.105 μA/cm2. It is at a similar level as a previous study with the same PAO1 strain, which generated approximately 0.150 μA/cm2 in a MFC (Wang et al., 2013). The coulombic efficiency was relative low, as it was not higher than 5%. Although the current generation and electron recovery from substrate was low, we could still analyze the effect of QS on the EET clearly. MFCs inoculated with pqsA mutants obtained the highest current density. The lasIrhlI-inoculated MFC produced lower current density than the other strains, due to its deficiency in rhlI and lasI. The AHLs QS systems induced cell lysis and extracellular DNA release which were responsible for biofilm structure formation in P. aeruginosa strains (Allesen-Holm et al., 2006). Additionally, the production of pyocyanin, which functions as an electron shuttle to enhance electron transport (Rabaey et al., 2005), is highly regulated by las and rhl (Brint and Ohman, 1995; Latifi et al., 1995; Pesci et al., 1997). The rhl QS system directly affect the EET as the production of pyocyanin was overproduced by the overexpression of the rhl genes (Yong et al., 2011). While the las QS system seemed to make indirect effect on the synthesis of pyocyanin, as it controls the rhl QS in two ways (Pesci et al., 1997). Thus, the susceptible biofilm (Allesen-Holm et al., 2006; Sakuragi and Kolter, 2007) and reduced production of pyocyanin may lead to the observed decrease in current generation of lasIrhlI-inoculated MFCs. The MFCs inoculated with PQS-deficient pqsA mutants obtained a much higher level of current generation than those inoculated with pqsL mutant, which overproduced PQS. PQS was demonstrated to inhibit bacteria growth (Toyofuku et al., 2008, 2010). Thus, large amount of PQS overproduced by the pqsL mutant might repress the production of electron shuttles, which limited the EET from EAB to the anode. However, to understand the regulation mechanism needs the more evidences of biofilm formation and transcriptome in the future. Conversely, PQS-deficient pqsA mutants could produce normal concentration level of electron shuttles under the directly regulation of rhl QS system and avoid negative effect of PQS, which promoted the EET with anode. Therefore, comparable experiments with the wild-type and mutant strains indicated that the overexpression of signaling molecules may limit the current generation in MFCs.
The difference in performance between the MEC and MFC was mainly due to differences in operating conditions. The configuration is an important factor that could affect the performance of MFCs (Logan et al., 2015) and MECs (Kadier et al., 2016). Previous studies suggest that MFCs fed with acetate as substrate produce higher power densities in small scale reactors in comparison to large-scale (Logan et al., 2015). In this study, the MFC volume (300 mL) was 60 folds larger than the MEC volume (5 mL). The MEC had a higher specific anode surface area (100 m2/m3) than the MFC (24.2 m2/m3). In addition, a higher ratio of inoculation was used in the MEC (10%) in comparison to the MFC (1.5%). Although the MEC could have higher current density and lower reagents costs because of its small size, the limited growth of P. aeruginosa under anaerobic conditions (Toyofuku et al., 2008) and insufficient biofilm formation resulted in the MEC being unable to be operated as long as the MFC. The oxygen level was also an important factor for the PQS system (Schertzer et al., 2010). Since oxygen was limited, the differences between different strains was not as visible in the MEC.
Effects of Biomass on Current Generation
Low production of currents in MFCs may be due to less enriched bacteria on the anode. To evaluate the hypothesis that anodic biomass may affect the current output, a measurement of protein concentration was made for biomass estimation during the third batch of MFC operation. Biomass of anode and solution in MFC were analyzed, respectively. Despite the highest anodic biomass, the lasIrhlI-inoculated MFC still had lower performance in electricity production (Figure 3A), while, the pqsL-inoculated MFC had a higher biomass in solution than other groups. This is largely due to the elevated release of extracellular DNA, as the QS-regulated DNA release can cause cell lysis (Webb et al., 2003). These results indicate that anodic biomass did not significantly affect the electricity production compared to QS.
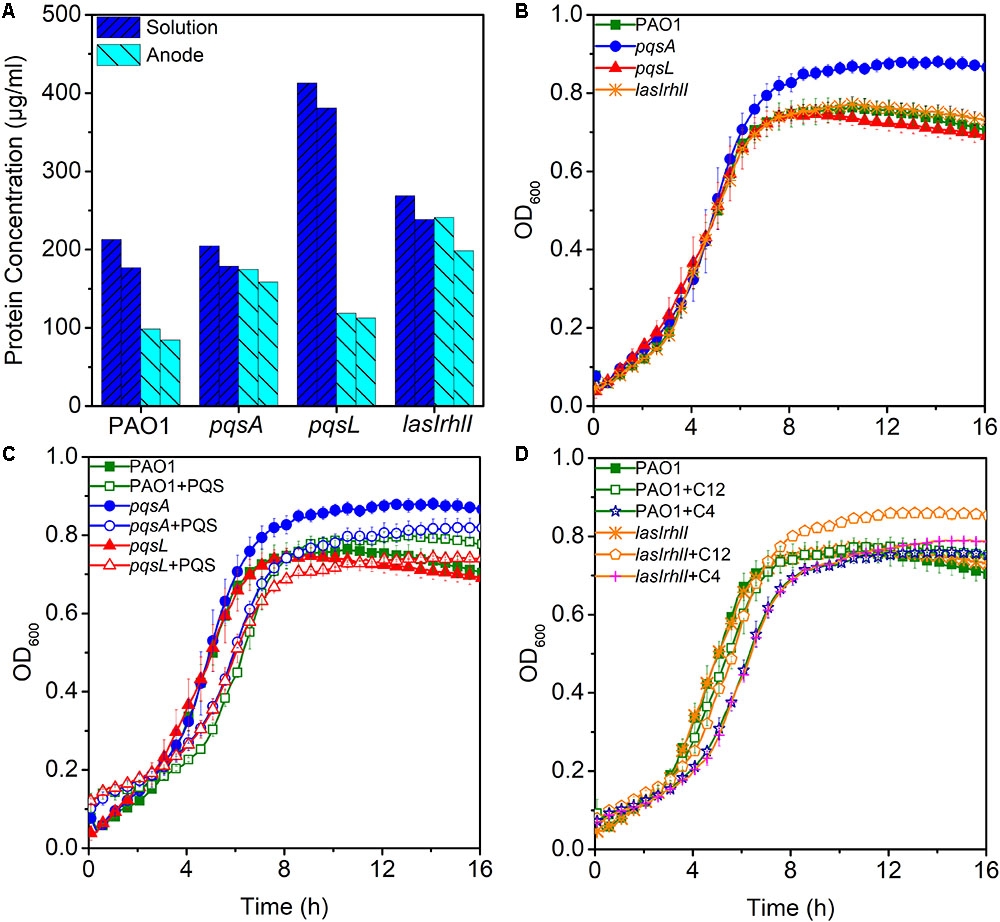
FIGURE 3. The maximum and minimum measured biomass of anode and solution in MFCs (A). Bacterial growth curves without signal molecules (B) and with PQS (C) and AHLs (D). Error bars represent the standard deviation of triplicate (B–D) tests.
Effects of QS Signals on Bacterial Growth
To examine the effect of different signal molecules on the growth of P. aeruginosa, the bacteria were grown in 96-well plates and signal molecules were added separately until a final concentration of 10 μM was reached. First, we examined the growth curve of different strains. There were no significant differences between them, except for pqsA which had a higher stationary phase OD than other strains (Figure 3B). When PQS was added to the cultures of PAO1, pqsA, and pqsL, a longer lag phase was observed. In addition, the pqsA+PQS had a shorter stationary-phase OD than pqsA (Figure 3C). These results indicate that PQS suppressed cell density and affected bacterial growth, as has been observed previously (Häussler and Becker, 2008; Toyofuku et al., 2008). Furthermore, the exogenous addition of 3-oxo-C12-HSL and C4-HSL increased the stationary phase OD of the lasIrhlI mutant strain (Figure 3D). The accumulation of extracellular DNA in the late-log phase was restored in the lasIrhlI mutant culture supplemented with signal molecules in a previous study (Allesen-Holm et al., 2006), which indicated that AHL QS regulated bacterial growth. The addition of signal molecules did not significantly influence the growth of the wild type planktonic cultures.
Effects of QS Signaling Molecules on the Performance of Pure-Culture MFCs
Quorum sensing played important roles in mediating the bioelectrochemical characteristics in MFCs. The pyocyanin-mediated EET from EAB to the anode is crucial for electricity generation in Pseudomonas-based MFC and the biosynthesis is regulated by QS systems (las, rhl, and PQS) (Rabaey et al., 2005; Yong et al., 2011; Wang et al., 2013). The electron shuttle biosynthesis is directly regulated by the rhl QS system as the overexpression of rhl QS system could lead a significant improvement in electricity generation (Yong et al., 2011). While the las QS system seemed to make indirect effect on the synthesis of pyocyanin, as it controls the rhl QS system and the mutations of las QS system decreased the pyocyanin production (Pesci et al., 1997). Besides, the PQS signaling system also regulates electron shuttle biosynthesis but this regulatory network may be inactivated due to the oxygen level (Schertzer et al., 2010). In order to further investigate the effect of QS on extracellular electron transfer regulation, the effect of the AHLs signal molecules on EET of wild-type and lasIrhlI mutant strains was studied in single-chamber air-cathode MFCs. 3-oxo-C12-HSL and C4-HSL were added until final concentration levels of 10 μM were reached. Results showed that adding 3-oxo-C12-HSL signal molecule caused no significant increase in EET of either wild-type or mutant strains, indicating that the las QS system may not directly affect EET in MFCs (Figure 4A). Although it showed that the las QS system could make indirect effect on the synthesis of pyocyanin by controlling the rhl QS system in P. aeruginosa, the rhl QS system could not be activated in the lasIrhlI mutant due to the deficiency of rhlI gene. However, a very significant increase was caused in lasIrhlI inoculated MFCs by the addition of C4-HSL signal molecule. This could increase the electricity production of mutant strains to a level similar to wild-type strain. In a previous related study, the engineered strains could attain much higher current than the wild-type parent strains by overexpressing the rhl QS system. This genetic manipulation resulted in an enhanced transcription level of the rhlI and rhlR genes, which increased the amount of signal molecules and transcriptional activators simultaneously and strengthened the current production when compared with wild-type (Yong et al., 2011). Thus, separately compensating for C4-HSL signal molecule in lasIrhlI mutant-inoculated MFCs restored the current generation to normal levels. Meanwhile, both signal molecules had little effect on wild-type strain-inoculated MFCs.
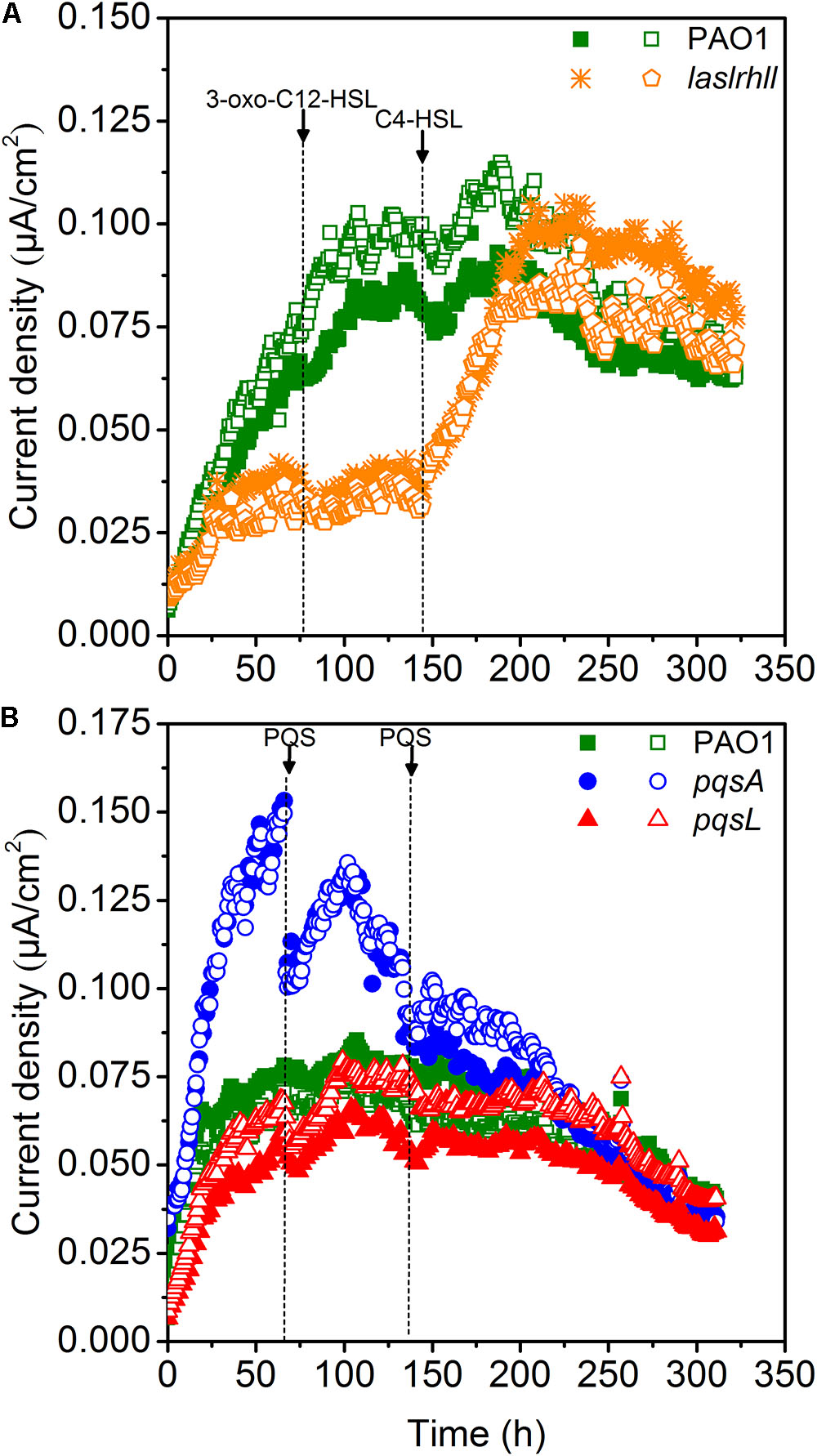
FIGURE 4. Current generation of MFCs after the addition of AHLs (A) and PQS (B). Arrows indicate the time point for adding QS signal molecules.
Microbial fuel cells inoculated with PAO1, pqsA and pqsL were supplemented with 10 μM of PQS. The maximum current decreased after adding PQS to pqsA-inoculated MFCs (Figure 4B). However, there was no significant effect on wild-type and pqsL mutants. In P. aeruginosa, PQS decreased growth rates under aerobic conditions and repressed anaerobic growth (Häussler and Becker, 2008; Toyofuku et al., 2008). Previous studies showed that P. aeruginosa didn’t produce detectable PQS anaerobically (Toyofuku et al., 2008; Schertzer et al., 2010). However, the special configuration of the air-cathodes formed a relatively micro-aerobic environment in the MFCs. Thus, the wild-type and pqsL mutants could both produce a considerable amount of PQS, which had negative effects on MFC performance. The addition of exogenous PQS to higher performance MFCs (pqsA-inoculated) decreased the current generation. This result is similar to a previous study (Wang et al., 2013), in which a PQS defective mutant inoculated MFC attained the lowest current generation. We hypothesized that this difference was likely caused by differences in reactor configuration. A higher oxygen level was available in the air-cathode MFCs than in dual chamber U-tube MFCs, which promoted bacterial growth (Toyofuku et al., 2008) and pyocyanin biosynthesis (Schertzer et al., 2010).
Conclusion
In summary, we investigated the effect of signal molecules on MFC performance and found that C4-HSL could increase the current generation of gene deficient variants to that of unmodified strains. PQS quorum sensing made no significant contribution to current output, and anodic biomass did not significantly alter electricity production when compared with QS. Thus, strategies to up-regulate the rhl QS system while limiting the PQS production are adoptable. However, the exogenous addition of signal molecules had little effect on the normal strain, which indicated that more efficient manipulation and utilization of QS needs to be further explored in mixed cultures MFCs. These findings will help provide suggestions for the enhancement of EET in MESs.
Author Contributions
DX designed the experiments. YY performed the specific experiments. YY, HZ, XM, BL, and DX contributed to analyze the experiment data. YY and DX wrote the manuscript. All authors were involved in revision of the manuscript and approved the final manuscript.
Funding
This study was supported by National Natural Science Foundation of China (No. 51422805), the Science Fund for Distinguished Young Scholars of Heilongjiang Province (Grant No. JC201407), the State Key Laboratory of Urban Water Resource and Environment (Harbin Institute of Technology) (No. 2016DX10).
Conflict of Interest Statement
The authors declare that the research was conducted in the absence of any commercial or financial relationships that could be construed as a potential conflict of interest.
References
Allesen-Holm, M., Barken, K. B., Yang, L., Klausen, M., Webb, J. S., Kjelleberg, S., et al. (2006). A characterization of DNA release in Pseudomonas aeruginosa cultures and biofilms. Mol. Microbiol. 59, 1114–1128. doi: 10.1111/j.1365-2958.2005.05008.x
Bi, L., Ci, S., Cai, P., Li, H., and Wen, Z. (2018). One-step pyrolysis route to three dimensional nitrogen-doped porous carbon as anode materials for microbial fuel cells. Appl. Surf. Sci. 427, 10–16. doi: 10.1016/j.apsusc.2017.08.030
Boles, B. R., and Horswill, A. R. (2008). agr-mediated dispersal of Staphylococcus aureus biofilms. PLoS Pathog. 4:e1000052. doi: 10.1371/journal.ppat.1000052
Bond, D. R., and Lovley, D. R. (2003). Electricity production by Geobacter sulfurreducens attached to electrodes. Appl. Environ. Microbiol. 69, 1548–1555. doi: 10.1128/AEM.69.3.1548-1555.2003
Brint, J. M., and Ohman, D. E. (1995). Synthesis of multiple exoproducts in Pseudomonas aeruginosa is under the control of RhlR-RhlI, another set of regulators in strain PAO1 with homology to the autoinducer-responsive LuxR-LuxI family. J. Bacteriol. 177, 7155–7163. doi: 10.1128/jb.177.24.7155-7163.1995
Call, D. F., and Logan, B. E. (2011). A method for high throughput bioelectrochemical research based on small scale microbial electrolysis cells. Biosens. Bioelectron. 26, 4526–4531. doi: 10.1016/j.bios.2011.05.014
Chen, S., Jing, X., Tang, J., Fang, Y., and Zhou, S. (2017). Quorum sensing signals enhance the electrochemical activity and energy recovery of mixed-culture electroactive biofilms. Biosens. Bioelectron. 97, 369–376. doi: 10.1016/j.bios.2017.06.024
Davies, D. G., Parsek, M. R., Pearson, J. P., Iglewski, B. H., Costerton, J. W., and Greenberg, E. P. (1998). The involvement of cell-to-cell signals in the development of a bacterial biofilm. Science 280, 295–298. doi: 10.1126/science.280.5361.295
Dong, H., Yu, H., Wang, X., Zhou, Q., and Feng, J. (2012). A novel structure of scalable air-cathode without Nafion and Pt by rolling activated carbon and PTFE as catalyst layer in microbial fuel cells. Water Res. 46, 5777–5787. doi: 10.1016/j.watres.2012.08.005
Gambello, M. J., and Iglewski, B. H. (1991). Cloning and characterization of the Pseudomonas aeruginosa lasR gene, a transcriptional activator of elastase expression. J. Bacteriol. 173, 3000–3009.
Häussler, S., and Becker, T. (2008). The Pseudomonas quinolone signal (PQS) balances life and death in Pseudomonas aeruginosa populations. PLoS Pathog. 4:e1000166. doi: 10.1371/journal.ppat.1000166
Juhas, M., Eberl, L., and Tümmler, B. (2005). Quorum sensing: the power of cooperation in the world of Pseudomonas. Environ. Microbiol. 7, 459–471. doi: 10.1111/j.1462-2920.2005.00769.x
Kadier, A., Kalil, M. S., Abdeshahian, P., Chandrasekhar, K., Mohamed, A., Azman, N. F., et al. (2016). Recent advances and emerging challenges in microbial electrolysis cells (MECs) for microbial production of hydrogen and value-added chemicals. Renew. Sust. Energ. Rev. 61, 501–525. doi: 10.1016/j.rser.2016.04.017
Karthikeyan, R., Wang, B., Xuan, J., Wong, J. W. C., Lee, P. K. H., and Leung, M. K. H. (2015). Interfacial electron transfer and bioelectrocatalysis of carbonized plant material as effective anode of microbial fuel cell. Electrochim. Acta 157, 314–323. doi: 10.1016/j.electacta.2015.01.029
Latifi, A., Winson, M. K., Foglino, M., Bycroft, B. W., Stewart, G. S. A. B., Lazdunski, A., et al. (1995). Multiple homologues of LuxR and LuxI control expression of virulence determinants and secondary metabolites through quorum sensing in Pseudomonas aeruginosa PAO1. Mol. Microbiol. 17, 333–343.
Liu, Q., Ren, Z. J., Huang, C., Liu, B., Ren, N., and Xing, D. (2016). Multiple syntrophic interactions drive biohythane production from waste sludge in microbial electrolysis cells. Biotechnol. Biofuels 9:162. doi: 10.1186/s13068-016-0579-x
Liu, W., Cai, W., Ma, A., Ren, G., Li, Z., Zhuang, G., et al. (2015). Improvement of bioelectrochemical property and energy recovery by acylhomoserine lactones (AHLs) in microbial electrolysis cells (MECs). J. Power Sources 284, 56–59. doi: 10.1016/j.jpowsour.2015.03.007
Logan, B. E. (2009). Exoelectrogenic bacteria that power microbial fuel cells. Nat. Rev. Microbiol. 7, 375–381. doi: 10.1038/nrmicro2113
Logan, B. E., Cheng, S., Watson, V., and Estadt, G. (2007). Graphite fiber brush anodes for increased power production in air-cathode microbial fuel cells. Environ. Sci. Technol. 41, 3341–3346. doi: 10.1021/es062644y
Logan, B. E., Hamelers, B., Rozendal, R. A., Schrorder, U., Keller, J., Freguia, S., et al. (2006). Microbial fuel cells: methodology and technology. Environ. Sci. Technol. 40, 5181–5192. doi: 10.1021/es0605016
Logan, B. E., Wallack, M. J., Kim, K. Y., He, W., Feng, Y., and Saikaly, P. E. (2015). Assessment of microbial fuel cell configurations and power densities. Environ. Sci. Technol. Lett. 2, 206–214. doi: 10.1021/acs.estlett.5b00180
Mcknight, S. L., Iglewski, B. H., and Pesci, E. C. (2000). The Pseudomonas quinolone signal regulates rhl quorum sensing in Pseudomonas aeruginosa. J. Bacteriol. 182, 2702–2708. doi: 10.1128/JB.182.10.2702-2708.2000
Mei, X., Guo, C., Liu, B., Tang, Y., and Xing, D. (2015). Shaping of bacterial community structure in microbial fuel cells by different inocula. RSC Adv. 5, 78136–78141. doi: 10.1039/c5ra16382j
Monzon, O., Yang, Y., Li, Q., and Alvarez, P. J. J. (2016). Quorum sensing autoinducers enhance biofilm formation and power production in a hypersaline microbial fuel cell. Biochem. Eng. J. 109, 222–227. doi: 10.1016/j.bej.2016.01.023
Ochsner, U. A., Koch, A. K., Fiechter, A., and Reiser, J. (1994). Isolation and characterization of a regulatory gene affecting rhamnolipid biosurfactant synthesis in Pseudomonas aeruginosa. J. Bacteriol. 176, 2044–2054. doi: 10.1128/jb.176.7.2044-2054.1994
Parsek, M. R., Schaefer, A. L., and Greenberg, E. P. (1997). Analysis of random and site-directed mutations in rhlI, a Pseudomonas aeruginosa gene encoding an acylhomoserine lactone synthase. Mol. Microbiol. 26, 301–310. doi: 10.1046/j.1365-2958.1997.5741935.x
Passador, L., and Iglewski, B. H. (1993). Expression of Pseudomonas aeruginosa virulence genes requires cell-to-cell communication. Science 260, 1127–1130. doi: 10.1126/science.8493556
Pesci, E. C., Milbank, J. B. J., Pearson, J. P., Mcknight, S., Kende, A. S., Greenberg, E. P., et al. (1999). Quinolone signaling in the cell-to-cell communication system of Pseudomonas aeruginosa. Proc. Natl. Acad. Sci. U.S.A. 96, 11229–11234. doi: 10.1073/pnas.96.20.11229
Pesci, E. C., Pearson, J. P., Seed, P. C., and Iglewski, B. H. (1997). Regulation of las and rhl quorum sensing in Pseudomonas aeruginosa. J. Bacteriol. 179, 3127–3132. doi: 10.1128/jb.179.10.3127-3132.1997
Rabaey, K., Boon, N., Höfte, M., and Verstraete, W. (2005). Microbial phenazine production enhances electron transfer in biofuel cells. Environ. Sci. Technol. 39, 3401–3408. doi: 10.1021/es048563o
Rabaey, K., Boon, N., Siciliano, S. D., Verhaege, M., and Verstraete, W. (2004). Biofuel cells select for microbial consortia that self-mediate electron transfer. Appl. Environ. Microbiol. 70, 5373–5382. doi: 10.1128/AEM.70.9.5373-5382.2004
Reguera, G., McCarthy, K. D., Mehta, T., Nicoll, J. S., Tuominen, M. T., and Lovley, D. R. (2005). Extracellular electron transfer via microbial nanowires. Nature 435, 1098–1101. doi: 10.1038/nature03661
Rinaldi, A., Mecheri, B., Garavaglia, V., Licoccia, S., Nardo, P. D., and Traversa, E. (2008). Engineering materials and biology to boost performance of microbial fuel cells: a critical review. Energy Environ. Sci. 1, 417–429. doi: 10.1039/b806498a
Sakuragi, Y., and Kolter, R. (2007). Quorum-sensing regulation of the biofilm matrix genes (pel) of Pseudomonas aeruginosa. J. Bacteriol. 189, 5383–5386. doi: 10.1128/JB.00137-07
Schertzer, J. W., Brown, S. A., and Whiteley, M. (2010). Oxygen levels rapidly modulate Pseudomonas aeruginosa social behaviours via substrate limitation of PqsH. Mol. Microbiol. 77, 1527–1538. doi: 10.1111/j.1365-2958.2010.07303.x
Swift, S., Throup, J. P., Williams, P., Salmond, G. P. C., and Stewart, G. S. A. B. (1996). Quorum sensing: a population-density component in the determination of bacterial phenotype. Trends Biochem. Sci. 21, 214–219.
Tian, X., Wang, C., Trzcinski, A. P., Lin, L., and Ng, W. J. (2015). Insights on the solubilization products after combined alkaline and ultrasonic pre-treatment of sewage sludge. J. Environ. Sci. 29, 97–105. doi: 10.1016/j.jes.2014.07.024
Toyofuku, M., Nakajima-kambe, T., Uchiyama, H., and Nomura, N. (2010). The effect of a cell-to-cell communication molecule, Pseudomonas quinolone signal (PQS), produced by P. aeruginosa on other bacterial species. Microbes Environ. 25, 1–7. doi: 10.1264/jsme2.ME09156
Toyofuku, M., Nomura, N., Kuno, E., Tashiro, Y., Nakajima, T., and Uchiyama, H. (2008). Influence of the Pseudomonas quinolone signal on denitrification in Pseudomonas aeruginosa. J. Bacteriol. 190, 7947–7956. doi: 10.1128/JB.00968-08
Valle, A., Bailey, M. J., Whiteley, A. S., and Manefield, M. (2004). N-acyl-L-homoserine lactones (AHLs) affect microbial community composition and function in activated sludge. Environ. Microbiol. 6, 424–433. doi: 10.1111/j.1462-2920.2004.00581.x
Wang, V. B., Chua, S.-L., Cao, B., Seviour, T., Nesatyy, V. J., Marsili, E., et al. (2013). Engineering PQS biosynthesis pathway for enhancement of bioelectricity production in Pseudomonas aeruginosa microbial fuel cells. PLoS One 8:e63129. doi: 10.1371/journal.pone.0063129
Webb, J. S., Thompson, L. S., James, S., Charlton, T., Tolkernielsen, T., Koch, B., et al. (2003). Cell death in Pseudomonas aeruginosa biofilm development. J. Bacteriol. 185, 4585–4592. doi: 10.1128/JB.185.15.4585-4592.2003
Williams, P., and Camara, M. (2009). Quorum sensing and environmental adaptation in Pseudomonas aeruginosa: a tale of regulatory networks and multifunctional signal molecules. Curr. Opin. Microbiol. 12, 182–191. doi: 10.1016/j.mib.2009.01.005
Xing, D., Tang, Y., Mei, X., and Liu, B. (2015). Electricity generation of microbial fuel cell with waterproof breathable membrane cathode. J. Power Sources 300, 491–495. doi: 10.1016/j.jpowsour.2015.09.106
Yong, Y.-C., Wu, X.-Y., Sun, J.-Z., Cao, Y.-X., and Song, H. (2015). Engineering quorum sensing signaling of Pseudomonas for enhanced wastewater treatment and electricity harvest: a review. Chemosphere 140, 18–25. doi: 10.1016/j.chemosphere.2014.10.020
Yong, Y.-C., Yu, Y.-Y., Li, C.-M., Zhong, J.-J., and Song, H. (2011). Bioelectricity enhancement via overexpression of quorum sensing system in Pseudomonas aeruginosa-inoculated microbial fuel cells. Biosens. Bioelectron. 30, 87–92. doi: 10.1016/j.bios.2011.08.032
Zhu, J., and Mekalanos, J. J. (2003). Quorum sensing-dependent biofilms enhance colonization in Vibrio cholerae. Dev. Cell 5, 647–656. doi: 10.1016/S1534-5807(03)00295-8
Keywords: quorum sensing, autoinducers, extracellular electron transfer, microbial fuel cell, biofilm
Citation: Yang Y, Zhou H, Mei X, Liu B and Xing D (2018) Dual-Edged Character of Quorum Sensing Signaling Molecules in Microbial Extracellular Electron Transfer. Front. Microbiol. 9:1924. doi: 10.3389/fmicb.2018.01924
Received: 02 June 2018; Accepted: 30 July 2018;
Published: 20 August 2018.
Edited by:
Fanghua Liu, Yantai Institute of Coastal Zone Research (CAS), ChinaReviewed by:
Oskar Modin, Chalmers University of Technology, SwedenKarthikeyan Rengasamy, Washington University in St. Louis, United States
Copyright © 2018 Yang, Zhou, Mei, Liu and Xing. This is an open-access article distributed under the terms of the Creative Commons Attribution License (CC BY). The use, distribution or reproduction in other forums is permitted, provided the original author(s) and the copyright owner(s) are credited and that the original publication in this journal is cited, in accordance with accepted academic practice. No use, distribution or reproduction is permitted which does not comply with these terms.
*Correspondence: Defeng Xing, ZHhpbmdAaGl0LmVkdS5jbg==