- Department of Basic Sciences, College of Veterinary Medicine, Mississippi State University, Starkville, MS, United States
Edwardsiella ictaluri is a Gram-negative intracellular pathogen causing enteric septicemia of channel catfish (ESC). Type six secretion system (T6SS) is a sophisticated nanomachine that delivers effector proteins into eukaryotic host cells as well as other bacteria. In the current work, we in-frame deleted the E. ictaluri evpB gene located in the T6SS operon by allelic exchange. The safety and efficacy of EiΔevpB as well as Aquavac-ESC, a commercial vaccine manufactured by Intervet/Merck Animal Health, were evaluated in channel catfish (Ictalurus punctatus) fingerlings and fry by immersion exposure. Our results showed that the EiΔevpB strain was avirulent and fully protective in catfish fingerlings. The EiΔevpB strain was also safe in catfish fry, and immersion vaccination with EiΔevpB at doses 106 and 107 CFU/ml in water resulted in 34.24 and 80.34% survival after wild-type immersion challenge compared to sham-vaccinated fry (1.79% survival). Catfish fry vaccinated with EiΔevpB at doses 106, 107, and 108 CFU/ml in water exhibited dose-dependent protection. When compared with Aquavac-ESC, EiΔevpB provided significantly higher protection in catfish fingerlings and fry (p < 0.05). Results indicate that the EiΔevpB strain is safe and can be used to protect catfish fingerlings and fry against E. ictaluri.
Introduction
Channel catfish (Ictalurus punctatus) is the most significant aquaculture commodity in the United States, and Edwardsiella ictaluri causes enteric septicemia of channel catfish (ESC) (Hawke, 1979). The disease occurs as acute enteric septicemia or chronic encephalitis (Shotts et al., 1986). Use of antibiotic-added feed (Smith et al., 1994; Plumb et al., 1995) and feed restriction (Wise et al., 2004) are traditional means used to control ESC. Although these practices could reduce mortalities, feed restriction results in reduced production through lost feeding days. Medicated feed is expensive, useful only in fish that accept feed, and could yield antibiotic-resistant strains.
Vaccination is a vital prophylactic strategy for prevention of bacterial diseases in aquaculture (Shoemaker et al., 2009; Villumsen et al., 2014). Because E. ictaluri species has been shown to be very homogeneous (Plumb and Vinitnantharat, 1989; Bertolini et al., 1990), a vaccine strain could potentially provide wide-range of protection against different E. ictaluri strains in different fish species. The commercial ESC vaccine Aquavac-ESC (RE-33) was developed by serial passage in increasing concentrations of rifampicin (Klesius and Shoemaker, 1997). Aquavac-ESC is safe in catfish fry (Klesius and Shoemaker, 1999), but it has not been accepted widely due to marginal economic returns (Bebak and Wagner, 2012). Another live attenuated E. ictaluri vaccine was developed by serial passage on media containing increasing concentrations of rifamycin, which protected catfish fingerlings when added in feed and administered orally (Wise et al., 2015). Under current catfish production practices, catfish fry are transferred from hatchery to nursery ponds when they are 1–2-week-old. Therefore, there is an urgent need for an effective ESC vaccine that can be delivered to catfish fry before their release into nursery ponds.
Type six secretion system (T6SS) is a virulence factor for many pathogenic bacteria (Rao et al., 2004; Pukatzki et al., 2006). This system is highly conserved and widely distributed in Gram-negative bacteria as one or more copies (Bingle et al., 2008). T6SS delivers protein effectors into the periplasm of the target cells directly upon cell-to-cell contact. Therefore, contributing to different processes ranging from inter-bacterial killing to pathogenesis. The number of genes encoded within T6SS clusters usually varies between 16 and 38 genes (Cascales, 2008; Murdoch et al., 2011), with a minimal set of 13 genes required to assemble a functional T6SS (Boyer et al., 2009; Lin et al., 2013). T6SS is also required to kill other bacterial cells by secreting anti-bacterial proteins (Ho et al., 2014).
Our previous proteomics study showed that EvpB protein is differentially regulated during in vitro iron-restricted conditions (Dumpala et al., 2015). Thus, we hypothesized that EvpB protein could have a crucial role in the T6SS of E. ictaluri. In the current work, we report the construction of an evpB in-frame deletion mutant and its vaccine potential in catfish fingerlings and fry.
Materials and Methods
Bacterial Strains, Plasmids, and Growth Conditions
Bacterial strains and plasmids used in this work are listed in Table 1. Wild-type E. ictaluri strain 93–146 (EiWT) was cultured in brain heart infusion (BHI) agar or broth (Difco, Sparks, MD, United States) and incubated at 30°C throughout the study. E. coli strains CC118λpir and SM10λpir were cultured on Luria-Bertani (LB) agar or broth (Difco, Sparks, MD, United States) and incubated at 37°C throughout the study. When required, media were supplemented with the following antibiotics and reagents: ampicillin (amp: 100 mg/ml), colistin sulfate (col: 12.5 mg/ml), sucrose (5%), and mannitol (0.35%) (Sigma-Aldrich, St. Louis, MO, United States).
Sequence Analysis
The nucleotide sequences of the T6SS operon were obtained from the E. ictaluri strain 93–146 genome (GenBank accession: CP001600) (Williams et al., 2012). The Basic Local Alignment Search Tool (BLAST) was used to determine the sequence of the evpB open reading frame and adjacent sequences.
Construction of EiΔevpB In-frame Deletion Mutant
In-frame deletion of the E. ictaluri evpB gene (NT01EI_RS11895) was accomplished by following the procedures described previously (Abdelhamed et al., 2013). Briefly, 1,210 bp upstream and 1,155 bp downstream regions of evpB were amplified from E. ictaluri strain 93–146 genomic DNA with A/B and C/D primer pairs (Table 2), respectively. These PCR products were mixed, diluted, and used as template in a splicing overlap extension PCR (Horton et al., 1990) with the A/D primers to generate ΔevpB deletion fragment (2,365 bp). The resulting ΔevpB deletion fragment was cloned into pMEG-375 suicide plasmid. The resulting plasmid, pEiΔevpB, was transformed into E. ictaluri by conjugation, and transformants were selected on BHI agar containing Amp and Col at 30°C for 2 days. To allow the second homologous recombination, a single Amp-resistant merodiploid colony was plated on BHI agar with sucrose and mannitol and incubated at 30°C for 3 days. Amp-sensitive colonies were screened by colony PCR using the A/D primers, and further confirmation was done by sequencing of A/D fragment.
Complementation of the evpB Gene
The 1,464 bp open reading frame of the evpB gene was amplified using primers listed in Table 2. The amplicon was cloned into a pBBR1-MCS4 plasmid (Kovach et al., 1995) at the SmaI and XbaI restriction sites. The resulting plasmid, pEievpB, was transferred to EiΔevpB by conjugation. Successful transformation was verified by observing plasmid profile of EiΔevpB. The resulting strain was designated as EiΔevpB+pEievpB.
Determination of Safety and Efficacy of EiΔevpB in Catfish Fingerlings
All fish experiments were approved by the Institutional Animal Care and Use Committee at Mississippi State University (protocol numbers: 12–042, 15–043, and 17–288). Virulence and vaccine efficacy of the EiΔevpB strain were assessed, as described (Abdelhamed et al., 2013). Briefly, 240 specific-pathogen-free (SPF) channel catfish fingerlings (13.88 ± 0.27 cm, 27.77 ± 1.04 g) were stocked in 40 l flow-through tanks (20 fish/tank) with constant aeration and allowed to acclimate for 1 week. Water temperature was maintained at 26 ± 2°C during the experiment. The tanks were randomly assigned into three groups, and each group contained four replicates. The three groups were EiΔevpB, EiWT (positive control), and BHI (sham control). The fish were vaccinated by immersion for 1 h in water containing approximately 3.32 × 107 CFU/ml, and then flow-through conditions were resumed. Mortalities were recorded daily, and the presence of E. ictaluri was confirmed by streaking anterior kidney onto BHI plates. At 21-days post-immunization, the vaccinated fish were challenged with EiWT (3.83 × 107 CFU/ml in water) by immersion for 1 h as described above. Mortalities were recorded daily.
Determination of Safety and Efficacy of EiΔevpB in Catfish Fry
Nine hundred 14-day-old SPF channel catfish fry were stocked in 18 tanks (50 fry/tank). Tanks were randomly assigned to six treatment groups with three replicates per group. Treatment groups consisted of high (3.32 × 107 CFU/ml in water) and low (3.32 × 106 CFU/ml in water) doses of EiΔevpB, EiWT, and BHI. Immersion vaccination was conducted same as fingerling challenge described above. At 21 days post-vaccination, fry were challenged with EiWT by immersion exposure at approximately 3.10 × 107 CFU/ml in water. Mortalities were recorded daily.
Evaluation of Various Challenge Doses of EiΔevpB in Catfish Fry
Vaccine efficacies of three separate doses of EiΔevpB were evaluated in 7-day-old fry to determine the optimal dose. Briefly, 750 fry were stocked into 15 tanks (50 fry/tank). The tanks were divided into five groups with three replicates per group. Vaccinated groups consisted of three doses of EiΔevpB (3.72 × l06, 3.72 × l07, and 3.72 × l08 CFU/ml in water), EiWT (positive control), and BHI (sham control). Fish were monitored daily, and mortalities were recorded from each tank. After 30 days post-vaccination, fry were challenged with the EiWT by immersion in water (3.80 × 107 CFU/ml) for 1 h. Mortalities were recorded daily.
Comparison of EiΔevpB and Aquavac-ESC in Catfish Fingerlings
Vaccine efficacy of EiΔevpB strain was compared with Aquavac-ESC in catfish fingerlings. Briefly, 320 channel catfish fingerlings (7.75 ± 0.08 cm, 4.50 ± 0.014 g) were stocked into 16 tanks (20 fish/tank). Each group included four replicate tanks. Vaccination groups consisted of Aquavac-ESC, EiΔevpB, EiWT (positive control), and BHI (sham control). Fingerlings were vaccinated by immersion in water containing approximately 4.5 × 107 CFU/ml for 1 h. Fish were monitored, and dead fish were removed daily. After 21 days, immunized fish were challenged with EiWT by immersion in water with 3.80 × 107 CFU/ml for 1 h. Mortalities were recorded daily.
Comparison of EiΔevpB and Aquavac-ESC in Catfish Fry
Vaccine efficacy of EiΔevpB was compared with Aquavac-ESC in 14-days post-hatch fry. Briefly, 800 channel catfish fry were stocked into 16 tanks (50 fish/tank). Each group included four replicate tanks. Vaccination groups consisted of EiΔevpB strain, Aquavac-ESC, EiWT (positive control), and BHI (sham control). Fry were vaccinated by immersion (3.72 × 107 CFU/ml in water) for 1 h. Fish were monitored, and dead fish were removed daily. After 21 days, immunized fish were challenged with EiWT by immersion (3.80 × 107 CFU/ml in water) for 1 h. Mortalities were recorded daily.
Comparison of EiΔevpB and Complemented Strain
Virulence of EiΔevpB+pEievpB strain was compared to EiΔevpB in 12-days post-hatch fry. Briefly, 360 channel catfish fry were stocked into 12 tanks (30 fish/tank). Experimental groups consisted of EiΔevpB, EiΔevpB+pEievpB, EiWT (positive control), and BHI (negative). Each group included three replicate tanks. Fry were challenged by immersion (4.60 × 107 CFU/ml in water) for 1 h. Mortalities were recorded daily.
Statistical Analysis
The percent mortality and survival values were arcsine transformed, and pairwise comparison of the means was performed with Tukey procedure. Analysis of variance (ANOVA) was done using PROC GLM in SAS for Windows v9.4 (SAS Institute, Inc., Cary, NC, United States) to assess significance. An alpha level of 0.05 was used in all analyses.
Results
T6SS in E. ictaluri Genome
Analysis of the E. ictaluri genome revealed the presence of a 20,724 bp operon containing 16 genes (evpP, plus genes from NT01EI_RS11890 to NT01EI_RS11960) that encode for the T6SS apparatus, chaperones, effectors, and regulators (Figure 1).

FIGURE 1. Type VI secretion system organization in the E. ictaluri genome. Arrows indicate the direction of transcription, and numbers at the beginning and the end indicate genomic coordinates.
Construction of the EiΔevpB Mutant
We successfully introduced an in-frame deletion to the evpB gene in the E. ictaluri chromosome. The resulting EiΔevpB strain contained a deletion of 1,167 bp out of 1,488 bp open reading frame (78.42%), resulting in loss of 389 amino acids from the E. ictaluri evpB gene. This in-frame deletion was verified by PCR and sequencing of the amplified fragment from the EiΔevpB strain.
Virulence and Vaccine Efficacy of EiΔevpB in Catfish Fingerlings
The virulence of EiΔevpB (0% mortality) was significantly lower than those fish challenged with EiWT (46.91% mortality) (P < 0.5) (Figure 2A). At 21 days post-vaccination, the EiΔevpB vaccinated group had significantly higher survival compared to the sham-vaccinated group (100% vs. 40.69% survival, respectively) (P < 0.05) (Figure 2B).
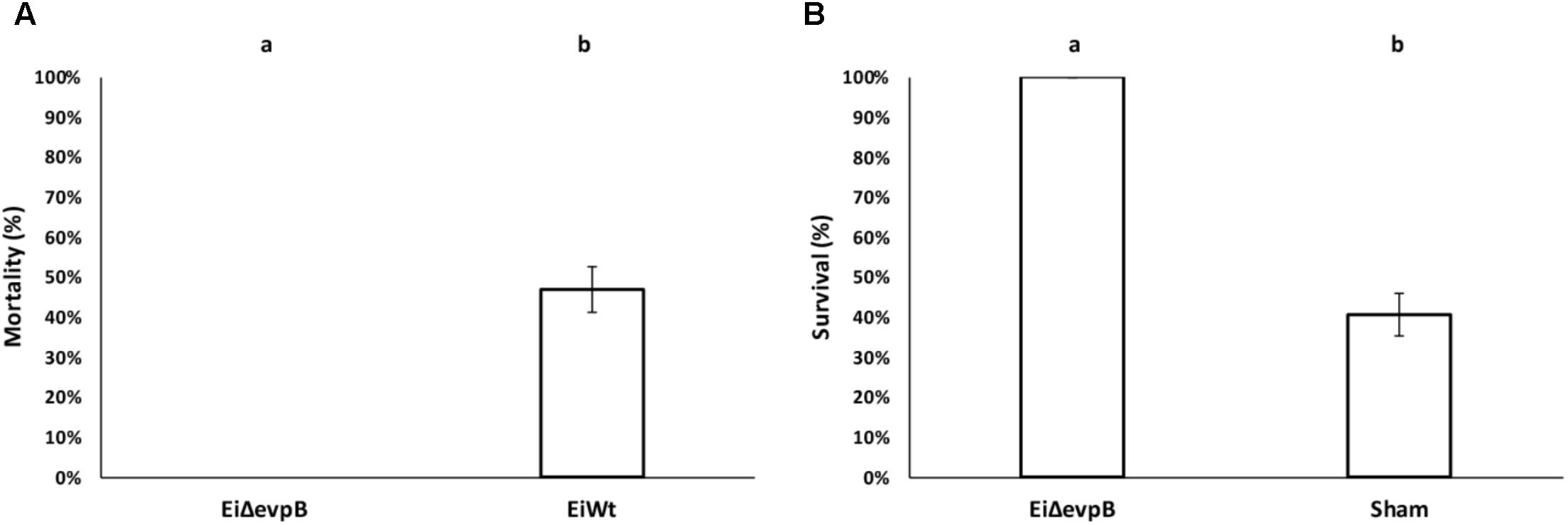
FIGURE 2. Results of virulence (A) and vaccine efficacy (B) trials of EiΔevpB in catfish fingerlings. Lowercase letters indicate significant differences (p < 0.05).
Virulence and Efficacy of EiΔevpB in Fry
No mortality was observed in the fry vaccinated with EiΔevpB at 106 and 107 CFU/ml in water. In contrast, 98.67 and 100% mortalities were observed in the fry exposed to EiWT at 106 and 107 CFU/ml in water doses, respectively (Figure 3A). The fry vaccinated with EiΔevpB showed 34.24% survival at 106 CFU/ml in water, and 80.34% survival at 107 CFU/ml in water at 21 days post-vaccination. On the contrary, the sham-vaccinated group had 1.79% survival (Figure 3B).
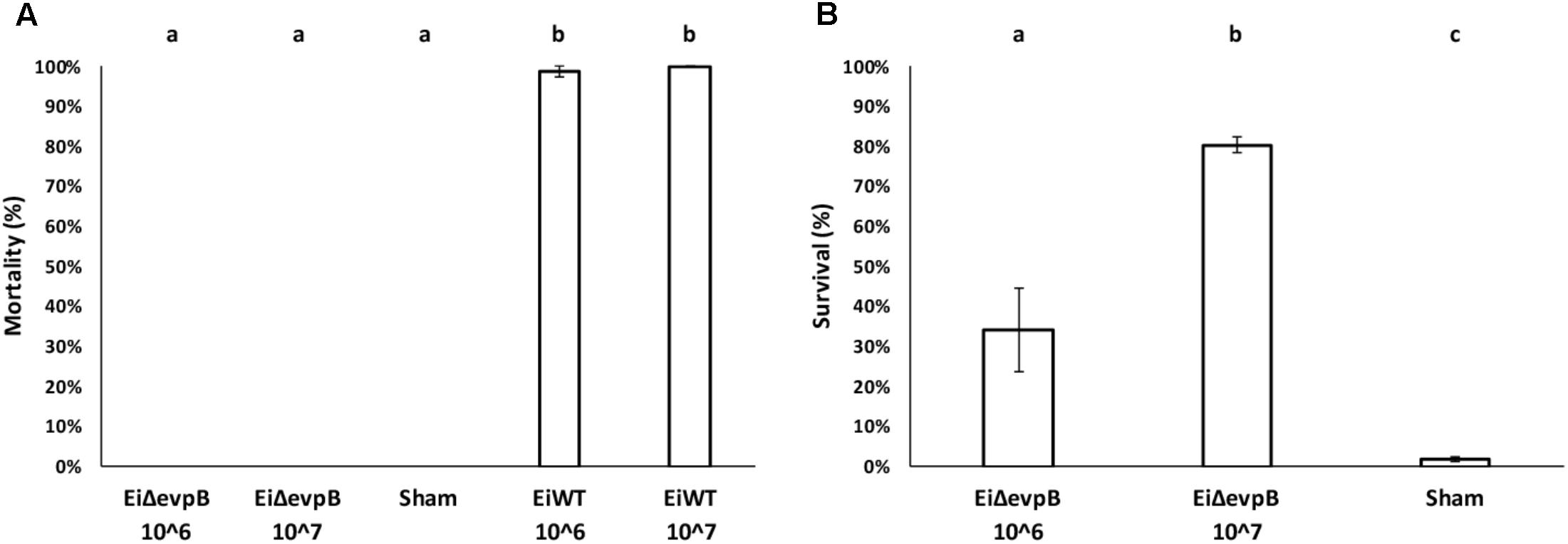
FIGURE 3. Results of virulence (A) and vaccine efficacy (B) trials in catfish fry vaccinated with two different doses of EiΔevpB (106 and 107CFU/ml in water). Lowercase letters indicate significant differences (p < 0.05).
Optimal Vaccine Dose of EiΔevpB in Catfish Fry
Mortality in 7-day old fry vaccinated with EiΔevpB (106, 107, and 108 CFU/ml in water) and sham group ranged between 2.53 and 5.13%, which was not statistically different. Dead fish collected from these treatments did not show any pathology, and E. ictaluri was not present in the fish. On the other hand, very high mortality (95.71%) was observed in EiWT exposed (107 CFU/ml in water) fry (Figure 4A). At 30-day post-vaccination with three different doses (106, 107, and108 CFU/ml in water), the percent survival in fry challenged with EiWT were significantly higher (57.74, 62.74, and 71.06%, respectively) compared to the sham-vaccinated fry (12.16% survival) (P < 0.05) (Figure 4B).
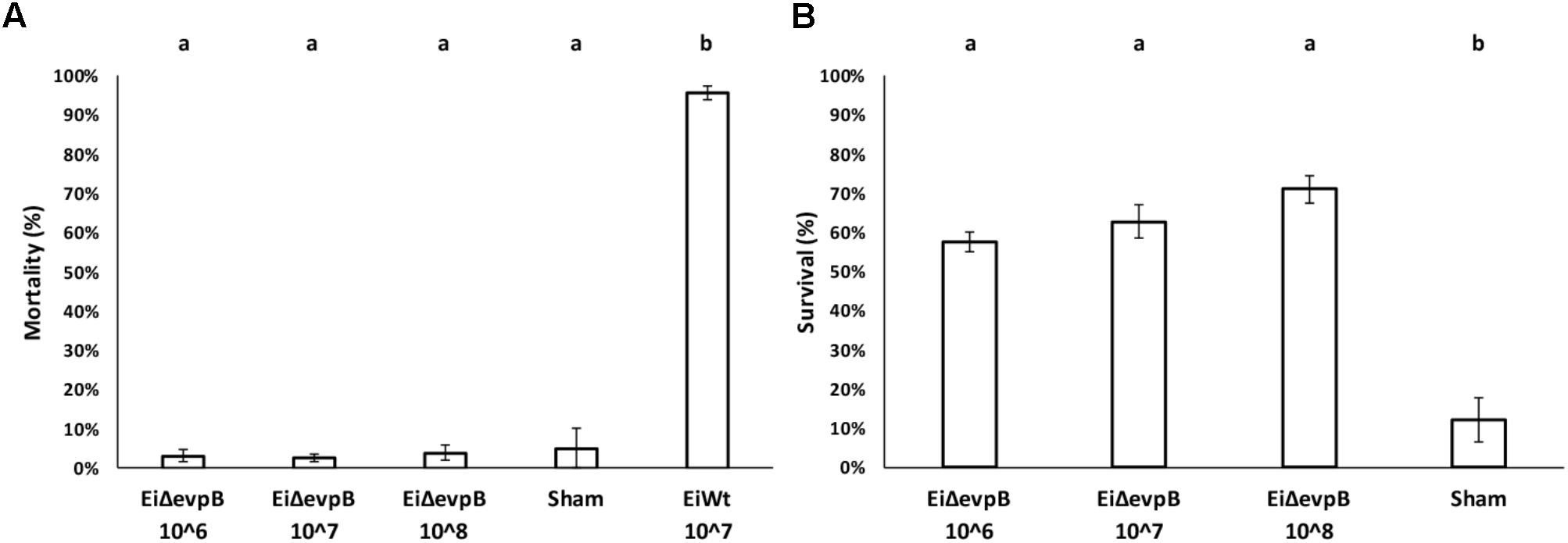
FIGURE 4. Results of virulence (A) and vaccine efficacy (B) trials of three different vaccination doses (106, 107, and 108 CFU/ml in water) of EiΔevpB in catfish fry. Lowercase letters indicate significant differences (p < 0.05).
Comparison of EiΔevpB to Aquavac-ESC in Catfish Fingerling
EiΔevpB, Aquavac-ESC, and sham control showed low mortalities (5.14, 1.45, and 1.67%, respectively) in catfish fingerlings. On the other hand, EiWT challenge caused 81.83% mortality (Figure 5A). Catfish fingerlings vaccinated with EiΔevpB elicited significantly higher protection (96.20% survival) compared to that of Aquavac-ESC (45.51% survival) and sham-vaccinated (6.67% survival) groups (p < 0.05) following EiWT challenge (Figure 5B).
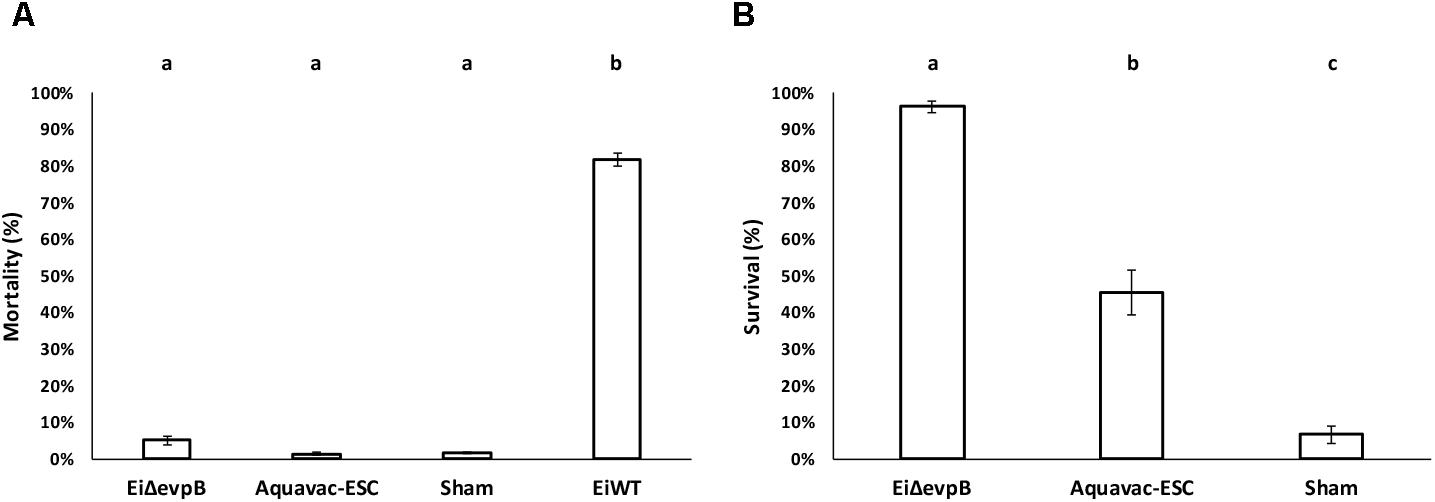
FIGURE 5. Results of virulence (A) and vaccine efficacy (B) trials of EiΔevpB and Aquavac-ESC in catfish fingerlings. Lowercase letters indicate significant differences (p < 0.05).
Comparison of EiΔevpB to Aquavac-ESC in Catfish Fry
EiΔevpB and Aquavac-ESC showed no mortalities, while negligible mortality was observed in the sham group (0.50%). EiWT group exhibited very high mortality (92.21% mortality) (Figure 6A). These results indicated that EiΔevpB and Aquavac-ESC were safe in catfish fry. In the vaccine efficacy experiment, EiΔevpB strain elicited significantly higher protection compared to Aquavac-ESC and sham-vaccinated fish (88.10, 27.40, and 5.56% survival, respectively) (p < 0.05) (Figure 6B).
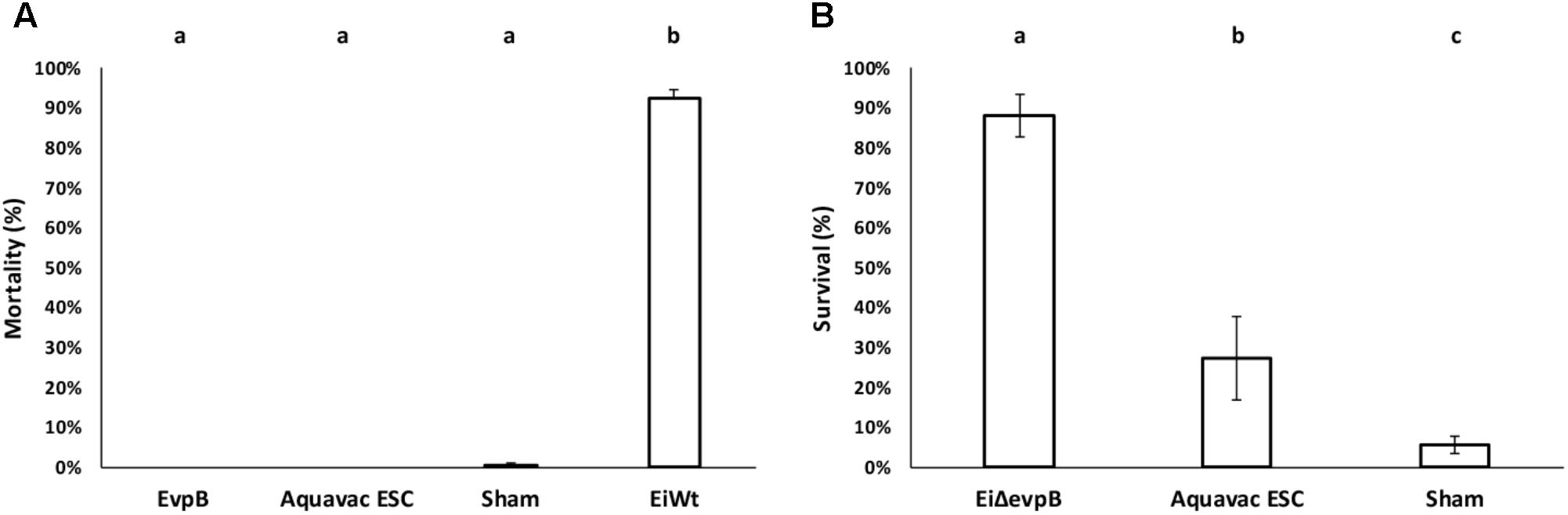
FIGURE 6. Results of virulence (A) and vaccine efficacy (B) trials of EiΔevpB and Aquavac-ESC in catfish fry. Lowercase letters indicate significant differences (p < 0.05).
Comparison of EiΔevpB to Complemented Strain
Complementation of EiΔevpB with pEievpB plasmid carrying the wild-type evpB gene restored the wild-type phenotype. The percent mortalities in 12-day-old fry vaccinated with EiΔevpB, EiΔevpB+pEievpB, sham, and EiWT groups were 7.78, 98.02, 8.89, and 100%, respectively (Figure 7).
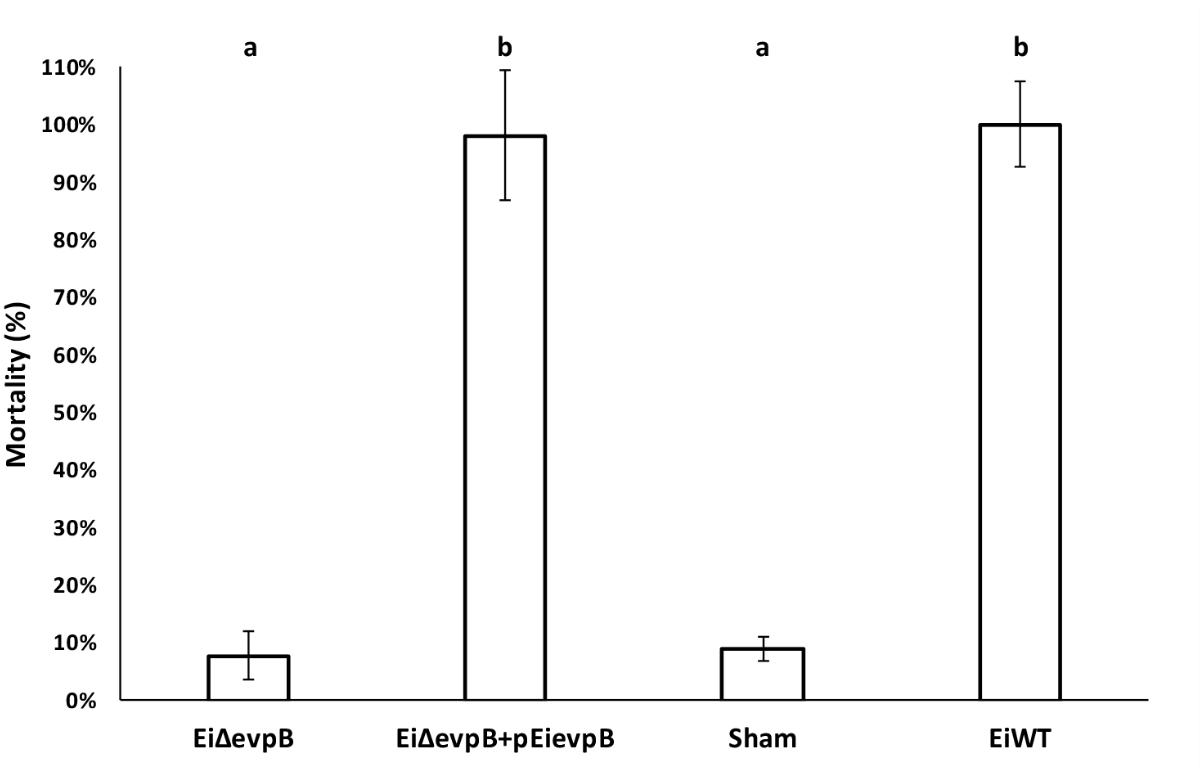
FIGURE 7. Mortalities observed in catfish fry vaccinated with EiΔevpB, complemented (EiΔevpB+pEievpB), sham, and EiWT groups. Lowercase letters indicate significant differences (p < 0.05).
Discussion
The primary objective of this study was to develop a live attenuated E. ictaluri vaccine strain based on mutation of the evpB gene, which is the second gene in the T6SS operon. The E. ictaluri EvpB protein was previously annotated as Eip55 (Moore et al., 2002), but it was not known that Eip55 was part of the T6SS. Eip55 is expressed during E. ictaluri infection and is antigenic to channel catfish. The percent sequence identity at the amino acid level between E. ictaluri and E. tarda EvpB (Rao et al., 2004) is high (96.5%). The first step of our work was construction of the EiΔevpB strain by in-frame deletion of the evpB gene leaving 189 bp at the 5′ end and 132 bp at the 3′ end. Mutant construction did not introduce any selective antibiotics to the EiΔevpB strain. Introduction of extraneous antibiotic resistance in vaccine strains is not desirable to avoid spread of antibiotic resistance genes.
The EiΔevpB strain was completely avirulent in catfish fingerlings. Moreover, vaccination of fingerlings with EiΔevpB provided full protection against subsequent challenge with EiWT at 21 days post-vaccination. However, U.S. catfish production practices limit immersion vaccination to 7–14 days post-hatch. At this age, 1000s of fry are housed in hatchery tanks and can be vaccinated cost-effectively. To conform to industry practices, the EiΔevpB was assessed in 14-day-old fry by immersion at two different doses (106 and 107 CFU/ml in water). The result demonstrated that the EiΔevpB strain was completely attenuated in channel catfish fry at both doses. Following vaccination, we found 80.34% survival at the 107 CFU/ml in water dose and 34.24% survival at the 106 CFU/ml in water dose. These results demonstrate that 107 CFU/ml in water dose of EiΔevpB could provide excellent protection levels in catfish fry against ESC.
Immunization of 7-day-old catfish fry with increasing doses of EiΔevpB (106, 107, and 108 CFU/ml in water) demonstrated that the protection levels of EiΔevpB, although was not statistically significant, tend to be higher with increasing vaccine dose. Challenge doses as high as 108 CFU/ml in water were safe under our experimental conditions, and the higher dose of EiΔevpB elicited better protection against EiWT. However, subsequent immunizations were conducted using a dose of 107 CFU/ml in water, which is a more achievable dose for commercial vaccine manufacturing.
We also compared vaccine efficacy of EiΔevpB to the commercial vaccine Aquavac-ESC in channel catfish fingerlings and fry by immersion. In both trials, EiΔevpB provided better protection than Aquavac-ESC. Besides the superior performance of EiΔevpB compared to Aquavac-ESC, EiΔevpB does not have an added antibiotic resistance gene while Aquavac-ESC is rifampicin resistant. Also, EiΔevpB has a known genotype, while the genetic basis for Aquavac-ESC attenuation is not described.
The results from fry and fingerling experiments showed that evpB is vital in E. ictaluri virulence, which is consistent with findings in E. tarda PPD130/91, where deletion of evpB led to reduced virulence in blue gourami and impaired replication in gourami phagocytes (Rao et al., 2004). Several T6SS proteins are important for bacterial pathogenesis. However, the function of most T6SS proteins remains unknown (Filloux et al., 2008; Silverman et al., 2012). This is the first report to our knowledge that evpB is required for E. ictaluri virulence. This is also the first study that linked T6SS and virulence in E. ictaluri.
The results shown here suggest that deletion of evpB gene provides an excellent live attenuated vaccine candidate. Live attenuated bacterial vaccines activate immune responses by mimicking the route of natural infection, possess intrinsic adjuvant properties, and can be administrated as mucosal vaccines. In the present study, only the immersion route of exposure was tested, which is the preferred vaccination method in commercial settings, because large numbers of small fish can be vaccinated quickly and cheaply (Stevenson, 1997; Chettri et al., 2013).
Live attenuated vaccines must achieve a precise balance between lack of pathogenicity and sufficient immunogenicity to provide protective immunity. An important consideration for the success of vaccination in catfish fry is that the immune system of fry may not be fully developed (Ellis, 1988). Therefore, fry may not be able to respond to vaccination effectively. The protective immunity in channel catfish is determined by cellular immune mechanisms, which generally precedes the development of humoral immunity (Shoemaker et al., 1997; Thune et al., 1997; Petrie-Hanson and Ainsworth, 2001). In previous studies, it has been reported that catfish fry failed to produce a significant antibody response before 3 weeks of age due to the poor organization of secondary lymphoid tissue (Patrie-Hanson and Jerald Ainsworth, 1999; Petrie-Hanson and Ainsworth, 2001). This could elucidate why attempts of early vaccination of fry are less likely to succeed. However, our results showed that vaccination of 2-week-old catfish fry with EiΔevpB could provide very high protection. It is clear that use of EiΔevpB at three or 4-week-old fry may provide full protection against EiWT, but current catfish practices do not permit housing fry in hatcheries beyond 10–14 days.
Conclusion
In conclusion, EiΔevpB described in this work is entirely safe and provides full protection for catfish fingerlings. Also, EiΔevpB is safe and highly protective in catfish fry. Further, EiΔevpB is well characterized genetically and does not carry any additional antibiotic resistance. Field trials in earthen ponds will allow us to assess the commercial potential of EiΔevpB under production conditions. Understanding the responses of the catfish immune system to EiΔevpB vaccination will help us develop a better vaccination strategy.
Author Contributions
AK and ML supervised the study. HA, ML, and AK designed the experiments and analyzed and interpreted the data. HA and AK performed the experiments. All authors wrote and approved the manuscript.
Funding
This project was supported by Agriculture and Food Research Initiative competitive Grant Nos. 2014-70007-22359 and 2016-67015-24909 from the USDA National Institute of Food and Agriculture.
Conflict of Interest Statement
The authors declare that the research was conducted in the absence of any commercial or financial relationships that could be construed as a potential conflict of interest.
Acknowledgments
We thank Drs. Jingjun Lu and Hasan C. Tekedar for their technical assistance. We also thank Dr. Robert Wills for his assistance in statistical analysis.
References
Abdelhamed, H., Lu, J., Shaheen, A., Abbass, A., Lawrence, M. L., and Karsi, A. (2013). Construction and evaluation of an Edwardsiella ictaluri fhuC mutant. Vet. Microbiol. 162, 858–865. doi: 10.1016/j.vetmic.2012.11.006
Bebak, J., and Wagner, B. (2012). Use of vaccination against enteric septicemia of catfish and columnaris disease by the U.S. catfish industry. J. Aquat. Anim. Health 24, 30–36. doi: 10.1080/08997659.2012.667048
Bertolini, J. M., Cipriano, R. C., Pyle, S. W., and McLaughlin, J. J. A. (1990). Serological investigation of the fish pathogen Edwardsiella ictaluri, cause of enteric septicemia of catfish. J. Wildl. Dis. 26, 246–252. doi: 10.7589/0090-3558-26.2.246
Bingle, L. E., Bailey, C. M., and Pallen, M. J. (2008). Type VI secretion: a beginner’s guide. Curr. Opin. Microbiol. 11, 3–8. doi: 10.1016/j.mib.2008.01.006
Boyer, F., Fichant, G., Berthod, J., Vandenbrouck, Y., and Attree, I. (2009). Dissecting the bacterial type VI secretion system by a genome wide in silico analysis: what can be learned from available microbial genomic resources? BMC Genomics 10:104. doi: 10.1186/1471-2164-10-104
Cascales, E. (2008). The type VI secretion toolkit. EMBO Rep. 9, 735–741. doi: 10.1038/embor.2008.131
Chettri, J. K., Deshmukh, S., Holten-Andersen, L., Jafaar, R. M., Dalsgaard, I., and Buchmann, K. (2013). Comparative evaluation of administration methods for a vaccine protecting rainbow trout against Yersinia ruckeri O1 biotype 2 infections. Vet. Immunol. Immunopathol. 154, 42–47. doi: 10.1016/j.vetimm.2013.04.001
Dozois, C. M., Daigle, F., and Curtiss, R. III (2003). Identification of pathogen-specific and conserved genes expressed in vivo by an avian pathogenic Escherichia coli strain. Proc. Natl. Acad. Sci. U.S.A. 100, 247–252. doi: 10.1073/pnas.232686799
Dumpala, P. R., Peterson, B. C., Lawrence, M. L., and Karsi, A. (2015). Identification of differentially abundant proteins of Edwardsiella ictaluri during iron restriction. PLoS One 10:e0132504. doi: 10.1371/journal.pone.0132504
Ellis, A. E. (1988). “Ontogeny of the Immune System in Teleost Fish,” in Fish Vaccination, ed. A.E. Ellis (London: Academic Press), 20–31.
Filloux, A., Hachani, A., and Bleves, S. (2008). The bacterial type VI secretion machine: yet another player for protein transport across membranes. Microbiology 154, 1570–1583. doi: 10.1099/mic.0.2008/016840-0
Hawke, J. P. (1979). A Bacterium associated with disease of pond cultured channel catfish, Ictalurus punctatus. J. Fisheries Res. Board Can. 36, 1508–1512. doi: 10.1139/f79-219
Herrero, M., de Lorenzo, V., and Timmis, K. N. (1990). Transposon vectors containing non-antibiotic resistance selection markers for cloning and stable chromosomal insertion of foreign genes in Gram-negative bacteria. J. Bacteriol. 172, 6557–6567. doi: 10.1128/jb.172.11.6557-6567.1990
Ho, B. T., Dong, T. G., and Mekalanos, J. J. (2014). A view to a kill: the bacterial type VI secretion system. Cell Host Microbe. 15, 9–21. doi: 10.1016/j.chom.2013.11.008
Horton, R. M., Cai, Z. L., Ho, S. N., and Pease, L. R. (1990). Gene splicing by overlap extension: tailor-made genes using the polymerase chain reaction. Biotechniques. 8, 528–535.
Klesius, P. H., and Shoemaker, C. A. (1997). Heterologous isolates challenge of channel catfish, Ictalurus punctatus, immune to Edwardsiella ictaluri. Aquaculture 157, 147–155. doi: 10.1016/S0044-8486(97)00076-8
Klesius, P. H., and Shoemaker, C. A. (1999). Development and use of modified live Edwardsiella ictaluri vaccine against enteric septicemia of catfish. Adv. Vet. Med. 41, 523–537. doi: 10.1016/S0065-3519(99)80039-1
Kovach, M. E., Elzer, P. H., Hill, D. S., Robertson, G. T., Farris, M. A., Roop, R. M., et al. (1995). Four new derivatives of the broad-host-range cloning vector pBBR1MCS, carrying different antibiotic-resistance cassettes. Gene 166, 175–176. doi: 10.1016/0378-1119(95)00584-1
Lawrence, M. L., Cooper, R. K., and Thune, R. L. (1997). Attenuation, persistence, and vaccine potential of an Edwardsiella ictaluri purA mutant. Infect. Immun. 65, 4642–4651.
Lin, J. S., Ma, L. S., and Lai, E. M. (2013). Systematic dissection of the agrobacterium type VI secretion system reveals machinery and secreted components for subcomplex formation. PLoS One 8:e67647. doi: 10.1371/journal.pone.0067647
Miller, V. L., and Mekalanos, J. J. (1988). A novel suicide vector and its use in construction of insertion mutations: osmoregulation of outer membrane proteins and virulence determinants in Vibrio cholerae requires toxr. J. Bacteriol. 170, 2575–2583. doi: 10.1128/jb.170.6.2575-2583.1988
Moore, M. M., Fernandez, D. L., and Thune, R. L. (2002). Cloning and characterization of Edwardsiella ictaluri proteins expressed and recognized by the channel catfish Ictalurus punctatus immune response during infection. Dis. Aquat. Organ. 52, 93–107. doi: 10.3354/dao052093
Murdoch, S. L., Trunk, K., English, G., Fritsch, M. J., Pourkarimi, E., and Coulthurst, S. J. (2011). The opportunistic pathogen Serratia marcescens utilizes type VI secretion to target bacterial competitors. J. Bacteriol. 193, 6057–6069. doi: 10.1128/JB.05671-11
Patrie-Hanson, L., and Jerald Ainsworth, A. (1999). Humoral immune responses of channel catfish (Ictalurus punctatus) fry and fingerlings exposed to Edwardsiella ictaluri. Fish Shellfish Immunol. 9, 579–589. doi: 10.1006/fsim.1999.0215
Petrie-Hanson, L., and Ainsworth, A. J. (2001). Ontogeny of channel catfish lymphoid organs. Vet. Immunol. Immunopathol. 81, 113–127. doi: 10.1016/S0165-2427(01)00331-2
Plumb, J. A., Sheifinger, C. C., Shryock, T. R., and Goldsby, T. (1995). Susceptibility of six bacterial pathogens of channel catfish to six antibiotics. J. Aquat. Anim. Health 7, 211–217. doi: 10.1577/1548-8667(1995)007<0211:SOSBPO>2.3.CO;2
Plumb, J. A., and Vinitnantharat, S. (1989). Biochemical, biophysical, and serological homogeneity of Edwardsiella ictaluri. J. Aquat. Anim. Health 1, 51–56. doi: 10.1577/1548-8667(1989)001<0051:BBASHO>2.3.CO;2
Pukatzki, S., Ma, A. T., Sturtevant, D., Krastins, B., Sarracino, D., Nelson, W. C., et al. (2006). Identification of a conserved bacterial protein secretion system in Vibrio cholerae using the Dictyostelium host model system. Proc. Natl. Acad. Sci. U.S.A. 103, 1528–1533. doi: 10.1073/pnas.0510322103
Rao, P. S., Yamada, Y., Tan, Y. P., and Leung, K. Y. (2004). Use of proteomics to identify novel virulence determinants that are required for Edwardsiella tarda pathogenesis. Mol. Microbiol. 53, 573–586. doi: 10.1111/j.1365-2958.2004.04123.x
Shoemaker, C. A., Klesius, P. H., Evans, J. J., and Arias, C. R. (2009). Use of modified live vaccines in aquaculture. J. World Aquac. Soc. 40, 573–585. doi: 10.1111/j.1749-7345.2009.00279.x
Shoemaker, C. A., Klesius, P. H., and Plumb, J. A. (1997). Killing of Edwardsiella ictaluri by macrophages from channel catfish immune and susceptible to enteric septicemia of catfish. Vet. Immunol. Immunopathol. 58, 181–190. doi: 10.1016/S0165-2427(97)00026-3
Shotts, E. B., Blazer, V. S., and Waltman, W. D. (1986). Pathogenesis of Experimental Edwardsiella ictaluri Infections in Channel Catfish (Ictalurus punctatus). Can. J. Fish. Aquat. Sci. 43, 36–42. doi: 10.1139/f86-005
Silverman, J. M., Brunet, Y. R., Cascales, E., and Mougous, J. D. (2012). Structure and regulation of the type VI secretion system. Annu. Rev. Microbiol. 66, 453–472. doi: 10.1146/annurev-micro-121809-151619
Smith, P., Hiney, M. P., and Samuelsen, O. B. (1994). Bacterial resistance to antimicrobial agents used in fish farming: a critical evaluation of method and meaning. Annu. Rev. Fish Dis. 4, 273–313. doi: 10.1016/0959-8030(94)90032-9
Stevenson, R. M. (1997). Immunization with bacterial antigens: yersiniosis. Dev. Biol. Stand. 90, 117–124.
Thune, R. L., Collins, L. A., and Penta, M. P. (1997). A Comparison of immersion, immersion/oral combination and injection methods for the vaccination of channel catfish Ictalurus punctatus Against Edwardsiella ictaluri. J. World Aquac. Soc. 28, 193–201. doi: 10.1111/j.1749-7345.1997.tb00856.x
Villumsen, K. R., Neumann, L., Ohtani, M., Strom, H. K., and Raida, M. K. (2014). Oral and anal vaccination confers full protection against enteric redmouth disease (ERM) in rainbow trout. PLoS One 9:e93845. doi: 10.1371/journal.pone.0093845
Williams, M. L., Gillaspy, A. F., Dyer, D. W., Thune, R. L., Waldbieser, G. C., Schuster, S. C., et al. (2012). Genome sequence of Edwardsiella ictaluri 93-146, a strain associated with a natural channel catfish outbreak of enteric septicemia of catfish. J. Bacteriol. 194, 740–741. doi: 10.1128/JB.06522-11
Wise, D. J., Camus, A., Schwedler, T., and Terhune, J. (2004). “Health management,” in Biology and Culture of the Channel Catfish, eds C. S. Tucker and J. A. Hargreaves (Amsterdam: Elsevier), 482–488.
Keywords: Edwardsiella ictaluri, type six secretion sysytem, T6SS, evpB, live attenuated vaccine
Citation: Abdelhamed H, Lawrence ML and Karsi A (2018) Development and Characterization of a Novel Live Attenuated Vaccine Against Enteric Septicemia of Catfish. Front. Microbiol. 9:1819. doi: 10.3389/fmicb.2018.01819
Received: 05 March 2018; Accepted: 19 July 2018;
Published: 07 August 2018.
Edited by:
Martin Stephen Llewellyn, University of Glasgow, United KingdomReviewed by:
Hetron Mweemba Munang’andu, Norwegian University of Life Sciences, NorwayPamela Del Carmen Mancha-Agresti, Universidade Federal de Minas Gerais, Brazil
Copyright © 2018 Abdelhamed, Lawrence and Karsi. This is an open-access article distributed under the terms of the Creative Commons Attribution License (CC BY). The use, distribution or reproduction in other forums is permitted, provided the original author(s) and the copyright owner(s) are credited and that the original publication in this journal is cited, in accordance with accepted academic practice. No use, distribution or reproduction is permitted which does not comply with these terms.
*Correspondence: Attila Karsi, karsi@cvm.msstate.edu