- 1Beijing Advanced Innovation Center for Food Nutrition and Human Health, College of Food Science and Nutritional Engineering, China Agricultural University, Beijing, China
- 2Beijing Fisheries Research Institute, Beijing, China
- 3Beijing Laboratory for Food Quality and Safety, China Agricultural University, Beijing, China
- 4School of Life Sciences, Inner Mongolia University, Hohhot, China
Sturgeon is an important fresh water-culture fish in China. A problem with sturgeon is its high susceptibility to spoilage. Food spoilage is reported to be regulated by quorum sensing (QS). To identify the QS signals acetylated homoserine lactones (AHLs) in sturgeon and test whether QS plays a role in the spoilage of sturgeon, we investigated the specific spoilage organisms (SSOs) in vacuum packaged sturgeon stored at 4°C and the production of AHLs by sturgeon SSOs. 16S rDNA sequencing and spoilage capabilities analysis revealed that Aeromonas veronii LP-11, Citrobacter freundii LPJ-2, and Raoultella ornithinolytica LPC-3 were the SSOs in sturgeon. Among the three SSOs, only A. veronii LP-11 induced the QS biosensors Agrobacterium tumefaciens KYC55 and Chromobacterium violaceum CV026, suggesting that it produced AHLs. Analysis by thin layer chromatography, high-performance liquid chromatography-triple quadrupole tandem mass spectrometry, and high-performance liquid chromatography quadrupole time-of-flight mass spectrometry (HPLC/qTOF-MS) identified that the AHLs produced by A. veronii were C6-SHL, C8-HSL, 3-oxo-C8-HSL, and 3-OH-C8-HSL. Our study revealed that QS system was probably involved in the regulation of sturgeon spoilage and for the first time reported the production of C8-HSL and 3-OH-C8-HSL by genus Aeromonas. As only HPLC/qTOF-MS effectively and accurately identified all the four AHLs produced by A. veronii LP-11, this study also showed that HPLC/qTOF-MS was the most efficient method for rapid analysis of AHLs in complex microbial sample. The study provides new insight into the microbiology of sturgeon spoilage which may be helpful for better sturgeon preservation.
Introduction
Sturgeon is economically one of the most important fresh water-cultured fish in China, of which the production in China takes up to 80% of the total production in the world. One problem with sturgeon is its high susceptibility to spoilage due to the high water content and nutrients. For this reason, refrigeration and vacuum packing are commonly used for the storage and transportation of sturgeon products. Growth of microorganism is the major cause of fish spoilage, which leads to the production of biogenic amines, organic acids, sulfides, alcohols, aldehydes, ketones, and unpleasant off-flavors (Gram and Dalgaard, 2002). The microflora that produces undesirable metabolites and are responsible for food spoilage are called specific spoilage organism (SSO; Gram et al., 2002).
Quorum sensing (QS) is a widespread process in bacteria that employs autoinducing chemical signals to coordinate diverse activities such as bioluminescence, biofilm formation, and exoenzyme secretion (Schuster et al., 2013). QS affects the microflora ecosystem in food, which may finally impacts the sensory quality and safety of food (Rul and Monnet, 2015). Thus, it is likely that QS plays a role in the spoilage of aquatic products. In fact, it has been reported that the secretion of a number of microbial extracellular enzymes, including lipases, nuclease, and protease, is regulated by QS (Skandamis and Nychas, 2012). In addition, QS signals have been detected in a variety of different spoiled commercial aquatic products, such as cold-smoked fish fillets, packed or refrigerated fish, and shrimp (Christensen et al., 2003; Flodgaard et al., 2005; Bai and Rai, 2011; Zhu et al., 2015, 2016). Acetylated homoserine lactones (AHLs) are utilized by a diverse range of Gram-negative bacteria as QS signals. N-(3-oxo-hexanoyl)-L-homoserine lactone (3-oxo-C6-HSL) which belongs to AHLs has been determined to be a QS signal in Serratia proteamaculans B5a isolated from spoiled cold-smoked salmon to modulate proteolytic activity of the strain, showing the involvement of QS in spoilage of fish (Christensen et al., 2003). AHLs are also detected in Shewanella putrefaciens, the SSO of refrigerated shrimp (Zhu et al., 2015). Taken together, these reports demonstrate that AHL-based QS system in Gram-negative bacteria is involved in the spoilage of some aquatic products. Using AHLs, lactonase which degrades AHLs could inhibit the spoilage progress of vacuum-packaged sturgeon stored at 4°C, indicating that QS signals AHLs are implicated in sturgeon spoilage (Gui et al., 2017). However, the mechanism of QS in sturgeon spoilage and their precise role in spoilage is still not clear.
To understand the role of QS in food spoilage, it is necessary to identify the QS signal molecules. As QS can be activated by very low level of AHLs for some strains (Mengsheng et al., 2005), the sensitivity of the analysis method should be very high to detect trace amounts of AHLs produced by the strain. AHL-sensitive bioassay is a convenient method to detect AHLs. Some high-sensitive AHL biosensors, such as Chromobacterium violaceum, Agrobacterium tumefaciens (TraR), and Pseudomonas aeruginosa (LasR), have been developed (Gould et al., 2006). However, there is an inherent detection bias of this method because the analysis relies on the specificity of the reporter strain (Steindler and Venturi, 2007). For this reason, mass spectrometry (MS) is increasingly being applied for the detection and identification of QS signals. MS is currently the most versatile method for rapid analysis of AHLs in complex microbial samples (Cataldi et al., 2013). With the development of MS technology, high-resolution mass spectrometry (HRMS) is becoming feasible and necessary for confident and contemporary QS analysis for it providing highly selective chemical information, such as accurate mass, isotope distribution, and characteristic fragmentation. So far, quadrupole time-of-flight (qTOF) MS as a representative HRMS has been successfully used to detect AHLs in Pseudomonas putida IsoF cell culture supernatant (Cataldi et al., 2013).
This objective of this study is to identify the AHLs in SSOs of sturgeon and test whether QS plays a role in the spoilage of sturgeon. For this purpose, we first identified the SSOs of vacuum packaged sturgeon stored at 4°C and then examined the presence of AHLs in SSO culture by different methods.
Materials and Methods
Strains and Culture Conditions
Agrobacterium tumefaciens KYC55 (pJZ372)(pJZ384)(pJZ410) (Zhu et al., 2003) and Chromobacterium violaceum CV026 (McClean et al., 1997) were kindly offered by Zhigang Zhou (Chinese Academy of Agricultural Sciences, Beijing, China) and used for the detection of AHLs as previous described (Ravn et al., 2001) A. tumefaciens KYC55 was grown in LB medium supplemented with 1 μg/mL tetracycline,100 μg/mL tetracycline, and 100 μg/mL spectinomycin. C. violaceum CV026 was gown in LB medium supplemented with 20 μg/mL kanamycin. N-butyryl-L-homoserine lactone (C4-HSL), N-hexanoyl-L-homoserine lactone (C6-HSL), N-octanoyl-L-homoserine lactone (C8-HSL), N-(3-oxohexanoyl)-D-homoserine lactone (3-oxo-C8-HSL), and N-(3-hydroxydodecanoyl)-DL-homoserine lactone (3-OH-C8-HSL) were purchased from Sigma-Aldrich (St. Louis, MO, United States). 5-bromo-4-chloro-3-indolyl-β-D-galactopyranoside (X-gal) was bought from Promega (Madison, WI, United States). All other chemicals were analytical grade.
Isolation and Identification of the Dominant Bacteria
Psychrotrophs, Enterobacteriaceae, and Aeromonas were identified as the dominant spoilage bacteria of vacuum packaged minced sturgeon (Acipenser schrenckii) stored at 4°C (Gui et al., 2014). Psychrotrophs were isolated using plate count agar (PCA, code HB0101, Qingdao Hope Biol-Technology Co., Ltd., China) after culture at 7°C for 7 days. Enterobacteriaceae were isolated using violet red bile glucose agar (VRBGA, code HB0176) after culture at 37°C for 24 h. Aeromonas were isolated using ampicillin macconey agar base (AMA, code HB8576) after culture at 28°C for 2 days; 32, 25, and 30 colonies of Psychrotrophs, Enterobacteriaceae, and Aeromonas, respectively, were selected and purified in nutrient agar plates by streaking, and then identified by 16S rDNA sequencing. For the sequencing, bacterial genomic DNA was extracted using Bacteria Genomic DNA extraction kit (Tiangen, Beijing, China). 16S rDNA was amplified by PCR using primers 27F (5′-AGAGTTTGATCCTGGCTCAG-3′) and 1495r (5′-CTACGGCTACCTTGTTACGA-3′). The PCR program was as follows: 30 cycles of denaturation at 94°C for 1 min, annealing at 52°C for 30 s, and extension at 72°C for 1.5 min, with a final elongation step at 72°C for 5 min. The PCR products were sequenced by the Beijing Biomed Biological Technology Co., Ltd. (Beijing, China). The sequences of 16S rDNA were searched against database GenBank by BLAST.
Spoilage Ability Analysis
Twelve strains of dominant spoilage bacteria, which were list as Q1–Q4, C1–C4, and N1–N4 (Table 1), were inoculated into nutrient broth (at 1% inoculum size) and cultured at 30°C for 12–18 h to reach 109 CFU/mL. Bacteria were collected by centrifugation at 12,000 g, 4°C for 10 min, resuspended in sterile saline solution, and diluted to 1.0 × 108 CFU/mL; 400 mL of sterile fish muscle juice was inoculated with 20 μL of prepared bacteria and stored at 4°C to evaluate the spoilage ability of inoculated bacteria. Spoilage ability was evaluated by the microbial growth, total volatile base nitrogen (TVB-N) and sensory score. The sturgeon muscle juice was prepared according to Gu et al. (2013). TVB-N was measured as previously described (Gui et al., 2014) and expressed as g TVB-N per kg minced sturgeon muscle. Quantitative descriptive analysis (QDA) was applied to evaluate the flavor of sturgeon juice. Seven trained people were selected for the sensory panel. Surgeon juice was scored on a 0–7 point scale with 6–7 meaning fresh, 4–6 no distinct odor, 2–4 incipient rancidity, and 0–2 rancid.
Screening of AHL-Producing Bacteria by Biosensors
Agrobacterium tumefaciens KYC55 (pJZ372)(pJZ384)(pJZ410) and Chromobacterium violaceum CV026 were used for the detection of AHLs in “T” streak assay as previously described by Piper et al. (1993).
Extraction of Homoserine Lactones From Culture Supernatants
Aeromonas veronii LP-11 was cultured in LB at 30°C, 200 rpm for 24 h. And AHLs were extracted from the supernatant of culture using method previously described (Ravn et al., 2001) with some modifications. A sample (600 mL) was centrifuged (4°C, 12,000 g for 10 min) and passed through 0.22 μm filter to remove cells. The supernatants were extracted three times with equal volumes of acidified ethylacetate (0.1% formic acid). The combined extract was first dried at 30°C using a rotatory evaporator .The small amount of residual liquid was dried by nitrogen gas flow. Residue was dissolved in 2 mL of HPLC-grade methanol and stored at −20°C until analysis.
Thin Layer Chromatography (TLC) Analysis of AHLs
Acetylated homoserine lactones extract was examined by thin layer chromatography (TLC) as described by Ravn et al. (2001) with some modifications. Briefly, 2 μL of AHL extract or synthetic AHL standards was applied to a 10 cm × 10 cm sizes C18 TLC plate (TLC aluminum sheets 20 cm × 20 cm, RP-18 F254S, Merck 64271 Darmstadt, Germany) and developed in 60:40 (v/v) methanol/water until the front reached the top. The TLC plates were dried for at least 10 min while an agar cover layer of either C. violaceum CV026 or A. tumefaciens KYC55 was prepared. To prepare the agar top layer, 0.5 mL of preculture of C. violaceum CV026 or A. tumefaciens KYC55 was inoculated into 50 mL LB medium and grown at 30°C for 24 h with aeration. The culture was then added with 100 mL of LB-agar (1.2% agar) and immediately poured onto the TLC plates. After the agar was solidified, the TLC plates were transferred to a sealed sterile container and cultured at 30°C for 48 h. AHL standards used included C4-HSL, C6-HSL, C8-HSL, and 3-oxo-C8-HSL.
HPLC/QqQ-MS Analysis of AHLs
HPLC/QqQ-MS analysis of AHLs extract was performed as described by Gould et al. (2006) with some modification; 10 μL of AHL extract was injected onto a C18 reverse-phase column (2.0 mm × 150.0 mm, Agilent). Mobile phase A was H2O containing 0.1% glacial acetic acid. Mobile phase B was methanol containing 0.1% glacial acetic acid. Elution started with 10% mobile phase B for 5 min, which then raised to 90% over 30 min and stayed at 90% for 15 min. The column was re-equilibrated for 10 min, and a blank run was performed between each analysis. The flow rate, column temperature and UV detection wavelength was 200 μL/min, 30°C, and 210 nm, respectively.
Mass spectrometric analysis was performed on Agilent 6460 triple-quadrupole tandem mass spectrometer. Precursor ion-scanning experiments were performed in positive-ion mode to monitor for m/z 102 in the range of m/z 50–400. The ion spray voltage, focusing potential, and collision energy were set at 4200, 200, and 25 V, respectively. To verify the AHLs detected in A. veronii extract, AHL reference standards including C6-HSL, C8-HSL, and 3-oxo-C8-HSL were analyzed in the same way.
HPLC/QTOF-MS Analysis
10 μL sample was injected onto an Agilent SB-C18 column (2.1 mm × 50.0 mm, Agilent) and eluted at a flow rate of 400 μL/min. Mobile phase A was H2O containing 0.1% formic acid. Mobile phase B was acetonitrile containing 0.1% formic acid. Elution started with 10% mobile phase B for 3 min, which then raised to 100% over 21 min, stayed at 100% for 2 min, reduced to 10% over 0.1 min, and stayed at 10% for 3.9 min. The column temperature and UV detection wavelength were 30°C and 210 nm, respectively. Mass spectrometric analysis was performed on a Waters XEVO-G2 QTOF of a quadrupole and orthogonal acceleration time-of-flight tandem mass spectrometer. The scan range was m/z 50–600. For positive-ion mode, the capillary and cone voltage were set at 3.5 kV and 40 V, respectively. The desolvation gas was set to 600 L/h at a temperature of 450°C. The ion source temperature was set to 110°C.All data were acquired and processed using Waters MassLynxv4.1 software.
Data Analysis
Statistical tests were performed using the SPSS 20.0 computer program (SPSS Statistical Software, Inc., Chicago, IL, United States). Data were reported as mean ± SDs of three measurements. Means were subjected to Duncan’s test and a p-value of <0.05 was considered significant.
Results
Identification of SSOs
A total of 87 strains of dominant spoilage bacteria were selected from selective mediums culture and subjected to identification by 16s rDNA sequencing. Totally, 13 species were identified from the 87 selected strains (Table 1). One strain from each of 12 most common identified species was selected for spoilage ability analysis. The selected 12 stains listed as Q1–Q4, C1–C4, and N1–N4 (Table 1) were inoculated into sterile sturgeon muscle juice. Viable counts, TVB-N values, and flavor of sturgeon muscle juice during storage were determined or evaluated. As shown in Figure 1, the viable count of Q1 rapidly exceeded 8 log CFU/g on day 4, which was significantly higher (P < 0.05) than those of all the other groups. At the end of the storage, the viable counts of Q1, N3, C1, and C4 became significantly higher (P < 0.05) than those of the others. The sensory scores of Q1, C1, and C4 were significantly lower (P < 0.05) than those of the other groups on day 6. In addition, the TVB-N values of Q1 increased rapidly after 2 days of storage and became significantly higher (P < 0.05) than those of all the other groups from day 4 to day8. On the final day 10 of storage, the TVB-N values of Q1, C1, and C4 increased to over 40 mg N/100 mL which were significantly higher (P < 0.05) than those of the other groups. Based on these data, it can be concluded that the spoilage ability of Q1, C1, and C4 was higher than that of the other tested strains. Strains Q1, C1, and C4 were classified as the SSOs of sturgeon in the present study and named as Aeromonas veronii LP-11, C. freundii LPJ-2, and R. ornithinolytica LPC-3, respectively.
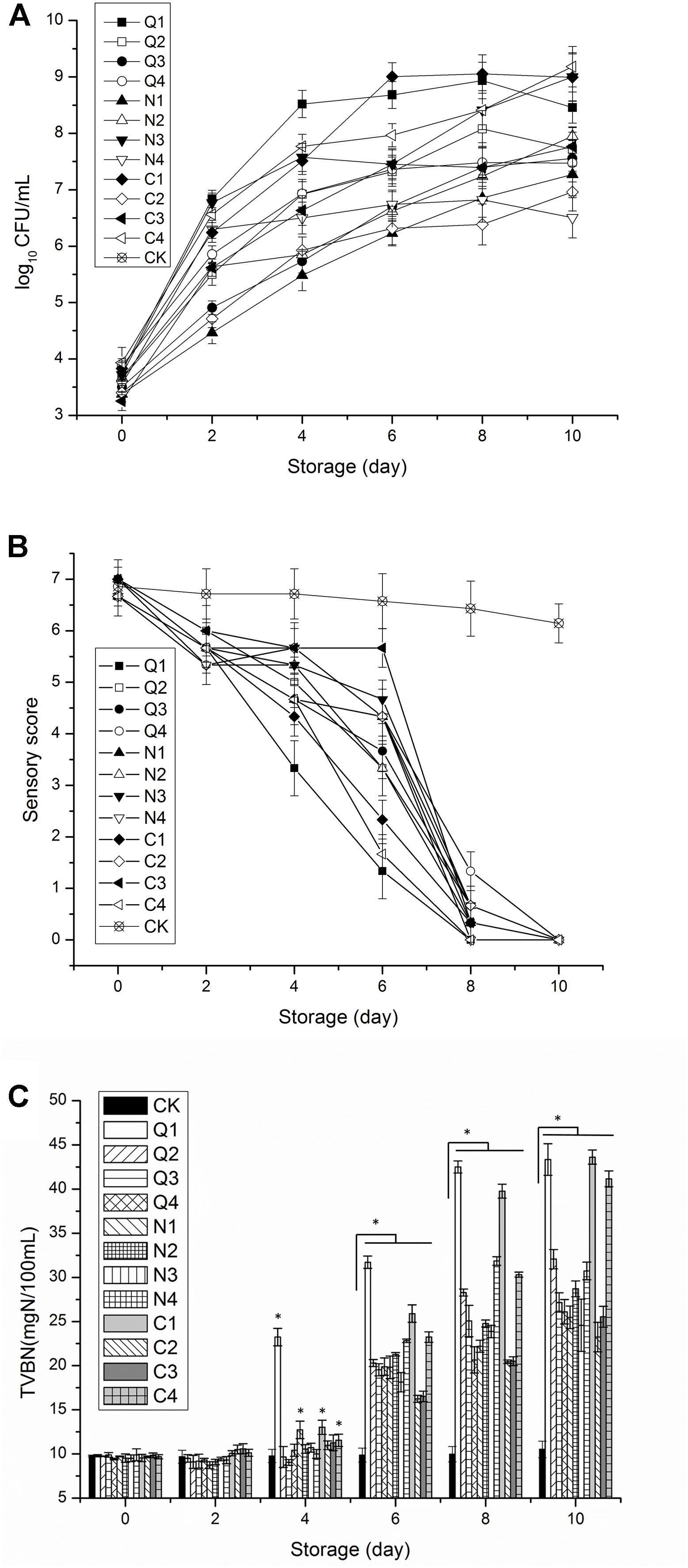
FIGURE 1. Comparison of spoilage capabilities of sterile fish juice inoculated with different bacteria (Q1–Q4, N1–N4, and C1–C4) during the storage at 4°C. (A) Total viable count, (B) sensory score, and (C) total volatile base nitrogen (TVB-N). The error bars indicate standard deviation (±SD). Statistical significance was marked by the asterisk (* represents P < 0.05).
Biosensor Analysis
Acetylated homoserine lactone biosensor C. violaceum CV026 responds well to AHLs with four to eight carbon acyl side chains but responds very poorly to AHLs with acyl chains of C10 or more (Steindler and Venturi, 2007). Another AHL biosensor A. tumefaciens KYC55 is sensitive to 3-oxo-substituted AHLs with acyl chain of lengths from 4 to 12 and 3-unsubstituted AHLs (Zhu et al., 2003). As each biosensor preferentially responds to AHLs with specific structure, using the two biosensors in combination can enlarge the detection range of AHLs. A. veronii LP-11 was able to induce violacein production in C. violaceum CV026 and β-galactosidase production in A. tumefaciens KYC55 (Figures 2A,B). The color of the two AHL biosensors was in gradient, with most strong response observed at the meeting point of the two strains, showing AHL production by A. veronii. In contrast, C. freundii LPJ-2 and R. ornithinolytica LPC-3 did not induce the QS phenomenon in the two biosensors (data was not shown). The AHLs produced by A.veronii LP-11 were further identified in the following study.
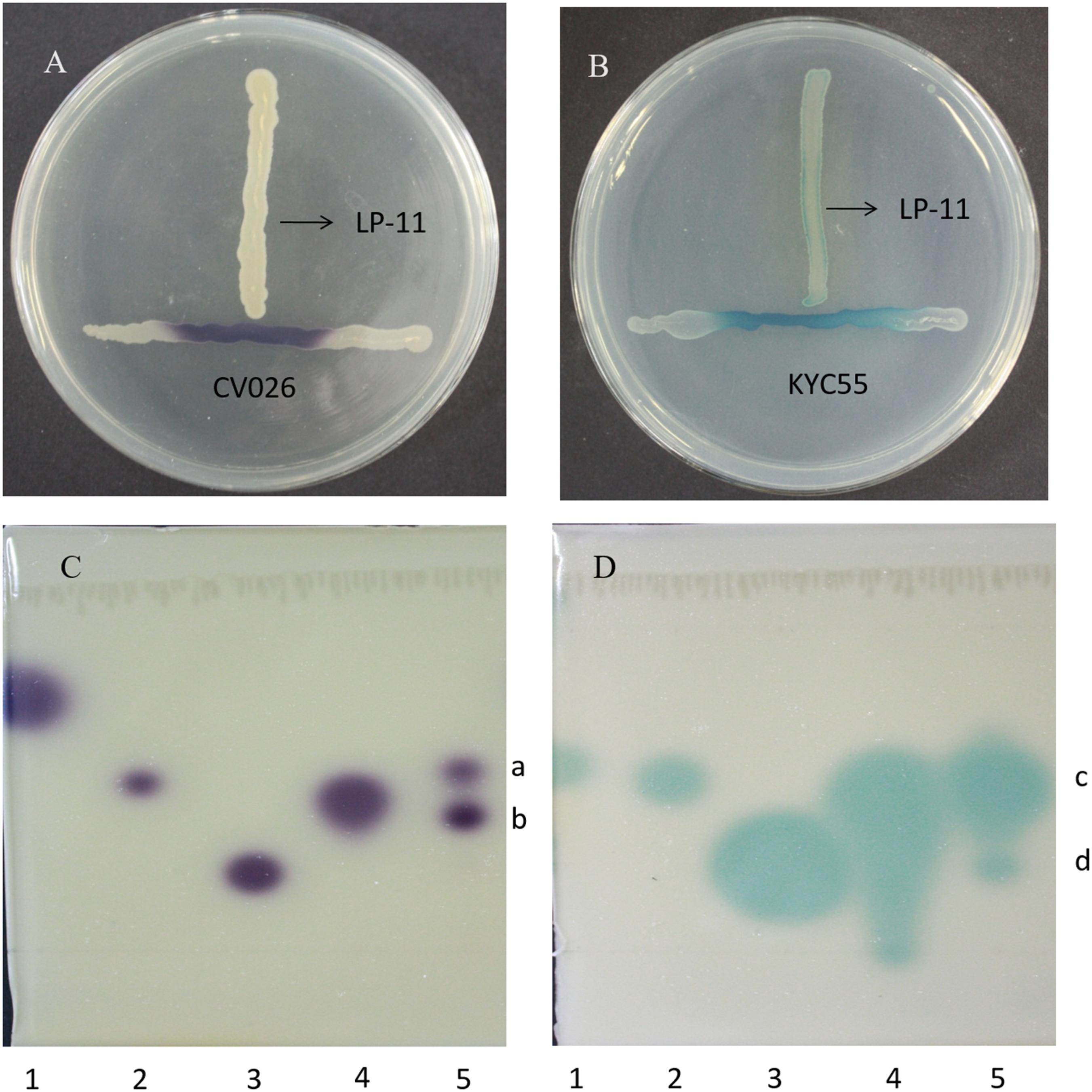
FIGURE 2. The detection of AHLs in A. veronii LP-11 in ‘T’ streak assay using biosensor (A) A. tumefaciens KYC55 and (B) C. violaceum CV026 and the detection of AHLs in A. veronii LP-11 extract by TLC using biosensor (C) A. tumefaciens KYC55 and (D) C. violaceum CV026. Lane 1, standard C4-HSL; lane 2, standard C6-HSL; lane 3, standard C8-HSL; and lane 4, standard 3-oxo-C8-HSL; and lane 5, A. veronii LP-11 extract.
TLC-Biosensor Analysis
Acetylated homoserine lactones can be partially characterized by TLC on C18 reversed-phase plates. Each AHL migrates with a characteristic mobility and results in a spot shape of response detected in a way depending on the reporter of the biosensor strain (Steindler and Venturi, 2007). A. veronii LP-11 induced two spots in the TLC plate coupled with A. tumefaciens KYC55 (Figure 2C). Spots a and b had similar mobility and shape as those of 3-oxo-C8-HSL and C8-HSL, respectively. At the same time, A. veronii LP-11 inducted two spots in the TLC plate coupled with C. violaceum CV026 (Figure 2D). The Rf value of spot c (∼0.49) was similar to that of the short chain AHL standard C6-HSL (Rf∼0.49), while the Rf value of spot d (∼0.37) was smaller than that of 3-oxo-C8-HSL (Rf∼0.41). From these results, it can be concluded that spots a, b, and c were 3-oxo-C8-HSL, C8-HSL and C6-HSL, respectively, but spot d was an unidentified AHL; these results demonstrated that at least four different AHLs were synthesized by A. veronii LP-11.
HPLC/QqQ-MS Analysis
To accurately identify the AHLs species synthesized by A. veronii LP-11, A. veronii LP-11 extract was analyzed by HPLC/ QqQ-MS. The ions used to identify AHLs are m/z 102, which corresponding to the loss of the acyl chain, and the corresponding m/z molecular ion (Morin et al., 2003). As shown in Figure 3, three AHLs were identified by HPLC/QqQ-MS. The prominent peak at m/z 242.8, 244.7, and 228.7 corresponded to the molecular ion [M + H]+ of 3-oxo-C8-HSL, 3-OH-C8-HSL, and C8-HSL, respectively. In addition, the specific retention times of molecular ions of m/z 244.2, 244.7, and 228.7 were in accordance with standards 3-oxo-C8-HSL (26.902 min), 3-OH-C8-HSL (27.678 min), and C8-HSL (32.932 min), respectively. Therefore, the molecular weight, retention time, and characteristic ion m/z 102 consistently confirmed the presence of 3-oxo-C8-HSL, 3-OH-C8-HSL, and C8-HSL in A. veronii LP-11 extract.
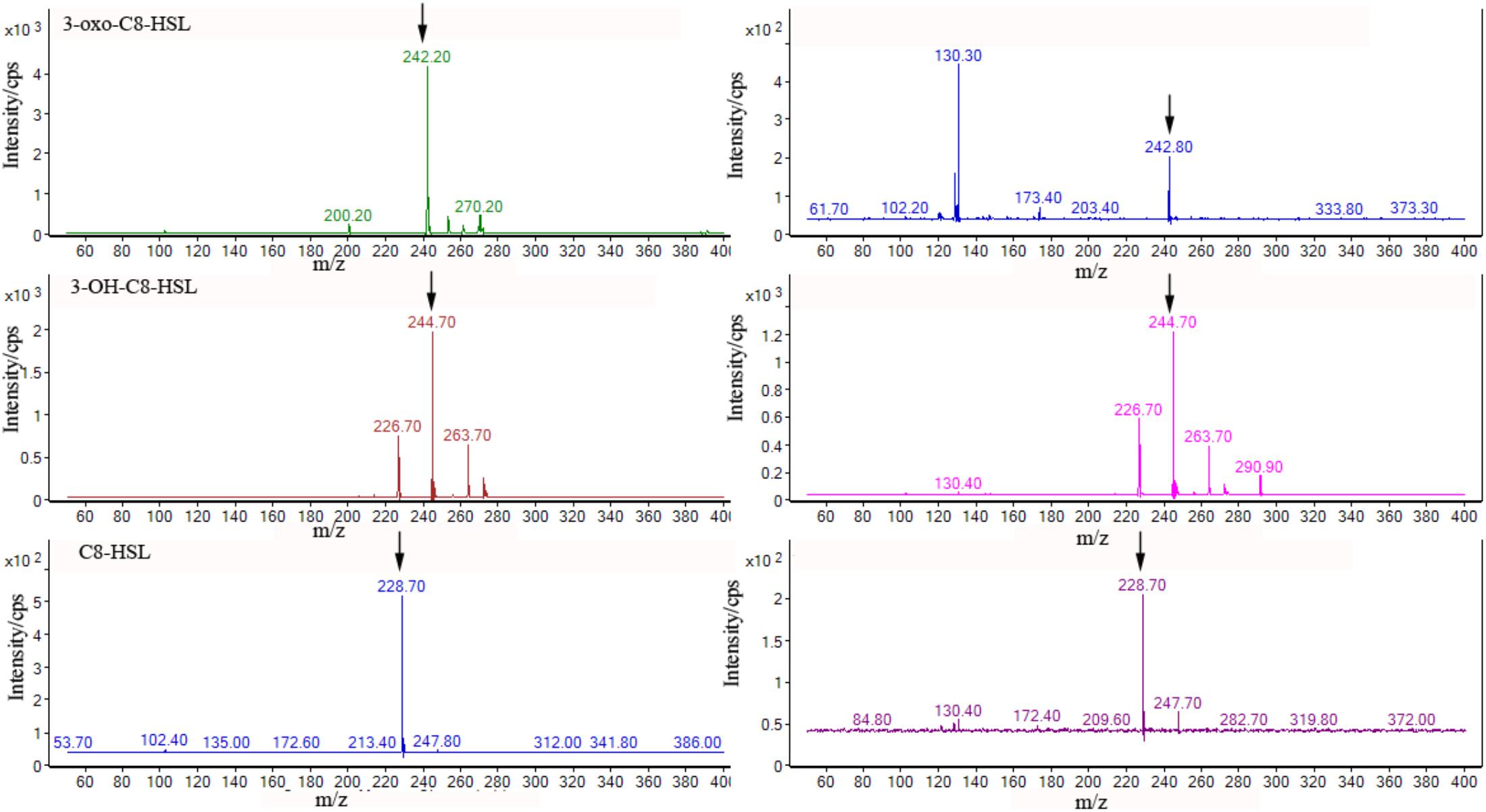
FIGURE 3. Product ion spectrum of AHLs standards (Left) and the corresponding peak (Right) in the chromatograms of A. veronii LP-11 extract.
HPLC/QTOF-MS Analysis
Thin layer chromatography analysis indicated that four different AHLs might present in A. veronii LP-11 extract, while QqQ-MS detected only three AHLs. To clarify the discrepancy, qTOF-MS with both high sensitivity and high mass accuracy for both precursor and product ions (Cataldi et al., 2013) was used to search the signaling molecules. The prominent precursor ions [M + H]+ at m/z 244.153, 242.1375, and 228.1579 in MS1 spectra corresponded to 3-OH-C8-HSL, 3-oxo-C8-HSL, and C8-HSL, respectively. Their intense characteristic protonated homoserine lactone product ion m/z 102 was observed in the MS2 spectra (Figures 4C–F). However, the precursor ions [M + H]+ at m/z 200.1269 which corresponded to C6-HSL were in low abundance in the MS1 spectra and no obvious characteristic product ions at m/z 102 were present in the MS2 spectra (Figures 4A,B). In order to confirm the presence of C6-HSL in the sample, standard C6-HSL was analyzed by the same method and the results showed that their retention time was the same, indicating the four compounds in Figure 4 were 3-OH-C8-HSL, 3-oxo-C8-HSL, and C8-HSL and C6-HSL, respectively. In addition, the typical mass errors of the four compounds were below 2.1 mDa and the elementary compositions of the four compounds calculated by Marker Lynx XS software were consistent with their predicted molecular formulas (Table 2), which substantiated the presence of the four AHLs in A. veronii LP-11extract.
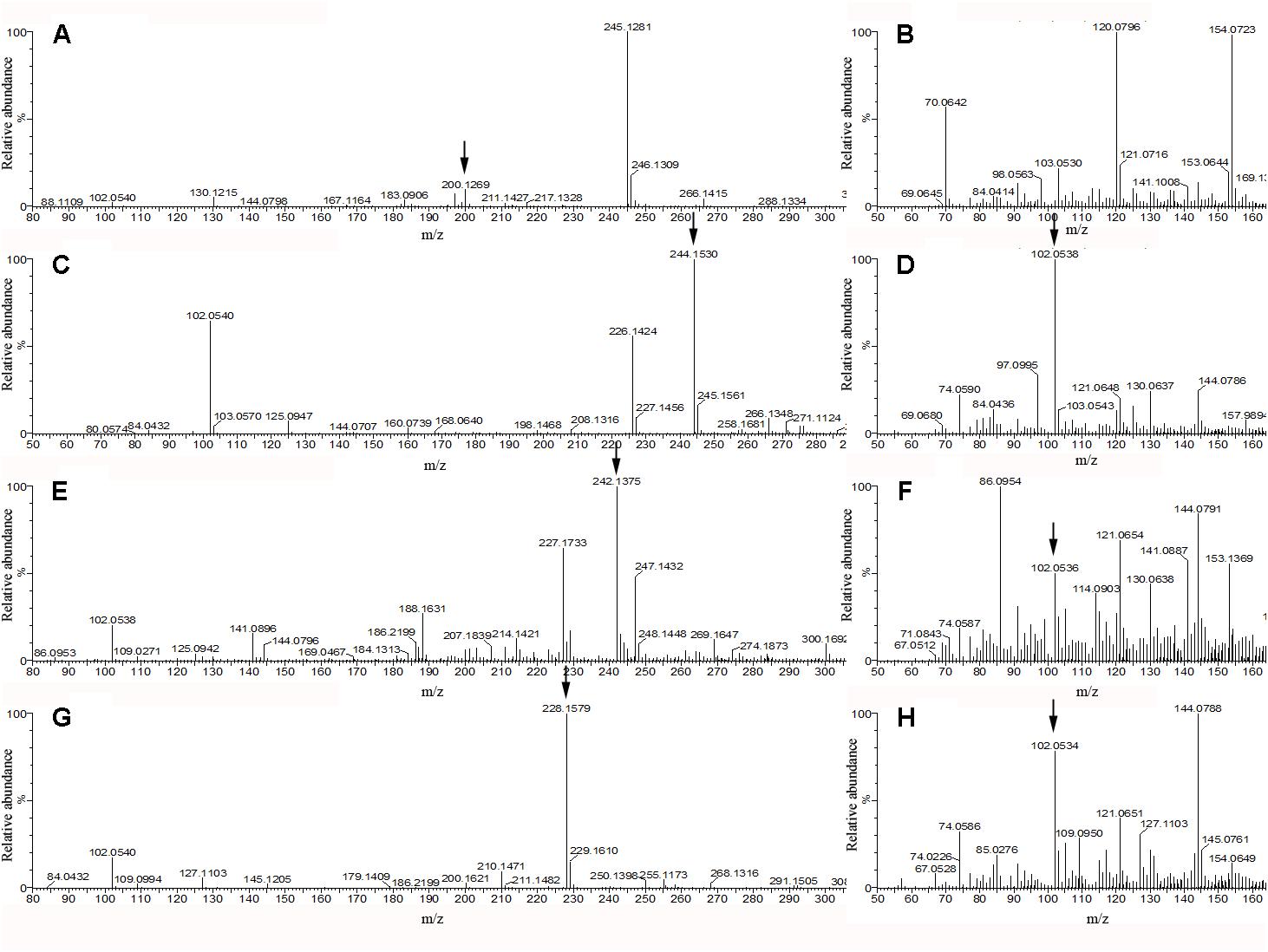
FIGURE 4. QTOF-MS spectra of four AHLs of A. veronii LP-11 extract. Panels (A,B) were the MS1 spectra and MS2 spectra, respectively of m/z 200.1269 ion; panels (C,D) were the MS1 spectra and MS2 spectra, respectively of m/z 244.153 ion; panels (E,F) were the MS1 spectra and MS2 spectra, respectively of m/z 242.1375 ion; and panels (G,H) were the MS1 spectra and MS2 spectra, respectively of m/z 228.1579 ion.
Discussion
Specific spoilage organisms refer to those organisms with the highest spoilage potential in specific products or storage conditions. Identification of SSOs is important for the understanding of food spoilage process and the development of spoilage control strategies. S. putrefaciens, Serratia liquefacines, Photobacterium phosphoreum, Brochothrix thermosphacta, Pseudomonas spp., Aeromonas spp., and Enterobacteriaceae have been identified to be the SSOs in marine or fresh fish in cold storage (Gram et al., 2002; Alfaro et al., 2013). For a certain fish, SSOs of multiple or single species have been reported (Gram et al., 2002). In the present study, A. veronii LP-11, Citrobater freundii LPJ-2, and Raoultella ornithinolytica LPC-3 which belong to Aeromonas and Enterobacteriaceae were identified to be the SSOs of vacuum packaged sturgeon stored at 4°C. Enterobacteriaceae have previously been reported to be the SSO of lightly preserved fish and chilled stored meat (Gram et al., 2002). They produce indole and putrescine, leading to the spoilage of food. Aeromonas, mostly A. hydrophila, is psychrotrophic and exhibits high proteolytic and lipolytic activities in many types of food, such as fish, poultry, beef, pork, and milk (Braun and Sutherland, 2005; Ravi et al., 2008). However, A. veronii is seldom reported to be an SSO in food and little is known about its spoilage potential.
Specific spoilage organisms are the main cause of food spoilage. And QS was reported to be played a role in the spoilage of aquatic products (Christensen et al., 2003; Flodgaard et al., 2005; Bai and Rai, 2011; Zhu et al., 2015, 2016). In order to find out whether QS is involved in the spoilage of sturgeon, we should first confirm that whether QS signals could be produced by sturgeon SSOs. AHLs, composed of a homoserine lactone ring and N-acyl chains varying in length, saturation level, and oxidation state, are QS signals of a great number of gram-negative bacteria. AHLs are synthesized by the enzyme of the LuxI family and sensed by the response transcriptional regulators of LuxR family (Ng and Bassler, 2009). In the present study, the discovery of AHLs in sturgeon SSO, A. veronii LP-11 suggested that QS is probably associated with spoilage of sturgeon, which was demonstrated previously (Gui et al., 2017), and this result advances our understanding of the microbiology of sturgeon spoilage which may be helpful for better sturgeon preservation. In addition, the four AHLs produced by A. veronii LP-11 in this study were different from AHLs produced by other Aeromonas. In a previous study, A. veronii MTCC3429 was reported to produce multiple AHLs, including 6-OH-HHL, C14-HSL, and methy-branched AHLs (Thiel et al., 2009; Jangid et al., 2012). It is very interesting that a species produces so many different AHLs. The specific LuxR/LuxI system of A. veronii LP-11, as well as the similarity and difference in the QS system between this strain and other Aeromonas, needs to be further defined. Most of the reported Aeromonas species that produce AHLs are A. hydrophila and A. salmonicida. C4-HSL and C6-HSL are the common two AHLs produce by the two species (Bruhn et al., 2005; Medina-Martinez et al., 2006a,b; Rasch et al., 2007), while C8-HSL and 3-OH-C8-HSL, produced by A. veronii LP-11 in the present study, have never been identified by MS in the genus Aeromonas or specie A. veronii in other studies.
At present, there is no accepted standard method to detect AHLs from bacteria. The combined use of different AHL biosensors was a fast and convenient method to screen pure cultures of bacteria for AHL production (Steindler et al., 2007). Using biosensors, we screened the three SSOs in sturgeon and only A. veronii LP-11 induced both the two biosensors (Table 1), indicating that it produced AHLs. C. freundii LPJ-2 and R. ornithinolytica LPC-3 were not found to produce AHLs by the two biosensors. This could be explained by two reasons: (i) the lack of a functional luxI homolog gene for production of AHLs; (ii) the amount of AHLs produced by these two strains was below the detection threshold of the biosensors (Chang et al., 2012). In addition, the deficiency of lasI homolog gene or the growth medium used in the study could be the reason for the low production of AHLs either (Zhu et al., 2002, 2004). Four AHLs including C4-HSL, C6-HSL, C8-HSL, and 3-oxo-C8-HSL, were identified in A. veronii LP-11 extract by qTOF MS. With this result, it can be determined that spots c and d in TLC plates coupled with A. tumefaciens KYC55 were 3-oxo-C8-HSL and C8-HSL, respectively, and spots a in TLC plates coupled with C. violaceum CV026 were C6-HSL. As 3-oxo- and 3-hydroxy derivatives of AHLs of the same chain length migrate with indistinguishable mobility in the methanol/water solvent system (Shaw et al., 1997), it can be deduced that spot b was 3-OH-C8-SHL. A. tumefaciens KYC55 has been reported to have the greatest sensitivity to AHLs and the broadest AHL range (Zhu et al., 2003), but it still failed to detect C6-HSL and 3-OH-C8-HSL in the present study. Interestingly, all the four AHLs were detected when A. tumefaciens KYC55 and C. violaceum CV026 used in combination, suggesting that the combined use of the two biosensers is necessary. QqQ-MS is an effective and sensitive approach for analysis of AHLs. It has been used to detect AHLs in Tenacibaculum maritimum, Escherichia coli, and P. aeruginosa extract (Gould et al., 2006; Romero et al., 2010). However, it was failed to detect C6-HSL in the present study. This may contributed by two reasons: (i) the concentration of C6-HSL in A. veronii LP-11 extract was too low to meet the detection limit of QqQ-MS and (ii) the interference of other compounds in the A. veronii LP-11 extract which had the same retention time of C6-HSL, produced too many fragment ions, and reduced the intensity of the target ion in the mass spectrum. These may also explain why C6-HSL showed low intensity of precursor ions [M + H]+ in the MS1 spectra and no obvious characteristic ion m/z 102 in the MS2 spectra in TOF MS. In contrast, the four AHLs were all accurately identified by qTOF-MS with typical mass errors below 2.1 mDa within 30 min, indicating that qTOF-MS was more effective and accurate than the methods of TLC and QqQ-MS.
Conclusion
The study demonstrated that A. veronii LP-11, C. freundii LPJ-2, and R. ornithinolytica LPC-3 were the SSOs of vacuum packaged sturgeon stored at 4°C. Among the three SSO, only A. veronii LP-11 produced C6-HSL, C8-HSL, 3-oxo-C8-HSL, and 3-OH-C8-HSL. So far as we know, this is the first report that C8-HSL and 3-OH-C8-HSL were detected by MS in the genus Aeromonas. Our results showed that HPLC/qTOF-MS was the most versatile detection method for rapid analysis of AHLs in complex microbial sample. This is the first attempt to characterize AHLs produced by sturgeon spoilage bacteria. Further studies are needed to clarify the QS regulation mechanisms of the four AHLs and the role of AHLs in sturgeon spoilage.
Author Contributions
MG and PL designed the study and drafted the manuscript. RW and LL collected test data and interpreted the results. SW and JH provided useful suggestions of the study.
Funding
This work was supported by Beijing Natural Science Foundation (Grant No. 6164033), Beijing Sturgeon and Trout Innovation Team (SCGWZJ20161105-2), and Youth Foundation of Beijing Academy of Agriculture and Forestry Sciences (QNJJ201523).
Conflict of Interest Statement
The authors declare that the research was conducted in the absence of any commercial or financial relationships that could be construed as a potential conflict of interest.
Acknowledgments
We thank Zhigang Zhou for kind donation of the biosensors and Dr. Lei Rao for thoroughly editing the manuscript.
References
Alfaro, B., Hernández, I., Le Marc, Y., and Pin, C. (2013). Modelling the effect of the temperature and carbon dioxide on the growth of spoilage bacteria in packed fish products. Food Control 29, 429–437. doi: 10.1016/j.foodcont.2012.05.046
Bai, A. J., and Rai, V. R. (2011). Bacterial quorum sensing and food industry. Compr. Rev. Food Sci. Food Saf. 10, 183–193. doi: 10.1111/j.1541-4337.2011.00150.x
Braun, P., and Sutherland, J. P. (2005). Predictive modelling of growth and measurement of enzymatic synthesis and activity by a cocktail of selected Enterobacteriaceae and Aeromonas hydrophila. Int. J. Food Microbiol. 105, 257–266. doi: 10.1016/j.ijfoodmicro.2005.04.005
Bruhn, J. B., Dalsgaard, I., Nielsen, K. F., Buchholtz, C., and Larsen, J. L. (2005). Quorum sensing signal molecules (acylated homoserine lactones) in gram-negative fish pathogenic bacteria. Dis. Aquat. Org. 65, 43–52. doi: 10.3354/dao065043
Cataldi, T. R. I., Bianco, G., Fonseca, J., and Schmitt-Kopplin, P. (2013). Perceiving the chemical language of Gram-negative bacteria: listening by high-resolution mass spectrometry. Anal. Bioanal. Chem. 405, 493–507. doi: 10.1007/s00216-012-6371-2
Chang, C., Koh, C., Sam, C., Chan, X., Yin, W. F., and Chan, K. G. (2012). Unusual long-chain N-acyl homoserine lactone production by and presence of quorum quenching activity in bacterial isolates from diseased tilapia fish. PLoS One 7:e44034. doi: 10.1371/journal.pone.0044034
Christensen, A. B., Riedel, K., Eberl, L., Flodgaard, L. R., Molin, S., Gram, L., et al. (2003). Quorum-sensing-directed protein expression in Serratia proteamaculans B5a. Microbiology 149, 471–483. doi: 10.1099/mic.0.25575-0
Flodgaard, L. R., Dalgaard, P., Andersen, J. B., Nielsen, K. F., Givskov, M., and Gram, L. (2005). Nonbioluminescent strains of Photobacterium phosphoreum produce the cell-to-cell communication signal N-(3-Hydroxyoctanoyl)homoserine lactone. Appl. Environ. Microbiol. 71, 2113–2120. doi: 10.1128/AEM.71.4.2113-2120.2005
Gould, T. A., Herman, J., Krank, J., Murphy, R. C., and Churchill, M. E. (2006). Specificity of acyl-homoserine lactone synthases examined by mass spectrometry. J. Bacteriol. 188, 773–783. doi: 10.1128/JB.188.2.773-783.2006
Gram, L., and Dalgaard, P. (2002). Fish spoilage bacteria–problems and solutions. Curr. Opin. Biotechnol. 13, 262–266. doi: 10.1016/S0958-1669(02)00309-9
Gram, L., Ravn, L., Rasch, M., Bruhn, J. B., Christensen, A. B., and Givskov, M. (2002). Food spoilage—interactions between food spoilage bacteria. Int. J. Bacteriol. 78, 79–97. doi: 10.1016/S0168-1605(02)00233-7
Gu, Q., Fu, L., Wang Y., and Lin, J. (2013). Identification and characterization of extracellular cyclic dipeptides as quorum-sensing signal molecules from Shewanella baltica, the specific spoilage organism of Pseudosciaena crocea during 4°C storage. J. Agric. Food Chem. 61, 11645–11652. doi: 10.1021/jf403918x
Gui, M., Binzhao, Song, J., Zhang, Z., Hui, P., and Li, P. (2014). Biogenic amines formation, nucleotide degradation and TVB-N accumulation of vacuum-packed minced sturgeon (Acipenser schrencki) stored at 4°C and their relation to microbiological attributes. J. Sci. Food Agric. 94, 2057–2063. doi: 10.1002/jsfa.6524
Gui, M., Wu, R. Y., Liu, L., Wang, S., Zhang, L., and Li, P. L. (2017). Effects of quorum quenching by AHL lactonase on AHLs, protease, motility and proteome patterns in Aeromonas veronii LP-11. Int. J. Food. Microbiol. 252, 61–68. doi: 10.1016/j.ijfoodmicro.2017.04.005
Jangid, K., Parameswaran, P. S., and Shouche, Y. S. (2012). A variant quorum sensing system in Aeromonas veronii MTCC 3249. Sensors 12, 3814–3830. doi: 10.3390/s120403814
McClean, K. H., Winson, M. K., Fish, L., Taylor, A., Chhabra, S. R., Camara, M., et al. (1997). Quorum sensing and Chromobacterium violaceum: exploitation of violacein production and inhibition for the detection of N-acylhomoserine lactones. Microbiology 143, 3703–3711. doi: 10.1099/00221287-143-12-3703
Medina-Martinez, M. S., Uyttendaele, M., Demolder, V., and Debevere, J. (2006a). Effect of temperature and glucose concentration on the N-butanoyl-l-homoserine lactone production by Aeromonas hydrophila. Food Microbiol. 23, 534–540. doi: 10.1016/j.fm.2005.09.010
Medina-Martinez, M. S., Uyttendaele, M., Demolder, V., and Debevere, J. (2006b). Influence of food system conditions on N-acyl-l-homoserine lactones production by Aeromonas spp. Int. J. Food Microbiol. 112, 244–252. doi: 10.1016/j.ijfoodmicro.2006.04.025
Mengsheng, G., Hancai, C., Anatol, E., Gronquist, M. R., Robinson, J. B., Rolfe, B. G., et al. (2005). sinI- and expR-dependent quorum sensing in Sinorhizobium meliloti. J. Bacteriol. 187, 7931–7944. doi: 10.1128/JB.187.23.7931-7944.2005
Morin, D., Grasland, B., Vallée-Réhel, K., Dufau, C., and Haras, D. (2003). On-line high-performance liquid chromatography–mass spectrometric detection and quantification of N-acylhomoserine lactones, quorum sensing signal molecules, in the presence of biological matrices. J. Chromatogr. A 1002, 79–92. doi: 10.1016/S0021-9673(03)00730-1
Ng, W. L., and Bassler, B. L. (2009). Bacterial quorum-sensing network architectures. Annu. Rev. Genet. 43, 197–222. doi: 10.1146/annurev-genet-102108-134304
Piper, K. R., von Bodman, S. B., and Farrand, S. K. (1993). Conjugation factor of Agrobacterium tumefaciens regulates Ti plasmid transfer by autoinduction. Nature 362, 448–450. doi: 10.1038/362448a0
Rasch, M., Kastbjerg, V. G., Bruhn, J. B., Dalsgaard, I., Givskov, M., and Gram, L. (2007). Quorum sensing signals are produced by Aeromonas salmonicida and quorum sensing inhibitors can reduce production of a potential virulence factor. Dis. Aquat. Org. 78, 105–113. doi: 10.3354/dao01865
Ravi, S. C. N., Lalitha, K. V., Jose, L., Manju, S., and Gopal, T. K. S. (2008). Effect of packaging atmosphere on the microbial attributes of pearlspot (Etroplus suratensis Bloch) stored at 0–2 °C. Food Microbiol. 25, 518–528. doi: 10.1016/j.fm.2007.12.003
Ravn, L., Christensen, A. B., Molin, S., Givskov, M., and Gram, L. (2001). Methods for detecting acylated homoserine lactones produced by Gram-negative bacteria and their application in studies of AHL-production kinetics. J. Microbiol. Methods 44, 239–251. doi: 10.1016/S0167-7012(01)00217-2
Romero, M., Avendaño-Herrera, R., Magariños, B., Cámara, M., and Otero, A. (2010). Acylhomoserine lactone production and degradation by the fish pathogen Tenacibaculum maritimum, a member of the Cytophaga–Flavobacterium–Bacteroides (CFB) group. FEMS Microbiol. Lett. 304, 131–139. doi: 10.1111/j.1574-6968.2009.01889.x
Rul, F., and Monnet, V. (2015). How microbes communicate in food: a review of signaling molecules and their impact on food quality. Curr. Opin. Food Sci. 2, 100–105. doi: 10.1016/j.cofs.2015.03.003
Schuster, M., Joseph Sexton, D., Diggle, S. P., and Peter Greenberg, E. (2013). Acyl-homoserine lactone quorum sensing: from evolution to application. Annu. Rev. Microbiol. 67, 43–63. doi: 10.1146/annurev-micro-092412-155635
Shaw, P. D., Ping, G., Daly, S. L., Cha, C., Cronan, J. E., Rinehart, K. L., et al. (1997). Detecting and characterizing N-acyl-homoserine lactone signal molecules by thin-layer chromatography. Proc. Natl. Acad. Sci. U.S.A. 94, 6036–6041. doi: 10.1073/pnas.94.12.6036
Skandamis, P. N., and Nychas, G. J. E. (2012). Quorum sensing in the context of food microbiology. Appl. Environ. Microbiol. 78, 5473–5482. doi: 10.1128/AEM.00468-12
Steindler, L., and Venturi, V. (2007). Detection of quorum-sensing N-acyl homoserine lactone signal molecules by bacterial biosensors. FEMS Microbiol. Lett. 266, 1–9. doi: 10.1111/j.1574-6968.2006.00501.x
Thiel, V., Kunze, B., Verma, P., Wagner Döbler, I., and Schulz, S. (2009). New structural variants of homoserine lactones in bacteria. Chembiochem 10, 1861–1868. doi: 10.1002/cbic.200900126
Zhu, H., Bandara, R., Conibear, T. C., Thuruthyil, S. J., Rice, S. A., Kjelleberg, S., et al. (2004). Pseudomonas aeruginosa with lasI quorum-sensing deficiency during corneal infection. Invest. Ophthalmol. Vis. Sci. 45, 1897–1903. doi: 10.1167/iovs.03-0980
Zhu, H., Thuruthyil, S. J., and Willcox, M. D. (2002). Determination of quorum-sensing signal molecules and virulence factors of Pseudomonas aeruginosa isolates from contact lens-induced microbial keratitis. J. Med. Microbiol. 51, 1063–1070. doi: 10.1099/0022-1317-51-12-1063
Zhu, J., Chai, Y., Zhong, Z., Li, S., and Winans, S. C. (2003). Agrobacterium bioassay strain for ultrasensitive detection of N-acylhomoserine lactone-type quorum-sensing molecules: detection of autoinducers in Mesorhizobium huakuii. Appl. Environ. Microbiol. 69, 6949–6953. doi: 10.1128/AEM.69.11.6949-6953.2003
Zhu, J., Zhao, A., Feng, L., and Gao, H. (2016). Quorum sensing signals affect spoilage of refrigerated large yellow croaker (Pseudosciaena crocea) by Shewanella baltica. Int. J. Food Microbiol. 217, 146–155. doi: 10.1016/j.ijfoodmicro.2015.10.020
Keywords: Aeromonas veronii, specific spoilage organism, sturgeon, acyl homoserine lactone, quorum sensing
Citation: Gui M, Liu L, Wu R, Hu J, Wang S and Li P (2018) Detection of New Quorum Sensing N-Acyl Homoserine Lactones From Aeromonas veronii. Front. Microbiol. 9:1712. doi: 10.3389/fmicb.2018.01712
Received: 17 November 2016; Accepted: 09 July 2018;
Published: 31 July 2018.
Edited by:
Lorenzo Morelli, Università Cattolica del Sacro Cuore, ItalyReviewed by:
Ana Cristina Esteves, University of Aveiro, PortugalIddya Karunasagar, Nitte University, India
Copyright © 2018 Gui, Liu, Wu, Hu, Wang and Li. This is an open-access article distributed under the terms of the Creative Commons Attribution License (CC BY). The use, distribution or reproduction in other forums is permitted, provided the original author(s) and the copyright owner(s) are credited and that the original publication in this journal is cited, in accordance with accepted academic practice. No use, distribution or reproduction is permitted which does not comply with these terms.
*Correspondence: Pinglan Li, lipinglan420@126.com