- 1State Key Laboratory of Applied Microbiology Southern China, Guangdong Provincial Key Laboratory of Microbial Culture Collection and Application, Guangdong Open Laboratory of Applied Microbiology, Guangdong Institute of Microbiology, Guangzhou, China
- 2College of Food Science, South China Agricultural University, Guangzhou, China
In this study, we characterized the β-lactamase genes and phenotypic resistance of cephalosporin-resistant Enterobacteriaceae isolated from retail foods in China. Of 1,024 Enterobacteriaceae isolates recovered from raw meat products, aquatic products, raw vegetables, retail-level ready-to-eat (RTE) foods, frozen foods, and mushrooms from 2011 to 2014, 164 (16.0%) showed cefotaxime (CTX) and/or ceftazidime (CAZ) cephalosporin resistance, and 96 (9.4%) showed the extended-spectrum β-lactamase (ESBL) phenotype. More than 30% isolates were resistant to all antimicrobial agents except carbapenems (MEM 3.1% and IPM 5.2%), cefoxitin (FOX 6.3%), and amoxicillin/clavulanic acid (AMC 26%), and 94.8% of the strains were resistant to up to seven antibiotics. Polymerase chain reaction analysis showed that blaTEM (81.9%) was the most common gene, followed by blaCTX-M (68.1%) and blaSHV (38.9%). Moreover, 16.8% (72/429) of food samples contained ESBL-positive Enterobacteriaceae, with the following patterns: 32.9% (23/70) in frozen foods, 27.2% (5/29) in mushrooms, 17.6% (24/131) in raw meats, 13.3% (4/30) in fresh vegetables, 11.1% (8/72) in RTE foods, and 9.3% (9/97) in aquatic products. In addition, 24 of 217 foods collected in South China (11.1%), 25 of 131 foods collected in North of the Yangtze River region (19.1%), and 23 of 81 foods collected in South of the Yangtze River region (28.4%) were positive for ESBL- Enterobacteriaceae. Conjugation experiments demonstrated that the 22 of 72 isolates were transconjugants that had received the β-lactamase gene and were resistant to β-lactam antibiotics as well as some non-β-lactam antibiotics. These findings demonstrated that retail foods may be reservoirs for the dissemination of β-lactam antibiotics and that resistance genes could be transmitted to humans through the food chain; and the predominant ESBL-producing Enterobacteriaceae in China was isolated from in frozen chicken-meat, followed by frozen pork, cold noodles in sauce, cucumber, raw chicken meat, frozen pasta, brine-soaked chicken and tomato.
Introduction
Enterobacteriaceae, a large family of gram-negative, non-spore-forming, rod-shaped, facultative anaerobes capable of fermenting sugars to various end products, are important hygiene indicators for process verification in food production, recently replacing the poorly defined group of coliforms (Anonymous, 2005). Several members of this group, including some species of Escherichia, Enterobacter, Klebsiella, Proteus, Citrobacter, Serratia, Salmonella, Shigella, and Yersinia, are among the most important causes of serious hospital-acquired and community-onset bacterial infections in humans (Jarzab et al., 2011).
Resistance to antimicrobial agents in Enterobacteriaceae has become an increasingly relevant problem. Because antibiotics are widely used in medical clinics and animal husbandry, high concentrations of antibiotics have appeared in aquacultures and other agricultural products, soil, water, and even food; consistent with this, antimicrobial-resistant Enterobacteriaceae have been appeared more and more frequently, and multiple drug-resistant strains have emerged (Capita and Alonso-Calleja, 2013; Laxminarayan et al., 2013). Beta-lactams (mainly extended-spectrum cephalosporins and carbapenems) and fluoroquinolones are the primary therapeutic choices to treat infections caused by Enterobacteriaceae (Bassetti et al., 2015). However, resistance to these antimicrobials has been increasing in recent years (Ben Sallem et al., 2012; Blaak et al., 2014; Durso and Cook, 2014).
β-Lactamases are the most frequent source of resistance to β-lactam antibiotics, and the production of β-lactamase is the primary mechanism of antibiotic resistance in Enterobacteriaceae. Various β-lactamases have been reported, including penicillinases, extended-spectrum β-lactamases (ESBLs), cephalosporinases (AmpC), metallo-β-lactamases (MBLs), and carbapenemases (KPCs) (Chagas et al., 2011; Pitout, 2012). Among these, ESBL-producing bacteria are particularly important (Bush and Jacoby, 2010; Nordmann et al., 2011). ESBLs are mainly produced in gram-negative bacilli, particularly Enterobacteriaceae (Poirel et al., 2012), including the well-known bacteria Escherichia coli and Klebsiella pneumoniae (Cantón et al., 2012; Poirel et al., 2012). ESBLs have the ability to hydrolyze penicillins and aztreonam, as well as first-, second-, and third-generation cephalosporins, but not cephamycins and carbapenems. Moreover, ESBLs are usually inhibited by so-called “classical” β-lactamase inhibitors, such as clavulanic acid, tazobactam, and sulbactam (Lee et al., 2012). Most ESBLs can also confer resistance to fourth-generation cephalosporins [such as cefepime (FEP) or cefpirome] (Bush and Jacoby, 2010). ESBLs include the classical extended-spectrum TEM-, SHV-, OXA-, and cefotaxime (CTX)-M type enzymes (Livermore, 2008; Pfeiier et al., 2010).
Previous studies have focused on investigating ESBLs in medical and veterinary clinics; however, relatively few reports have investigated ESBLs in foods (Reuland et al., 2014; Ben Said et al., 2015; Tekiner and Özpinar, 2016). Because of the wide use of broad-spectrum antibiotics in the livestock and animal husbandry industries, ESBL-producing bacteria have evolved and shown increased incidence owing to mutations, selection, and the spread of drug-resistant genes in animal and food products of animal origin (Ojer-Usoz et al., 2013; Tekiner and Özpinar, 2016), as well as other types of foods, such as seafood (Nguyen et al., 2016), raw vegetables (Reuland et al., 2014; Ben Said et al., 2015), ready-to-eat (RTE) foods (Campos et al., 2015; Kim et al., 2015), and milk (Odenthal et al., 2016). Furthermore, these ESBL bacteria can readily be transferred to humans through consumption of contaminated food, contributing to the spread and persistence of antibiotic-resistant bacteria in the general population and environment (Huijbers et al., 2016). Thus, in order to identify changes in antimicrobial resistance as early as possible and control the spread of ESBL-producing strains in foods, implementing a system for monitoring the prevalence patterns of ESBL-producing Enterobacteriaceae in foods from different areas and performing regular surveillance of the susceptibility of isolates to antimicrobial agents are necessary.
In China, most studies of ESBL-producing Enterobacteriaceae have focused on characterizing isolates from the clinical setting (Xiao et al., 2011, 2015), aquatic environment (Zou et al., 2012), animals (Wang et al., 2012; Bai et al., 2016), and animal food sources (Zheng et al., 2012; Yang et al., 2014) in particular areas or provinces. In these studies, only limited types of food samples were examined. No systematic studies have been conducted on a nationwide scale in China.
Accordingly, based on our prior research on the prevalence of Enterobacteriaceae in certain cities (Yang et al., 2016; Zhang et al., 2016), we undertook this study with the goal of providing new knowledge on the diversity of Enterobacteriaceae from different types of fresh food, frozen food and retail-level RTE food samples collected from 2011 to 2014, covering most provincial capitals of China, characterize their resistance to antibiotics, and determine the presence of ESBL- producers in the recovered isolates.
Materials and Methods
Bacterial Strains
A total of 1,024 Enterobacteriaceae strains were studied; these strains were isolated from 429 retail food samples (including raw meat products, aquatic products, raw vegetables, retail-level RTE foods, frozen foods, and mushrooms) from various cities in China from 2011 to 2014 (Table S1). The strains were selected from the collection of the Guangdong Institute of Microbiology in Guangzhou, China. In brief, for isolation of E. coli, 25 g food sample was placed into a sterile bag containing 225 mL Butterfield's phosphate-buffered water (Huankai, Guangzhou, China) and homogenized at 230 rpm for 2 min using a stomacher (Huankai); serial 10-fold dilutions was inoculated lactose broth (Huankai) and fermentation tubes (Huankai) at 37°C for 24–48 h, a loopful of suspension from positive cultures (lactose fermentation positive and gas production positive) was streaked onto Chromagar E. coli agar plates (Huankai) and incubated at 37°C for 18–24 h; Subsequently, presumptive E. coli colonies were selected from each plate and biochemically identified by API 20E (BioMe′rieux, Marcy I′Etoile, France). For isolation of Salmonella, 25 g food sample was pre-enriched in 225 ml of buffered peptone broth (Huankai); one milliliter cultures were incubated in 10 ml of selenite cystine broth (SC) (Huankai) at 37°C and 10 ml of tetrathionate brilliant green broth (TTB) at 42°C for 24 h, respectively; loopfuls of SC and TTB cultures were streaked onto xyloselysine-tergitol 4 (XLT4) selective agar plates (Difco, Detroit, MI, USA) and chromogenic Salmonella agar plates (Huankai), then incubated at 37°C for 24 h; presumptive colonies were picked from each plate, stabbed into a triple sugar iron slant (Huankai), and incubated at 37°C for 24 h; isolates with typical Salmonella phenotypes were further confirmed using API 20E test strips (BioMe′rieux, Marcy I′Etoile, France).
Phenotypic ESBL Testing
Disk diffusion tests were used for initial screening of ESBL-producing isolates. Mueller Hinton agar (Huankai, Guangzhou, China) was swabbed using a suspension of a pure culture (0.5McF), and antibiotic disks were then loaded. CTX (30 μg) and ceftazidime (CAZ, 30 μg) were used for screening of suspicious ESBL-producing isolates (Maravić et al., 2015). Isolates with reduced susceptibility to CTX and/or CAZ were assessed for the presence of ESBLs using a combination of the double-disk synergy test (DDST) with CTX and CAZ with and without clavulanic acid (Mast Diagnostics, Merseyside, UK) according to the 2011 guidelines of the Clinical and Laboratory Standards Institute (CLSI). An increase in the zone diameter of more than 5 mm for either antimicrobial agent tested in combination with clavulanic acid vs. the zone diameter of the agent when tested alone confirmed the presence of an ESBL-producing organism.
Antimicrobial Susceptibility Testing
The susceptibility of antimicrobials to other commonly used antibiotics was assessed using the disk diffusion method according to 2011 CLSI guidelines for the following antibiotics: ampicillin (AMP, 30 μg), amoxicillin/clavulanic acid (AMC, 20/10 μg), aztreonam (AZM, 30 μg), imipinem (IPM, 10 μg), meropenem (MEM, 10 μg), FEP (30 μg), gentamicin (CN, 10 μg), tobramycin (TM, 10 μg), tetracycline (TE, 30 μg), ciprofloxacin (CIP, 5 μg), levofloxacin (LEV, 5 μg), trimethroprim/sulfamethoxazole (SXT, 1.25/23.75 μg), sulfonamide (SSS, 300 μg), chloramphenicol (C, 30 μg), cephalothin (KF, 30 μg), cefoxitin (FOX, 30 μg), ceftiofur (EFT, 30 μg), CTX (30 μg), and CAZ (30 μg; all from Oxoid Ltd., Basingstoke, UK). Staphylococcus aureus ATCC 25923 and Escherichia coli ATCC 25922 were used as quality control strains for this study. Zones of inhibition were measured with a precision caliper to the nearest 0.01 mm. Isolates exhibiting resistance to at least three antimicrobial agents tested were considered as multidrug-resistant strains.
Detection of Beta-Lactamase Genes
All isolates that exhibited a positive phenotype in ESBL screening tests were evaluated by PCR for the presence of genes encoding TEM, SHV, OXA, and CTX-M as previously described, with minor modifications. Genomic DNA was extracted from ESBL-producing isolates using a Bacterial Genomic DNA Purification Kit (Dongsheng Biotech, Guangzhou, China) according to the manufacturer's instruction. Genomic DNA concentration was determined at 260 nm using a Nano Drop®ND-1000UVeVis Spectrophotometer (Thermo Fisher Scientific, MA, USA). The primer sequences and their positions, PCR conditions, and references are summarized in Table S1.
Detection of Integrons
The presence of intI1 and intI2 genes (encoding class 1 and class 2 integrases, respectively) was examined by PCR (Table S1). Genomic DNA was extracted according to 2.4.
Conjugation Mating Experiments
Conjugation experiments were performed with the plasmid-free recipient strain E. coli DH5α (Kallová et al., 1995). Briefly, single colonies of the donor and recipient were inoculated in LB broth (Huankai, Guangzhou, China) and grown overnight at 37°C. Subsequently, equal volumes of the donor and recipient cultures were mixed and incubated overnight at 37°C without shaking. Serial dilutions were then plated on Luria-Bertani agar (Huankai, Guangzhou, China) selection plates supplemented with 50 μg/mL AMP (National Institutes for Food and Drug Control, Beijing, China). For all transconjugation experiments, the donor strain alone and acceptor strain alone were used as controls to ensure the effectiveness of the selective plates used. Transconjugants growing on the selection plates were subjected to DDSTs and PCR to confirm the presence of the ESBL phenotype.
Statistics
The independent sample Kruskal-Wallis test (multiple groups) were used to compare proportions of the resistances to antimicrobials for ESBL-producing strains between species, regions, and food types groups by SPSS Statistics V21.0; the independent sample Kruskal-Wallis test (multiple groups) were also used to compare proportions of ESBL-positive Enterobacteriaceae between food type and regions groups by SPSS Statistics V21.0.
Results
Identification of ESBL-Producing Enterobacteriaceae
A total of 164 (16.0%) isolates that showed the resistance or intermediate resistance to CTX and/or CAZ by the disc diffusion method were subjected to an initial screening of ESBL-producing isolates. Based on the results, 96 (9.4%) isolates were designated as ESBLs by DDSTs. Table 1 shows the identities of the 96 isolated ESBL-producing Enterobacteriaceae. A high prevalence of E. coli strains was found, followed by Salmonella spp. The remaining strains were identified as Citrobacter freundii, C. koseri, C. amalonaticus, and Serratia marcescens.
Sensitivity Profiles of ESBL-Producing Strains
Antimicrobial susceptibility tests using the standard disk diffusion method confirmed the presence of 96 ESBL-producing strains from DDSTs. The β-lactam sensitivity patterns showed high resistances to AMP, CTX, KF, and EFT (97.9, 97.8, 96.9, and 95.8%, respectively). The remaining sensitivity patterns were as follows: AZM, 51.0% resistant and 22.9% intermediate; CAZ, 42.7% resistant and 19.8% intermediate; FEP, 30.2% resistant and 17.7% intermediate; AMC, 26% resistant and 45.8% intermediate; and FOX, 6.3% resistant and 93.7% sensitive. The resistance against carbapenems was low; approximately 5.2% and 5.2% of strains showed resistance and intermediate resistance to IPM and MEM, respectively. Additionally, resistance patterns were observed for SSS (92.7% resistant and 2.1% intermediate), SXT (81.3% resistant), TE (83.3% resistant and 1.1% intermediate), and C (83.3% resistant and 2.1% intermediate). In contrast, approximately 36.5–47.9% of the strains were sensitive to CN, TM, LEV, and CIP (47.9.8, 40.6, 37.5, and 36.5%, respectively; Table 2). In addition, with the exception of one strain that was resistant to only one antimicrobials and one strain that was resistant to two antibiotic, all strains exhibited a multidrug-resistant phenotype, showing resistance to antibiotics from at least three different classes. Additionally, 94.8% of strains were resistant to at least seven antibiotics.
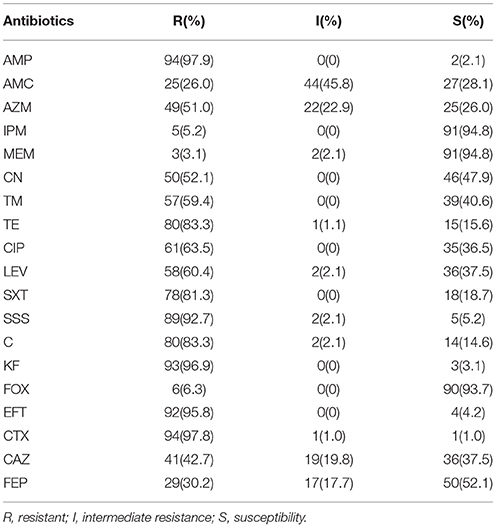
Table 2. Results of antimicrobial resistance of ESBL-producing Enterobacteriaceae from retail food in China.
By species, more than 92% of E. coli, Salmonella spp., Citrobacter spp., and Serratia spp. strains showed resistance to AMP and SSS. High levels of resistance to CTX, KF, and EFT were observed for E. coli and Salmonella spp. strains (≥92%), Citrobacter spp. and Serratia spp. strains (75–87.5%). A high percentage of E. coli, Salmonella spp., Citrobacter spp., and Serratia spp. strains were resistant to antibiotics tested, such as TE, C, SXT, and AMC (≥60%) and LEV, CIP, TM and CN (48.4%-69.2%). Moreover, the highest prevalence of recorded was against AZM, CAZ, and FEP in Salmonella spp. strains (92.3, 84.6, and 73.1%), followed by E. coli (69.4, 56.5, and 41.9%), Citrobacter spp. and Serratia spp. (50, 37.5, and 12.5%). The resistance against carbapenems and FOX was low in E. coli and Salmonella spp. strains (3.2–4.8%), while 12.5, 25, and 37.5% of Citrobacter spp. and Serratia spp. strains showed resistance and intermediate resistance to IPM, MEM and FOX respectively (Figure 1A). By regions, high levels of resistance to AMP, SSS, EFT and CTX (≥85.3%), and AMC, AZM, TE, SXT and C (≥67.7%) were observed. Moreover, a high percentage of the isolates resistance to CIP and LEV (70–81.3%), TM and CN (56.7–73.4%) were observed in all regions except for South China. The resistance against carbapenems and FOX was low (3.1–8.8%) (Figure 1B). By food types, the highest prevalence recorded was against AMP, SSS, KF, EFT, and CTX (≥92%) in all kinds of retail food. A high percentage of the isolates were resistant to other antibiotics tested, such as AMC and TE (≥70%), CAZ (44.5–61.3%) in retail food except for raw vegetables. Moreover, high levels of resistance to CIP, LEV, SXT, and C (≥67.7%), TM (60–77.8%) and CN (44.5–80%) in retail food except for RTE foods were also observed. The resistance rate to FOX, carbapenems varied from 0 to 25% in retail food (Figure 1C). Overall, there were no statistically significant difference in the resistances to antimicrobials for ESBL-producing strains by species (Figure 1A), regions (Figure 1B), and food types (Figure 1C; P >0.05). In addition, 35 strains were isolated from 16 food samples which each one sample was detected 2–3 E. coli or Salmonella spp. strains, and the same species from the same food sample had the same resistant pattern. One isolate belong to each resistant pattern was selected from each food sample. Thus, 72 isolates which were selected from 96 ESBL-producing strains were analyzed for further study. Information on the 72 ESBL-producing strains is shown in Table 3.
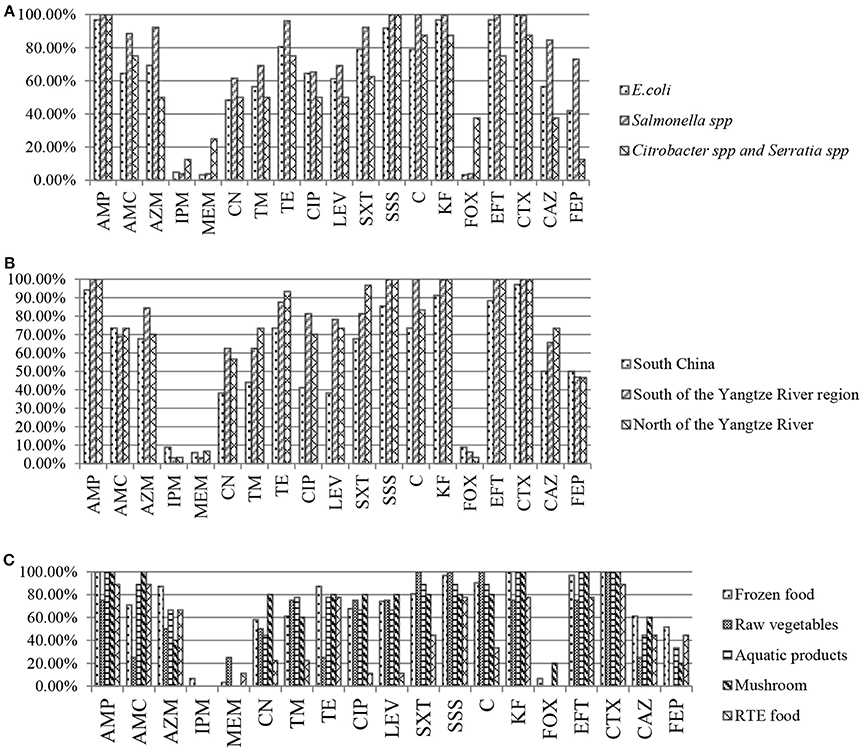
Figure 1. Results of antibiotic resistance rate of Enterobacteriaceae isolates from retail food in China. (A) Results of antibiotic resistance rate by species. (B) Results of antibiotic resistance rate by regions. (C) Results of antibiotic resistance rate by food types.
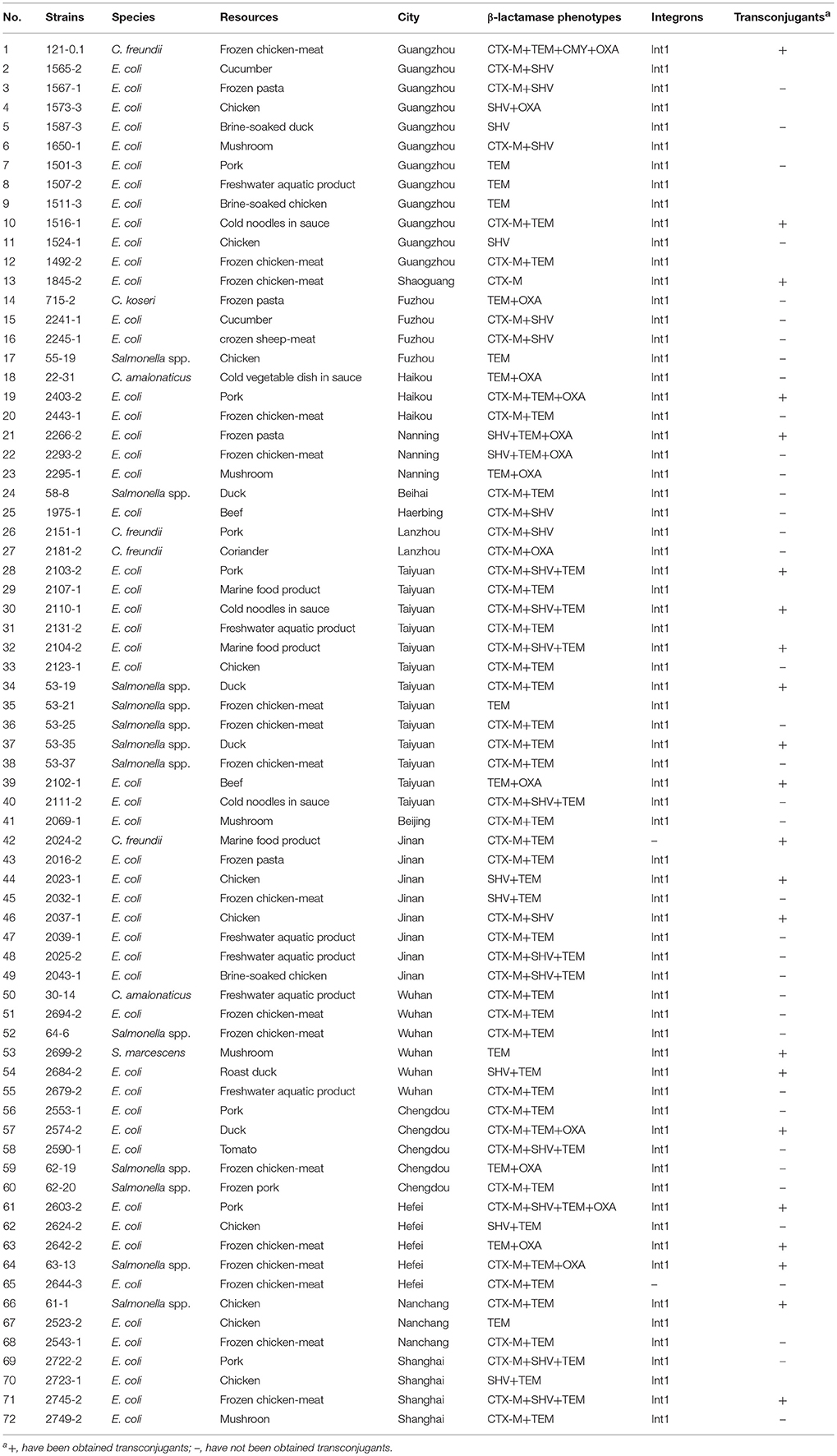
Table 3. Results of β-lactamase gene types, integrons and transconjugants of β-lactamase -producing Enterobacteriaceae from retail food in China.
Characterization of β-Lactamase Genes
One or more β-lactamase genes were detected in all of the 72 isolated strains. The TEM type was clearly prevalent in our isolates (81.9%), followed by the CTX-M type (68.1%), SHV type (38.9%), and OXA type (20.8%). CTX-M in combination with TEM β-lactamase (34.7%) was the most frequent ESBL type. TEM β-lactamase was detected alone in seven isolates (four E. coli, one Serratia marcescens, and two Salmonella spp.). SHV ESBL was only detected in E. coli isolates (alone or in combination with other types of β-lactamases), but for one C. freundii 2151-1 isolate, was identified in combination with CTX-M. OXA β-lactamase was detected in combination with other types of β-lactamases (Table 3).
Prevalence of ESBL-Producing Enterobacteriaceae in Retail Food Products
From our analysis of 429 food samples using the disk diffusion method, we detected ESBL-producing Enterobacteriaceae in 72 (16.8%). Some specific differences were present among distinct food varieties. For example, ESBL-positive Enterobacteriaceae were detected in 23 of 70 (32.9%) frozen food samples, five of 29 (27.2%) mushroom samples, 23 of 131 (17.6%) raw meats, four of 30 (13.3%) fresh vegetables, eight of 72 (11.1%) RTE foods, and nine of 97 (9.3%) aquatic products (Table 4). By regions, our study detected ESBL-positive Enterobacteriaceae in 16 of 24 cities surveyed (66.7%), with prevalence ranging from 6.7% in cities in ha'erbin to 72.7% in Jinan. The prevalence of ESBL-positive Enterobacteriaceae varied with different latitude: 24 of 217 foods collected in South China (11.1%), 25 of 131 foods collected in North of the Yangtze River region (19.1%), and 23 of 81 foods collected in South of the Yangtze River region (28.4%) were positive for ESBL-positive Enterobacteriaceae (Figure 2). Overall, there were no statistically significant difference in the prevalence of ESBL-positive Enterobacteriaceae by regions (Figure 2) and by food type (Table 4; P > 0.05).
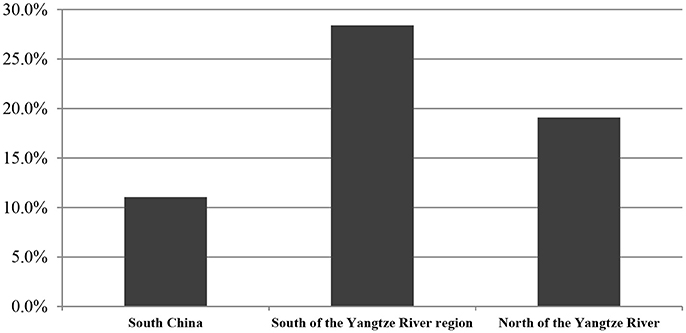
Figure 2. The occurrence of ESBL- producing Enterobacteriaceae isolated from retail foods by regions in China. % of isolates were include the prevalence of resistance or intermediate resistance to the antibiotics. AMP, ampicillin; AMC, amoxicillin/clavulanic acid; AZM, aztreonam; IPM, imipinem; MEM, meropenem; CN, gentamicin; TM, tobramycin; TE, tetracycline; CIP, ciprofloxacin; LEV, levofloxacin; SXT, trimethoprim/sulfamethoxazole; SSS, sulfonamide; C, chloramphenicol; KF, cephalothin; FOX, cefoxitin; EFT, ceftiofur; CTX, cefotaxime; CAZ, ceftazidime; FEP, cefepime.
Detection of Integrons
Class 1 integrons were present in 70 of the 72 ESBL strains, whereas class 2 integrons were not identified in this collection (Table 3).
Conjugal Transfer of ESBL-Encoding Genes
In the conjugation experiments, transfer of the ESBL phenotype was demonstrated in 22 out of 72 ESBL strains tested (Table 3); however, transconjugants could not be recovered in the other strains. All of the obtained transconjugants received β-lactamase genes and acquired the ESBL phenotype. In addition, seven transconjugants acquired the ESBL phenotype and resistance to SSS, CIP, CN, SXT, TE, and C (Table 5).
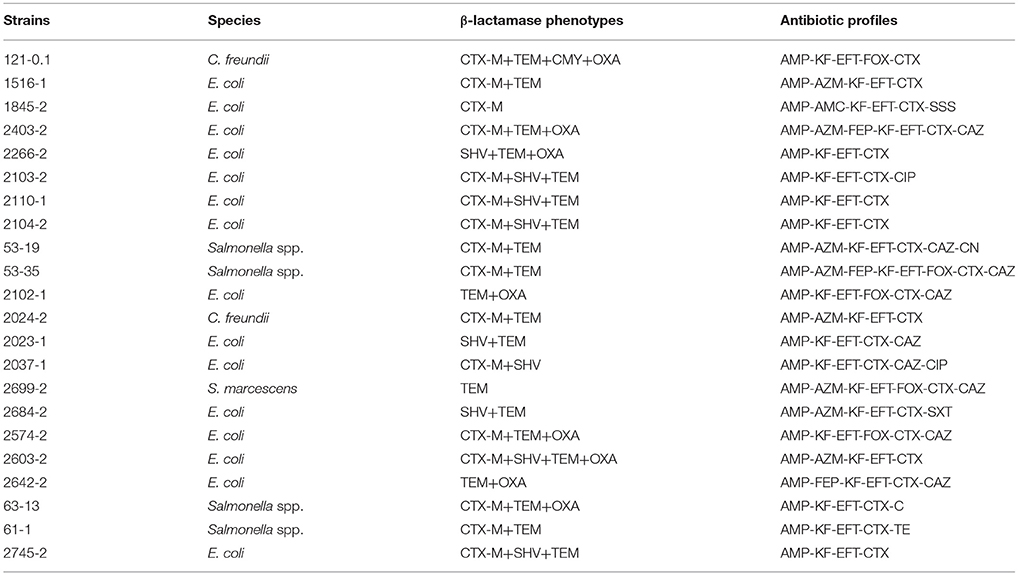
Table 5. Characterization of transconjugants of β-lactamase -producing Enterobacteriaceae from retail foods in China.
Discussion
Contaminated food is a major source of gastrointestinal microbial pathogens and has caused numerous foodborne disease outbreaks in the developing world. Because of the wide use of third- and fourth-generation cephalosporin antibiotics, the prevalence of multidrug-resistant Enterobacteriaceae has increased yearly, around the globe. The production of ESBLs is the primary mechanism of antibiotic resistance in Enterobacteriaceae, and ESBL-producing isolates are widespread in China and other countries. Accordingly, antibiotic resistance owing to ESBLs is a major public health concern. Although ESBL-producing isolates have not been frequently reported in food products, some reported have described ESBL-producing isolates in chicken, pork, beef, other raw meats (Ojer-Usoz et al., 2013; Tekiner and Özpinar, 2016), and even fresh vegetables (Reuland et al., 2014; Ben Said et al., 2015; Kim et al., 2015; Nguyen et al., 2016; Freitaga et al., 2018). Additionally, some recent studies have been undertaken to determine the prevalence of ESBL-producing Enterobacteriaceae from animals or animal sources of foods in China (Zheng et al., 2012; Yang et al., 2014) and fewer reports have described other types of foods (Yang et al., 2014). Moreover, in these studies, only a limited number of food samples or food sources of a particular type were examined, and no systematic studies have been conducted on a nationwide scale in China.
This is the first nationwide survey of the prevalence of ESBL-producing Enterobacteriaceae in foods in China. This survey was a large-scale investigation of ESBL-producing Enterobacteriaceae in fresh, frozen, and RTE food products collected from more of the provincial capitals of China, and the types of food sampled were more culturally relevant than those in some previous studies. Of the 429 food samples examined, we found an overall prevalence of 16.8%, with certain differences being present among the distinct food varieties. Moreover, ESBL-producing Enterobacteriaceae varied from 9.3% of 97 isolates in aquatic products to 32.9% of 70 isolates in frozen food samples. This result was higher than those found by van Hoek et al. (2015) (3.5% of 1216 isolates), Reuland et al. (2014) (6% of 119 isolates), and Maravić et al. (2015) (4.2% of 1351 isolates). Our results indicated ESBL-producing Enterobacteriaceae has the highest incidence in frozen foods (32.9% of 70 isolates), ranging from 14.3% of 7 isolates in frozen sheep-meat to 42.5% of 40 isolates in frozen chicken-meat. The risks of ESBL-producing strains in these food products should draw public attention. Raw meat products have often been suggested as the main source of ESBL-producing Enterobacteriaceae, and several studies were carried out to assess the prevalence of ESBL-producing Enterobacteriaceae in these products. 23 of the 131 raw meat samples were confirmed positive for the presence of ESBL-producing Enterobacteriaceae (17.6%), which is comparable to results from some other countries (Schilla et al., 2017) (20.6% of 63 isolates). Prevalence in poultry products (23.8% of 42 isolates in chicken and 17.4% of 23 isolates in duck) was higher than in pork (13.7% of 51 isolates) or beef (13.3% of 15 isolates), which is a lower prevalence than has been reported for many countries (84% of 45 isolates in poultry, 55% of 47 isolates in pork and 59% of 49 isolates in beef) (Ojer-Usoz et al., 2013). The prevalence of ESBL-producing Enterobacteriaceae in frozen foods was higher than that in raw meat products, indicating that frozen foods may be susceptible to cross-contamination in the production process. Our results found a high prevalence of ESBL-producing Enterobacteriaceae in raw vegetables (13.3% of 30 isolates) than previous studies (3.5% of 1216 isolates) (van Hoek et al., 2015). RTE foods such as cold noodles in sauce, cold vegetable dish in sauce, brine-soaked or roast meat (chicken, duck, pork and beef) and milk are commonly consumed in China. Of the 72 RTE food samples examined, we found an overall prevalence of 11.1%, with some differences among different varieties of food. No ESBL-producing Enterobacteriaceae were isolated from brine-soaked or roast pork, brine-soaked beef, milk and roast chicken. By contrast, a high prevalence of ESBL-producing Enterobacteriaceae in cold noodles in sauce (27.3% of 11 isolates), brine-soaked chicken (20% of 10 isolates), cold vegetable dish in sauce (14.3% of 7 isolates), brine-soaked duck (12.5% of 8 isolates) and roast duck (8.3% of 12 isolates) was observed, and we still need to pay attention to contamination of these food.
Categorized by genera, E. coli was the predominant ESBL producer, consistent with the results of other studies (Lu et al., 2010; Mokracka et al., 2012; Korzeniewska and Harnisz, 2013). However, the rate of ESBL-positive E. coli was lower than those found in clinical settings (Xiao et al., 2015) (42.3% of 634 isolates), but is consistent with a previous report in animals in China (Zheng et al., 2012) (13.1% of 896 isolates). Citrobacter and Serratia are mainly detected in the clinical setting and were present at lower prevalence in our study than in previous clinical reports (Parka et al., 2005). The differences in the occurrence of ESBL-producing Enterobacteriaceae in retail foods may also be associated with regional differences. Our study detected ESBL-positive Enterobacteriaceae in 16 of 24 cities surveyed (66.7%), with prevalence ranging from 6.7% in cities in ha'erbin to 72.7% in Jinan. The prevalence of ESBL-positive Enterobacteriaceae varied with different latitude, and the most occurrence was observed in South of the Yangtze River region (28.4%), followed in North of the Yangtze River region (19.1%) and in South China (11.1%). Thus, measures for governmental agencies to play a larger role in controlling the risks of ESBL-producing Enterobacteriaceae are recommended.
Many studies have shown that the generation of ESBL enzymes is the primary mechanism of antibiotic resistance in Enterobacteriaceae. ESBL-producing Enterobacteriaceae can exhibit strong antimicrobial resistance to penicillins, third-generation cephalosporins, aminoglycosides, C, quinolones, and TE (Rupp and Fey, 2003). In this study, we found that over 50% of ESBL-positive strains were resistant to AZM, TE, SXT, SSS, C, KF, CTX, and EFT, and these results are consistent with a previous report (Yang et al., 2014). Currently, the most reliable therapies used for infection by ESBL-producing Enterobacteriaceae are FEP and carbapenems (Paterson et al., 2001). In the present study, 47.9% of β-lactamase-producing strains showed resistance to FEP, in contrast with previous studies (Chen et al., 2012; Maravić et al., 2015). This phenomenon may be explained by the varied use of FEP in different countries. Previous studies have reported that few ESBL-positive isolates were resistant to carbapenems, even in the clinical setting (Chen et al., 2014; Hu et al., 2015). However, 5.2% of strains were resistant to IPM and MEM in the present study. Nevertheless, carbapenems may still be effective for the treatment of ESBL-producing Enterobacteria. Taken together, these data demonstrated that the emergence of ESBL-producing strains and the wide use of β-lactam antimicrobial agents had a direct causal relationship; therefore, measures for controlling the overuse of third-generation cephalosporins should be implemented to reduce the emergence of drug-resistant strains.
ESBLs include TEM-, SHV-, OXA-, and CTX-M-type enzymes (Livermore, 2008; Pfeiier et al., 2010). In the 1980s, SHV- and TEM-type ESBLs were the primary cause of third-generation cephalosporin resistance among Enterobacteriaceae (Pitout, 2012). In contrast, since 2000, the CTX-M-type ESBL became predominant over TEM- and SHV-type enzymes (Cantón et al., 2012; Peirano et al., 2012; Doi et al., 2013). Because different countries and regions have different strategies for antibiotics use, the dominance of ESBL-enzyme types differs among countries. CTX-M has been reported to be dominant in China and is the most common type of ESBL globally (Cantón et al., 2012; Peirano et al., 2012). Genotypic characterization by PCR assays revealed that blaTEM was the most frequent gene, followed by blaCTX-M and blaSHV, consistent with previous reports in other countries (Ferreira et al., 2014; Kawamura et al., 2014; Zarfel et al., 2014). Class 1 integrons were present in 70 of the 72 ESBL -producing strains, but class 2 integrons were not identified in this collection, which was consistent with other reports (Schmiedel et al., 2014; Ben Said et al., 2015). In the conjugation transfer experiments, 22 isolates were detected as transconjugants, demonstrating the prevalence of ESBL-producing Enterobacteriaceae in China. Moreover, transconjugants received the β-lactamase gene and showed resistance to β-lactam antibiotics and some non-β-lactam antibiotics. These results indicated that plasmids could not only carry genes encoding ESBL β-lactamases but also could spread horizontally among Enterobacteriaceae, thereby contributing to resistance to antimicrobials, including β-lactams and some non-β-lactams, resulting in high prevalence of multidrug resistance.
We here report the results of the first investigation of the prevalence of ESBL- -producing Enterobacteriaceae isolated from retail foods in China. Our results showed that frozen foods, mushrooms, raw meat products, raw vegetables, and aquatic products were important vehicles for the dissemination of ESBL-producing Enterobacteria and posed a critical health risk for Chinese consumers. Therefore, close surveillance of antimicrobial resistance in bacteria from food-producing and food-derived products should be established as a priority. In addition, our results could provide a basis for further investigations into ESBL-positive isolates from food products in order to avoid jeopardizing the safety of final products.
Author Contributions
QY contributed to designed the work that led to the submission, acquired data, and played an important role in interpreting the results. QW contributed to revised the manuscript and approved the final version. SZ, GY helped to acquired data. JZ, MC, LX, and JW helped perform the analysis with constructive discussions.
Funding
This work was supported by the National Key Research and Development Program of China (2016YFD0401204); Science and Technology Program of Guangdong Province (2016A050502033); Natural Science Foundation of Guangdong Province, China (2018A030313442); GDAS' Special Project of Science and Technology Development (2018GDASCX-0401; 2018GDASCX-0910; 2017GDASCX-0201).
Conflict of Interest Statement
The authors declare that the research was conducted in the absence of any commercial or financial relationships that could be construed as a potential conflict of interest.
Supplementary Material
The Supplementary Material for this article can be found online at: https://www.frontiersin.org/articles/10.3389/fmicb.2018.01709/full#supplementary-material
References
Anonymous (2005). Commission Regulation (EC) No 2073/2005 of 15 November 2005 on Microbiological Criteria for Foodstuffs. Available online at: https://eur-lex.europa.eu/eli/reg/2005/2073/oj
Bai, L., Hurley, D., Li, J., Meng, Q., Wang, J., Fanning, S., et al. (2016). Characterisation of multidrug-resistant Shiga toxin-producing Escherichia coli cultured from pigs in China: co-occurrence of extended-spectrum β-lactamase- and mcr-1-encoding genes on plasmids. Int. J. Antimicrob. Agents 48, 445–448. doi: 10.1016/j.ijantimicag.2016.06.021
Bassetti, M., Pecori, D., Sibani, M., Corcione, S., and Rosa, F. G. (2015). Epidemiology and Treatment of MDR Enterobacteriaceae. Curr. Treat Options Infect. Dis. 7, 291–316. doi: 10.1007/s40506-015-0065-1
Ben Sallem, R., Ben Slama, K., Sáenz, Y., Rojo-Bezares, B., Estepa, V., Jouini, A., et al. (2012). Prevalence and characterization of extended-spectrum beta-lactamase (ESBL)- and CMY-2-producing Escherichia coli Isolates from healthy food-producing animals in Tunisia. Foodborne Pathog. Dis. 9, 1137–1142. doi: 10.1089/fpd.2012.1267
Ben Said, L., Jouini, A., Klibi, N., Dziri, R., Alonso, C. A., Boudabous, A., et al. (2015). Detection of extended-spectrum beta-lactamase (ESBL)-producing Enterobacteriaceae in vegetables, soil and water of the farm environment in Tunisia[J]. Int. J. Food Microbiol. 203, 86–92. doi: 10.1016/j.ijfoodmicro.2015.02.023
Blaak, H., van Hoek, A. H., Veenman, C., Docters, A. E. L., Lynch, G., Overbeek, W. M., et al. (2014). Extended spectrum ß-lactamaseand constitutively AmpC-producing Enterobacteriaceae on fresh produce and in the agricultural environment. Int. J. Food Microbiol. 168–169, 8–16. doi: 10.1016/j.ijfoodmicro.2013.10.006
Bush, K., and Jacoby, G. A. (2010). Updated functional classification of beta-lactamases. Antimicrob. Agents Chemother. 54, 969–976. doi: 10.1128/AAC.01009-09
Campos, J., Gil, J., Mourão, J., Peixe, L., and Antunes, P. (2015). Ready-to-eat street-vended food as a potential vehicle of bacterial pathogens and antimicrobial resistance: an exploratory study in Porto region, Portugal. Int. J. Food Microbiol. 206, 1–6. doi: 10.1016/j.ijfoodmicro.2015.04.016
Cantón, R., González-Alba, J. M., and Galán., J. C (2012). CTX-M enzymes: origin and diffusion. Front. Microbiol: 3:110. doi: 10.3389/fmicb.2012.00110
Capita, R., and Alonso-Calleja, C. (2013). Antibiotic-resistant bacteria: a challenge for the food industry. Crit. Rev. Food Sci. 53, 11–48. doi: 10.1080/10408398.2010.519837
Chagas, T. P., Seki, I. M., Cury, J. C., Oliveira, J. A., Davila, A. M., Silva, D. M., et al. (2011). Multiresistance beta-lactamse-encoding genes and bacterial diversity in hospital wastewater in Rio de Janeiro, Brazil. J. Appl. Microbiol. 111, 572–581. doi: 10.1111/j.1365-2672.2011.05072.x
Chen, J., Jin, M., Qiu, Z. G., Guo, C., Chen, Z. L., Shen, Z. Q., et al. (2012). A Survey of Drug Resistance bla Genes Originating from Synthetic Plasmid Vectors in Six Chinese Rivers. Environ. Sci. Technol. 46, 13448–13454. doi: 10.1021/es302760s
Chen, Z. J., Sun, Z. Y., Hu, Z. D., Li, J., Wei, L. H., Wu, L., et al. (2014). CHINET 2012 surveillance of antibiotic resistance in Enterobacter spp. in China. Chin. J. Infect Chemother 14, 387–391. doi: 10.16718/j.1009-7708.2014.05.006
Doi, Y., Park, Y. S., Rivera, J. I., Adams-Haduch, J. M., Hingwe, A., Sordillo, E. M., et al. (2013). Community-associated extended-spectrum beta-lactamaseproducing Escherichia coli infection in the United States. Clin. Infect. Dis. 56, 641–648. doi: 10.1093/cid/cis942
Durso, L. M., and Cook, K. L. (2014). Impacts of antibiotic use in agriculture: what are the benefits and risks? Curr. Opin. Microbiol. 19, 37–44. doi: 10.1016/j.mib.2014.05.019
Ferreira, J. C., Filho, R. A. C. P., Andrade, L. N., Junior, A. B., and Darini, A. L. C. (2014). IncI1/ST113 and IncI1/ST114 conjugative plasmids carrying blaCTX-M-8 in Escherichia coli isolated from poultry in Brazil. Diagn. Microbiol. Infect. Dis. 80, 304–306. doi: 10.1016/j.diagmicrobio.2014.09.012
Freitaga, C., Michael, G. B., Lid, J., Kadle, K., Wang, Y., Hassel, M., et al. (2018). Occurrence and characterisation of ESBL-encoding plasmids among Escherichia coli isolates from fresh vegetables. Vet. Microbiol. 219, 63–69. doi: 10.1016/j.vetmic.2018.03.028
Hu, F. P., Zhu, D. M., Wang, F., Jiang, X. F., Xu, Y. C., Zhang, X. J., et al. (2015). CHINET 2014 surveillance of bacterial resistance in China. Chin. J. Infect. Chemother 15, 401–410. doi: 10.3969/j.issn.1009-7708.2015.05.001
Huijbers, P. M. C., Graat, E. A. M., Hoek, A. H. A. M., Veenman, C., Jong, M. C. M., and Duijkeren, E. (2016). Transmission dynamics of extended-spectrum β-lactamase and AmpCβ-lactamase-producing Escherichia coli in a broiler flock without antibiotic use. Prev. Vet. Med. 131, 12–19. doi: 10.1016/j.prevetmed.2016.07.001
Jarzab, A., Gorska-Fraczek, S., Rybka, J., and Witkowaka, D. (2011). Enterobacteriaceae infection-diagnosis, antibitioc resistance and prevention. Postepy Hig. Med. Dosw. 65, 55–72. doi: 10.5604/17322693.933273
Kallová, J., Macicková, T., Majtánová, A., Aghová, A., Adam, D., and Kettner, M. (1995). Transferable amikacin resistance in gram-negative bacterial isolates. Chemotherapy 41, 187–192. doi: 10.1159/000239342
Kawamura, K., Goto, K., Nakane, K., and Arakawa, Y. (2014). Molecular epidemiology of extended-spectrum β-lactamases and Escherichia coli isolated from retail foods including chicken meat in Japan. Foodborne Pathog Dis. 11, 104–110. doi: 10.1089/fpd.2013.1608
Kim, H. S., Chon, J. W., Kim, Y. J., Kim, D. H., Kim, M. S., and Seo, K. H. (2015). Prevalence and characterization of extended-spectrum-β-lactamase-producing Escherichia coli and Klebsiella pneumoniae in ready-to-eat vegetables. Int. J. Food Microbiol. 207, 83–86. doi: 10.1016/j.ijfoodmicro.2015.04.049
Korzeniewska, E., and Harnisz, M. (2013). Beta-lactamase-producing Enterobacteriaceae in hospital effluents. J. Environ. Manage. 123, 1–7. doi: 10.1016/j.jenvman.2013.03.024
Laxminarayan, R., Duse, A., Wattal, C., Zaidi, A. K. M., Wertheim, H. F. L., Sumpradit, N., et al. (2013). Antibiotic resistance-the need for global solutions. Lancet Infect. Dis. 13, 1057–1098. doi: 10.1016/S1473-3099(13)70318-9
Lee, J. H., Bae, I. K., and Lee, S. H. (2012). New definitions of extended-spectrum beta-lactamase conferring worldwide emerging antibiotic resistance. Med. Res. Rev. 32, 216–232. doi: 10.1002/med.20210
Livermore, D. M. (2008). Defining an extended-spectrum beta-lactamase. Clin. Microbiol. Infect. 14(Suppl. 1), 3–10. doi: 10.1111/j.1469-0691.2007.01857.x
Lu, S. Y., Zhang, Y. L., Geng, S. N., Li, T. Y., Ye, Z. M., Zhang, D. S., et al. (2010). High diversity of extended-spectrum beta-lactamase-producing bacteria in an urban river sediment habitat. Appl. Environ. Microbiol. 76, 5972–5976. doi: 10.1128/AEM.00711-10.
Maravić, A., Skočibušić, M., Cvjetan, S., Šamanić, I., Fredotović, Ž., and Puizina, J. (2015). Prevalence and diversity of extended-spectrum-β-lactamase-producing Enterobacteriaceae from marine beach waters. Marine Pollut. Bull. 90, 60–67. doi: 10.1016/j.marpolbul.2014.11.021
Mokracka, J., Koczura, R., and Kaznowski, A. (2012). Multiresistant Enterobacteriaceae with class 1 and class 2 integrons in a municipal wastewater treatment plant. Water Res. 46, 3353–3363. doi: 10.1016/j.watres.2012.03.037
Nguyen, D. T. A., Kanki, M., Nguyen, P., Le, H. T., Ngo, P. T., Tran, D. N. M., et al. (2016). Prevalence, antibiotic resistance, and extended-spectrum and AmpC β-lactamase productivity of Salmonella isolates from raw meat and seafood samples in Ho Chi Minh City, Vietnam. Int. J. Food Microbiol. 236, 115–122. doi: 10.1016/j.ijfoodmicro.2016.07.017
Nordmann, P., Naas, T., and Poirel, L. (2011). Global spread of carbapenemase-producing Enterobacteriaceae. Emerg. Infect. Dis. 17, 1791–1798. doi: 10.3201/eid1710.110655
Odenthal, S., Akineden, Ö., and Usleber, E. (2016). Extended-spectrum β-lactamase producing Enterobacteriaceae in bulk tank milk from German dairy farms. Int. J. Food Microbiol. 238, 72–78. doi: 10.1016/j.ijfoodmicro.2016.08.036
Ojer-Usoz, E., González, D., Vitas, A. I., Leiva, J., García-Jalón, I., Febles-Casquero, A., et al. (2013). Prevalence of extended-spectrum β-lactamase-producing Enterobacteriaceae in meat products sold in Navarra. Meat. Sci. 93, 316–321. doi: 10.1016/j.meatsci.2012.09.009
Parka, Y. J., Parka, S. Y., Oha, E. J., Parka, J. J., Leea, K. Y., Woob, G. J., et al. (2005). Occurrence of extended-spectrum β-lactamases among chromosomal AmpC-producing Enterobacter cloacae, Citrobacter freundii, and Serratia marcescens in Korea and investigation of screening criteria. Diagn. Microbiol. Inf. Dis. 51, 265–269. doi: 10.1016/j.diagmicrobio.2004.11.009
Paterson, D., Ko, W. C., and Gottberg, A. (2001). Outcome of cephalosporin treatment for serious infections due to apparently susceptible organisms producing extended-spectrum β-lactamases: implications for the clinical microbiology laboratory. J. Clin. Microbiol. 39, 2206–2212. doi: 10.1128/JCM.39.6.2206-2212.2001
Peirano, G., Sang, J. H., Pitondo-Silva, A., Laupland, K. B., and Pitout, J. D. (2012). Molecular epidemiology of extended-spectrum-beta-lactamase-producing Klebsiella pneumoniae over a 10 year period in Calgary, Canada. J. Antimicrob. Chemother. 67, 1114–1120. doi: 10.1093/jac/dks026
Pfeiier, Y., Cullik, A., and Witte, W. (2010). Resistance to cephalosporins and carbapenems in Gram-negative bacterial pathogens. Int. J. Med. Microbiol. 300, 371–379. doi: 10.1016/j.ijmm.2010.04.005
Pitout, J. D. (2012). Extraintestinal pathogenic Escherichia coli: an update on antimicrobial resistance, laboratory diagnosis and treatment. Expert Rev. Anti Infect. Ther. 10, 1165–1176. doi: 10.1586/eri.12.110.
Poirel, L., Bonnin, R. A., and Nordmann, P. (2012). Genetic support and diversity of acquired extended-spectrum β-lactamases in Gram-negative rods[J]. Infect. Genet. Evol. 12, 883–893. doi: 10.1016/j.meegid.2012.02.008
Reuland, E. A., AlNaiemi, N., Raadsen, S. A., Savelkoul, P. H. M., Kluytmans, J. A. J. W., and Vandenbroucke-Grauls, C. M. J. E. (2014). Prevalence of ESBL-producing Enterobacteriaceae in raw vegetables. Eur. J. Clin. Microbiol. Infect. Dis. 33, 1843–1846. doi: 10.1007/s10096-014-2142-7
Rupp, M. E., and Fey, P. D. (2003). Extended spectrum β-lactamase (ESBL) producing Enterobacteriaceae considerations for diagnosis, prevention and drug treatment. Drugs. 64, 353–365. doi: 10.2165/00003495-200363040-00002
Schilla, F., Abdulmawjood, A., Klein, G., and Reich, F. (2017). Prevalence and characterization of extended-spectrum β-lactamase (ESBL) and AmpC β-lactamase producing Enterobacteriaceae in fresh pork meat at processing level in Germany. Int. J. Food Microbiol. 257, 58–66. doi: 10.1016/j.ijfoodmicro.2017.06.010
Schmiedel, J., Falgenhauer, L., Domann, E., Bauerfeind, R., Prenger-Berninghoff, E., Imirzalioglu, C., et al. (2014). Multiresistant extended-spectrum β-lactamase-producing Enterobacteriaceae from humans, companion animals and horses in central Hesse, Germany. BMC Microbiol. 14:187. doi: 10.1186/1471-2180-14-187
Tekiner, I. H., and Özpinar, H. (2016). Occurrence and characteristics of extended spectrum beta-lactamases-producing Enterobacteriaceae from foods of animal origin. Braz. J. Microbiol. 47, 444–451. doi: 10.1016/j.bjm.2015.11.034
van Hoek, A. H., Veenman, C., Overbeek, W. M., Lynch, G., Husman, A. M. R., and Blaak, H. (2015). Prevalence and characterization of ESBL- and AmpC-producing Enterobacteriaceae on retail vegetables. Int. J. Food Microbiol. 204, 1–8. doi: 10.1016/j.ijfoodmicro.2015.03.014
Wang, Y., He, T., Han, J., Wang, J., Foley, S. L., Yang, G. Y., et al. (2012). Prevalence of ESBLs and PMQR genes in fecal Escherichia coli isolated from the non-human primates in six zoos in China. Vet. Microbiol. 159, 53–59. doi: 10.1016/j.vetmic.2012.03.009
Xiao, Y. H., Giske, C. G., Wei, Z. Q., Shen, P., Heddini, A., and Li, L. J. (2011). Epidemiology and characteristics of antimicrobial resistance in China. Drug Resist Updat 14, 236–250. doi: 10.1016/j.drup.2011.07.001
Xiao, Y. H., Wei, Z. Q., Shen, P., Ji, J. R., Sun, Z. Y., Yu, H., et al. (2015). Bacterial-resistance among outpatients of county hospitals in China: significant geographic distinctions and minor differences between central cities. Microbes Infect. 17, 417–425. doi: 10.1016/j.micinf.2015.02.001
Yang, B. W., Wang, Q. N., Cui, S. H., Wang, Y., Shi, C., Xia, X. D., et al. (2014). Characterization of extended-spectrum beta-lactamases-producing Salmonella strains isolated from retail foods in Shaanxi and Henan Province, China. Food Microbiol. 42, 14–18. doi: 10.1016/j.fm.2014.02.003
Yang, X. J., Huang, J. H., Wu, Q. P., Zhang, J. M., Liu, S. R., Guo, W. P., et al. (2016). Prevalence, antimicrobial resistance and genetic diversity of Salmonella isolated from retail ready-to-eat foods in China. Food Control. 60, 50–56. doi: 10.1016/j.foodcont.2015.07.019
Zarfel, G., Galler, H., Luxner, J., Petternel, C., Reinthaler, F. F., Haas, D., et al. (2014). Multiresistant bacteria isolated from chicken meat in Austria. Int. J. Environ. Res. Public Health 11, 12582–12593. doi: 10.3390/ijerph111212582
Zhang, S. H., Wu, Q. P., Zhang, J. M., Lai, Z. B., and Zhu, X. M. (2016). Prevalence, genetic diversity, and antibiotic resistance of enterotoxigenic Escherichia coli in retail ready-to-eat foods inChina. Food Control. 68, 236–243. doi: 10.1016/j.foodcont.2016.03.051
Zheng, H. Q., Zeng, Z. L., Chen, S., Liu, Y. H., Yao, Q. F., Deng, Y. T., et al. (2012). Prevalence and characterisation of CTX-M -lactamases amongst Escherichia coli isolates from healthy food animals in China. Int. J. Antimicrobial Agents 39, 305–310. doi: 10.1016/j.ijantimicag.2011.12.00
Keywords: Enterobacteriaceae, extended spectrum β-lactamase, antimicrobial resistance, β-lactamase, retail food, China
Citation: Ye Q, Wu Q, Zhang S, Zhang J, Yang G, Wang J, Xue L and Chen M (2018) Characterization of Extended-Spectrum β-Lactamase-Producing Enterobacteriaceae From Retail Food in China. Front. Microbiol. 9:1709. doi: 10.3389/fmicb.2018.01709
Received: 03 April 2018; Accepted: 09 July 2018;
Published: 08 August 2018.
Edited by:
Chunbao Li, Nanjing Agricultural University, ChinaReviewed by:
Vesna Milanović, Università Politecnica delle Marche, ItalyMichael P. Ryan, University of Limerick, Ireland
Copyright © 2018 Ye, Wu, Zhang, Zhang, Yang, Wang, Xue and Chen. This is an open-access article distributed under the terms of the Creative Commons Attribution License (CC BY). The use, distribution or reproduction in other forums is permitted, provided the original author(s) and the copyright owner(s) are credited and that the original publication in this journal is cited, in accordance with accepted academic practice. No use, distribution or reproduction is permitted which does not comply with these terms.
*Correspondence: Qingping Wu, d3VxcDIwM0AxNjMuY29t