- 1Department of Endodontics, Faculty of Dentistry, Tanta University, Tanta, Egypt
- 2Department of Restorative Dental Sciences, College of Dentistry, King Khalid University, Abha, Saudi Arabia
- 3Department of Pharmaceutical Microbiology, Faculty of Pharmacy, Tanta University, Tanta, Egypt
- 4Biopharmacetical Product Research Department, Genetic Engineering and Biotechnology Research Institute, City of Scientific Research and Technology Applications, Alexandria, Egypt
This study was conducted to investigate the antimicrobial-biofilm activity of chitosan (Ch-NPs), silver nanoparticles (Ag-NPs), ozonated olive oil (O3-oil) either separately or combined together against endodontic pathogens. While testing the antimicrobial activity, Ch-NPs showed the least minimum inhibitory concentration (MIC) and minimum bactericidal concentration (MBC) values exerting eightfold higher bactericidal activity than O3-oil against both Enterococcus faecalis and Streptococcus mutans as well as fourfold higher fungicidal activity against Candida albicans. Antimicrobial synergy test revealed synergism between O3-oil and Ch-NPs against the test pathogens (FIC index ≤ 0.5). Ch-NPs was superior at inhibiting immature single and mixed-species biofilm formations by 97 and 94%, respectively. Both of O3-oil and Ch-NPs had a complete anti-fibroblast adherent effect. The safety pattern results showed that O3-oil was the safest compound, followed by Ch-NPs. The double combination of Ch-NPs and O3-oil reduced the mature viable biofilm on premolars ex vivo model by 6-log reductions, with a fast kill rate, indicating potential use in treating root canals. Therefore, the double combination has the potential to eradicate mature mixed-species biofilms and hence it is potent, novel and safe.
Introduction
The primary goal of endodontic therapy is to eradicate microbial infection and promote periapical tissue healing. Endodontic infections are polymicrobial and made up of various microorganisms that differ between failed endodontic cases and primary endodontic infections (Nair, 2006; Tirali et al., 2017).
Gram-negative, black-pigmented anaerobes are typically associated with primary endodontic infections, whereas Gram-positive streptococci and enterococci, particularly Enterococcus faecalis and Streptococcus mutans, and Candida albicans are more common in failed or persistent endodontic infections (Peciuliene et al., 2000; Nair, 2006; Tirali et al., 2017).
In endodontic microbiology, biofilm-mediated infection is the primary cause of endodontic disease. Bacterial biofilm is made up of bacterial cell colonies that form an extracellular matrix substrate that adheres to organic surfaces. Persistent and chronic endodontic infections depend in part on the density, diversity and resistance of bacterial biofilms to both host defense mechanisms and antimicrobial agents. Other contributing factors are the complexity of the root canal anatomy including the lateral canals, ramifications and tubular nature of the dentin that harbors bacterial colonies (Kishen, 2010; Siqueira et al., 2010).
Chemomechanical debridement of root canals is reported to be effective for eliminating bacterial biofilm and disinfecting root canals (Kishen, 2010). However, the current intracanal medicament and irrigation solutions can be harmful and cytotoxic to the host tissue and do not completely eradicate bacterial biofilm from the root canal. The insufficient disinfection capability of root canal chemomechanical debridement advocated the development of more advanced root canal disinfection methods (Casal et al., 2009; Kishen, 2010; Siqueira et al., 2010).
Recently, nanoparticles, which are particles sized less than 100 nm, have demonstrated broad spectrum antimicrobial activity (Samuel and Guggenbichler, 2004). Biocompatibility was defined by Williams (2008) as the ability of nanoparticles to exert their biological activity without causing adverse reactions neither local nor systemic in the patients receiving the therapy. On the reverse, toxicity of NPs means their ability to disturb the normal physiology as well as damaging tissues and organs. Some studies showed that NPs could weaken the alveolar macrophage activity. For instance, silver NPs showed lung toxicity in the form of alveolar inflammation and granulomatous changes leading to a reduced pulmonary function (Sung et al., 2008). On the contrary, Chitosan is a non-toxic natural biopolymer of de-acetylated chitin that is of interest in the dental profession for its antimicrobial activity. Chitosan may also contribute to dentin remineralization by bonding to dentin calcium at its functional phosphate group. It is also a biodegradable, biocompatible broad-spectrum antimicrobial agent. Chitosan’s antimicrobial activity is affected by its solvent activity and molecular weight, and this activity increases at lower pH values (No et al., 2002; Xu et al., 2011; Li et al., 2012).
Over the last decade, ozone has been used in the medical field for its antiviral, antifungal and bactericidal activity as well as its high biocompatibility (Valacchi et al., 2005). Additionally, ozonated oils have been used to topically treat chronic ulcers and mucosal infections (Martinez-Sanchez et al., 2005). Ozonated olive oil exhibits high germicidal and oxygenating power against many microorganisms, favoring tissue healing and regeneration, and thus has been used on post-extraction alveolitis (Agapov et al., 2002).
Little is known about the efficacy of ozonated olive oil, silver nanoparticles or chitosan nanoparticles against microbial species isolated from persistent endodontic infections. The current study was conducted to evaluate the antimicrobial effect of these preparations against E. faecalis, S. mutans, and C. albicans, the predominant microbes in failed endodontic cases.
Materials and Methods
Test Materials
This study evaluated three test substances: an ozonated extra virgin olive oil (Novox®, MOSS S.r.l., Lesa – Novara, Italy) containing active oxygen in the form of peroxide ranged between 560–590 mmol-equiv/kg, prepared by introducing olive oil through a device generating ozone (Díaz et al., 2006); chitosan NPs (Ch-NPs) prepared by an ionotropic gelation technique (Sailaja et al., 2011) (NanoTech Egypt company for Photo-Electronics); and silver nanoparticles prepared by a chemical reduction method (Ratyakshi and Chauhan, 2009) (NanoTech Egypt company for Photo-Electronics).
Stock solutions (100 mg/ml) of Ag-NPs in sterile distilled water, Ch-NPs in 5% glacial acetic acid (HAC) and O3-oil in dimethylsulfoxide (DMSO) were prepared.
Test Microorganisms
Freeze-dried reference microorganisms, S. mutans ATCC 2419, E. faecalis OG1RF, and C. albicans MTCC 227, were used, which were obtained from the Department of Pharmaceutical Microbiology, Faculty of Pharmacy, Tanta University, Egypt.
Cell Line
Human Gingival Fibroblast cells (ATCC® PCS-201-012TM) were obtained from the laboratory of the Tissue Culture Department of the Holding Company for Biological Products and Vaccines (VACSERA), Cairo, Egypt.
Susceptibility of the Test Strains to Different Root Canal Medicaments
The antimicrobial activity of the tested root canal disinfectants was examined by determining the minimum inhibitory concentrations (MICs), minimum bactericidal concentrations (MBCs) and minimum fungicidal concentrations (MFCs) against endodontic pathogens using broth microdilution assay. The turbidity of each microbial suspension was adjusted to that of a 0.5 MacFarland standard then diluted in RPMI 1640 to give a final inoculum of 106 CFU/ml. This inoculum was added to the wells of a 96-well microtitration plate containing twofold serial dilutions of the test medicament ranged from 0.039–40 mg/ml. Medium without inoculum was considered as a blank control. Inoculated medium without treatment was used as positive control. The wells were incubated for 24 h at 37°C, and then the OD530 was recorded using TECAN SunriseTM microtitre plate reader (Austria). MIC was defined as the lowest concentration of each medicament that inhibited the microbial growth compared to the untreated control culture. MBC was determined by performing viable count (CFU/mL) following 24 h of incubation at 37°C and was defined as the lowest medicament concentration that reduced the viable cells by more than 3 log10 steps (>99.9%) compared to the untreated control cultures. Independent assays were performed in triplicates (Gahlaut and Chhillar, 2013; Clinical and Laboratory Standards Institute [CLSI], 2016; Kaur et al., 2017). The antimicrobial synergy effect of different medicaments combinations was also evaluated in vitro using Checkerboard method as previously described by Tin et al. (2009). Briefly, for testing the combined effect of O3-oil and Ch-NPs, 96-well microtitre plate was used where the rows contained the same concentrations of O3-oil which was diluted twofold (from 20 to 0.3125 mg/ml) along the columns. The same concentrations of Ch-NPs are present in the columns which was diluted twofold (from 2.5 to 0.0391 mg/ml) along the rows. The wells were inoculated by the test pathogen at 106 CFU/ml and the plates were incubated at 37°C for 24 h. For detecting the combined synergy effect, fractional inhibitory concentration (FIC) index was calculated as follows:
Where FIC A = MIC of drug A in the combination/MIC of drug A alone.
FIC B = MIC of drug B in combination/MIC of drug B alone.
The results were interpreted as synergism if the FIC index ≤0.5; additive effect if the FIC index of >0.5 and ≤4; and antagonism if the FIC index >4. The procedure was replicated three times. Similarly, the antimicrobial synergy effect of Ch-NPs/Ag-NPs and O3-oil/Ag-NPs was assessed by the same procedure.
Cytotoxicity Analysis of the Test Medicaments on Human Fibroblast
The safety of the test compounds was evaluated on normal human fibroblasts. Briefly, approximately 100 μl of each of serially diluted compound was incubated with pre-cultured (6 × 104 cell/ml) on 96-well plates. After 48 h, the cellular cytotoxic effects were quantified using the neutral red assay protocol (Borenfreund and Puerner, 1985). Half maximal inhibitory concentration (IC50) was calculated for each test medicament.
Biofilm Assay Using Crystal Violet Quantitative Assay
The effect of the test medicaments on the biofilm biomass was evaluated by crystal violet binding assay (O’Toole, 2011). Overnight culture of each test strain was standardized to contain 106 CFU/ml in trypticase soy broth. For generating dual or triple species biofilm, equal proportions of the standardized microbial suspensions were mixed together. About 100 μl of either of single, double or triple microbial suspension was dispensed to the corresponding well in a polystyrene 96-well plate containing the IC50 of the test compounds. Blank controls (medium without inoculum) were considered and inoculated medium without treatment was used as a positive control. After incubation at 37°C for 24 h, the planktonic cells were removed by aspiration of the spent media. All wells were washed twice using phosphate buffered saline solution (PBS) (pH 7.4) to remove loosely bound microbial cells and media components. Next, 125 μl of 0.1% crystal violet solution was added to each well to stain biofilm forming cells, incubated for 10 min at room temperature then rinsed three times with distilled water. For the quantitative assay, crystal violet was solubilized by adding 125 μl of 30% acetic acid to each well and incubated for 10 min at room temperature. The absorbance was measured for both the control and test groups by a plate reader at 550 nm with a reference filter of 570 nm.
Effect of the Test Medicaments on Microbial Adhesion as a Virulence Factor Using Human Fibroblasts
Fibroblasts were cultured in Dulbecco’s Modified Eagle’s Medium (DMEM) (Lonza, United States) supplemented with 10% fetal bovine serum (FBS) (Lonza, United States) and 1% streptomycin/penicillin in 6-well plates (Greiner Bio-one, Germany) at approximately 1 × 105 cells/ml then incubated at 37°C in a 5% CO2 atmosphere until they formed semi-confluent monolayers. Twenty-four hours post-incubation, the exhausted media were replaced with fresh DMEM. Overnight cultures of the test microbes were standardized to a final inoculum of 107 CFU/ml using 0.5 MacFarland standard and confirmed by viable count. Approximately 50 μl of each standardized microbial cell cultures, pretreated with non-toxic doses of the medicaments, were incubated at 37°C for 3 h. After incubation, the medium was discarded and the wells were washed three times with PBS to remove the unbound microbial cells. One milliliter of methanol was added to each well for 1 min, then replaced with 4 ml of freshly diluted Giemsa stain (1:10) and incubated for 30 min at room temperature. The stain was discarded, and the fibroblast monolayers were washed sequentially with tap water, acidified water (1 ml concentrated H2SO4 in 1 L of tap water) and tap water again. The wells were examined at 20× magnification under a light microscope (Olympus, Carlsbad, CA, United States). The microbial strains were considered adherent if they formed characteristic microcolonies on >40% of the fibroblast cell surface. The data represented at least three trials (Cravioto et al., 1979; Presterl et al., 2003).
Antibiofilm Challenge of the Test Medicaments Against Endodonotic Pathogens Using an ex Vivo Premolar Teeth Model
Among the tested medicaments, Ch-NPs and O3-oil were selected due to antibiofilm activity at their IC50. The effect of the later medicaments, either separate or combined together, was evaluated on the mature multi-species biofilm ex vivo using extracted premolar teeth. The effect of DMSO or 5% HAC, as vehicles, on the microbial biofilm was also assessed.
Preparation of the Extracted Premolar Teeth
A total of 168 intact single rooted human premolars with single straight root canals extracted for periodontal or orthodontic reasons were collected after obtaining the patients’ written consent. This was based on the protocol approved by The Research Ethics Committee at the Faculty of Dentistry, Tanta University, Egypt. The teeth were collected in 2.5% sodium hypochlorite solution then mechanically cleaned and decoronated under water coolant using a diamond disk (Edenta AG, AU/SG, and Switzerland). The step-back technique was used to chemomechanically debride the canals using stainless steel k-files (Dentsply/Maillefer, Ballaigues, Switzerland) and 2.5% sodium hypochlorite as an irrigant with a master apical file size 40.
The apical 3 mm as well as crown (2–3 mm) of each root specimen was cut using the diamond disk to obtain 12 mm-long standardized root specimens. The canal lumen for each specimen was prepared to a standardized size by passing rotating peeso drills, sizes 1 and 2, from the coronal end until just visible at the apical end. A 2 mm-deep cavity was prepared on the apical ends of each canal using #4 slow speed round bur (Dentsply/Maillefer) and sealed with acrylic resin to prevent micro leakage. The prepared root canals were conditioned with 10 mL of 17% EDTA (Canal +, Septodont, France) for 60 s to remove the smear layer, washed with 10 mL of 5.25% NaOCl, flushed with 10 mL of saline solution and dried with paper points (Dentsply-Maillefer, Ballaigues, Switzerland). Teeth were subjected to sterilization by steam autoclave for 15 m at 121°C (Samuel and Guggenbichler, 2004; Gomes-Filho et al., 2010).
Generation of Mature Biofilm in Premolars
A single colony from the overnight cultures of each microbial strain was suspended in sterile brain heart infusion broth to prepare 106 CFU/ml using the 0.5 McFarland standard. Equal portions of each microbial suspension were mixed together in a Falcon tube to be used for generating multiple species biofilm. Premolar teeth were aseptically transferred to the tube containing the mixed microbial suspension, then incubated under anaerobic conditions at 37°C for 1 week to obtain a mature multi-species biofilm. The culture broth was refreshed every 2nd day to ensure bacterial viability and remove dead cells. At the end of the incubation period, specimens were aseptically removed from the tubes and washed with sterile PBS to remove unbound microbes and culture media. Another Falcon tube contained teeth in a medium without inoculation was used as negative control (Flemming and Wingender, 2010).
Application of Different Medicaments Into Biofilm Containing Premolars
Fifty six teeth were divided into eight groups (n = 7) to test the three different intracanal medicaments including; O3-oil, Ch-NPs and a combination of both. The control group (G.4, n = 7) was inoculated and kept untreated in the incubator for the remainder of the test. The negative control group (G.5, n = 7) in which the medium was free from inoculum and treatment to exclude contamination throughout the experiment. Vehicles (DMSO or 5% HAC) were also tested to exclude their effect on mature biofilm (G.6 and G.7, respectively). The positive control group (G. 8) which test calcium hydroxide suspension. Test samples were irrigated with sterile saline for 2 m and dried with paper points. The test medicaments were injected into the corresponding root using syringe and canal orifices, then aseptically sealed with modeling wax (Cavex, Holland). The specimens were transferred into sterile petri dishes and covered with humid sterile gauze. The plates were incubated at 37°C for 1 week and evaluated daily for viability of microbial cells (Flemming and Wingender, 2010). This experiment was repeated three times.
Quantitative Determination of Viable Microbes in the Biofilm
Viable microbes were quantified in the biofilm post-treatment. The wax seal was removed from the canal orifice of one root from each group daily. Each canal was flushed with sterile saline solution along with light circumferential filing using the master apical file to remove intracanal medicament. Root canals were dried with sterile paper points.
A 400 μm-deep sample from the dentin lining of each canal was collected by passing a rotating size 4 peeso drill from the coronal to the apical ends of each sample. Dentin debris obtained from the canal walls were immediately collected onto separate Eppendorf tubes to a weight of 10 mg and serially diluted. The harvested dentin debris was added to 1 ml of sterile saline, then vortexed for 30 s to collect the microbes on the dentin debris. Each sample was serially diluted twofold for five dilutions, then 10 μL of each specimen were plated out on brain heart infusion agar plates and incubated at 37°C for 24 h. Viable microbes were counted and represented as colony-forming units (CFU/ml). This procedure was repeated three times. The CFU values were converted to log10 and the mean log values with standard deviations (SD) were calculated (Flemming and Wingender, 2010).
Statistical Analysis
The data were analyzed using IBM SPSS statistics for Windows, version 21.0 (IBM Corp., Armonk, NY, United States). One-way ANOVA, and Tukey’s test at α = 0.05 were used to determine significant differences between the groups. Graph Pad Prism was used to calculate the IC50 from the plotted curves of the cytotoxicity assay.
Results
Broth microdilution assay revealed that all of the test medicaments had high MIC and MBC values as recorded in Table 1. Ch-NPs exerted 4- and 8-fold increases in the microbicidal activity compared to O3-oil against C. albicans, and both of E. faecalis and S. mutans, respectively. Synergism (FIC index ≤ 0.5) was recorded for O3-oil/Ch-NPs combination when it was assessed for the antimicrobial synergy in vitro against the test endodontic pathogens. Moreover, an additive effect (FIC index of >0.5 and ≤4) was noticed when O3-oil/Ag-NPs and Ch-NPs/Ag-NPs combinations were tested as recorded in Table 2.
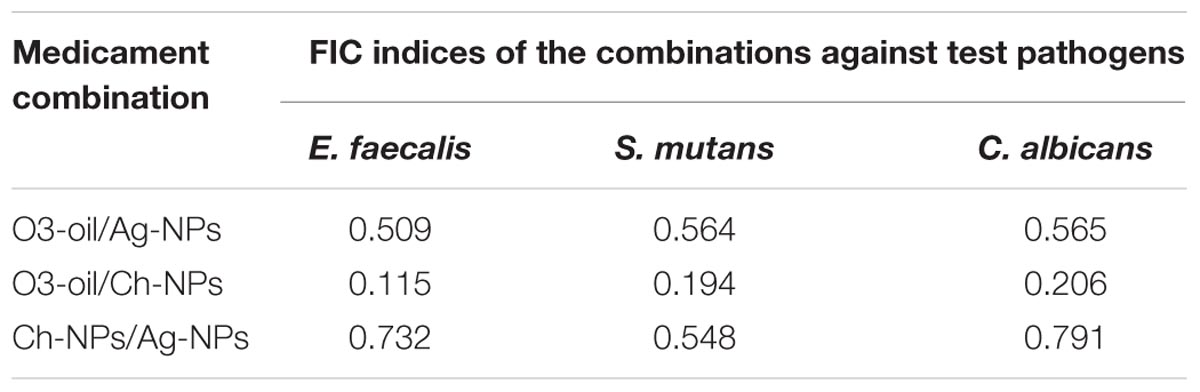
TABLE 2. FIC indices for different combinations of the test root canal medicaments against persistent endodontic pathogens.
The neutral red assay results for testing cytotoxicity on human fibroblasts are shown in (Figure 1). The IC50 for the three test disinfectants was calculated from the plotted curves and ranged from 0.039–10 mg/ml. One-way ANOVA revealed a significant difference (p < 0.001) between the test groups and the untreated control group. The IC50 of O3-oil was the safest, followed by the Ch-NPs (1.25 mg/ml).
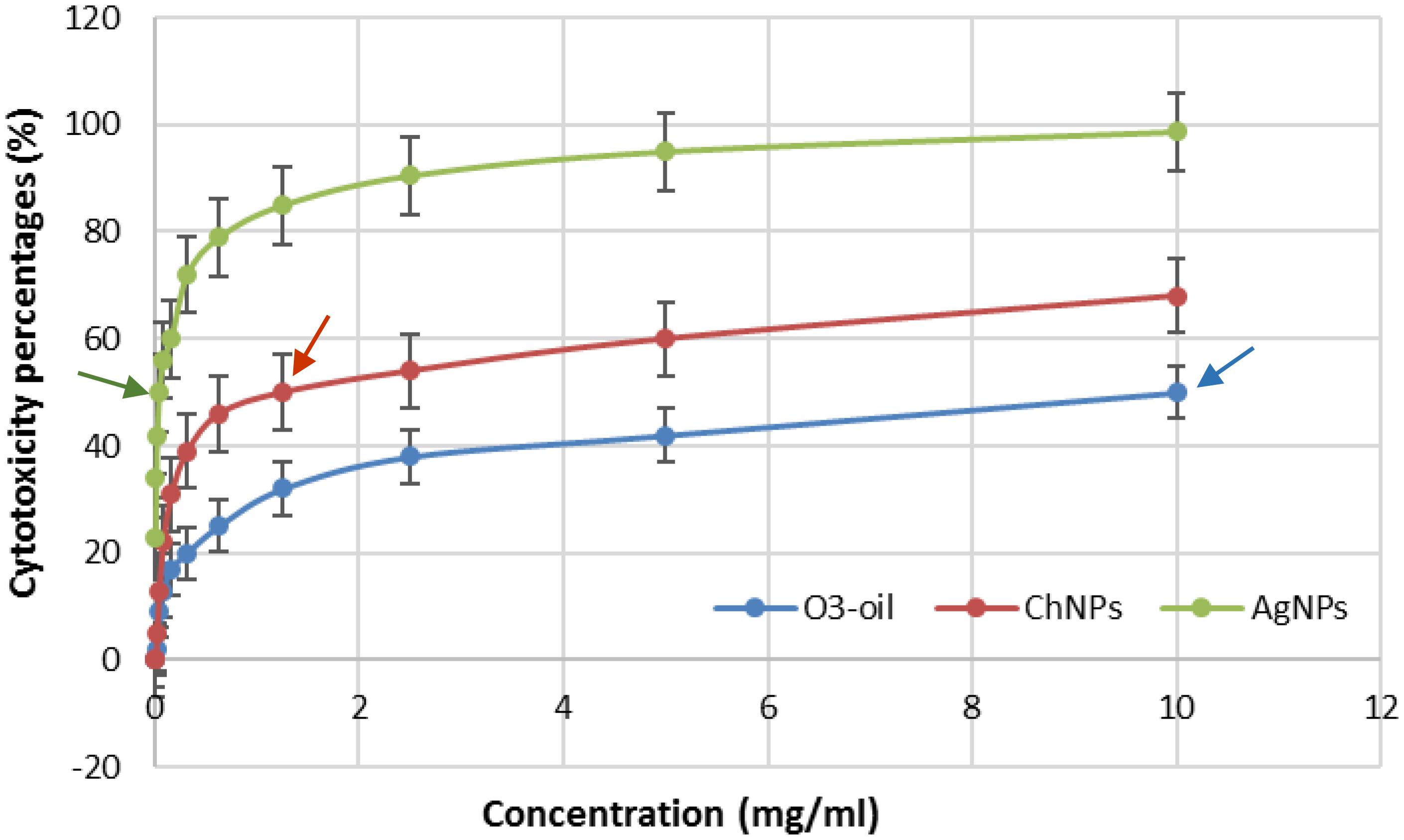
FIGURE 1. Cytotoxicity test of Ch-NPs, O3-oil, and Ag-NPs on human fibroblast. Arrows point to the IC50 of each medicament.
Effect of the test medicaments on the formation of single or mixed microbial species biofilms was evaluated by a crystal violet assay that measures only biomass by binding of positively charged CV to all negatively charged residues in the biofilm. The Ch-NP solutions had the lowest mean value of absorbance, followed by O3-oil then the Ag-NP solutions, while the control group had greatest mean value (Figure 2). Ch-NPs reduced single and mixed species biofilm by 97 and 94%, respectively. Furthermore, O3-oil exerted 86 and 79% reduction in biofilm, respectively. However, Ag-NPs showed non-significant reduction. Notably, the control group was significantly different from the other groups.
Antiadherent test was assessed using Giemsa staining and microscopy (Figure 3). It revealed that the Ch-NPs and O3-oil were the most effective at reducing the fibroblast-microbial cell adhesion by reducing the number of fibroblast-associated microbes to almost zero (p < 0.05) compared to the untreated microbial cells (control), which showed high adhesion indices. Non-significant reduction in fibroblast-bacterial cell adhesion was also noted with Ag-NPs. Moreover, it had less effect on C. albicans-fibroblast adhesion (Table 3). The time-kill kinetics for the different test treatments showed that killing was time-dependent throughout the 7-day incubation with the mature biofilm. ANOVA revealed a significant difference between groups (p < 0.05). The fastest kill rate was recorded for the double combination (O3-oil/Ch-NPs), as it significantly reduced (6-log reduction) the number of survivors by the 2nd day of treatment. The separate medicaments had slower kill rates than the combined one (Figure 4). There were no significant effect for DMSO or 5% HAC on the microbial biofilm as compared to the untreated control group (Figure 4).
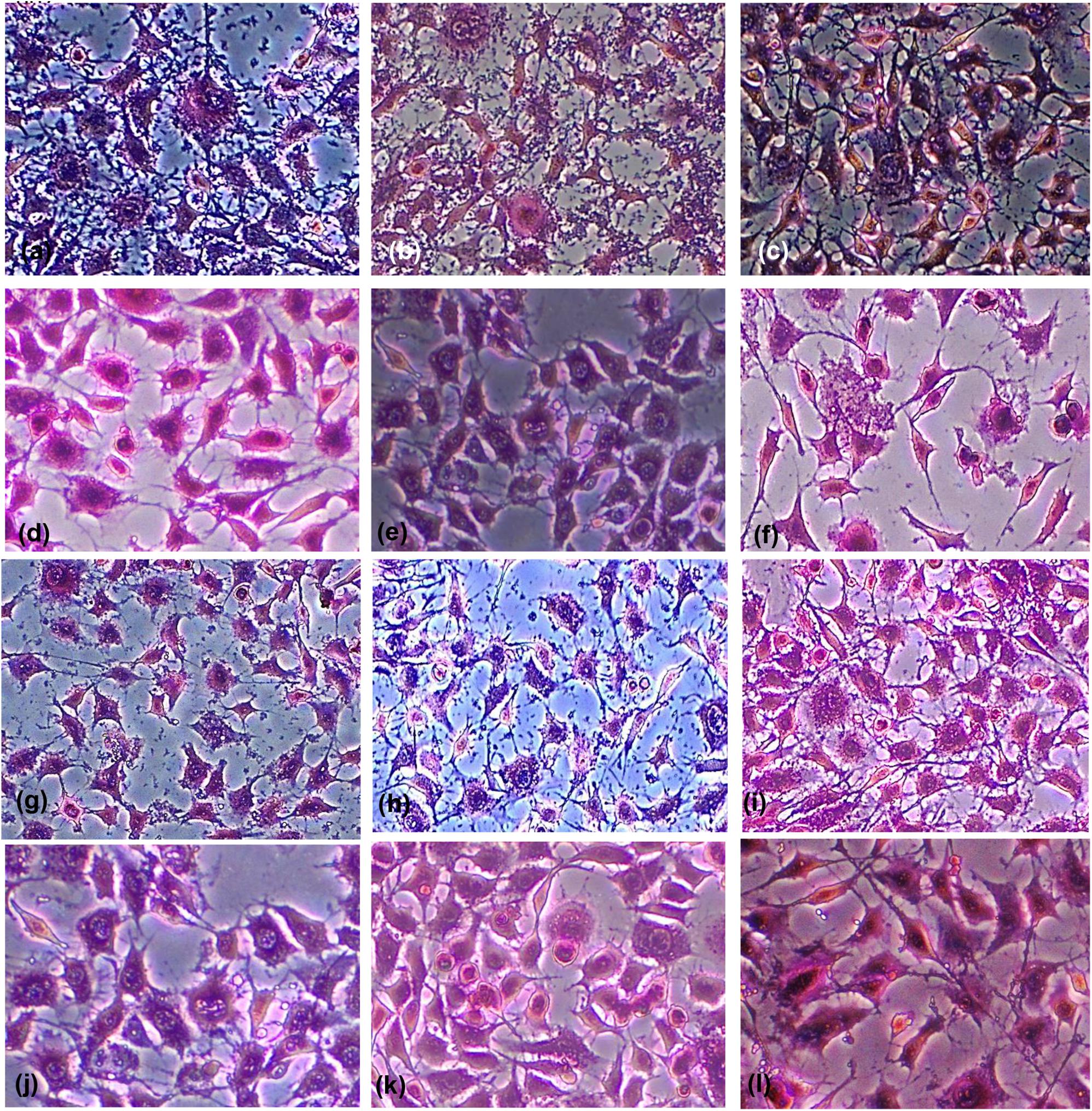
FIGURE 3. Light micrographs of Giemsa-stained untreated fibroblast cells showing adherence of (a) Enterococcus faecalis, (b) Streptococcus mutans, and (c) Candida albicans, to the cells a swell as the polystyrene of the microtitration plate. The light micrographs (d–f) show cells treated with Ch-NPs, (g–i) treated with Ag-NPs, (j–l) treated with O3-oil. Magnification 20×.
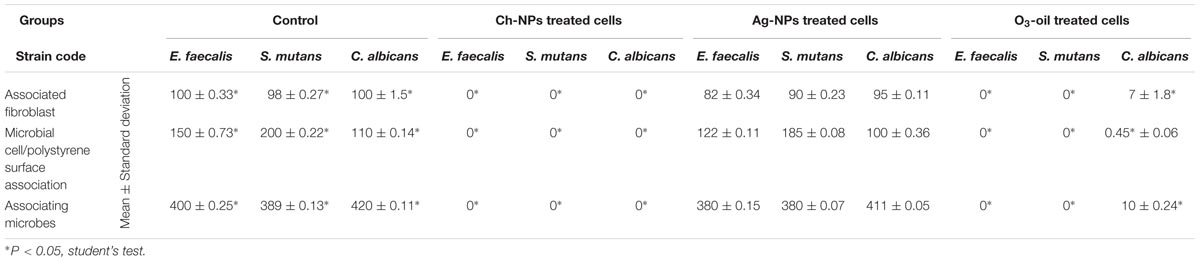
TABLE 3. The mean values of the associating microbial cells to the fibroblast or the polystyrene surface as well as the number of fibroblast associated with the microbial cells, in the presence or absence of the test medicaments.
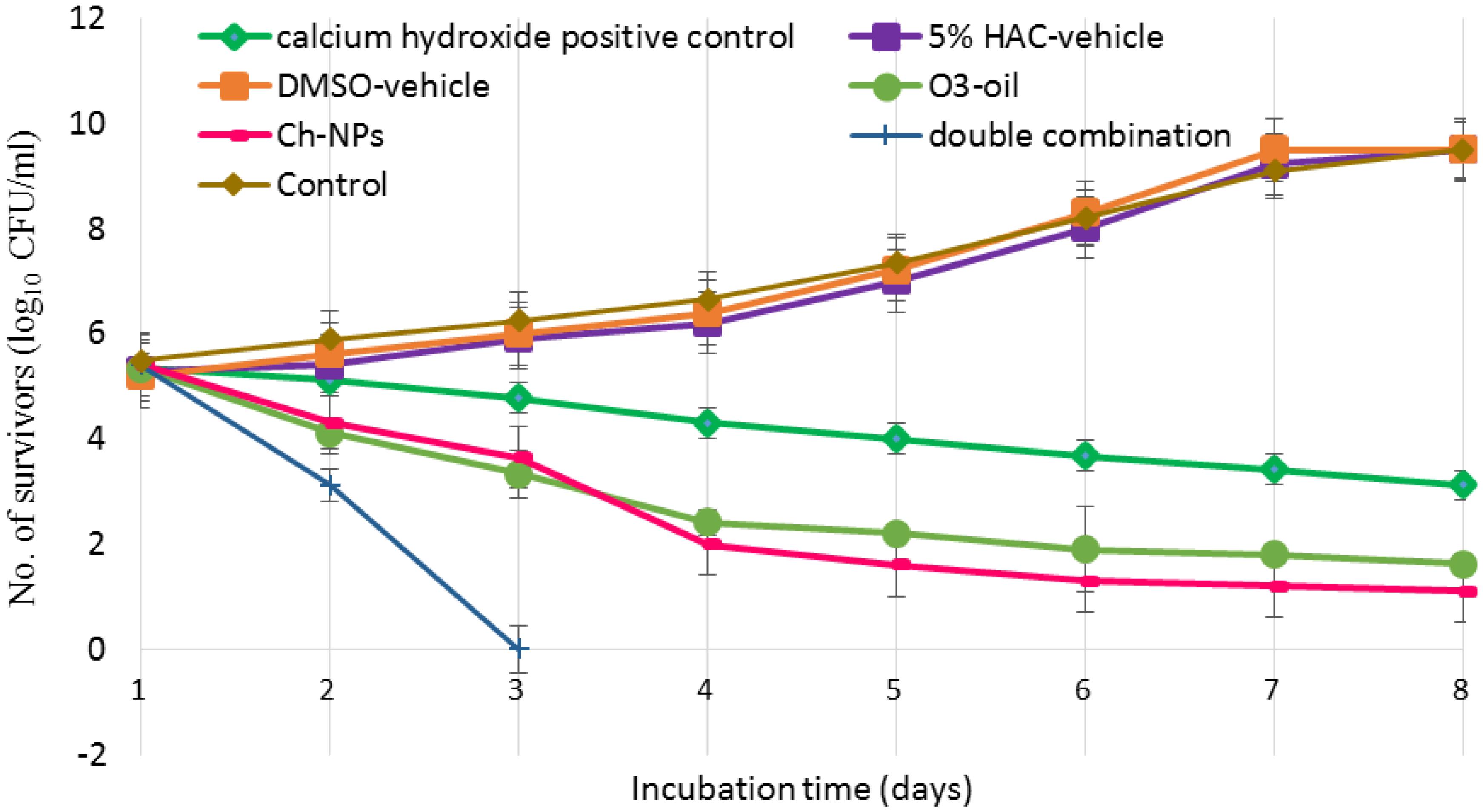
FIGURE 4. Time-kill curves showing the number of microbial survivors recorded day to day in the debris of the dentinal tubules at 400 microns to assess the effect of O3-oil, Ch-NPs, alone or in combination on the mature biofilm developed for 1 week. Data represent mean ± standard deviation.
Discussion
Microbial biofilm is a survival mechanism in which microbes form a strong shield against chemotherapeutic attack (Flemming and Wingender, 2010). Pulpo-periapical diseases are biofilm-mediated infections that are difficult to treat with conventional antimicrobials (Römling and Balsalobre, 2012). The physicochemical environment of the biofilm is markedly modified by C. albicans, which produces β-1,3 glucan, providing stability to the biofilm’s 3D matrix. In addition, extracellular polymeric substance (EPS) secretion helps other pathogens, such as S. mutans, adhere and form microcolonies that enable growth in the presence of C. albicans. The acidity of the biofilm increases as it matures in the presence of acidogenic pathogens such as S. mutans. The coexistence of E. faecalis with these pathogens stimulates production of superoxides that trigger hyphae formation in C. albicans. This results in a more complex biofilm due to the complexity of the bacterial-fungal association (Xiao et al., 2012; Falsetta et al., 2014). Accordingly, innovative approaches are necessary to prevent and eradicate biofilm formation. This study was conducted to assess the antimicrobial, antibiofilm and antivirulent efficacy of Ch-NPs, Ag-NPs, and O3-oil against the S. mutans, E. faecalis, and C. albicans bacterial-fungal biofilm that is encountered in many resistant endodontic infections. The three test disinfectants, along with a novel mixture, were assessed in an ex vivo model of infected root canal premolar teeth specimens.
The antimicrobial effect of Ch-NPs, Ag-NPs, and O3-oil on S. mutans, E. faecalis, and C. albicans was evaluated by routine microbiological techniques such as MIC, MBC, and MFC tests. Aliasghari et al. (2016) reported that 5 mg/ml of Ch-NPs inhibited the growth of S. mutans, showing an inhibition zone diameter of 15 mm. Berenji et al. (2014) stated that O3-oil had powerful bactericidal and fungicidal activity. They reported that the MIC of ozonated olive oil was 233.33 mg/ml against C. albicans. This indicates that our preparation was 47 times more effective. Additionally, Krishnan et al. (2015) stated that the optimum MIC of Ag-NPs against E. faecalis was 5 mg/ml, similar to that of the current study. Conversely, Lotfi et al. (2011) reported that the MIC was 50 μg/ml, and this variation may be due to differences in the Ag-NPs preparation methods as well as particle size. A synergistic effect was recorded for O3-oil/ChNPs combinations against endodontic pathogens with FIC index ≤ 0.5 giving a strong evidence on the better combination of both candidates against the test microbes. A similar result was reported by Tin et al. (2009).
Nanotechnology has gained a great interest due to several health benefits and industrial applications such as drug delivery, cancer treatment, gene therapy and medical imaging. Although nanoparticles has many advantages, nanotoxicity to human and environment gained the attention of the researchers (Ray et al., 2009). These smaller materials might be biodegraded within the cell resulting in their accumulation leading to intracellular changes such as alterations of genes. Argyria is a condition in which the skin is blue–gray colored due to the exposure to high levels of silver NPs, however, the low levels of this NPs might result in breathing problems and allergic reactions (Ray et al., 2009). The study conducted by Burd et al. (2007) tested the cytotoxicity effect of commercially available dressings containing Ag-NPs in a cell culture monolayer. Their study showed that the cytotoxicity was concentration dependent. However, Hernández-Sierra et al. (2011) and Soleimani et al. (2015) reported that Ag-NPs (80–100 nm) showed no apparent cytotoxicity on fibroblasts due to the low concentration. In contrast, the cytotoxicity results in our investigation showed that the most cytotoxic effect was exerted by the Ag-NPs, while the safest effect was reported for O3-oil. This high cytotoxicity of the Ag-NPs was confirmed by previous studies that related this to the toxicity from cytochrome C release, together with the induction of reactive oxygen species, which produce oxidative stress leading to a series of cellular events, including inflammation, membrane lipid peroxidation, apoptosis and DNA damage (Gomes-Filho et al., 2010; Kishen, 2010; Guzman et al., 2012). On the reverse, chitosan was previously approved by FDA as safe polymer (Dash et al., 2011). Additionally, several non-specific enzymes could degrade it. Furthermore, it is able to stabilize the membranes of RBCs and hence it is hemostatic (Aranaz et al., 2010). Similarly, FDA allowed the use of ozonated olive oil on food to fight bacteria. It has also a positive impact on tissue repair and wound healing due to its potent anti-inflammatory effect on the NF-kappa B system and the ability to induce fibroblast proliferation confirming its safety when reach the systemic circulation (Ramzy et al., 2005). Therefore, we used the IC50, safe on the fibroblast, for all medicaments to be tested as anti-biofilm and anti-adherent.
One of the most important problems in treating endodontic disease is the infectious process of the biofilm formation (Jhajharia et al., 2015). In the current study, CV assay was used to measures the biomass by binding of positively charged CV to all negatively charged residues in the biofilm. Both of Ch-NPs and O3-oil significantly (P < 0.05) reduced the total biofilm mass compared to the control groups, specifically the Ch-NPs, which showed 97 and 94% reductions for single and mixed species biofilms, respectively. This agrees with the results of Costa et al. (2014), who reported that Ch-NPs significantly reduced biofilm formation in C. albicans and S. mutans up to 92.5 and 93.4%, respectively. This supports the finding that our medications acted against the biofilm. The slightly lesser effect of the treatments on the mixed species biofilm in this study can be explained by the findings of Bachtiar et al. (2016), who stated that C. albicans was resistant in the presence of the encapsulated E. faecalis ATCC 29212 biofilm at the intermediate stage (24-h). E. faecalis produces peptidoglycan that induces hyphal growth in C. albicans. Consequently, this stimulatory effect at the intermediate stage of biofilm development indicates the ability of E. faecalis and C. albicans to cohabitate. Additionally, Huycke et al. (1998) stated that E. faecalis produces extracellular superoxides that can trigger reactive oxidative stress, inducing filamentation in C. albicans. However, this relationship can become antagonistic with unencapsulated E. faecalis isolates in mature biofilm. Similarly, S. mutans can promote C. albicans biofilm due to their mutualistic relationship (Falsetta et al., 2014). However, the interaction between E. faecalis and S. mutans is variable and strain-dependent (Li et al., 2014).
The first step in biofilm formation depends on the microbe’s ability to adhere to solid surfaces (Shen et al., 2011). One of the mechanisms of action of our treatments was as an antibiofilm. In this work, 1.25 mg/ml of Ch-NPs completely inhibited all test microbes from adhering to normal human fibroblasts as well as to the polystyrene surface of microtitration plates. Aliasghari et al. (2016) reported that 5 mg/ml of Ch-NPs reduced the adhesiveness of cariogenic S. mutans by 93.4%, indicating the effectiveness of our medicine. Additionally, Di Giulio et al. (2013) reported that the chitosan-silver mixture reduced the adherence of streptococci to polystyrene surfaces.
In our study, mixed species mature biofilm was developed in premolars to simulate clinical cases. The effect of the medicaments on mature biofilm in premolars was evaluated using viable counts of CFU/ml to test their viability in biofilm within the dentinal tubules. The O3-oil/Ch-NPs combination worked the best, exerting a significant killing effect in the form of a 6-log reduction in viable cells (i.e., killing 99.9999% of the viable microbes in 2 days). It worked faster than the other treatments and the traditional medications that need at least 1 week to provide acceptable results. Calcium hydroxide was used as a positive control in our study. It was less effective than O3-oil, Ch-NPs, and their combination. This result was supported by the findings of Upadya et al. (2011) who reported that calcium hydroxide was ineffective for treatment of root canal infections forming either single of multispecies biofilms. Del Carpio-Perochena et al. (2017) mentioned that a combination of calcium hydroxide and Ch-NPs could potentiate the killing of bacteria forming the biofilm within 7–14 days. Moreover, Devaraj et al. (2016) stated that the triple antibiotic paste was more effective than calcium hydroxide against E. faecalis biofilm. Valacchi et al. (2005), who reported that ozonated olive oil had the strongest effect on mature biofilm. The oil’s potent antimicrobial activity is attributed to the stabilization of ozone as ozonide in addition to other products including peroxide, hydroperoxide, diperoxide, polyperoxide and aldehydes, which are generated by the interaction between ozone and olive oil (Pryor and Uppu, 1993). Di Giulio et al. (2013) reported that chitosan nanocomposite system exerted similar result on biofilm. Chitosan antibiofilm activity can be explained by several mechanisms, among which is its ability to penetrate and damage the biofilm due to its cationic properties (Carlson et al., 2008). A recent study reported that using light-activated curcumin as an antibiofilm killed the most E. faecalis cells at 200 and 400 micron depths compared to the control; however, this did not differ significantly from the triple antibiotic paste (Devaraj et al., 2016).
In view of the current in vitro study results, further in vivo investigations are recommended to determine the best concentration, and application time for each medicament, as well as the optimum ratio of the double combination, to obtain the best antimicrobial effect with the least adverse reactions.
Based on the current study, ozonated olive oil had the least cytotoxic effects, while chitosan nanoparticles showed better antimicrobial activity against the tested endodontic pathogens when used at its MIC and MBC/MFC compared to both silver nanoparticles and ozonated olive oil. Our tested double combination has the potential to prevent microbial biofilm formation and eradicate mature mixed-species biofilms. Incorporating the tested medicaments, either separately or in the novel double combination, into intracanal treatments, root canal sealers and irrigating solutions can prevent biofilm formation and eradicate resistant endodontic pathogens from the root canal, thereby increasing the success rate of endodontic treatment. These medicines might also be valuable if added to toothpastes and/or mouth rinses to combat harmful oral microbial biofilms.
Author Contributions
LA-M and NE-D conceived the experiments. LA-M, NE-D, and WG conducted the experiments. LA-M and NE-D analyzed the results. All authors wrote and reviewed the manuscript.
Conflict of Interest Statement
The authors declare that the research was conducted in the absence of any commercial or financial relationships that could be construed as a potential conflict of interest.
References
Agapov, V.-S., Smirnov, S.-N., Shulakov, V.-V., and Tsarev, V.-N. (2002). Ozone therapy in treatment of local sluggish suppurative inflammation of maxillofacial soft tissues. Stomatologija 80, 23–27.
Aliasghari, A., Khorasgani, M., Vaezifar, S., Rahimi, F., Younesi, H., Maryam, R., et al. (2016). Evaluation of antibacterial efficiency of chitosan and chitosan nanoparticles on cariogenic streptococci: an in vitro study. Iran. J. Microbiol. 8, 93–100.
Aranaz, I., Harris, R., and Heras, A. (2010). Chitosan amphiphilic derivates. Chemistry and applications. Curr. Org. Chem. 14, 308–330. doi: 10.2174/138527210790231919
Bachtiar, E.-W., Dewiyani, S., Surono, S.-M., and Bachtia, B.-M. (2016). Inhibition of Candida albicans biofilm development by unencapsulated Enterococcus faecalis cps2. J. Dent. Sci. 11, 323–330. doi: 10.1016/j.jds.2016.03.012
Berenji, F., Rajabi, O., Azish, M., and Minoochehr, N. (2014). Comparing the effect of ozonized olive oil with clotrimazole on three Candida species C. albiacans, C. glabrata, C. krusei. J. Microbiol. Res. 2, 009–013.
Borenfreund, E., and Puerner, J.-A. (1985). A simple quantitative procedure using monolayer cultures for cytotoxicity assays (HTD/NR90). J. Tissue Cult. Methods 9, 7–9. doi: 10.1007/BF01666038
Burd, A., Kwok, C. H., Hung, S. C., Chan, H. S., Gu, H., Lam, W. K., et al. (2007). A comparative study of the cytotoxicity of silver-based dressings in monolayer cell, tissue explant, and animal models. Wound Repair Regen. 15, 94–104. doi: 10.1111/j.1524-475X.2006.00190.x
Carlson, R.-P., Taffs, R., Davison, W.-M., and Stewart, P.-S. (2008). Anti-biofilm properties of chitosan-coated surfaces. J. Biomater. Sci. Polym. Ed. 19, 1035–1046. doi: 10.1163/156856208784909372
Casal, M.-M., Cause, M., Sol, F., Rodrız, F., and Casal, M. (2009). Investigation of Enterococcus faecalis antimicrobial resistance. Rev. Esp. Quimioter. 22, 117–119.
Clinical and Laboratory Standards Institute [CLSI] (2016). Performance Standards for Antimicrobial Susceptibility Testing; 26th Informational Supplement. CLSI Document M100-S26. Wayne, PA: Clinical and Laboratory Standards Institute.
Costa, E.-M., Silva, S., Madureira, A. R., Cardelle-Cobas, A., Tavaria, F. K., and Pintado, M. M. (2014). A comprehensive study into the impact of a chitosan mouthwash upon oral microorganism’s biofilm formation in vitro. Carbohydr. Polym. 101, 1081–1086. doi: 10.1016/j.carbpol.2013.09.041
Cravioto, A., Gross, R.-J., Scotland, S.-M., and Rowe, B. (1979). An adhesive factor found in strains of Escherichia coli belonging to the traditional infantile enteropathogenic serotypes. Curr. Microbiol. 3, 95–99. doi: 10.1007/BF02602439
Dash, M., Chiellini, F., Ottenbrite, R. M., and Chiellini, E. (2011). Chitosan – a versatile semisynthetic polymer in biomedical applications. Prog. Polym. Sci. 36, 981–1014. doi: 10.1016/j.progpolymsci.2011.02.001
Del Carpio-Perochena, A., Kishen, A., Felitti, R., Bhagirath, A. Y., Medapati, M. R., Lai, C., et al. (2017). Antibacterial properties of chitosan nanoparticles and propolis associated with calcium hydroxide against single- and multispecies biofilms: an in vitro and in situ study. J. Endod. 43, 1332–1336. doi: 10.1016/j.joen.2017.03.017
Devaraj, S., Jagannathan, N., and Neelakantan, P. (2016). Antibiofilm efficacy of photoactivated curcumin, triple and double antibiotic paste, 2% chlorhexidine and calcium hydroxide against Enterococcus faecalis in vitro. Sci. Rep. 6:24797. doi: 10.1038/srep24797
Di Giulio, M., Di Bartolomeo, S., Di Campli, E., Sancilio, S., Marsich, E., Travan, A., et al. (2013). The effect of a silver nanoparticle polysaccharide system on streptococcal and saliva-derived biofilms. Int. J. Mol. Sci. 14, 13615–13625. doi: 10.3390/ijms140713615
Díaz, M. F., Hernández, R., Martínez, G., Vidal, G., Gómez, M., Fernández, H., et al. (2006). Comparative study of ozonized olive oil and ozonized sunflower oil. J. Braz. Chem. Soc. 17, 403–407. doi: 10.1590/S0103-50532006000200026
Falsetta, M.-L., Klein, M. I., Punsiri, M., Colonne, P. M., Kathleen, S.-A. K., Chia-Hua, P., et al. (2014). Symbiotic relationship between Streptococcus mutans and Candida albicans synergizes virulence of plaque biofilms In vivo. Infect. Immun. 82, 1968–1981. doi: 10.1128/IAI.00087-14
Flemming, H.-C., and Wingender, J. (2010). The biofilm matrix. Nat. Rev. Microbiol. 8, 623–633. doi: 10.1038/nrmicro2415
Gahlaut, A., and Chhillar, A.-K. (2013). Evaluation of antibacterial potential of plant extracts using resazurin based microtiter dilution assay. Int. J. Pharm. Pharm. Sci. 5, 372–376.
Gomes-Filho, J., Silva, F. O., Watanabe, S., Cintra, L. T. A., Tendoro, K. V., Dalto, L. G., et al. (2010). Tissue reaction to silver nanoparticles dispersion as an alternative irrigating solution. J. Endod. 36, 1698–1702. doi: 10.1016/j.joen.2010.07.007
Guzman, M., Dille, J., and Godet, S. (2012). Synthesis and antibacterial activity of silver nanoparticles against gram-positive and gram-negative bacteria. Nanomedicine 8, 37–45. doi: 10.1016/j.nano.2011.05.007
Hernández-Sierra, J. F., Galicia-Cruz, O., Salinas-Acosta, A., Ruíz, F., Pierdant-Pérez, M., and Pozos-Guillé, A. J. (2011). In vitro cytotoxicity of silver nanoparticles on human periodontal fibroblasts. J. Clin. Pediatr. Dent. 36, 37–41. doi: 10.17796/jcpd.36.1.d677647166398886
Huycke, M.-M., Sahm, D.-F., and Gilmore, M.-S. (1998). Multiple-drug resistant enterococci: nature of the problem and an agenda for the future. Emerg. Infect. Dis. 4, 239–249. doi: 10.3201/eid0402.980211
Jhajharia, K., Parolia, A., Shetty, K.-V., and Mehta, L.-K. (2015). Biofilm in endodontics: a review. J. Int. Soc. Prev. Commun. Dent. 5, 1–12. doi: 10.4103/2231-0762.151956
Kaur, G., Balamurugan, P., Vasudevan, S., Jadav, S., and Princy, S. A. (2017). Antimicrobial and antibiofilm potential of acyclic amines and diamines against multi-drug resistant Staphylococcus aureus. Front. Microbiol. 8:1767. doi: 10.3389/fmicb.2017.01767
Kishen, A. (2010). Advanced therapeutic options for endodontic biofilms. Endod. Topics. 22, 99–123. doi: 10.1111/j.1601-1546.2012.00284.x
Krishnan, R., Arumugam, V., and Vasaviah, S.-K. (2015). The MIC and MBC of silver nanoparticles against Enterococcus faecalis - a facultative anaerobe. J. Nanomed. Nanotechnol. 6:3. doi: 10.4172/2157-7439.1000285
Li, X., Hoogenkamp, M.-A., Ling, J., and Crielaard, W. (2014). Diversity of Streptococcus mutans strains in bacterial interspecies interactions. J. Basic Microbiol. 54, 97–103. doi: 10.1002/jobm.201200457
Li, X., Wang, L., Fan, Y., Feng, Q., and Cui, F. (2012). Biocompatibility and toxicity of nanoparticles and nanotubes. J. Nanomater. 2012:548389. doi: 10.1155/2012/548389
Lotfi, M., Vosoughhosseini, S., Ranjkesh, B., Khani, S., Saghiri, M., and Zand, V. (2011). Antimicrobial efficacy of nanosilver, sodium hypochlorite and chlorhexidine gluconate against Enterococcus faecalis. J. Biotech. 10, 6799–6803.
Martinez-Sanchez, G., Al-Dalain, S. M., Menendez, S., Re, L., Giuliani, A., Candelario-Jalil, E., et al. (2005). Therapeutic efficacy of ozone in patients with diabetic foot. Eur. J. Pharmacol. 523, 151–161. doi: 10.1016/j.ejphar.2005.08.020
Nair, P.-N. (2006). On the causes of persistent apical periodontitis: a review. Int. Endod. J. 39, 249–281. doi: 10.1111/j.1365-2591.2006.01099.x
No, H. K., Park, N. Y., Lee, S. H., and Meyers, S. P. (2002). Antibacterial activity of chitosans and chitosan oligomers with different molecular weights. Int. J. Food Microbiol. 74, 65–72. doi: 10.1016/S0168-1605(01)00717-6
O’Toole, G. A. (2011). Microtiter dish biofilm formation assay. J. Vis. Exp. 30:2437. doi: 10.3791/2437
Peciuliene, V., Balciuniene, I., Eriksen, H.-M., and Haapasalo, M. (2000). Isolation of Enterococcus faecalis in previously root-filled canals in a Lithuanian population. J. Endod. 26, 593–595. doi: 10.1097/00004770-200010000-00004
Presterl, E., Zwick, R. H., Reichmann, S., Aichelburg, A., Winkler, S., Kremsner, P. G., et al. (2003). Frequency and virulence properties of diarrheagenic Escherichia coli in children with diarrhoea in Gabon. Am. J. Trop. Med. Hyg. 69, 406–410.
Pryor, W.-A., and Uppu, R.-M. (1993). A kinetic model for the competitive reactions of ozone with amino acid residues in proteins in reverse micelles. J. Biol. Chem. 268, 3120–3126.
Ramzy, M. I., Gonna, H. E., Mostafa, M. I., and Zaki, B. M. (2005). Management of aggressive periodontitis using ozonized water. Egypt. Med. J. NRC 6, 229–245.
Ratyakshi, N., and Chauhan, R.-P. (2009). Colloidal synthesis of silver nano particles. Asian J. Chem. 21, 113–116.
Ray, P. C., Yu, H., and Fu, P. P. (2009). Toxicity and environmental risks of nanomaterials: challenges and future needs. J. Environ. Sci. Health C Environ. Carcinog. Ecotoxicol. Rev. 27, 1–35. doi: 10.1080/10590500802708267
Römling, U., and Balsalobre, C. (2012). Biofilm infections, their resilience to therapy and innovative treatment strategies. J. Intern. Med. 272, 541–561. doi: 10.1111/joim.12004
Sailaja, A.-K., Amareshwar, P., and Chakravarty, P. (2011). Different techniques used for the preparation of nanoparticles using natural polymers and their application. Int. J. Pharm. Pharm. Sci. 3, 45–50.
Samuel, U., and Guggenbichler, J. P. (2004). Prevention of catheter-related infections: the potential of a new nano-silver impregnated catheter. Int. J. Antimicrob. Agents 1, 75–78. doi: 10.1016/j.ijantimicag.2003.12.004
Shen, Y., Stojicic, S., and Haapasalo, M. (2011). Antimicrobial efficacy of chlorhexidine against bacteria in biofilms at different stages of development. J. Endod. 37, 657–661. doi: 10.1016/j.joen.2011.02.007
Siqueira, J.-F., Rocas, I. N., and Ricucci, D. (2010). Biofilm in endodontic infection. Endod. Top. 22, 33–49. doi: 10.1111/j.1601-1546.2012.00279.x
Soleimani, N., Mobarez, A. M., Olia, M. S., and Atyabi, F. (2015). Synthesis, characterization and effect of the antibacterial activity of chitosan nanoparticles on vancomycin-resistant Enterococcus and other gram negative or gram positive bacteria. Int. J. Pure Appl. Sci. Technol. 2015, 14–23.
Sung, J. H., Ji, J. H., Yoon, J. U., Kim, D. S., Song, M. Y., Jeong, J., et al. (2008). Lung function changes in Sprague-Dawley rats after Prolonged inhalation exposure to silver nanoparticles. Inhal. Toxicol. 20, 567–574. doi: 10.1080/08958370701874671
Tin, S., Sakharkar, K. R., Lim, C. S., and Sakharkar, M. K. (2009). Activity of Chitosans in combination with antibiotics in Pseudomonas aeruginosa. Int. J. Biol. Sci. 2009, 153–160. doi: 10.7150/ijbs.5.153
Tirali, R.-E., Bodur, H., Sipahi, B., and Sungurtekin, E. (2017). Evaluation of the antimicrobial activities of chlorhexidine gluconate, sodium hypochlorite and octenidine hydrochloride in vitro. Aust. Endod. J. 39, 15–18. doi: 10.1111/j.1747-4477.2010.00266.x
Upadya, M., Shrestha, A., and Kishen, A. (2011). Role of efflux pump inhibitors on the antibiofilm efficacy of calcium hydroxide, chitosan nanoparticles, and light-activated disinfection. J. Endod. 37, 1422–1426. doi: 10.1016/j.joen.2011.06.017
Valacchi, G., Fortino, V., and Bocci, V. (2005). The dual action of ozone on the skin. Br. J. Dermatol. 2005, 1096–1100. doi: 10.1111/j.1365-2133.2005.06939.x
Williams, D. F. (2008). On the mechanisms of biocompatibility. Biomaterials29, 2941–2953. doi: 10.1007/s10544-015-9929-x
Xiao, J., Klein, M. I., Falsetta, M. L., Lu, B., Delahunty, C. M., Yates, J. R., et al. (2012). The exopolysaccharide matrix modulates the interaction between 3D architecture and virulence of a mixed-species oral biofilm. PLoS Pathog. 2012:e1002623. doi: 10.1371/journal.ppat.1002623
Keywords: anti-biofilm, root canal, ozonated olive oil, chitosan nanoparticles, endodontic pathogens
Citation: Elshinawy MI, Al-Madboly LA, Ghoneim WM and El-Deeb NM (2018) Synergistic Effect of Newly Introduced Root Canal Medicaments; Ozonated Olive Oil and Chitosan Nanoparticles, Against Persistent Endodontic Pathogens. Front. Microbiol. 9:1371. doi: 10.3389/fmicb.2018.01371
Received: 19 March 2018; Accepted: 06 June 2018;
Published: 03 July 2018.
Edited by:
Farhat Afrin, Taibah University, Saudi ArabiaReviewed by:
Jozsef Dudas, Innsbruck Medical University, AustriaDong Seok Lee, Inje University, South Korea
Fabian Cieplik, University of Regensburg, Germany
Copyright © 2018 Elshinawy, Al-Madboly, Ghoneim and El-Deeb. This is an open-access article distributed under the terms of the Creative Commons Attribution License (CC BY). The use, distribution or reproduction in other forums is permitted, provided the original author(s) and the copyright owner(s) are credited and that the original publication in this journal is cited, in accordance with accepted academic practice. No use, distribution or reproduction is permitted which does not comply with these terms.
*Correspondence: Lamiaa A. Al-Madboly, bGFtaWEueW91c3NpZkBwaGFybS50YW50YS5lZHUuZWc=
†These authors have contributed equally to this work.