- 1Key Laboratory of Soil Environment and Pollution Remediation, Institute of Soil Science, Chinese Academy of Sciences, Nanjing, China
- 2College of Environment and Ecology, Jiangsu Open University, Nanjing, China
- 3School of Engineering, Newcastle University, Newcastle upon Tyne, United Kingdom
Fifteen antibiotic resistance genes (ARGs) and intI1, a gene involved in horizontal gene transfer (HGT) of ARGs, were quantified in three different soil samples from a 22 year old field experiment that had received inorganic fertilizer (NPK), organic manure (OM; a mixture of wheat straw, soybean oil cake and cotton cake), and control fields that had received no fertilizer and manure (CK). Tet(L) was the most abundant ARG in OM, which also contained considerable levels of intI1. Molecular analysis of yearly collected archived soils over the past 22 years showed that tet(L) and intI1 were higher in OM soils than in NPK soils. The relative abundance of tet(L) was essentially constant during these years, while the level of intI1 in OM soils decreased over time. The main genotype of tet(L) was the same in archived and in fresh soil, OM, and irrigation water. Phylogenetic analysis of the 16S rRNA genes of tetracycline-resistant bacteria (TRB) isolates indicated that the Firmucutes carrying tet(L) in OM were similar to those in the OM soil, suggesting that OM transferred TRB into the OM soils where they survived. Almost all of the TRB isolated from OM carried tet(L) and belonged to the Firmicutes. Survival of bacteria from the organic manure that carried tet(L) may be the cause of the increased level of tet(L) in OM soil.
Introduction
Antibiotic resistance genes (ARGs) in the environment are posing a global threat to public health (Pruden et al., 2013). Antibiotic misuse and over-prescription have been regarded as driving forces promoting the selection and dissemination of resistance (D'Costa et al., 2007). Horizontal gene transfer (HGT) of mobile genetic elements harboring ARGs substantially increases the epidemiological risks associated with antibiotic resistance, because these elements can be readily transferred between bacteria on both an inter- and intra-species level or bi-directionally from non-pathogenic to pathogenic bacteria (Forsberg et al., 2012; Cytryn, 2013).
Previous studies have focused on the effect of animal manure on antibiotic resistance in agricultural soils, showing that use of animal manure as fertilizer increases resistance of cultivable soil microorganism to tetracycline and sulfonamide antibiotics (Sengeløv et al., 2003; Byrne-Bailey et al., 2009). The occurrence, abundance, and transferability of ARGs in soil especially increased
after field application of manure from antibiotic-treated animals (Jechalke et al., 2013; Kyselková et al., 2013; Zhu et al., 2013; Peng et al., 2015, 2017). In addition, it has been reported that manure from cattle that had not been treated with antibiotics can also lead to increased populations of resident soil bacteria harboring β-lactam resistance genes (Udikovic-Kolic et al., 2014).
Application of organic manure (OM) from plants rather than animals usually increases soil microbial biomass (Chu et al., 2007) and crop yield (Chen et al., 2010), and ameliorates soil properties, both abiotically, and biotically (Feng et al., 2015). OM fertilization has a large influence on bacterial community composition, larger than fertilization with chemical fertilizers (Feng et al., 2015). A previous study has reported that the different effects of pig manure with that of chemical fertilizers and straw on the soil resistome was accompanied by a change in the bacterial community (Liu et al., 2017). OM from plants is quite different from straw and animal manure, both chemically and microbiologically. In addition, OM is frequently composted and fungi and bacteria are known to play important role during the organic waste composting process (Neher et al., 2013; Gu et al., 2017). The present study was directed toward investigating the impact of long-term application of OM on the soil resistome and the main factor directing the impact.
Archived soil samples are a very valuable resource for microbial ecological studies (Dolfing et al., 2004; Cary and Fierer, 2014; Dolfing and Feng, 2015). Knapp et al. (2010) have assayed DNA extracted from dried archived soils, and found that the presence of ARGs in agricultural soils has substantially increased since 1940, concomitant with the increase in industrial antibiotic production. Graham et al. (2016) analyzed soils archived since 1923 and found that “total” β-lactam ARG levels were significantly higher in manured soils than in inorganic fertilized soils after 1940; in addition, these authors showed that when non-therapeutic antibiotic use was banned in Denmark, the levels of the extended-spectrum β-lactamase gene blaCTX−M declined in manured soils. Dolfing et al. (2004) showed that air-dried soils stored for extended periods of time is feasible for application of molecular techniques, generating valuable information on bacterial community changes due to agricultural practice, even though drying and storage can affect microbial biomass and activity (De Nobili et al., 2006). Tzeneva et al. (2009) also found that soil drying and storage affected the detectable community structure in their samples, but did not materially impair their capacity to identify the effect of soil parameters studied in long-term grassland experiments. Given the high scientific value of archived soil samples, it is relevant to evaluate the effect of long-term manure application on the abundance of ARGs with archived samples.
Based on the above considerations, ARG abundance in fresh soil samples from a 22 years field fertilizer experiment, including non-animal waste OM fertilization and chemical fertilizations with NPK (nitrogen-phosphorus-potassium) as well as a control without fertilization, were analyzed by qPCR. Furthermore, archived soils yearly collected from 1989 onwards were analyzed to assess the change of the main ARG over the past 20 years. To compare the historical effect of non-animal organic manure vs. inorganic fertilizer, phylogenetic analysis of the main ARG was also conducted to obtain information on the potential source of this gene. The resistant bacteria carried with the main ARG was isolated and identified to further confirm the source of main ARG. Present study comprehensively investigated the influence of OM from plant on soil ARGs and the results also can help us better understand the mechanism of the influence on soil resistome caused by animal manure.
Materials and Methods
Description of the Long-Term Experiment
The long-term field fertilizer experiment was conducted at Fengqiu Agro-ecological Experimental Station, Chinese Academy of Sciences, Fengqiu County, Henan Province, China (35°00′N, 114°24′E). The soil was a sandy loam (alluvial-aquic) soil with alkaline characteristics. Historical soil samples were acquired from Feng et al. (2015), soil physicochemical properties were described in detail in that article. Winter wheat (Triticum aestivum L.) and summer maize (Zea mays L.) were rotated planted, and agronomic practice was not substantially changed for more than 20 years. At the beginning of the experiment in 1989, the soil contained 5.83 g kg−1 of organic matter, 0.45 g total N kg−1, 0.50 g total P kg−1, and 18.6 g total K kg−1 with a pH of 8.65. Three treatments with four replicates in completely randomized blocks were established. were selected for analysis in this study: (1) OM: applied with organic manure (P and K were supplemented as chemical fertilizers for the same amount of nutrients as other treatments); (2) NPK: balanced fertilizations with NPK; (3) Control: no fertilization. The composition of OM was constant during these years; OM consisted of wheat straw, soybean oil cake and cotton cake at a ratio of 100:40:45, as described in detail by Meng et al. (2005) and Gong et al. (2009).
Sample Collection and Determination
Fresh soil samples were collected on 18 September 2011 (at maize harvesting stage) from 16 points at a depth of 0–15 cm from each field as described in Feng et al. (2015). Sub-samples were air dried and kept at room temperature. Fresh samples were kept at −80°C until further use. A series of soil samples from 1989 to 2009 under NPK, OM, and control fertilization regimes was collected as described in Feng et al. (2015).
Soil and Organic Manure DNA Extraction
DNA was extracted from soil using the FastDNA® SPIN Kit for soil (MP Biomedicals) according to the manufacturer's protocol. DNA of OM was extracted using the FastDNA® SPIN Kit for feces samples. The extracted DNA was dissolved in 50-μL of TES buffer, quantified by a microspectrophotometry (NanoDrop ND-1000, NanoDrop Technologies, Wilmington, DE), and stored at −20°C until use. The DNA samples were diluted to 1:10 and 1:50 to avoid inhibitors to the PCR reaction.
Quantification of ARGs, 16S-rRNA, and Class I Integrons
qPCR analysis was used to determine the abundance of ARGs and class I integrons (intI1) by C1000™ Thermal Cycler equipped with the CFX96 Touch™ Real-Time PCR Detection System (Bio-Rad, USA). All qPCR assays were performed in triplicate using the SYBR Premix Ex Taq™ Kit (TaKaRa) with the primer sets shown in Table S1. A 10-fold dilution series of plasmids carrying each targeted ARG was made to generate a six-point calibration curve for qPCR. The abundance of ARGs per microliter of plasmid solution was calculated according to Zhang et al. (2009). All samples were assayed in duplicate, as were the standards, and positive and negative controls. Bacterial 16S rRNA genes were also quantified as described by Biddle et al. (2008), so that ARGs or intI1 abundance could be normalized to the total bacterial 16S rRNA gene counts.
Sequencing and Phylogenetic Analysis of tet(L)
Archived OM soil samples of 1991, 1993, 1997, 1999, 2001, 2003, 2005, 2007, 2009, and 2011, as well as fresh NPK soil and OM soil from 2011, fresh OM, irrigation water were selected for phylogenetic analysis of tet(L). tet(L) gene sequencing was performed by Tianhao Genomics Institute (Shanghai, China) using the SMRT cell PACBIO RS II platform with primers tet(L)-F (5′-GTTGCGCGCTATATTCCAAA-3′) and tet(L)-R (5′-TTAAGCAAACTCATTCCAGC-3′) (You et al., 2012). The number of clean reads of every sample was more than 1900. Sequences were clustered into an genotype at a level of 99% sequence similarity, and one representative sequence was selected for subsequent similarity analysis online using BLASTn and for phylogenetic tree construction. The neighbor joining trees were constructed using MEGA version 5.1.
Isolation and Identification of Tetracycline-Resistant Bacteria Carrying tet(L)
Tetracycline-resistant bacteria (TRB) and culturable bacteria were counted in fresh OM, fresh soils from the NPK and OM treated fields, and in the archived OM soil from 1989 and 2011. Soil samples were suspended in saline and plated onto R2A agar (Popowska et al., 2012) medium supplemented with and without antibiotics. Each of the antibiotic medium plates contained 8 μg/mL of tetracycline, based on Sengeløv et al. (2003). The plates were incubated at room temperature for 3–4 days. Twenty strains of TRB were randomly isolated from each soil sample. The purified strains were stored at 4°C on antibiotic agar plates.
The colony PCR method was used for the amplification of 16S rRNA and tet(L) genes. Amplification of 16S rRNA genes was performed with primers 27F (5′-AGAGTTTGATCCTGGCTCAG-3′) and 1492R (5′-GGTTACCTTGTTACGACTT-3′) under the conditions published by Popowska et al. (2012). Subsequently the isolated TRB were analyzed for presence of tet(L). The 16S rRNA gene PCR products of those bacteria carrying tet(L) were selected and ligated into a pEASY-T3 vector (TransGen Biotech, Beijing) and then cloned into a Trans1-T1 phage resistant chemically competent cell (TransGen Biotech, Beijing). Positive clones were sequenced, and identification to the species level was performed. Bacteria sharing ≥99% identity with 16S rRNA gene sequences in EzBioCloud and Genbank were identified to the species level; those with 95–98.9% identity were identified to the genus level (Popowska et al., 2012).
Heavy Metal Analysis
Eight typical heavy metals, viz. Hg, As, Cd, Cr, Pb, Cu, Zn, and Ni, were quantified using the method developed by Lu (2000). These metals are found frequently in the environmena as a result of human activities or are commonly used as additives in livestock feed (Chen et al., 2009; Ji et al., 2012). Samples were digested in teflon crucibles at 170–210. Hg and As levels in soils was quantified by an atomic fluorescence spectrophotometer AFS-8120 as described by GB/T 22105-2008. The other six heavy metals were quantified using high performance liquid chromatography inductively coupled plasma-mass spectroscopy (HPLC-ICP-MS).
Statistical Analysis
Statistical analysis was carried out using the software package SPSS 17.0 for Windows (SPSS Inc., USA). Figures were plotted with OriginPro 8.0 (OriginLab Corporation, USA). Mean separation was assessed by ANOVA. Duncan's multiple range test was used to evaluate the significance of differences between samples. P < 0.05 was considered statistically significant. Correlations between the relative abundance of tet(L) and intI1 or heavy metal content (Pearson's correlation coefficient) were calculated with SPSS 17.0.
Results
Abundance of ARGs in OM, Irrigation Water, and Fresh Soil Samples
The distribution of 15 ARGs and two intI genes, normalized to 16S rRNA gene copies, in OM and irrigation water was evaluated by real-time PCR. ARGs are abundant in OM. The most abundant ARG was tet(L) (Table 1), which accounted for 67.49% of the total relative abundance of the 15 ARGs, followed by erm(C), sul1, and sul2. Irrigation water also contained considerable amounts of ARGs, of which the most abundant ARGs are erm(C), sul2, sul1, and tet(G) (Table 1). In the fresh soil samples collected in 2011, the relative abundances of tet(L), tet(M), sul1, and erm(C) were significantly increased after continuous application of OM for 22 years; tet(O) and sul2 had also increased to a detectable level (Figure 1). Among these ARGs, tet(L) had increased the most: the relative abundance of tet(L) in OM soil was 120 times more than that in NPK soil, followed by tet(M), which was increased by 74.5 times. In our previous studies, we found that tet(L) also had increased considerably in soil after long-term fertilization with animal manure (Peng et al., 2015, 2017); therefore this gene was selected for comprehensive analysis in this study.
Change of the tet(L) Abundance After Soils Were Air Dried
We compared the relative abundance of tet(L) in fresh soils and air-dried soils, and found that tet(L) was increased in NPK and CK soils after the soils was air-dried, but not significantly changed in OM soil (Figure 2). Simultaneously, the abundance of the 16S rRNA gene was significantly reduced in NPK and CK after these soils were air-dried, while its abundance in the soil sampled from the fields fertilized with OM was not measurably changed (Figure 2). The abundance of tet(L) was not influenced by air-drying in OM soil.
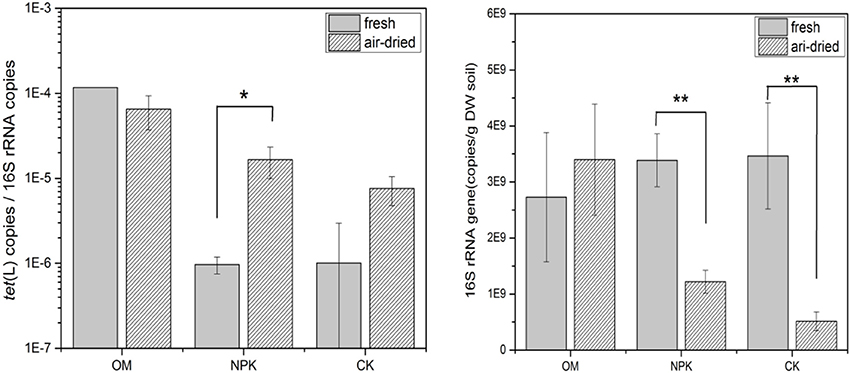
Figure 2. The relative abundance of tet(L) and the absolute abundance of the 16S rRNA gene in fresh or air-dried soil fertilized with OM or NPK and CK. * and ** indicate statistically significant differences at P < 0.05 and P < 0.01, respectively.
Abundance of tet(L) and intI1, and Concentrations of Heavy Metals in Yearly Archived Soil
The relative abundance of tet(L) in CK, NPK, and OM treated soils fluctuated over time (Figure 3), but there was no distinct difference in relative abundance of the tet(L) between CK and NPK soils. tet(L) was markedly higher in OM soils than in NPK and CK soils (Figure 1), and this difference was consistent over 20 years (Figure 3). ANOVA with repeated measures revealed that these three treatments had no significant effect on the absolute abundance of tet(L) in the 22-years time series measurements.
The abundance of intI1 was at a very low level in those soils that were not exposed to OM; however, the relative abundance of intI1 was higher in OM soils (Figure 4). Both the absolute and the relative intI1 abundance decreased over time (Figure 4). A repeated measures one-way ANOVA revealed that all three treatments exerted no significant influence on the abundance of intI1 among the 22 times measurement. IntI2 was below the detection limit. intI1 was positively correlated with tet(L) (r = 0.694, P < 0.01, Figure S1).
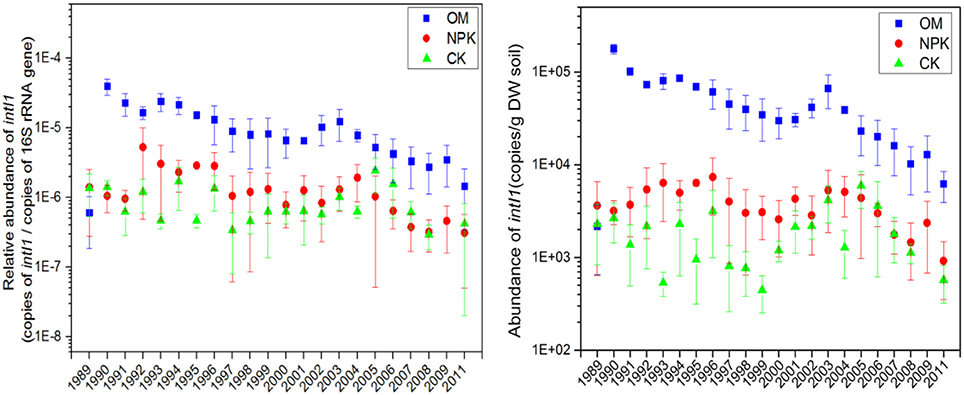
Figure 4. The variation trend in the abundance of the intI1 gene in different fertilizer treated archived soils.
The heavy metal concentrations (Cu, As, Zn, Cr, Ni, Pb, As, and Hg) in archived soils of 1989, 1996, 2002, 2008, and 2011 did not significantly change over time (Table S3). Table S4 gives a summary of the correlation analyses among the detected heavy metals and tet(L), intI1 in the soils. Cu and Pb were positively correlated with tet(L).
The Main tet(L) Genotype in OM and OM Soil
44896 tet(L) sequences were obtained and grouped into 230 genotypes under 99% identity. There were seven genotypes (genotype 1–genotype 7) containing more than two sequences, comprising 99.5% of the total library. The sequences of the seven genotypes have been deposited in GenBank under the accession number of MG821379–MG821385. Genotype1 was the main tet(L) genotype in all of the samples conducted for phylogenetic analysis, more than 97% sequences of every sample were grouped into genotype 1 (Figure S2). BLAST searches of the GenBank database confirmed that genotype 1 matched known tet(L) genes (Figure 5), with sequence identities of 100%. This most common tet(L) type was identical to the sequences of tet(L) found in plasmids and chromosomes of different Gram-positive bacteria.
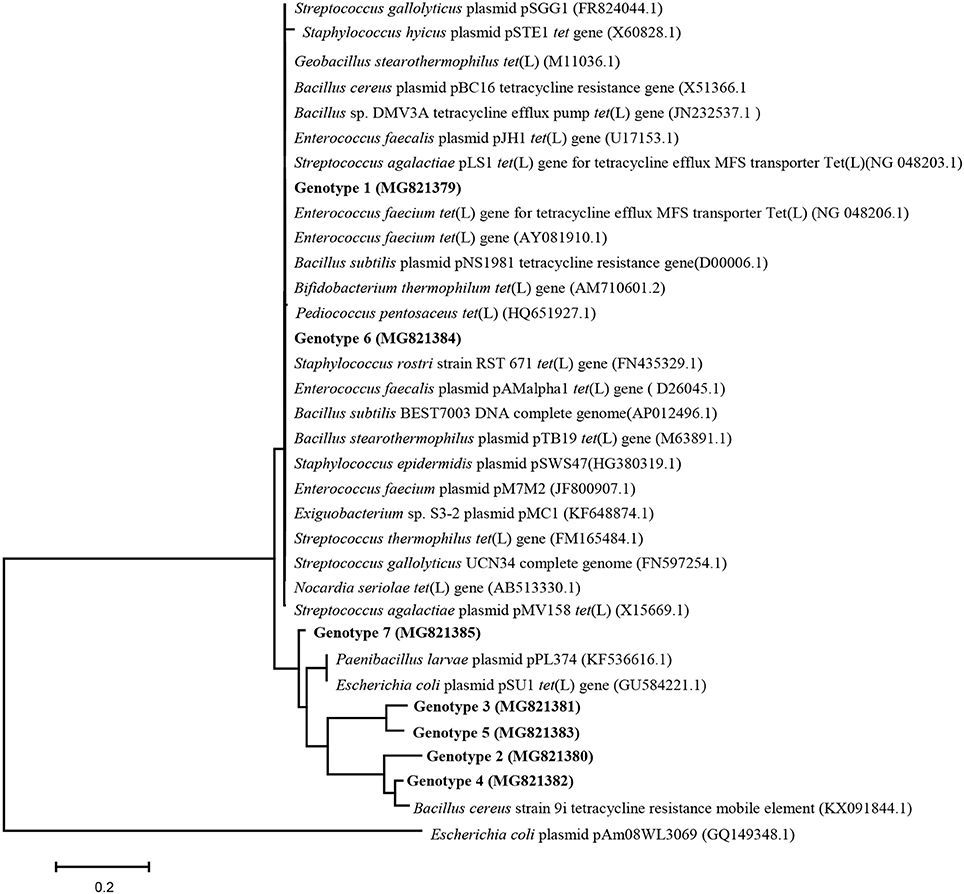
Figure 5. Neighbor-joining phylogenetic analysis of genotypes genotype 1~7 of tet(L) displayed in bold font. The sequences in GenBank that were most similar to genotype1–genotype 7 were retrieved for phylogenetic tree construction. The tree was constructed using MEGA version 5.1, and bootstrap analysis with 500 replicates was used to evaluate the significance of the nodes.
Isolation and Identification of TRB Carrying tet(L)
In order to find which species of ARB were transmitted into soil by OM and caused the difference of tet(L) abundance between OM soils and NPK soils, approximately 280 TRB were isolated from fresh OM, fresh soils of OM and NPK, and from the archived soil samples collected in 1989 and 2011 from OM treatment (Table 2). Fertilization with OM increased the number of TRB carrying tet(L). There were more diverse types of TRB carrying tet(L) in fresh soils fertilized with OM than in fresh soils fertilized with NPK (Table 2). Thirtythree of the 60 TRB (55%) isolated from the archived soils of 1989 carried tet(L); in the archived soils of 2011, the percentage of TRB carrying tet(L) increased to 91.7%. Almost all the TRB isolated from OM were carrying tet(L); they were mainly Bacillus sp., Lysinibacillus sp., Solibacillus sp., Rummeliibacillus sp., and Sporosarcina sp., all belonging to the order of the Bacillales.
Discussion
OM and Irrigation Water Contained Considerable Amounts of ARGs
Feng et al. (2015) has argued and illustrated that OM fertilization is more sustainable than NPK, and can boost nutrient levels in arable soils to adequate levels. The nutrient-levels (including organic C, available N, available P, and available K) at the Fengqiu fieldsite indeed all responded positively to OM fertilization (Table S2). The composition of OM is different from that of animal manure; it consisted of wheat straw, soybean oil cake, and cotton cake; these materials were machine ground into 3–5 mm sized particles, mixed thoroughly with a limited amount of water and composted for 2 months (Meng et al., 2005). Therefore, OM is intrinsically unlikely to contain bioactive compounds like antibiotics or heavy metals that are common in animal manure and may select for antibiotic resistance (Berg et al., 2010; Wu et al., 2010). We recently quantified these ARGs in pig manure and cow manure (Peng et al., 2017), and found that ARGs in OM were less diverse and less abundant, and that most of them are common in the environment. The ARGs in OM would be randomly carried by microbes that inhabit the antibiotic free ecosystem. There are several possibilities why this was not the case, e.g., (i) OM was polluted by antibiotics like tetracycline or related compounds, (ii) The composting process may enrich some microbes that produce the antibiotics. e.g., Actinobacteria and Fungi. etc., (iii) OM was colonized by bacteria (Firmicutes) carrying ARGs during the storage of the ingredients or proliferating during the composting procedure, resulting in the abundance of ARGs in OM (Table 1).
Irrigation water also contained considerable amounts of ARGs, of which the most abundant ARGs are erm(C), sul2, sul1, and tet(G) (Table 1). In this long term-fertilizer experiment, the irrigation water was ground water which was always pumped from a well; yet this irrigation water contained considerable amounts of ARGs. ARGs and ARB have been detected extensively in natural environments, such as surface rivers (Luo et al., 2011), groundwater (Koike et al., 2007; Böckelmann et al., 2009) and sediments (Devarajan et al., 2015). Even in the product water of some drinking water treatment plants (DWTPs), many ARGs have been detected (Xi et al., 2009), with sul1, sul2, tet(C), and tet(G) the four most abundant ARGs in DWTPs (Guo et al., 2014). The relative abundance of these ARGs was more than one order of magnitude higher than in the ground water of the present study. Considering that a much higher tet(L) gene abundance was detectable in the OM, it is possible that the irrigation water in this study was not the primary source for tet(L).
Application of OM Markedly Increased the Abundance of tet(L) and intI1
Tet(L) was markedly higher in OM soils than in NPK and CK soils, and this difference was consistent over 20 years (Figure 3). We speculate that there are two reasons for the difference observed. First, OM is likely the source of this elevated level of tet(L), which is the most abundant ARG in OM (Table 1). OM might have been colonized by ARB during storage or during the composting process, and these bacteria survived in soil. These ARB may be the key species which could promote composting of OM. However, they were not proliferated or increased continuously along with repeating application of OM, so that the abundance of tet(L) was in general essentially constant or even gradually lower (Figure 3). Second, tet(L) is also present in the CK and NPK soils, so there is indeed the possibility that tet(L) was already in the soil and is proliferating because the organisms carrying it are favored by OM. Previous studies found that fierce microbe–microbe competition should select for increased antibiotic production and resistance (Fierer et al., 2012), and that soil carbon is positively related to ARG abundance (Wepking et al., 2017), therefore, the increase in carbon in soil fertilized with OM may lead to increased microbial competition and a subsequent increase in tet(L). The difference of ARG abundance between NPK and OM soils was also observed in the data of the fresh soils (Figure 1), but from the time series we now know that this is a long standing difference. In the study of Graham et al. (2016), the level of ARGs dropped dramatically in manure fertilized soils when the source of ARGs was removed. Whether tet(L) would disappear when we would stop adding OM, or when we would switch to sterilized OM or a virtually tet(L)-free OM is still unknown.
In yearly archived samples, the abundance of tet(L) in NPK treated soils was essentially the same as in the CK soils, just as it was in fresh soils (Figures 1, 3). Graham et al. (2016) also reported that the level of ARGs in inorganic fertilized soils was essentially constant over time, but the time series in that study were sampled less frequently. Furthermore, a weakness of that study was that there were no unfertilized controls.
IntI1 is commonly linked to genes conferring resistance to antibiotics (Gillings et al., 2015) and may play a role in the dissemination of ARGs (Heuer and Smalla, 2007; Gillings et al., 2008; Wang et al., 2014). In this study, intI1 was significantly positively correlated with tet(L) (r = 0.694), this may imply that OM application has increased the intrinsic potential of OM fertilized soil for HGT. When evaluating the time series, we found that the difference of intI1 between the three soils is decreasing (Figure 4); maybe this suggests that the amount of the bacteria carrying intI1 is decreasing in OM or intI1 was lost by the TRB in soil. Furthermore, the time series change of intI1 in OM soil was somewhat similar to which in NPK soil (Figure 4), intI1 is frequently detected in diverse environments, even in drinking water also exist abundant intI1 (Ma et al., 2017), the improvement of the irrigation water quality may be one of the factors.
Heavy metals can exert a continuous selection pressure for antibiotic resistance in agricultural soils adjacent to feedlots (Ji et al., 2012). In this study, Cu and Pb were found weakly positively correlated with tet(L) (r = 0.309 and 0.452, respectively), this result was in agreement with results of a previous study which reported that soil ARGs might associate with Cu and Zn levels in soil (Lin et al., 2016). Cu has been shown to co-select for resistance to clinically important antibiotics in microbial soil communities under field conditions (Berg et al., 2010). Knapp et al. (2011) found that many ARGs positively correlated with soil Cu levels in archived Scottish soils. Metals can coselect for ARGs because the resistance mechanisms employed by microorganisms often leads to resistance to both and the genes are located on the same mobile elements (Knapp et al., 2011; Devarajan et al., 2015).
OM Soil Bacteria Shared Same Common Genotype of tet(L) With NPK Soil and OM
Tet(L) is generally located on small transmissible plasmids, which on occasion become integrated into the chromosome of staphylococci or the chromosome of Bacillus subtilis or into larger staphylococcal plasmids (Chopra and Roberts, 2001). Results of tet(L) phylogenetic analysis showed that the main genotype of tet(L) was same in the archived soil samples treated with OM, fresh NPK soil and OM soil, fresh OM, and irrigration water. This indicated that this main genotype of tet(L) is common in the environment, and that it was not affected by air-drying and OM application. Although long-term application of OM increased the abundance of tet(L), sequences of tet(L) carried by bacteria in OM soil was conservative and has not been essentially changed. The low diversity of the tet(L) genotypes in OM and OM soil probably results from a narrow host range of the genes carried by bacterial genera, or from the refining selection of the gene function. The high microbial density and diversity, high densities of various mobile elements, and high level of tetracycline were thought to contributed to nucleotide alterations on tet gene fragments (Zhang and Zhang, 2011); all three or the latter two factors may not exist in OM soil, resulting in the low diversity in tet(L) sequences observed.
Survival of the Bacteria Carrying tet(L) Caused the Increased Level of tet(L) in OM Soil
Microorganisms from animal manure have been shown to contribute considerably to the accumulation of TRB and tetracycline resistance genes in soil (Peng et al., 2016). In this study, the bacteria carrying tet(L) in fresh OM were identified as Bacillus sp., Lysinibacillus sp., Solibacillus sp., Rummeliibacillus sp., and Sporosarcina sp. They all belong to the order Bacillales, and were also present in OM soil except Sporosarcina sp. (Table 2). Therefore we speculate that many Bacillales from OM were co-transferred with the OM to the soil, where their presence caused an increase in the tet(L) level.
Furthermore, almost all the identified TRB isolates belonged to the Firmicutes although a few members of the Actinobacteria (two Streptomyces sp.) and Proteobacteria (one Achromobacter insolitus) were also detected. Feng et al. (2015) have shown that OM fertilization significantly increased the relative abundance of Firmicutes: from 5% in the CK up to 14%. Firmicutes was found positively correlated with ARGs (Chen et al., 2016; Peng et al., 2017), and was also previously demonstrated to contribute to the elevated abundance and enrichment of ARGs (Zhang et al., 2016). It is possible that the transfer of Firmicutes (Bacillales) carrying tet(L) from OM to soil contributed considerably to the abundance of tet(L) genes in OM treated soil.
Although a wide variety of genes capable of conferring antibiotic resistance has been identified in soil microorganisms, it has been found that few of these genes are shared by human pathogens; furthermore, there is limited transfer of ARGs within the soil community and from the soil to other bacteria (Forsberg et al., 2012; Sommer, 2014). In addition, mobile ARGs have been found to be significantly enriched in Proteobacteria (Hu et al., 2016). Although we cannot neglect that cultivation-based approaches detect only a small fraction (usually about 1% of soil bacteria) of the total bacterial community (Amann et al., 1995), this result suggests that survival of the bacteria carrying tet(L) is the main reason for the increased level of tet(L) in OM soil, although we have found no evidence that the gene is transferred to other organisms, more in depth studies are warranted.
Molecular Analysis of Archived Soils Can Provide Meaningful Information on the Relative Abundance of ARGs in Soils at the Time of Sampling
Dried soil samples from many sources have been stored in archives world-wide over the years (Dolfing and Feng, 2015). Previous data had shown that DNA can be effectively obtained from dried archive soils (Dolfing et al., 2004; Knapp et al., 2010, 2011; Graham et al., 2016), but the effect of drying on ARG recovery was still not studied. The abundance of the 16S rRNA gene was not obviously changed in OM soils after these soils were air-dried, while they were significantly reduced in NPK and CK soils. In the archived soil, the abundance of 16S rRNA gene fluctuated more gently in OM than in NPK and CK (Figure S3). These results were in accordance with that of Clark and Hirsch (2008); these authors found that DNA yields declined less and may be preserved by desiccation in the soil treated with farmyard manure than in the soil fertilized with NPK, which suggests that DNA stability and/or recovery during extraction were protected by the elevated level of soil organic matter.
In the present study, only in OM soil, the influence of air-drying on the relative abundance of tet(L) was not significant. This result may be caused by the different bacterial community structure in soil samples of OM soils compared to the communities in NPK and CK soils, it has reported that OM fertilization resulted in significantly more Firmicutes, significantly increased Bacilli abundance (p < 0.05) and changes in Bacilli composition (Feng et al., 2015). Furthermore, Tzeneva et al. (2009) found that soil drying and storage affected the bacteria community structure, probably some types of bacteria carried with tet(L) were tolerant to drought and survived, or persisted as spores (if they are predominantly Bacilli), results in this study supported this speculation: 63.6% of the isolated TRB carrying tet(L) belonged to Bacilli in fresh OM soil from 2011, while it's 98.18% in the archived OM soil from 2011, and 100% in the archived OM soil from 1989 (Table 2). Although air-drying well affected the recovery of the 16S rRNA gene in NPK and CK soil samples, the relative abundance of tet(L) was not reduced among all three fertilization treatments. Therefore, analyzing the abundance of tet(L) in archived soils by normalizing to 16S rRNA genes can be reliable: while absolute numbers are elusive, relative numbers, and consequently comparisons between different time series appear sound.
Conclusion
In this study, we assessed the abundance of 15 ARGs and intI1 in fresh OM soil and NPK for 22 years, and found that the abundance of tet(L) was noticeably increased by application of OM. We then used yearly collected archived soils to assess time series change of the tet(L) and intI1 in OM, NPK, and CK soils over the past 22 years. Results indicate that tet(L) and intI1 were markedly higher in OM soils than in NPK and CK soils, and that this difference was consistent over 20 years. Despite this unwanted phenomenon, our results provide cause for optimism: the normalized tet(L) content in the three plots is essentially constant over time. Furthermore, in OM soil, not only tet(L) did not increase over time (in spite of the annual dose of tet(L) supplied via the addition of OM), the level of intI1 in OM soil was even decreasing in the time series, even though intI1 was significantly and positively correlated to tet(L). In addition, the main genotyope of tet(L) in the archived OM soils, in fresh NPK soil and OM soil, in fresh OM, as well as in irrigation water was always the same, and tet(L) was always associated with the same culturable organisms in OM and OM soils. The increase of tet(L) in OM soils was a corollary result accompanied by an increase in Firmicutes (Bacillales). Application of OM has previously been found to push B. asahii to the fore in alkaline soils, which subsequently played a key role in increased crop yield and soil fertility. Although we here report that application of OM also increased the relative abundance of tet(L), OM application may not further the risk of tet(L) dissemination, because the abundance of tet(L) in OM soils was essentially constant and, importantly, was always associated with the same genotype and the same organisms as in the OM itself. This result lends further support for the use of OM to maintain soil fertility.
Author Contributions
JD, YF, and XL initiated the project. YF, YW, and XL supplied soil materials and reagents. JD contributed his scientific advice during the work and MS revision. SP performed the experiments, analyzed the data, and wrote the paper. All authors reviewed the manuscript.
Conflict of Interest Statement
The authors declare that the research was conducted in the absence of any commercial or financial relationships that could be construed as a potential conflict of interest.
Acknowledgments
Research in this work was funded by National Natural Science Foundation of China (41501275 and 21377137), National Key Research and Development Plan (2016YFD0800206), China Postdoctoral Science Foundation (2016M591940), and Jiangsu Planned Projects for Postdoctoral Research Funds (1501077B).
Supplementary Material
The Supplementary Material for this article can be found online at: https://www.frontiersin.org/articles/10.3389/fmicb.2018.01140/full#supplementary-material
References
Amann, R. I., Ludwig, W., and Schleifer, K. H. (1995). Phylogenetic identification and in situ detection of individual microbial cells without cultivation. Microbiol. Rev. 59, 143–169.
Berg, J., Thorsen, M. K., Holm, P. E., Jensen, J., Nybroe, O., and Brandt, K. K. (2010). Cu exposure under field conditions coselects for antibiotic resistance as determined by a novel cultivation-independent bacterial community tolerance assay. Environ. Sci. Technol. 44, 8724–8728. doi: 10.1021/es101798r
Biddle, J. F., Fitz-Gibbon, S., Schuster, S. C., Brenchley, J. E., and House, C. H. (2008). Metagenomic signatures of the Peru Margin subseafloor biosphere show a genetically distinct environment. Proc. Natl. Acad. Sci. U.S.A. 105, 10583–10588. doi: 10.1073/pnas.0709942105
Böckelmann, U., Dorries, H. H., Ayuso-Gabella, M. N., de Marcay, M. S., Tandoi, V., Levantesi, C., et al. (2009). Quantitative PCR monitoring of antibiotic resistance genes and bacterial pathogens in three European artificial groundwater recharge systems. Appl. Environ. Microbiol. 75, 154–163. doi: 10.1128/AEM.01649-08
Byrne-Bailey, K. G., Gaze, W. H., Kay, P., Boxall, A. B., Hawkey, P. M., and Wellington, E. M. (2009). Prevalence of sulfonamide resistance genes in bacterial isolates from manured agricultural soils and pig slurry in the United Kingdom. Antimicrob. Agents Chemother. 53, 696–702. doi: 10.1128/AAC.00652-07
Cary, S. C., and Fierer, N. (2014). The importance of sample archiving in microbial ecology. Nat. Rev. Microbiol. 12, 789–790. doi: 10.1038/nrmicro3382
Chen, C. Q., Hu, Q. Y., Sun, B., and Zhang, J. B. (2010). Simulating trends in soil organic carbon of in calcareous fluvo-aquic soil under long-term fertilization using the DNDC model. Plant Nutrit. Fertil. Sci. 16, 1410–1417. doi: 10.3390/su9071129
Chen, Q., An, X., Li, H., Su, J., Ma, Y., and Zhu, Y. G. (2016). Long-term field application of sewage sludge increases the abundance of antibiotic resistance genes in soil. Environ. Int. 92–93, 1–10. doi: 10.1016/j.envint.2016.03.026
Chen, T., Liu, X., Li, X., Zhao, K., Zhang, J., Xu, J., et al. (2009). Heavy metal sources identification and sampling uncertainty analysis in a field-scale vegetable soil of Hangzhou, China. Environ. Pollut. 157, 1003–1010. doi: 10.1016/j.envpol.2008.10.011
Chopra, I., and Roberts, M. (2001). Tetracycline antibiotics: mode of action, applications, molecular biology, and epidemiology of bacterial resistance. Microbiol. Mol. Biol. Rev. 65, 232–260. doi: 10.1128/MMBR.65.2.232-260.2001
Chu, H., Lin, X., Fujii, T., Morimoto, S., Yagi, K., Hu, J., et al. (2007). Soil microbial biomass, dehydrogenase activity, bacterial community structure in response to long-term fertilizer management. Soil Biol. Biochem. 39, 2971–2976. doi: 10.1016/j.soilbio.2007.05.031
Clark, I. M., and Hirsch, P. R. (2008). Survival of bacterial DNA and culturable bacteria in archived soils from the Rothamsted Broadbalk experiment. Soil Biol. Biochem. 40, 1090–1102. doi: 10.1016/j.soilbio.2007.11.021
Cytryn, E. (2013). The soil resistome: the anthropogenic, the native, and the unknown. Soil Biol. Biochem. 63, 18–23. doi: 10.1016/j.soilbio.2013.03.017
D'Costa, V. M., Griffiths, E., and Wright, G. D. (2007). Expanding the soil antibiotic resistome: exploring environmental diversity. Curr. Opin. Microbiol. 10, 481–489. doi: 10.1016/j.mib.2007.08.009
De Nobili, M., Contin, M., and Brookes, P. (2006). Microbial biomass dynamics in recently air-dried and rewetted soils compared to others stored air-dry for up to 103 years. Soil Biol. Biochem. 38, 2871–2881. doi: 10.1016/j.soilbio.2006.04.044
Devarajan, N., Laffite, A., Graham, N. D., Meijer, M., Prabakar, K., Mubedi, J. I., et al. (2015). Accumulation of clinically relevant antibiotic-resistance genes, bacterial load, and metals in freshwater lake sediments in central europe. Environ. Sci. Technol. 49, 6528–6537. doi: 10.1021/acs.est.5b01031
Dolfing, J., and Feng, Y. (2015). The importance of soil archives for microbial ecology. Nature Rev. Microbiol. 13:1. doi: 10.1038/nrmicro3382-c1
Dolfing, J., Vos, A., Bloem, J., Ehlert, P. A. I., Naumova, N. B., and Kuikman, P. J. (2004). Microbial diversity in archived soils. Science 306:813. doi: 10.1126/science.306.5697.813a
Feng, Y., Chen, R., Hu, J., Zhao, F., Wang, J., Chu, H., et al. (2015). Bacillus asahii comes to the fore in organic manure fertilized alkaline soils. Soil Biol. Biochem. 81, 186–194. doi: 10.1016/j.soilbio.2014.11.021
Fierer, N., Leff, J. W., Adams, B. J., Nielsen, U. N., Bates, S. T., Lauber, C. L., et al. (2012). Cross-biome metagenomic analyses of soil microbial communities. Proc. Nat. Acad. Sci. U.SA. 109, 21390–21395. doi: 10.1073/pnas.1215210110
Forsberg, K. J., Reyes, A., Wang, B., Selleck, E. M., Sommer, M. O., and Dantas, G. (2012). The shared antibiotic resistome of soil bacteria and human pathogens. Science 337, 1107–1111. doi: 10.1126/science.1220761
Gillings, M. R., Gaze, W. H., Pruden, A., Smalla, K., Tiedje, J. M., and Zhu, Y. G. (2015). Using the class 1 integron-integrase gene as a proxy for anthropogenic pollution. ISME J. 9, 1269–1279. doi: 10.1038/ismej.2014.226
Gillings, M. R., Krishnan, S., Worden, P. J., and Hardwick, S. A. (2008). Recovery of diverse genes for class 1 integron-integrases from environmental DNA samples. FEMS Microbiol. Lett. 287, 56–62. doi: 10.1111/j.1574-6968.2008.01291.x
Gong, W., Yan, X. Y., Wang, J. Y., Hu, T. X., and Gong, Y. B. (2009). Long-term manuring and fertilization effects on soil organic carbon pools under a wheat-maize cropping system in North China Plain. Plant Soil 314, 67–76. doi: 10.1007/s11104-008-9705-2
Graham, D. W., Knapp, C. W., Christensen, B. T., McCluskey, S., and Dolfing, J. (2016). Appearance of beta-lactam resistance genes in agricultural soils and clinical isolates over the 20(th) century. Sci. Rep. 6:21550. doi: 10.1038/srep21550
Gu, W., Lu, Y., Tan, Z., Xu, P., Xie, K., Li, X., et al. (2017). Fungi diversity from different depths and times in chicken manure waste static aerobic composting. Bioresour. Technol. 239, 447–453. doi: 10.1016/j.biortech.2017.04.047
Guo, X., Li, J., Yang, F., Yang, J., and Yin, D. (2014). Prevalence of sulfonamide and tetracycline resistance genes in drinking water treatment plants in the Yangtze River Delta, China. Sci. Total Environ. 493, 626–631. doi: 10.1016/j.scitotenv.2014.06.035
Heuer, H., and Smalla, K. (2007). Manure and sulfadiazine synergistically increased bacterial antibiotic resistance in soil over at least two months. Environ. Microbiol. 9, 657–666. doi: 10.1111/j.1462-2920.2006.01185.x
Hu, Y., Yang, X., Li, J., Lv, N., Liu, F., Wu, J., et al. (2016). The bacterial mobile resistome transfer network connecting the animal and human microbiomes. Appl. Environ. Microbiol. 82, 6672–6681. doi: 10.1128/AEM.01802-16
Jechalke, S., Kopmann, C., Rosendahl, I., Groeneweg, J., Weichelt, V., Krogerrecklenfort, E., et al. (2013). Increased abundance and transferability of resistance genes after field application of manure from sulfadiazine-treated pigs. Appl. Environ. Microbiol. 79, 1704–1711. doi: 10.1128/AEM.03172-12
Ji, X., Shen, Q., Liu, F., Ma, J., Xu, G., Wang, Y., et al. (2012). Antibiotic resistance gene abundances associated with antibiotics and heavy metals in animal manures and agricultural soils adjacent to feedlots in Shanghai; China. J. Hazard. Mater. 235–236, 178–185. doi: 10.1016/j.jhazmat.2012.07.040
Knapp, C. W., Dolfing, J., Ehlert, P. A., and Graham, D. W. (2010). Evidence of increasing antibiotic resistance gene abundances in archived soils since 1940. Environ. Sci. Technol. 44, 580–587. doi: 10.1021/es901221x
Knapp, C. W., McCluskey, S. M., Singh, B. K., Campbell, C. D., Hudson, G., and Graham, D. W. (2011). Antibiotic resistance gene abundances correlate with metal and geochemical conditions in archived scottish soils. PLoS ONE 6:e27300. doi: 10.1371/journal.pone.0027300
Koike, S., Krapac, I. G., Oliver, H. D., Yannarell, A. C., CheeSanford, J. C., Aminov, R. I., et al. (2007). Monitoring and source tracking of tetracycline resistance genes in lagoons and groundwater adjacent to swine production facilities over a 3-year period. Appl. Environ. Microbiol. 73, 4813–4823 doi: 10.1128/AEM.00665-07
Kyselková, M., Jirout, J., Chronáková, A., Vrchotová, N., Bradley, R., Schmitt, H., et al. (2013). Cow excrements enhance the occurrence of tetracycline resistance genes in soil regardless of their oxytetracycline content. Chemosphere 93, 2413–2418. doi: 10.1016/j.chemosphere.2013.08.058
Lin, H., Sun, W., Zhang, Z., Chapman, S. J., Freitag, T. E., Fu, J., et al. (2016). Effects of manure and mineral fertilization strategies on soil antibiotic resistance gene levels and microbial community in a paddy-upland rotation system. Environ. Pollut. 211, 332–337. doi: 10.1016/j.envpol.2016.01.007
Liu, P., Jia, S., He, X., Zhang, X., and Ye, L. (2017). Different impacts of manure and chemical fertilizers on bacterial community structure and antibiotic resistance genes in arable soils. Chemosphere 188, 455–464. doi: 10.1016/j.chemosphere.2017.08.162
Lu, R. K. (2000). Methods of Soil Agricultural Chemical Analysis. Beijing: Agricultural Science and Technology Publishing House.
Luo, Y., Xu, L., Rysz, M., Wang, Y. Q., Zhang, H., and Alvarez, P. J. J. (2011). Occurrence and transport of tetracycline, sulfonamide, quinolone, and macrolide antibiotics in the Haihe River Basin, China. Environ. Sci. Technol. 45, 1827–1833. doi: 10.1021/es104009s
Ma, L., Li, A. D., Yin, X. L., and Zhang, T. (2017). The prevalence of Integrons as the carrier of antibiotic resistance genes in natural and man-made environments. Environ. Sci. Technol. 51, 5721–5728. doi: 10.1021/acs.est.6b05887
Meng, L., Ding, W. X., and Cai, Z. C. (2005). Long-term application of organic manure and nitrogen fertilizer on N2O emissions, soil quality and crop production in a sandy loam soil. Soil Biol. Biochem. 37, 2037–2045. doi: 10.1016/j.soilbio.2005.03.007
Neher, D. A., Weicht, T. R., Bates, S. T., Leff, J. W., and Fierer, N. (2013). Changes in bacterial and fungal communities across compost recipes, preparation methods, and composting times. PLoS ONE 8:e79512. doi: 10.1371/journal.pone.0079512
Peng, S., Feng, Y., Wang, Y., Guo, X., Chu, H., and Lin, X. (2017). Prevalence of antibiotic resistance genes in soils after continually applied with different manure for 30 years. J. Hazard. Mat. 340, 16–25. doi: 10.1016/j.jhazmat.2017.06.059
Peng, S., Wang, Y., Zhou, B., and Lin, X. (2015). Long-term application of fresh and composted manure increase tetracycline resistance in the arable soil of eastern China. Sci. Total Environ. 506–507, 279–286. doi: 10.1016/j.scitotenv.2014.11.010
Peng, S., Zhou, B., Wang, Y., Lin, X., Wang, H., and Qiu, C. (2016). Bacteria play a more important role than nutrients in the accumulation of tetracycline resistance in manure-treated soil. Biol. Fertil. Soil 52, 655–663. doi: 10.1007/s00374-016-1105-9
Popowska, M., Rzeczycka, M., Miernik, A., Krawczyk-Balska, A., Walsh, F., and Duffy, B. (2012). Influence of soil use on prevalence of tetracycline, streptomycin, and erythromycin resistance and associated resistance genes. Antimicrob. Agents Chemother. 56, 1434–1443. doi: 10.1128/AAC.05766-11
Pruden, A., Larsson, D. G., Amezquita, A., Collignon, P., Brandt, K. K., Graham, D. W., et al. (2013). Management options for reducing the release of antibiotics and antibiotic resistance genes to the environment. Environ. Health Perspect. 121, 878–885. doi: 10.1289/ehp.1206446
Sengeløv, G., Agersø, Y., Halling-Sørensen, B., Baloda, S. B., Andersen, J. S., and Jensen, L. B. (2003). Bacterial antibiotic resistance levels in Danish farmland as a result of treatment with pig manure slurry. Environ. Int. 28, 587–595. doi: 10.1016/S0160-4120(02)00084-3
Sommer, M. O. A. (2014). Barriers to the spread of resistance. Nature 509, 567–568. doi: 10.1038/nature13342
Tzeneva, V. A., Salles, J. F., Naumova, N., de Vos, W. M., Kuikman, P. J., Dolfing, J., et al. (2009). Effect of soil sample preservation, compared to the effect of other environmental variables, on bacterial and eukaryotic diversity. Res. Microbiol. 160, 89–98. doi: 10.1016/j.resmic.2008.12.001
Udikovic-Kolic, N., Wichmann, F., Broderick, N. A., and Handelsman, J. (2014). Bloom of resident antibiotic-resistant bacteria in soil following manure fertilization. Proc. Natl. Acad. Sci. U.S.A. 111, 15202–15207. doi: 10.1073/pnas.1409836111
Wang, F. H., Qiao, M., Lv, Z. E., Guo, G. X., Jia, Y., Su, Y. H., et al. (2014). Impact of reclaimed water irrigation on antibiotic resistance in public parks, Beijing, China. Environ. Pollut. 184, 247–253. doi: 10.1016/j.envpol.2013.08.038
Wepking, C., Avera, B., Badgley, B., Barrett, J. E., Franklin, J., Knowlton, K. F., et al. (2017). Exposure to dairy manure leads to greater antibiotic resistance and increased mass-specific respiration in soil microbial communities. Proc. R. Soc. B. 284, 2016–2233 doi: 10.1098/rspb.2016.2233
Wu, N., Qiao, M., Zhang, B., Cheng, W. D., and Zhu, Y. G. (2010). Abundance and diversity of tetracycline resistance genes in soils adjacent to representative swine feedlots in China. Environ. Sci. Technol. 44, 6933–6939. doi: 10.1021/es1007802
Xi, C., Zhang, Y., Marrs, C. F., Ye, W., Simon, C., Foxman, B., et al. (2009). Prevalence of antibiotic resistance in drinking water treatment and distribution systems. Appl. Environ. Microbiol. 75, 5714–5718. doi: 10.1128/AEM.00382-09
You, Y., Hilpert, M., and Ward, M. J. (2012). Detection of a common and persistent tet(L)-carrying plasmid in chicken-waste-impacted farm soil. Appl. Environ. Microbiol. 78, 3203–3213. doi: 10.1128/AEM.07763-11
Zhang, J., Chen, M., Sui, Q., Tong, J., Jiang, C., Lu, X., et al. (2016). Impacts of addition of natural zeolite or a nitrification inhibitor on antibiotic resistance genes during sludge composting. Water Res. 91, 339–349. doi: 10.1016/j.watres.2016.01.010
Zhang, T., Zhang, M., Zhang, X., and Fang, H. H. (2009). Tetracycline resistance genes and tetracycline resistant lactose-fermenting Enterobacteriaceae in activated sludge of sewage treatment plants. Environ. Sci. Technol. 43, 3455–3460. doi: 10.1021/es803309m
Zhang, X. X., and Zhang, T. (2011). Occurrence, abundance, and diversity of tetracycline resistance genes in 15 sewage treatment plants across China and other global locations. Environ. Sci. Technol. 45, 2598–2604. doi: 10.1021/es103672x
Keywords: antibiotic resistance genes, class 1 integron, organic manure, archived soil, real-time PCR
Citation: Peng S, Dolfing J, Feng Y, Wang Y and Lin X (2018) Enrichment of the Antibiotic Resistance Gene tet(L) in an Alkaline Soil Fertilized With Plant Derived Organic Manure. Front. Microbiol. 9:1140. doi: 10.3389/fmicb.2018.01140
Received: 07 November 2017; Accepted: 14 May 2018;
Published: 31 May 2018.
Edited by:
Satoru Suzuki, Ehime University, JapanReviewed by:
Xuesong Luo, Huazhong Agricultural University, ChinaMichael S. Strickland, University of Idaho, United States
Copyright © 2018 Peng, Dolfing, Feng, Wang and Lin. This is an open-access article distributed under the terms of the Creative Commons Attribution License (CC BY). The use, distribution or reproduction in other forums is permitted, provided the original author(s) and the copyright owner are credited and that the original publication in this journal is cited, in accordance with accepted academic practice. No use, distribution or reproduction is permitted which does not comply with these terms.
*Correspondence: Xiangui Lin, xglin@issas.ac.cn