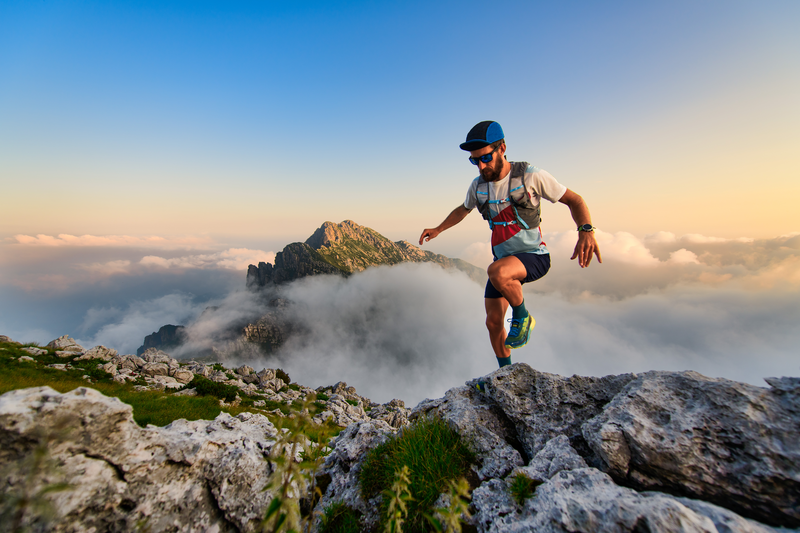
94% of researchers rate our articles as excellent or good
Learn more about the work of our research integrity team to safeguard the quality of each article we publish.
Find out more
ORIGINAL RESEARCH article
Front. Microbiol. , 23 May 2018
Sec. Food Microbiology
Volume 9 - 2018 | https://doi.org/10.3389/fmicb.2018.01051
This article is part of the Research Topic Advances in Postharvest Pathology of Fruits and Vegetables View all 17 articles
The main goal of this study was to investigate the influences of Phomopsis longanae Chi infection on activities of cell wall-degrading enzymes (CWDEs), and contents of cell wall components in pericarp of harvested “Fuyan” longan (Dimocarpus longan Lour. cv. Fuyan) fruit and its relation to disease development. The results showed that, compared with the control samples, P. longanae-inoculated longans showed higher fruit disease index, lower content of pericarp cell wall materials (CWMs), as well as lower contents of pericarp cell wall components (chelate-soluble pectin (CSP), sodium carbonate-soluble pectin, hemicelluloses, and cellulose), but higher content of pericarp water-soluble pectin (WSP). In addition, the inoculation treatment with P. longanae significantly promoted the activities of CWDEs including pectinesterase, polygalacturonase, β-galactosidase, and cellulase. The results suggested that the P. longanae stimulated-disease development of harvested longans was due to increase in activities of pericarp CWDEs, which might accelerate the disassembly of pericarp cell wall components. In turn, resulting in the degradation of pericarp cell wall, reduction of pericarp mechanical strength, and subsequently leading to the breakdown of longan pericarp tissues. Eventually resulting in development of disease development and fruit decay in harvested longans during storage at 28°C.
In developed countries, fruit decay caused by pathogens affects 20–25% of the harvested fruits during post-harvest handling and storage. While in developing countries, the situation is even worse due to inadequate transportation, storage, and preservation facilities for fruits (Al-Hindi et al., 2011). Infection by pathogenic bacteria and fungi could take place in almost every step of fruit production from pre-harvest to post-harvest storage and marketing (Yao and Tian, 2005; Aghdam and Fard, 2017; Li et al., 2017).
In botanic cells, the cell wall is the first barrier against the infection by fungal pathogens (Kubicek et al., 2014). Cell wall-degrading enzymes (CWDEs) secreted by pathogens play a key role in penetrating the cell wall to utilize the nutrients (Kang and Buchenauer, 2000; Lalaoui et al., 2000; Kikot et al., 2009; Tian et al., 2009; Gharbi et al., 2015; Ramos et al., 2016). There is a diverse array of CWDEs, including polygalacturonase (PG), pectin methylgalacturonase (PMG), pectinesterase (PE), pectin lyase (PL), pectate lyase (PNL), cellulase (CX), β-glucosidase, and xylanases (Al-Hindi et al., 2011; Li et al., 2012; Kubicek et al., 2014). Ramos et al. (2016) found that Macrophomina phaseolina induced the cell wall degradation of maize and sunflower, which was initiated by the pectinase that was the first CWDE secreted by M. phaseolina. The activities of PG and PMG were higher than CX that appeared in the later stage of the degradation process (Ramos et al., 2016). This sequence promoted the initial tissue maceration before the degradation of cell wall materials (CWMs). It was reported that Fusarium culmorum was able to secrete CWDEs including cellulases, xylanases, and pectinases. These CWDEs degraded the wheat spike plant tissues (cellulose, xylan, and pectin) and enabled the invasion to the tissues by F. culmorum (Kang and Buchenauer, 2000). Kang and Buchenauer (2000) also reported that in the cell wall, the degree of pectin degradation was higher compared with cellulose and xylan at the early stage of infection, which implied that there might be earlier secretion or higher activity of pectinases over cellulases or xylanases. Moreover, Li et al. (2012) showed that Botryodiplodia theobromae Pat. caused the stem-end rot of mangoes by producing PG, PMG, and CX that disrupted the fruit tissues in the deterioration process. Therefore, it can be concluded that the secretion of CWDEs plays an important role in the degradation of plant cell wall during pathogenesis.
Longan is a well-known subtropical fruit with a short shelf life at room temperature due to its high susceptibility to pathogenic infections (Chen et al., 2014; Lin et al., 2017a,b,c, 2018; Zhang et al., 2017, 2018; Sun et al., 2018). Our previous studies demonstrated that Phomopsis longanae Chi (P. longanae) is one of the dominating pathogens that can cause postharvest decay of longans (Chen et al., 2011a,b, 2014; Lin et al., 2017a). To date, there has been no report on the types or activities of the CWDEs produced by P. longanae, and there is still a lack of research regarding the effect of these enzymes on infected longan fruits. The objective of this study was to investigate the changes of CWDEs activities during disease development in P. longanae-inoculated longan fruits and their effect on the degradation of cell wall. This study also aimed to elucidate the CWDEs’ function during the infection of P. longanae on harvested longan fruits.
Phomopsis longanae culturing and the preparation of spore suspension were performed as described in our previous publication (Chen et al., 2014). The concentration of spore suspension was diluted to 1 × 104 spores mL-1 and used for inoculation.
“Fuyan” longan (Dimocarpus longan Lour. cv. Fuyan) fruit at commercial maturity were handpicked from a longan orchard (Quanzhou, Fujian, China). The harvested fruit were carefully packed and transported to a research laboratory in Fujian Agriculture and Forestry University (Fuzhou, Fujian, China) within 3 h and stored at 4°C. Fruit in uniform maturity and size were selected for the experiment and any rotten or damaged fruit were excluded.
The fruits were dipped in 0.5% sodium hypochlorite solution for 10 s to eliminate surface microorganisms, and then air-dried. A total of 150 fruits were employed to evaluate the properties of harvested fruits on day 0. The remaining 3000 fruits were randomly divided into two lots (1500 fruits per lot) for the control and P. longanae-inoculated treatment. The control group (1500 fruits) was dipped into the sterile deionized water for 5 min. The P. longanae-inoculated group (1500 fruits) was immersed into the P. longanae spore suspension (1 × 104 spores mL-1) for 5 min. All fruits were then air dried and packed in a polyethylene bag with a thickness of 0.015 mm. Each bag contained 50 longan fruits and 30 bags were used for each treatment. The samples were then stored at 28°C with a relative humidity of 90%. For each treatment, three bags of fruit (total 150 longan fruits) were randomly selected on a daily basis during the storage period and used for the assessments of longan fruit. All the evaluations were conducted in triplicate.
Longan fruit disease was assessed based on our previous study (Chen et al., 2014). The lesion proportion on fruit surface of 50 individual longan fruits was measured and defined to five disease scales. The calculations of fruit disease index were performed based on the method of Chen et al. (2014).
Extraction of CWM was based on the modified procedures described in Duan et al. (2011) and Chen et al. (2017a,b). Ten grams of frozen longan pericarp were homogenized in 200 mL of 80 % ethanol. The mixture was boiled for 30 min with stirring. The solution was then cooled to room temperature, followed by filtration with filter papers (ϕ11 cm Medium-Speed, Whatman, Zhejiang, China). The residues were subsequently washed with 200 mL of 80% ethanol, immersed into 50 mL of 90 % dimethyl sulfoxide for 8 h to remove starches. It was subsequently washed with 200 mL of acetone, dried for 3 days at 40°C to report the final weight as CWM.
Cell wall components fractionation and analysis were followed the procedures reported in Rugkong et al. (2010) and Chen et al. (2017a,b) with some modifications. Water-soluble pectin (WSP) was extracted via dispersing CWM (300 mg) in sodium acetate buffer (50 mmol L-1, pH 6.5) for 6 h with shaking (SKY-200B, SUKUN, Shanghai, China). The mixture was then centrifuged (10,000 × g, 4°C) for 10 min (Centrifuge 5810R, Eppendorf AG, Hamburg, Germany). The sediment was immersed in 20 mL of 50 mmol L-1 ethylene diamine tetraacetic acid (EDTA) with 1 mol L-1 NaCl (pH 6.8). After shaking and centrifugation, the supernatant containing chelate-soluble pectin (CSP) was collected. Residues were further immersed in 20 mL of 50 mmol L-1 Na2CO3 with 20 mmol L-1 NaBH4 for another shaking and centrifugation. The supernatant containing Na2CO3-soluble pectin (NSP) was collected. The remaining residue was further dipped in 10 mL of 4 mmol L-1 KOH solution with 100 mmol L-1 NaBH4 for shaking and centrifugation, and the supernatant collected was considered as hemicellulose. A volume of 1% sodium sulfite and 50 mL of 8 mol L-1 KOH solution with 10 mmol L-1 NaBH4 were then added to the remaining residue for shaking and centrifugation, and the supernatant containing cellulose was collected. The amounts of WSP, CSP, and NSP were determined via m-hydroxydiphenyl method (Wang et al., 2015; Chen et al., 2017a,b). The amount of hemicellulose and cellulose were determined based on the anthrone method (Wang et al., 2015; Chen et al., 2017a,b).
Enzyme extraction was based on the procedures described by Andrews and Li (1995) and Chen et al. (2017a,b). Briefly, frozen longan pericarp (1 g) was ground with 8 mL of 40 mmol L-1 sodium acetate buffer (containing 100 mmol L-1 NaCl, 2% mercaptoethanol and 5% PVP, pH 5.2). The homogenous solution was centrifuged (12,000 × g, 20 min) at 4°C. The collected supernatant was used to measure the activities of PE, PG, β-galactosidase and cellulase.
Pectinesterase activity was measured by combining 3 mL of crude enzyme with 10 mL of 1% pectin for the titration using 0.01 mol L-1 NaOH (pH 7.4 at 37°C for 30 min). The amount of enzyme that consumed 1 μmol NaOH solution per hour was used to define one unit of PE activity.
Polygalacturonase activity was determined by mixing 5 mL of 20 mmol L-1 sodium acetate (pH 4.0), 2 mL of 1% (w/v) polygalacturonic acid, and 1 mL of crude enzyme extract, followed by incubation at 37°C for the 30 min. A volume of 2 mL of 10 mmol L-1 Na2B4O7 was then added to the reaction mixture before terminating the reaction with 0.1 mL of 1% (w/v) 2-cyanoacetamide and boiling for 5 min. The boiled reaction mixture without adding substrate was used as the blank. The concentrations of the reducing groups were measured at 276 nm with D-galacturonic acid as the standard. The amount of enzyme producing 1 μmol galacturonic acid per hour was considered as one unit of PG activity.
To determine the β-galactosidase activity, 5 mL of 20 mmol L-1 sodium acetate (pH 4.7), 2 mL of 3 mmol L-1 p-nitrophenyl-β-D-galactopyranoside, and 1 mL of crude enzyme were combined and incubated at 37°C for 30 min. A volume of 2 mL of 0.2 mmol L-1 Na2CO3 was then added to the mixture. The concentration of the reducing product was determined at 400 nm with p-nitrophenol (PNP) as a standard. One unit of β-galactosidase activity referred to the amount of enzyme that produced 1 μmol PNP per hour.
Cellulase activity was assayed by mixing 5 mL of 20 mmol L-1 sodium acetate (pH 4.0), 1 mL of 0.25% carboxymethyl cellulose, and 1 mL of crude enzyme extract. The mixture was incubated at 37°C for 30 min followed by addition of 2 mL of 10 mmol L-1 Na2B4O7 and 0.1 mL of 1% (w/v) 2-cyanoacetamide. The reaction was stopped by heating in a boiling water bath for 5 min. A blank was prepared for each sample by boiling the reaction mixture before addition of substrate. One unit of cellulase activity referred to the amount of enzyme that produced 1 μg D-glucose per hour.
The activities of CWDEs were presented as U mg-1 protein. Protein content was measured following the method of Bradford (1976) using bovine serum as standard.
All experiments were repeated three time and data were acquired. The values in figures were expressed in the format of the mean values and standard errors. Analysis of variance (ANOVA) was used to analyze the data using the software (SPSS version 17.0). Student’s t-test was used to compare the mean values of the data set. A P-value of less than or equal to 0.05 or 0.01 was considered statistically significant.
As indicated in Figure 1, control fruits were intact without lesion during the first two storage days, and then the fruit disease index gradually increased with further storage. But the disease index of P. longanae-inoculated fruit increased rapidly throughout the storage period. Statistical analysis reveals that P. longanae-inoculated fruit had consistently higher fruit disease index than the control fruit at the same storage time (P < 0.01). After 5 days of storage, the disease index of P. longanae-inoculated longans was 0.9, which was almost twice as high as that of the control longans. This clearly demonstrates that inoculation treatment could significantly increase disease development of longans during storage.
FIGURE 1. Effects of Phomopsis longanae infection on fruit disease index of harvested longan fruit during storage at 28°C. The asterisks indicate significant difference between control and P. longanae-inoculated fruit (∗∗P < 0.01). ∘, control; •, P. longanae-inoculation treatment.
As shown in Figure 2, the pericarp CWM content decreased rapidly during storage and CWM readings of P. longanae-inoculated longans was significantly (P < 0.05) lower than the control longans on each storage day. After 5 days of storage, the CWM in pericarp of control longans decreased from 12.18 to 6.23 mg g-1, while P. longanae-inoculated longans has a pericarp CWM value of 5.08 mg g-1 on storage day 5. Correlation analysis indicates that there was a significant negative correlation between disease index (y) and CWM content (x) (y = 1.5173-0.1362 x, r = -0.915, P < 0.05) for the P. longanae-inoculated longans during storage. These findings indicate that the disease development or loss of disease resistance of longan fruits during storage could lead to cell wall disassembly.
FIGURE 2. Effects of P. longanae infection on cell wall material (CWM) in pericarp of harvested longan fruit during storage at 28°C. The asterisks indicate significant difference between control and P. longanae-inoculated fruit (∗P < 0.05). ∘, control; •, P. longanae-inoculation treatment.
Cell wall components including pectic substances, hemicelluloses, and cellulose constitute the material basis for the mechanical properties of cell wall, and also for maintaining the mechanical strength of the pericarp (Huang et al., 1999; Vorwerk et al., 2004). Pectic substances like WSP, CSP, and NSP are located in the primary cell wall and the middle lamella. The degradation of pectic substances led to cellulose and hemicellulose disassembly, which caused pericarp tissue loosing or fruit softening (Duan et al., 2008; Zhou et al., 2011; Chen et al., 2017a,b). In a similar study performed on litchi fruit, higher levels of structural materials like insoluble pectin, hemicellulose, and cellulose were observed in the cell walls of ‘Huaizhi’ litchi fruit pericarp compared with ‘Nuomici’ litchis, which might notably correlate to better pericarp structural strength (Huang et al., 1999).
The data acquired from this work indicate that P. longanae-inoculated longans had a faster increase in pericarp WSP than the control longans (Figure 3A). P. longanae-inoculated longans also had faster reduction in pericarp CSP contents (Figure 3B), NSP (Figure 3C), hemicellulose (Figure 4A), and cellulose (Figure 4B) than the control longans. Furthermore, fruit disease index (y) shows negative correlations with CSP content (x) (y = 1.6142-8.7271 x, r = -0.971, P < 0.01), NSP content (x) (y = 1.1252-2.9018 x, r = -0.962, P < 0.01), hemicellulose (x) (y = 1.0903-2.584 x, r = -0.916, P < 0.05) and cellulose (x) (y = 1.58-0.4145 x, r = -0.956, P < 0.01) in pericarp of P. longanae-inoculated longan fruit during storage. However, a positive correlation between fruit disease index (y) and WSP content (x) (y = -0.5308 + 8.3717 x, r = 0.972, P < 0.01) in pericarp of P. longanae-inoculated longan fruit was observed. In short, P. longanae-inoculation treatment accelerated the degradation of the cell wall components including CSP, NSP, hemicellulose, and cellulose in longans pericarp cell wall and middle lamella; however, the degraded cell wall components like WSP was elevated. Therefore, the mechanical strength of the cell wall of longan pericarp was decreased during disease development. Cell wall disassembly of longan pericarp may facilitate further pathogen invasion and dissemination.
FIGURE 3. Effects of P. longanae infection on water-soluble pectin (WSP) (A), chelate-soluble pectin (CSP) (B) and sodium carbonate-soluble pectin (C) in pericarp of harvested longan fruit during storage at 28°C. The asterisks indicate significant difference between control and P. longanae-inoculated fruit (∗P < 0.05, ∗∗P < 0.01). ∘, control; •, P. longanae-inoculation treatment.
FIGURE 4. Effects of P. longanae infection on hemicellulose (A) and cellulose (B) in pericarp of harvested longan fruit during storage at 28°C. The asterisks indicate significant difference between control and P. longanae-inoculated fruit (∗P < 0.05, ∗∗P < 0.01). ∘, control; •, P. longanae-inoculation treatment.
To further explain the change of cell wall components during disease development, changes in CWDEs were measured. PE and PG activities in pericarp of control fruit rose gradually toward the maximum on day 4 and decreased afterwards (Figures 5A,B). The PE and PG activities in pericarp of P. longanae-inoculated longans followed a similar trend as the control longans but had significantly (P < 0.05) higher activities (Figures 5A,B). It has been reported that the softening of pericarp tissues were associated with the changes in pectic substances, which could be attributed to the action of PE and PG (Liu et al., 2006). Specifically, PE can remove the methoxyl groups and catalyze the decomposition of galacturonic acid polymer to polygalacturonic acid, which enables PG to hydrolyze 1, 4-α-D-galacturonic bond of polygalacturonic acid to generate galacturonic acid (Liu et al., 2006; Lin et al., 2007; Rugkong et al., 2010; Wei et al., 2010). The degradation of pectic substances by the joint action of PE and PG can destroy the structure of middle lamella and decrease the mechanical strength of cell wall (Deng et al., 2005; Liu et al., 2006). In the present work, PE and PG activities increased significantly after inoculation treatment, which promoted the depolymerization and dissolution of pectin.
FIGURE 5. Effects of P. longanae infection on activities of pectinesterase (A), polygalacturonase (B), β-galactosidase (C), and cellulase (D) in pericarp of harvested longan fruit during storage at 28°C. The asterisks indicate significant difference between control and P. longanae-inoculated fruit (∗P < 0.05, ∗∗P < 0.01). ∘, control; •, P. longanae-inoculation treatment.
As indicated in Figure 5C, β-galactosidase activity in pericarp of P. longanae-inoculated longans exhibited a sharp increase on the first day of storage, then changed mildly on the second storage day, followed by a quick decline from days 2 to 4, and a rapid increase on day 5. However, the β-galactosidase activity in pericarp of the control longans increased steadily with progressing storage time, with significantly (P < 0.01) lower level in contrast to that of P. longanae-inoculated fruit from day 1 to day 4 (Figure 5C). β-galactosidase also plays a key role in the depolymerization and dissolution of pectic substances in fruits. It can hydrolyze β-1, 4-galactan bonds and separate galactosyl residues from pectin side chains, which may trigger some adverse reactions such as the production of ethylene and stress reaction, and thus further accelerate the disruption of cell wall structure (Liu et al., 2006; Lin et al., 2007; Wei et al., 2010; Chen et al., 2017a,b). The results of this study suggest that higher levels of β-galactosidase activity in inoculated fruits during storage contributed to the degradation of pectin polysaccharides.
Cellulose activity increased gradually in both control and P. longanae-inoculated longans during storage days 1–4 and then decreased (Figure 5D). After 5 days of storage, P. longanae-inoculated longans showed 72.5% higher value of pericarp cellulose activity as compared with the control longans. Cellulase is a multi-enzyme system including endo-1, 4-β-D-glucanase, exo-1, 4-β-D-glucanase, and β-1, 4-glucosidase (Lin et al., 2007). Cellulase could cause the degradation of cellulose and xyloglucan in cell wall structure, which resulted in pericarp tissue loosing and fruit softening (Deng et al., 2005; Liu et al., 2006; Zhou et al., 2011; Bu et al., 2013; Chen et al., 2017a,b). In this study, the enhanced activity of cellulase due to the P. longanae-inoculation treatment correlated well with cellulose degradation (Figure 4B) in pericarp of longan fruit during storage.
In summary, as compared with the control, fruit inoculated with P. longanae could lead to significantly higher fruit disease index, increased activity of CWDEs (e.g., PE, PG, β-galactosidase and cellulose), and lower levels of CWM and cell wall components (such as CSP, NSP, hemicelluloses, and cellulose) in pericarp of harvested longan fruit. These results indicate that P. longanae infection can accelerate the cell wall degradation of longan pericarp during disease development by promoting CWDEs activities, which decreased the mechanical strength of the cell walls, resulting in longan pericarp tissue softening, and eventually leading to fruit decay.
YC and HL designed the research. SZ, JZS, YL, and HW conducted the experiments and analyzed the data. YC and SZ wrote the manuscript. HL revised the manuscript. ML and JS edited English language of the manuscript. All authors approved the submission and publication of the manuscript.
This work was supported by the National Natural Science Foundation of China (Grant Nos. 31772035, 31671914, and 31171776), the Natural Science Foundation of Fujian Province of China (Grant No. 2017J01429), the Construction Projects of Top University at Fujian Agriculture and Forestry University of China (Grant No. 612014042), the Science Fund for Distinguished Young Scholars at Fujian Province of China (Grant No. KLa16036A), and the Science Fund for Distinguished Young Scholars at Fujian Agriculture and Forestry University of China (Grant No. XJQ201512).
The authors declare that the research was conducted in the absence of any commercial or financial relationships that could be construed as a potential conflict of interest.
Aghdam, M. S., and Fard, J. R. (2017). Melatonin treatment attenuates postharvest decay and maintains nutritional quality of strawberry fruits (Fragaria × anannasa cv. Selva) by enhancing GABA shunt activity. Food Chem. 221, 1650–1657. doi: 10.1016/j.foodchem.2016.10.123
Al-Hindi, R. R., Al-Najada, A. R., and Mohamed, S. A. (2011). Isolation and identification of some fruit spoilage fungi: screening of plant cell wall degrading enzymes. Afr. J. Microbiol. Res. 5, 443–448. doi: 10.5897/AJMR10.896
Andrews, P. K., and Li, S. L. (1995). Cell wall hydrolytic enzyme activity development of nonclimacteric sweet cherry (Prunus avium L.) fruit. J. Hortic. Sci. 70, 561–567. doi: 10.1080/14620316.1995.11515327
Bradford, M. M. (1976). A rapid and sensitive method for the quantitation of microgram quantities of protein utilizing the principle of protein-dye binding. Anal. Biochem. 72, 248–254. doi: 10.1016/0003-2697(76)90527-3
Bu, J. W., Yu, Y. C., Aisikaer, G., and Ying, T. J. (2013). Postharvest UV-C irradiation inhibits the production of ethylene and the activity of cell wall-degrading enzymes during softening of tomato (Lycopersicon esculentum L.) fruit. Postharvest Biol. Technol. 86, 337–345. doi: 10.1016/j.postharvbio.2013.07.026
Chen, Y. H., Hung, Y. C., Chen, M. Y., and Lin, H. T. (2017a). Effects of acidic electrolyzed oxidizing water on retarding cell wall degradation and delaying softening of blueberries during postharvest storage. LWT Food Sci. Technol. 84, 650–657. doi: 10.1016/j.lwt.2017.06.011
Chen, Y. H., Sun, J. Z., Lin, H. T., Hung, Y. C., Zhang, S., Lin, Y. F., et al. (2017b). Paper-based 1-MCP treatment suppresses cell wall metabolism and delays softening of Huanghua pears during storage. J. Sci. Food Agric. 97, 2547–2552. doi: 10.1002/jsfa.8072
Chen, Y. H., Lin, H. T., Jiang, Y. M., Zhang, S., Lin, Y. F., and Wang, Z. H. (2014). Phomopsis longanae Chi-induced pericarp browning and disease development of harvested longan fruit in association with energy status. Postharvest Biol. Technol. 93, 24–28. doi: 10.1016/j.postharvbio.2014.02.003
Chen, Y. H., Lin, H. T., Lin, Y. F., Zhang, J. N., and Zhao, Y. F. (2011a). Effects of Phomopsis longanae Chi infection on browning and active oxygen metabolism in pericarp of harvested longan fruits. Sci. Agric. Sin. 44, 4858–4866. doi: 10.3864/j.issn.0578-1752.2011.23.012
Chen, Y. H., Lin, H. T., Lin, Y. F., Zhao, Y. F., and Zhang, J. N. (2011b). Effects of Phomopsis longanae Chi infection on lipoxygenase activity and fatty acid constituents of membrane lipids in pericarp of harvested longan fruits. J. Trop. Subtrop. Bot. 19, 260–266. doi: 10.3969/j.issn.1005-3395.2011.03.011
Deng, Y., Wu, Y., and Li, Y. F. (2005). Changes in firmness, cell wall composition and cell wall hydrolases of grapes stored in high oxygen atmospheres. Food Res. Int. 38, 769–776. doi: 10.1016/j.foodres.2005.03.003
Duan, X. W., Cheng, G. P., Yang, E., Yi, C., Ruenroengklin, N., Lu, W. J., et al. (2008). Modification of pectin polysaccharides during ripening of postharvest banana fruit. Food Chem. 111, 144–149. doi: 10.1016/j.foodchem.2008.03.049
Duan, X. W., Zhang, H. Y., Zhang, D. D., Sheng, J. F., Lin, H. T., and Jiang, Y. M. (2011). Role of hydroxyl radical in modification of cell wall polysaccharides and aril breakdown during senescence of harvested longan fruit. Food Chem. 128, 203–207. doi: 10.1016/j.foodchem.2011.03.031
Gharbi, Y., Alkher, H., Triki, M. A., Barkallah, M., Emna, B., Trabelsi, R., et al. (2015). Comparative expression of genes controlling cell wall-degrading enzymes in Verticillium dahliae isolates from olive, potato and sunflower. Physiol. Mol. Plant Pathol. 91, 56–65. doi: 10.1016/j.pmpp.2015.05.006
Huang, X. M., Wang, H. C., Gao, F. F., and Huang, H. B. (1999). A comparative study of the pericarp of litchi cultivars susceptible and resistant to fruit cracking. J. Hortic. Sci. Biotechnol. 74, 351–354. doi: 10.1080/14620316.1999.11511120
Kang, Z., and Buchenauer, H. (2000). Ultrastructural and cytochemical studies on cellulose, xylan and pectin degradation in wheat spikes infected by Fusarium culmorum. J. Phytopathol. 148, 263–275. doi: 10.1046/j.1439-0434.2000.00489.x
Kikot, G. E., Hours, R. A., and Alconada, T. M. (2009). Contribution of cell wall degrading enzymes to pathogenesis of Fusarium graminearum: a review. J. Basic Microb. 49, 231–241. doi: 10.1002/jobm.200800231
Kubicek, C. P., Starr, T. L., and Glass, N. L. (2014). Plant cell wall-degrading enzymes and their secretion in plant-pathogenic fungi. Annu. Rev. Phytopathol. 52, 427–451. doi: 10.1146/annurev-phyto-102313-045831
Lalaoui, F., Halama, P., Dumortier, V., and Paul, B. (2000). Cell wall-degrading enzymes produced in vitro by isolates of Phaeosphaeria nodorum differing in aggressiveness. Plant Pathol. 49, 727–733. doi: 10.1046/j.1365-3059.2000.00491.x
Li, J. K., Lei, H. H., Song, H. M., Lai, T. F., Xu, X. B., and Shi, X. Q. (2017). 1-methylcyclopropene (1-MCP) suppressed postharvest blue mold of apple fruit by inhibiting the growth of Penicillium expansum. Postharvest Biol. Technol. 125, 59–64. doi: 10.1016/j.postharvbio.2016.11.005
Li, M., Gao, Z. Y., Hu, M. J., Zhou, S., Yang, D. P., Yang, B., et al. (2012). Pathogenicity of cell wall degrading enzymes produced by Botryodiplodia theobromae Pat. against mangoes. Agric. Biotechnol. 1, 18–21.
Lin, H. T., Zhao, Y. F., and Xi, Y. F. (2007). Changes in cell wall components and cell wall-degrading enzyme activities of postharvest longan fruit during aril breakdown. J. Plant Physiol. Mol. Biol. 33, 137–145. doi: 10.3321/j.issn:1671-3877.2007.02.007
Lin, Y. F., Chen, M. Y., Lin, H. T., Hung, Y. C., Lin, Y. X., Chen, Y. H., et al. (2017a). DNP and ATP induced alteration in disease development of Phomopsis longanae Chi-inoculated longan fruit by acting on energy status and reactive oxygen species production-scavenging system. Food Chem. 228, 497–505. doi: 10.1016/j.foodchem.2017.02.045
Lin, Y. F., Lin, Y. X., Lin, H. T., Chen, Y. H., Wang, H., and Shi, J. (2018). Application of propyl gallate alleviates pericarp browning in harvested longan fruit by modulating metabolisms of respiration and energy. Food Chem. 240, 863–869. doi: 10.1016/j.foodchem.2017.07.118
Lin, Y. F., Lin, Y. X., Lin, H. T., Ritenour, M. A., Shi, J., Zhang, S., et al. (2017b). Hydrogen peroxide-induced pericarp browning of harvested longan fruit in association with energy metabolism. Food Chem. 225, 31–36. doi: 10.1016/j.foodchem.2016.12.088
Lin, Y. F., Lin, Y. X., Lin, H. T., Shi, J., Chen, Y. H., and Wang, H. (2017c). Inhibitory effects of propyl gallate on membrane lipids metabolism and its relation to increasing storability of harvested longan fruit. Food Chem. 217, 133–138. doi: 10.1016/j.foodchem.2016.08.065
Liu, X. D., Wu, Z. X., Han, D. M., Chen, W. X., and Su, M. X. (2006). Changes of the cell-wall metabolism enzymes of pericarp of longan under Storage. Chin. J. Trop. Crops 2, 24–28. doi: 10.3969/j.issn.1000-2561.2006.02.005
Ramos, A. M., Gally, M., Szapiro, G., Itzcovich, T., Carabajal, M., and Levin, L. (2016). In vitro growth and cell wall degrading enzyme production by Argentinean isolates of Macrophomina phaseolina, the causative agent of charcoal rot in corn. Rev. Argent. Microbiol. 48, 267–273. doi: 10.1016/j.ram.2016.06.002
Rugkong, A., Rose, J. K. C., Lee, S. J., Giovannoni, J. J., O’Neill, M. A., and Watkins, C. B. (2010). Cell wall metabolism in cold-stored tomato fruit. Postharvest Biol. Technol. 57, 106–113. doi: 10.1016/j.postharvbio.2010.03.004
Sun, J. Z., Lin, H. T., Zhang, S., Lin, Y. F., Wang, H., Lin, M. S., et al. (2018). The roles of ROS production-scavenging system in Lasiodiplodia theobromae (Pat.) Griff. & Maubl.-induced pericarp browning and disease development of harvested longan fruit. Food Chem. 247, 16–22. doi: 10.1016/j.foodchem.2017.12.017
Tian, C. M., Zhao, P., and Cao, Z. M. (2009). Role of cell wall degrading enzymes in the interaction of poplar and Melampsora larici-populina Kleb. Front. For. China 4:111. doi: 10.1007/s11461-009-0006-6
Vorwerk, S., Somerville, S., and Somerville, C. (2004). The role of plant cell wall polysaccharide composition in disease resistance. Trends Plant Sci. 4, 203–209. doi: 10.1016/j.tplants.2004.02.005
Wang, L., Jin, P., Wang, J., Jiang, L. L., Shan, T. M., and Zheng, Y. H. (2015). Effect of β-aminobutyric acid on cell wall modification and senescence in sweet cherry during storage at 20°C. Food Chem. 175, 471–477. doi: 10.1016/j.foodchem.2014.12.011
Wei, J. M., Ma, F. W., Shi, S. G., Qi, X. D., Zhu, X. Q., and Yuan, J. W. (2010). Changes and postharvest regulation of activity and gene expression of enzymes related to cell wall degradation in ripening apple fruit. Postharvest Biol. Technol. 56, 147–154. doi: 10.1016/j.postharvbio.2009.12.003
Yao, H. J., and Tian, S. P. (2005). Effects of pre- and post-harvest application of salicylic acid or methyl jasmonate on inducing disease resistance of sweet cherry fruit in storage. Postharvest Biol. Technol. 35, 253–262. doi: 10.1016/j.postharvbio.2004.09.001
Zhang, S., Lin, H. T., Lin, Y. F., Lin, Y. X., Hung, Y. C., Chen, Y. H., et al. (2017). Energy status regulates disease development and respiratory metabolism of Lasiodiplodia theobromae (Pat.) Griff. & Maubl.-infected longan fruit. Food Chem. 231, 238–246. doi: 10.1016/j.foodchem.2017.03.132
Zhang, S., Lin, Y. Z., Lin, H. T., Lin, Y. X., Chen, Y. H., Wang, H., et al. (2018). Lasiodiplodia theobromae (Pat.) Griff. & Maubl.-induced disease development and pericarp browning of harvested longan fruit in association with membrane lipids metabolism. Food Chem. 244, 93–101. doi: 10.1016/j.foodchem.2017.10.020
Zhou, R., Li, Y. F., Yan, L. P., and Xie, J. (2011). Effect of edible coatings on enzymes, cell-membrane integrity, and cell-wall constituents in relation to brittleness and firmness of Huanghua pears (Pyrus pyrifolia Nakai, cv. Huanghua) during storage. Food Chem. 124, 569–575. doi: 10.1016/j.foodchem.2010.06.075
Keywords: longan (Dimocarpus longan Lour.), Phomopsis longanae Chi, disease development, cell wall components, cell wall-degrading enzymes, cell wall disassembly
Citation: Chen Y, Zhang S, Lin H, Sun J, Lin Y, Wang H, Lin M and Shi J (2018) Phomopsis longanae Chi-Induced Changes in Activities of Cell Wall-Degrading Enzymes and Contents of Cell Wall Components in Pericarp of Harvested Longan Fruit and Its Relation to Disease Development. Front. Microbiol. 9:1051. doi: 10.3389/fmicb.2018.01051
Received: 31 March 2018; Accepted: 03 May 2018;
Published: 23 May 2018.
Edited by:
Nengguo Tao, Xiangtan University, ChinaReviewed by:
Jia Liu, Chongqing University of Arts and Sciences, ChinaCopyright © 2018 Chen, Zhang, Lin, Sun, Lin, Wang, Lin and Shi. This is an openaccess article distributed under the terms of the Creative Commons Attribution License (CC BY). The use, distribution or reproduction in other forums is permitted, provided the original author(s) and the copyright owner are credited and that the original publication in this journal is cited, in accordance with accepted academic practice. No use, distribution or reproduction is permitted which does not comply with these terms.
*Correspondence: Hetong Lin, aGV0b25nbGluQDEyNi5jb20=; aGV0b25nbGluQDE2My5jb20=
Disclaimer: All claims expressed in this article are solely those of the authors and do not necessarily represent those of their affiliated organizations, or those of the publisher, the editors and the reviewers. Any product that may be evaluated in this article or claim that may be made by its manufacturer is not guaranteed or endorsed by the publisher.
Research integrity at Frontiers
Learn more about the work of our research integrity team to safeguard the quality of each article we publish.