- 1Department of Biosciences, COMSATS Institute of Information Technology, Islamabad, Pakistan
- 2Cancer Research, Institute of Biomedical and Genetic Engineering, Islamabad, Pakistan
- 3Al-Sayed Hospital, Rawalpindi, Pakistan
- 4Department of Microbiology, Quaid-i-Azam University, Islamabad, Pakistan
- 5PARC Institute for Advanced Studies in Agriculture (PIASA), National Agricultural Research Centre (NARC), Islamabad, Pakistan
- 6Industrial Biotechnology Division, National Institute of Biotechnology and Genetic Engineering, Faisalabad, Pakistan
Natural antimicrobial agents, particularly essential oils present an excellent alternative to current antibiotics due to their potent and broad-spectrum antimicrobial potential, unique mechanisms of action and low tendency to induce resistance. However their potential as a viable therapeutic alternative is greatly compromised due to their hydrophobic and volatile nature. The objective of the current research was to explore the anti-pathogenic potential of essential oils in a bio-based nano-carrier system. Six different essential oils were tested on multidrug-resistant bacterial pathogens. However, cardamom oil was selected for nano-encapsulation because of most potent anti-microbial activity. Cardamom oil loaded chitosan nano-particles were prepared by ionic gelation method with an encapsulation efficiency of more than 90% and size was estimated to be 50–100 nm. The Zeta potential was more than +50 mV that indicate a stable nano-dispersion. Cytotoxicity analysis indicated non haemolytic and non-cytotoxic behaviour on human corneal epithelial cells and HepG2 cell lines. Cardamom oil loaded chitosan nano-particles were found to exhibit excellent anti-microbial potential against extended spectrum β lactamase producing Escherichia coli and methicillin resistant Staphylococcus aureus. Our results suggested safety and efficacy of cardamom oil loaded chitosan nano-particles for treating multidrug-resistant pathogens hence offer an effective alternative to current antibiotic therapy.
Introduction
Community associated or hospital-acquired infectious diseases resulting from multidrug-resistant pathogens are considered leading cause of morbidity and mortality, especially in developing countries (Laxminarayan et al., 2013). The injudicious use of antibiotics is the single most important factor leading to antibiotic resistance around the world (Centres for Disease Control, and Prevention [CDC], 2013). Center for Disease Control (CDC) has endorsed the need for development of new antibiotics. However, the development of new antibiotics is expensive and time consuming. Meanwhile the genetic plasticity in pathogens results in development of resistance at a rapid rate (Centres for Disease Control, and Prevention [CDC], 2013; Lee et al., 2013). To tackle this issue there is a dire need to search for safe alternative therapies (Jamil et al., 2015).
Natural products have garnered the attention of many researchers in recent years as complementary and alternative antimicrobial agents (Jeon et al., 2011; Calo et al., 2015). Essential oils (EOs) are natural plant products comprised of complex mixtures of biologically active substances and offer potential novel template molecules and bioactive compounds (Maida et al., 2014). EOs are composed of volatile secondary metabolites having antibacterial, antifungal, anti-inflammatory, antioxidant, anticancer, and antiviral activities (Espina et al., 2011; Hossain et al., 2014; Aumeeruddy-Elalfi et al., 2015; Sharifi-Rad et al., 2015). The efficiency of the EOs depends on its chemical composition, genotypes, environmental, and agronomic conditions (Mohamed et al., 2014). Recent studies have highlighted the antimicrobial potential of EOs with few reports against multidrug-resistant bacteria (Kon and Rai, 2012; Coelho and Pereira, 2013; Nazzaro et al., 2013). Furthermore, the use of EOs as food preservatives has also been described by many to control food pathogens (Djenane et al., 2013; Perricone et al., 2015). In the pharmaceutical field, EOs have been included in the composition of many dosage forms to impart flavor and natural preservation (Mohamed et al., 2014).
Despite the excellent antimicrobial activity of EOs against pathogenic microorganisms, their utilization is very limited due to low water solubility and less stability to environmental factors including heat, moisture, and oxygen. To improve water dispersion and protect EOs from degradation, nano-sized formulations emerge as a viable solution (São Pedro et al., 2009; Donsì et al., 2011; Acevedo-Fani et al., 2015; El Asbahani et al., 2015). Moreover, nano-encapsulation improves the antibacterial activity of several antibiotics (El Asbahani et al., 2015; Jamil et al., 2016).
Chitosan (CS) has proven to be a cost effective carrier for many pharmaceutical agents (Piras et al., 2015). Major characteristics that make it an ideal carrier system are its biodegradability, biocompatibility, availability, safety, cationic charge and innate antimicrobial potential. The present work has been done to investigate the microscopic, physico-chemical and cytotoxic attributes of cardamom EOs functionalized engineered CS nanoparticles (CSNPs). Not yet reported cardamom EO potential at nano-scale to treat multidrug-resistant pathogens was also explored which may provide a bio-based alternative to overcome existing therapeutic challenges posed by multidrug-resistant pathogens.
Materials and Methods
Cardamom, Lemon, rose, peppermint, eucalyptus, and orange EOs were purchased from local market. CS medium molecular weight was purchased from Sigma–Aldrich (Product number 448877, 75–85% deacetylation, 200–800 cP viscosity of 1% w/v in 1% v/v acetic acid). Pentasodium tripolyphospate (TPP) and glacial acetic acid were also procured from Sigma–Aldrich. All antibiotic disks were obtained from Oxoid.
Microbial Culture Collection
All microbial cultures were collected from Al-Sayed Hospital, Rawalpindi and they were identified and characterized by standard biochemical tests and by using Analytical Profile Index (API) kit. These pathogens were preserved in glycerol broth and kept at -20°C.
Both Gram positive [Methicillin-resistant S. aureus (MRSA)] and Gram negative (E. coli) microbes were employed in the study. Resistance pattern of selected microbes was performed by Kirby Bauer disk diffusion method. Following antibiotic disks were used, Ceftazidime (CAZ 30 μg), Cefazolin (KZ 30 μg), Cefotaxime (CTX 30 μg), Imipenem (IPM), Cefepime (FEP), Cefoxitin (FOX 30 μg) Ceftriaxone (CRO 30 μg), Aztreonam (ATM 30 μg), Ampicillin (AMP 25 μg), Vancomycin (VA 30 μg), Meropenem (MEM 10 μg), and Augmentin (AMC 30 μg) and zones of inhibitions were compared with CLSI guidelines (CLSI, 2016; EUCAST, 2016).
EO Screening for Anti-bacterial Activity
Screening of EOs to compare their antimicrobial potential was performed by agar well diffusion method. Briefly, inoculum was prepared in normal saline from pure culture and turbidity was compared to that of 0.5% McFarland solution. With the help of sterilized cotton bud microbial lawn was prepared on agar surface in all directions by rotating the plate at 90°angle. Wells were prepared on inoculated agar surface with the help of sterilized borer. Different EOs were poured in each well (50 μL). Plates were then incubated at 37°C for overnight incubation. Next day zones of inhibitions were measured. EO with the best antimicrobial activity was chosen for nano-encapsulation.
Determination of Minimum Inhibitory Concentration (MIC)
Minimum inhibitory concentration of cardamom oil was carried out on both solid (by agar well diffusion method) and liquid media (broth dilution method). Cardamom oil was first serially diluted in different ratios with distilled water. Each dilution was poured in wells prepared on inoculated agar Petri plate. It was then incubated at 37°C. Next day highest dilution displaying zone of inhibition was marked and recorded as MIC.
Broth microdilution method was performed according to the protocol mentioned by Duarte et al. (2015) with some modifications. Briefly, 9 mL nutrient broth was sterilized in test tubes and each test tube was inoculated with 10 μL inoculum after matching turbidity with turbidity standard. Then 1 mL of each of cardamom oil dilution was added in each inoculated test tube. All test tubes were then incubated at 37°C for 18 h. After incubation highest dilution giving visibly clear broth was marked as MIC. Both positive and negative controls were also added. Negative control had only the nutrient broth and positive control had the inoculated broth (Duarte et al., 2015).
Preparation of CSNPs
Chitosan nano-particles were prepared using ionic gelation process as described earlier (Piras et al., 2015; Jamil et al., 2016). For this purpose, two solutions were prepared. CS and tripolyphosphate (TPP) solution.
Chitosan solution was prepared by adding 0.3 g CS in 1% acetic acid solution (100 mL). It was subjected to continuous stirring until a clear solution was obtained. Whereas TPP solution was prepared by adding 0.1 g TPP in water (10 mL). TPP solution (4 mL) was added drop wise to the CS solution (100 mL) with continuous stirring. Nano-particles (NPs) formed spontaneously upon addition of TPP aqueous solution. The mixture was stirred at room temperature for 2 more hours. The NPs were then subjected to extensive ultrasonication (Cole-parmer ultrasonic processor) at 35 Hz for at least 30 min on ice bath to avoid temperature raise. It was subjected to centrifugation at 14000 g for 30 min at 4°C (Eppendorf 5804R Benchtop Centrifuge). For the preparation of cardamom oil loaded CSNPs, 4 mL of the cardamom oil was taken and mixed with 4 mL of TPP solution.
Characterization of NPs
Scanning Electron Microscopy (SEM)
Scanning electron microscopy is a method for high-resolution imaging of surfaces. SEM analysis was done by using Jeol JSM 6490A analytical scanning electron microscope. Sample was prepared on a glass slide (1 × 1 cm) after washing it with ethanol. A tiny drop of nanodispersion was spreaded evenly over glass slide and allowed to air dry. In order to make it conductive, gold coating with Jeol Quick Auto Coater was performed (JFC-1500). The NPs were then subjected to SEM analysis under ambient conditions.
3D Structure Evaluation: Atomic Force Microscopy (AFM)
While SEM) provides 2 dimensional (2D) images of sample, AFM studies were performed to get the 3 dimensional (3D) images. AFM Imaging was carried out by using Scanning Probe Microscope (Model: SPM 9600 Company: Shimadzu) in tap mode. Samples were prepared in a similar fashion as for SEM analysis without any further sample treatment.
Interaction and Bonding Analysis of EOs with NPs
Fourier transform infrared spectroscopy (FTIR) studies were conducted to get information about the interaction pattern and release of EOs from nano-scaffolds. For an effective drug delivery system, it is necessary that the active agent must be released slowly from the carrier moiety. If the interaction between carrier and drug is strong, drug will not be released. FTIR was conducted using Bruker-Tensor 27 Fourier transform spectrometer (Bruker Daltonik GmbH, Bremen, Germany) in order to observe the existing and newly formed functional groups between CS and EOs.
Determination of Zeta Potential
Nanoparticles have the natural tendency to form agglomerates due to van der Walls attractive forces dominating over repulsive forces between the particles. Zeta potential is the charge on diffused aqueous layer formed on NP surface as it is kept in water. Zeta potential was measured through Malvern zeta sizer (NanoZS) at room temperature.
Determination of Encapsulation Efficiency
The quantity of EO loading in CSNPs was determined by the method of Abreu with slight modifications (Abreu et al., 2012). λmax was calculated for various dilutions of cardamom oil by using nanophotometer (Implen). At 295 nm (λmax), a clear peak was observed. Based upon readings of various dilutions, standard curve was constituted. The trend line was obtained by linear regression with standard equation (Y = 0.7979X - 1.0021) and R2 value (0.9848). Drug loaded CSNPs were isolated from the free drug by centrifugation (16,000 g, 25 min, Eppendorf 5415D, Eppendorf, Germany) and supernatant was quantified by spectrophotometer at λmax (295 nm). The encapsulation efficiency (EE) of cardamom EO loaded CSNPs was determined using the following equation:
Cytotoxicity Studies of NPs
These tests were done to assess the safety of NPs for in vivo application (Dehn et al., 2004).
In vitro Hemolysis Analysis
Whole blood was collected in acid-citrate-dextrose (ACD) Vacutainer. Blood sample was mixed with the NPs suspension in 1% concentration and incubated at 37°C for 45 min. Unexposed samples (negative control), distilled H2O (vehicle control) and 1% SDS (positive control) were included as experimental controls. Samples were centrifuged at 14000 rpm for 5 min and absorbance of the supernatant was measured at 540 nm using Nanodrop2000 (Dobrovolskaia et al., 2008). Percentage hemolysis was calculated relative to the untreated control.
Cytotoxicity Screening on Human Hepatocellular Carcinoma Cell Line
Human hepatocellular carcinoma cell line HepG2 (ATCC HB-8065TM) was sub-cultured and maintained in DMEM supplemented with fetal bovine serum (FBS) 10%, Na-pyruvate (1 mM), L-glutamine (2 mM), penicillin (100 U/mL) and streptomycin (100 μg/mL) at 37°C, and 5% CO2 under humidified conditions.
Potential cytotoxic effects of nanoparticles were determined via sulforhodamine B (SRB) assay as described previously (Skehan et al., 1990). In short, HepG2 cultures (10000 cells/well) were exposed to the cardamom oil loaded nanoparticles and incubated for 24 h at 37°C and 5% CO2. As negative control, untreated cultures were also included in each experiment. At the end of the incubation time, cultures were fixed by adding 50% pre-chilled trichloroacetic acid (TCA) and further incubated at 4°C for 1 hour. The samples were thoroghly washed with deionized water and air dried. Cells were then stained with SRB solution (0.4%) for 30 min and washed with acetic acid (1%) and dried overnight. Dried samples were photographed with a Olympus IMT-2 inverted microscope equipped with digital camera.
Cytotoxicity Screening on Human Corneal Epithelial Cells
Human corneal epithelial (HCEC; RIKEN Bio Resource Center, Japan) cells were maintained in a mixture (ratio 1:1) of Ham’s F12 and Dulbecco’s Modified Eagle Medium (DMEM), supplemented with FBS (10%), L-glutamine (2 mM), Na-pyruvate (1 mM), penicillin–streptomycin (100 U/ml) at 37°C in a 5% CO2 incubator, under humidified environment. Cell harvesting was done by trypsin/EDTA (0.5 mM) at room temperature.
To analyze the toxic effects of the nanoparticles, pre-seeded HCEC cells (>90% viability; 15,000 cells/well) were exposed to the nanoparticles at 100 μg/ml for 24 h. Unexposed samples and non-cellular background (culture media only and media + NPs only) were included as experimental controls. Cell viability was assessed using MTT [3-(4,5-Dimethylthiazol-2-yl)-2,5-Diphenyltetrazolium Bromide] assay as described earlier (Mosmann, 1983). Briefly, at the end of incubation period, MTT reagent (0.5 mg/ml) was added and further incubated for 3 h at 37°C. The resulting Formazan crystals were dissolved by the addition of an equal amount of acidified 10% SDS for overnight. Absorbance was taken at 565 nm by using microplate reader (AMP PLATOS R-496) and percentage viabilities of the samples were determined relative to unexposed sample using following formula:
where Abs (565) test sample and Abs (565) unexposed represent the optical density (OD) values of exposed and unexposed samples, respectively. Abs (565) media + NPs only and Abs (565) media are representatives of background OD measured for ‘media + NPs only’ and ‘media only’ samples.
Kinetics of Bacterial Inactivation
Antibacterial activity of antibiotic loaded CSNPs was determined through standard micro-dilution broth assays. Bacterial turbidity of E. coli and Methicillin-resistant S. aureus inoculated in nutrient broth was compared to Mc-farland standards after 24 h of incubation. Ten microliters of the bacterial suspensions were added to 9 mL of sterilized broth and identical concentrations of antibiotic and nano-antibiotic formulations were added in inoculated broth and all test tubes were incubated in shaker incubator at 37°C. After 24 h OD values were measured at 595 nm on ELISA Multiplate reader. Experiment was run in triplicate and both positive and negative controls were added.
Results
Resistance Spectra of Selected Pathogens
In the present investigation, E. coli and S. aureus were used to determine antimicrobial efficacy of our Cardamom oil-loaded CSNPs. Both microbes were subjected to disk diffusion method to determine their resistance pattern (Table 1). S. aureus was resistant to FOX disk that is why it was called MRSA. While the E. coli was found to be extended spectrum β lactamase (ESBL) producer, as zone augmentation was observed between AMC disk and other cephalosporin disks. Both of the pathogens were observed to be multidrug resistant.
EO Screening and MIC Determination
Essential oils were subjected to agar well diffusion assay to determine efficacy of selected EOs against multidrug-resistant pathogens (Table 2). It was observed that cardamom oil was most active against both MRSA and ESBL producing E. coli so it was selected for encapsulation in NPs. Cardamom oil emulsion was prepared in various dilutions with distilled water. MIC was calculated to be 25 % (Percent v/v) for E. coli and (10 %) (Percent v/v) for MRSA.
Characterization of NPs
Chitosan NPs were prepared by ionic gelation method and then subjected to various characterization techniques to ascertain their size and shape. SEM (Figure 1) and AFM (Figure 2) analysis depicted CSNPs size to be 50–100 nm with quite uniformity in both size and shape. More than 90% encapsulation efficiency of cardamom oil loaded CSNPs was observed.
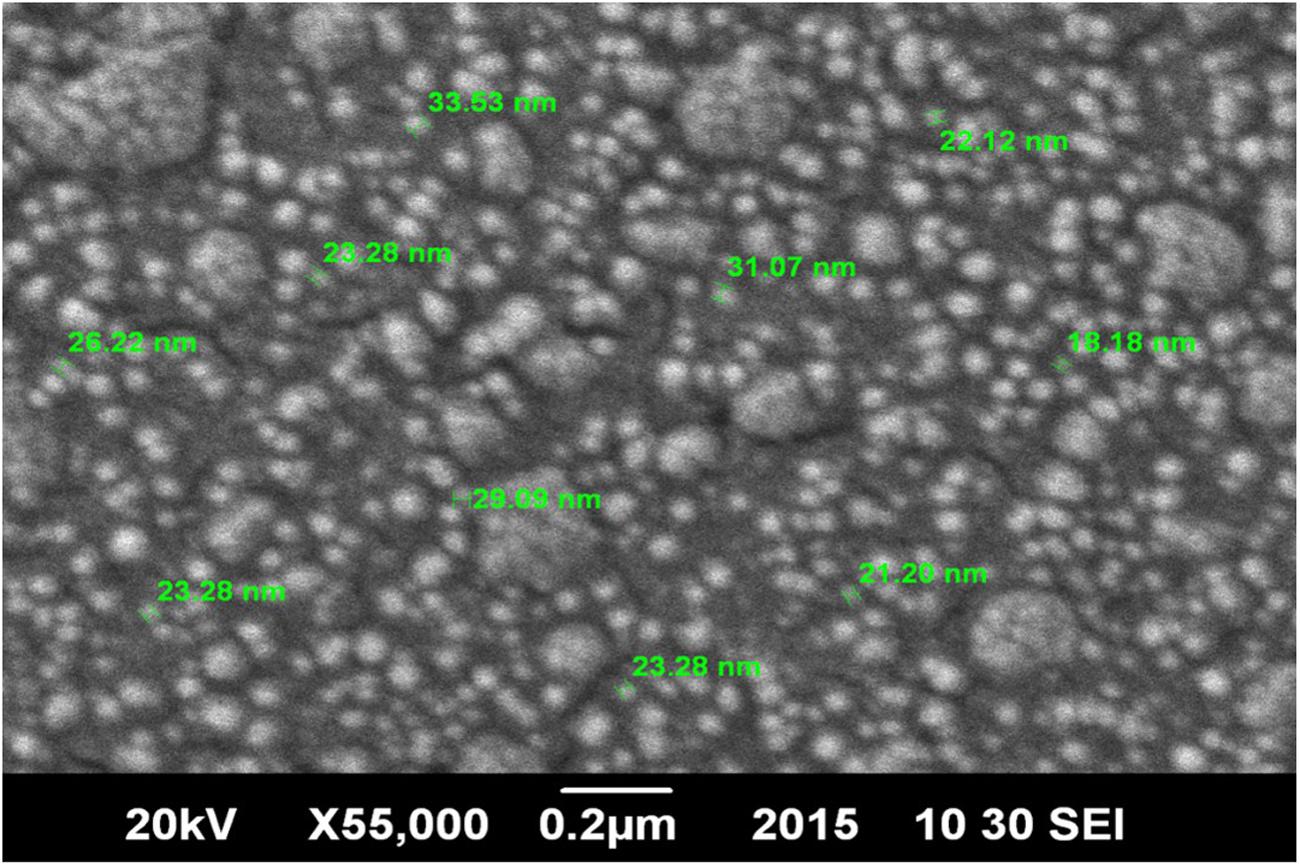
FIGURE 1. Scanning Electron Microscope (SEM) micrographs. Morphological evaluation of chitosan nano-particles (CSNPs) by scanning electron microscope (SEM) micrographs at 55000×.
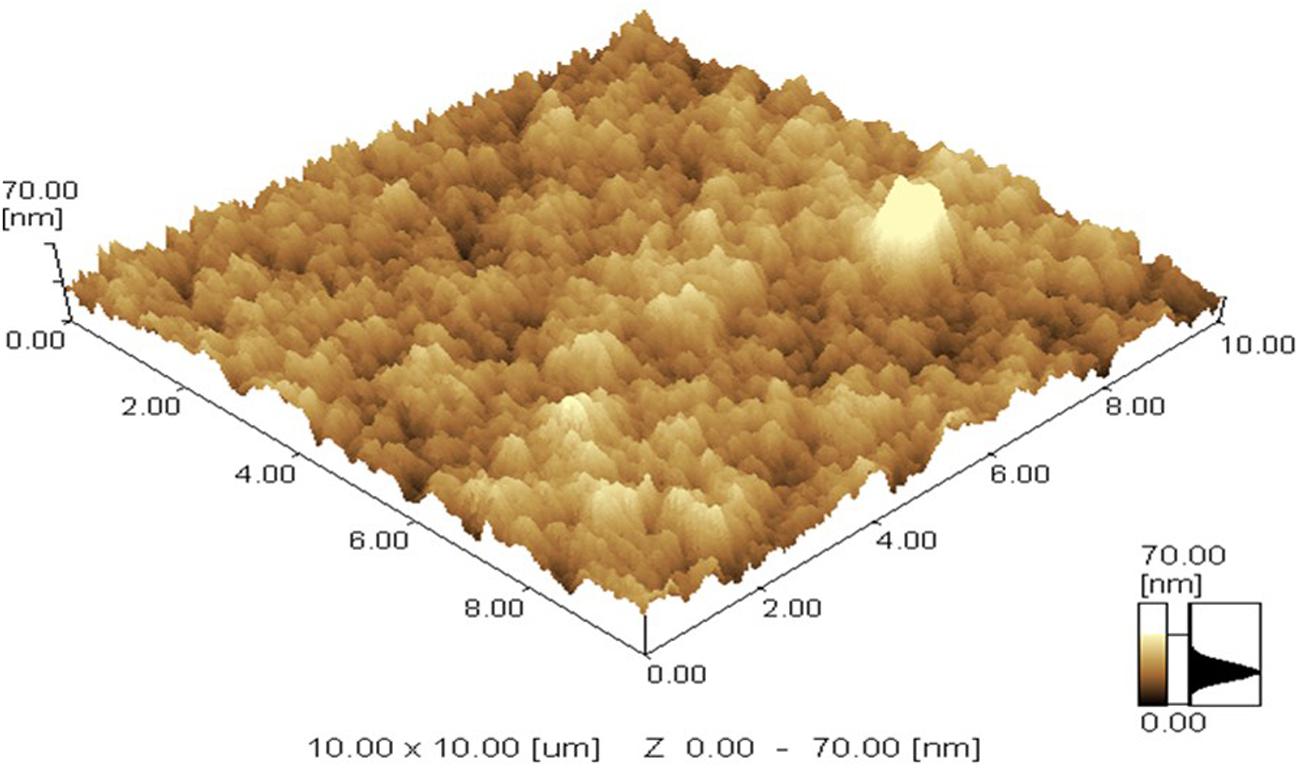
FIGURE 2. Atomic Force Microscope (AFM) image. AFM micrograph of CSNPs was taken at room temperature. Maximum height of 70 nm was observed.
Interaction Studies of Cardamom Oil with CSNPs
Fourier transform infrared spectroscopy spectra of CS powder shows characteristic peaks at 3433 cm-1 (OH and NH2 stretching), 2920 cm-1 (CH stretching), 1647 cm-1 (amide I), 1088 cm-1 (C–O–C stretching; Figure 3A). Pure cardamom oil spectra displays sharp characteristic peaks at 3331 cm-1 (OH stretching), 2971 cm-1 (OH stretching), 1710 cm-1 (C = O stretch representing aldehyde or ketones), 1458 cm-1 (CH2 bending), 1377 cm-1 (CH bending), and 1333 cm-1 (C–N stretching; Figure 3B). For empty CSNPs (Figure 3C), the amide I (NH2 bending) peak shifted from 1647 to 1641 cm-1, and new peaks appeared at 1280 cm-1 (C–N stretch) implying the complex formation via electrostatic interaction between NH3+ groups of CS and phosphoric groups of TPP within the nanoparticles. All the above characteristic peaks also appear in the spectra of cardamom oil loaded CSNPs at almost the same wave number indicating no modification or interaction between the EO and CSNPs (Figure 3D).
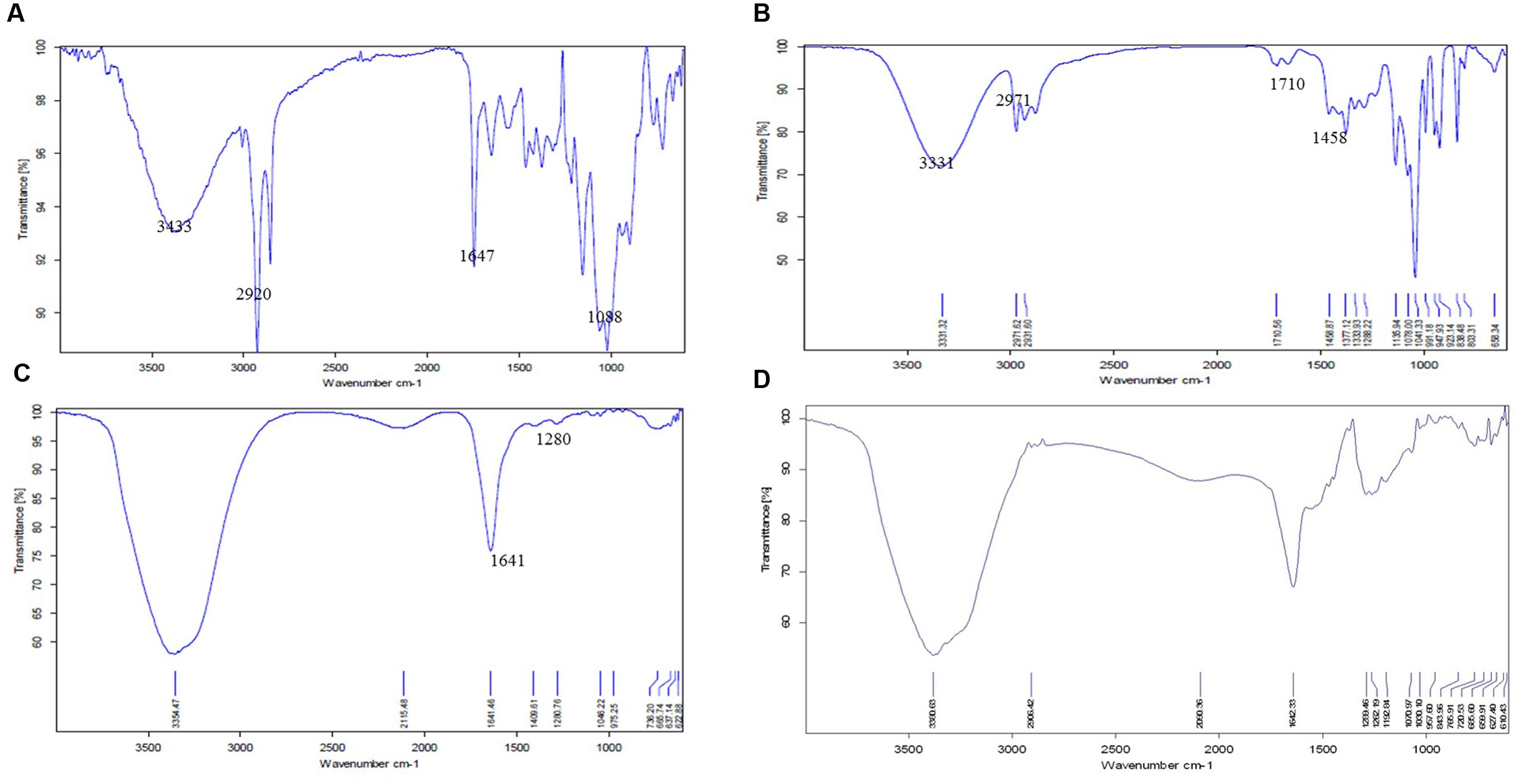
FIGURE 3. Fourier transform infrared spectroscopic (FTIR) spectra of (A) CS raw material, (B) cardamom oil raw material, (C) blank CSNPs, and (D) cardamom oil-loaded CSNPs. From these images it can be observed that the FTIR image of CSNPs and EOs loaded CSNPs are almost superimposable, indicating no chemical reaction between CS and EOs. The entrapment of EOs is mere physical.
Stability of Cardamom Oil with CSNPs
Zeta potential studies predicted long term stability as the zeta potential values were more than 50 mV in both empty and cardamom oil-loaded CSNPs (Figure 4).
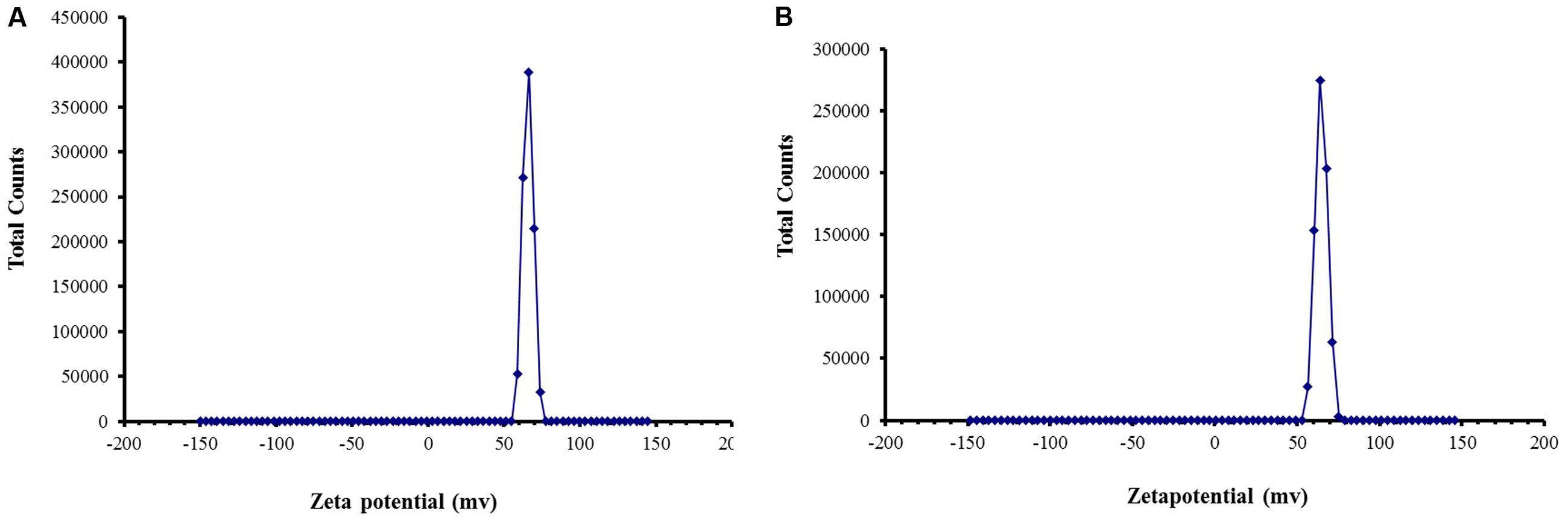
FIGURE 4. Zeta Potential of (A) blank CSNPs and (B) cardamom oil-loaded CSNPs. Zeta potential was measured through Malvern Instrument. A positive zeta potential of more than 50 mV was observed for both blank and EOs loaded CSNPs.
Cytotoxicity Studies
Potential cytotoxic effects of the cardamom oil loaded CSNPs was performed via hemolysis and potential to induce morphological alterations in mammalian cells. Both empty and EOs-loaded CSNPs did not display any hemolytic effect (Figure 5). Furthermore, no necrotic damage to monolayer cell lines or any change in cell morphology was observed (Figures 6 and 7).
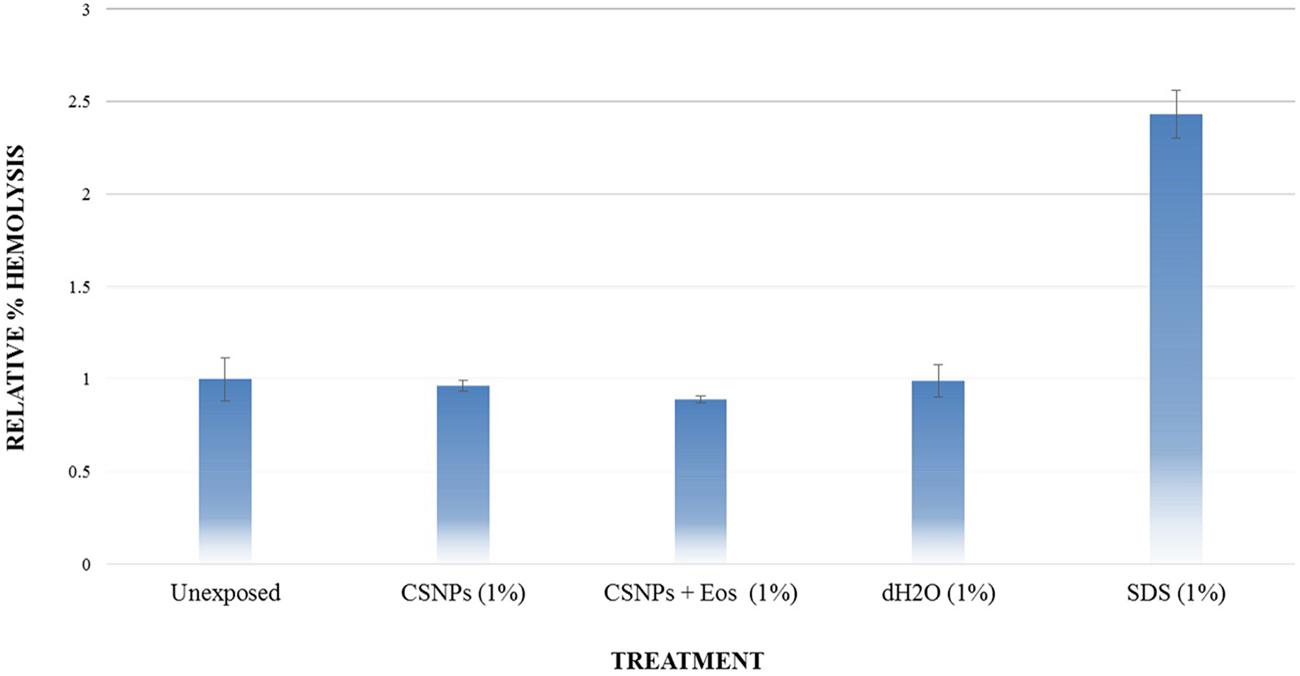
FIGURE 5. Hemolysis analysis. Evaluation of % hemolysis induced by chitosan NP and cardamom oil-loaded CSNPs. It can be observed that both empty and EOs-loaded CSNPs are not causing hemolysis.
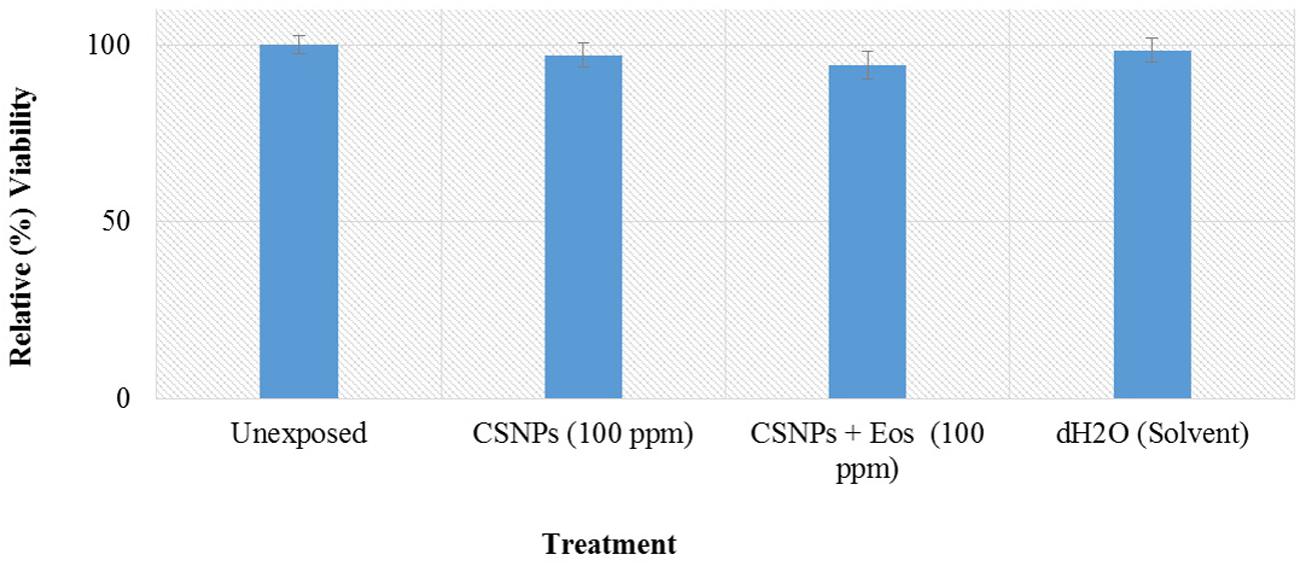
FIGURE 6. Cytotoxicity analysis on Human corneal epithelial cells. Growth and survival of Human corneal epithelial cells was assessed by MTT [3-(4,5-Dimethylthiazol-2-yl)-2,5-Diphenyltetrazolium Bromide] assay.
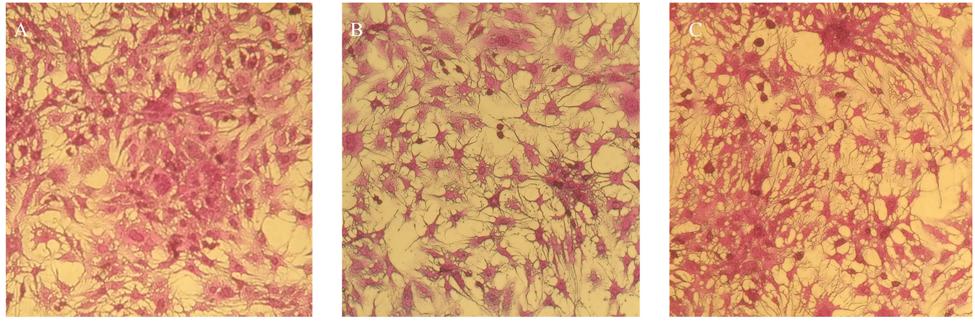
FIGURE 7. Cytotoxicity analysis on HepG2 cancerous cell line (A) untreated HepG2 cells and (B) blank CSNPs (C) essential oil-loaded CSNPs. Cytotoxicity testing was done to check the safety of NPs on human cell. It can be observed that both the blank and EOs loaded chitosan NPs were not causing any cell damage.
Antimicrobial Activity of Cardamom Oil-Loaded CSNPs
According to the results of the current study, cardamom oil alone failed to inhibit both pathogens because cardamom oil was incorporated below MIC (Figure 8). Both empty CSNPs and EOs-loaded CSNPs effectively controlled the growth of pathogens for first 48 h. However, after 48 h growth appeared with empty CSNPs whereas the EOs-loaded CSNPs maintained their anti-microbial potential till 7 days (Figure 8).
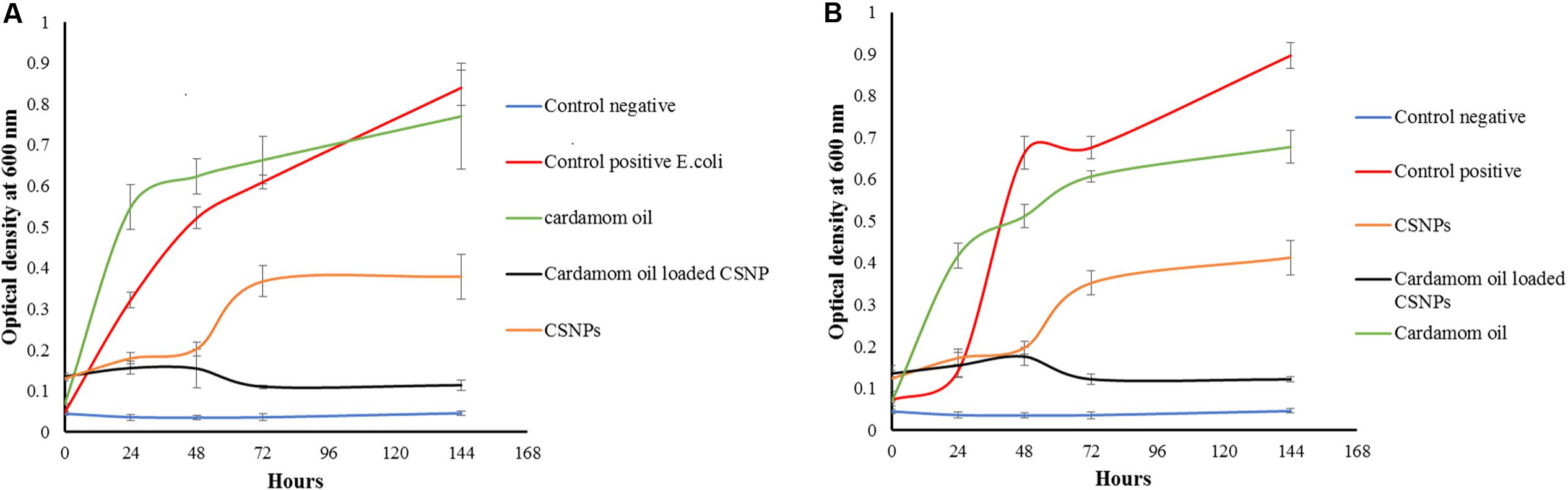
FIGURE 8. Growth kinetics of (A) Escherichia coli (B) Methicillin-resistant Staphylococcus aureus. It can be observed that the cardamom oil-loaded CSNPs have completely eradicated both pathogens whereas, bare CSNPs initially controlled the pathogens for 2 days after that a rapid growth was observed.
Discussion
Essential oils of plant origin are used both domestically and industrially. According to an estimate more than 3000 EOs have been produced by different plants. However, almost 300 are important from the commercial point of view. EO market all over the world is estimated to be 700 million US$ (Raut and Karuppayil, 2014).
In the present study we encapsulated EOs in CSNPs and then their antimicrobial potential on multidrug-resistant S. aureus and E. coli was determined. The S. aureus was confirmed to be MRSA because it was exhibiting resistance against cefoxitin disk. The proportion of S. aureus isolates that are resistant to methicillin is increasing rapidly. Previously, it was considered as nosocomial pathogen. However, infections caused by oxacillin (methicillin)-resistant S. aureus (MRSA) are a problem not only in health care institutions but also in community as well (Klevens et al., 2006). E. coli used in the study was ESBL positive. The prevalence of (ESBL) positive E. coli is also reported to be very high. According to a study it was estimated that almost 70–90% of E. coli population is ESBL positive (Kumarasamy et al., 2010). ESBL positive pathogens are considered to be resistant to whole cephalosporin range and the only safe treatment for curing ESBL positive pathogens is carbapenem and now the pathogens have developed resistance against carbapenem as well. So the clinicians are left with no safe antibiotics and there is a dire need to search for new antibiotics against which microbes cannot develop resistance rapidly.
Elettaria cardamomum (cardamom, family; Scitaminaceae) is a perennial herb, indigenous to India, Pakistan, Burma, and Sri Lanka (Khan et al., 2011). In addition to its wide use for culinary purpose, cardamom has folkloric repute as carminative, stomachic, diuretic, antibacterial, antiviral, antifungal and is considered useful in treatment of constipation, colic, diarrhoea, dyspepsia, vomiting, headache, epilepsy, and cardiovascular diseases (Gilani et al., 2008). Besides cardamom oil, the antimicrobial properties of several other EOs against a number of pathogenic microorganisms involved in foodborne illness have been demonstrated in previous investigations (Roby et al., 2013; Zhang et al., 2016).
Previous studies have reported MIC of cardamom EO at 0.4% against S. aureus ATCC 6538 and 0.8% against E. coli ATCC 8739 (Gochev et al., 2012). However, in the present study we tested the EOs against multi drug resistant pathogens that is why higher MIC values were achieved as compare to previous reports.
Numerous studies have reported an enhancement of the physical and antimicrobial properties of EOs-loaded nano-emulsions as compared to their conventional emulsions (Buranasuksombat et al., 2011; Liang et al., 2012; Salvia-Trujillo et al., 2014; Severino et al., 2015). The encapsulation of eugenol and carvacrol into nanometric surfactant micelles also resulted in enhanced antimicrobial activity. However, not yet reported previously, cardamom oil was encapsulated in CSNPs to protect it and to achieve antimicrobial activity at low dose below MIC value against MDR pathogens.
Hosseini et al. (2013) reported oregano loaded CSNPs by incorporating Tween, however, in the present study, cardamom oil-loaded CSNPs were prepared directly by ionic gelation method after mixing EO with TPP. It was observed after SEM (Figure 1) and AFM (Figure 2) analysis that NPs were in the range of 50–100 nm and they were uniformly distributed. CS is a very popular material as nano-carrier and has been widely studied by numerous authors. However, varying sizes in the range of 40–500 nm have been reported previously (Abreu et al., 2012; Hosseini et al., 2013).
While studying the interaction of cardamom oil with CS scaffolds, the materials were subjected to FTIR analysis. CS powder shows characteristic peaks that were in accordance to previously reported spectra (Khan et al., 2002; Vitali et al., 2006). The results of FTIR indicated that cardamom oil might be encapsulated into the CSNPs. Evidence of EOs and CS interaction was in good agreement with previous literature (Abreu et al., 2012). The CS and EOs-loaded CSNPs were subjected to stability testing by means of zeta potential analysis. Zeta potential studies predicted long term stability as the zeta potential values were more than + 60 mV in both empty and cardamom oil loaded CSNPs (Figure 4). Results of this study were in good accordance to the previous data (Calvo et al., 1997; Raja et al., 2013; Chang et al., 2015). A charge of more than + 30 mV is consider as an indicator of stability as the mutual repulsive forces would be more as compare to van der walls attractive forces between the NPs. In addition to stability the positive charge on both empty and loaded CSNPs indicated good interaction with pathogens as well. Bacterial cell wall carries negative charge and our developed NPs are carrying positive charge so there is a prediction of very strong interaction.
Our results of cytotoxicity were also found to be in accordance with the results of De Campos, where it was observed that both HCEC and HepG2 cell lines after CSNPs treatment yielded 100% cells viability (De Campos et al., 2004). Hemolysis or destruction of red blood cells is a very significant aspect to be considered for in vivo applications of all pharmaceutical agents as it can lead to anemia, jaundice and other pathological conditions. CSNPs were found to be absolutely non-hemolytic. Our results were found to be in good accordance to the findings of Zhou et al. (2015).
The antimicrobial potential of medicinal plants is well documented. Donsì investigated the nano-encapsulation of EO and has reported enhanced antimicrobial effect against multiple pathogens causing food spoilage (Donsì et al., 2011; Aumeeruddy-Elalfi et al., 2015). Moreover, Liang also reported enhanced and prolonged antimicrobial activity of EOs after nano-encapsulation (Liang et al., 2012). However, this is the first report on nano-encapsulation of cardamom oil in CSNPs. According to our findings cardamom oil loaded CSNPs were highly effective in combating both ESBL-producing E. coli and MRSA. Whereas, cardamom oil alone failed to eradicate them. However, the CSNP inhibited the growth of pathogens till first 48 h after that there was a rapid increase in optical density of both pathogens.
The positive charge due to the presence of –NH+3 have been mentioned as important factors that influence the antibacterial activities of CS (Chang et al., 2015). The strong adhesive properties of CS with bacteria are due to these opposite charges. However, to maintain the antimicrobial potential of CS, it must be incorporated with some antimicrobial agents and naturally occurring antimicrobials are the best choice for this purpose. It serves dual purpose, protecting the EOs from degradation for prolonged antimicrobial efficacy and sustaining the innate inhibitory effect of CS to inhibit resistant superbugs.
Conclusion
Cardamom EO-loaded CS nano-capsules were observed to be highly effective in controlling multi drug resistant E. coli and MRSA in vitro without showing any signs of toxicity to human cells.
Author Contributions
BJ performed all research work and compiled data and wrote manuscript. RA performed cytotoxicity analysis and helped in manuscript write up. SA helped in collecting and identifying pathogens. MI helped for FTIR analysis. SK helped in studying growth kinetics studies on ELISA multi plate reader. AI helped in doing zeta potential analysis. SJ provided technical assistance in experimentation and write up. HB also provided technical assistance in experimentation and manuscript write up and MI∗ provided technical assistance in experimentation and compilation of data and manuscript write up.
Funding
Funding for this study was provided by internal department.
Conflict of Interest Statement
The authors declare that the research was conducted in the absence of any commercial or financial relationships that could be construed as a potential conflict of interest.
References
Abreu, F. O., Oliveira, E. F., Paula, H. C., and de Paula, R. C. (2012). Chitosan/cashew gum nanogels for essential oil encapsulation. Carbohydr. Polym. 89, 1277–1282. doi: 10.1016/j.carbpol.2012.04.048
Acevedo-Fani, A., Salvia-Trujillo, L., Rojas-Graü, M. A., and Martín-Belloso, O. (2015). Edible films from essential-oil-loaded nanoemulsions: physicochemical characterization and antimicrobial properties. Food Hydrocoll. 47, 168–177. doi: 10.1016/j.foodhyd.2015.01.032
Aumeeruddy-Elalfi, Z., Gurib-Fakim, A., and Mahomoodally, F. (2015). Antimicrobial, antibiotic potentiating activity and phytochemical profile of essential oils from exotic and endemic medicinal plants of Mauritius. Ind. Crops Prod. 71, 197–204. doi: 10.1016/j.indcrop.2015.03.058
Buranasuksombat, U., Kwon, Y. J., Turner, M., and Bhandari, B. (2011). Influence of emulsion droplet size on antimicrobial properties. Food Sci. Biotechnol. 20, 793–800. doi: 10.1007/s10068-011-0110-x
Calo, J. R., Crandall, P. G., O’Bryan, C. A., and Ricke, S. C. (2015). Essential oils as antimicrobials in food systems–a review. Food Control 54, 111–119. doi: 10.1016/j.foodcont.2014.12.040
Calvo, P., Remunan-Lopez, C., Vila-Jato, J. L., and Alonso, M. J. (1997). Novel hydrophilic chitosan-polyethylene oxide nanoparticles as protein carriers. J. Appl. Polym. Sci. 63, 125–132. doi: 10.1002/(SICI)1097-4628(19970103)63:1<125::AID-APP13>3.0.CO;2-4
Centres for Disease Control, and Prevention [CDC] (2013). Antibiotic resistance threats in the United States, 2013. Atlanta, GA: Centres for Disease Control, and Prevention.
Chang, S. H., Lin, H. T. V., Wu, G. J., and Tsai, G. J. (2015). pH Effects on solubility, zeta potential, and correlation between antibacterial activity and molecular weight of chitosan. Carbohydr. Polym. 134, 74–81. doi: 10.1016/j.carbpol.2015.07.072
CLSI (2016). Performances Standards for Antimicrobial Susceptibility Testing; Twenty-Fourth Informational Supplement. M100-S26. Wayne, PA: Clinical Laboratory Standards Institute.
Coelho, F. A. B. L., and Pereira, M. O. (2013). “Exploring new treatment strategies for Pseudomonas aeruginosa biofilm infections based on plant essential oils,” in Microbial Pathogens and Strategies for Combating Them: Science, Technology and Education, Vol. 1, ed. A. Mendez-Vilas (Badajoz: Formatex).
De Campos, A. M., Diebold, Y., Carvalho, E. L., Sánchez, A., and Alonso, M. J. (2004). Chitosan nanoparticles as new ocular drug delivery systems: in vitro stability, in vivo fate, and cellular toxicity. Pharm. Res. 21, 803–810. doi: 10.1023/B:PHAM.0000026432.75781.cb
Dehn, P. F., White, C. M., Conners, D. E., Shipkey, G., and Cumbo, T. A. (2004). Characterization of the human hepatocellular carcinoma (hepg2) cell line as an in vitro model for cadmium toxicity studies. In Vitro Cell. Dev. Biol. Anim. 40, 172–182. doi: 10.3109/15376516.2012.714006
Djenane, D., Yangüela, J., Roncalés, P., and Aider, M. (2013). Use of essential oils as natural food preservatives: effect on the growth of Salmonella enteritidis in liquid whole eggs stored under abuse refrigerated conditions. J. Food Res. 2, 65–78. doi: 10.5539/jfr.v2n3p65
Dobrovolskaia, M. A., Clogston, J. D., Neun, B. W., Hall, J. B., Patri, A. K., and McNeil, S. E. (2008). Method for analysis of nanoparticle hemolytic properties in vitro. Nano lett. 8, 2180–2187. doi: 10.1021/nl0805615
Donsì, F., Annunziata, M., Sessa, M., and Ferrari, G. (2011). Nanoencapsulation of essential oils to enhance their antimicrobial activity in foods. LWT Food Sci. Technol. 44, 1908–1914. doi: 10.1016/j.lwt.2011.03.003
Duarte, A., Martinhoa, A., Luís,Â, Figueirasa, A., Oleastro, M., and Domingues, F. C. (2015). Resveratrol encapsulation with methyl-β-cyclodextrin for antibacterial and antioxidant delivery applications. LWT Food Sci. Technol. 63, 1254–1260. doi: 10.1016/j.lwt.2015.04.004
El Asbahani, A., Miladi, K., Badri, W., Sala, M., Addi, E. A., Casabianca, H., et al. (2015). Essential oils: from extraction to encapsulation. Int. J. Pharm. 483, 220–243. doi: 10.1016/j.ijpharm.2014.12.069
Espina, L., Somolinos, M., Lorán, S., Conchello, P., García, D., and Pagán, R. (2011). Chemical composition of commercial citrus fruit essential oils and evaluation of their antimicrobial activity acting alone or in combined processes. Food control 22, 896–902. doi: 10.1016/j.foodcont.2010.11.021
EUCAST (2016). European Committee on Antimicrobial Susceptibility Testing. Breakpoint tables for interpretation of MICs and zone diameters Version 6.0. Available at: www.eucast.org
Gilani, A. H., Jabeen, Q., Khan, A. U., and Shah, A. J. (2008). Gut modulatory, blood pressure lowering, diuretic and sedative activities of cardamom. J. Ethnopharmacol. 115, 463–472. doi: 10.1016/j.jep.2007.10.015
Gochev, V., Girova, T., Stoilova, I., Atanasova, T., Nenov, N., Stanchev, V., et al. (2012). Low temperature extraction of essential oil bearing plants by liquefied gases. 7. Seeds from cardamom (Elettaria cardamomum (L.) Maton). J. BioSci. Biotechnol 1, 135–139.
Hossain, M. A., Harbi, S. R. A., Weli, A. M., Al-Riyami, Q., and Al-Sabahi, J. N. (2014). Comparison of chemical constituents and antimicrobial activities of three essential oils from three different brands’ clove samples collected from Gulf region. Asian Pac. J. Trop. Dis. 4, 262–268. doi: 10.1016/S2222-1808(14)60570-3
Hosseini, S. F., Zandi, M., Rezaei, M., and Farahmandghavi, F. (2013). Two-step method for encapsulation of oregano essential oil in chitosan nanoparticles: preparation, characterization and in vitro release study. Carbohydr. Polym. 95, 50–56. doi: 10.1016/j.carbpol.2013.02.031
Jamil, B., Bokhari, H., and Imran, M. (2015). Mechanism of action: how nano-antimicrobials act? Curr. Drug Targets doi: 10.2174/1389450116666151019101826 [Epub ahead of print].
Jamil, B., Habib, H., Abbasi, S., Nasir, H., Rahman, A., Rehman, A., et al. (2016). Cefazolin loaded chitosan nanoparticles to cure multi drug resistant Gram-negative pathogens. Carbohydr. Polym. 136, 682–691. doi: 10.1016/j.carbpol.2015.09.078
Jeon, J. G., Rosalen, P. L., Falsetta, M. L., and Koo, H. (2011). Natural products in caries research: current (limited) knowledge, challenges and future perspective. Caries Res. 45, 243–263. doi: 10.1159/000327250
Khan, A. U., Khan, Q. J., and Gilani, A. H. (2011). Pharmacological basis for the medicinal use of cardamom in asthma. Bangladesh J. Pharmacol. 6, 34–37. doi: 10.1038/sj.bjp.0706437
Khan, T. A., Peh, K. K., and Ch’ng, H. S. (2002). Reporting degree of deacetylation values of chitosan: the influence of analytical methods. J. Pharm. Pharm. Sci. 5, 205–212.
Klevens, R. M., Edwards, J. R., Tenover, F. C., McDonald, L. C., Horan, T., Gaynes, R., et al. (2006). Changes in the epidemiology of methicillin-resistant Staphylococcus aureus in intensive care units in US hospitals, 1992–2003. Clin. Infect. Dis. 42, 389–391. doi: 10.1086/499367
Kon, K. V., and Rai, M. K. (2012). Plant essential oils and their constituents in coping with multidrug-resistant bacteria. Expert Rev. Anti Infect. Ther. 10, 775–790. doi: 10.1586/eri.12.57
Kumarasamy, K. K., Toleman, M. A., Walsh, T. R., Bagaria, J., Butt, F., Balakrishnan, R., et al. (2010). Emergence of a new antibiotic resistance mechanism in India, Pakistan, and the UK: a molecular, biological, and epidemiological study. Lancet Infect. Dis. 10, 597–602. doi: 10.1016/S1473-3099(10)70143-2
Laxminarayan, R., Duse, A., Wattal, C., Zaidi, A. K., Wertheim, H. F., Sumpradit, N., et al. (2013). Antibiotic resistance—the need for global solutions. Lancet Infect. Dis. 13, 1057–1098. doi: 10.1016/S1473-3099(13)70318-9
Lee, C. R., Cho, I. H., Jeong, B. C., and Lee, S. H. (2013). Strategies to minimize antibiotic resistance. Int. J. Environ. Res. Public Health 10, 4274–4305. doi: 10.3390/ijerph10094274
Liang, R., Xu, S., Shoemaker, C. F., Li, Y., Zhong, F., and Huang, Q. (2012). Physical and antimicrobial properties of peppermint oil nanoemulsions. J. Agric. Food Chem. 60, 7548–7555. doi: 10.1021/jf301129k
Maida, I., Lo Nostro, A., Pesavento, G., Barnabei, M., Calonico, C., Perrin, E., et al. (2014). Exploring the anti-Burkholderia cepacia complex activity of essential oils: a preliminary analysis. Evid. Based Complement. Alternat. Med. 2014:573518. doi: 10.1155/2014/573518
Mohamed, A. A., Ali, S. I., El-Baz, F. K., Hegazy, A. K., and Kord, M. A. (2014). Chemical composition of essential oil and in vitro antioxidant and antimicrobial activities of crude extracts of Commiphora myrrha resin. Ind. Crops Prod. 57, 10–16. doi: 10.1016/j.indcrop.2014.03.017
Mosmann, T. (1983). Rapid colorimetric assay for cellular growth and survival: application to proliferation and cytotoxicity assays. J. Immunol. Methods 65, 55–63. doi: 10.1016/0022-1759(83)90303-4
Nazzaro, F., Fratianni, F., De Martino, L., Coppola, R., and De Feo, V. (2013). Effect of essential oils on pathogenic bacteria. Pharmaceuticals 6, 1451–1474. doi: 10.3390/ph6121451
Perricone, M., Arace, E., Corbo, M. R., Sinigaglia, M., and Bevilacqua, A. (2015). Bioactivity of essential oils: a review on their interaction with food components. Front. Microbiol. 6:76. doi: 10.3389/fmicb.2015.00076
Piras, A. M., Maisetta, G., Sandreschi, S., Gazzarri, M., Bartoli, C., Grassi, L., et al. (2015). Chitosan nanoparticles loaded with the antimicrobial peptide temporin B exert a long-term antibacterial activity in vitro against clinical isolates of Staphylococcus epidermidis. Front. Microbiol. 6:372. doi: 10.3389/fmicb.2015.00372
Raja, M. A. G., Katas, H., Hamid, Z. A., and Razali, N. A. (2013). Physicochemical properties and in vitro cytotoxicity studies of chitosan as a potential carrier for dicer-substrate siRNA. J. Nanomater. 2013:653892. doi: 10.1155/2013/653892
Raut, J. S., and Karuppayil, S. M. (2014). A status review on the medicinal properties of essential oils. Ind. Crops Prod. 62, 250–264. doi: 10.1016/j.indcrop.2014.05.055
Roby, M. H. H., Sarhan, M. A., Selim, K. A. H., and Khalel, K. I. (2013). Antioxidant and antimicrobial activities of essential oil and extracts of fennel (Foeniculum vulgare L.) and chamomile (Matricaria chamomilla L.). Ind. Crops Prod. 44, 437–445. doi: 10.1016/j.indcrop.2012.10.012
Salvia-Trujillo, L., Rojas-Graü, M. A., Soliva-Fortuny, R., and Martín-Belloso, O. (2014). Impact of microfluidization or ultrasound processing on the antimicrobial activity against Escherichia coli of lemongrass oil-loaded nanoemulsions. Food Control 37, 292–297. doi: 10.1016/j.foodcont.2013.09.015
São Pedro, A., Cabral-Albuquerque, E., Ferreira, D., and Sarmento, B. (2009). Chitosan: an option for development of essential oil delivery systems for oral cavity care? Carbohydr. Polym. 76, 501–508. doi: 10.1016/j.carbpol.2008.12.016
Severino, R., Ferrari, G., Vu, K. D., Donsì, F., Salmieri, S., and Lacroix, M. (2015). Antimicrobial effects of modified chitosan based coating containing nanoemulsion of essential oils, modified atmosphere packaging and gamma irradiation against Escherichia coli O157: H7 and Salmonella Typhimurium on green beans. Food Control 50, 215–222. doi: 10.1016/j.foodcont.2014.08.029
Sharifi-Rad, J., Hoseini-Alfatemi, S. M., Sharifi-Rad, M., and Da Silva, J. A. T. (2015). Antibacterial, antioxidant, antifungal and anti-inflammatory activities of crude extract from Nitraria schoberi fruits. 3 Biotech 5, 677–684. doi: 10.1007/s13205-014-0266-1
Skehan, P., Storeng, R., Scudiero, D., Monks, A., McMahon, J., Vistica, D., et al. (1990). New colorimetric cytotoxicity assay for anticancer-drug screening. J. Natl. Cancer Inst. 82, 1107–1112. doi: 10.1093/jnci/82.13.1107
Vitali, L., Justi, K. C., Laranjeira, M., and Fávere, V. T. (2006). Impregnation of chelating agent 3, 3-bis-N, N bis-(carboxymethyl) aminomethyl-o-cresolsulfonephthalein in biopolymer chitosan: adsorption equilibrium of Cu (II) in aqueous medium. Polímeros 16, 116–122. doi: 10.1590/S0104-14282006000200011
Zhang, Y., Liu, X., Wang, Y., Jiang, P., and Quek, S. (2016). Antibacterial activity and mechanism of cinnamon essential oil against Escherichia coli and Staphylococcus aureus. Food Control 59, 282–289. doi: 10.1016/j.foodcont.2015.05.032
Keywords: natural active agents, cardamom essential-oil, multidrug resistance, chitosan nanocapsules, MRSA, cytotoxicity
Citation: Jamil B, Abbasi R, Abbasi S, Imran M, Khan SU, Ihsan A, Javed S, Bokhari H and Imran M (2016) Encapsulation of Cardamom Essential Oil in Chitosan Nano-composites: In-vitro Efficacy on Antibiotic-Resistant Bacterial Pathogens and Cytotoxicity Studies. Front. Microbiol. 7:1580. doi: 10.3389/fmicb.2016.01580
Received: 27 May 2016; Accepted: 21 September 2016;
Published: 04 October 2016.
Edited by:
Octavio Luiz Franco, Universidade Católica de Brasília, BrazilReviewed by:
Juliane Viana, Universidade Ceuma, BrazilPramod Kumar, National Center for Disease Control and Public Health, India
Copyright © 2016 Jamil, Abbasi, Abbasi, Imran, Khan, Ihsan, Javed, Bokhari and Imran. This is an open-access article distributed under the terms of the Creative Commons Attribution License (CC BY). The use, distribution or reproduction in other forums is permitted, provided the original author(s) or licensor are credited and that the original publication in this journal is cited, in accordance with accepted academic practice. No use, distribution or reproduction is permitted which does not comply with these terms.
*Correspondence: Muhammad Imran, m.imran@comsats.edu.pk Bushra Jamil, bushrajamil2000@gmail.com