- 1Department of Advanced Biomedical Sciences, Medical School, “Federico II” University of Naples, Naples, Italy
- 2Department of Histopathology, Imperial College London, Hammersmith Hospital, London, United Kingdom
Superoxide dismutase is widespread in the human body, including skin and its appendages. Here, we focus on human skin copper/zinc superoxide dismutase, the enzyme that protects skin and its appendages against reactive oxygen species. Human skin copper/zinc superoxide dismutase resides in the cytoplasm of keratinocytes, where up to 90% of cellular reactive oxygen species is produced. Factors other than cell type, such as gender, age and diseased state influence its location in skin tissues. We review current knowledge of skin copper/zinc superoxide dismutase including recent studies in an attempt to contribute to solving the question of its remaining unexplained functions. The research described here may be applicable to pathologies associated with oxidative stress. However, recent studies on copper/zinc superoxide dismutase in yeast reveal that its predominant function may be in signaling pathways rather than in scavenging superoxide ions. If confirmed in the skin, novel approaches might be developed to unravel the enzyme's remaining mysteries.
Introduction
Skin envelops the entire surface of human body and is daily exposed to environmental insults such as pathogens, injuries and ultraviolet (UV) radiation. Its important functions include regulation of body temperature, defense, and sensation as well as production of vitamin D. One of the skin's several defense mechanisms against environmental insults involves its structural organization: both skin layers, epidermis and dermis, contain cells, enzymes and other substances that play a critical role in defense. Several enzyme families contribute specifically to skin defense by scavenging pathogen-, injury- and UV radiation-derived molecules. The noxious molecules called free radicals can only be destroyed by the antioxidant activity of enzymes such as catalase, superoxide dismutase, peroxidase, and some supporting enzymes. We will focus here on human skin superoxide dismutase (SOD) highlighting, in addition to its best-known function of scavenging, further functions already shown in other models.
ROS
Free radicals have a single unpaired electron on the outer orbit. Derived from oxygen and also known as reactive oxygen species (ROS), they are generated by normal cellular aerobic respiration and are produced in mitochondria, endoplasmic reticulum, and peroxisomes, and are involved in several biochemical reactions that regulate fundamental cellular signaling pathways such as cell proliferation, apoptosis, and autophagy (1, 2). The energy created by their unstable configuration is freed through reactions with adjacent molecules, such as inorganic and organic substances, proteins, lipids and carbohydrates, and with key membrane molecules and nucleic acids (3–5). Oxygen radicals and other reactive species cause modifications in the amino acids of proteins, which frequently result in functional or structural changes of enzymatic proteins (6). They are able to induce covalent bonds with Kelch-like ECH-associated protein 1 (Keap1), a keratinocyte cytoplasmic protein that is normally linked to nuclear factor erythroid 2-related factor 2 (Nrf2), which, according to Dinkova-Kostova et al. (7), dissociates from Keap1 and transmigrates to the nucleus where it acts as a transcription factor and induces the production of antioxidant enzymes including copper/zinc SOD (Cu/Zn SOD) (Figure 1). ROS generation can also trigger autocatalytic reactions resulting in more free radicals that propagate the damage chain. These are inherently unstable and generally decay spontaneously. The superoxide anion, for example, is unstable and decays spontaneously in the presence of water to release oxygen and hydrogen peroxide (12).
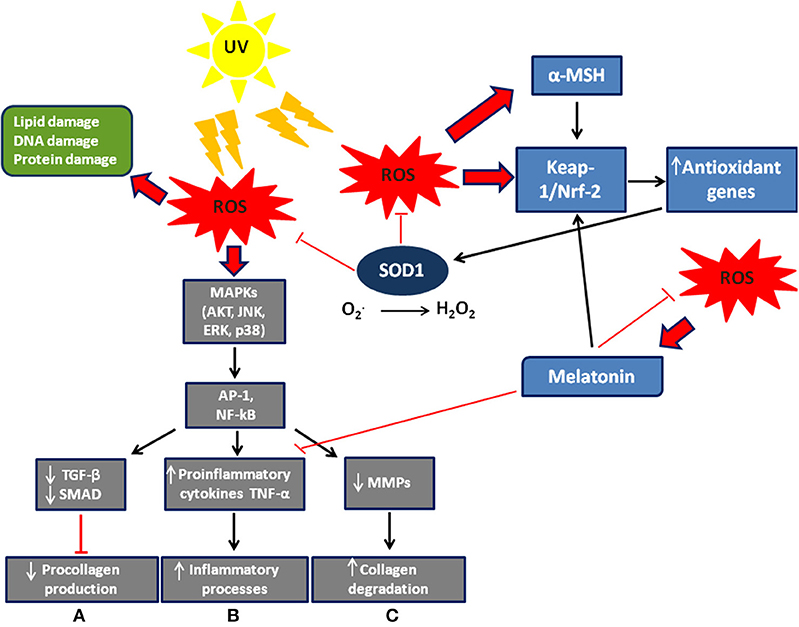
Figure 1. Scheme of ROS signaling/effects and defense system in the skin. Exogenous and endogenous agents of skin cells generate ROS, whose excessive levels can cause cell damage. ROS modulate MAP signaling pathways leading to activation of the transcription factor AP-1 (activator protein 1) and NF-kB (nuclear factor kappa-light-chain-enhancer of activated B cells). They also induce: (A) a decrease in procollagen synthesis via blocking TGF-β/SMAD signaling; (B) an increase in the inflammatory processes; (C) an increase in collagen degradation via synthesis of matrix degenerating MMPs. Furthermore, ROS allow nuclear factor Nrf2 to detach from its cytoplasmic inhibitor, keap-1, to translocate to the nucleus and activate transcription of antioxidant genes. Melatonin (8) and α-MSH (9) are also stimulated by ROS to activate Nrf2 dependent-pathways and then the expression of antioxidant genes. The Nrf2 pathway is activated not only in different skin cells such as keratinocytes and melanocytes, but also in fibroblasts (10). Modified by Sardy (11).
There are many other exogenous factors, such as pollutants and UV that can induce ROS production. Excessive ROS production can lead to skin aging and development of skin cancer (13–15). Human skin is constantly exposed to three main types of UV [(16–18)1]. However, epidermis, by means of its self-protecting cells, keratinocytes, undergoes cornification. And among the proteins that make up the thickened cell envelope, small proline rich proteins, also known as stress-inducible proteins, are expressed that protect keratinocytes from ROS (19).
Superoxide Dismutase
SOD is a highly conserved enzyme, that is abundantly expressed in the cytoplasm of aerobic organisms and plays a fundamental role in protecting cells from oxidative stress. It belongs to a family of enzymes that catalyze the dismutation of the superoxide radical (20, 21). These radicals are generated in many cellular processes (22) such as products of normal respiration and oxidative bursts from immune cells. There are various forms of SOD that incorporate different covalently bound substances (Mn, Zn, Cu, Fe) (23), and can inactivate both intra- and extra-cellular superoxides (20). Work on their subcellular localization in hepatocytes shows that Cu/Zn SOD (SOD1), which contains copper and zinc, may be found in the nuclei, cytoplasm, peroxisomes and lysosomes, but also in mitochondrial intermembrane space (24–26). Mn SOD (SOD2) contains manganese and it is predominantly found in the mitochondrial matrix (27). The third one, Cu/Zn extracellular SOD (SOD3), also contains copper and zinc and is secreted in the extracellular space (28). Superoxide radical anions are unstable molecules, commonly produced by aerobic metabolism. Oxidative stress, induced by the uncontrolled production of superoxide and its reaction products, is implicated in the development of pathologies including neurodegenerative diseases, premature aging, cancer, diabetes, and dermatitis. There is a clear increase in ROS generation of the skin after UV exposure (6). Epithelial cells and thymus-based fibroblasts can release antioxidant enzymes, especially under stress conditions (29). All mammalian cells express both mitochondrial Mn SOD and cytosolic Cu/Zn SOD, while the extra-cellular SOD high molecular weight isoform appears to be expressed only in specific cell populations (30, 31). Extra-cellular SOD was discovered by Marklund (30, 32) who showed that it is localized in the fluid and in the extra-cellular matrix of tissues; its role in cancer cells has been reviewed by Griess et al. (33) who suggested that extra-cellular SOD increase generated by ROS might work as a cancer suppressor (33). Immunohistochemistry has shown that EC SOD is also present in connective tissue. It is absent, however, in the thymus, stomach and skeletal muscle (34). Although it has been observed that extra-cellular SOD protects the extracellular space and the endothelial cell surface, its activity is low (32). These observations suggest that the enzyme can act both by a paracrine mechanism and on remote cells (35, 36). The hydrogen peroxide generated by SOD activity acts as an inhibitor of the enzyme. In this case the superoxide radical is not neutralized and inhibits the enzymes involved in subsequent reactions (e.g., catalase) (37–39).
Cu/Zn SOD is a stable 15.9 kDa homodimer. The dimerization is held by hydrophobic contacts (40) that reduce solvent accessibility and increase its stability (41). Each monomer contains two metals, a copper ion and a zinc ion, which together have either a structural or catalytic function. Initially Cu/Zn SOD was detected in the cytosol (24), in the outer membrane and/or in the intermembranous space between mitochondria and peroxisomes, which generate superoxide radicals (42). In fibroblasts, Cu/Zn SOD appears to be localized in peroxisomes (43). An important novel function of Cu/Zn SOD has been reported recently in yeasts and humans. In response to oxidative stress, high levels of H2O2 promote Cu/Zn SOD nuclear translocation and as a transcription factor the enzyme regulates the expression of oxidative resistance and repair genes (44). In addition, in yeasts, only a small amount of Cu/Zn SOD was shown to scavenge superoxides while the majority of Cu/Zn SOD mediated peroxide signaling (45). There are as yet few similar investigations on vertebrate cells including human; it would therefore be of great interest to confirm the data obtained in Saccharomyces cerevisiae. Actually, it has been reported that 25% of genes related to human degenerative pathologies overlap almost completely with those of yeast pathologies, thus allowing the study of homologous antioxidant response genes in much simpler eukaryotic organisms (46).
Cu/Zn SOD and Skin
In a recently published immunocytochemical study Altobelli et al. localized Cu/Zn-SOD in human skin in several conditions (Figures 2A–F). Some studies show that during the processes of natural aging and photo-aging the activity of SOD does not change in the skin, while that of catalase is increased in the epidermis and considerably reduced in the dermis (48). The activity of antioxidant enzymes seems to vary among cell types; for instance, fibroblasts have high levels of catalase, glutathione peroxidase and SOD compared to keratinocytes (43). In the skin, environmental direct contact-induced ROS production can cause aging, skin diseases, and cancer. Photo-aging depends on the degree of exposure and the type of skin. For example, individuals who live in warm environments are more exposed to light and are consequently prone to photo-aging (49). However, according to Hellemans (50), the seasonal activity of SOD in the stratum corneum does not seem to vary.
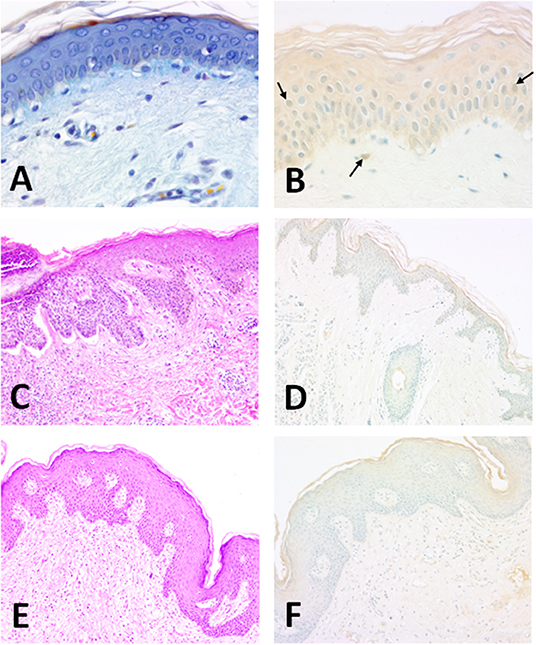
Figure 2. Structure of the normal human epidermis (man, aged 25; forearm skin). (A) Section of human skin showing the different layers of the epidermis. OPA trichrome staining (47). 40 x magnification; (B) The enzyme is clearly present in epithelial keratinocytes. Horseradish peroxidase development. Methyl green counterstaining allows a better identification of SOD1-immunoreactive nuclei (arrows). Specimens are subsequently evaluated by histo-densitometry of Cu/Zn SOD immunoreactive areas. 10x magnification. Illustration of BCC (man, aged 71; back, scapular skin). (C) Structure of the epidermis showing a nodular lesion in the dermis. Hematoxylin-Eosin. 10x magnification; (D) Densitometric measurements of Cu/Zn SOD for basal carcinomas were made at the level of the surface epidermis and in the tumor areas invaginated in the dermis. Faint staining of epidermis. Horseradish peroxidase development. Methyl green counterstaining. 10x magnification. Illustration of SCC (woman, aged 65; back, scapular skin). (E) Structure of the SCC-affected skin. Hematoxylin-Eosin. 10x magnification; (F) Densitometric measurements of Cu/Zn SOD in SCC-affected human skin were made at the level of the surface epidermis and in the tumor areas invaginated in the dermis. Epidermal staining is weaker than normal tissue. Horseradish peroxidase development. Methyl green counterstaining. 10x magnification.
UV rays induce the synthesis of matrix metalloproteinases (MMP) in the skin, leading to the destruction of collagen (51) (Figure 1). The greatest effect is observed in photo-aging, where there is a greater activity of MMP and an increase in the accumulation of hydrogen peroxide, due to reduced dermal catalase. The accumulation of hydrogen peroxide changes the activity of mitogen-activated protein (MAP) kinases involved in the synthesis of pro-collagen and consequently induces aging (50, 52–54). In vitro studies of the effects of SOD on cellular antioxidant metabolism have shown its relationship with MMP and its consequent regulation of extracellular matrix degradation (55). These data were later confirmed in a study in vivo on a pig model. Clinical results of radiation-induced fibrosis have provided some evidence that Cu/Zn SOD could become an anti-fibrotic drug, its therapeutic effect relating to down-regulation of transforming growth factor beta 1 (TGF-beta1). In fact, SOD significantly reduces the expression of TGF-beta1 while an increase of TGF-beta1 expression is associated with fibrotic diseases (56).
Direct murine skin-exposure to various oxidative stresses requires a high antioxidant capacity to maintain a low oxidant balance (57). Measurements of enzymes and antioxidant substances present in human skin showed that the activity of SOD was higher in the epidermis than the dermis in both young and aging skin (48). These results were then confirmed by histo-densitometry and showed a reverse relationship between human cutaneous Cu/Zn SOD and aging, as well as a higher level in the male than the female (58). In addition, exposed skin expressed more of the enzyme than non-exposed skin (Figure 2B). Interestingly, it has been proved that carbazole induces ROS production in the human keratinocyte cell line, HaCaT. Carbazole is an aromatic heterocyclic compound so called because it contains one or more aromatic rings in its structure. It has been shown that when carbazole is present in human skin, for example in tattoo ink, prolonged exposure to sunlight resulting in photosensitized carbazole causes downregulation of antioxidant genes (hmox-1, keap-1, nrf-2, and bcl2) in HaCaT cells and of course an increase of ROS, with consequent apoptotic cell death (59). In vitro experiments with HaCaT (59, 60) provide important information on the physiology of SOD and oxidative stress-linked skin diseases. HaCaT cell lines have also been used to unravel an unexpected relationship of the NO/NOS system with Cu/Zn SOD. NO is thought to up-regulate Cu/Zn SOD expression that in turn might inhibit the mechanism of keratinocyte proliferation (61).
Thus, SOD protects human keratinocytes from UV-induced damage that includes aging caused by fragmentation of collagen and elastic fibers and activation of metalloproteases (62). A comprehensive review of mainly plant-derived natural antioxidants for human skin has been produced by Dunaway et al. (63): it has been reported that antioxidants such as green tea, vitamin C, vitamin E, CoQ10 and hydroxytyrosol could reduce the effect of UV radiation (64–69). In human skin, a single exposure to UV rays involves a transient reduction of SOD activity; however, after chronic UVB irradiation, activity of epidermal SOD is induced (6). Studies on mouse skin have shown that UVB lowers the level of Cu/Zn SOD, while Mn SOD is influenced by UVA. Seasonal alternation, however, does not cause a variation in SOD concentration in either exposed or non-exposed skin (50).
It seems that HaCaT cell lines become apoptotic in a dose-dependent way 24 h after radiation with 150 J/m2 of UVB and only 35 J/m2 of UVC (70). These negative effects are reduced in the body thanks to the activity of antioxidant enzymes.
Basal Cell and Spinocellular Carcinomas
The skin is the organ generally most affected by tumors because it is the most exposed to environmental insults. The importance of SOD in the skin is not always clear, i.e., its expression may be influenced by cytokines (71).
The activity of SOD is variable: it is reduced in the presence of melanomas, epitheliomas, and carcinomas (72). Immunohistochemical studies have shown in fact that in carcinomas the activity of both Cu/Zn SOD and Mn SOD decreases (58, 73). EC SOD, however, is present both in the dermis and in the epidermis and its level of expression is seven times greater in the dermis (71). In another study both basal and spinous layers contained Cu/Zn SOD with a prominent expression in the upper epidermal layers (58) (Figures 2C,D). In addition, a weak intracellular distribution was observed throughout the human epidermis and especially in the stratum corneum (6).
Chronic exposure of the skin to sunlight promotes premature aging, reduces its immunological response to environmental antigens and is the main risk factor in the development of a variety of precancerous and malignant skin neoplasms due to the uncontrolled growth of keratinocytes and melanocytes (74–76). Although ultraviolet rays are responsible for tanning, the UVB component in particular can damage skin cell DNA (77). Damage to the DNA induces in turn the modulation of genes concerned with the activation of various signal transduction pathways, promoting tumor growth from initiated cells, which can grow into tumors because they divide much more rapidly than normal ones (78).
Skin tumors develop mainly in areas most exposed to the sun: face, ears, neck and scalp (79). Basal cell carcinoma (BCC) is particularly frequent and is the most common invasive skin carcinoma in humans, followed by spinocellular carcinoma (SCC). Melanoma is rarer, but better known and more dangerous. Malignant skin tumors develop relatively slowly and are largely treatable if detected early and then treated promptly (80). Immunohistochemical analysis of BCC and SCC revealed a reduction of immunoreactive Cu/Zn SOD and Mn SOD, indicating a UV-dependent depletion of antioxidant defenses; this reduction seems to be inversely proportional to the proliferation of malignant cells (81). In another study there was a noticeable increase in SOD activity at 24 h from exposure to UVB rays, with a significant increase in Cu/Zn SOD and a reduction in Mn SOD levels (82). Notably, it has been reported that melatonin has a protective action against UV radiation (UVR)-induced 8-hydroxydeoxyguanosine formation and depletion of antioxidative enzymes. The experiment used ex vivo human full-thickness skin exposed to UVR in a dose- (0, 100, 300 mJ/cm2) and time- (0, 24, 48 h post-UVR) dependent manner. Thus, melatonin plays a crucial role as a potent antioxidant and DNA protectant against UVR-induced oxidative damage in human skin (83).
Benign epithelial neoplasms are common and generally biologically harmless. These tumors, deriving from the multi-layered keratinized basal epithelium of the epidermis, hair follicles, and the ductal epithelium of the cutaneous glands, generally maintain the characteristics of their cells of origin. With regard to malignant tumors, the incidence of BCCs increases significantly in immunosuppressed patients and in those with congenital defects of DNA repair mechanisms and affects individuals over 45 years of age (84, 85). These tumors can take various forms and can affect every part of the body but most often the head. Approximately 33% patients a year develop another primary BCC. SCCs occur on light-exposed skin of elderly subjects. Except for lesions of the lower limbs, these tumors have a higher incidence among men. They are more aggressive than BCCs, expanding more rapidly and occasionally (<5%) metastasizing to nearby lymph nodes where they are generally profoundly invasive (85).
The main predisposing factor is exposure to UVR from the sun and consequent DNA damage; other factors include industrial carcinogens, chronic ulcers and draining osteomyelitis, old burn scars, ingestion of arsenical substances, ionizing radiation and (for the oral cavity) mastication of tobacco (86, 87). Sunlight, in addition to its effects on DNA, also seems to have a direct transient immunosuppressive effect on the skin, altering the normal immune-surveillance function of its Langerhans cells. SCCs, often preceded by preneoplastic lesions (actinic keratoses), are usually diagnosed and operated early when they heal in 95% of cases, otherwise they can invade the deep tissues and destroy the bone and cartilage, especially around the eyes, nose, and ear. Morphologically these epitheliomas are nodular, flat (cicatricial), sclerodermiform and pigmented, and may be ulcerated. Both BCCs and SCCs express Cu/Zn SOD enzyme mostly in the upper epidermis (Figures 2E,F). Furthermore, histo-densitomentric evaluation of these tumors has recently demonstrated increased human epidermal SOD activity with respect to dermal one, and more expression in human male skin than in female as well as more in young than old people (58).
Conclusion
Cu/Zn SOD has long been known as an enzymatic protein, but morphological studies in skin began only in the late nineteen-eighties, first in pig and then in human (88, 89). The first observations allowed the localization of two types of SOD, the Cu/Zn-type and a Mn-type. However, these initial morphological studies were aimed at understanding the relationship between SOD activity and epidermal proliferation. Then, using cultured human keratinocytes, different roles were established for Cu/Zn SOD and Mn SOD. Cu/Zn SOD activity increases after irradiation as a reaction to ROS-generated oxidative stress, therefore acquiring an antioxidant function (89). Furthermore, it was suggested that cytokines IL-1 alpha and TNF-alpha produced by keratinocytes could mediate SOD recovery after the defense reaction. During the past decade understanding has changed, as described above: many other Cu/Zn SOD functions have been proposed; for example, the role of transcription factor and, with the help of the yeast, S. cerevisiae, and HaCaT cells, the function of oxidative signaling, which predominate over that of scavenging. Thus, Cu/Zn SOD crosses the nuclear membrane to join promoters and activate transcription of determinate signaling pathways. In fact, Cu/Zn SOD-containing cells show immune-positive nuclei as well as cytoplasm. It remains to be clarified why nuclear labeling by immunogold doesn't occur in Kobayashi's skin preparations (89). In another investigation, Cu/Zn SOD nuclear translocation was shown in yeast and human fibroblast (44). Additional evidence for the anti-oxidant role of this enzyme comes from work on skin atrophy and delayed wound healing in SOD-deficient mice which can be reversed with various anti-oxidants, such as syringaresinol, vitamin C derivatives and Palladium/Platinum nanoparticle mixtures (90–93). It would be useful if all these data could be confirmed in the human epidermis. Skin is a very complex biochemical laboratory. Many aspects of antioxidant enzymes have been discovered during the past 5 years but there are more to be elucidated before the definitive functions of Cu/Zn SOD and other antioxidant enzymes in human skin are fully understood.
Author Contributions
VC and GA: conceptualization and design of the study. VC: drafting of original manuscript. VC, GA, and AB: literature reviewing. SV: editing and revising manuscript critically for intellectual content. GA, SV, AB, and VC: final approval of the manuscript as it has been submitted.
Conflict of Interest
The authors declare that the research was conducted in the absence of any commercial or financial relationships that could be construed as a potential conflict of interest.
Acknowledgments
Thanks to of Drs. Giorgia Chalkiadaki and Marita Georgia Riccardi for their assistance during their apprenticeships for BSc degrees.
Footnotes
References
1. Di Meo S, Reed TT, Venditti P, Victor VM. Role of ROS and RNS sources in physiological and pathological conditions. Oxid Med Cell Longev. (2016) 2016:7909186. doi: 10.1155/2016/1245049
2. He L, He T, Farrar S, Ji L, Liu T, Ma X. Antioxidants maintain cellular redox homeostasis by elimination of reactive oxygen species. Cell Physiol Biochem. (2017) 44:532–53. doi: 10.1159/000485089
3. Yla-Herttuala S. Oxidized LDL and atherogenesis. Ann N Y Acad Sci. (1999) 874:134–7. doi: 10.1111/j.1749-6632.1999.tb09231.x
4. Stadtman ER, Levine RL. Protein oxidation. Ann N Y Acad Sci. (2000) 899:191–8. doi: 10.1111/j.1749-6632.2000.tb06187.x
5. Marnett LJ. Oxyradicals and DNA damage. Carcinogenesis. (2000) 21:361–70. doi: 10.1093/carcin/21.3.361
6. Sander CS, Chang H, Salzmann S, Müller CS, Ekanayake-Mudiyanselage S, Elsner P, et al. Photoaging is associated with protein oxidation in human skin in vivo. J Invest Dermatol. (2002) 118:618–25. doi: 10.1046/j.1523-1747.2002.01708.x
7. Dinkova-Kostova AT, Holtzclaw WD, Cole RN, Itoh K, Wakabayashi N, Katoh Y, et al. Direct evidence that sulfhydryl groups of Keap1 are the sensors regulating induction of phase 2 enzymes that protect against carcinogens and oxidants. Proc Natl Acad Sci USA. (2002) 99:11908–913. doi: 10.1073/pnas.172398899
8. Janjetovic Z, Jarrett SG, Lee EF, Duprey C, Reiter RJ, Slominski AT. Melatonin and its metabolites protect human melanocytes against UVB-induced damage: involvement of NRF2-mediated pathways. Sci Rep. (2017) 7:1274–86. doi: 10.1038/s41598-017-01305-2
9. Kokot A, Metze D, Mouchet N, Galibert MD, Schiller M, Luger TA, et al. Alpha-melanocyte-stimulating hormone counteracts the suppressive effect of UVB on Nrf2 and Nrf-dependent gene expression in human skin. Endocrinology. (2009) 150:3197–106. doi: 10.1210/en.2008-1315
10. Gegotek A, Skrzydlewska E. The role of transcription factor Nrf2 in skin cells metabolism. Arch Dermatol Res. (2015) 307:385–96. doi: 10.1007/s00403-015-1554-2
11. Sárdy M. Role of matrix metalloproteinases in skin ageing. Connect Tissue Res. (2009) 50:132–38. doi: 10.1080/03008200802585622
12. Bielski BHJ, Cabelli BH, Arudi RL, Ross AB. Reactivity of RO2/O2. Radicals in aqueous solution. J Phys Chem Ref Data. (1985) 14:1041–1100. doi: 10.1063/1.555739
13. Rinnerthaler M, Bischof J, Streubel MK, Trost A, Richter K. Oxidative stress in aging human skin. Biomolecules. (2015) 5:545–89. doi: 10.3390/biom5020545
14. Narendhirakannan RT, Hannah MA. Oxidative stress and skin cancer: an overview. Indian J Clin Biochem. (2013) 28:110–5. doi: 10.1007/s12291-012-0278-8
15. Nishigori C, Hattori Y, Toyokuni S. Role of reactive oxygen species in skin carcinogenesis. Antioxid Redox Signal. (2004) 6:561–70. doi: 10.1089/152308604773934314
16. Dong PD, Munson CA, Norton W, Crosnier C, Pan X, Gong Z, et al. Fgf10 regulates hepatopancreatic ductal system patterning and differentiation. Nat Genet. (2007) 39:397–402. doi: 10.1038/ng1961
17. Stolarski R, Bojkov R, Bishop L, Zerefos C, Staehelin J, Zawodny J. Measured trends in stratospheric ozone. Science. (1992) 256:342–49. doi: 10.1126/science.256.5055.342
18. World Health Organization. Environmental Health Criteria: Ultraviolet Radiation. Geneva: WHO (1994). p. 352.
19. Vermeij WP, Backendorf C. Skin cornification proteins provide global link between ROS detoxification and cell migration during wound healing. PLoS ONE. (2010) 5:e11957. doi: 10.1371/journal.pone.0011957
20. Wang Y, Branicky R, Noë A, Hekimi S. Superoxide dismutases: dual roles in controlling ROS damage and regulating ROS signaling. J Cell Biol. (2018) 217:1915–28. doi: 10.1083/jcb.201708007
21. Tainer JA, Getzoff ED, Beem KM, Richardson JS, Richardson DC. Determination and analysis of the 2 A-structure of copper, zinc superoxide dismutase. J Mol Biol. (1982) 160:181–217.
22. Raha S, Robinson BH. Mitochondria, oxygen free radicals, disease and ageing. Trends Biochem Sci. (2000) 25:502–8. doi: 10.1016/S0968-0004(00)01674-1
23. Zelko IN, Mariani TJ, Folz RJ. Superoxide dismutase multigene family: a comparison of the CuZn-SOD (SOD1), Mn-SOD (SOD2), and EC-SOD (SOD3) gene structures, evolution, and expression. Free Radic Biol Med. (2002) 33:337–49. doi: 10.1016/s0891-5849(02)00905-x
24. Chang LY, Slot JW, Geuze HJ, Grapo JD. Molecular immunocytochemistry of the CuZn superoxide dismutase in rat hepatocytes. J Cell Biol. (1988) 107:2169–79. doi: 10.1083/jcb.107.6.2169
25. Crapo JD, Oury T, Rabouille C, Slot JW, Chang LY. Copper,zinc superoxide dismutase is primarily a cytosolic protein in human cells. Proc Natl Acad Sci USA. (1992) 89:10405–9. doi: 10.1073/pnas.89.21.10405
26. Okado-Matsumoto A, Fridovich I. Subcellular distribution of superoxide dismutases (SOD) in rat liver: Cu, Zn-SOD in mitochondria. J Biol Chem. (2001) 276:38388–93. doi: 10.1074/jbc.M105395200
27. Slot JW, Geuze HJ, Freeman BA, Grapo JD. Intracellular localization of the copper-Zinc and manganese superoxide dismutases in the liver parenchymal cells. Lab Invest. (1986) 55:363–71.
28. Fukai T, Folz RJ, Landmesser U, Harrison DG. Extracellular superoxide dismutase and cardiovascular disease. Cardiovasc Res. (2002) 55:239–49. doi: 10.1016/S0008-6363(02)00328-0
29. Cimini V, Ruggiero G, Buonomo T, Seru R, Sciorio S, Zanzi C, et al. CuZn-superoxide dismutase in human thymus: immunocytochemical localisation and secretion in thymus-derived epithelial and fibroblast cell lines. Histochem Cell Biol. (2002) 118:163–69. doi: 10.1007/s00418-002-0429-8
30. Marklund SL. Human copper containing superoxide dismutase of high molecular weight. Proc Natl Acad Sci USA. (1982) 79:7634–38. doi: 10.1073/pnas.79.24.7634
31. Tibell L, Hjalmarsson K, Edlund T, Skogman G, Engström A, Marklund SL. Expression of human extracellular superoxide dismutase in Chinese hamster ovary cells and characterization of the product. Proc Natl Acad Sci USA. (1987) 84:6634–38. doi: 10.1073/pnas.84.19.6634
32. Marklund SL. Extracellular SOD in human tissues and human cells. J Clin Invest. (1984) 74:1398–03. doi: 10.1172/JCI111550
33. Griess B, Tom E, Domann F, Teoh-Fitzgerald M. Extracellular superoxide dismutase and its role in cancer. Free Radic Biol Med. (2017) 112:464–79. doi: 10.1016/j.freeradbiomed.2017.08.013
34. Ookawara T, Imazeki N, Matsubara O, Kizaki T, Oh-Ishi S, Nakao C, et al. Tissue distribution of immunoreactive mouse extracellular superoxide dismutase. Am J Physiol. (1998) 275:C840–47. doi: 10.1152/ajpcell.1998.275.3.C840
35. Mondola P, Annela T, Santillo M. Evidence for secretion of cytosolic Cu-Zn superoxide dismutase by HEPG2 cells and human fibroblasts. Int J Biochem Cell Biol. (1996) 28:677–81. doi: 10.1016/1357-2725(96)00004-0
36. Mruk D, Cheng CH, Cheng YH, Mo MY, Grima J, Silvestrini B, et al. Rat testicular extracellular superoxide dismutase: its purification, cellular distribution, and regulation. Biol Reprod. (1998) 59:298–08. doi: 10.1095/biolreprod59.2.298
37. Hodgson EK, Fridovich I. The interaction of bovine erythrocyte superoxide dismutase with hydrogen peroxide: inactivation of the enzyme. Biochemistry. (1975) 14:5294–99. doi: 10.1021/bi00695a010
38. Hodgson EK, Fridovich I. The interaction of bovine erythrocyte superoxide dismutase with hydrogen peroxide: chemiluminescence and peroxidation. Biochemistry. (1975) 14:5299–303. doi: 10.1021/bi00695a011
40. Tainer JA, Getzoff ED, Richardson JS, Richardson DC. Structure and mechanism of copper, zinc superoxide dismutase. Nature. (1983) 306:284–87. doi: 10.1038/306284a0
41. Goodsell DS, Olson AJ. Structural symmetry and protein function. Annu Rev Biophys Biomol Struct. (2000) 29:105–53. doi: 10.1146/annurev.biophys.29.1.105
42. Yukimi K, Eisuke F, Masayasu I. Association of Cu, Zn type SOD with mitochondria and peroxisomes. Arch Biochem Biophys. (2002) 399:96–102. doi: 10.1006/abbi.2001.2738
43. Thérond P, Gerbaud P, Dimon S, Anderson WB, Evain-Broin D, Raynaudt F. Antioxidant enzymes in psoriatic fibroblasts and erythrocytes. J Invest Dermatol. (1996) 106:1325–28. doi: 10.1111/1523-1747.ep12349055
44. Tsang K, Liu Y, Thomas J, Zhang Y, Zheng XF. Superoxide dismutase 1 acts as a nuclear transcription factor to regulate oxidative stress resistance. Nat Commun. (2014) 5:3446. doi: 10.1038/ncomms4446
45. Montllor-Albalate C, Colin AE, Chandrasekharan B, Bolaji N, Andersen JL, Wayne Outten F, et al. Extra-mitochondrial Cu/Zn superoxide dismutase (Sod1) is dispensable for protection against oxidative stress but mediates peroxide signaling in Saccharomyces cerevisiae. Redox Biol. (2019) 21:101064. doi: 10.1016/j.redox.2018.11.022
46. Eleutherio E, Brasil AA, França MB, de Almeida DSG, Rona GB, Magalhães RSS. Oxidative stress and aging: learning from yeast lessons. Fungal Biol. (2018) 122:514–25. doi: 10.1016/j.funbio.2017.12.003
47. Tajana G, Marotta M, de Palma L. Practical staining method: T-OPA triple stain. Boll Soc Ital Biol Sper. (1980) 56:2026–29.
48. Rhie G, Shin MH, Seo JY, Choi WW, Cho KH, Kim KH, et al. Aging- and photoaging-dependent changes of enzymic and nonenzymic antioxidants in the epidermis and dermis of human skin in vivo. J Invest Dermatol. (2001) 117:1212–7. doi: 10.1046/j.0022-202x.2001.01469.x
49. Fisher GJ, Kang S, Varani J, Bata-Csorgo Z, Wan Y, Datta S, et al. Mechanisms of photoaging and chronological skin aging. Arch Dermatol. (2002) 138:1462–70. doi: 10.1001/archderm.138.11.1462
50. Hellemans L, Corstjens H, Neven A, Declerq L, Maes D. Antioxidant enzyme activity in human stratum corneum shows seasonal variation with an age-dependent recovery. J Invest Dermatol. (2003) 120:434–39. doi: 10.1046/j.1523-1747.2003.12056.x
51. Fisher GJ, Wang ZQ, Datta SC, Varani J, Kang S. Pathophysiology of premature skin aging induced by ultraviolet light. N Engl J Med. (1997) 337:1419–28. doi: 10.1056/NEJM199711133372003
52. Shin MH, Rhie GE, Kim YK, Park CH, Cho KH, Kim KH, et al. H2O2 accumulation by catalase reduction changes MAP kinase signaling in aged human skin in vivo. J Invest Dermatol. (2005) 125:221–9. doi: 10.1111/j.0022-202X.2005.23823.x
53. Chung JH, Seo JY, Choi HR, Lee MK, Youn CS, Rhie G, et al. Modulation of skin collagen metabolism in aged and photoaged human skin in vivo. J Invest Dermatol. (2001) 117:1218–24. doi: 10.1046/j.0022-202x.2001.01544.x
54. Davis R, Chen A, Bend D. Raf and mitogen activated protein kinase regulate stellate cell collagen gene expression. J Biol Chem. (1996) 271:1039–42. doi: 10.1074/jbc.271.19.11039
55. Delanian S, Martin M, Bravard A, Luccioni C, Lefaix JL. Cu/Zn superoxide dismutase modulates phenotypic changes in cultured fibroblasts from human skin with chronic radiotherapy damage. Radiother Oncol. (2001) 58:325–31. doi: 10.1016/S0167-8140(00)00332-7
56. Emerit J, Samuel D, Pavio N. Cu-Zn super oxide dismutase as a potential antifibrotic drug for hepatitis C related fibrosis. Biomed Pharmacother. (2006) 60:1–4. doi: 10.1016/j.biopha.2005.09.002
57. Shindo Y, Witt E, Han D, Packer L. Dose-response effects of acute ultraviolet irradiation on antioxidants and molecular markers of oxidation in murine epidermis and dermis. J Invest Dermatol. (1994) 102:470–75. doi: 10.1111/1523-1747.ep12373027
58. Altobelli GG, van Noorden S, Cimini V. Copper/zinc-superoxide dismutase in human epidermis: an immunochemical study. Front Med. (2019) 6:258. doi: 10.3389/fmed.2019.00258
59. Srivastav AK, Dubey D, Chopra D, Singh J, Negi S, Mujtaba SF, et al. Oxidative stress-mediated photoactivation of carbazole inhibits human skin cell physiology. J Cell Biochem. (2019) 121:1273–82. doi: 10.1002/jcb.29360
60. Barygina V, Becatti M, Lotti T, Moretti S, Taddei N, Fiorillo C. ROS-challenged keratinocytes as a new model for oxidative stress-mediated skin diseases. J Cell Biochem. (2019) 120:28–36. doi: 10.1002/jcb.27485
61. Frank S, Kämpfer H, Podda M, Kaufmann R, Pfeilschifter J. Identification of copper/zinc superoxide dismutase as a nitric oxide-regulated gene in human (HaCaT) keratinocytes: implications for keratinocyte proliferation. Biochem J. (2000) 346:719–28. doi: 10.1042/bj3460719
62. Sasaki H, Akamatsu H, Horio T. Protective role of copper, zinc superoxide dismutase against UVB-induced injury of the human keratinocyte cell line HaCaT. J Invest Dermatol. (2000) 114:502–07. doi: 10.1046/j.1523-1747.2000.00914.x
63. Dunaway S, Odin R, Zhou L, Ji L, Zhang Y, Kadekaro AL. Natural antioxidants: multiple mechanisms to protect skin from solar radiation. Front Pharmacol. (2018) 9:392. doi: 10.3389/fphar.2018.00392
64. Geesin JC, Darr D, Kaufman R, Murad S, Pinnell SR. Ascorbic acid specifically increases type I and type III procollagen messenger RNA levels in human skin fibroblast. J Invest Dermatol. (1988) 90:420–24. doi: 10.1111/1523-1747.ep12460849
65. Nachbar F, Korting HC. The role of vitamin E in normal and damaged skin. J Mol Med. (1995) 73:7–17. doi: 10.1007/BF00203614
66. Traikovich SS. Use of topical ascorbic acid and its effects on photodamaged skin topography. Arch. Otolaryngol. Head Neck Surg. (1999) 125:1091–98. doi: 10.1001/archotol.125.10.1091
67. Elmets CA, Singh D, Tubesing K, Matsui M, Katiyar S, Mukhtar H. Cutaneous photoprotection from ultraviolet injury by green tea polyphenols. J Am Acad Dermatol. (2001) 44:425–32. doi: 10.1067/mjd.2001.112919
68. Žmitek K, Pogačnik T, Mervic L, Žmitek J, Pravst I. The effect of dietary intake of coenzyme Q10 on skin parameters and condition: results of a randomised, placebo-controlled, double-blind study. Biofactors. (2017) 43:132–40. doi: 10.1002/biof.1316
69. Karković Marković A, Torić J, Barbarić M, Jakobušić Brala C. Hydroxytyrosol, tyrosol and derivatives and their potential effects on human health. Molecules. (2019) 24:2001. doi: 10.3390/molecules24102001
70. Takasawa R, Nakamura H, Mori T, Tanuma S. Differential apoptotic pathways in human keratinocyte HaCaT cells exposed to UVB and UVC. Apoptosis. (2005) 10:1121–30. doi: 10.1007/s10495-005-0901-8
71. Choung BY, Byun SJ, Suh JG, Kim TY. Extracellular superoxide dismutase tissue distribution and the patterns of superoxide dismutase mRNA expression following ultraviolet irradiation on mouse skin. Exp Dermatol. (2004) 13:691–99. doi: 10.1111/j.0906-6705.2004.00209.x
72. Hamanaka H, Miyachi Y, Tachibana T, Imamura S. Lowered Cu, Zn-Superoxide dismutase activity in human malignant skin tumors. J Dermatol. (1991) 18:258–61. doi: 10.1111/j.1346-8138.1991.tb03079.x
73. Kobayashi T, Matsumoto M, Iizuka H, Suzuki K, Taniguchi N. Superoxide dismutase in psoriasis, squamous cell carcinoma and basal cell epithelioma: an immunohistochemical study. Br J Dermatol. (1991) 124:555–9. doi: 10.1111/j.1365-2133.1991.tb04950.x
74. Rittié L, Fisher GJ. Natural and sun-induced aging of human skin. Cold Spring Harb Perspect Med. (2015) 5:a015370. doi: 10.1101/cshperspect.a015370
75. Kim Y, He YY. Ultraviolet radiation-induced non-melanoma skin cancer: regulation of DNA damage repair and inflammation. Genes Dis. (2014) 1:188–98. doi: 10.1016/j.gendis.2014.08.005
76. Jhappan C, Noonan FP, Merlino G. Ultraviolet radiation and cutaneous malignant melanoma. Oncogene. (2003) 22:3099–12. doi: 10.1038/sj.onc.1206450
77. Chen H, Weng QY, Fisher DE. UV signaling pathways within the skin. J Invest Dermatol. (2014) 134:2080–85. doi: 10.4045/tidsskr.14.1072
78. Liu K, Yu D, Cho YY, Bode AM, Ma W, Yao K, et al. Sunlight UV-induced skin cancer relies upon activation of the p38α signaling pathway. Cancer Res. (2013) 73:2181–88. doi: 10.1158/0008-5472.CAN-12-3408
79. Kansara S, Bell D, Weber R. Surgical management of non melanoma skin cancer of the head and neck. Oral Oncol. (2019) 100:104485. doi: 10.1016/j.oraloncology.2019.104485
80. Liu-Smith F, Jia J, Zheng Y. UV-induced molecular signaling differences in melanoma and non-melanoma skin cancer. Adv Exp Med Biol. (2017) 996:27–40. doi: 10.1007/978-3-319-56017-5_3
81. Nogués MR, Giralt M, Cervelló I, Del Castillo D, Espeso O, Argany N, et al. Parameters related to oxygen free radicals in human skin: a study comparing healthy epidermis and skin cancer tissue. J Invest Dermatol. (2002) 119:645–52. doi: 10.1046/j.1523-1747.2002.00077.x
82. Shaw SR, Breur J, Ahma N. Enhancement of UVB radiation – mediated apoptosis by sanguinarine in HaCat human immortalized keratinocytes. Mol Cancer Ther. (2006) 5:418–29. doi: 10.1158/1535-7163.MCT-05-0250
83. Fischer TW, Kleszczynski K, Hardkop LH, Kruse N, Zillikens D. Melatonin enhances antioxidative enzyme gene expression (CAT, GPx, SOD), prevents their UVR-induced depletion, and protects against the formation of DNA damage (8-hydroxy-2'-deoxyguanosine) in ex vivo human skin. J Pineal Res. (2013) 54:303–12. doi: 10.1111/jpi.12018
84. Kasper M, Jaks V, Hohl D, Toftgård R. Basal cell carcinoma - molecular biology and potential new therapies. J Clin Invest. (2012) 122:455–63. doi: 10.1172/JCI58779
85. Muzic JG, Schmitt AR, Wright AC, Alniemi DT, Zubair AS, Olazagasti Lourido JM, et al. Incidence and trends of basal cell Carcinoma and cutaneous squamous cell Carcinoma: a population-based study in olmsted county, Minnesota, 2000 to 2010. Mayo Clin. Proc. (2017) 92:890–98. doi: 10.1016/j.mayocp.2017.02.015
86. Proia NK, Paszkiewicz GM, Nasca MA, Franke GE, Pauly JL. Smoking and smokeless tobacco-associated human buccal cell mutations and their association with oral cancer–a review. Cancer Epidemiol Biomarkers Prev. (2006) 15:1061–77. doi: 10.1158/1055-9965.EPI-05-0983
87. Li C, Athar M. Ionizing radiation exposure and basal cell Carcinoma pathogenesis. Radiat Res. (2016) 185:217–28. doi: 10.1667/RR4284.S1
88. Ohkuma N, Matsuo S, Iizuka H, Ohkawara A. Superoxide dismutase in epidermis. J Dermatol. (1987) 14:218–23. doi: 10.1111/j.1346-8138.1987.tb03564.x
89. Kobayashi T, Saito N, Takemori N, Iizuka S, Suzuki K, Taniguchi N, et al. Ultrastructural localization of superoxide dismutase in human skin. Acta Derm Venereol. (1993) 73:41–5.
90. Murakami K, Inagaki J, Saito M, Ikeda Y, Tsuda C, Noda Y, et al. Skin atrophy in cytoplasmic SOD-deficient mice and its complete recovery using a vitamin C derivative. Biochem Biophys Res Commun. (2009) 382:457–61. doi: 10.1016/j.bbrc.2009.03.053
91. Iuchi Y, Roy D, Okada F, Kibe N, Tsunoda S, Suzuki S, et al. Spontaneous skin damage and delayed wound healing in SOD1-deficient mice. Mol Cell Biochem. (2010) 341:181–94. doi: 10.1007/s11010-010-0449-y
92. Shibuya S, Ozawa Y, Watanabe K, Izuo N, Toda T, Yokote K, et al. Palladium and platinum nanoparticles attenuate aging-like skin atrophy via antioxidant activity in mice. PLoS ONE. (2014) 9:e109288. doi: 10.1371/journal.pone.0109288
93. Kim J, Toda T, Watanabe K, Shibuya S, Ozawa Y, Izuo N, et al. Syringaresinol reverses age-related skin atrophy by suppressing FoxO3a-mediated matrix metalloproteinase-2 activation in Copper/Zinc superoxide dismutase-deficient mice. J Invest Dermatol. (2019) 139:648–55. doi: 10.1016/j.jid.2018.10.012
Keywords: Cu/Zn superoxide dismutase, human skin, immunochemistry, skin tumors, ROS
Citation: Altobelli GG, Van Noorden S, Balato A and Cimini V (2020) Copper/Zinc Superoxide Dismutase in Human Skin: Current Knowledge. Front. Med. 7:183. doi: 10.3389/fmed.2020.00183
Received: 24 February 2020; Accepted: 17 April 2020;
Published: 12 May 2020.
Edited by:
Ivan V. Litvinov, McGill University, CanadaReviewed by:
Takahiko Shimizu, National Center for Geriatrics and Gerontology (NCGG), JapanSalvador Gonzalez, University of Alcalá, Spain
Copyright © 2020 Altobelli, Van Noorden, Balato and Cimini. This is an open-access article distributed under the terms of the Creative Commons Attribution License (CC BY). The use, distribution or reproduction in other forums is permitted, provided the original author(s) and the copyright owner(s) are credited and that the original publication in this journal is cited, in accordance with accepted academic practice. No use, distribution or reproduction is permitted which does not comply with these terms.
*Correspondence: Giovanna G. Altobelli, Z2dhbHRvYmUmI3gwMDA0MDt1bmluYS5pdA==; Vincenzo Cimini, Y2ltaW5pJiN4MDAwNDA7dW5pbmEuaXQ=