- 1Renal Group, Basic Medical Research Centre, Medical College of Nantong University, Nantong, China
- 2Department of Nephrology, Nantong-Leicester Joint Institute of Kidney Science, Affiliated Hospital of Nantong University, Nantong, China
- 3Department of Cardiovascular Sciences, University of Leicester, University Hospitals of Leicester, Leicester, United Kingdom
- 4Department of Urology, Zhongshan Hospital, Fudan University, Shanghai, China
- 5Shanghai Key Laboratory of Organ Transplantation, Shanghai, China
Ischemia-reperfusion (IR) injury often affects transplant and native kidneys alike. IR injury is one of the main causes of acute kidney injury (AKI) and further associated with the progression of chronic kidney disease. Our previous study revealed the renoprotection of erythropoietin derived cyclic helix-B surface peptide (CHBP) against IR injury. However, the precise role and underlying mechanism of endoplasmic reticulum stress (ERS) in the injury and the renoprotection induced by IR or CHBP, respectively, have not been fully defined. This study using mouse kidney epithelial cells (TCMK-1) revealed that the level of CHOP (a key marker of ERS), PERK, and JNK (regulators of CHOP) was gradually increased by the prolonged time of hydrogen peroxide (H2O2) stimulation. In addition, CHOP mRNA and protein were significantly reduced by small interfering RNA (siRNA) target CHOP, as were apoptotic and inflammatory mediator caspase-3 and HMGB-1, and early apoptosis. Furthermore, CHOP mRNA was correlated positively with PERK protein, active caspase-3, HMGB-1 and apoptosis, but negatively with cell viability in vitro, while CHOP protein was also correlated positively with the level of tubulointerstitial damage and active caspase-3 protein in vivo. Finally, CHBP improved the viability of TCMK-1 cells subjected to H2O2 stimulation time-dependently, with reduced level of CHOP mRNA. CHBP also inhibited the increase of CHOP protein, not only in TCMK-1 cells, but also in the IR injury kidneys at 2 weeks. Moreover, CHBP reduced the expression of PERK mRNA and protein, JNK and HMGB-1 protein, as well as early and later apoptosis. In addition, raised CHOP at 12 h post IR injury might be an early time window for intervention. In conclusion, the differential role of ERS and CHBP in IR-related injury was proved in mouse TCMK-1 cells and kidneys, in which the mechanistic signaling pathway was associated with CHOP/PERK/JNK, HMGB-1/caspase-3, and apoptosis. CHOP might be a potential biomarker and CHBP might be therapeutic drug for IR-induced AKI.
Introduction
Renal ischemia-reperfusion (IR) injury is inevitable in kidney transplantation, which greatly affects the survival of allograft (1–3). IR injury also often occurs in native kidneys subjected to a variety of causes such as cardiovascular surgery, dehydration, and sepsis. IR injury is a major inducement of acute kidney injury (AKI) and further associated with its progression to chronic kidney disease (CKD). It is imperative to disclose the mechanism of IR injury in order to achieve time diagnosis and specific treatment for IR-induced AKI and prevent CKD. Renal tubular epithelial cells (TECs) are most vulnerable to renal IR insult, underwent to autophagy, apoptosis or necrosis, which contributes to the loss of renal function, and subsequent repair/recovery or fibrosis (4–6). The underlying mechanisms of IR injury and repair are complicated including endoplasmic reticulum stress (ERS), mitochondria dysfunction, cell death, and inflammation (7–10). Improving ERS and TEC apoptosis have been considered to be effective methods to alleviate renal IR injury (3, 11).
Newly synthesized proteins fold in the lumen of ER prior to exit into various compartments of the cell and extracellular space. In response to adverse conditions, these proteins could folded incorrectly and unable to exit, but accumulate inside ER resulting in ERS (9, 12). A series of adaptions were subsequently activated, called the unfolded protein response (UPR) (9, 13, 14). The UPR is attributed to the activation of one ER transmembrane protein, named protein kinase RNA-like ER kinase (PERK) (15–17). Growing evidence has shown that ERS associated with apoptosis and inflammation plays important roles in IR injury (18, 19). UPR could gradually develop into apoptosis and further induces inflammation, if ERS is too severe to overcome. CCAAT/enhancer-binding protein homologous protein (CHOP) is an ERS-induced transcriptional regulator and key to ERS-mediated apoptotic pathway (20, 21). The over expression of CHOP plays an important role in the activation of C-Jun N-terminal kinase (JNK) that contributes to apoptotic cell death (22, 23). CHOP-mediated apoptosis and inflammation exacerbate myocardial IR injury, whereas CHOP−/− mice have been shown to be resistant to apoptosis in various disease models (24).
Erythropoietin (EPO), on the other hand, could protect various organs including kidney against IR injury (25). The renoprotection of EPO is through a heterodimer EPO receptor and β-common receptor (EPOR/βcR). EPOR/βcR is pharmacologically distinct from the homodimer (EPOR)2 that mediates erythropoiesis (26–28). The renoprotection of EPO against IR injury has been shown by our previous studies, in terms of reducing tubular cell apoptosis, but promoting inflammatory apoptotic cell clearance (29, 30). However, the large dosage of EPO was required to achieve tissue protection, which often causes hypertension and thrombosis in vivo (31). Therefore, a helix B surface peptide (HBSP) derived from EPO was developed, which is composed by 11 amino acids (QEQLERALNSS) and interacts with EPOR/βcR only, but with very short serum half-life (32). A metabolic stable cyclic HBSP (CHBP) was further generated, having prolonged half-life and potent tissue protection. The renoprotection of HBSP and CHBP against IR injury has also been confirmed in our previous research projects (33, 34). The pretreatment of CHBP reduced the active level of oxidative damage and ERS induced hydrogen peroxide (H2O2) in human proximal tubular cell line, HK-2 cells (35), but its underlying mechanisms have still not been well-defined, especially the impact of CHBP on ERS regulators in TECs, as well as in kidneys post IR injury with extended time to 2 and 8 weeks.
We hypothesized that ERS and CHBP plays differential role in renal IR-related injury, which is associated CHOP and its regulators, apoptotic and inflammatory mediators, as well as subsequent apoptosis. To verify this hypothesis, TCMK-1 cells, mouse kidney epithelial cell line, stimulated by H2O2 and mouse kidneys subjected to IR injury were used to evaluate the change of CHOP, the key marker of ERS, and its regulators PERK and JNK, and their relations to apoptotic and inflammatory mediators caspase-3 and HMGB-1, and apoptosis. The impacts of intervention using siRNA target CHOP and CHBP treatment, on above biological events were further studied. It has been proved in this study that ERS plays key roles in IR-related injury and CHBP induced renoprotection in mouse TCMK-1 cells at the early stage and in kidneys with prolonged IR time, and the underlying mechanistic pathway is associated with CHOP/PERK/JNK, HMGB-1/ caspase-3, and apoptosis. CHOP might be a potential biomarker and CHBP might be therapeutic drug for IR-induced AKI.
Methods and Materials
TCMK-1 Cell Culture and H2O2 Stimulation
TCMK-1 cells (a mouse kidney epithelial cell line, CCL-139™, American Type Culture Collection, Manassas, USA) were cultured in DMEM/F12 medium, supplemented with 10% (v/v) of fetal bovine serum (FBS, Gibco Technologies, Logan, USA), 100 unit/ml penicillin G (Gibco), and 100 μg/ml streptomycin (Gibco), defined as completed medium, at 37°C in 5% CO2 atmosphere. TCMK-1 cells grown about 80% confluent in monolayer in 6-well plates were treated with or without 500 μmol/L H2O2 (Merck KGaA, Darmstadt, Germany) diluted in basic culture medium. At 4, 8, 12 h after the exposure of H2O2, the cells were washed three times with PBS (Ji Nuo, Hangzhou, China) and harvested for further analysis. Twenty ng/ml of CHBP (synthesized by Shanghai Institute of Materia Medica, Chinese Academy of Sciences) was added at the same time upon H2O2 stimulation and cultured for 12 h (35).
According to the instruction of manufacturer, the small interfering RNA (siRNA) was diluted to 5 nM transfected into TCMK-1 cells using Lipofectamine@ RNAiMAX (Invitrogen, Carlsbad, USA). A double-stranded CHOP siRNA (Thermo Fisher Scientific, Waltham, USA) targeting mouse CHOP mRNA was designed and constructed. The sequences of CHOP siRNA are: sense: GGAAGAACUAGGAAACGGATT, antisense: UCCGUUUCCUAGUUCUUCCTT. The negative control siRNA (# 4390843, Thermo Fisher Scientific) does not target any known mammalian genes. The transfected cells were cultured at 37°C for 8 h before 12-h H2O2 stimulation.
Renal IR Injury Model
Male BALB/c mice weighing 20–25 g were obtained from the Experimental Animal Center of Nantong University, China. They were housed in constant temperature (25°C) and humidity (55%) on a 12-h light/dark cycle, fed ad libitum on standard laboratory mouse chow and tap water. All animal procedures were performed according to the guidelines of the Animal Care and Use Committee of Nantong University and the Jiangsu Province Animal Care Ethics Committee (36).
The mice were anesthetized using 80 mg/kg sodium pentobarbital by intraperitoneal injections. The bilateral renal pedicles were exposed by flank incision and clamped for 30 min. For reperfusion, the clamping was released and confirmed blood reflow by monitoring the color change of the kidney before suturing the incision. The mice were randomly divided into 7 groups with different reperfusion time: control, 6, 12, 24, 72 h, and 1 w or 3 groups (n = 6): (1) Control group: the abdominal cavity and renal pedicles were exposed without clamping-induced IR injury, (2) IR group: mice were subjected to ischemia 30 min followed by reperfusion for 2 and 8 weeks, (3) IR + CHBP group: IR injury with 24 nmol/kg CHBP dissolved in 0.9% saline intraperitoneally injected after reperfusion every 3 days (37).
Cell Viability Analysis
TCMK-1 cells (1 × 105) were seeded in 96-well plates and cultured in the completed medium 24 h before adding 500 μmol/L of H2O2 apart from the control group. In the treatment groups, 20 ng/ml of CHBP was added at the same time upon H2O2 stimulation. Ten microliter of CCK-8 (Beyotime, Nantong, China) was then added after 1, 4, and 12 h and further incubated for 1–4 h. The cell viability was measured by absorbance using a microplate reader (MDC, Hayward, USA).
Quantitative Real-Time Polymerase Chain Reaction
Total RNA was extracted from cultured cells with Trizol reagent (Invitrogen) according to the manufacturer's instructions. Complementary DNA (cDNA) was synthesized using 1 μg of total RNA, oligo d(T) 18 Primer and Reverse Transcription Kit (Bio-Rad, Hercules, USA). Quantitative RT-PCR analysis was performed in the real time PCR system (CFX96, Bio-Rad) with AceQ® qPCR SYBR® Green system (Vazyme biotech, Nanjing, China). The oligonucleotide primers for target genes were used as follows: CHOP: forward 5′- ATGTGCGTGTGACCTCTGTT-3′ and reverse 5′-TATCTCATCCCCAGGAAACG, PERK: forward 5′-TAGATGAAACCAAGGAACCAGA-3′, and reverse 5′-ATCAGCACTTTAGATGGACGAA-3′). We amplified 2 μl cDNA for each sample in a 20 μl reaction system containing SYBR Green Master Mix and primers with the following cycling conditions: 2 min at 95°C, 40 cycles of 95°C for 10 s, then 60°C for 10 s. Expression levels were normalized with GAPDH in the same samples using the 2−ΔΔCt method.
Western Blot Analysis
RIPA lysis buffer (Beyotime) containing phenylmethylsulfonyl fluoride, protease inhibitor, and phosphatase inhibitor was used to crack renal tissues or cells. Total protein was isolated according to the standard methods (34). The protein was measured by Pierce BCA Protein Quantitation Kit (Pierce, Rockland, USA). Twenty five micrograms of protein from TCMK-1 cells or kidney cortex was separated on a 12–15% (w/v) polyacrylamide denaturing gel and electronically blotted onto 0.45 μm polyvinylidene difluoride (PVDF) membranes (Roche Diagnostics GmbH, Mannheim, Germany) on 14 volts for 16 h at 4°C or 100 volts for 1 h at room temperature. After 2-h blocking with 5% milk, caspase-3 or CHOP antibody (both at 1:200, Santa Cruz Biochemicals, Santa Cruz, USA), or PERK, JNK, HMGB-1, β-actin or α-Tubulin antibody (all at 1:1,000, Cell Signaling Technology, Beverly, USA) was incubated overnight at 4°C. The horseradish peroxidase-conjugated secondary antibody (Jackson ImmunoResearch Laboratories, West Grove, USA) was then incubated for 2 h at room temperature. Antibody binding was detected by enhanced chemiluminescent (ECL, Pierce) and a Molecular Imager, Chemi Doc, XRS+ System (Bio-Rad, Berkeley, USA) (36). Developed images were semi quantitatively analyzed by scanning volume density using Alpha View Software 3.3 (Cell Biosciences Inc., Santa Clara, USA). The expression of target protein was normalized against to the expression of β-actin or α-Tubulin, and presented as an ratio.
Determination of Apoptosis by Flow Cytometry
Adherent TCMK-1 cells were harvested by tripsinizing and centrifuged at 1,000 rpm/min for 5 min, then washed twice with PBS. The pellet was resuspended in 100 μl of 1× binding buffer and incubated with 5 μl of FITC-conjugated annexin-V and 5 μl of propidium iodide (PI) for 15 min at room temperature in the dark. Another three tubes: annexin-V only, PI only and none dye was prepared as controls. Four hundred μl of 1× binding buffer was added to each sample tube, and the samples were analyzed by BD FACS Calibur flow cytometer (Becton Dickinson, San Jose, USA) using Cell Quest Research Software (BD Biosciences, Franklin Lakes, USA), a minimum of 10,000 cells were counted.
The results were shown as quadrant dot plots with survival cells (Annexin V-/PI-), early apoptotic cells (Annexin V+/PI-), later apoptotic cells or necrotic cells (Annexin V+/PI+). The number of each type of cells was expressed as the percentage of each type of cells against the total number of gated cells.
Correlation Analysis
The correlation analysis was used to measure the correlation between two parameters. The range of R value between 0 and 1 or −1 and P < 0.05 implies positive or negative correlation (38). This method was used to analyze the correlation between CHOP and PERK, JNK, apoptosis, or cell viability in the in vitro model.
Statistical Analysis
All data are representative of at least three independent experiments, while the data are presented as the mean ± standard error of the mean (SEM). The results among three or more groups were compared by one-way analysis of variance (ANOVA), while those in any two groups were compared using a two-tailed independent Student's t-test. All statistical analysis were performed using GraphPad Prism 6.0 (GraphPad Software Inc., San Diego, USA), with P < 0.05 considered statistically significant.
Results
ERS Induced by H2O2 in TCMK-1 Cells
To study the dynamic change of CHOP and its regulator PERK, the expression of CHOP mRNA and protein, and PERK mRNA was measured by qPCR and western blot in TCMK-1 cells treated with 500 μmol/L H2O2 for 4, 8, 12 h. The mRNA expression of CHOP and PERK was gradually increased with the time, and reached significance at 8 h compared with the control, and peaked at 12 h compared with the control or 4 h (all P < 0.01, Figures 1A,B). The expression of CHOP protein was also increased at 12 h (1.06 ± 0.13) compared with the control or 4 h, respectively (0.79 ± 0.04 or 0.67 ± 0.10, both P < 0.05, Figures 1C,D).
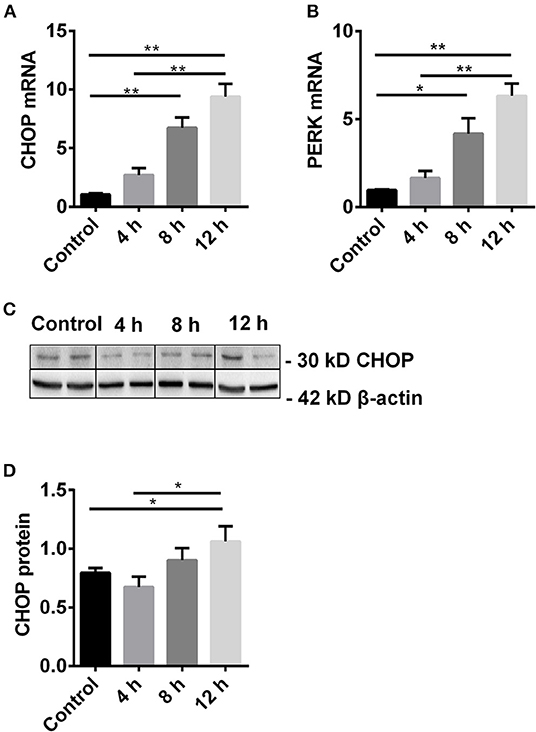
Figure 1. The dynamic change of CHOP and its regulator PERK. (A,B) The mRNA level of CHOP and PERK was gradually increased and reached the significance at 8 h and peaked at 12 h. (C,D) The expression of CHOP protein was significantly increased at 12 h. Data are expressed as mean ± SEM (n = 3). The volume density of western blots was corrected by against 42 kD β-actin as a loading control. *P < 0.05, **P < 0.01. h, hours.
CHOP siRNA Downregulated Its mRNA and Protein in vitro
In the TCMK-1 cells transfected with CHOP siRNA, the expression of CHOP mRNA was reduced by 53% and 56% compared to the cells treated by H2O2 or the negative control (NC) siRNA, respectively (both P < 0.01, Figure 2A). In addition, CHOP siRNA also reduced the expression of CHOP protein (0.69 ± 0.08 vs. 1.17 ± 0.17 or 1.42 ± 0.10, P < 0.05 or 0.01, Figures 2B,C) in the same comparisons.
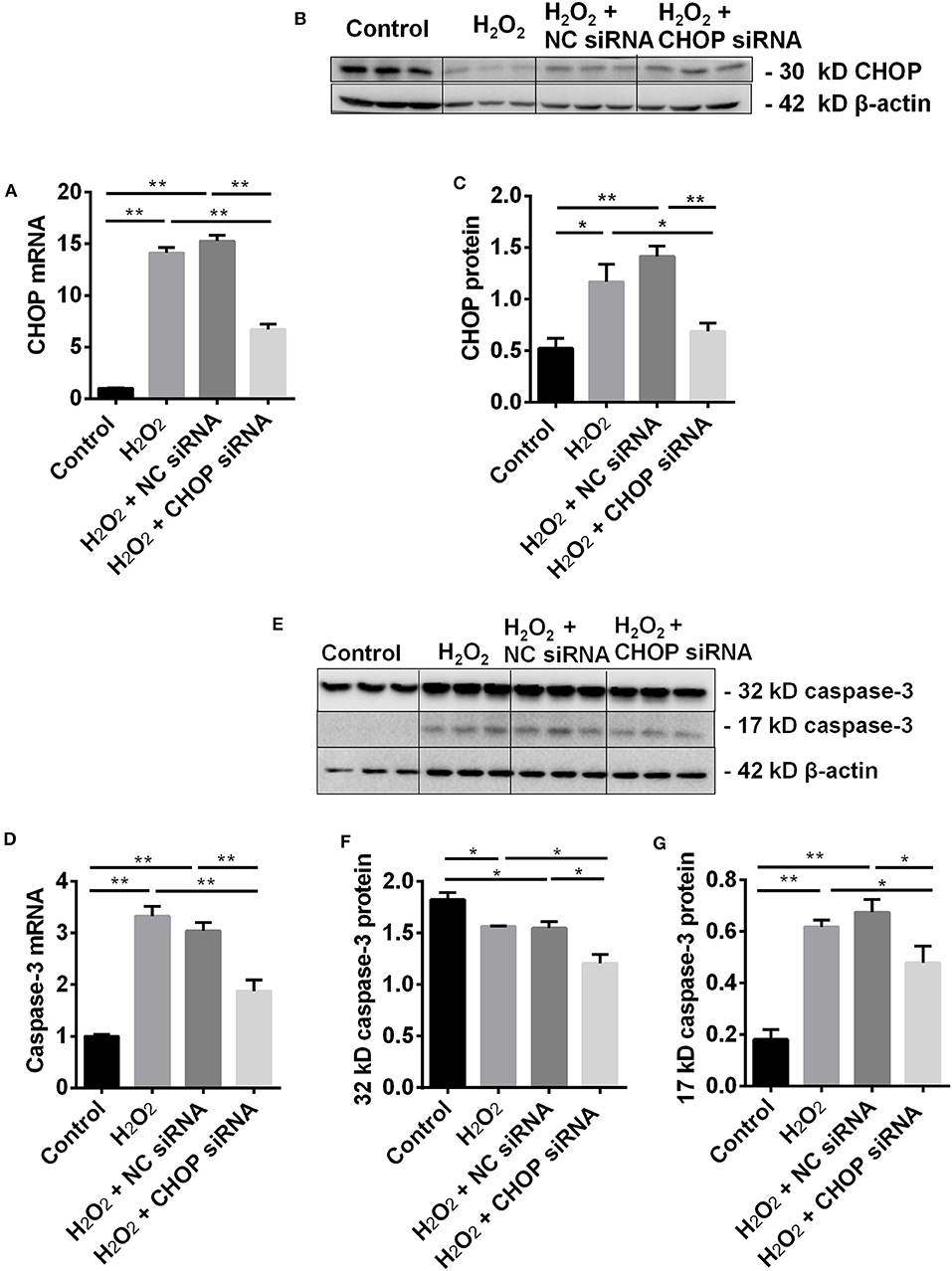
Figure 2. CHOP siRNA downregulated its mRNA, protein and caspase-3 in TCMK-1 cells. (A) The expression of CHOP mRNA in the TCMK-1 cells transfected with CHOP siRNA was reduced by 53% and 56% compared to the cells treated by the H2O2 and the NC siRNA. (B,C,E,G) The expression of CHOP protein as well as caspase-3 mRNA (D) and 17 kD caspase-3 protein was increased at the H2O2 and the NC siRNA group but reversed by CHOP siRNA. (E,F) The expression of 32 kD caspase 3 protein was decreased by H2O2 or NC siRNA and further decreased by CHOP siRNA. Data are expressed as mean ± SEM (n = 3). The volume density of western blots was corrected by against 42 kD β-actin as a loading control. *P < 0.05, **P < 0.01.
CHOP siRNA Decreased Caspase-3 and HMGB-1 Expression in TCMK-1 Cells
The effect of CHOP siRNA on caspase-3 expression was further investigated. The level of caspase-3 mRNA (Figure 2D) and 17 kD protein (Figures 2E,G), contrary to 32 kD protein (Figures 2E,F), was increased in the H2O2 or NC siRNA group. However, they all were significantly decreased by CHOP siRNA (mRNA: 1.88 ± 0.21 vs. 3.33 ± 0.19 or 3.04 ± 0.17, 17 kD: 1.21 ± 0.08 vs. 1.57 ± 0.01 or 1.55 ± 0.07, 32 kD: 0.34 ± 0.04 vs. 0.62 ± 0.03 or 0.60 ± 0.07, P < 0.05 or 0.01).
The level of HMGB-1 protein was significantly up-regulated in the H2O2 group (P < 0.05, Figures 3A,B), but was down-regulated by CHOP siRNA compared to the H2O2 and NC siRNA group (0.81 ± 0.11 vs. 1.27 ± 0.34 and 1.18 ± 0.11, P < 0.05).
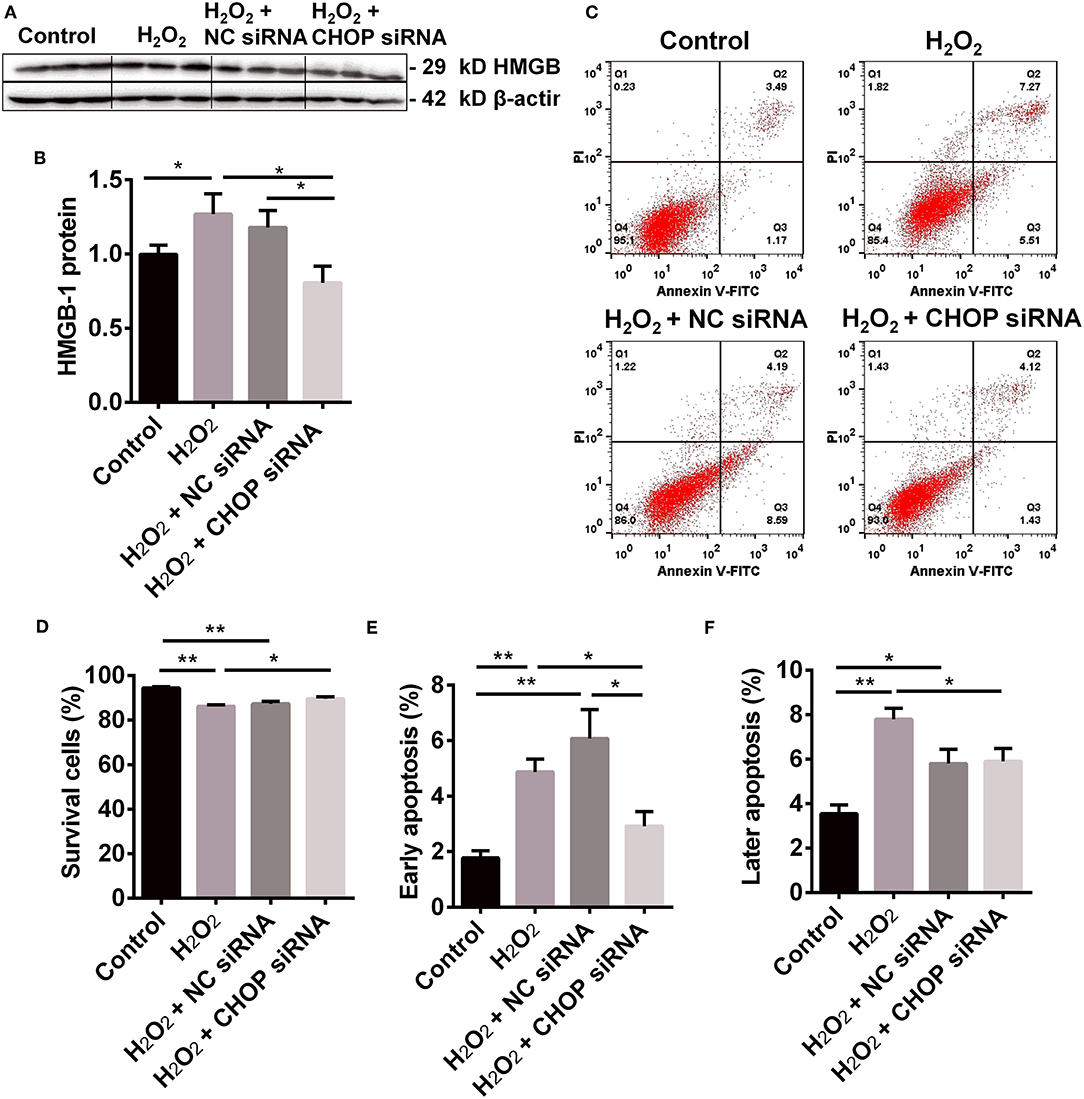
Figure 3. The renoprotection of CHBP associated with ERS improvement, apoptosis and inflammation. (A,B) HMGB-1 protein was significantly up-regulated by H2O2, but was down-regulated by CHOP siRNA compared to the H2O2 and NC siRNA groups. (C,D) The percentage of living cells was decreased by H2O2 and H2O2 + NC siRNA but reversed by H2O2 + CHOP siRNA. (E,F) The both early and later apoptotic cells were increased by H2O2 and H2O2 + NC siRNA, but only the early apoptotic cells were reduced by H2O2 + CHOP siRNA. Data are expressed as mean ± SEM (n = 3). The volume density of western blots was corrected by against 42 kD β-actin as a loading control. *P < 0.05, **P < 0.01.
CHOP siRNA Decreased Apoptosis in TCMK-1 Cells
The cells stimulated by H2O2, NC siRNA or CHOP siRNA post H2O2 stimulation were presented (Figure 3C). The percentage of survival cells was decreased by H2O2 and NC siRNA compared with the control group, but reversed by CHOP siRNA (81.55 ± 1.74 vs. 75.66 ± 1.54, P < 0.05, Figure 3D). The early apoptotic cells and later apoptotic cells were increased, respectively, by H2O2 and NC siRNA treatment compared with the control group (Figures 3E,F). Only the early apoptotic cells were reduced by CHOP siRNA compared to the H2O2 and NC siRNA group (7.07 ± 1.28 vs. 14.58 ± 1.71 and 16.84 ± 2.74, P < 0.01, and 0.05).
CHOP Correlated With PERK, Caspase-3, HMGB-1, and Apoptosis Positively, but Cell Viability Negatively
To further investigate the role of CHOP in IR-related injuries, the correlation between CHOP mRNA and PERK, 17 kD active caspase-3, HMGB-1, apoptosis, and cell viability was analyzed, respectively. CHOP mRNA was correlated positively with PERK protein, active caspase-3, HMGB-1 and apoptosis (R = 0.7219, 0.9343, 0.7129, and 0.8823, all P < 0.01, Figure 4), but negatively with cell viability (R = −0.5248, P < 0.05) in the in vitro model.
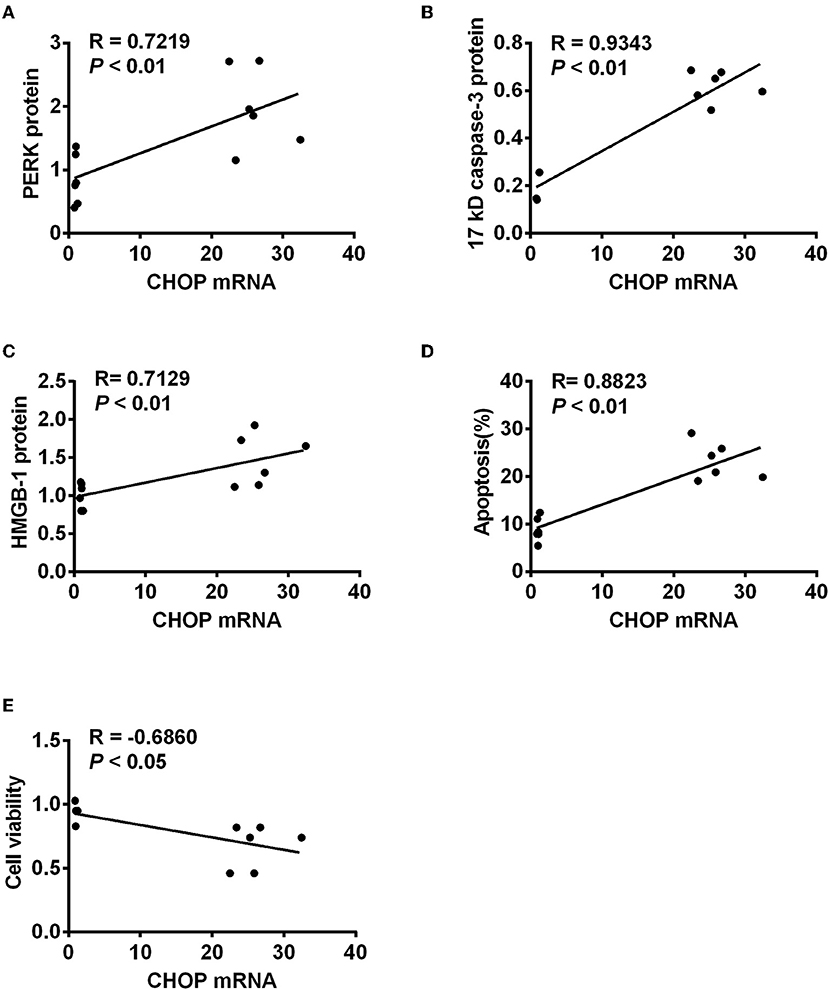
Figure 4. The correlation between CHOP mRNA and other detected parameters. CHOP mRNA was positively correlated with PERK protein (A), active caspase-3 (B), HMGB-1 (C), and apoptosis (D) (R = 0.7219, 0.9343, 0.7129, and 0.8823, all P < 0.01) but negatively with cell viability (E, R = −0.5248, P < 0.05) in the in vitro model.
CHBP Increased TCMK-1 Cell Viability Post H2O2 Stimulation
In order to observe the effect of CHBP, renoprotective peptide derived from erythropoietin, on cell viability, the CCK-8 assay was used to detect the value of optical density (OD) at 1, 4, 12 h after H2O2 stimulation (Figure 5A). The cell viability gradually decreased with the time of H2O2 stimulation with or without CHBP and reached the significance from 1 to 12 h compared with the control (P < 0.01 or 0.05).
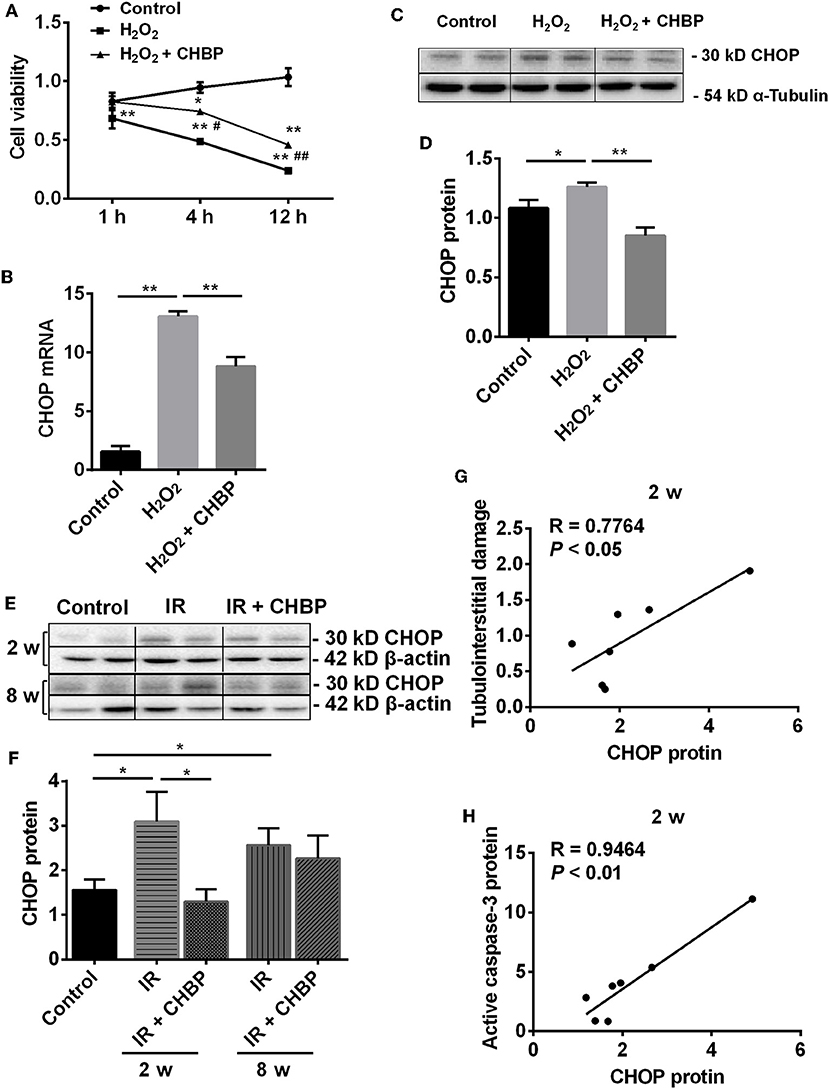
Figure 5. The dynamic change of cell viability and the expression of CHOP mRNA and protein in TCMK-1 cells and kidneys. (A) The cell viability was gradually decreased with the time of H2O2 treatment, reached the significance from 1 to 12 h, but was significantly improved in the H2O2 + CHBP group after 4 h. (B–D) The mRNA and protein of CHOP were significantly increased in the TCMK-1 cells stimulated by H2O2, but reversed by CHBP. (E,F) CHOP protein was also significantly increased in the IR group at 2 and 8 weeks, but was decreased by CHBP only at 2 weeks. Data are expressed as mean ± SEM (n = 3–6). The volume density of western blots was corrected by against 42 kD β-actin or 54 kD α-Tubulin as a loading control. (G,H) CHOP protein was positively correlated with the level of TID and active caspase-3 protein at 2 weeks. *, #P < 0.05, **, ##P < 0.01 vs. Control, 4 h or H2O2 + CHBP.
More importantly, the cell viability was significantly increased in the H2O2 + CHBP group at 4 and 12 h (0.74 ± 0.08 and 0.46 ± 0.06) compared with the H2O2 group (0.49 ± 0.06 and 0.24 ± 0.04, P < 0.05 and 0.01).
CHBP Reduced ERS Induced by H2O2 and IR Injury
To further dissect the mechanism of CHBP renoprotection in association with ERS, the mRNA and protein of CHOP were measured in the TCMK-1 cells stimulated by H2O2 for 12 h (Figures 5B–D). It has been revealed that the mRNA and protein of CHOP were increased (P < 0.01 or 0.05), whereas the increased CHOP mRNA and protein were reversed by CHBP treatment (mRNA: 8.82 ± 0.79 vs. 13.06 ± 0.44, protein: 0.85 ± 0.06 vs. 1.26 ± 0.04, P < 0.01).
In addition, the expression of CHOP protein was significantly increased in the IR kidneys at 2 and 8 weeks (both P < 0.05), which was decreased by CHBP only at 2 weeks (1.30 ± 0.28 vs. 3.10 ± 0.66, P < 0.05, Figures 5E,F). The CHOP protein was also correlated positively with the level of tubulointerstitial damage (TID) and active caspase-3 protein at 2 weeks (R = 0.7764 and 0.9464, P < 0.05 or 0.01, Figures 5G,H). Besides that, a trend of positive correlation between CHOP protein and apoptosis or interstitial fibrosis was showed at 2 weeks, respectively, even though there was no statistical significance (R = 0.5612, P = 0.1478; R = 0.4467, P = 0.2671). However, the original data of TID, active caspase-3 protein, apoptosis and interstitial fibrosis have not been shown here.
Moreover, the level of PERK mRNA and protein (Figures 6A–C), JNK protein (Figures 6D,E) and HMGB-1 protein (Figures 6F,G) was significantly up-regulated by H2O2 (P < 0.05 or 0.01), which was down-regulated by CHBP (PERK mRNA: 2.24 ± 0.22 vs. 3.20 ± 0.26, PERK protein: 1.15 ± 0.15 vs. 1.98 ± 0.17, JNK protein: 1.10 ± 0.08 vs. 1.48 ± 0.11, HMGB-1 protein: 0.89 ± 0.04 vs. 1.21 ± 0.11, all P < 0.05).
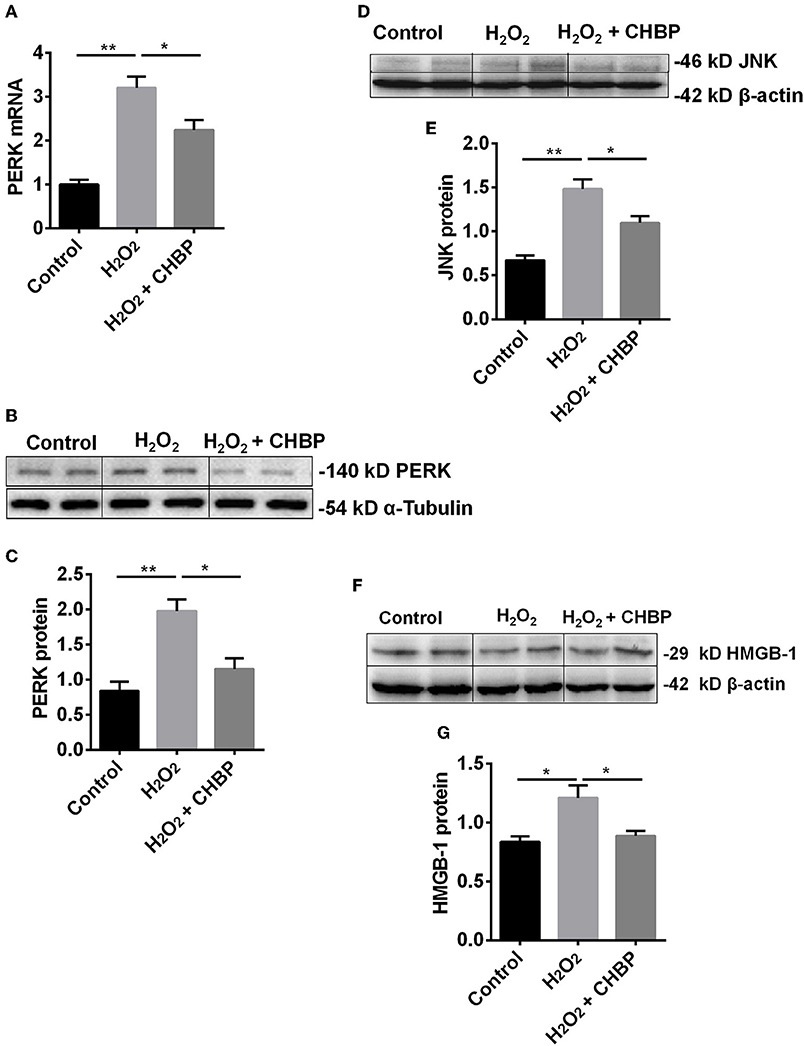
Figure 6. The expression of PERK, JNK and HMGB-1 protein and/or mRNA. The mRNA (A) and protein (B,C) of PERK, as well as JNK protein (D,E) and HMGB-1 protein (F,G), were significantly increased by H2O2 in the TCMK-1 cells. However, these effects were reversed by CHBP. Data are expressed as mean ± SEM (n = 3). The volume density of western blots was corrected by against 42 kD β-actin or 54 kD α-Tubulin as a loading control. *P < 0.05, **P < 0.01.
CHBP Inhibited Cell Apoptosis in vitro
Apoptotic cells were detected by flow cytometry using Annexin V/PI staining. The population of cells in the control, H2O2, CHBP, or CHBP post H2O2 stimulation group was presented (Figure 7A). The percentage of survival cells (Annexin V-/PI-) was decreased by H2O2 treatment compared with the control groups with or without CHBP treatment, but effectively reversed by CHBP (88.15 ± 0.57 vs. 43.23 ± 2.88, P < 0.01, Figure 7B). Both the early apoptotic cells (Annexin V+/PI-) and later apoptotic cells (Annexin V+/PI+) were increased, respectively, by H2O2 treatment compared with the control group and the CHBP group (Figures 7C,D), but reduced by CHBP almost to the baseline level (1.85 ± 0.22 vs. 6.67 ± 0.13, 7.88 ± 0.61 vs. 45.55, both P < 0.01).
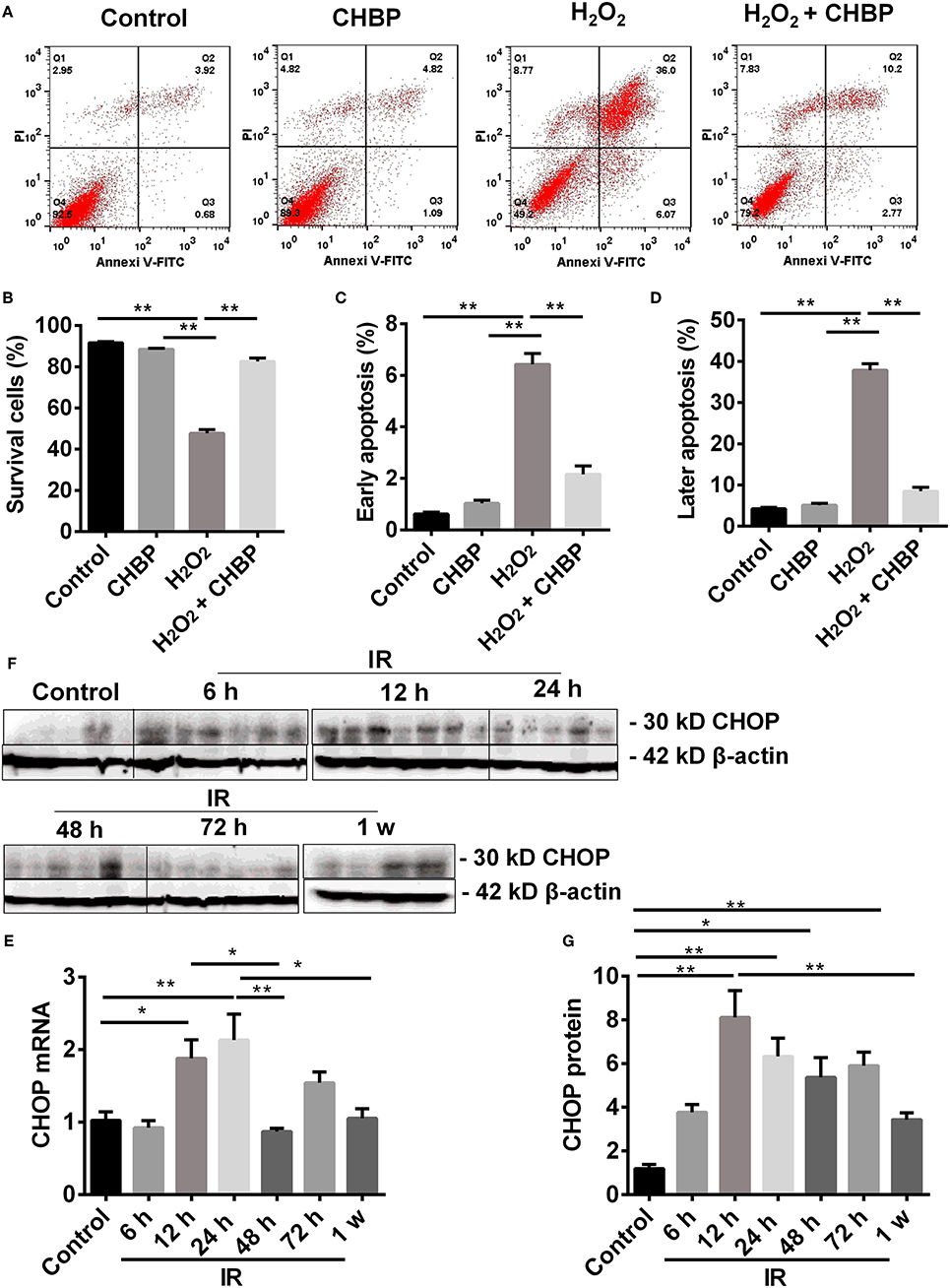
Figure 7. Cell apoptosis analyzed by flow cytometry and CHOP protein detected by western blot. (A,B: Annexin V-FITC-/PI-) The percentage of living cells was decreased by H2O2, but reversed by CHBP. (A,C: Annexin V +/PI-; A,D: Annexin V +/PI+) The early apoptotic cells, as well as later apoptotic cells were increased, respectively, by H2O2, but reduced by CHBP. (E–G) The dynamic expression of CHOP at mRNA and protein level. (E,F) CHOP protein was significantly raised and picked at 12 h. (G) CHOP mRNA was also significantly increased at 12 h and peaked at 24 h after IR injury. Both CHOP protein and mRNA were decreased at 1 week. Data are expressed as mean ± SEM (n = 3). The volume density of western blots was corrected by against 42 kD β-actin as a loading control. *P < 0.05, **P < 0.01.
Dynamic Change of CHOP at Different Time Post IR Injury
The expression of CHOP mRNA was significantly increased from 12 h after IR injury, peaked at 24 h, and then significantly decreased at 48 h compared with both 12 and 24 h, and also at 1 week compared with 24 h. CHOP protein was also significantly raised at 12, 24, 48, and 72 h compared with the control with a peak at 12 h, and decreased at 1 week compared with 12 h (P < 0.05 or 0.01, Figures 7E–G).
Discussion
The role and its underlying mechanism of ERS in IR-related injury and CHBP-induced renoprotection have been well-investigated in this study using in vitro and in vivo models. The expression of CHOP, a key marker of ERS, was increased in IR-related and IR injury. In addition, the intervening CHOP using siRNA reduced CHOP expression at both mRNA and protein level, subsequent decreased caspase-3 and HMGB-1 protein, and early apoptosis. Furthermore, CHBP treatment significantly improved ERS, in terms of decreased CHOP expression in TCMK-1 cells and kidneys subjected to IR-related and IR injury, as well as its regulators PERK and JNK, apoptotic and inflammatory mediator HMGB-1, and early and later apoptosis in vitro. Therefore, the key role of ERS in IR-related injury and CHBP induced renoprotection was proved in both mouse TCMK-1 cells and kidneys, in which its mechanistic signaling pathway was associated with CHOP/PERK/JNK, HMGB-1/caspase-3, and apoptosis (Figure 8).
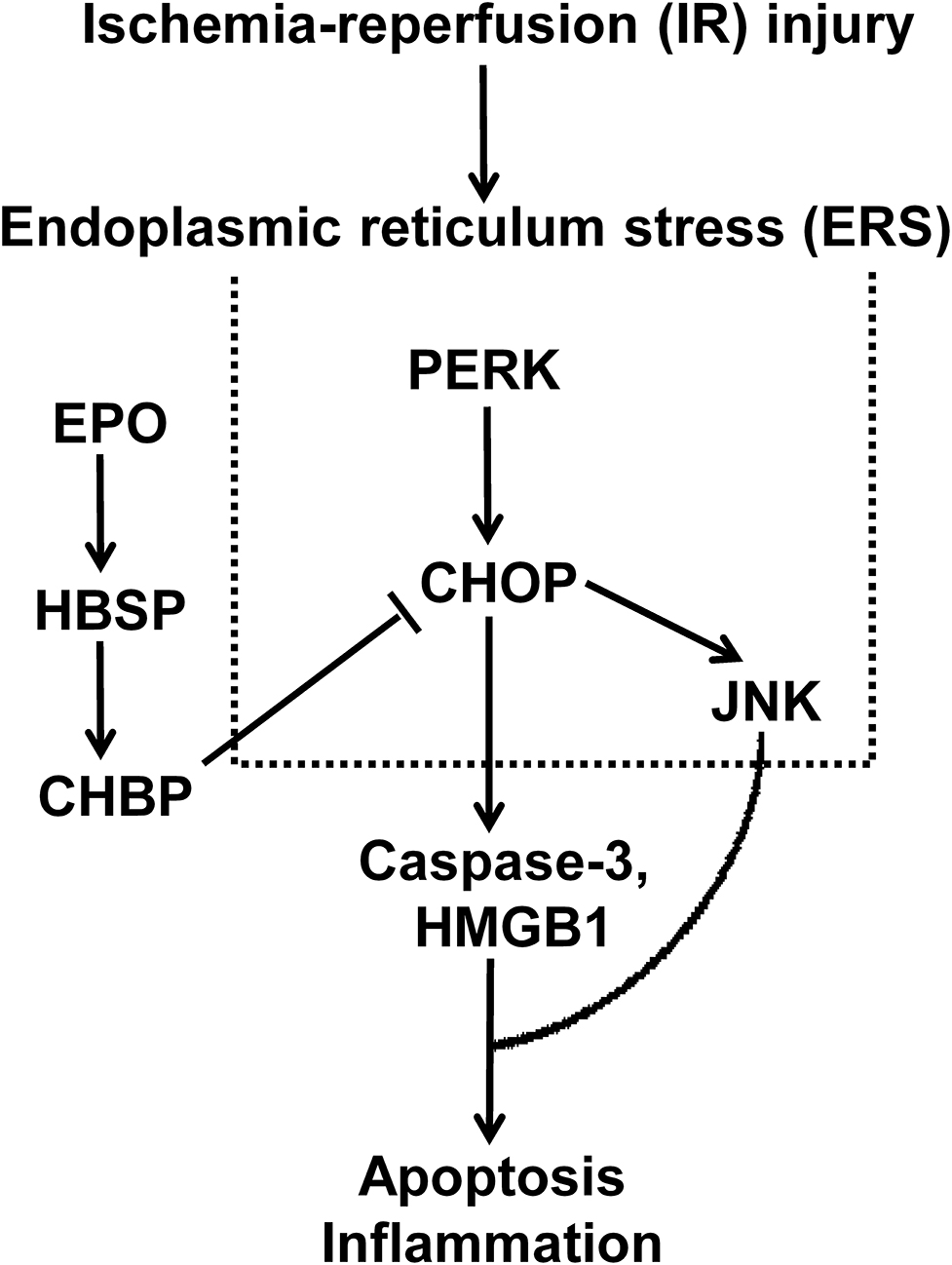
Figure 8. The schematic illustration shows that the key role of ERS in IR-related injury, and CHBP-incuced renoprotection was associated with CHOP/PERK/JNK, HMGB-1/caspase-3, and apoptosis.
The dynamic change of CHOP and PERK mRNA was evaluated in TCMK-1 at different time points after H2O2 stimulation. The consequential increase of these key parameters was observed, which reflected the gradual occurrence of ERS. The expression of CHOP protein was also increased significantly with time from 8 to 12 h compared with the control and 4 h. The change of CHOP at mRNA level represents transcriptional change, while that of CHOP at protein level represents translational change. The divergence of both at 4 h reflected differential regulations between transcription and translation. In addition, the temporary decline of CHOP protein at 4 h may be due to consumption involved in apoptosis, a self-protective regulation of cells, which subsequently stimulated the increase of CHOP at mRNA. It has been confirmed that 500 μmol/L H2O2 for stimulation over a period of 12 h were the most suitable experimental condition for the following investigation.
CHOP is a key player in ERS-mediated apoptotic pathway (20, 39). In response to severe ERS, CHOP activates the expression of Bim, leading to caspase-3 cleavage and apoptosis (40). Caspase-3 associated with apoptosis and inflammation involved in renal IR-related and IR injury. The precursor of caspase-3 could be cleaved into 17 and 12 kD subunits, both of which contribute to caspase-3 activity (34, 37, 41, 42). The changes of CHOP activate the ER-associated caspase cascades, including caspase-12 and caspases-3 (37). Therefore, we further investigate the effect of CHOP intervention by its siRNA on apoptotic and inflammatory mediator caspase-3 and HMGB-1, and apoptosis in renal IR-related injury. CHOP siRNA decreased the expression of CHOP mRNA and protein, as well as caspase-3 mRNA, 32 and 17 kD caspase-3, and HMGB-1 protein. Moreover, the number of early apoptotic cells was also reduced by CHOP siRNA compared to the H2O2 and NC siRNA groups, and the cell survival was improved. These data were consistent to the CHOP−/− knockout model in vivo (21, 43, 44). Furthermore, the correlation analysis revealed that CHOP mRNA was positively correlated with PERK, 17 kD active caspase-3 and HMGB-1, and apoptosis, but negatively related to cell viability. These results were in accordance with some other studies (40, 45, 46). Therefore, our data convincingly proved that CHOP was involved in the process of apoptosis, especially in its early stage, in renal IR-related injury.
The decreased cell viability upon H2O2 stimulation, in addition, was significantly reversed by CHBP treatment time-dependently, which confirmed the cellular protection of CHBP. More interestingly, CHBP also reduced CHOP mRNA and protein expression in TCMK-1 cells, while increased CHOP protein expression in the IR kidneys at prolonged time points was also decreased by CHBP treatment at 2 weeks, even though not at 8 weeks. Therefore, our data demonstrated that CHOP involved in renal IR-related and IR injuries, and CHBP-induced renoprotection might be through regulating CHOP or improving ERS. However, when UPR response cannot restore the homeostasis of endoplasmic reticulum upon IR injury, PERK pathway could trigger off downstream apoptosis via the activation of CHOP, caspase-12 and Bcl-2 family (44, 47, 48). Additional correlation analysis revealed that CHOP protein was positively correlated with the level of TID and active caspase-3 protein at 2 weeks. A trend of positive correlation between CHOP protein and interstitial fibrosis, apoptosis was also revealed at 2 weeks. But because the sample size is insufficient, it was not reached statistical significance. Therefore, CHBP might be renoprotective against IR-induced apoptosis and tubular damage mediated by CHOP, which could be more effective at 2 weeks than 8 weeks, as the level of CHOP could be alleviated with the time post IR injury naturally. These results also indicate that the efficacy of CHBP renoprotection might depend on the level of CHOP in certain degree, the higher of ERS, the better of CHBP renoprotection. In order to study the underlying relationship between CHBP and ERS, the changes in two main up and down-stream regulators of CHOP, PERK, and JNK, were further explored. PERK mRNA and protein, and JNK protein, were all increased significantly in the TCMK-1 cells stimulated by H2O2, but was reversed by CHBP treatment. These results imply that the renoprotection of CHBP was attributed to the improvement of CHOP associated ERS, especially at the early stage of renal IR injury. Furthermore, both early and later apoptotic cells increased by H2O2 stimulation all reversed by CHBP treatment that was more potent than CHOP siRNA. However, there was no impact of CHPB on apoptosis at baseline level. The change of HMGB-1 protein (49), a mediator of apoptosis and inflammation, has a similar trend with that of CHOP, PERK, and JNK in vitro. These results revealed that the protective effects of CHBP were related to not only ERS improvement, but also apoptotic and inflammatory mediator, and apoptosis. To confirm whether ERS plays key roles at the early stage of IR injury, we established a time course model with reperfusion at 6, 12, 24, 48, 72 h, and 1 week. The dynamic change of CHOP showed that the expression of CHOP mRNA and protein was significantly increased as early as 12 h post IR injury peaked at 24 and 12 h respectively, and then both decreased at 1 week. Therefore, the intervention at early time points is worth to be further investigated.
In conclusion, ERS and CHBP play differential roles in IR-related injury in TCMK-1 cells and kidneys. The mechanistic signaling pathway is associated with CHOP/PERK/JNK, HMGB-1/caspase-3, and apoptosis. This study, therefore, indicates that CHOP might be a potential biomarker for diagnosis and treatment of IR-induced acute kidney injury, and CHBP might also have therapeutic application clinically.
Data Availability Statement
The datasets generated for this study are available on request to the corresponding author.
Ethics Statement
The animal study was reviewed and approved by Ethics committee of affiliated hospital of Nantong University.
Author Contributions
BY, YF, and TZ conceived and designed the study. YZ, QW, AL, YW, FL, and HW acquired, analyzed, and interpreted the data. YZ and BY wrote the paper. BY final approval of submitted version. All authors participated in the design, interpretation of the studies and analysis of the data and review of the manuscript.
Funding
This research was funded by the National Natural Science Foundation of China (81170689, 81570677, and 81873622 to BY), and the Science and Technology Projects of Nantong (MS12015117 to YZ).
Conflict of Interest
The authors declare that the research was conducted in the absence of any commercial or financial relationships that could be construed as a potential conflict of interest.
Acknowledgments
We would like to acknowledge the Basic Medical Research Center of Nantong University for providing all facilities to accommodate this study. In addition, we thank Professor Yaqiu Long (Key Laboratory of Receptor Research, Shanghai Institute of Materia Medica, Chinese Academy of Sciences) for providing CHBP for this study.
References
1. Lobb I, Davison M, Carter D, Liu W, Haig A, Gunaratnam L, et al. Hydrogen sulfide treatment mitigates renal allograft ischemia-reperfusion injury during cold storage and improves early transplant kidney function and survival following allogeneic renal transplantation. J Urol. (2015) 194:1806–15. doi: 10.1016/j.juro.2015.07.096
2. Chatauret N, Badet L, Barrou B, Hauet T. Ischemia-reperfusion: from cell biology to acute kidney injury. Prog Urol. (2014) 24 (Suppl. 1):S4–12. doi: 10.1016/S1166-7087(14)70057-0
3. Chiang WC, Huang YC, Fu TI, Chen PM, Chang FC, Lai CF, et al. Angiopoietin 1 influences ischemic reperfusion renal injury via modulating endothelium survival and regeneration. Mol Med. (2019) 25:5. doi: 10.1186/s10020-019-0072-7
4. Dong G, Liu Y, Zhang L, Huang S, Ding HF, Dong Z. mTOR contributes to ER stress and associated apoptosis in renal tubular cells. Am J Physiol Renal Physiol. (2015) 308:F267–74. doi: 10.1152/ajprenal.00629.2014
5. Li L, Wang ZV, Hill JA, Lin F. New autophagy reporter mice reveal dynamics of proximal tubular autophagy. J Am Soc Nephrol. (2014) 25:305–15. doi: 10.1681/ASN.2013040374
6. Rong S, Hueper K, Kirsch T, Greite R, Klemann C, Mengel M, et al. Renal PKC-epsilon deficiency attenuates acute kidney injury and ischemic allograft injury via TNF-alpha-dependent inhibition of apoptosis and inflammation. Am J Physiol Renal Physiol. (2014) 307:F718–26. doi: 10.1152/ajprenal.00372.2013
7. Wadosky KM, Shourideh M, Goodrich DW, Koochekpour S. Riluzole induces AR degradation via endoplasmic reticulum stress pathway in androgen-dependent and castration-resistant prostate cancer cells. Prostate. (2019) 79:140–50. doi: 10.1002/pros.23719
8. Wang J, Wen Y, Lv LL, Liu H, Tang RN, Ma KL, et al. Involvement of endoplasmic reticulum stress in angiotensin II-induced NLRP3 inflammasome activation in human renal proximal tubular cells in vitro. Acta Pharmacol Sin. (2015) 36:821–30. doi: 10.1038/aps.2015.21
9. Senft D, Ronai ZA. UPR, autophagy, and mitochondria crosstalk underlies the ER stress response. Trends Biochem Sci. (2015) 40:141–8. doi: 10.1016/j.tibs.2015.01.002
10. Ozkok A, Ravichandran K, Wang Q, Ljubanovic D, Edelstein CL. NF-kappaB transcriptional inhibition ameliorates cisplatin-induced acute kidney injury (AKI). Toxicol Lett. (2016) 240:105–13. doi: 10.1016/j.toxlet.2015.10.028
11. Pressly JD, Hama T, Brien SO, Regner KR, Park F. TRIP13-deficient tubular epithelial cells are susceptible to apoptosis following acute kidney injury. Sci Rep. (2017) 7:43196. doi: 10.1038/srep43196
12. Simon N, Hertig A. Alteration of fatty acid oxidation in tubular epithelial cells: from acute kidney injury to renal fibrogenesis. Front Med. (2015) 2:52. doi: 10.3389/fmed.2015.00052
13. Cao SS. Endoplasmic reticulum stress and unfolded protein response in inflammatory bowel disease. Inflamm Bowel Dis. (2015) 21:636–44. doi: 10.1097/MIB.0000000000000238
14. Hetz C. The unfolded protein response: controlling cell fate decisions under ER stress and beyond. Nat Rev Mol Cell Biol. (2012) 13:89–102. doi: 10.1038/nrm3270
15. O'Hayre M, Vazquez-Prado J, Kufareva I, Stawiski EW, Handel TM, Seshagiri S, et al. The emerging mutational landscape of G proteins and G-protein-coupled receptors in cancer. Nat Rev Cancer. (2013) 13:412–24. doi: 10.1038/nrc3521
16. Hottenrott C. Next-generation, genome- and mutational landscape heterogeneity-based novel biomarkers for personalized neoadjuvant treatment and laparoscopic rectal cancer resection. Surg Endosc. (2013) 27:1441–3. doi: 10.1007/s00464-012-2570-3
17. Sommerweiss D, Gorski T, Richter S, Garten A, Kiess W. Oleate rescues INS-1E β-cells from palmitate-induced apoptosis by preventing activation of the unfolded protein response. Biochem Biophys Res Commun. (2013) 441:770–6. doi: 10.1016/j.bbrc.2013.10.130
18. Lv Z, Liu C, Zhai M, Zhang Q, Li J, Zheng F, et al. LPS pretreatment attenuates cerebral ischaemia/reperfusion injury by inhibiting inflammation and apoptosis. Cell Physiol Biochem. (2018) 45:2246–56. doi: 10.1159/000488170
19. Yang JR, Yao FH, Zhang JG, Ji ZY, Li KL, Zhan J, et al. Ischemia-reperfusion induces renal tubule pyroptosis via the CHOP-caspase-11 pathway. Am J Physiol Renal Physiol. (2014) 306:F75–84. doi: 10.1152/ajprenal.00117.2013
20. Mei S, Livingston M, Hao J, Li L, Mei C, Dong Z. Autophagy is activated to protect against endotoxic acute kidney injury. Sci Rep. (2016) 6:22171. doi: 10.1038/srep22171
21. Tian H, Li Y, Kang P, Wang Z, Yue F, Jiao P, et al. Endoplasmic reticulum stress-dependent autophagy inhibits glycated high-density lipoprotein-induced macrophage apoptosis by inhibiting CHOP pathway. J Cell Mol Med. (2019) 23:2954–69. doi: 10.1111/jcmm.14203
22. Guo G, Meng Y, Tan W, Xia Y, Cheng C, Chen X, et al. Induction of apoptosis coupled to endoplasmic reticulum stress through regulation of CHOP and JNK in bone marrow mesenchymal stem cells from patients with systemic lupus erythematosus. J Immunol Res. (2015) 2015:183738. doi: 10.1155/2015/183738
23. Wolberg AS, Cushman M. Illustrated review article: a new format for disseminating scientific progress. Res Pract Thromb Haemost. (2018) 2:405–06. doi: 10.1002/rth2.12124
24. Miyazaki Y, Kaikita K, Endo M, Horio E, Miura M, Tsujita K, et al. C/EBP homologous protein deficiency attenuates myocardial reperfusion injury by inhibiting myocardial apoptosis and inflammation. Arterioscler Thromb Vasc Biol. (2011) 31:1124–32. doi: 10.1161/ATVBAHA.111.224519
25. Aronson JP, Katnani HA, Pomerantseva I, Shapir N, Tse H, Miari R, et al. Sustained intrathecal therapeutic protein delivery using genetically transduced tissue implants in a freely moving rat model. Int J Pharm. (2017) 534:42–9. doi: 10.1016/j.ijpharm.2017.10.002
26. van Rijt WG, van Goor H, Ploeg RJ, Leuvenink HG. Erythropoietin-mediated protection in kidney transplantation: nonerythropoietic EPO derivatives improve function without increasing risk of cardiovascular events. Transpl Int. (2014) 27:241–8. doi: 10.1111/tri.12174
27. Uversky VN, Redwan EM. Erythropoietin and co.: intrinsic structure and functional disorder. Mol Biosyst. (2016) 13:56–72. doi: 10.1039/C6MB00657D
28. Zhang Y, Chen W, Wu Y, Yang B. Renoprotection and mechanisms of erythropoietin and its derivatives helix b surface peptide in kidney injuries. Curr Protein Pept Sci. (2017) 18:1183–90. doi: 10.2174/1389203717666160909144436
29. Yang B, Hosgood SA, Bagul A, Waller HL, Nicholson ML. Erythropoietin regulates apoptosis, inflammation and tissue remodelling via caspase-3 and IL-1beta in isolated hemoperfused kidneys. Eur J Pharmacol. (2011) 660:420–30. doi: 10.1016/j.ejphar.2011.03.044
30. Hu L, Yang C, Zhao T, Xu M, Tang Q, Yang B, et al. Erythropoietin ameliorates renal ischemia and reperfusion injury via inhibiting tubulointerstitial inflammation. J Surg Res. (2012) 176:260–6. doi: 10.1016/j.jss.2011.06.035
31. Forbes JA, Reig AS, Tomycz LD, Tulipan N. Intracranial hypertension caused by a depressed skull fracture resulting in superior sagittal sinus thrombosis in a pediatric patient: treatment with ventriculoperitoneal shunt insertion. J Neurosurg Pediatr. (2010) 6:23–8. doi: 10.3171/2010.3.PEDS09441
32. Brines M, Patel NS, Villa P, Brines C, Mennini T, De Paola M, et al. Nonerythropoietic, tissue-protective peptides derived from the tertiary structure of erythropoietin. Proc Natl Acad Sci USA. (2008) 105:10925–30. doi: 10.1073/pnas.0805594105
33. Yang C, Hosgood SA, Meeta P, Long Y, Zhu T, Nicholson ML, et al. Cyclic helix B peptide in preservation solution and autologous blood perfusate ameliorates ischemia-reperfusion injury in isolated porcine kidneys. Transplant Direct. (2015) 1:e6. doi: 10.1097/TXD.0000000000000515
34. Wu Y, Zhang J, Liu F, Yang C, Zhang Y, Liu A, et al. Protective effects of HBSP on ischemia reperfusion and cyclosporine a induced renal injury. Clin Dev Immunol. (2013) 2013:758159. doi: 10.1155/2013/758159
35. Li L, Lin M, Zhang L, Huang S, Hu C, Zheng L, et al. Cyclic helix B peptide protects HK2 cells from oxidative stress by inhibiting ER stress and activating Nrf2 signalling and autophagy. Mol Med Rep. (2017) 16:8055–61. doi: 10.3892/mmr.2017.7588
36. Liu A, Wu J, Yang C, Wu Y, Zhang Y, Zhao F, et al. TRPM7 in CHBP-induced renoprotection upon ischemia reperfusion-related injury. Sci Rep. (2018) 8:5510. doi: 10.1038/s41598-018-22852-2
37. Yang C, Zhang Y, Wang J, Li L, Wang L, Hu M, et al. A novel cyclic helix B peptide inhibits dendritic cell maturation during amelioration of acute kidney graft rejection through Jak-2/STAT3/SOCS1. Cell Death Dis. (2015) 6:e1993. doi: 10.1038/cddis.2015.338
38. Wang L, Mo Q, Wang J. MIrExpress: a database for gene coexpression correlation in immune cells based on mutual information and pearson correlation. J Immunol Res. (2015) 2015:140819. doi: 10.1155/2015/140819
39. Shu S, Zhu J, Liu Z, Tang C, Cai J, Dong Z. Endoplasmic reticulum stress is activated in post-ischemic kidneys to promote chronic kidney disease. EBioMedicine. (2018) 37:269–80. doi: 10.1016/j.ebiom.2018.10.006
40. Decuypere JP, Ceulemans LJ, Agostinis P, Monbaliu D, Naesens M, Pirenne J, et al. Autophagy and the kidney: implications for ischemia-reperfusion injury and therapy. Am J Kidney Dis. (2015) 66:699–709. doi: 10.1053/j.ajkd.2015.05.021
41. Yang C, Xu Z, Zhao Z, Li L, Zhao T, Peng D, et al. A novel proteolysis-resistant cyclic helix B peptide ameliorates kidney ischemia reperfusion injury. Biochim Biophys Acta. (2014) 1842:2306–17. doi: 10.1016/j.bbadis.2014.09.001
42. Yang C, Zhao T, Lin M, Zhao Z, Hu L, Jia Y, et al. Helix B surface peptide administered after insult of ischemia reperfusion improved renal function, structure and apoptosis through beta common receptor/erythropoietin receptor and PI3K/Akt pathway in a murine model. Exp Biol Med. (2013) 238:111–9. doi: 10.1258/ebm.2012.012185
43. Shang L, Dong P, Du L, Yang X, Wang H, Li S. SERP1 prevents hypoxia-reoxygenation-induced H9c2 apoptosis through activating JAK2/STAT3 pathway-dependent attenuation of endoplasmic reticulum stress. Biochem Biophys Res Commun. (2019) 508:256–62. doi: 10.1016/j.bbrc.2018.11.119
44. Choi AY, Choi JH, Hwang KY, Jeong YJ, Choe W, Yoon KS, et al. Correction to: licochalcone A induces apoptosis through endoplasmic reticulum stress via a phospholipase Cgamma1-, Ca(2+)-, and reactive oxygen species-dependent pathway in HepG2 human hepatocellular carcinoma cells. Apoptosis. (2019) 24:200–03. doi: 10.1007/s10495-018-1493-4
45. Bhavsar D, Subramanian K, Sethuraman S, Krishnan UM. Translational siRNA therapeutics using liposomal carriers: prospects & challenges. Curr Gene Ther. (2012) 12:315–32. doi: 10.2174/156652312802083611
46. Coselli JS, Amarasekara HS, Green SY, Price MD, Preventza O, de la Cruz KI, et al. Open repair of thoracoabdominal aortic aneurysm in patients 50 years old and younger. Ann Thorac Surg. (2017) 103:1849–57. doi: 10.1016/j.athoracsur.2016.09.058
47. Melk A, Baisantry A, Schmitt R. The yin and yang of autophagy in acute kidney injury. Autophagy. (2016) 12:596–7. doi: 10.1080/15548627.2015.1135284
48. Yang TY, Wu YJ, Chang CI, Chiu CC, Wu ML. The effect of bornyl cis-4-hydroxycinnamate on melanoma cell apoptosis is associated with mitochondrial dysfunction and endoplasmic reticulum stress. Int J Mol Sci. (2018) 19:1370. doi: 10.3390/ijms19051370
Keywords: apoptosis, CHOP, cyclic helix-B surface peptide (CHBP), endoplasmic reticulum stress (ERS), inflammation, ischemia-reperfusion injury
Citation: Zhang Y, Wang Q, Liu A, Wu Y, Liu F, Wang H, Zhu T, Fan Y and Yang B (2020) Erythropoietin Derived Peptide Improved Endoplasmic Reticulum Stress and Ischemia-Reperfusion Related Cellular and Renal Injury. Front. Med. 7:5. doi: 10.3389/fmed.2020.00005
Received: 01 November 2019; Accepted: 08 January 2020;
Published: 24 January 2020.
Edited by:
Songjie Cai, Brigham and Women's Hospital, United StatesReviewed by:
Matsuda Yoshiko, University of California, San Francisco, United StatesRyoichi Imamura, Osaka University, Japan
Copyright © 2020 Zhang, Wang, Liu, Wu, Liu, Wang, Zhu, Fan and Yang. This is an open-access article distributed under the terms of the Creative Commons Attribution License (CC BY). The use, distribution or reproduction in other forums is permitted, provided the original author(s) and the copyright owner(s) are credited and that the original publication in this journal is cited, in accordance with accepted academic practice. No use, distribution or reproduction is permitted which does not comply with these terms.
*Correspondence: Bin Yang, by5@le.ac.uk; dryangbin@hotmail.com
†These authors have contributed equally to this work