- Department of Radiation Oncology, Ghent University Hospital and Ghent University, Ghent, Belgium
For many years, conventional oncologic treatments such as surgery, chemotherapy, and radiotherapy (RT) have dominated the field of non-small-cell lung cancer (NSCLC). The recent introduction of immunotherapy (IT) in clinical practice, especially strategies targeting negative regulators of the immune system, so-called immune checkpoint inhibitors, has led to a paradigm shift in lung cancer as in many other solid tumors. Although antibodies against programmed death protein-1 (PD-1) and programmed death ligand-1 (PD-L1) are currently on the forefront of the immuno-oncology field, the first efforts to eradicate cancer by exploiting the host's immune system date back to several decades ago. Even then, researchers aimed to explore the addition of RT to IT strategies in NSCLC patients, attributing its potential benefit to local control of target lesions through direct and indirect DNA damage in cancer cells. However, recent pre-clinical and clinical data have shown RT may also modify antitumor immune responses through induction of immunogenic cell death and reprogramming of the tumor microenvironment. This has led many to reexamine RT as a partner therapy to immuno-oncology treatments and investigate their potential synergy in an exponentially growing number of clinical trials. Herein, the authors review the rationale of combining IT and RT across all NSCLC disease stages and summarize both historical and current clinical evidence surrounding these combination strategies. Furthermore, an overview is provided of active clinical trials exploring the IT-RT concept in different settings of NSCLC.
Introduction
Radiotherapy (RT) has earned its place as one of three main pillars in non-small cell lung cancer (NSCLC) treatment, alongside surgery and systemic agents. Traditionally considered as a means of achieving local tumor control through induction of irreversible deoxyribonucleic acid (DNA) damage in irradiated tumor cells, RT is used in routine clinical practice across all NSCLC disease stages, whether with curative or palliative intent (1). However, since as early as 1953, reports have been published describing tumor regression outside the radiation fields (2). This so-called “abscopal effect” has more recently been postulated to be the result of a RT-induced antitumor immune response. With the advent of modern immunotherapy (IT), the potential for immune activation by RT has become even more relevant. Indeed, mounting pre-clinical and clinical evidence suggests a potential synergy between RT and IT, creating opportunities for combining these two treatment strategies, in NSCLC as in many other tumor types. In order to harness these synergistic effects however, it is important to understand the underlying mechanisms in which key factors such as the type of IT used, the irradiated volume, as well as timing, dose and fractionation of RT play a crucial role. Herein, we review the rationale for combining IT and RT across different NSCLC disease stages and summarize the current clinical evidence surrounding these novel treatment approaches. Furthermore, an overview is provided of active clinical trials exploring the IT-RT concept in different settings of NSCLC.
For this review, we conducted a search of PubMed, Embase and Web of Science for original research, review articles and meta-analyses relevant to the combination of RT and IT in NSCLC from inception until March 2019, yielding a total reference count of 708. After removal of duplicates, 394 abstracts were screened by one reviewer, of which 62 qualified for full text screening. In case of multiple publications reporting on the same study population, manuscripts with the longest follow-up were selected for inclusion. The cited and citing references of the included studies were checked for additional relevant publications. Finally, a total of 42 published original research papers and abstracts were included and their results will be discussed below. Furthermore, using the search terms “radiotherapy,” “immunotherapy,” “immune,” “vaccine,” and “checkpoint,” the international clinical study database Clinicaltrials.gov was queried for currently active trials in the area of NSCLC combining both treatment modalities.
Immunologic Effects of Radiotherapy
It has been over a decade since new insights into the complex interplay between cancer and the host's immune system, known as the cancer immunoediting hypothesis (3), have revolutionized our understanding and approach to this disease. Besides sparking interest in the development of novel immunotherapeutic drugs targeting different aspects of the so-called cancer-immunity cycle (4), it also prompted researchers to reassess the role of conventional oncological therapies—most notably RT—in anticancer immunity.
In-situ Vaccination
The principal mechanism of action of ionizing radiation is the induction of irreparable DNA damage in tumor cells—either directly or indirectly through free radicals. Under the right circumstances, radiation-damaged tumor cells may in turn undergo a phenomenon called “immunogenic cell death,” whereby an increased expression of calreticulin facilitates their phagocytosis by dendritic cells (DCs) and promotes the secretion of pro-inflammatory cytokines (Figure 1) (5). In addition, radiation-induced DNA damage leads to the accumulation of cytosolic DNA, which stimulates the production of type I interferons (IFN-I) through cyclic guanosine monophosphate-adenosine monophosphate synthase (cGAS)/stimulator of IFN genes (STING) nucleic acid-sensing pathways (6–8). RT also triggers the release of several other danger-associated molecular patterns, including adenosine triphosphate and high mobility group box 1, which together with IFN-I, prompt DC recruitment and activation (5, 9). After subsequently migrating to the tumor-draining lymph node, DCs will present tumor-associated antigen (TAA) to cluster of differentiation 8 positive (CD8+) T-cells so that cross-priming and activation of these cytotoxic T-cells can occur (10, 11). T-cell trafficking back to the tumor microenvironment is aided by radiation-induced chemokines such as C-X-C chemokine ligand 16 (CXCL16) by the tumor and intercellular (ICAM) and vascular cell adhesion molecules expression by the endothelial cells (12, 13). There, cytotoxic T lymphocytes will meet residual irradiated tumor cells that show increased expression of major histocompatibility complex class I (MHC-I), Fas and natural killer group 2, member D ligands, thus rendering them more sensitive to cell killing (14–16). In theory, these TAA-specific T-cells could also home to cancerous lesions outside of the radiation field, thereby leading to abscopal responses.
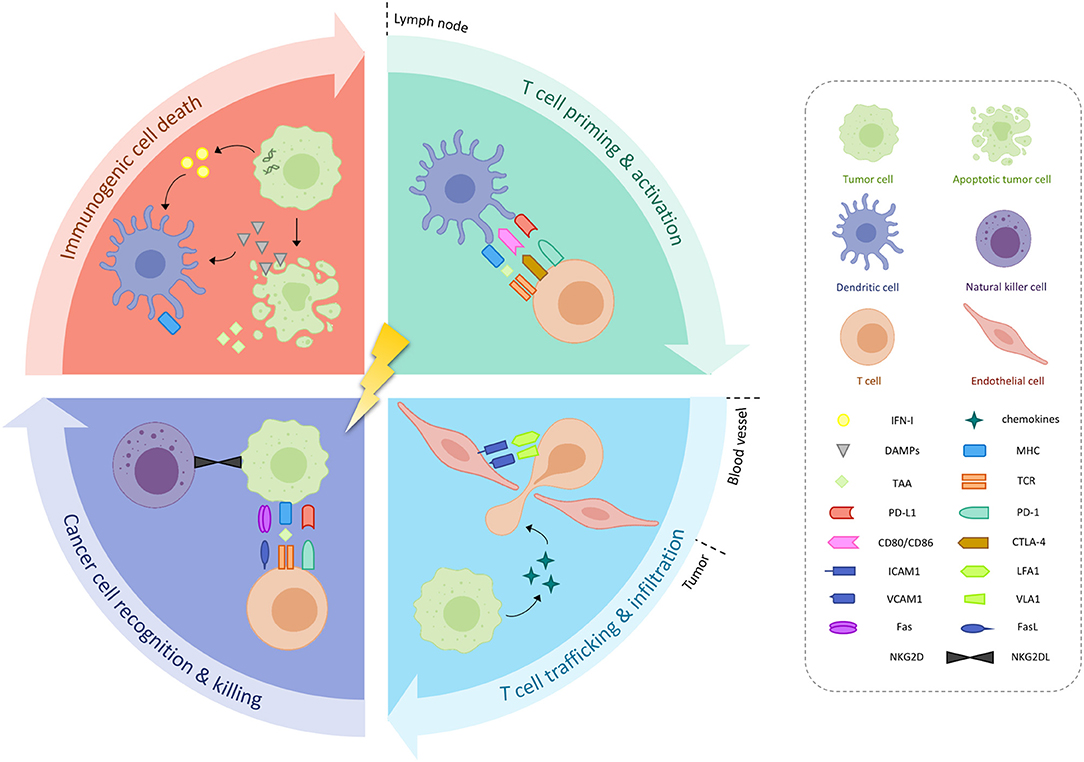
Figure 1. Immunological effects or radiotherapy. Radiotherapy may induce immunogenic cancer cell death, characterized by increased expression of danger-associated molecular patterns (DAMPs) and type I interferon (IFN-I), in turn causing the release of tumor-associated antigens (TAAs). Activated dendritic cells (DCs) will present these TAAs to T-cells located in the tumor-draining lymph node, which also carry inhibitory receptors programmed death protein 1 (PD-1) and cytotoxic T-lymphocyte-associated antigen 4 (CTLA-4) on their cell surface. T-cell homing back to the tumor microenvironment is aided by radiation-induced chemokines, as well as upregulation of intercellular (ICAM) and vascular cell adhesion molecules (VCAM) on endothelial cells. Increased expression of major histocompatibility complex (MHC), Fas and natural killer group 2, member D (NKG2D) by residual irradiated tumor cells facilitates their destruction. CD, cluster of differentiation; L, ligand; LFA1, lymphocyte function associated antigen 1; PD-L1, programmed death ligand-1; TCR, T-cell receptor; VLA1, integrin alpha 1.
The Role of RT Dose
It has been stipulated that RT in conventional dose-fractionation regimens [i.e., 1.8–2 Gray (Gy) per fraction] may elicit profound immunosuppressive responses in tumors. Such effects include recruitment of notoriously pro-tumorigenic myeloid-derived suppressor cells (MDSCs) and M2 tumor-associated macrophages (TAM), as well as a preferential increase of the regulatory T-cell (Treg) population, either independently, due to their intrinsic radioresistance or as a consequence of RT-induced upregulation of transforming growth factor beta (3, 17–20). Conversely, 2 Gy daily RT fractions may also have the potential to boost antitumor immune responses through in-situ vaccination, as demonstrated by the detection of TAA-specific CD8+ T-cells in the circulation of colorectal (21) and prostate cancer patients (22) receiving standard (chemo)radiation. Nevertheless, in pre-clinical experiments comparing immunologic effects of conventional RT doses to those of hypofractionated regimens, more specifically if ≥6 Gy per fraction is being delivered, or even single high-dose radiation, profound differences are observed (9). For example, Reits et al. showed that the expression of MHC-I and associated tumor peptides was higher with increasing RT doses (16). Other reports demonstrate that higher doses may lead to greater upregulation of other stimulatory immune signals such as Fas and ICAM, as well as enhanced tumor-specific CD8+ T-cell infiltration (23, 24).
Interestingly, the enhanced immunogenicity of increasing radiation doses does not seem to extend beyond a certain dose range (24). This could be explained in part by the induction of DNA exonuclease Trex1 at doses larger than 18–20 Gy per fraction, which degrades cytosolic DNA, thus preventing activation of the cGAS/STING pathway and thereby abrogating a potential antitumor immune response (8).
The Role of RT Timing and Sequencing
Immunologic effects of RT may not only be sensitive to variations in dose and fractionation, they also appear to be time-dependent. For instance, in vitro data demonstrate an increase in the MHC-I-associated peptide pool after approximately 8 h and up to 11 days or more following high doses of radiation (16). Kinetics of RT-induced intratumoral immune cell activation were also studied in vivo, particularly in cervical cancer patients treated with conventionally fractionated RT and concurrent chemotherapy (25). Repeated cervical brushings showed an enrichment of activated DCs at the tumor site during the first week of chemoradiation, potentially corresponding to treatment-induced antigen presentation. Meanwhile, the proportion of activated and proliferating T-cells experienced an initial decline in week 1, but quickly recovered and even increased in weeks 3–5, supporting the in-situ vaccination hypothesis. Interestingly, changes in the populations and/or activation status of myeloid and T-cells appeared to be more pronounced at the tumor site in comparison to peripheral blood samples. Findings such as these provide important insights into immune dynamic changes in the tumor microenvironment and could therefore further the development of rational IT-RT combination strategies, especially regarding optimal treatment sequencing.
The antitumor effects of antigen-specific immunotherapy (ASI), for instance, rely on the generation of a potent cellular immune response against a specific TAA. A prime example of this is tecemotide, a peptide vaccine containing a liposomal formulation of tumor-associated mucin 1 (MUC1), which is designed to elicit a MUC1-specific T-cell proliferative response. As the initial depletion of effector cell types from the tumor microenvironment caused by repeated moderate doses of RT could abrogate any previously induced immune infiltrate, ASI such as tecemotide may prove most useful when administered after RT, serving as a booster for the immune cells generated by in-situ vaccination. Moreover, the RT-induced expression of chemotactic signals such as CXCL16 may facilitate homing of any TAA-specific T-cells to the irradiated lesion.
Similarly, RT may be used to prime tumors in order to render them more susceptible to adoptive IT, such as lymphokine-activated killer cell (LAK), DC, cytokine-induced killer cell (CIK), tumor-infiltrating lymphocyte (TIL) and chimeric antigen receptor (CAR) T-cell therapy. Important work by Klug et al. demonstrated in murine as well as xenotransplanted and human primary tumors that even single low doses of RT ( ≤ 2 Gy) may be capable of reprogramming the tumor microenvironment—in particular polarizing TAMs to an M1 phenotype—causing inducible nitric oxide synthase-mediated vascular normalization, and thus facilitating tumor homing of transferred T-cells (26). In addition, RT may enhance cytotoxicity of CIKs through upregulation of signaling pathways required for their antitumor activity, including NK-cell receptor ligands and Fas. In turn, CIKs are known to secrete proinflammatory cytokines such as tumor necrosis factor alpha and interferon gamma (IFNɤ), thereby enriching local immune responses (27). With regards to CAR T-cell therapy, De Selm et al. demonstrated increased efficacy after previous exposure of tumors to low-dose RT in their orthotopic pancreatic cancer model (28).
Of all IT-RT combinations, treatment regimens integrating RT and immune checkpoint blockade (ICB) have garnered the most interest in recent years. Simply put, the rationale of a potential synergy between both treatments is that the inhibition of immune checkpoints, such as cytotoxic T lymphocyte antigen 4 (CTLA-4), programmed cell death protein 1 (PD-1) and programmed cell death 1 ligand 1 (PD-L1), liberates T-cells from immunosuppression, thereby increasing the in-situ vaccination effect of RT. Additional benefits of ICB in the context of RT include: depletion of intratumoral Treg cells (anti-CTLA-4), counteracting RT-induced T-cell exhaustion through upregulation of PD-L1 (anti-PD-(L)1) and a reduction of MDSC populations in the tumor microenvironment (anti-PD-(L)1) (29). RT can also lead to upregulation of PD-L1 expression in tumors, which has been shown to be mediated by IFNɤ and may be an important mechanism of radioresistance (30). Altogether, these findings suggest concurrent administration of ICB with RT would be optimal in order to exploit their full synergistic potential. Nevertheless, encouraging results have been achieved with sequential treatment regimens as well, in particular with the adjuvant use of checkpoint inhibitors to CRT. In these types of treatment schedules, timing may play an even greater role, as in the PACIFIC trial, where multivariate analysis suggested that initiation of anti-PD-L1 treatment before 2 weeks after completion of chemoradiation was correlated with better overall survival (OS) in comparison to initiation after 14 days or more (31). Nevertheless, further analysis showed that durvalumab initiated after longer time intervals still provided clinical benefit in terms of progression-free survival (PFS) and time to distant metastasis (32).
Less is known about the underlying mechanisms of interaction between RT and non-specific immunomodulatory drugs, such as levamisole, or so-called active immunotherapy (i.e., Bacillus Calmette-Guérin, BCG), making it more difficult to theorize their optimal sequencing.
The Role of RT Target Volume and Organs at Risk
As illustrated above, lymphocytes play a crucial role in antitumor immunity. Unfortunately, one of the major drawbacks of conventional oncological treatments is their tendency to cause systemic lymphopenia, which is increasingly being recognized to profoundly influence outcome. Indeed, several reports have shown radiation-induced lymphopenia (RIL) to be an independent predictor for poor survival in solid tumors (33, 34). More specifically in NSCLC, besides the obvious consideration of whether or not a patient is amenable to receive concurrent chemotherapy, the definition of RT target volumes seems to be a determining factor in the incidence of RIL. First, the sheer size of the radiation field has a direct impact on lymphocyte nadirs, as was demonstrated in a retrospective study by Tang et al. (35). This effect could be explained by taking into consideration unintentional RT doses to sites of lymphopoiesis (bone marrow) and lymphocyte storage (spleen, lymph nodes), as well as the amount of circulating immune cells passing through the radiation field (36, 37). The latter is of particular importance in thoracic RT, where organs at risk (OARs) characterized by high blood flow such as the heart and lungs reside, thus exposing a large proportion of circulating lymphocytes to radiation (33). Due to their intrinsic radiosensitivity to doses even below 1 Gy (38) large volumes irradiated to relatively low doses (i.e., the low-dose bath), inherent even to modern photon RT techniques such as intensity-modulated radiotherapy, may significantly impact the incidence of RIL. In theory, more conformal (e.g., proton therapy) and faster (e.g., higher dose rate) dose delivery could aid in limiting this exposure.
In addition to the direct hit of lymphocytes by RT, pre-clinical data suggest irradiation of the draining lymph nodes may also attenuate adaptive immune responses through altered intratumoral chemokine expression and CD8+ T-cell trafficking as compared to RT to the primary tumor alone (39). This in turn adversely affected treatment outcome when RT was combined with ICB.
Adding to the list of OARs to consider, Price et al. demonstrated irradiating major areas of skin may also have detrimental effects on antitumor immune responses. Their experiments showed RT mobilizes Langerhans cells, a DC subset present in the epidermis, which may subsequently migrate to the draining lymph node where they cause an accumulation of Treg cells (40).
On the other end of the benefit-risk balance of IT-RT strategies stands the possibility of increased toxicity of combined treatment. RT-induced immune-cell infiltration and subsequent inflammation-associated normal tissue responses underlying the occurrence of radiation-induced lung injury (i.e., radiation pneumonitis and fibrosis) warrant further inquiry into a potential interaction with immunotherapy (41). Most notably, it has been postulated that combining RT with ICB may pose a particular risk, as these drugs alone are known to cause severe, even potentially life-threatening, pneumonitis in a small subset of patients. Reports of high-grade lung toxicities with concurrent RT and anti-PD-(L)1 caution the use of such treatment schedules outside of clinical trials (42). Even so, higher rates of pneumonitis may be expected in a non-trial-enrolled population, as is the case with ICB monotherapy (43). Regardless of the setting, all available measures should be taken to minimize the incidence of RT-related pulmonary toxicity, such as careful patient and tumor selection, as well as optimal treatment planning and delivery.
Combining radiotherapy and immunotherapy in NSCLC
Locally-Advanced NSCLC
Approximately one in four new NSCLC cases are diagnosed with locally-advanced (LA-NSCLC) disease (44). In this setting, RT may be offered as an adjunct to surgery in operable patients, be it in the preoperative setting or after incomplete resection, but will most frequently be used as definitive treatment combined with chemotherapy (concurrently of sequentially) in stage IIIB or (unresectable) stage IIIA disease. When combined with platinum-based doublet chemotherapy, RT doses ranging between 60 and 66 Gy in 2 Gy daily fractions over 6–7 weeks are advocated (1). Despite many historical efforts to optimize treatment schedules, LA-NSCLC treated with concurrent chemo-radiotherapy is characterized by a poor 2-year overall and progression-free survival, typically <60 and 30% respectively, with median OS ranging between 2 and 2.5 years, even in the most recent randomized series (45, 46). Analyses of failure patterns after CRT reveal a substantial contribution of locoregional recurrence, but an even greater proportion of about 50% of patients experiencing distant progression (47). Thus, it seems neither RT's qualities in terms of local control, nor the systemic antitumor effects of chemotherapy are sufficient to offer long-term disease control in all LA-NSCLC patients. Therefore, this setting may represent an exciting opportunity for the development of innovative strategies integrating immunotherapeutic agents into combined modality treatment. This is why it is not surprising that LA-NSCLC was the first clinical entity in lung cancer in which the concept of immune modulation with RT was explored.
Available Clinical Evidence (Table 1)
The earliest clinical data on IT-RT combinations in NSCLC date back to the 1970's and 80's, when immuno-oncology was still in its infancy. At the time, experience in leveraging the immune system in order to eradicate cancer cells was mostly limited to the use of non-specific immunostimulants.
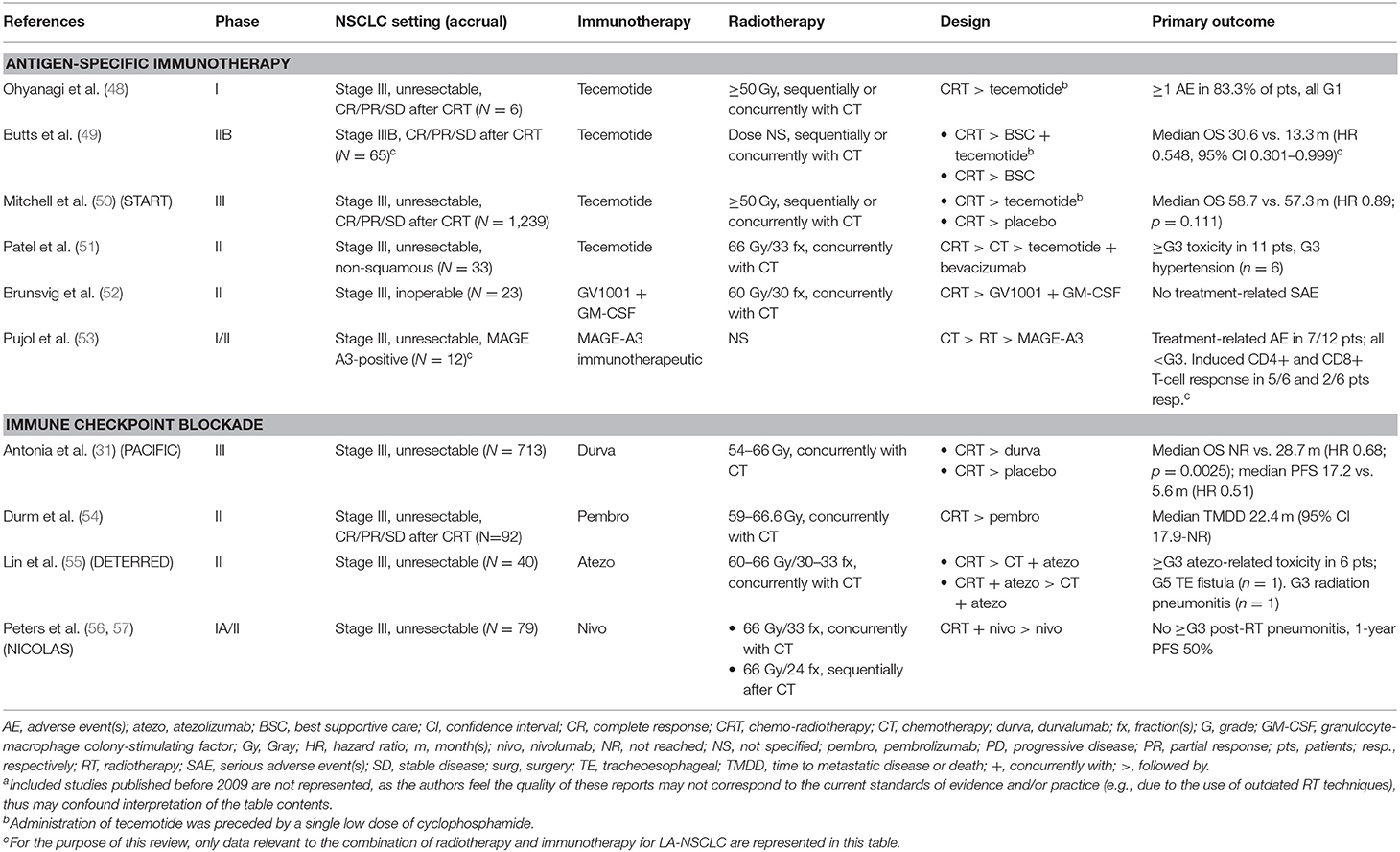
Table 1. Clinical studies evaluating immunotherapy-radiotherapy combinations in locally advanced non-small cell lung cancer (LA-NSCLC), with primary endpoint results published or presented during the last decade (2009–2019)a.
One in particular, BCG, had garnered substantial interest due to its encouraging results in hematologic malignancies, which soon inspired the development of several pre-clinical experiments and clinical studies in various other cancer types (58). Specifically in lung cancer, trials evaluating BCG or its methanol extraction residue in combination with (chemo)radiation in LA-NSCLC unfortunately failed to show any benefit in terms of survival (59–62). An interesting finding however, was that when administered during or after RT, these drugs seemed to inhibit the development of distant metastasis (59, 60), suggesting that their potential immunogenic effects may be more pronounced in the context of minimal (residual) disease.
The same allegedly applied to levamisole, a drug used as an anthelmintic before its immunotropic properties were discovered in animal cancer models. Several trials investigated levamisole in conjunction with (chemo)radiation, among which a phase III study initiated by the Radiation Therapy Oncology Group (RTOG), unfortunately showing no difference in OS and PFS compared to placebo, in both resectable and unresectable NSCLC (63–67).
Meanwhile in Japan, research efforts were aimed at combining RT with other non-specific immunopotentiators, namely Picibanil (or OK-432) and polysaccharide K (PSK or Krestin). In their non-randomized trial, Ogawa et al. demonstrated an increased survival associated with the use of OK-432 and/or PSK in addition to chemo-radiotherapy, but did not report significance (68).
A decade later, the first clinical data on experimental RT regimens integrating interferons as radiosensitizers in LA-NSCLC started to emerge. Two smaller studies showed that combining natural alpha-interferon or human recombinant IFNɤ with hyperfractionated RT (60 Gy in 1.25–1.5 Gy fractions, twice-daily) resulted in a marked increase in severe treatment-related toxicity (i.e., esophagitis, radiation pneumonitis, and fibrosis) (69, 70). While initial phase I/II results of concurrent recombinant beta-interferon and conventionally fractionated RT (60 Gy in 2 Gy fractions) were more encouraging, a phase III study initiated by the RTOG demonstrated greater rates of both acute and late side effects and failed to confirm any improvement in overall survival (71). Although interferons play a vital role in in-situ vaccination, it seems increasing systemic exposure during RT may overstimulate these pathways, thereby inducing radiation damage where it is least needed. Whether this effect could be mitigated if RT doses were delivered more precisely and conformally is an interesting hypothesis but remains unproven.
A final member of the non-specific immunomodulatory drug family, referred to as bovine dialyzable leukocyte extract, was evaluated concomitantly with chemoradiation in a phase I study, demonstrating its safety and ability to increase certain T-cell subpopulations (72).
As described above, RT and vaccine therapy may also offer the potential of synergistic effects. One of the most extensively studied ASI in LA-NSCLC is tecemotide (or L-BLP25). As maintenance treatment after chemoradiation for stage III disease, the anti-MUC1 vaccine first demonstrated an acceptable safety and tolerability profile (48). Subsequent phase II results were promising, thus warranting initiation of one of the largest phase III IT-RT studies to date (49). The START trial enrolled over 1,500 patients and randomly assigned them two to one, to receive either tecemotide or placebo within 4–12 weeks after completion of chemo-radiotherapy, with the primary aim to improve OS (73). Though this primary endpoint was not met overall, the investigators found that patients receiving tecemotide after concurrent chemoradiation did show a significant increase in OS of about 10 months (HR 0.78, p = 0.016), as opposed to those who had received sequential chemo-radiotherapy (HR 1.12, p = 0.38). This was confirmed in the updated results published a few years later by Mitchell et al., adding exploratory biomarker analyses which demonstrated a potential positive predictive value of high blood levels of soluble MUC1 (sMUC1) for tecemotide therapy (50). Given that negative prognostic associations were observed between high sMUC1 and OS in the placebo group, researchers proposed that an abundance of sMUC1 in the circulation may reflect increased MUC1 expression by tumors and therefore indicate a target for tecemotide-induced T-cell immunity. A subsequent phase III trial, narrowing its study population to concurrent chemoradiation, attempted to confirm the previously demonstrated OS benefit of adjuvant tecemotide but was terminated prematurely due to the discontinuation of the tecemotide program in NSCLC.
More recently, Patel et al. hypothesized that vascular endothelial growth factor-inhibitor bevacizumab may provide additional immunomodulatory benefits when combined with tecemotide following definitive chemoradiation for LA-NSCLC (51). Their phase I study met its safety endpoint, but the authors did report grade 4 (1/70) and 5 (1/70) toxicity during maintenance treatment.
A second peptide-based vaccine studied in LA-NSCLC, called GV1001, is derived from the functional domain of human telomerase reverse transcriptase. Overexpression of this enzyme in cancer cells helps maintain the integrity of telomere sequences, thereby allowing them to avoid senescence. In their phase II trial of maintenance vaccination following radiotherapy and docetaxel for stage III NSCLC, Brunsvig et al. demonstrated a trend toward improved PFS in patients exhibiting a GV1001-specific T-cell response (52). The benefits of GV1001 in the context of radiation may not be limited to immune activation, as they may also include antifibrotic effects (74).
The cancer/testis antigen, MAGE-A3, has also been proposed as a target for ASI in NSCLC and was investigated in one phase I trial (53). One of four cohorts (n = 12) integrated RT into protocol treatment, adding the MAGE-A3 immunotherapeutic after sequential chemoradiation in unresectable stage III NSCLC. In addition to demonstrating an acceptable toxicity profile, a higher prevalence and magnitude of MAGE-A3-specific T-cell responses were observed following chemoradiation in comparison to other study arms not incorporating RT, suggesting irradiated tumor tissue may present an ideal substrate for this particular vaccine.
Several reports, originating primarily from Asian countries, have described therapeutic efficacy of combining chemoradiation with adoptive immunotherapy. A meta-analysis by Qian et al. concluded that the addition of immune cell reinfusion (i.e., DC-CIK, CIK, LAK, or TIL) to conventional NSCLC treatments significantly improved 2-year OS (OR 2.45, 1.60–3.75; p < 0.001) (75). Even more recently, a second meta-analysis focusing on CIK treatment in lung cancer demonstrated increased objective response and disease control rates, with 1- and 2-year OS in favor of combined IT-RT treatment in the NSCLC subgroup analyses (76).
Whereas, the previously discussed immunotherapeutics are either no longer under investigation in conjunction with RT for LA-NSCLC, or still in experimental stages of development, a true paradigm shift in the treatment of LA-NSCLC was brought about by the introduction of ICB. In this context, most studies to date have investigated RT in conjunction with monoclonal antibodies targeting the PD(L)-1 receptor interaction. First, consolidation pembrolizumab (anti-PD-1) after chemoradiation in patients with unresectable stage III NSCLC demonstrated increased 2-year PFS (44.6%) and time to metastatic disease or death (TMDD; median 22.4 months) compared to historical controls (54), but the most convincing results to date were achieved with PD-L1 inhibition. The PACIFIC study, a randomized phase III trial evaluating durvalumab or placebo after concurrent chemo-radiotherapy for unresectable stage III disease, initially achieved very convincing results in terms of PFS (HR 0.52) and TMDD (median 23.2 months, HR 0.52) (77). More recently, the trial also showed practice-changing evidence through a significantly prolonged OS of maintenance durvalumab as compared with placebo (HR 0.68, p = 0.0025) across all prespecified subgroups (31). This subsequently led to its FDA and EMA approval as standard treatment for tumors with a PD-L1 expression of ≥1% in the majority of jurisdictions. In addition, although there is currently no formal evidence on the effect of durvalumab following sequential chemo-radiotherapy in LA-NSCLC, some countries have already granted approval for this setting as well. Nevertheless, further research is ongoing to define the impact of durvalumab after sequential chemoradiation, as it is recognized that results may not simply be transferable due to aspects inherent to the treatment approach as such and/or because of a difference in patient population, as clearly demonstrated by the START trial. Moreover, since detailed data of the RT delivered in the PACIFIC study, such as dose-volume parameters, are lacking, more thorough RT quality assurance will be of utmost importance in future clinical trials.
The proof of durvalumab's manageable safety profile as a sequential treatment after chemoradiation, in line with previous studies of ICB monotherapy, encouraged researchers to attempt combining all three treatment modalities concurrently. In the DETERRED trial, 40 LA-NSCLC patients were randomized 3:1 to receive standard chemo-radiotherapy with or without concomitant atezolizumab (anti-PD-L1) followed by atezolizumab maintenance treatment (55). No increased toxicity was found when comparing both regimens. Similarly, the recently published interim safety analysis of the ETOP NICOLAS study (n = 21) established that the addition of nivolumab (anti-PD-1) concurrently to chemoradiation followed by nivolumab maintenance, did not lead to the occurrence of grade ≥3 pneumonitis by 3 months post-RT (56). Regrettably, phase II results of this trial presented at the 2019 European Society of Medical Oncology congress showed it did not meet its primary efficacy endpoint with a 1-year PFS of 50% (57). Further evidence from other ongoing trials (Table 3) are eagerly awaited.
Metastatic NSCLC
About half of all lung cancer patients are diagnosed with advanced disease (44). Though the therapeutic landscape of metastatic NSCLC (M-NSCLC) has changed tremendously over the past decade, 5-year relative survival remains low at around 6%. Traditionally, RT is offered as a palliative treatment option for these patients (78). In recent years, however, there is growing evidence that RT could also be offered as a local—potentially curative—treatment in a subset of M-NSCLC patients who present with a limited number of metastases, termed oligometastatic disease (79). In this context, stereotactic body radiotherapy (SBRT), using high doses per fraction and precise dose delivery, is often proposed to ablate visible lesions, built upon the finding that further disease progression most often originates from these known disease sites (80). Recent randomized controlled trials (RCTs) have confirmed this hypothesis, the ablative approach yielding OS and PFS benefit (81–83). Nevertheless, this evidence was gathered at a time when IT had not yet made its way into standard first- and/or second-line treatment for M-NSCLC. Considering the potential immunogenicity of RT, perhaps its benefits could extend beyond the oligometastatic setting, boosting immunotherapeutic efficacy through in-situ vaccination and the induction of abscopal responses, while debulking sites of gross disease in polymetastatic patients. IT, in turn, may reduce tumor loads or slow down the process of metastatic spread, creating a window of opportunity for local ablative treatments, such as SBRT.
Available Clinical Evidence (Table 2)
As in LA-NSCLC, research efforts into IT-RT combinations for the treatment of M-NSCLC date back to a time when ICB had not yet been developed. Studies with limited patient numbers have explored the potential benefit of different IT-RT combinations.
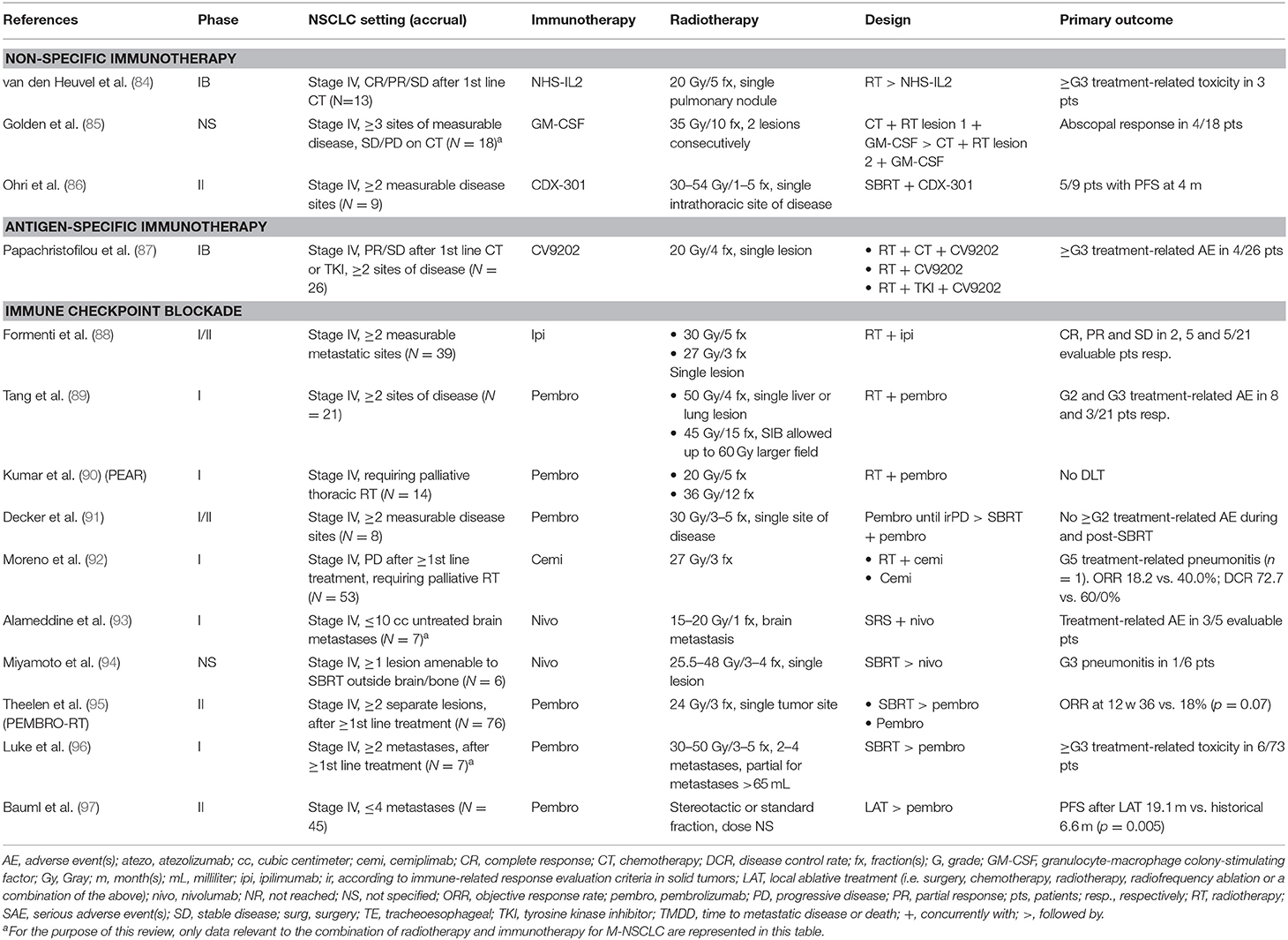
Table 2. Clinical studies evaluating immunotherapy-radiotherapy combinations in metastatic non-small cell lung cancer (M-NSCLC), with primary endpoint results published or presented during the last decade (2009–2019).
Among the first experiments with RT and immunocytokine therapy in lung cancer demonstrated an acceptable safety profile and potential immunogenicity of Selectikine or NHS-IL2 (genetically modified interleukin-2 fused with DNA-targeting antibody NHS76), administered after local irradiation of a single pulmonary lesion in 13 M-NSCLC patients (84). Interestingly, both long-term survivors developed thyroiditis during treatment, which was only seen in one other patient, indicating that the incidence of immune-related adverse events may be linked to intrinsic IT susceptibility.
Recognizing the importance of DCs in RT-induced immune activation, Golden et al. attempted to stimulate DC maturation by combining radiation with granulocyte-macrophage colony-stimulating factor (GM-CSF), ultimately aiming to elicit abscopal responses (85). Almost half of the 41 patients studied had M-NSCLC (n = 18), four of whom demonstrated a partial (2/4) or complete (2/4) response in non-irradiated lesions. In addition, patients experiencing an abscopal response (11 in total) showed significantly better OS (HR 2.06).
Similar to GM-CSF, the feline McDonough sarcoma-like tyrosine kinase 3 ligand CDX-301 has the ability to induce differentiation of bone marrow precursor cells into distinct subsets of DCs. Preliminary results from an ongoing trial investigating SBRT to an intrathoracic disease site and concurrent CDX-301 in M-NSCLC are promising, with more than half of patients (5/9) achieving partial remission (86). Of note, all five responders had been previously treated with ICB.
Though disappointing phase III results, such as those of the START trial in LA-NSCLC (50, 73) and the STOP trial investigating maintenance belagenpumatucel-L in M-NSCLC (98), might have negatively impacted general enthusiasm for antigen-specific immunotherapeutic approaches in NSCLC, novel mRNA-based vaccines may be more promising. Targeting five and six different TAAs respectively, CV9201 and its successor CV9202 have shown an acceptable safety profile and evidence of immune activation when delivered after or concurrently with local fractionated RT for stage IIIB-IV disease (87, 99). Additional research is required to determine whether this combination treatment can improve long-term outcome.
The largest body of available IT-RT evidence in M-NSCLC describes the marriage of radiation and ICB. While Formenti et al. performed a pioneering study on abscopal effects with ipilimumab (88), research subsequently published focused mainly on combining RT with anti-PD-1 antibodies, due to their proven efficacy as monotherapy in M-NSCLC.
Early results of two phase I trials, comparing different—radical or palliative—RT dose-fractionation schedules given concurrently with escalating doses of pembrolizumab, demonstrated acceptable toxicity of combined treatment and encouraging disease control rates (DCR) of up to 68% (89, 90). Moreover, Kumar et al. observed a trend for improved PFS with higher RT doses, supporting the notion that these could potentially be more immunogenic (90). Another cleverly designed study attempted to elucidate the role of radiation as a salvage treatment for M-NSCLC refractory to ICB, adding SBRT onto pembrolizumab treatment only at the time of disease progression (100). Stable disease and systemic responses were achieved in 10 and 2 of the 21 patients who completed study treatment, respectively.
Early safety data on the concurrent use of other anti-PD-1 drugs (e.g., cemiplimab) with RT appear to be reassuring (92), although caution is advised when treating brain metastases radiosurgically in conjunction with nivolumab, especially in the presence of significant peritumoral edema (93).
Sequential approaches were also evaluated, initially for nivolumab (94), but more recent phase I and II trials focused on pembrolizumab after local ablative treatment for M-NSCLC. For instance, Theelen et al. performed a RCT of pembrolizumab either without or after SBRT (3 × 8 Gy) of a single NSCLC metastasis (95). While the study's primary endpoint criteria were not met, a significant improvement of DCR was observed in the experimental arm (64 vs. 40%; p = 0.04). Moreover, subgroup analyses showed patients benefiting most from SBRT were those with PD-L1 negative tumors at baseline. This finding is particularly intriguing, since this is a population for which single-agent PD-(L)1 inhibition is known to be of limited benefit. Perhaps, pembrolizumab following SBRT may represent a less toxic alternative to chemoimmunotherapy when aiming to enhance response rates in M-NSCLC patients with a low PD-L1 tumor proportion score.
Despite promising results, some authors have advocated abandoning the single-site abscopal approach and instead propose to irradiate as much of the tumor burden as can be safely achieved (101). They hypothesize that targeting only one lesion may fail to account for tumor heterogeneity and immunosuppressive features of bulky disease, thereby limiting the probability of RT-induced systemic antitumor immune activation. Luke et al. applied this logic in their phase I study, allowing multisite SBRT of 2–4 metastases up to 1 week before starting pembrolizumab in patients with advanced solid tumors (96). Translating this concept into the oligometastatic NSCLC setting, Bauml et al. initiated a phase II trial offering adjuvant pembrolizumab to patients with a limited tumor burden (≤4 metastases) after eradication of all known sites of disease (97). Median PFS exceeded that of historical controls, but whether this benefit could be explained by a potential IT-RT synergy remains unclear, as subgroup analyses comparing irradiated to radiation-naive patients could not be performed. Questions like these should be answered in RCTs, preferably stratifying patients according to tumor burden, such as the ongoing IMMUNOSABR2 study. Other ongoing IT-RT trials in M-NSCLC are shown in Table 3.
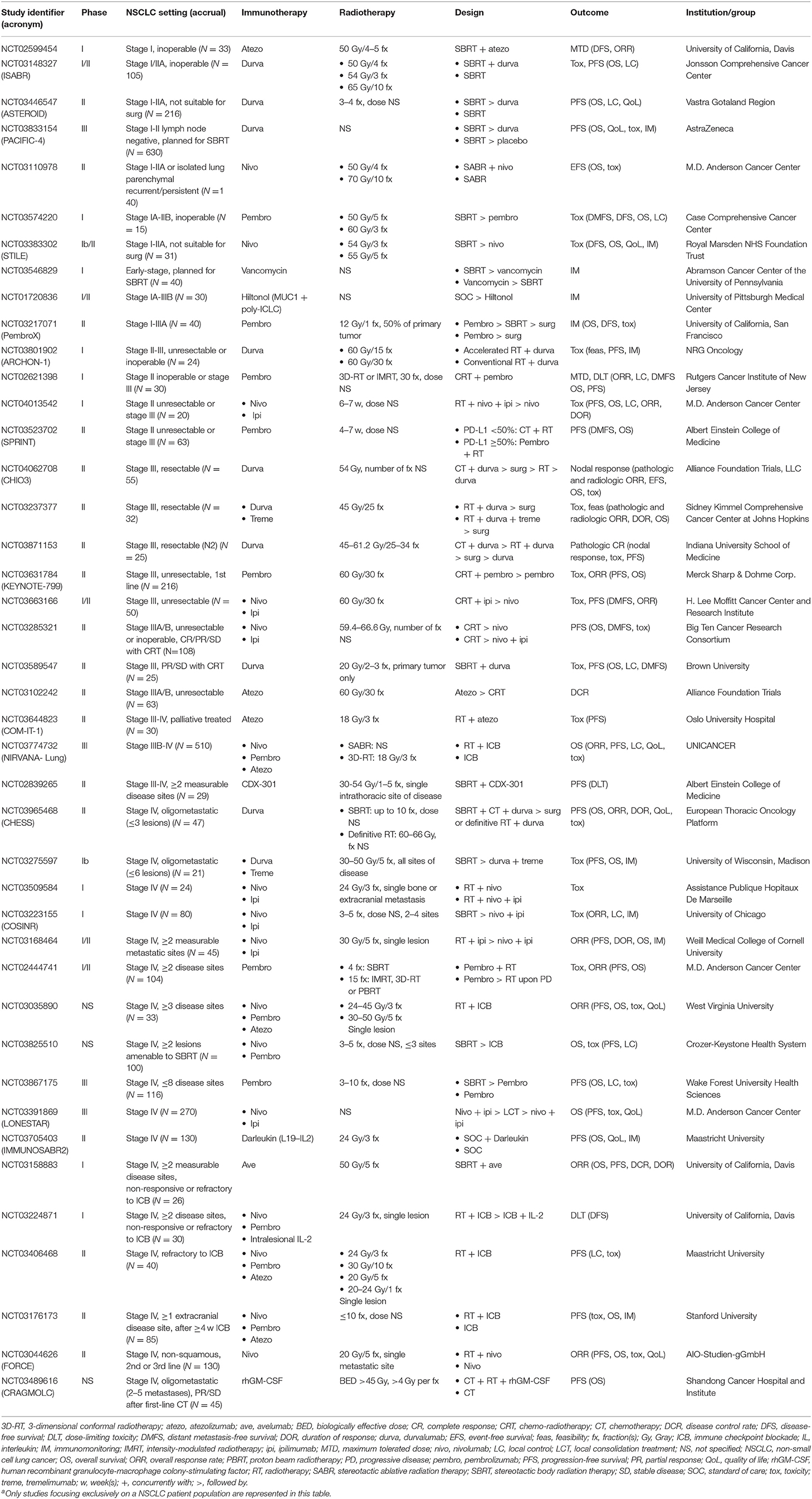
Table 3. Currently ongoing trials (i.e. not yet recruiting, recruiting and enrolling by invitation) evaluating immunotherapy-radiotherapy combinations in non-small cell lung cancera.
Early-Stage NSCLC
Early-stage NSCLC (ES-NSCLC) represents about 15–20% of all new lung cancer diagnoses (44). According to the latest consensus guidelines, surgery remains the treatment of choice for operable ES-NSCLC patients. For those unfit for or unwilling to undergo surgical resection, SBRT is now the gold standard, with an excellent safety profile and local control rates of approximately 90% at 5 years (1). Unfortunately, as in LA-NSCLC, the issue remains distant relapse, occurring in up to 20% or more (102–104). This number rises to over 30% in most reports when regional recurrences are taken into account. Certain clinical and molecular features associated with poor prognosis have been identified (105, 106), justifying possible treatment intensification in a subset of ES-NSCLC patients. In this context, systemic treatment options could be considered in order to eradicate micrometastatic disease and, in doing so, improve long-term outcome. However, as factors determining patients' operability often coincide with those affecting their eligibility for chemotherapy, a unique opportunity presents itself for treatments with a relatively modest toxicity profile, such as IT. Moreover, IT may offer additional benefits in terms of potential synergistic effects with SBRT as detailed above. This rationale is further supported by immune mechanistic studies demonstrating hypofractionated RT for stage I NSCLC may stimulate immune activation (107). As of yet, clinical data of combined IT-RT approaches in ES-NSCLC are lacking, but trials investigating their safety and efficacy are well underway (Table 3).
Conclusion
As of yet, the lion's share of evidence demonstrating clinical benefit with combined immunotherapy-radiotherapy strategies for NSCLC is situated in locally-advanced disease. Even so, a growing body of pre-clinical and clinical data has allowed further insights into their synergy, providing a strong rationale for extending this potential to other disease entities, such as metastatic NSCLC. In addition, the excellent tolerability profile of many novel immunotherapeutic drugs, both as monotherapy and in conjunction with radiotherapy, as well as technological and technical radiotherapy advances have created a window of opportunity for the development of combined strategies in earlier disease stages. Nevertheless, the success of future trials will require well-reasoned hypotheses for radiotherapy timing, dose and fractionation, in addition to selection of the appropriate partner immunotherapy.
Author Contributions
MS performed the literature review and search of ongoing clinical trials, on which both authors structured and synthesized the evidence for the manuscript. YL critically revised all the drafts and approved the final version for submission.
Funding
This work was supported by research grants from the Flemish League against Cancer and Varian.
Conflict of Interest
YL reports expert positions for AstraZeneca and RaySearch, outside the current work.
The remaining author declares that the research was conducted in the absence of any commercial or financial relationships that could be construed as a potential conflict of interest.
References
1. Postmus PE, Kerr KM, Oudkerk M, Senan S, Waller DA, Vansteenkiste J, et al. Early and locally advanced non-small-cell lung cancer (NSCLC): ESMO Clinical Practice Guidelines for diagnosis, treatment and follow-up. Ann Oncol. (2017) 28(suppl_4):iv1–21. doi: 10.1093/annonc/mdx222
2. Abuodeh Y, Venkat P, Kim S. Systematic review of case reports on the abscopal effect. Curr Probl Cancer. (2016) 40:25–37. doi: 10.1016/j.currproblcancer.2015.10.001
3. Dunn GP, Bruce AT, Ikeda H, Old LJ, Schreiber RD. Cancer immunoediting: from immunosurveillance to tumor escape. Nat Immunol. (2002) 3:991–8. doi: 10.1038/ni1102-991
4. Chen DS, Mellman I. Oncology meets immunology: the cancer-immunity cycle. Immunity. (2013) 39:1–10. doi: 10.1016/j.immuni.2013.07.012
5. Golden EB, Pellicciotta I, Demaria S, Barcellos-Hoff MH, Formenti SC. The convergence of radiation and immunogenic cell death signaling pathways. Front Oncol. (2012) 2:88. doi: 10.3389/fonc.2012.00088
6. Durante M, Formenti SC. Radiation-induced chromosomal aberrations and immunotherapy: micronuclei, cytosolic DNA, and interferon-production pathway. Front Oncol. (2018) 8:192. doi: 10.3389/fonc.2018.00192
7. Deng L, Liang H, Xu M, Yang X, Burnette B, Arina A, et al. STING-Dependent cytosolic DNA sensing promotes radiation-induced type I interferon-dependent antitumor immunity in immunogenic tumors. Immunity. (2014) 41:843–52. doi: 10.1016/j.immuni.2014.10.019
8. Vanpouille-Box C, Alard A, Aryankalayil MJ, Sarfraz Y, Diamond JM, Schneider RJ, et al. DNA exonuclease Trex1 regulates radiotherapy-induced tumour immunogenicity. Nat Commun. (2017) 8:15618. doi: 10.1038/ncomms15618
9. Hellevik T, Martinez-Zubiaurre I. Radiotherapy and the tumor stroma: the importance of dose and fractionation. Front Oncol. (2014) 4:1. doi: 10.3389/fonc.2014.00001
10. Gupta A, Probst HC, Vuong V, Landshammer A, Muth S, Yagita H, et al. Radiotherapy promotes tumor-specific effector CD8+ T cells via dendritic cell activation. J Immunol. (2012) 189:558–66. doi: 10.4049/jimmunol.1200563
11. Lee Y, Auh SL, Wang Y, Burnette B, Wang Y, Meng Y, et al. Therapeutic effects of ablative radiation on local tumor require CD8+ T cells: changing strategies for cancer treatment. Blood. (2009) 114:589–95. doi: 10.1182/blood-2009-02-206870
12. Martinez-Zubiaurre I, Chalmers AJ, Hellevik T. Radiation-induced transformation of immunoregulatory networks in the tumor stroma. Front Immunol. (2018) 9:1679. doi: 10.3389/fimmu.2018.01679
13. Matsumura S, Wang B, Kawashima N, Braunstein S, Badura M, Cameron TO, et al. Radiation-induced CXCL16 release by breast cancer cells attracts effector T cells. J Immunol. (2008) 181:3099–107. doi: 10.4049/jimmunol.181.5.3099
14. Kim JY, Son YO, Park SW, Bae JH, Chung JS, Kim HH, et al. Increase of NKG2D ligands and sensitivity to NK cell-mediated cytotoxicity of tumor cells by heat shock and ionizing radiation. Exp Mol Med. (2006) 38:474–84. doi: 10.1038/emm.2006.56
15. Chakraborty M, Abrams SI, Camphausen K, Liu K, Scott T, Coleman CN, et al. Irradiation of tumor cells up-regulates Fas and enhances CTL lytic activity and CTL adoptive immunotherapy. J Immunol. (2003) 170:6338–47. doi: 10.4049/jimmunol.170.12.6338
16. Reits EA, Hodge JW, Herberts CA, Groothuis TA, Chakraborty M, Wansley EK, et al. Radiation modulates the peptide repertoire, enhances MHC class I expression, and induces successful antitumor immunotherapy. J Exp Med. (2006) 203:1259–71. doi: 10.1084/jem.20052494
17. Vatner RE, Formenti SC. Myeloid-derived cells in tumors: effects of radiation. Semin Radiat Oncol. (2015) 25:18–27. doi: 10.1016/j.semradonc.2014.07.008
18. Kachikwu EL, Iwamoto KS, Liao YP, DeMarco JJ, Agazaryan N, Economou JS, et al. Radiation enhances regulatory T cell representation. Int J Radiat Oncol Biol Phys. (2011) 81:1128–35. doi: 10.1016/j.ijrobp.2010.09.034
19. Biswas S, Guix M, Rinehart C, Dugger TC, Chytil A, Moses HL, et al. Inhibition of TGF-beta with neutralizing antibodies prevents radiation-induced acceleration of metastatic cancer progression. J Clin Invest. (2017) 127:1116. doi: 10.1172/JCI93333
20. Ostrand-Rosenberg S, Horn LA, Ciavattone NG. Radiotherapy both promotes and inhibits myeloid-derived suppressor cell function: novel strategies for preventing the tumor-protective effects of radiotherapy. Front Oncol. (2019) 9:215. doi: 10.3389/fonc.2019.00215
21. Schaue D, Comin-Anduix B, Ribas A, Zhang L, Goodglick L, Sayre JW, et al. T-cell responses to survivin in cancer patients undergoing radiation therapy. Clin Cancer Res. (2008) 14:4883–90. doi: 10.1158/1078-0432.CCR-07-4462
22. Tabi Z, Spary LK, Coleman S, Clayton A, Mason MD, Staffurth J. Resistance of CD45RA- T cells to apoptosis and functional impairment, and activation of tumor-antigen specific T cells during radiation therapy of prostate cancer. J Immunol. (2010) 185:1330–9. doi: 10.4049/jimmunol.1000488
23. Garnett CT, Palena C, Chakraborty M, Tsang KY, Schlom J, Hodge JW. Sublethal irradiation of human tumor cells modulates phenotype resulting in enhanced killing by cytotoxic T lymphocytes. Cancer Res. (2004) 64:7985–94. doi: 10.1158/0008-5472.CAN-04-1525
24. Schaue D, Ratikan JA, Iwamoto KS, McBride WH. Maximizing tumor immunity with fractionated radiation. Int J Radiat Oncol Biol Phys. (2012) 83:1306–10. doi: 10.1016/j.ijrobp.2011.09.049
25. Dorta-Estremera S, Colbert LE, Nookala SS, Yanamandra AV, Yang G, Delgado A, et al. Kinetics of intratumoral immune cell activation during chemoradiation for cervical cancer. Int J Radiat Oncol Biol Phys. (2018) 102:593–600. doi: 10.1016/j.ijrobp.2018.06.404
26. Klug F, Prakash H, Huber PE, Seibel T, Bender N, Halama N, et al. Low-dose irradiation programs macrophage differentiation to an iNOS(+)/M1 phenotype that orchestrates effective T cell immunotherapy. Cancer Cell. (2013) 24:589–602. doi: 10.1016/j.ccr.2013.09.014
27. Meng Y, Yu Z, Wu Y, Du T, Chen S, Meng F, et al. Cell-based immunotherapy with cytokine-induced killer (CIK) cells: From preparation and testing to clinical application. Hum Vaccin Immunother. (2017) 13:1–9. doi: 10.1080/21645515.2017.1285987
28. DeSelm C, Palomba ML, Yahalom J, Hamieh M, Eyquem J, Rajasekhar VK, et al. Low-dose radiation conditioning enables CAR T cells to mitigate antigen escape. Mol Ther. (2018) 26:2542–52. doi: 10.1016/j.ymthe.2018.09.008
29. Weichselbaum RR, Liang H, Deng L, Fu YX. Radiotherapy and immunotherapy: a beneficial liaison? Nat Rev Clin Oncol. (2017) 14:365–79. doi: 10.1038/nrclinonc.2016.211
30. Deng L, Liang H, Burnette B, Beckett M, Darga T, Weichselbaum RR, et al. Irradiation and anti-PD-L1 treatment synergistically promote antitumor immunity in mice. J Clin Invest. (2014) 124:687–95. doi: 10.1172/JCI67313
31. Antonia SJ, Villegas A, Daniel D, Vicente D, Murakami S, Hui R, et al. Overall survival with durvalumab after chemoradiotherapy in stage III NSCLC. N Engl J Med. (2018) 379:2342–50. doi: 10.1056/NEJMoa1809697
32. Faivre-Finn C, Spigel DR, Senan S, Langer CJ, Raben D, Perz B, et al. Efficacy and safety evaluation based on time from completion of radiotherapy to randomization with durvalumab or placebo in pts from PACIFIC. Ann Oncol. (2018) 29(suppl_8):mdy291. doi: 10.1093/annonc/mdy291
33. Venkatesulu BP, Mallick S, Lin SH, Krishnan S. A systematic review of the influence of radiation-induced lymphopenia on survival outcomes in solid tumors. Crit Rev Oncol Hematol. (2018) 123:42–51. doi: 10.1016/j.critrevonc.2018.01.003
34. Grassberger C, Ellsworth SG, Wilks MQ, Keane FK, Loeffler JS. Assessing the interactions between radiotherapy and antitumour immunity. Nat Rev Clin Oncol. (2019). doi: 10.1038/s41571-019-0238-9 [Epub ahead of print].
35. Tang C, Liao Z, Gomez D, Levy L, Zhuang Y, Gebremichael RA, et al. Lymphopenia association with gross tumor volume and lung V5 and its effects on non-small cell lung cancer patient outcomes. Int J Radiat Oncol Biol Phys. (2014) 89:1084–91. doi: 10.1016/j.ijrobp.2014.04.025
36. Yovino S, Kleinberg L, Grossman SA, Narayanan M, Ford E. The etiology of treatment-related lymphopenia in patients with malignant gliomas: modeling radiation dose to circulating lymphocytes explains clinical observations and suggests methods of modifying the impact of radiation on immune cells. Cancer Invest. (2013) 31:140–4. doi: 10.3109/07357907.2012.762780
37. Ladbury CJ, Rusthoven CG, Camidge DR, Kavanagh BD, Nath SK. Impact of radiation dose to the host immune system on tumor control and survival for stage III non-small cell lung cancer treated with definitive radiation therapy. Int J Radiat Oncol Biol Phys. (2019) 105:346–55. doi: 10.1016/j.ijrobp.2019.05.064
38. Sellins KS, Cohen JJ. Gene induction by gamma-irradiation leads to DNA fragmentation in lymphocytes. J Immunol. (1987) 139:3199–206.
39. Marciscano AE, Ghasemzadeh A, Nirschl TR, Theodros D, Kochel CM, Francica BJ, et al. Elective nodal irradiation attenuates the combinatorial efficacy of stereotactic radiation therapy and immunotherapy. Clin Cancer Res. (2018) 24:5058–71. doi: 10.1158/1078-0432.CCR-17-3427
40. Price JG, Idoyaga J, Salmon H, Hogstad B, Bigarella CL, Ghaffari S, et al. CDKN1A regulates Langerhans cell survival and promotes Treg cell generation upon exposure to ionizing irradiation. Nat Immunol. (2015) 16:1060–8. doi: 10.1038/ni.3270
41. Wirsdörfer F, de Leve S, Jendrossek V. Combining radiotherapy and immunotherapy in lung cancer: can we expect limitations due to altered normal tissue toxicity? Int J Mol Sci. (2018) 20:E24. doi: 10.3390/ijms20010024
42. Louvel G, Bahleda R, Ammari S, Le Péchoux C, Levy A, Massard C, et al. Immunotherapy and pulmonary toxicities: can concomitant immune-checkpoint inhibitors with radiotherapy increase the risk of radiation pneumonitis? Eur Respir J. (2018) 51:1701737. doi: 10.1183/13993003.01737-2017
43. Suresh K, Voong KR, Shankar B, Forde PM, Ettinger DS, Marrone KA, et al. Pneumonitis in non-small cell lung cancer patients receiving immune checkpoint immunotherapy: incidence and risk factors. J Thorac Oncol. (2018) 13:1930–9. doi: 10.1016/j.jtho.2018.08.2035
44. Lu T, Yang X, Huang Y, Zhao M, Li M, Ma K, et al. Trends in the incidence, treatment, and survival of patients with lung cancer in the last four decades. Cancer Manag Res. (2019) 11:943–53. doi: 10.2147/CMAR.S187317
45. Bradley JD, Paulus R, Komaki R, Masters G, Blumenschein G, Schild S, et al. Standard-dose versus high-dose conformal radiotherapy with concurrent and consolidation carboplatin plus paclitaxel with or without cetuximab for patients with stage IIIA or IIIB non-small-cell lung cancer (RTOG 0617): a randomised, two-by-two factorial phase 3 study. Lancet Oncol. (2015) 16:187–99. doi: 10.1016/S1470-2045(14)71207-0
46. Senan S, Brade A, Wang LH, Vansteenkiste J, Dakhil S, Biesma B, et al. PROCLAIM: randomized phase III trial of pemetrexed-cisplatin or etoposide-cisplatin plus thoracic radiation therapy followed by consolidation chemotherapy in locally advanced nonsquamous non-small-cell lung cancer. J Clin Oncol. (2016) 34:953–62. doi: 10.1200/JCO.2015.64.8824
47. Garg S, Gielda BT, Kiel K, Turian JV, Fidler MJ, Batus M, et al. Patterns of locoregional failure in stage III non-small cell lung cancer treated with definitive chemoradiation therapy. Pract Radiat Oncol. (2014) 4:342–8. doi: 10.1016/j.prro.2013.12.002
48. Ohyanagi F, Horai T, Sekine I, Yamamoto N, Nakagawa K, Nishio M, et al. Safety of BLP25 liposome vaccine (L-BLP25) in Japanese patients with unresectable stage III NSCLC after primary chemoradiotherapy: preliminary results from a Phase I/II study. Jpn J Clin Oncol. (2011) 41:718–22. doi: 10.1093/jjco/hyr021
49. Butts C, Murray N, Maksymiuk A, Goss G, Marshall E, Soulières D, et al. Randomized phase IIB trial of BLP25 liposome vaccine in stage IIIB and IV non-small-cell lung cancer. J Clin Oncol. (2005) 23:6674–81. doi: 10.1200/JCO.2005.13.011
50. Mitchell P, Thatcher N, Socinski MA, Wasilewska-Tesluk E, Horwood K, Szczesna A, et al. Tecemotide in unresectable stage III non-small-cell lung cancer in the phase III START study: updated overall survival and biomarker analyses. Ann Oncol. (2015) 26:1134–42. doi: 10.1093/annonc/mdv104
51. Patel J, Lee J, Wagner H Jr, Carbone D, Shanker A, Horn L, et al. Phase II study of immunotherapy with tecemotide and bevacizumab after chemoradiation in unresectable stage III NS-NSCLC. J Thorac Oncol. (2018) 13:S370. doi: 10.1016/j.jtho.2018.08.349
52. Brunsvig PF, Kyte JA, Kersten C, Sundstrøm S, Møller M, Nyakas M, et al. Telomerase peptide vaccination in NSCLC: a phase II trial in stage III patients vaccinated after chemoradiotherapy and an 8-year update on a phase I/II trial. Clin Cancer Res. (2011) 17:6847–57. doi: 10.1158/1078-0432.CCR-11-1385
53. Pujol JL, Vansteenkiste JF, De Pas TM, Atanackovic D, Reck M, Thomeer M, et al. Safety and Immunogenicity of MAGE-A3 Cancer Immunotherapeutic with or without Adjuvant Chemotherapy in Patients with Resected Stage IB to III MAGE-A3-Positive Non-Small-Cell Lung Cancer. J Thorac Oncol. (2015) 10:1458–67. doi: 10.1097/JTO.0000000000000653
54. Durm G, Althouse S, Sadiq A, Jalal S, Jabbour S, Zon R, et al. Updated results of a phase II trial of concurrent chemoradiation with consolidation pembrolizumab in patients with unresectable stage III NSCLC. J Thorac Oncol. (2018) 13:S321. doi: 10.1016/j.jtho.2018.08.238
55. Lin S, Lin X, Clay D, Yao L, Mok I, Gomez D, et al. DETERRED: phase II trial combining atezolizumab concurrently with chemoradiation therapy in locally advanced non-small cell lung cancer. J Thorac Oncol. (2018) 13:S320–1. doi: 10.1016/j.jtho.2018.08.237
56. Peters S, Felip E, Dafni U, Belka C, Guckenberger M, Irigoyen A, et al. Safety evaluation of nivolumab added concurrently to radiotherapy in a standard first line chemo-radiotherapy regimen in stage III non-small cell lung cancer-The ETOP NICOLAS trial. Lung Cancer. (2019) 133:83–7. doi: 10.1016/j.lungcan.2019.05.001
57. Peters S, FeliP E, Dafni U, Tufman A, Guckenberger M, Irigoyen A, et al. Efficacy evaluation of concurrent nivolumab addition to a first-line, concurrent chemo-radiotherapy regimen in unresectable locally advanced NSCLC: results from the European Thoracic Oncology Platform (ETOP 6–14) NICOLAS phase II trial. Ann Oncol. (2019) 30(Suppl. 5):mdz259. doi: 10.1093/annonc/mdz259
58. Alexandroff AB, Jackson AM, O'Donnell MA, James K. BCG immunotherapy of bladder cancer: 20 years on. Lancet. (1999) 353:1689–94. doi: 10.1016/S0140-6736(98)07422-4
59. Pines A. A 5-year controlled study of B.C.G. and radiotherapy inoperable lung cancer. Lancet. (1976) 1:380–1. doi: 10.1016/S0140-6736(76)90213-0
60. Robinson E, Bartal A, Cohen Y, Haasz R, Mekori T. Treatment of lung cancer by radiotherapy, chemotherapy, and methanol extraction residue of BCG (MER): clinical and immunological studies. Cancer. (1977) 40:1052–9. doi: 10.1002/1097-0142(197709)40:3<1052::AID-CNCR2820400313>3.0.CO;2-G
61. Robinson E, Haim N, Segal R, Veseley Z, Mekori T. Combined-modality treatment of inoperable lung cancer (i.v. immunotherapy, chemotherapy, and radiotherapy). Cancer Treat Rep. (1985) 69:251–8.
62. Ruckdeschel JC, de Leve S, Jendrossek V. Regional immunotherapy has a detrimental effect on the response to combined irradiation and chemotherapy in locally advanced non-small cell bronchogenic carcinoma. Cancer Immunol Immunother. (1981) 11:277–82. doi: 10.1007/BF00198972
63. White JE, Chen T, Reed R, Mira J, Stuckey WJ, Weatherall T, et al. Limited squamous cell carcinoma of the lung: a Southwest Oncology Group randomized study of radiation with or without doxorubicin chemotherapy and with or without levamisole immunotherapy. Cancer Treat Rep. (1982) 66:1113–20.
64. Perez CA, Bauer M, Emami BN, Byhardt R, Brady LW, Doggett RL, et al. Thoracic irradiation with or without levamisole (NSC #177023) in unresectable non-small cell carcinoma of the lung: a phase III randomized trial of the RTOG. Int J Radiat Oncol Biol Phys. (1988) 15:1337–46. doi: 10.1016/0360-3016(88)90229-5
65. Herskovic A, Bauer M, Seydel HG, Yesner R, Doggett RL, Perez CA, et al. Post-operative thoracic irradiation with or without levamisole in non-small cell lung cancer: results of a Radiation Therapy Oncology Group Study. Int J Radiat Oncol Biol Phys. (1988) 14:37–42. doi: 10.1016/0360-3016(88)90048-X
66. Van Houtte P, Bondue H, Rocmans P, Michel J, Wybran J, Dalesio O, et al. Adjuvant immunotherapy by levamisole in resectable lung cancer: a control study. Eur J Cancer. (1980) 16:1597–601. doi: 10.1016/0014-2964(80)90033-X
67. Krauss S, Comas F, Perez C, Gordon D, Philpott G, Broun G, et al. Treatment of inoperable non-small cell carcinoma of the lung with radiation therapy, with or without levamisole. A randomized trial of the Southeastern Cancer Study group. Am J Clin Oncol. (1984) 7:405–12. doi: 10.1097/00000421-198410000-00003
68. Ogawa Y, Kimura S, Imajo Y, Hamada F, Miyaji C, Imanaka K, et al. [Evaluation of concomitant use of non-specific immunopotentiator on 172 cases of primary lung cancer (stage III, IV) treated with radiation combined with chemotherapy (author's transl)]. Rinsho Hoshasen. (1982) 27:451–4.
69. Maasilta P, Holsti LR, Halme M, Kivisaari L, Cantell K, Mattson K. Natural alpha-interferon in combination with hyperfractionated radiotherapy in the treatment of non-small cell lung cancer. Int J Radiat Oncol Biol Phys. (1992) 23:863–8. doi: 10.1016/0360-3016(92)90660-A
70. Shaw EG, Deming RL, Creagan ET, Nair S, Su JQ, Levitt R, et al. Pilot study of human recombinant interferon gamma and accelerated hyperfractionated thoracic radiation therapy in patients with unresectable stage IIIA/B nonsmall cell lung cancer. Int J Radiat Oncol Biol Phys. (1995) 31:827–31. doi: 10.1016/0360-3016(94)00462-5
71. Bradley JD, Scott CB, Paris KJ, Demas WF, Machtay M, Komaki R, et al. A phase III comparison of radiation therapy with or without recombinant beta-interferon for poor-risk patients with locally advanced non-small-cell lung cancer (RTOG 93-04). Int J Radiat Oncol Biol Phys. (2002) 52:1173–9. doi: 10.1016/S0360-3016(01)02797-3
72. Franco-Molina MA, Mendoza-Gamboa E, Zapata-Benavides P, Vera-García ME, Castillo-Tello P, García de la Fuente A, et al. IMMUNEPOTENT CRP (bovine dialyzable leukocyte extract) adjuvant immunotherapy: a phase I study in non-small cell lung cancer patients. Cytotherapy. (2008) 10:490–6. doi: 10.1080/14653240802165681
73. Butts C, Socinski MA, Mitchell PL, Thatcher N, Havel L, Krzakowski M, et al. Tecemotide (L-BLP25) versus placebo after chemoradiotherapy for stage III non-small-cell lung cancer (START): a randomised, double-blind, phase 3 trial. Lancet Oncol. (2014) 15:59–68. doi: 10.1016/S1470-2045(13)70510-2
74. Chen W, Shin KH, Kim S, Shon WJ, Kim RH, Park NH, et al. hTERT peptide fragment GV1001 demonstrates radioprotective and antifibrotic effects through suppression of TGFbeta signaling. Int J Mol Med. (2018) 41:3211–20. doi: 10.3892/ijmm.2018.3566
75. Qian H, Wang H, Guan X, Yi Z, Ma F. Adoptive immunotherapy combined chemoradiotherapy for non-small-cell lung cancer: a meta-analysis. Anticancer Drugs. (2016) 27:433–8. doi: 10.1097/CAD.0000000000000346
76. Xiao Z, Wang CQ, Zhou MH, Li NN, Liu SY, He YJ, et al. Clinical efficacy and safety of CIK plus radiotherapy for lung cancer: a meta-analysis of 16 randomized controlled trials. Int Immunopharmacol. (2018) 61:363–75. doi: 10.1016/j.intimp.2018.06.012
77. Antonia SJ, Villegas A, Daniel D, Vicente D, Murakami S, Hui R, et al. Durvalumab after chemoradiotherapy in stage III non-small-cell lung cancer. N Engl J Med. (2017) 377:1919–29. doi: 10.1056/NEJMoa1709937
78. Planchard D, Popat S, Kerr K, Novello S, Smit EF, Faivre-Finn C, et al. Metastatic non-small cell lung cancer: ESMO Clinical Practice Guidelines for diagnosis, treatment and follow-up. Ann Oncol. (2019) 30:863–70. doi: 10.1093/annonc/mdy474
79. Dingemans AC, Hendriks LEL, Berghmans T, Levy A, Hasan B, Faivre-Finn C, et al. Definition of synchronous oligo-metastatic non-small cell lung cancer - a consensus report. J Thorac Oncol. (2019). doi: 10.1016/j.jtho.2019.07.025. [Epub ahead of print].
80. Rusthoven KE, Hammerman SF, Kavanagh BD, Birtwhistle MJ, Stares M, Camidge DR. Is there a role for consolidative stereotactic body radiation therapy following first-line systemic therapy for metastatic lung cancer? A patterns-of-failure analysis. Acta Oncol. (2009) 48:578–83. doi: 10.1080/02841860802662722
81. Gomez DR, Tang C, Zhang J, Blumenschein GR, Hernandez M, Lee JJ, et al. Local consolidative therapy vs. maintenance therapy or observation for patients with oligometastatic non-small-cell lung cancer: long-term results of a multi-institutional, phase ii, randomized study. J Clin Oncol. (2019) 37:1558–65. doi: 10.1200/JCO.19.00201
82. Iyengar P, Wardak Z, Gerber DE, Tumati V, Ahn C, Hughes RS, et al. Consolidative radiotherapy for limited metastatic non-small-cell lung cancer: a phase 2 randomized clinical trial. JAMA Oncol. (2018) 4:e173501. doi: 10.1001/jamaoncol.2017.3501
83. Palma DA, Olson R, Harrow S, Gaede S, Louie AV, Haasbeek C, et al. Stereotactic ablative radiotherapy versus standard of care palliative treatment in patients with oligometastatic cancers (SABR-COMET): a randomised, phase 2, open-label trial. Lancet. (2019) 393:2051–8. doi: 10.1016/S0140-6736(18)32487-5
84. van den Heuvel MM, Verheij M, Boshuizen R, Belderbos J, Dingemans AM, De Ruysscher D, et al. NHS-IL2 combined with radiotherapy: preclinical rationale and phase Ib trial results in metastatic non-small cell lung cancer following first-line chemotherapy. J Transl Med. (2015) 13:32. doi: 10.1186/s12967-015-0397-0
85. Golden EB, Chhabra A, Chachoua A, Adams S, Donach M, Fenton-Kerimian M, et al. Local radiotherapy and granulocyte-macrophage colony-stimulating factor to generate abscopal responses in patients with metastatic solid tumours: a proof-of-principle trial. Lancet Oncol. (2015) 16:795–803. doi: 10.1016/S1470-2045(15)00054-6
86. Ohri N, Halmos B, Cheng H, Abrahmam T, Yahya T, Garg M, et al. FLT3 ligand (CDX-301) and stereotactic radiotherapy for advanced non-small cell lung cancer. Cancer Res. (2018) 78(13 Suppl.):CT005. doi: 10.1158/1538-7445.AM2018-CT005
87. Papachristofilou A, Hipp MM, Klinkhardt U, Früh M, Sebastian M, Weiss C, et al. Phase Ib evaluation of a self-adjuvanted protamine formulated mRNA-based active cancer immunotherapy, BI1361849 (CV9202), combined with local radiation treatment in patients with stage IV non-small cell lung cancer. J Immunother Cancer. (2019) 7:38. doi: 10.1186/s40425-019-0520-5
88. Formenti SC, Rudqvist NP, Golden E, Cooper B, Wennerberg E, Lhuillier C, et al. Radiotherapy induces responses of lung cancer to CTLA-4 blockade. Nat Med. (2018) 24:1845–51. doi: 10.1038/s41591-018-0232-2
89. Tang C, de Groot P, Hess K, Shabaan S, Gomez DR, Chang JY, et al. Phase 1 study of pembrolizumab and stereotactic or hypofractionated radiation for metastatic non-small cell lung cancer. Int J Radiat Oncol Biol Phys. (2017) 99:S160–1. doi: 10.1016/j.ijrobp.2017.06.370
90. Kumar R, Walder DP, Pejanaute A, Gunapala R, Bhosle J, Yousef N, et al. Phase I dose escalation of pembrolizumab given concurrently with palliative thoracic radiotherapy (RT) for NSCLC. Ann Oncol. (2018) 29(Suppl. 8):mdy292.087. doi: 10.1093/annonc/mdy292.087
91. Decker R, Goldberg S, Nath S, Husain Z, Lilenbaum R, Schalper K, et al. A phase i/ii trial evaluating the combination of stereotactic body radiotherapy and pembrolizumab in metastatic NSCLC: topic: IT. J Thorac Oncol. (2017) 12:S1303–4. doi: 10.1016/j.jtho.2016.11.1843
92. Moreno V, Gil-Martin M, Johnson M, Aljumaily R, Lopez-Crido MP, Northfelt D, et al. Cemiplimab, a human monoclonal anti-PD-1, alone or in combination with radiotherapy: phase 1 NSCLC expansion cohorts. J Thorac Oncol. (2018) 13:S366. doi: 10.1016/j.jtho.2018.08.340
93. Alameddine R, Wong P, Masucci L, Roberge D, Menard C, Routy B, et al. Early safety data of a phase I/II combining nivolumab and stereotactic brain radiosurgery for treatment of brain metastases in patients with NSCLC. J Thorac Oncol. (2018) 13:S385. doi: 10.1016/j.jtho.2018.08.384
94. Miyamoto S, Nomura R, Sato K, Awano N, Kuse N, Inomata M, et al. Nivolumab and stereotactic radiation therapy for the treatment of patients with Stage IV non-small-cell lung cancer. Jpn J Clin Oncol. (2019) 49:160–4. doi: 10.1093/jjco/hyy171
95. Theelen WSME, Peulen HMU, Lalezari F, van der Noort V, de Vries JF, Aerts JGJV, et al. Effect of pembrolizumab after stereotactic body radiotherapy vs pembrolizumab alone on tumor response in patients with advanced non-small cell lung cancer: results of the PEMBRO-RT Phase 2 randomized clinical trial. JAMA Oncol. (2019) 5:1276–82. doi: 10.1001/jamaoncol.2019.1478
96. Luke JJ, Lemons JM, Karrison TG, Pitroda SP, Melotek JM, Zha Y, et al. Safety and clinical activity of pembrolizumab and multisite stereotactic body radiotherapy in patients with advanced solid tumors. J Clin Oncol. (2018) 36:1611–8. doi: 10.1200/JCO.2017.76.2229
97. Bauml JM, Mick R, Ciunci C, Aggarwal C, Davis C, Evans T, et al. Pembrolizumab after completion of locally ablative therapy for oligometastatic non-small cell lung cancer: a phase 2 trial. JAMA Oncol. (2019) 5:1283–90. doi: 10.1001/jamaoncol.2019.1449
98. Giaccone G, Bazhenova LA, Nemunaitis J, Tan M, Juhász E, Ramlau R, et al. A phase III study of belagenpumatucel-L, an allogeneic tumour cell vaccine, as maintenance therapy for non-small cell lung cancer. Eur J Cancer. (2015) 51:2321–9. doi: 10.1016/j.ejca.2015.07.035
99. Sebastian M, Schröder A, Scheel B, Hong HS, Muth A, von Boehmer L, et al. A phase I/IIa study of the mRNA-based cancer immunotherapy CV9201 in patients with stage IIIB/IV non-small cell lung cancer. Cancer Immunol Immunother. (2019) 68:799–812. doi: 10.1007/s00262-019-02315-x
100. Campbell AM, Cai WL, Burkhadt D, Gettinger SN, Goldberg SB, Amodio M, et al. Final results of a phase ii prospective trial evaluating the combination of stereotactic body radiotherapy (sbrt) with concurrent pembrolizumab in patients with metastatic non-small cell lung cancer (NSCLC). Int J Radiat Oncol Biol Phys. (2019) 105:S36–7. doi: 10.1016/j.ijrobp.2019.06.453
101. Brooks ED, Chang JY. Time to abandon single-site irradiation for inducing abscopal effects. Nat Rev Clin Oncol. (2019) 16:123–35. doi: 10.1038/s41571-018-0119-7
102. van den Berg LL, Klinkenberg TJ, Groen HJ, Widder J. Patterns of recurrence and survival after surgery or stereotactic radiotherapy for early stage NSCLC. J Thorac Oncol. (2015) 10:826–31. doi: 10.1097/JTO.0000000000000483
103. Chi A, Liao Z, Nguyen NP, Xu J, Stea B, Komaki R. Systemic review of the patterns of failure following stereotactic body radiation therapy in early-stage non-small-cell lung cancer: clinical implications. Radiother Oncol. (2010) 94:1–11. doi: 10.1016/j.radonc.2009.12.008
104. Senthi S, Lagerwaard FJ, Haasbeek CJ, Slotman BJ, Senan S. Patterns of disease recurrence after stereotactic ablative radiotherapy for early stage non-small-cell lung cancer: a retrospective analysis. Lancet Oncol. (2012) 13:802–9. doi: 10.1016/S1470-2045(12)70242-5
105. Matsuo Y, Shibuya K, Nagata Y, Takayama K, Norihisa Y, Mizowaki T, et al. Prognostic factors in stereotactic body radiotherapy for non-small-cell lung cancer. Int J Radiat Oncol Biol Phys. (2011) 79:1104–11. doi: 10.1016/j.ijrobp.2009.12.022
106. Leeman JE, Rimner A, Montecalvo J, Hsu M, Zhang Z, von Reibnitz D, et al. Histologic subtype in core lung biopsies of early-stage lung adenocarcinoma is a prognostic factor for treatment response and failure patterns after stereotactic body radiation therapy. Int J Radiat Oncol Biol Phys. (2017) 97:138–45. doi: 10.1016/j.ijrobp.2016.09.037
Keywords: non-small-cell lung cancer, immunotherapy, checkpoint inhibitor, stereotactic body radiation therapy, radiotherapy
Citation: Spaas M and Lievens Y (2019) Is the Combination of Immunotherapy and Radiotherapy in Non-small Cell Lung Cancer a Feasible and Effective Approach? Front. Med. 6:244. doi: 10.3389/fmed.2019.00244
Received: 31 August 2019; Accepted: 14 October 2019;
Published: 07 November 2019.
Edited by:
Thierry Berghmans, Institut Jules Bordet, BelgiumReviewed by:
Paul Jules Van Houtte, Institut Jules Bordet, BelgiumAntonin Levy, Institut Gustave Roussy, France
Copyright © 2019 Spaas and Lievens. This is an open-access article distributed under the terms of the Creative Commons Attribution License (CC BY). The use, distribution or reproduction in other forums is permitted, provided the original author(s) and the copyright owner(s) are credited and that the original publication in this journal is cited, in accordance with accepted academic practice. No use, distribution or reproduction is permitted which does not comply with these terms.
*Correspondence: Yolande Lievens, yolande.lievens@uzgent.be