- 1Department of Pneumology, CHU Grenoble Alpes, Grenoble, France
- 2Medicine Faculty, Université Grenoble Alpes, Grenoble, France
- 3Departement of Pathological Anatomy and Cytology, Pôle de Biologie et Pathologie, CHU Grenoble Alpes, Grenoble, France
- 4UGA/INSERM U1209/CNRS 5309-Institute for Advanced Biosciences - Université Grenoble Alpes, Grenoble, France
- 5Department of Biochemistry, Molecular Biology and Environmental Toxicology, CHU Grenoble Alpes, Grenoble, France
- 6Cancer Research Center Lyon, Centre Léon Bérard, Lyon, France
Non-small-cell lung cancers (NSCLC) represent 85% of all lung cancers, with adenocarcinoma as the most common subtype. Since the 2000's, the discovery of molecular alterations including epidermal growth factor receptor (EGFR) mutations and anaplastic lymphoma kinase (ALK) rearrangements together with the development of specific tyrosine kinase inhibitors (TKIs) has facilitated the development of personalized medicine in the management of this disease. This review focuses on the biology of molecular alterations in NSCLC as well as the diagnostic tools and therapeutic alternatives available for each targetable alteration. Rapid and sensitive methods are essential to detect gene alterations, using tumor tissue biopsies or liquid biopsies. Massive parallel sequencing or Next Generation Sequencing (NGS) allows to simultaneously analyze numerous genes from relatively low amounts of DNA. The detection of oncogenic fusions can be conducted using fluorescence in situ hybridization, reverse-transcription polymerase chain reaction, immunohistochemistry, or NGS. EGFR mutations, ALK and ROS1 rearrangements, MET (MET proto-oncogenereceptor tyrosine kinase), BRAF (B-Raf proto-oncogen serine/threonine kinase), NTRK (neurotrophic tropomyosin receptor kinase), and RET (ret proto-oncogene) alterations are described with their respective TKIs, either already authorized or still in development. We have herein paid particular attention to the mechanisms of resistance to EGFR and ALK-TKI. As a wealth of diagnostic tools and personalized treatments are currently under development, a close collaboration between molecular biologists, pathologists, and oncologists is crucial.
Key Concepts
• Massive parallel sequencing, known as NGS, could be particularly suited to multiplexed assessment of driving somatic alterations.
• Gene rearrangements are rare events, with no single detection technique shown to be 100% sensitive and specific. NGS platforms present several key advantages over FISH, IHC, or RT-PCR.
• Despite an initial response to EGFR inhibitors, patients develop acquired resistance and relapse. The most frequent mechanism of resistance (50–60%) is the T790M mutation in exon 20.
• The strategy of sequencing ALK inhibitors was associated with a median overall survival of 81–89.6 months.
Introduction
Lung cancer is the leading cause of cancer mortality, accounting for 1,600,000 annual deaths worldwide and results in a 5-years survival rate of 19%. Non-small-cell lung cancers (NSCLC) represent 85% of all lung cancers, with adenocarcinoma as the most common subtype. Lung cancers are often diagnosed at an advanced stage, with worse prognosis (1).
In the 2000's, the discovery of epidermal growth factor receptor (EGFR) mutations and a specific tyrosine kinase inhibitor (TKI) gefitinib, led to the development of personalized treatments for NSCLC (2). Over the last 2 decades, other NSCLC genetic alterations have been described, such as anaplastic lymphoma kinase (ALK), ROS proto-oncogene 1 (ROS1), rearranged during transfection proto-oncogene gene (RET), neurotrophin kinase (NTRK) gene rearrangements, B-Raf proto-oncogene, serine/threonine kinase (BRAF) mutations, MET proto-oncogene (MET) amplification, as well as MET exon 14 skipping (3–9). These genes encode tyrosine kinase receptors (TKR) and their alterations have been shown to induce their constitutive activation, thereby driving carcinogenesis through intra-cellular signaling. Their inhibition by specific TKIs leads to cellular apoptosis. Several EGFR-, ALK,- or ROS1-inhibitors, currently used in clinical settings, have considerably improved NSCLC patients' overall response rates (ORR), progression-free survival (PFS), and overall survival (OS), compared to standard chemotherapy.
Consequently, molecular profiling of patients with advanced NSCLC is now systematically performed in lung adenocarcinoma patients, with a targetable molecular alteration found in 15–20% of Caucasian patients (10). In clinical practice, different molecular diagnostic tools are employed for detecting these alterations, such as immunochemistry (IHC), fluorescent in situ hybridization (FISH), and DNA- or RNA-based sequencing.
This review focuses on the biology of molecular alterations in NSCLC, and on diagnostic tools and therapeutic alternatives for each targetable alteration (Table 1).
Biology of EGFR and ALK alterations in NSCLC
EGFR Mutations
EGFR, a member of the EGFR family, is a TKR that activates multiple signal transduction pathways. In NSCLC, EGFR mutations correspond to somatic gain-of-function mutations, occurring within the tyrosine kinase domain (14). These alterations commonly consist of in-frame deletions in exon 19 (45–50%) or the L858R substitution in exon 21 (40–45%). Uncommon alterations represent 10% of EGFR mutations, which induce a heterogeneous response to EGFR-TKIs, along with a poorer prognosis than that of more frequent mutations (15, 16). Exon 18 G719X are the most frequent alterations in this subgroup, accounting for 28% of all rare mutations, is followed by exon 21 L861Q (16–35% of cases) and exon 20 S768I (5% of cases) alterations (17, 18).
EGFR mutations are identified in 11% of NSCLCs and in 44% of non-smoker patients (10). These alterations are mainly observed in non-smoking, Asian, and female patients.
ALK Rearrangements
The ALK gene is located on the short arm of chromosome 2 and encodes a TKR, member of the insulin receptor family. The ALK receptor is activated by two ligands: FAM150A and FAM150B (19, 20). The precise role of the ALK protein in humans is still unknown, whereas the ALK gene plays a role in mice's neuronal development and testicular function (21, 22). The ALK protein is physiologically not expressed in the lung tissue. These alterations correspond to either an inversion or translocation, leading to a fusion between the 3′ portion of ALK and 5′ portion of a partner gene. These fusion genes encode a fusion protein that activates signaling pathways (e.g., PI3K-AKT, JAK-STAT, MAPK pathways), promoting carcinogenesis (23).
In NSCLC, several fusion partners have been described. The most common of these is echinoderm microtubule-associated protein like-4 (EML4), located on the short arm of chromosome 2 but separated from ALK by 12 Mb (24). The breakpoint site in the partner gene can occur within different exons, thereby defining the fusion variant. Consequently, different fusion variants have been identified, the most frequent being EML4(13)-ALK(20), which consists of a fusion between exon 13 of EML4 and exon 20 of ALK. This is also known as EML4-ALK variant 1. Three characteristics are shared by the reported ALK fusion variants. First, the entire ALK kinase domain is conserved. Second and third, the partner promoter and its oligomerization domain are both preserved, inducing an aberrant expression and constitutive activation of the fusion protein (25). As a result, levels of ALK fusion protein expression, along with invasion or proliferation capacities of the tumor cells, could depend on the nature of the fusion variant (26). Moreover, the breakpoint site within the gene partner could affect protein stability and, thus, treatment sensitivity (27, 28). Some clinical data showed a link between the variant nature and the TKI response (29).
ALK rearrangements are rare. They are identified in 2–7% of NSCLCs and 15% of non-smoker patients, mainly in young patients (3, 10, 30, 31).
Diagnostic Tools for Detecting Molecular Alterations in a Clinical Setting
Accurate and timely detection of these oncogenic alterations, has been proven crucial, as the products of these alterations can be targeted by a growing list of inhibitors, leading to tumor growth inhibition and regression.
Methods for Genotyping Tumor Tissues or Liquid Biopsies
Genotyping of somatic genetic alterations has become routine practice for patient management from baseline to disease's progression following targeted therapies. Lung cancers are predominantly diagnosed through biopsy, but the quantity of tumor cells in each biopsy varies, largely depending on tumor cellularity and size of the specimen acquired. Furthermore, most tumor tissues are preserved in formalin-fixed paraffin-embedded (FFPE) blocks, which crosslink the nucleic acids, thereby resulting in fragmented DNA. Finally, EGFR testing should be available as soon as possible to enable first-line therapy using EGFR antagonists. Therefore, genotyping of NSCLC biopsies requires rapid and sensitive technologies that only require a small amount of input DNA.
While Sanger sequencing, the gold standard technology that characterizes a mutation in hereditary disease, proves to be not sensitive enough, numerous alternative sequencing methods have been developed and validated for the clinic (Table 2). In France, a multicenter study named ERMETIC (Evaluation of EGFR Mutation Status for the administration of EGFR-TKIs in Non-Small Cell Lung Carcinoma) was conducted to validate numerous methods based on restriction enzyme analysis, allele-specific amplification, single-base extensions, fluorogenic allele-specific oligonucleotide hybridization probe, pyro-sequencing, or high-resolution melting (33).
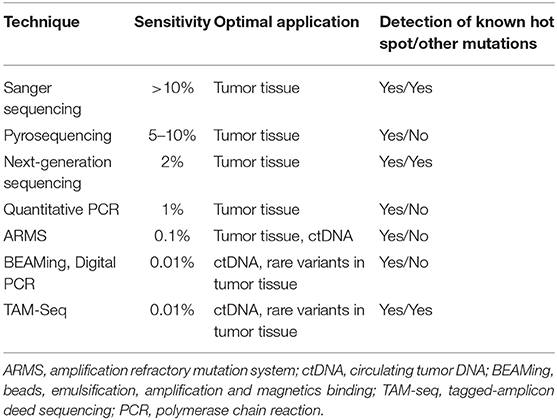
Table 2. Methodologies for detecting mutations [modified according to Diaz and Bardelli (32)].
All these methods are single-gene approaches and must be multiplied by a rapidly growing number of predictive markers. Massive parallel sequencing, also known as next-generation sequencing (NGS), is particularly well-suited to the multiplexed assessment of somatic alterations (34). Associated with panel development, bioinformatic supports permit to evaluate the sequencing coverage and to characterize and annotate not only known hot spot mutation but also other molecular anomalies [non-hot-spot mutations, gene copy number (CNV)]. For clinical applications, targeted sequencing of a limited set of essential genes has so far proven to be the most practical approach. Indeed, targeted panels offer the advantage of high depth (>300X), as well as high overall exon coverage (>99%). These two quality markers secure the sensitivity of NGS at around 2%. Thus, the choice of a specific clinical NGS assay requires careful consideration of panel size, inclusion of appropriate markers, the ability to detect multiple genomic aberration types, and compatibility with a low quality and quantity of nucleic acids (35). This choice is also dependent of the analysis's cost. Sabatine et al. (36) have demonstrated that NGS price varies from about 600–3,400$ depending on the number of genes including in the panel. Recently, Simarro et al. (37) have compared cost of conventional molecular analysis for detection of EGFR, ALK, and ROS1 anomalies (367.66€) to targeted NGS analysis (421.23€) and conclude that NGS could be implemented to routine diagnosis at reasonable costs.
When tumor tissue specimens are insufficient, not contributive, or not obtainable, molecular analyses are conducted on liquid biopsies. Usually, a liquid biopsy corresponds to circulating tumor DNA (ctDNA), which represents only a small proportion (<1%) of circulating free DNA (cfDNA). Discriminating ctDNA from normal cfDNA is aided by the fact that tumor DNA is defined by the presence of somatic mutations. Recently, the development of sensitive and accurate assays has facilitated the detection and quantification of rare variants among a large excess of normal sequences. At this time, the commercially available Cobas EGFR mutation test is the only technology approved by the United-Stated (US) Food and Drug Administration (FDA) for the molecular analysis of liquid biopsy specimens in NSCLC. Meanwhile, several digital genomic technologies have been validated for clinical use (38, 39). While these methods exhibit very high sensitivity (around 0.01%), they remain single-gene approaches. New NGS developments are particularly focused on molecular barcodes called unique molecular identifiers (UMIs) and improved bioinformatics pipelines, able to discriminate mutations with a very low allele frequency from sequencing background (40). The MOSCATO trial has demonstrated a good concordance between tumor biopsies and cfDNA NGS analyses (41). This study also demonstrate that molecular diagnosis (comprising tumor biopsy, dispatch of biological samples, histological control, CGH array, targeted NGS, bioinformatics analysis and multidisciplinary molecular tumor board) represent a modest part (6%) of the overall cost of a molecular-guided therapy (42).
Tools for Detecting Oncogenic Fusions
In NSCLC, kinase genes, such as ALK, ROS1, RET, NTRK1, and NTRK3 [but also BRAF, MET, EGFR, and fibroblast growth factor receptor (FGFR)] or other proto-oncogenes such as neuregulin 1 (NRG1), may all be subject to gene rearrangements that lead to constitutive downstream pathway activation (3, 6, 11, 43–45). Since 2007, a wealth of literature exploring ALK rearrangements has shown that the detection of gene fusions can prove challenging, because the genomic rearrangements leading to these fusions can be either intra- or interchromosomal (3). In addition, these ALK rearrangements have been shown to have multiple partner genes (46–49). In these somatic rearrangements, the 5′ portion of a gene that is expressed by the tumor cell progenitor is fused to the 3′ portion of the proto-oncogene. If an in-frame fusion gene is formed, it is then transcribed into an mRNA fusion transcript that encodes a fusion protein. In the case of kinase gene rearrangements, the fusion protein consists of the N-terminus of the fusion partner, generally containing a dimerization domain, fused to the C-terminus portion of the target gene, containing the kinase domain (Figure 1).
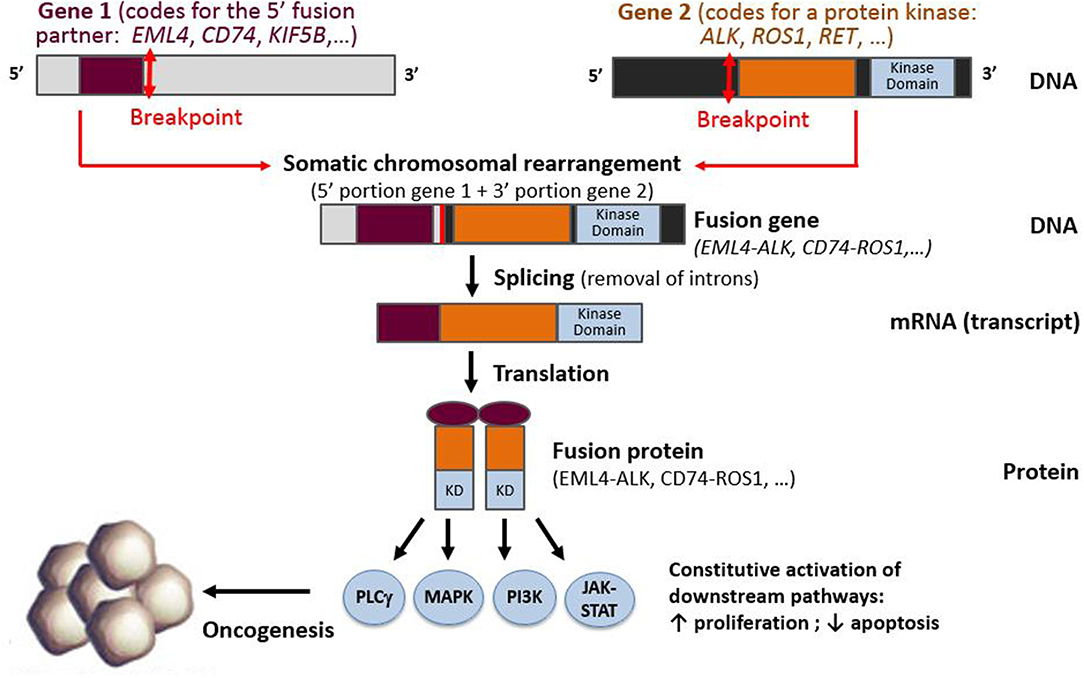
Figure 1. Schematic representation of a fusion between the 3′ portion of a protein kinase gene (containing the kinase domain) and 5′ part of a fusion partner gene, resulting in the production of a fusion gene, fusion transcript, and fusion protein.
Several molecular tools are currently available for identifying oncogenic fusions in tumor specimens, enabling the detection of these fusions at the DNA, RNA, or protein levels:
- FISH allows the detection of gene rearrangements at the DNA level. The most common assays are based on the use of specific break-apart probes, which are located on either side of the breakpoint in the target gene. This technique was initially considered the gold standard for detecting gene rearrangements, and ALK FISH was FDA-approved as a companion diagnostic to help identify patients eligible for crizotinib treatment. However, FISH can lead to false-positive results, because some rearrangements detected on the DNA may not produce a fusion transcript. This approach can additionally miss some small intrachromosomal rearrangements, leading to false-negative results (50–55). Moreover, this technique is not able to provide any indication regarding the nature of the fusion variant (i.e., the fusion partner and introns/exons involved in the fusion).
- Reverse-transcription PCR (RT-PCR) allows the detection of fusion transcripts, although a high number of primer pairs is required for it to be able to detect all known fusions. This is associated with a high risk of false-negatives, considering the diversity of fusions possible, and this approach misses the detection of unknown fusions (56, 57).
- Immunohistochemistry (IHC) can be used to detect fusion proteins, provided a sensitive, specific, and clinically validated antibody is available. This approach is based on the assumption that these antibodies are not specifically directed against fusion proteins, but rather toward their active domain. ALK immunohistochemistry (clone D5F3) has received FDA approval as a companion diagnostic tool, and comparable results have been obtained with the 5A4 clone, provided validated methods are employed (56–63). There are currently no approved IHC-based tests for detecting other fusion proteins, though commercial antibodies are available for identifying ROS1 and TRK proteins.
- NGS, or massive parallel sequencing, assays represent a multiplexed approach to tumor samples' molecular characterization. Considering the high number of molecular alterations described so far in NSCLCs, these assays are likely to experience a bright future in the clinical setting. NGS assays performed on either tissue DNA or RNA enable full characterization of the genes involved in the fusion (rearranged gene and partner gene). However, some of the DNA panels currently available are not designed to detect all possible fusions. Indeed, some panels display only partial intron coverage, especially for genes containing large introns, such as the NTRK genes, or introns with repetitive regions. This can lead to false-negative results, because most breakpoints occur within introns. As with FISH, DNA-based NGS can generate false-positive results, given that some gene rearrangements detected on DNA are unlikely to produce a fusion transcript (50, 64). Conversely, Davies and colleagues recently compared DNA-based and RNA-based NGS assays on 14 ROS1-positive samples. In their study, these authors pointed out that the genomic breakpoint was an unreliable predictor of the transcript-level breakpoint. Moreover, some DNA breakpoints predicted to yield an out-of-frame transcript by DNA-sequencing actually produced an in-frame transcript that was detected by RNA-sequencing (47). Using RNA-based NGS assays for detecting gene rearrangements thus appears to be preferable, because these techniques allow for detecting fusions within the coding regions, in addition to fusions that are likely to result in an expressed fusion protein. This is, however, only applicable if the extracted RNA is of sufficient quality and quantity, which is, undoubtedly, the most critical limitation of these assays (56, 65, 66). Last but not least, NGS approaches permit the full characterization of the introns/exons involved in the fusions, which could provide essential information for the clinicians, if specific fusion variants are found to display different biologic and clinical features, as previously proposed in ALK- or RET-rearranged lung cancers (67–69).
Gene rearrangements are rare events, and no single detection technique has been shown to be 100% sensitive and specific. However, NGS platforms present several key advantages over FISH, IHC, or RT-PCR, given that these platforms not only allow for multiplex testing of both point mutations and rearrangements, but additionally aid molecular characterization of the fusion variant produced. This kind of information could later gain clinical relevance, if some variants prove to be superior predictors of response than others (56, 65, 70–74).
Therapeutics and Resistance
EGFR
Since 2009, several first-line Phase III trials have revealed the impressive clinical activity of first- (gefitinib and erlotinib) and second- (afatinib) generation EGFR-TKIs over platinum-doublet chemotherapy, for advanced NSCLC EGFR-mutated patients (75–82). Despite the lack of proven evidence regarding OS benefits, probably due to the high percentage of crossed-over patients, these first- and second-generation EGFR-TKIs are clearly associated with a significant benefit in terms of PFS and ORR, namely 9–14 months and 60–70%, respectively. Dacomitinib, a second-generation EGFR-TKI, was investigated in the Phase III ARCHER 1050 trial. This agent was shown to significantly improve both PFS (9.2 vs. 14.7 months, HR 0.59; p < 0.0001) and OS (34.1 vs. 26.8 months, HR 0.76; p = 0.0438) compared to gefitinib (83, 84). Nevertheless, all four drugs received FDA approbation in the first-line setting, without any distinction made in terms of patient selection (Table 3).
Despite an initial response to EGFR inhibitors, patients are most likely to develop acquired resistance and relapse after 8–13 months of treatment (89). Several mechanisms have been proposed to account for this. The most common (50–60% of cases) is the T790M mutation in exon 20, which changes the conformation of the protein and prevents TKIs' binding to the ATP pocket (90, 91). Another resistance mechanism is the activation of bypass signaling pathways, such as HER2 or MET (92, 93), as well as EGFR downstream signaling pathways (94). Additionally, phenotypical transformations, such as SCLC transformation and epithelial mesenchymal transition (EMT), have been described as resistance mechanisms to EGFR-TKIs (95, 96).
Osimertinib, a third-generation EGFR-TKI, was recently approved as either first-line agent for metastatic and locally advanced EGFR mutant patients or second-line drug for those presenting the T790M resistance mechanism (97). In the Phase III FLAURA trial, osimertinib nearly doubled the median PFS, compared to erlotinib or gefitinib (18.9 vs. 10.2 months, HR 0.46; p < 0.001). It should, however, be noted that the OS data were not yet mature at analysis (98). Considering these crowded scenarios, the first-line choice should primarily be driven by the patient's characteristics and tolerability profile. In the FLAURA trial, osimertinib had a lower rate of Grade 3–4 undesirable events compared to first-generation EGFR-TKIs (34 vs. 45%). Furthermore, the hazard ratio for PFS was similar in patients either with or without known brain metastasis (0.47 and 0.46, respectively). These data have been summarized in Figure 2.
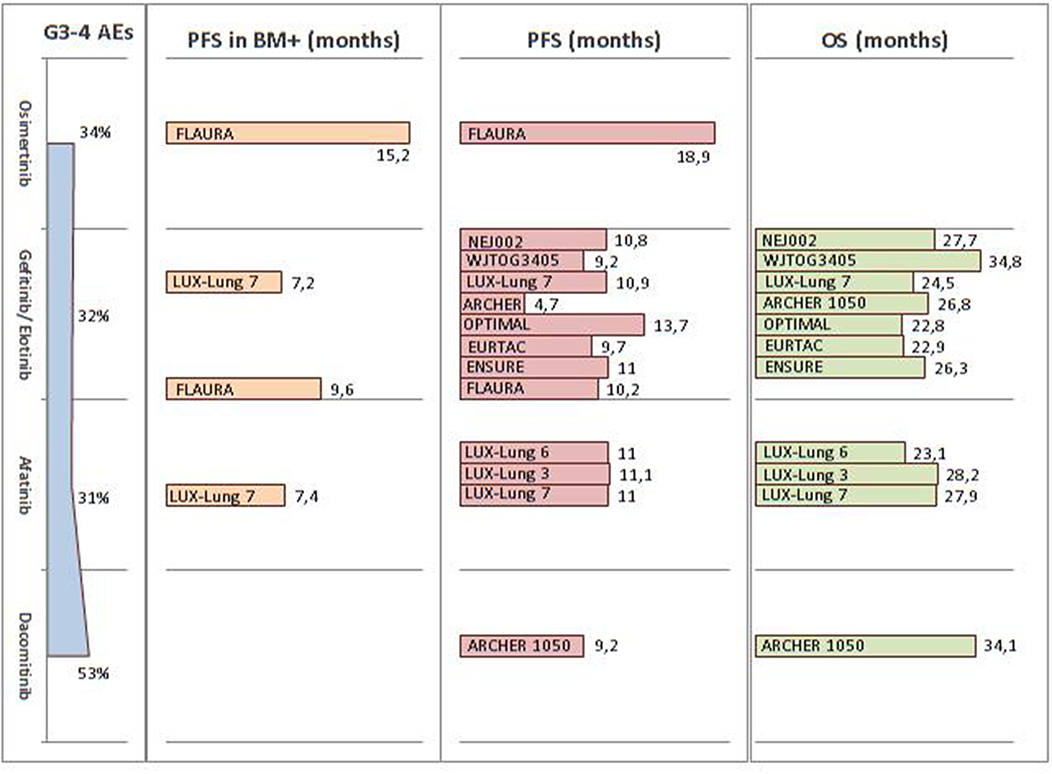
Figure 2. Summary of FDA and EMA approved EGFR-TKI efficacy and tolerability across Phase III trials in the first-line setting. FDA, Food and Drug Administration; EMA, European Medicines Agency; EGFR-TKI, epidermal growth factor receptor tyrosine kinase inhibitors; AE, adverse event; PFS, progression-free survival; OS, overall survival; BM, brain metastasis.
Despite the impressive efficacy of third-generation EGFR TKIs in T790M positive NSCLC, acquired resistance invariability is likely to occur via several, though only partially-known, mechanisms. These resistance mechanisms can be divided into three categories: 1) reactivation of the EGFR pathway through EGFR novel mutations; 2) activation of bypass signaling pathways; 3) histological shift able to bypass the EGFR-dependence in form of or small-cell lung cancer (SCLC) transformation (99–101). At resistance, around 50% of patients were demonstrated, across different series, to maintain the T790M mutation. Novel EGFR mutations represent the most commonly acquired resistance mechanism in this group, while the C797S mutation accounts for 22–40% of all progressions under third-generation EGFR-TKIs (100, 102). Interestingly, cell lines harboring dual C797S and T790M alteration in trans were sensitive to a combination of first- and third-generation EGFR-TKIs, as opposed to a mutation in cis (103). Conversely, patients with a loss of T790M alteration at resistance more frequently presented an EGFR bypass alteration, either by downstream EGFR (RAS-MAPK pathway) signaling or activating parallel signaling pathways, such as MET or ERBB2 amplifications, PIK3CA mutations, and PTEN loss (99–101). These data highlight the urgent need for investigative or combination therapies to effectively prevent or treat the drug resistance complexity in EGFR-mutant lung cancer.
Other uncommon alterations (exon 18 G719, exon 21 L861Q, and exon 20 S768I) are recognized as sensitizing EGFR-TKIs, with different magnitudes of response. In a large cohort of EGFR-mutated patients treated with first-generation EGFR-TKIs, ORR was significantly lower in patients with uncommon alterations, compared to classical EGFR mutations (47.5 vs. 74.1%; p < 0.001) (104). Other studies have similarly reported data concerning the effectiveness of EGFR-TKIs on these rare mutations. These include a combined post-hoc analysis of LUX-Lung 2, LUX-Lung 3, and LUX-Lung 6 (105), in addition to the Phase II trial KCSG-LU15-09 (106). Among the upcoming target agents, poziotinib demonstrated promising activity in a Phase II trial that enrolled pretreated NSCLC patients with EGFR exon 20 mutation (excluding acquired T790M). This resulted in 55% ORR and 5.5 months mPFS, along with a high rate of Grade 3–4 undesirable events (56%) (107).
ALK
Five ALK inhibitors are currently available: first generation (crizotinib), second generation (alectinib, ceritinib, and brigatinib), and third generation (lorlatinib). These therapies have dramatically changed patients' prognosis, as they have improved the median OS from diagnosis made in the metastatic stage. Indeed, the strategy using sequencing ALK inhibitors has resulted in a median 81 months OS in two recent studies (108, 109).
Crizotinib, a molecule-targeting MET, ROS1, and ALK, was the first TKI to generate promising results in ALK-positive patients (30). Compared to standard chemotherapy in ALK-positive advanced NSCLC, crizotinib demonstrated a longer median PFS (10.9 vs. 7 months; HR 0.45), along with a higher ORR (74 vs. 45%) (110). After crossover adjustments, OS was increased in the crizotinib arm (HR 0.346) (111). Compared to chemotherapy, crizotinib was found to be well-tolerated, primarily causing color vision disorders, digestive disorders, or edema. Ceritinib was shown associated with a doubled median PFS (16.6 months), in comparison to chemotherapy (8.1 months) (HR 0.55) (88). In this Phase 3 study, among 22 patients with brain metastases, the intracranial ORR was 72.7% in the ceritinib group and 27.3% in the chemotherapy group. The most common undesirable events of ceritinib included digestive disorders and increased alanine aminotransferase levels. The ALEX trial, which compared alectinib to crizotinib in the first-line setting, has proven an higher alectinib efficiency, with a comparable safety profile (86, 87). Indeed, the median PFS in the alectinib arm was 34.8 months vs. 10.9 in the crizotinib arm (HR 0.43). Furthermore, alectinib exhibited a better cerebral activity, with a median PFS of 27.7 months in patients with central nervous system (CNS) metastases at baseline, compared to 7.4 months for the crizotinib group (HR 0.35; 95% CI: 0.22–0.56). Alectinib was shown to be associated with a lower incidence of CNS progression (four times less) in patients without CNS metastasis at diagnosis, compared to crizotinib. Considering its safety profile, alectinib primarily induced myalgia, anemia, and liver disorders. Finally, in the first-line setting, brigatinib has been proven superior over crizotinib, in terms of efficacy (85). Indeed, the 12-months PFS in the brigatinib group was higher (67%) than in the crizotinib arm (43%), with statistically significant results (HR 0.49; 95%CI: 0.33–0.74; p < 0.001). Likewise, brigatinib was associated with an intracranial ORR of 78%, compared to 29% in the crizotinib group. Furthermore, their safety profiles were comparable. The most common undesirable events of brigatinib conprised digestive disorders, hypertension, and increased alanine aminotransferase ALAT and lipase levels. Lastly, lorlatinib has been tested in a Phase II study that enrolled 228 ALK-positive patients, with or without previous ALK-TKI or chemotherapy exposure (112). Lorlatinib showed an ORR of 90% in the treatment-naïve group (30 patients) and of 47% beyond the first-line setting (198 patients). Likewise, an intracranial response was observed in 66.7% of the treatment-naïve patients (n = 3) and in 63% of previously-treated patients (n = 81). Lorlatinib was found to induce dyslipidemia, peripheral neuropathy, and mood or cognitive effects.
However, ALK inhibitors have been shown to consistently cause the development of resistance mechanisms. After a first ALK-inhibitor, treatment choice can be guided by a new sample with the identification of an ALK-resistance mechanism (113). These mechanisms can be either ALK-dependent or ALK-independent. ALK-dependent mechanisms correspond to secondary mutations in the ALK kinase domain. These mutations induce kinase and signaling reactivation by preventing the binding of TKIs to their targets. These mutations occur in 20–30% of patients treated with crizotinib, and in 50–70% of patients after second-generation ALK inhibitor exposure (113). With second-generation inhibitors, the highly resistant G1202R mutation was shown to be more common, which then requires initiating the third-generation TKI lorlatinib (114, 115). Indeed, an in vitro study has shown different sensitivity profiles of ALK inhibitors, with respect to various resistance mutations (113). ALK amplifications were revealed to be less frequent than ALK mutations, most commonly occurring following crizotinib therapy. ALK-independent mechanisms consist of the activation of bypass signaling pathways, such as EGFR activation, MET amplification, MEK reactivation, or phenotypic changes like EMT or SCLC transformation (25, 116–119). An overexpression of P-glycoprotein (PGP), an efflux pump, was additionally identified as a potential resistance mechanism (114). PGP was shown to be responsible for decreased ALK inhibitor (crizotinib or ceritinib) concentrations in brain tissues (120, 121).
MET
The MET gene encodes a transmembrane receptor that is normally activated by the binding of its ligand, the hepatocyte growth factor (HGF). MET pathway activation is thought to occur through diverse mechanisms that influence properties affecting cancer cell survival, growth, and invasiveness (122). Somatic dysregulation at MET occurs through several different mechanisms that are non-exclusive in NSCLC, including protein overexpression, gene amplification, mutation, and rearrangement. MET amplification was revealed to be uncommon in previously-untreated NSCLC patients and was found in about 2–4% of cases. MET amplification appears to play a role in acquired resistance to EGFR inhibitors and is observed in about 5–20% of patients in this setting (12, 123, 124). MET alterations that result in exon 14 skipping were observed in about 3–4% of NSCLCs (12, 125). METex14 mutations were more frequently identified in adenocarcinoma and sarcomatoid carcinoma patients. These mutations were found more frequently in older rather than younger patients. Of note is that these mutations were revealed to be mutually exclusive to other driven mutations (126).
Several clinical trials have been carried out so far, but most of them did not produce positive results (127–129). Preliminary data on capmatinib revealed encouraging results in patients with METex14 mutations in either previously-treated or treatment-naïve NSCLC patients. The GEOMETRY trial showed a clinically meaningful ORR of 39.1% and 71.4% in previously-treated and treatment-naïve patients, respectively, with a manageable toxicity profile (130). Tepotinib, a MET-selective TKI, generated promising activity in METex14 patients, with an ORR of 59%. In terms of safety, more than 50% of patients experienced tepotinib-related treatment-emergent adverse events (TRTEAEs), including serious TRTEAEs in three cases (8.8%) (131). Crizotinib obtained FDA breakthrough designation in METex14 NSCLC, based on the results of an expansion cohort from the Phase I PROFILE 1001 study, which included 69 METex14 patients. Among 65 evaluable patients, there were three complete responses (4.6%), 18 (27.7%) partial responses, and 29 stable disease cases. Median time to response was 7.6 weeks, with a median duration of response (DOR) of 9.1 months and PFS of 7.3 months (132). The AcSé program enrolled 25 patients with MET amplification and 29 with MET mutation (25 METex14). Crizotinib showed activity in both MET-amplified and MET-mutated NSCLC (ORR: 32 and 40%, respectively) patients, whereas response correlated with the number of MET copies in the amplified group. Median PFS was around 3.5 months in both groups, while OS was longer in the METex14 population (9.5 vs. 7.7 months) (133).
BRAF
BRAF (B-Raf proto-oncogene, serine/threonine kinase) base substitutions are present in approximately 2–5% of NSCLCs; about half of these mutations result in V600E amino acid substitution (13, 134, 135). The BRAF mutation induces the activation of the MAPK pathway, promoting cell growth, proliferation, and survival. Gene fusions that are biologically distinct from V600E mutations have been identified more rarely, in 0.2% NSCLC cases (136). Most patients with BRAF mutations were revealed to be former smokers, whereas non-V600E mutations were more commonly found in heavy smokers (44).
Preliminary data, pertaining to a single-agent BRAF inhibitor like dabrafenib or vemurafenib, consisted of a 30–40% ORR and 5–7 months median PFS (137, 138). The most interesting results were, nevertheless, obtained when combining dabrafenib with the MEK inhibitor trametinib. A Phase 2 trial in chemotherapy-pretreated NSCLC patients generated an OS of 18 months, compared to 12.7 months observed under dabrafenib monotherapy (139). Another Phase 2 trial in BRAF(V600E)-positive, chemo-naïve patients confirmed this combination's efficacy, reflected by a 64% ORR, along with a PFS and OS of 11 and 25 months, respectively (140). Based on these results, both the FDA and European Medicines Agency (EMA) approved in 2017 the combination of dabrafenib and trametinib for patients with advanced NSCLC harboring a V600 mutation, regardless of the therapy line.
ROS1
ROS1 rearrangements are detected in approximately 1–2% of lung adenocarcinomas (43). In Europe, ROS1-rearrangement testing is recommended in never smokers with advanced EGFR/KRAS/ALK-negative NSCLC. In current and former smokers, ROS1 testing is indicated only in non-squamous histology (141). The high amino-acid sequence homology between ROS1 and ALK kinase domains explains the significant clinical activity of crizotinib in both ROS1 and ALK-driven tumors. Indeed, in the expansion cohort of the Phase I trial PROFILE 1001, 50 ROS1-positive NSCLCs received crizotinib, reaching an ORR of 72% and a median PFS of 19.2 month (142). Median OS was 51.4 months and no correlations were observed between overall survival and specific ROS1 fusion partners (143). Several Phase II trials conducted on European and Asian cohorts showed similar results, with reported ORR of about 70% and PFS ranging from 10 to 13 months (144–146). As with other oncogene-addictions, mechanisms of acquired resistance also occur in ROS1-positive NSCLC cancers, mainly through mutations in the target itself or via activation of alternative pathways. Several molecules have shown promising results in overcoming resistance to crizotinib in ROS1+ NSCLCs. For example, ceritinib showed an ORR of 62% and a median PFS of 9.3 months in a phase II trial conducted on 28 pretreated or untreated patients with advanced ROS1-rearranged NSCLC. The median PFS was even longer (19.3 months) for crizotinib-naïve patients and the median overall survival (OS) was 24 months (147). Alectinib and brigatinib, have little to no established ROS1 inhibitory activity. By contrast, lorlatinib also appears to be able to overcome acquired resistance to crizotinib in ROS1-positive NSCLC, including disease in the CNS (112). Newer ROS1 inhibitors are in development, and investigational agents such as cabozantinib, entrectinib, repotrectinib, and DS-6051b have emerging results in early-phase clinical trials in ROS1-rearranged NSCLC (148–150).
NTRK
The NTRK genes, consisting of NTRK1, NTRK2, and NTRK3, encode the tropomyosin receptor tyrosine kinases TRKA, TRKB, and TRKC, respectively, which function during normal neuronal development and maintenance (151–153). While the frequency of NTRK fusions proves to be low in common cancer types, including NSCLC, NTRK3 fusions were revealed to be almost ubiquitous among rare cancer types, such as mammary analog secretory carcinoma and infantile fibrosarcoma (44). In NSCLC, NTRK fusions are estimated to occur at a frequency of approximately 0.1 to 1% (7, 44), whereas the clinical and pathologic features of the patients harboring such fusions have not yet been well-characterized.
Considering the rarity of these fusions, drug development has been primarily derived from basket trials. Among them, larotrectinib (LOXO-101) and entrectinib (RXDX-101) produced promising results, in fusion-positive NSCLC patients (132, 154). A Phase 1 study involving adults only, Phase 1–2 study involving children only, and Phase 2 study involving both adolescents (n = 55) and adults treated with larotrectinb showed an ORR of 75%, with the median DOR and PFS not yet reached at data cut-off. Larotrectinib tolerability was acceptable, with predominantly Grade 1 undesirable events reported (132). Entrectinib, an oral TKI of TRKA/B/C, ROS1, and ALK, was evaluated in two Phase 1 studies. These trials revealed a good toxicity profile and rapid and durable responses in patients with brain metastases (154). A Phase 2 trial with entrectinib in solid tumor patients harboring NTRK1/2/3, ROS1, or ALK gene rearrangements is still ongoing (NCT02568267), as are several other trials involving TKIs against TRKA/B/C, including TSR-011 (NCT02048488), DS-6051b (NCT02279433), and PLX7486 (NCT01804530).
RET
The RET (rearranged during transfection proto-oncogene) fusion oncogene and fusions proteins resulting from chromosomal rearrangement were initially described in thyroid carcinoma patients (155). RET fusions were subsequently identified in approximately 1–2% of NSCLC patients; it was found to be mutually exclusive with other oncogenic drivers (5, 6, 11, 156, 157). At least 12 different RET gene partners have been described, the most common being KIF5B (70% of cases), followed by CCDC6 (20% of cases) (48). Data from the Global Multicenter RET registry did not reveal any significant difference in incidences between men and women, whereas the majority of affected patients had never been smokers (63%) and suffered from a metastatic adenocarcinoma (48).
In NSCLC, many multi-targeted kinase inhibitors have been tested to date. These include cabozantinib, vandetanib, sunitinib, sorafenib, alectinib, lenvatinib, nintedanib, ponatinib, and regorafenib. Yet, their activity was shown to be clearly inferior to the responses and survival outcomes seen with selective TKIs in other oncogene-addicted NSCLC models (48, 158, 159). To date, no drugs have been approved for RET-rearranged NSCLCs (126). New RET selective inhibitors are, however, in development (e.g., LOXO-292 and BLU-667). LOXO-292 was found to generate encouraging clinical activity in RET-altered solid tumors in a Phase 1 trial, with a 77% ORR for NSCLCs patients, including those with brain metastases. At data cut-off, the median DOR was not yet reached, while the longest response already exceeded 10 months (160, 161). Based on these data, further studies are needed to further confirm this drug's potential benefit.
Conclusion
In advanced NSCLC, identifying molecular alterations proves to be a daily challenge. Tumor samples can be either tissues with low tumor cellularity or liquid biopsies. Biologists increasingly use efficient techniques to identify these oncogenic alterations. NGS platforms, therefore, occupy a central place. Other promising techniques are also emerging, such as radiogenomics, for example, which combine non-invasive imaging and molecular analysis, study the link between genomic and phenotypic information. These techniques could help for the continuous monitoring of patients with advanced NSCLC and to follow their treatment responses (162).
For patients with EGFR mutations or ALK/ROS1 rearrangements, several TKIs are currently available, designed either for front-line therapy or patients at progression. These TKIs are more effective than chemotherapy, well-tolerated and remain the reference treatment. For the other oncogenic alterations (MET, BRAF, NTRK, and RET), while several new molecules appear promising, further studies are still required. Concerning immune checkpoint inhibitors, according to a recent study, these molecules have not demonstrated a better efficacy and should be used after TKIs and chemotherapy failures, especially in ALK-positive NSCLCs (163).
As a wealth of diagnostic tools and personalized treatments are already available or still under development. A close relationship between molecular biologists, pathologists, and oncologists is crucial.
Author Contributions
JP, AM-F, MG, FF, CE, EG, and A-CT wrote/edited the manuscript and approved it for submission.
Conflict of Interest
JP reports personal fees or grants from MSD, Pierre Fabre, Roche, Pfizer, and Takeda. AM-F reports personal fees from Pfizer, Takeda, AstraZeneca, and Boehringer, research grants from Pfizer, Novartis, and Takeda, and non-financial support from Roche, Clinisciences, AstraZeneca, Takeda. MG reports personal fees from BMS, Roche, Novartis, MSD, and Astra Zeneca, as well as grants from BMS. FF reports personal fees from Roche diagnostic and AstraZeneca. EG reports personal fees from Astrazeneca, grants and personal fees from Bristol-Myers Squibb, personal fees from Roche, and personal fees from Merck Sharpe and Dohme, outside the submitted work. A-CT reports grants, personal fees, and non-financial support from Roche; grants, personal fees and non-financial support from AbbVie; personal fees and non-financial support from MSD; personal fees and non-financial support from BMS; personal fees and non-financial support from Boehringer Ingelheim; personal fees and non-financial support from Astra Zeneca; personal fees from Vifor Pharma; personal fees from Novartis.
The remaining author declares that the research was conducted in the absence of any commercial or financial relationships that could be construed as a potential conflict of interest.
Acknowledgments
The authors thank Dr. Gabrielle Cremer for providing language help and writing assistance.
References
1. Siegel R, DeSantis C, Virgo K, Stein K, Mariotto A, Smith T, et al. Cancer treatment and survivorship statistics, 2012. CA Cancer J Clin. (2012) 62:220–41. doi: 10.3322/caac.21149
2. Lynch TJ, Bell DW, Sordella R, Gurubhagavatula S, Okimoto RA, Brannigan BW, et al. Activating mutations in the epidermal growth factor receptor underlying responsiveness of non-small-cell lung cancer to gefitinib. N Engl J Med. (2004) 350:2129–39. doi: 10.1056/NEJMoa040938
3. Soda M, Choi YL, Enomoto M, Takada S, Yamashita Y, Ishikawa S, et al. Identification of the transforming EML4-ALK fusion gene in non-small-cell lung cancer. Nature. (2007) 448:561–6. doi: 10.1038/nature05945
4. Rikova K, Guo A, Zeng Q, Possemato A, Yu J, Haack H, et al. Global survey of phosphotyrosine signaling identifies oncogenic kinases in lung cancer. Cell. (2007) 131:1190–203. doi: 10.1016/j.cell.2007.11.025
5. Lipson D, Capelletti M, Yelensky R, Otto G, Parker A, Jarosz M, et al. Identification of new ALK and RET gene fusions from colorectal and lung cancer biopsies. Nat Med. (2012) 18:382–4. doi: 10.1038/nm.2673
6. Kohno T, Ichikawa H, Totoki Y, Yasuda K, Hiramoto M, Nammo T, et al. KIF5B-RET fusions in lung adenocarcinoma. Nat Med. (2012) 18:375–7. doi: 10.1038/nm.2644
7. Vaishnavi A, Capelletti M, Le AT, Kako S, Butaney M, Ercan D, et al. Oncogenic and drug-sensitive NTRK1 rearrangements in lung cancer. Nat Med. (2013) 19:1469–72. doi: 10.1038/nm.3352
8. Davies H, Bignell GR, Cox C, Stephens P, Edkins S, Clegg S, et al. Mutations of the BRAF gene in human cancer. Nature. (2002) 417:949–54. doi: 10.1038/nature00766
9. Park S, Choi YL, Sung CO, An J, Seo J, Ahn MJ, et al. High MET copy number and MET overexpression: poor outcome in non-small cell lung cancer patients. Histol Histopathol. (2012) 27:197–207. doi: 10.14670/HH-27.197
10. Barlesi F, Mazieres J, Merlio JP, Debieuvre D, Mosser J, Lena H, et al. Routine molecular profiling of patients with advanced non-small-cell lung cancer: results of a 1-year nationwide programme of the French Cooperative Thoracic Intergroup (IFCT). Lancet. (2016) 387:1415–26. doi: 10.1016/S0140-6736(16)00004-0
11. Takeuchi K, Soda M, Togashi Y, Suzuki R, Sakata S, Hatano S, et al. RET, ROS1 and ALK fusions in lung cancer. Nat Med. (2012) 18:378–81. doi: 10.1038/nm.2658
12. Cancer Genome Atlas Research N. Comprehensive molecular profiling of lung adenocarcinoma. Nature. (2014) 511:543–50. doi: 10.1038/nature13385
13. Tissot C, Couraud S, Tanguy R, Bringuier PP, Girard N, Souquet PJ. Clinical characteristics and outcome of patients with lung cancer harboring BRAF mutations. Lung Cancer. (2016) 91:23–8. doi: 10.1016/j.lungcan.2015.11.006
14. Sharma SV, Bell DW, Settleman J, Haber DA. Epidermal growth factor receptor mutations in lung cancer. Nat Rev Cancer. (2007) 7:169–81. doi: 10.1038/nrc2088
15. Leduc C, Merlio JP, Besse B, Blons H, Debieuvre D, Bringuier PP, et al. Clinical and molecular characteristics of non-small-cell lung cancer (NSCLC) harboring EGFR mutation: results of the nationwide French Cooperative Thoracic Intergroup (IFCT) program. Ann Oncol. (2017) 28:2715–24. doi: 10.1093/annonc/mdx404
16. Lee CK, Wu YL, Ding PN, Lord SJ, Inoue A, Zhou C, et al. Impact of specific epidermal growth factor receptor (EGFR) mutations and clinical characteristics on outcomes after treatment with EGFR tyrosine kinase inhibitors versus chemotherapy in EGFR-mutant lung cancer: a meta-analysis. J Clin Oncol. (2015) 33:1958–65. doi: 10.1200/JCO.2014.58.1736
17. Beau-Faller M, Prim N, Ruppert AM, Nanni-Metellus I, Lacave R, Lacroix L, et al. Rare EGFR exon 18 and exon 20 mutations in non-small-cell lung cancer on 10 117 patients: a multicentre observational study by the French ERMETIC-IFCT network. Ann Oncol. (2014) 25:126–31. doi: 10.1093/annonc/mdt418
18. Krawczyk P, Reszka K, Ramlau R, Powrozek T, Pankowski J, Wojas-Krawczyk K, et al. Prevalence of rare EGFR gene mutations in nonsmall-cell lung cancer: a multicenter study on 3856 Polish Caucasian patients. Ann Oncol. (2016) 27:358–9. doi: 10.1093/annonc/mdv553
19. Reshetnyak AV, Murray PB, Shi X, Mo ES, Mohanty J, Tome F, et al. Augmentor alpha and beta (FAM150) are ligands of the receptor tyrosine kinases ALK and LTK: hierarchy and specificity of ligand-receptor interactions. Proc Natl Acad Sci USA. (2015) 112:15862–7. doi: 10.1073/pnas.1520099112
20. Guan J, Umapathy G, Yamazaki Y, Wolfstetter G, Mendoza P, Pfeifer K, et al. FAM150A and FAM150B are activating ligands for anaplastic lymphoma kinase. Elife. (2015) 4:e09811. doi: 10.7554/eLife.09811
21. Bilsland JG, Wheeldon A, Mead A, Znamenskiy P, Almond S, Waters KA, et al. Behavioral and neurochemical alterations in mice deficient in anaplastic lymphoma kinase suggest therapeutic potential for psychiatric indications. Neuropsychopharmacology. (2008) 33:685–700. doi: 10.1038/sj.npp.1301446
22. Witek B, El Wakil A, Nord C, Ahlgren U, Eriksson M, Vernersson-Lindahl E, et al. Targeted disruption of alk reveals a potential role in hypogonadotropic hypogonadism. PLoS ONE. (2015) 10:e0123542. doi: 10.1371/journal.pone.0123542
23. Hallberg B, Palmer RH. The role of the ALK receptor in cancer biology. Ann Oncol. (2016) 27(Suppl. 3):iii4–15. doi: 10.1093/annonc/mdw301
24. Du X, Shao Y, Qin HF, Tai YH, Gao HJ. ALK-rearrangement in non-small-cell lung cancer (NSCLC). Thorac Cancer. (2018) 9:423–30. doi: 10.1111/1759-7714.12613
25. Lin JJ, Riely GJ, Shaw AT. Targeting ALK: precision medicine takes on drug resistance. Cancer Discov. (2017) 7:137–55. doi: 10.1158/2159-8290.CD-16-1123
26. Armstrong F, Duplantier MM, Trempat P, Hieblot C, Lamant L, Espinos E, et al. Differential effects of X-ALK fusion proteins on proliferation, transformation, and invasion properties of NIH3T3 cells. Oncogene. (2004) 23:6071–82. doi: 10.1038/sj.onc.1207813
27. Eichenmuller B, Everley P, Palange J, Lepley D, Suprenant KA. The human EMAP-like protein-70 (ELP70) is a microtubule destabilizer that localizes to the mitotic apparatus. J Biol Chem. (2002) 277:1301–9. doi: 10.1074/jbc.M106628200
28. Richards MW, Law EW, Rennalls LP, Busacca S, O'Regan L, Fry AM, et al. Crystal structure of EML1 reveals the basis for Hsp90 dependence of oncogenic EML4-ALK by disruption of an atypical beta-propeller domain. Proc Natl Acad Sci USA. (2014) 111:5195–200. doi: 10.1073/pnas.1322892111
29. Duruisseaux M, Mc Leer-Florin A, Moro-Sibilot D, Cadranel J. Are ALK rearrangement variants promising predictive biomarker of ALK tyrosine kinase inhibitors efficacy? Ann Oncol. (2017) 28:1401. doi: 10.1093/annonc/mdx116
30. Kwak EL, Bang YJ, Camidge DR, Shaw AT, Solomon B, Maki RG, et al. Anaplastic lymphoma kinase inhibition in non-small-cell lung cancer. N Engl J Med. (2010) 363:1693–703. doi: 10.1056/NEJMoa1006448
31. Inamura K, Takeuchi K, Togashi Y, Hatano S, Ninomiya H, Motoi N, et al. EML4-ALK lung cancers are characterized by rare other mutations, a TTF-1 cell lineage, an acinar histology, and young onset. Mod Pathol. (2009) 22:508–15. doi: 10.1038/modpathol.2009.2
32. Diaz LA Jr, Bardelli A. Liquid biopsies: genotyping circulating tumor DNA. J Clin Oncol. (2014) 32:579–86. doi: 10.1200/JCO.2012.45.2011
33. Beau-Faller M, Blons H, Domerg C, Gajda D, Richard N, Escande F, et al. A multicenter blinded study evaluating EGFR and KRAS mutation testing methods in the clinical non-small cell lung cancer setting–IFCT/ERMETIC2 Project Part 1: comparison of testing methods in 20 French molecular genetic National Cancer Institute platforms. J Mol Diagn. (2014) 16:45–55. doi: 10.1016/j.jmoldx.2013.07.009
34. Metzker ML. Sequencing technologies - the next generation. Nat Rev Genet. (2010) 11:31–46. doi: 10.1038/nrg2626
35. Fisher KE, Zhang L, Wang J, Smith GH, Newman S, Schneider TM, et al. Clinical validation and implementation of a targeted next-generation sequencing assay to detect somatic variants in non-small cell lung, melanoma, and gastrointestinal malignancies. J Mol Diagn. (2016) 18:299–315. doi: 10.1016/j.jmoldx.2015.11.006
36. Sabatini LM, Mathews C, Ptak D, Doshi S, Tynan K, Hegde MR, et al. Genomic sequencing procedure microcosting analysis and health economic cost-impact analysis: a report of the association for molecular pathology. J Mol Diagn. (2016) 18:319–28. doi: 10.1016/j.jmoldx.2015.11.010
37. Simarro J, Murria R, Perez-Simo G, Llop M, Mancheno N, Ramos D, et al. Development, implementation and assessment of molecular diagnostics by next generation sequencing in personalized treatment of cancer: experience of a public reference healthcare hospital. Cancers. (2019) 11:E1196. doi: 10.3390/cancers11081196
38. Thress KS, Brant R, Carr TH, Dearden S, Jenkins S, Brown H, et al. EGFR mutation detection in ctDNA from NSCLC patient plasma: a cross-platform comparison of leading technologies to support the clinical development of AZD9291. Lung Cancer. (2015) 90:509–15. doi: 10.1016/j.lungcan.2015.10.004
39. Li M, Diehl F, Dressman D, Vogelstein B, Kinzler KW. BEAMing up for detection and quantification of rare sequence variants. Nat Methods. (2006) 3:95–7. doi: 10.1038/nmeth850
40. Newman AM, Lovejoy AF, Klass DM, Kurtz DM, Chabon JJ, Scherer F, et al. Integrated digital error suppression for improved detection of circulating tumor DNA. Nat Biotechnol. (2016) 34:547–55. doi: 10.1038/nbt.3520
41. Jovelet C, Ileana E, Le Deley MC, Motte N, Rosellini S, Romero A, et al. Circulating cell-free tumor DNA analysis of 50 genes by next-generation sequencing in the prospective MOSCATO trial. Clin Cancer Res. (2016) 22:2960–8. doi: 10.1158/1078-0432.CCR-15-2470
42. Pages A, Foulon S, Zou Z, Lacroix L, Lemare F, de Baere T, et al. The cost of molecular-guided therapy in oncology: a prospective cost study alongside the MOSCATO trial. Genet Med. (2017) 19:683–90. doi: 10.1038/gim.2016.174
43. Bergethon K, Shaw AT, Ou SH, Katayama R, Lovly CM, McDonald NT, et al. ROS1 rearrangements define a unique molecular class of lung cancers. J Clin Oncol. (2012) 30:863–70. doi: 10.1200/JCO.2011.35.6345
44. Farago AF, Azzoli CG. Beyond ALK and ROS1: RET, NTRK, EGFR and BRAF gene rearrangements in non-small cell lung cancer. Transl Lung Cancer Res. (2017) 6:550–9. doi: 10.21037/tlcr.2017.08.02
45. Fernandez-Cuesta L, Plenker D, Osada H, Sun R, Menon R, Leenders F, et al. CD74-NRG1 fusions in lung adenocarcinoma. Cancer Discov. (2014) 4:415–22. doi: 10.1158/2159-8290.CD-13-0633
46. Rosenbaum JN, Bloom R, Forys JT, Hiken J, Armstrong JR, Branson J, et al. Genomic heterogeneity of ALK fusion breakpoints in non-small-cell lung cancer. Mod Pathol. (2018) 31:791–808. doi: 10.1038/modpathol.2017.181
47. Davies KD, Le AT, Sheren J, Nijmeh H, Gowan K, Jones KL, et al. Comparison of molecular testing modalities for detection of ROS1 rearrangements in a cohort of positive patient samples. J Thorac Oncol. (2018) 13:1474–82. doi: 10.1016/j.jtho.2018.05.041
48. Gautschi O, Milia J, Filleron T, Wolf J, Carbone DP, Owen D, et al. Targeting RET in patients with RET-rearranged lung cancers: results from the global, multicenter RET registry. J Clin Oncol. (2017) 35:1403–10. doi: 10.1200/JCO.2016.70.9352
49. Gatalica Z, Xiu J, Swensen J, Vranic S. Molecular characterization of cancers with NTRK gene fusions. Mod Pathol. (2019) 32:147–53. doi: 10.1038/s41379-018-0118-3
50. Hechtman JF, Benayed R, Hyman DM, Drilon A, Zehir A, Frosina D, et al. Pan-Trk immunohistochemistry is an efficient and reliable screen for the detection of NTRK fusions. Am J Surg Pathol. (2017) 41:1547–51. doi: 10.1097/PAS.0000000000000911
51. Kozuma Y, Toyokawa G, Seto T. ALK testing methods: is there a winner or loser? Expert Rev Anticancer Ther. (2019) 19:237–44. doi: 10.1080/14737140.2019.1562343
52. Soria JC, Ho SN, Varella-Garcia M, Iafrate AJ, Solomon BJ, Shaw AT, et al. Correlation of extent of ALK FISH positivity and crizotinib efficacy in three prospective studies of ALK-positive patients with non-small-cell lung cancer. Ann Oncol. (2018) 29:1964–71. doi: 10.1093/annonc/mdy242
53. Mescam-Mancini L, Lantuejoul S, Moro-Sibilot D, Rouquette I, Souquet PJ, Audigier-Valette C, et al. On the relevance of a testing algorithm for the detection of ROS1-rearranged lung adenocarcinomas. Lung Cancer. (2014) 83:168–73. doi: 10.1016/j.lungcan.2013.11.019
54. FunkhouserWK Jr, Lubin IM, Monzon FA, Zehnbauer BA, Evans JP, Ogino S, et al. Relevance, pathogenesis, and testing algorithm for mismatch repair-defective colorectal carcinomas: a report of the association for molecular pathology. J Mol Diagn. (2012) 14:91–103. doi: 10.1016/j.jmoldx.2011.11.001
55. Ilie MI, Bence C, Hofman V, Long-Mira E, Butori C, Bouhlel L, et al. Discrepancies between FISH and immunohistochemistry for assessment of the ALK status are associated with ALK ‘borderline'-positive rearrangements or a high copy number: a potential major issue for anti-ALK therapeutic strategies. Ann Oncol. (2015) 26:238–44. doi: 10.1093/annonc/mdu484
56. Letovanec I, Finn S, Zygoura P, Smyth P, Soltermann A, Bubendorf L, et al. Evaluation of NGS and RT-PCR methods for ALK rearrangement in European NSCLC patients: results from the European thoracic oncology platform lungscape project. J Thorac Oncol. (2018) 13:413–25. doi: 10.1016/j.jtho.2017.11.117
57. Lantuejoul S, Rouquette I, Blons H, Le Stang N, Ilie M, Begueret H, et al. French multicentric validation of ALK rearrangement diagnostic in 547 lung adenocarcinomas. Eur Respir J. (2015) 46:207–18. doi: 10.1183/09031936.00119914
58. Ma H, Yao WX, Huang L, Jin SH, Liu DH, Liu Y, et al. Efficacy of D5F3 IHC for detecting ALK gene rearrangement in NSCLC patients: a systematic review and meta-analysis. Oncotarget. (2016) 7:70128–42. doi: 10.18632/oncotarget.11806
59. Conde E, Hernandez S, Prieto M, Martinez R, Lopez-Rios F. Profile of Ventana ALK (D5F3) companion diagnostic assay for non-small-cell lung carcinomas. Expert Rev Mol Diagn. (2016) 16:707–13. doi: 10.1586/14737159.2016.1172963
60. Savic S, Diebold J, Zimmermann AK, Jochum W, Baschiera B, Grieshaber S, et al. Screening for ALK in non-small cell lung carcinomas: 5A4 and D5F3 antibodies perform equally well, but combined use with FISH is recommended. Lung Cancer. (2015) 89:104–9. doi: 10.1016/j.lungcan.2015.05.012
61. Wynes MW, Sholl LM, Dietel M, Schuuring E, Tsao MS, Yatabe Y, et al. An international interpretation study using the ALK IHC antibody D5F3 and a sensitive detection kit demonstrates high concordance between ALK IHC and ALK FISH and between evaluators. J Thorac Oncol. (2014) 9:631–8. doi: 10.1097/JTO.0000000000000115
62. von Laffert M, Warth A, Penzel R, Schirmacher P, Kerr KM, Elmberger G, et al. Multicenter immunohistochemical ALK-testing of non-small-cell lung cancer shows high concordance after harmonization of techniques and interpretation criteria. J Thorac Oncol. (2014) 9:1685–92. doi: 10.1097/JTO.0000000000000332
63. Uruga H, Mino-Kenudson M. ALK (D5F3) CDx: an immunohistochemistry assay to identify ALK-positive NSCLC patients. Pharmgenomics Pers Med. (2018) 11:147–55. doi: 10.2147/PGPM.S156672
64. Kummar S, Lassen UN. TRK inhibition: a new tumor-agnostic treatment strategy. Target Oncol. (2018) 13:545–56. doi: 10.1007/s11523-018-0590-1
65. McLeer-Florin A, Duruisseaux M, Pinsolle J, Dubourd S, Mondet J, Phillips Houlbracq M, et al. ALK fusion variants detection by targeted RNA-next generation sequencing and clinical responses to crizotinib in ALK-positive non-small cell lung cancer. Lung Cancer. (2018) 116:15–24. doi: 10.1016/j.lungcan.2017.12.004
66. Vollbrecht C, Lenze D, Hummel M, Lehmann A, Moebs M, Frost N, et al. RNA-based analysis of ALK fusions in non-small cell lung cancer cases showing IHC/FISH discordance. BMC Cancer. (2018) 18:1158. doi: 10.1186/s12885-018-5070-6
67. Drilon A, Hu ZI, Lai GGY, Tan DSW. Targeting RET-driven cancers: lessons from evolving preclinical and clinical landscapes. Nat Rev Clin Oncol. (2018) 15:150. doi: 10.1038/nrclinonc.2017.175
68. Wu J, Lin Y, He X, Yang H, He P, Fu X, et al. Comparison of detection methods and follow-up study on the tyrosine kinase inhibitors therapy in non-small cell lung cancer patients with ROS1 fusion rearrangement. BMC Cancer. (2016) 16:599. doi: 10.1186/s12885-016-2582-9
69. Yoshida A, Tsuta K, Wakai S, Arai Y, Asamura H, Shibata T, et al. Immunohistochemical detection of ROS1 is useful for identifying ROS1 rearrangements in lung cancers. Mod Pathol. (2014) 27:711–20. doi: 10.1038/modpathol.2013.192
70. Schram AM, Chang MT, Jonsson P, Drilon A. Fusions in solid tumours: diagnostic strategies, targeted therapy, and acquired resistance. Nat Rev Clin Oncol. (2017) 14:735–48. doi: 10.1038/nrclinonc.2017.127
71. Yoshida T, Oya Y, Tanaka K, Shimizu J, Horio Y, Kuroda H, et al. Differential crizotinib response duration among ALK fusion variants in ALK-positive non-small-cell lung cancer. J Clin Oncol. (2016) 34:3383–9. doi: 10.1200/JCO.2015.65.8732
72. Heuckmann JM, Balke-Want H, Malchers F, Peifer M, Sos ML, Koker M, et al. Differential protein stability and ALK inhibitor sensitivity of EML4-ALK fusion variants. Clin Cancer Res. (2012) 18:4682–90. doi: 10.1158/1078-0432.CCR-11-3260
73. Woo CG, Seo S, Kim SW, Jang SJ, Park KS, Song JY, et al. Differential protein stability and clinical responses of EML4-ALK fusion variants to various ALK inhibitors in advanced ALK-rearranged non-small cell lung cancer. Ann Oncol. (2017) 28:791–7. doi: 10.1093/annonc/mdw693
74. Sabir SR, Yeoh S, Jackson G, Bayliss R. EML4-ALK variants: biological and molecular properties, and the implications for patients. Cancers. (2017) 9:E118. doi: 10.3390/cancers9090118
75. Maemondo M, Inoue A, Kobayashi K, Sugawara S, Oizumi S, Isobe H, et al. Gefitinib or chemotherapy for non-small-cell lung cancer with mutated EGFR. N Engl J Med. (2010) 362:2380–8. doi: 10.1056/NEJMoa0909530
76. Mitsudomi T, Morita S, Yatabe Y, Negoro S, Okamoto I, Tsurutani J, et al. Gefitinib versus cisplatin plus docetaxel in patients with non-small-cell lung cancer harbouring mutations of the epidermal growth factor receptor (WJTOG3405): an open label, randomised phase 3 trial. Lancet Oncol. (2010) 11:121–8. doi: 10.1016/S1470-2045(09)70364-X
77. Zhou C, Wu YL, Chen G, Feng J, Liu XQ, Wang C, et al. Erlotinib versus chemotherapy as first-line treatment for patients with advanced EGFR mutation-positive non-small-cell lung cancer (OPTIMAL, CTONG-0802): a multicentre, open-label, randomised, phase 3 study. Lancet Oncol. (2011) 12:735–42. doi: 10.1016/S1470-2045(11)70184-X
78. Rosell R, Carcereny E, Gervais R, Vergnenegre A, Massuti B, Felip E, et al. Erlotinib versus standard chemotherapy as first-line treatment for European patients with advanced EGFR mutation-positive non-small-cell lung cancer (EURTAC): a multicentre, open-label, randomised phase 3 trial. Lancet Oncol. (2012) 13:239–46. doi: 10.1016/S1470-2045(11)70393-X
79. Wu YL, Zhou C, Liam CK, Wu G, Liu X, Zhong Z, et al. First-line erlotinib versus gemcitabine/cisplatin in patients with advanced EGFR mutation-positive non-small-cell lung cancer: analyses from the phase III, randomized, open-label, ENSURE study. Ann Oncol. (2015) 26:1883–9. doi: 10.1093/annonc/mdv270
80. Wu YL, Zhou C, Hu CP, Feng J, Lu S, Huang Y, et al. Afatinib versus cisplatin plus gemcitabine for first-line treatment of Asian patients with advanced non-small-cell lung cancer harbouring EGFR mutations (LUX-Lung 6): an open-label, randomised phase 3 trial. Lancet Oncol. (2014) 15:213–22. doi: 10.1016/S1470-2045(13)70604-1
81. Sequist LV, Yang JC, Yamamoto N, O'Byrne K, Hirsh V, Mok T, et al. Phase III study of afatinib or cisplatin plus pemetrexed in patients with metastatic lung adenocarcinoma with EGFR mutations. J Clin Oncol. (2013) 31:3327–34. doi: 10.1200/JCO.2012.44.2806
82. Yang JC, Wu YL, Schuler M, Sebastian M, Popat S, Yamamoto N, et al. Afatinib versus cisplatin-based chemotherapy for EGFR mutation-positive lung adenocarcinoma (LUX-Lung 3 and LUX-Lung 6): analysis of overall survival data from two randomised, phase 3 trials. Lancet Oncol. (2015) 16:141–51. doi: 10.1016/S1470-2045(14)71173-8
83. Wu YL, Cheng Y, Zhou X, Lee KH, Nakagawa K, Niho S, et al. Dacomitinib versus gefitinib as first-line treatment for patients with EGFR-mutation-positive non-small-cell lung cancer (ARCHER 1050): a randomised, open-label, phase 3 trial. Lancet Oncol. (2017) 18:1454–66. doi: 10.1016/S1470-2045(17)30608-3
84. Mok TS, Cheng Y, Zhou X, Lee KH, Nakagawa K, Niho S, et al. Improvement in overall survival in a randomized study that compared dacomitinib with gefitinib in patients with advanced non-small-cell lung cancer and EGFR-activating mutations. J Clin Oncol. (2018) 36:2244–50. doi: 10.1200/JCO.2018.78.7994
85. Camidge DR, Kim HR, Ahn MJ, Yang JC, Han JY, Lee JS, et al. Brigatinib versus crizotinib in ALK-positive non-small-cell lung cancer. N Engl J Med. (2018) 379:2027–39. doi: 10.1056/NEJMoa1810171
86. Camidge DR, Dziadziuszko R, Peters S, Mok T, Noe J, Nowicka M, et al. Updated efficacy and safety data and impact of the EML4-ALK fusion variant on the efficacy of alectinib in untreated ALK-positive advanced non-small cell lung cancer in the global phase III ALEX study. J Thorac Oncol. (2019) 14:1233–43. doi: 10.1016/j.jtho.2019.03.007
87. Peters S, Camidge DR, Shaw AT, Gadgeel S, Ahn JS, Kim DW, et al. Alectinib versus crizotinib in untreated ALK-positive non-small-cell lung cancer. N Engl J Med. (2017) 377:829–38. doi: 10.1056/NEJMoa1704795
88. Soria JC, Tan DSW, Chiari R, Wu YL, Paz-Ares L, Wolf J, et al. First-line ceritinib versus platinum-based chemotherapy in advanced ALK-rearranged non-small-cell lung cancer (ASCEND-4): a randomised, open-label, phase 3 study. Lancet. (2017) 389:917–29. doi: 10.1016/S0140-6736(17)30123-X
89. Vecchiarelli S, Bennati C. Oncogene addicted non-small-cell lung cancer: current standard and hot topics. Future Oncol. (2018) 14:3–17. doi: 10.2217/fon-2018-0095
90. Pao W, Miller VA, Politi KA, Riely GJ, Somwar R, Zakowski MF, et al. Acquired resistance of lung adenocarcinomas to gefitinib or erlotinib is associated with a second mutation in the EGFR kinase domain. PLoS Med. (2005) 2:e73. doi: 10.1371/journal.pmed.0020073
91. Kobayashi S, Boggon TJ, Dayaram T, Janne PA, Kocher O, Meyerson M, et al. EGFR mutation and resistance of non-small-cell lung cancer to gefitinib. N Engl J Med. (2005) 352:786–92. doi: 10.1056/NEJMoa044238
92. Takezawa K, Pirazzoli V, Arcila ME, Nebhan CA, Song X, de Stanchina E, et al. HER2 amplification: a potential mechanism of acquired resistance to EGFR inhibition in EGFR-mutant lung cancers that lack the second-site EGFRT790M mutation. Cancer Discov. (2012) 2:922–33. doi: 10.1158/2159-8290.CD-12-0108
93. Engelman JA, Zejnullahu K, Mitsudomi T, Song Y, Hyland C, Park JO, et al. MET amplification leads to gefitinib resistance in lung cancer by activating ERBB3 signaling. Science. (2007) 316:1039–43. doi: 10.1126/science.1141478
94. Asao T, Takahashi F, Takahashi K. Resistance to molecularly targeted therapy in non-small-cell lung cancer. Respir Investig. (2019) 57:20–6. doi: 10.1016/j.resinv.2018.09.001
95. Sequist LV, Waltman BA, Dias-Santagata D, Digumarthy S, Turke AB, Fidias P, et al. Genotypic and histological evolution of lung cancers acquiring resistance to EGFR inhibitors. Sci Transl Med. (2011) 3:75ra26. doi: 10.1126/scitranslmed.3002003
96. Ferrer L, Giaj Levra M, Brevet M, Antoine M, Mazieres J, Rossi G, et al. A brief report of transformation from NSCLC to SCLC: molecular and therapeutic characteristics. J Thorac Oncol. (2019) 14:130–4. doi: 10.1016/j.jtho.2018.08.2028
97. Cross DA, Ashton SE, Ghiorghiu S, Eberlein C, Nebhan CA, Spitzler PJ, et al. AZD9291, an irreversible EGFR TKI, overcomes T790M-mediated resistance to EGFR inhibitors in lung cancer. Cancer Discov. (2014) 4:1046–61. doi: 10.1158/2159-8290.CD-14-0337
98. Soria JC, Ohe Y, Vansteenkiste J, Reungwetwattana T, Chewaskulyong B, Lee KH, et al. Osimertinib in untreated EGFR-mutated advanced non-small-cell lung cancer. N Engl J Med. (2018) 378:113–25. doi: 10.1056/NEJMoa1713137
99. Le X, Puri S, Negrao MV, Nilsson MB, Robichaux J, Boyle T, et al. Landscape of EGFR-dependent and -independent resistance mechanisms to osimertinib and continuation therapy beyond progression in EGFR-mutant NSCLC. Clin Cancer Res. (2018) 24:6195–203. doi: 10.1158/1078-0432.CCR-18-1542
100. Oxnard GR, Hu Y, Mileham KF, Husain H, Costa DB, Tracy P, et al. Assessment of resistance mechanisms and clinical implications in patients with EGFR T790M-positive lung cancer and acquired resistance to osimertinib. JAMA Oncol. (2018) 4:1527–34. doi: 10.1001/jamaoncol.2018.2969
101. Piotrowska Z, Niederst MJ, Karlovich CA, Wakelee HA, Neal JW, Mino-Kenudson M, et al. Heterogeneity underlies the emergence of EGFRT790 wild-type clones following treatment of T790M-positive cancers with a third-generation EGFR inhibitor. Cancer Discov. (2015) 5:713–22. doi: 10.1158/2159-8290.CD-15-0399
102. Thress KS, Paweletz CP, Felip E, Cho BC, Stetson D, Dougherty B, et al. Acquired EGFR C797S mutation mediates resistance to AZD9291 in non-small cell lung cancer harboring EGFR T790M. Nat Med. (2015) 21:560–2. doi: 10.1038/nm.3854
103. Niederst MJ, Hu H, Mulvey HE, Lockerman EL, Garcia AR, Piotrowska Z, et al. The allelic context of the C797S mutation acquired upon treatment with third-generation EGFR inhibitors impacts sensitivity to subsequent treatment strategies. Clin Cancer Res. (2015) 21:3924–33. doi: 10.1158/1078-0432.CCR-15-0560
104. Wu JY, Yu CJ, Chang YC, Yang CH, Shih JY, Yang PC. Effectiveness of tyrosine kinase inhibitors on “uncommon” epidermal growth factor receptor mutations of unknown clinical significance in non-small cell lung cancer. Clin Cancer Res. (2011) 17:3812–21. doi: 10.1158/1078-0432.CCR-10-3408
105. Yang JC, Sequist LV, Geater SL, Tsai CM, Mok TS, Schuler M, et al. Clinical activity of afatinib in patients with advanced non-small-cell lung cancer harbouring uncommon EGFR mutations: a combined post-hoc analysis of LUX-Lung 2, LUX-Lung 3, and LUX-Lung 6. Lancet Oncol. (2015) 16:830–8. doi: 10.1016/S1470-2045(15)00026-1
106. Chiu CH, Yang CT, Shih JY, Huang MS, Su WC, Lai RS, et al. Epidermal growth factor receptor tyrosine kinase inhibitor treatment response in advanced lung adenocarcinomas with G719X/L861Q/S768I mutations. J Thorac Oncol. (2015) 10:793–9. doi: 10.1097/JTO.0000000000000504
107. Yang Z, Tchekmedyian N, Chu DT, Reddy G, Bhat G, Socinski MA. A phase 2 study of poziotinib in patients with EGFR or HER2 exon 20 mutation-positive non-small cell lung cancer. J Clin Oncol. (2018) 36:TPS9106. doi: 10.1200/JCO.2018.36.15_suppl.TPS9106
108. Duruisseaux M, Besse B, Cadranel J, Perol M, Mennecier B, Bigay-Game L, et al. Overall survival with crizotinib and next-generation ALK inhibitors in ALK-positive non-small-cell lung cancer (IFCT-1302 CLINALK): a French nationwide cohort retrospective study. Oncotarget. (2017) 8:21903–17. doi: 10.18632/oncotarget.15746
109. Pacheco JM, Gao D, Smith D, Purcell T, Hancock M, Bunn P, et al. Natural history and factors associated with overall survival in stage IV ALK-rearranged non-small cell lung cancer. J Thorac Oncol. (2019) 14:691–700. doi: 10.1016/j.jtho.2018.12.014
110. Solomon BJ, Mok T, Kim DW, Wu YL, Nakagawa K, Mekhail T, et al. First-line crizotinib versus chemotherapy in ALK-positive lung cancer. N Engl J Med. (2014) 371:2167–77. doi: 10.1056/NEJMoa1408440
111. Solomon BJ, Kim DW, Wu YL, Nakagawa K, Mekhail T, Felip E, et al. Final overall survival analysis from a study comparing first-line crizotinib versus chemotherapy in ALK-mutation-positive non-small-cell lung cancer. J Clin Oncol. (2018) 36:2251–8. doi: 10.1200/JCO.2017.77.4794
112. Solomon BJ, Besse B, Bauer TM, Felip E, Soo RA, Camidge DR, et al. Lorlatinib in patients with ALK-positive non-small-cell lung cancer: results from a global phase 2 study. Lancet Oncol. (2018) 19:1654–67. doi: 10.1016/S1470-2045(18)30649-1
113. Gainor JF, Dardaei L, Yoda S, Friboulet L, Leshchiner I, Katayama R, et al. Molecular mechanisms of resistance to first- and second-generation ALK inhibitors in ALK-rearranged lung cancer. Cancer Discov. (2016) 6:1118–33. doi: 10.1158/2159-8290.CD-16-0596
114. Katayama R, Shaw AT, Khan TM, Mino-Kenudson M, Solomon BJ, Halmos B, et al. Mechanisms of acquired crizotinib resistance in ALK-rearranged lung Cancers. Sci Transl Med. (2012) 4:120ra117. doi: 10.1126/scitranslmed.3003316
115. Ignatius Ou SH, Azada M, Hsiang DJ, Herman JM, Kain TS, Siwak-Tapp C, et al. Next-generation sequencing reveals a Novel NSCLC ALK F1174V mutation and confirms ALK G1202R mutation confers high-level resistance to alectinib (CH5424802/RO5424802) in ALK-rearranged NSCLC patients who progressed on crizotinib. J Thorac Oncol. (2014) 9:549–53. doi: 10.1097/JTO.0000000000000094
116. Sasaki T, Koivunen J, Ogino A, Yanagita M, Nikiforow S, Zheng W, et al. A novel ALK secondary mutation and EGFR signaling cause resistance to ALK kinase inhibitors. Cancer Res. (2011) 71:6051–60. doi: 10.1158/0008-5472.CAN-11-1340
117. Gouji T, Takashi S, Mitsuhiro T, Yukito I. Crizotinib can overcome acquired resistance to CH5424802: is amplification of the MET gene a key factor? J Thorac Oncol. (2014) 9:e27–8. doi: 10.1097/JTO.0000000000000113
118. Hrustanovic G, Olivas V, Pazarentzos E, Tulpule A, Asthana S, Blakely CM, et al. RAS-MAPK dependence underlies a rational polytherapy strategy in EML4-ALK-positive lung cancer. Nat Med. (2015) 21:1038–47. doi: 10.1038/nm.3930
119. Levacq D, D'Haene N, de Wind R, Remmelink M, Berghmans T. Histological transformation of ALK rearranged adenocarcinoma into small cell lung cancer: a new mechanism of resistance to ALK inhibitors. Lung Cancer. (2016) 102:38–41. doi: 10.1016/j.lungcan.2016.10.012
120. Tang SC, Nguyen LN, Sparidans RW, Wagenaar E, Beijnen JH, Schinkel AH. Increased oral availability and brain accumulation of the ALK inhibitor crizotinib by coadministration of the P-glycoprotein (ABCB1) and breast cancer resistance protein (ABCG2) inhibitor elacridar. Int J Cancer. (2014) 134:1484–94. doi: 10.1002/ijc.28475
121. Kort A, Sparidans RW, Wagenaar E, Beijnen JH, Schinkel AH. Brain accumulation of the EML4-ALK inhibitor ceritinib is restricted by P-glycoprotein (P-GP/ABCB1) and breast cancer resistance protein (BCRP/ABCG2). Pharmacol Res. (2015) 102:200–7. doi: 10.1016/j.phrs.2015.09.003
122. Drilon A, Cappuzzo F, Ou SI, Camidge DR. Targeting MET in lung cancer: will expectations finally be MET? J Thorac Oncol. (2017) 12:15–26. doi: 10.1016/j.jtho.2016.10.014
123. Wu YL, Soo RA, Locatelli G, Stammberger U, Scagliotti G, Park K. Does c-Met remain a rational target for therapy in patients with EGFR TKI-resistant non-small cell lung cancer? Cancer Treat Rev. (2017) 61:70–81. doi: 10.1016/j.ctrv.2017.10.003
124. Piotrowska Z, Thress KS, Mooradian M, Heist RS, Azzoli CG, Temel JS, et al. MET amplification (amp) as a resistance mechanism to osimertinib. J Clin Oncol. (2017) 35:9020. doi: 10.1200/JCO.2017.35.15_suppl.9020
125. Frampton GM, Ali SM, Rosenzweig M, Chmielecki J, Lu X, Bauer TM, et al. Activation of MET via diverse exon 14 splicing alterations occurs in multiple tumor types and confers clinical sensitivity to MET inhibitors. Cancer Discov. (2015) 5:850–9. doi: 10.1158/2159-8290.CD-15-0285
126. Planchard D, Popat S, Kerr K, Novello S, Smit EF, Faivre-Finn C, et al. Metastatic non-small cell lung cancer: ESMO Clinical Practice Guidelines for diagnosis, treatment and follow-up. Ann Oncol. (2019) 30:863–70. doi: 10.1093/annonc/mdy474
127. Spigel DR, Ervin TJ, Ramlau RA, Daniel DB, Goldschmidt JH Jr, Blumenschein GR Jr, et al. Randomized phase II trial of Onartuzumab in combination with erlotinib in patients with advanced non-small-cell lung cancer. J Clin Oncol. (2013) 31:4105–14. doi: 10.1200/JCO.2012.47.4189
128. Scagliotti G, von Pawel J, Novello S, Ramlau R, Favaretto A, Barlesi F, et al. Phase III multinational, randomized, double-blind, placebo-controlled study of tivantinib (ARQ 197) plus erlotinib versus erlotinib alone in previously treated patients with locally advanced or metastatic nonsquamous non-small-cell lung cancer. J Clin Oncol. (2015) 33:2667–74. doi: 10.1200/JCO.2014.60.7317
129. Sequist LV, von Pawel J, Garmey EG, Akerley WL, Brugger W, Ferrari D, et al. Randomized phase II study of erlotinib plus tivantinib versus erlotinib plus placebo in previously treated non-small-cell lung cancer. J Clin Oncol. (2011) 29:3307–15. doi: 10.1200/JCO.2010.34.0570
130. Wolf J, Seto T, Han JY, Reguart N, Garon EB, Groen HJM, et al. Capmatinib (INC280) in METΔex14-mutated advanced non-small cell lung cancer (NSCLC): efficacy data from the phase II GEOMETRY mono-1 study. J Clin Oncol. (2019) 37:9004. doi: 10.1200/JCO.2019.37.15_suppl.9004
131. Felip E, Sakai H, Patel J, Horn L, Veillon R, Griesinger F, et al. OA12.01 Phase II data for the MET inhibitor tepotinib in patients with advanced NSCLC and MET exon 14-skipping mutations. J Thoracic Oncol. (2018) 13:S347. doi: 10.1016/j.jtho.2018.08.299
132. Drilon A, Laetsch TW, Kummar S, DuBois SG, Lassen UN, Demetri GD, et al. Efficacy of larotrectinib in TRK fusion-positive cancers in adults and children. N Engl J Med. (2018) 378:731–9. doi: 10.1056/NEJMoa1714448
133. Moro-Sibilot D, Cozic N, Pérol M, Mazières J, Otto J, Souquet PJ, et al. Crizotinib in c-MET- or ROS1-positive NSCLC: results of the AcSé phase II trial. Ann Oncol. (2019). doi: 10.1093/annonc/mdz407. [Epub ahead of print].
134. Cardarella S, Ogino A, Nishino M, Butaney M, Shen J, Lydon C, et al. Clinical, pathologic, and biologic features associated with BRAF mutations in non-small cell lung cancer. Clin Cancer Res. (2013) 19:4532–40. doi: 10.1158/1078-0432.CCR-13-0657
135. Marchetti A, Felicioni L, Malatesta S, Grazia Sciarrotta M, Guetti L, Chella A, et al. Clinical features and outcome of patients with non-small-cell lung cancer harboring BRAF mutations. J Clin Oncol. (2011) 29:3574–9. doi: 10.1200/JCO.2011.35.9638
136. Ross JS, Wang K, Chmielecki J, Gay L, Johnson A, Chudnovsky J, et al. The distribution of BRAF gene fusions in solid tumors and response to targeted therapy. Int J Cancer. (2016) 138:881–90. doi: 10.1002/ijc.29825
137. Planchard D, Kim TM, Mazieres J, Quoix E, Riely G, Barlesi F, et al. Dabrafenib in patients with BRAF(V600E)-positive advanced non-small-cell lung cancer: a single-arm, multicentre, open-label, phase 2 trial. Lancet Oncol. (2016) 17:642–50. doi: 10.1016/S1470-2045(16)00077-2
138. Hyman DM, Puzanov I, Subbiah V, Faris JE, Chau I, Blay JY, et al. Vemurafenib in multiple nonmelanoma cancers with BRAF V600 mutations. N Engl J Med. (2015) 373:726–36. doi: 10.1056/NEJMoa1502309
139. Planchard D, Besse B, Groen HJM, Souquet PJ, Quoix E, Baik CS, et al. Dabrafenib plus trametinib in patients with previously treated BRAF(V600E)-mutant metastatic non-small cell lung cancer: an open-label, multicentre phase 2 trial. Lancet Oncol. (2016) 17:984–93. doi: 10.1016/S1470-2045(16)30146-2
140. Planchard D, Smit EF, Groen HJM, Mazieres J, Besse B, Helland A, et al. Dabrafenib plus trametinib in patients with previously untreated BRAF(V600E)-mutant metastatic non-small-cell lung cancer: an open-label, phase 2 trial. Lancet Oncol. (2017) 18:1307–16. doi: 10.1016/S1470-2045(17)30679-4
141. Bubendorf L, Buttner R, Al-Dayel F, Dietel M, Elmberger G, Kerr K, et al. Testing for ROS1 in non-small cell lung cancer: a review with recommendations. Virchows Arch. (2016) 469:489–503. doi: 10.1007/s00428-016-2000-3
142. Shaw AT, Ou SH, Bang YJ, Camidge DR, Solomon BJ, Salgia R, et al. Crizotinib in ROS1-rearranged non-small-cell lung cancer. N Engl J Med. (2014) 371:1963–71. doi: 10.1056/NEJMoa1406766
143. Shaw AT, Riely GJ, Bang YJ, Kim DW, Camidge DR, Solomon BJ, et al. Crizotinib in ROS1-rearranged advanced non-small-cell lung cancer (NSCLC): updated results, including overall survival, from PROFILE 1001. Ann Oncol. (2019) 30:1121–6. doi: 10.1093/annonc/mdz131
144. Landi L, Chiari R, Tiseo M, D'Inca F, Dazzi C, Chella A, et al. Crizotinib in MET deregulated or ROS1 rearranged pretreated non-small-cell lung cancer (METROS): a phase II, prospective, multicentre, two-arms trial. Clin Cancer Res. (2019) 2019:994. doi: 10.1158/1078-0432.CCR-19-0994
145. Moro-Sibilot D, Faivre L, Zalcman G, Pérol M, Barlesi F, Otto J, et al. Crizotinib in patients with advanced ROS1-rearranged non-small cell lung cancer (NSCLC). Preliminary results of the ACSé phase II trial. J Clin Oncol. (2015) 33:8065–5. doi: 10.1200/jco.2015.33.15_suppl.8065
146. Wu YL, Yang JC, Kim DW, Lu S, Zhou J, Seto T, et al. Phase II study of crizotinib in East Asian patients with ROS1-positive advanced non-small-cell lung cancer. J Clin Oncol. (2018) 36:1405–11. doi: 10.1200/JCO.2017.75.5587
147. Lim SM, Kim HR, Lee JS, Lee KH, Lee YG, Min YJ, et al. Open-label, multicenter, phase II study of ceritinib in patients with non-small-cell lung cancer harboring ROS1 rearrangement. J Clin Oncol. (2017) 35:2613–8. doi: 10.1200/JCO.2016.71.3701
148. Katayama R, Kobayashi Y, Friboulet L, Lockerman EL, Koike S, Shaw AT, et al. Cabozantinib overcomes crizotinib resistance in ROS1 fusion-positive cancer. Clin Cancer Res. (2015) 21:166–74. doi: 10.1158/1078-0432.CCR-14-1385
149. Doebele R, Ahn M, Siena S, Drilon A, Krebs M, Lin C, et al. OA02.01 Efficacy and safety of entrectinib in locally advanced or metastatic ROS1 fusion-positive non-small cell lung cancer (NSCLC). J Thoracic Oncol. (2018) 13:S321–2. doi: 10.1016/j.jtho.2018.08.239
150. Cho BC, Drilon AE, Doebele RC, Kim D-W, Lin JJ, Lee J, et al. Safety and preliminary clinical activity of repotrectinib in patients with advanced ROS1 fusion-positive non-small cell lung cancer (TRIDENT-1 study). J Clin Oncol. (2019) 37:9011. doi: 10.1200/JCO.2019.37.15_suppl.9011
151. Klein R, Jing SQ, Nanduri V, O'Rourke E, Barbacid M. The trk proto-oncogene encodes a receptor for nerve growth factor. Cell. (1991) 65:189–97. doi: 10.1016/0092-8674(91)90419-Y
152. Klein R, Nanduri V, Jing SA, Lamballe F, Tapley P, Bryant S, et al. The trkB tyrosine protein kinase is a receptor for brain-derived neurotrophic factor and neurotrophin-3. Cell. (1991) 66:395–403. doi: 10.1016/0092-8674(91)90628-C
153. Martin-Zanca D, Hughes SH, Barbacid M. A human oncogene formed by the fusion of truncated tropomyosin and protein tyrosine kinase sequences. Nature. (1986) 319:743–8. doi: 10.1038/319743a0
154. Drilon A, Siena S, Ou SI, Patel M, Ahn MJ, Lee J, et al. Safety and antitumor activity of the multitargeted pan-TRK, ROS1, and ALK inhibitor entrectinib: combined results from two phase I trials (ALKA-372-001 and STARTRK-1). Cancer Discov. (2017) 7:400–9. doi: 10.1158/2159-8290.CD-16-1237
155. Nikiforov YE. Thyroid carcinoma: molecular pathways and therapeutic targets. Mod Pathol. (2008) 21 Suppl 2:S37–43. doi: 10.1038/modpathol.2008.10
156. Kato S, Subbiah V, Marchlik E, Elkin SK, Carter JL, Kurzrock R. RET aberrations in diverse cancers: next-generation sequencing of 4,871 patients. Clin Cancer Res. (2017) 23:1988–97. doi: 10.1158/1078-0432.CCR-16-1679
157. Ju YS, Lee WC, Shin JY, Lee S, Bleazard T, Won JK, et al. A transforming KIF5B and RET gene fusion in lung adenocarcinoma revealed from whole-genome and transcriptome sequencing. Genome Res. (2012) 22:436–45. doi: 10.1101/gr.133645.111
158. Drilon A, Bergagnini I, Delasos L, Sabari J, Woo KM, Plodkowski A, et al. Clinical outcomes with pemetrexed-based systemic therapies in RET-rearranged lung cancers. Ann Oncol. (2016) 27:1286–91. doi: 10.1093/annonc/mdw163
159. Lee SH, Lee JK, Ahn MJ, Kim DW, Sun JM, Keam B, et al. Vandetanib in pretreated patients with advanced non-small cell lung cancer-harboring RET rearrangement: a phase II clinical trial. Ann Oncol. (2017) 28:292–7. doi: 10.1093/annonc/mdw559
160. Drilon AE, Subbiah V, Oxnard GR, Bauer TM, Velcheti V, Lakhani NJ, et al. A phase 1 study of LOXO-292, a potent and highly selective RET inhibitor, in patients with RET-altered cancers. J Clin Oncol. (2018) 36:102. doi: 10.1200/JCO.2018.36.15_suppl.102
161. Oxnard G, Subbiah V, Park K, Bauer T, Wirth L, Velcheti V, et al. OA12.07 clinical activity of LOXO-292, a highly selective RET inhibitor, in patients with RET fusion+ non-small cell lung cancer. J Thoracic Oncol. (2018) 13:S349–50. doi: 10.1016/j.jtho.2018.08.304
162. Rizzo S, Savoldi F, Rossi D, Bellomi M. Radiogenomics as association between non-invasive imaging features and molecular genomics of lung cancer. Ann Transl Med. (2018) 6:447. doi: 10.21037/atm.2018.11.17
Keywords: non-small-cell lung cancer, molecular alterations, next-generation sequencing, liquid biopsy, ALK rearrangement, EGFR mutation, tyrosine kinase inhibitors
Citation: Pinsolle J, McLeer-Florin A, Giaj Levra M, de Fraipont F, Emprou C, Gobbini E and Toffart A-C (2019) Translating Systems Medicine Into Clinical Practice: Examples From Pulmonary Medicine With Genetic Disorders, Infections, Inflammations, Cancer Genesis, and Treatment Implication of Molecular Alterations in Non-small-cell Lung Cancers and Personalized Medicine. Front. Med. 6:233. doi: 10.3389/fmed.2019.00233
Received: 29 July 2019; Accepted: 03 October 2019;
Published: 29 October 2019.
Edited by:
Laurent Pierre Nicod, University of Lausanne, SwitzerlandReviewed by:
Christophe Guignabert, Institut National de la Santé et de la Recherche Médicale (INSERM), FranceRudolf Maria Huber, Ludwig Maximilian University of Munich, Germany
Copyright © 2019 Pinsolle, McLeer-Florin, Giaj Levra, de Fraipont, Emprou, Gobbini and Toffart. This is an open-access article distributed under the terms of the Creative Commons Attribution License (CC BY). The use, distribution or reproduction in other forums is permitted, provided the original author(s) and the copyright owner(s) are credited and that the original publication in this journal is cited, in accordance with accepted academic practice. No use, distribution or reproduction is permitted which does not comply with these terms.
*Correspondence: Anne-Claire Toffart, YXRvZmZhcnRAY2h1LWdyZW5vYmxlLmZy