- 1Comprehensive Cancer Center Eugène Marquis Rennes, France
- 2Univ Rennes Inra, Inserm, Institut NUMECAN (Nutrition, Métabolismes et Cancer)—UMR_A 1341, UMR_S 1241, Rennes, France
- 3Angers University Hospital Angers, France
- 4Univ Angers Univ Nantes, Inserm, CNRS, CRCINA (Centre de Recherche en Cancérologie et Immunologie Nantes—Angers)—UMR 1232, ERL 6001, Nantes, France
- 5Univ Rennes CNRS, ISCR (Institut des Sciences Chimiques de Rennes)—UMR 6226, Rennes, France
- 6Univ Angers PRIMEX (Plateforme de Radiobiologie et d'Imagerie EXperimentale), Angers, France
- 7ICO (Institut de Cancérologie de l'Ouest) Comprehensive Cancer Center René Gauducheau, Saint-Herblain, France
- 8ENSCR (Ecole Nationale Supérieure de Chimie de Rennes) Rennes, France
- 9Emeritus Medical Radioisotopes Program, ORNL (Oak Ridge National Laboratory), Oak Ridge, TN, United States
Rhenium-188 (188Re) is a high energy beta-emitting radioisotope with a short 16.9 h physical half-life, which has been shown to be a very attractive candidate for use in therapeutic nuclear medicine. The high beta emission has an average energy of 784 keV and a maximum energy of 2.12 MeV, sufficient to penetrate and destroy targeted abnormal tissues. In addition, the low-abundant gamma emission of 155 keV (15%) is efficient for imaging and for dosimetric calculations. These key characteristics identify 188Re as an important therapeutic radioisotope for routine clinical use. Moreover, the highly reproducible on-demand availability of 188Re from the 188W/188Re generator system is an important feature and permits installation in hospital-based or central radiopharmacies for cost-effective availability of no-carrier-added (NCA) 188Re. Rhenium-188 and technetium-99 m exhibit similar chemical properties and represent a “theranostic pair.” Thus, preparation and targeting of 188Re agents for therapy is similar to imaging agents prepared with 99mTc, the most commonly used diagnostic radionuclide. Over the last three decades, radiopharmaceuticals based on 188Re-labeled small molecules, including peptides, antibodies, Lipiodol and particulates have been reported. The successful application of these 188Re-labeled therapeutic radiopharmaceuticals has been reported in multiple early phase clinical trials for the management of various primary tumors, bone metastasis, rheumatoid arthritis, and endocoronary interventions. This article reviews the use of 188Re-radiopharmaceuticals which have been investigated in patients for cancer treatment, demonstrating that 188Re represents a cost effective alternative for routine clinical use in comparison to more expensive and/or less readily available therapeutic radioisotopes.
Introduction
During the last decades, new radionuclide-based targeted therapies have arisen as efficient tools for cancer and inflammatory lesions treatment. They are based on the use of unsealed radioactive sources emitting β− or α particles, or Auger or low energy conversion electrons and aim at delivering tumoricidal ionizing radiation to tumor cells, while sparing healthy tissues (1–8). Several therapeutic radionuclides, essentially β− emitters, are routinely used in clinics or actively investigated in clinical trials. Some of them are summarized in Table 1. Among them, 188Re is particularly attractive, thanks to its ideal properties [t1/2 = 16.9 h, Eβmax = 2.12 MeV, Eγ = 155 keV (15%)] and its on-demand availability at high-specific activity through its generator mode of production.
Rhenium is the 3rd-row congener of transition metal elements in Group VIIB, after manganese and technetium, which, with its isotope technetium-99 m (t1/2 = 6 h, Eγ = 141 keV), has been the workhorse of nuclear medicine for more than half a century (9–11). It has a rich chemistry, with oxidation states ranging from −1 to +7 and coordination numbers up to nine. Rhenium is able to complex with a variety of ligands and bifunctional chelating agents (12–16). It possesses two potentially useful therapeutic isotopes, 186Re and 188Re (1). Both can be produced non-carrier-added (nca), but 188Re is produced with high specific activities, thanks to its generator mode of production, while 186Re is essentially reactor-produced with low specific activity, but research is currently conducted on cyclotron production of nca 186Re (17, 18). Likewise, both possess γ emissions which allow for imaging and dosimetry calculations. 186Re has a lower β− emission with a maximum tissue penetration of 4.5 mm, which is more or less half that of 188Re (11 mm), making 186Re particular suitable for treating small to mid-sized tumors while 188Re is a better match for larger-sized tumors. Considering half-lives, 188Re has a relatively short one (17 h) which restricts its use to agents with rapid target uptake and non-target tissue clearance, while 186Re can also be employed in targeting agents with longer biological half-lives, like antibodies. Based on chemical similarities and the availability of non-radioactive isotopes—which is not the case for technetium—rhenium has been used as a surrogate for technetium-99 m to elucidate structures and mechanisms (19–21). On the other hand, 99mTc-labeled radiopharmaceuticals likewise serve as a model to prepare 186/188Re-radiotracers using similar labeling methods (22, 23). However, despite close properties, there are notable differences in the reactivity of technetium and rhenium, particularly concerning their reaction kinetics and redox behaviors (24, 25). Perrhenate is much more difficult to reduce than pertechnetate, which is of prime importance, since this is the form obtained from the generators. This rich but difficult chemistry—which has been thoroughly reviewed recently and do not enter the scope of this review (26), coupled with the current limited availability of pharmaceutical-grade rhenium-188, may explain why 188Re-radiopharmaceuticals have not yet gained wide acceptance, while the use of more convenient therapeutic isotopes (simple, straightforward chemistry, and high production capacities), such as 90Y and 177Lu, is steadily increasing. This is clearly visible when making a bibliographical search on these isotopes, combined with “clinical” research term (Figure 1), despite the expected considerably higher costs. There are nonetheless research groups actively working on 188Re-labeled compounds all over the world, aiming at demonstrating the potential clinical usefulness of 188Re-radiopharmaceuticals for the treatment of various benign and malignant conditions. 188Re, under different forms, from small labeled molecules to large antibodies, or loaded into particles, from nanosized colloids to microspheres, has been investigated in various malignant diseases. Several clinical trials are currently going in progress, and some very promising new compounds are in advanced preclinical evaluation and deserve further investigation in patients.
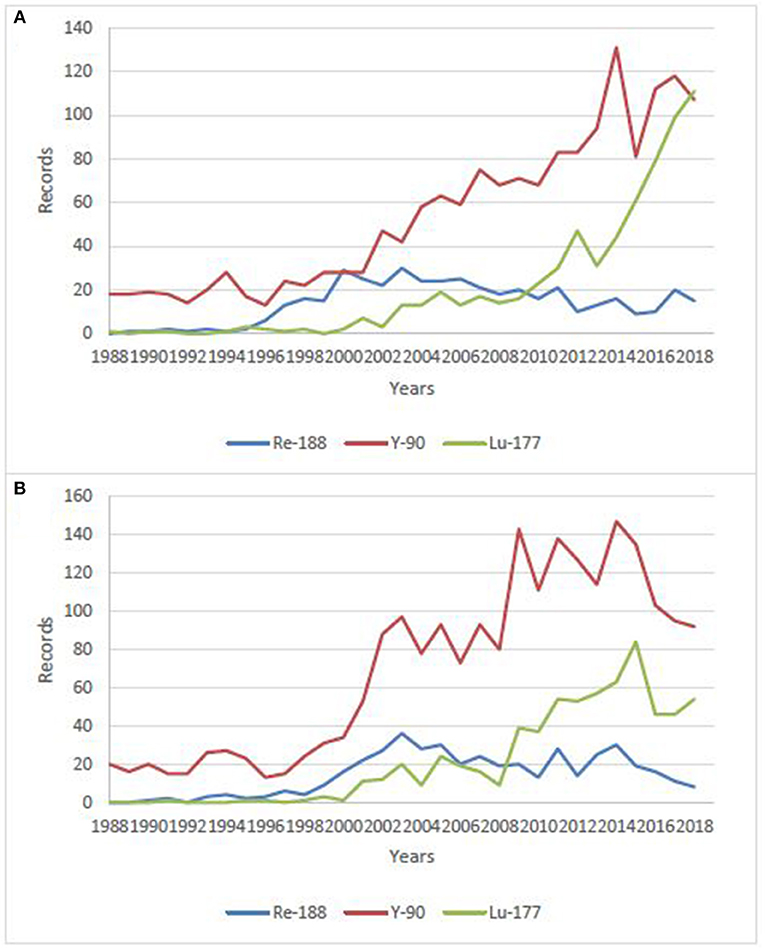
Figure 1. Number of publications/year on clinical use of 188Re, 177Lu and, 90Y over the last 30 years. (A) data from Sci-Finder, ©2019 American Chemical Society, (B) data from Web of Science, ©2019 Clarivate Analytics.
188Re Production
The attractive performance properties of the alumina-based 188W/188Re generator system have been widely described (27–32). However, factors which will affect the hopeful broader use of 188Re in routine clinical practice include the costs and required routine reactor production of sufficient activity levels of 188W. These are key issues which have challenged the broader use and routine clinical introduction of 188Re-labeled radiopharmaceuticals. One very attractive characteristic for routine clinical use of the 188W/188Re generator is the relatively rapid 188Re daughter in-growth (~60% in 24 h) following bolus elution, which means the generator can be used on a daily basis to optimize clinical use of 188Re-labeled therapeutic agents (Figure 2). The many advantages for radiotherapy with 188Re would be expected to maintain broad interest in the continued availability of the 188W/188Re generator system. Unfortunately, efficient generator utilization has generally not been the case at most institutions evaluating the early stage clinical trial-based evaluation of 188Re therapeutic agents. The limited ad hoc use of the 188W/188Re at many institutions has been often particularly inefficient, because of relatively high generator costs, discussed below. To offset these high costs, one strategy for the most cost-effective generator use, is installation of the generator at a central radiopharmacy site located in a high-density patient population area, where unit 188Re doses can be dispensed to surrounding clinics. Another strategy would be generator installation at specialized regional clinical centers where patients could be referred from the surrounding area. The cost-effective use of the 188W/188Re generator is particularly attractive for use in developing countries because of the low unit dose costs generator system is effectively used (33).
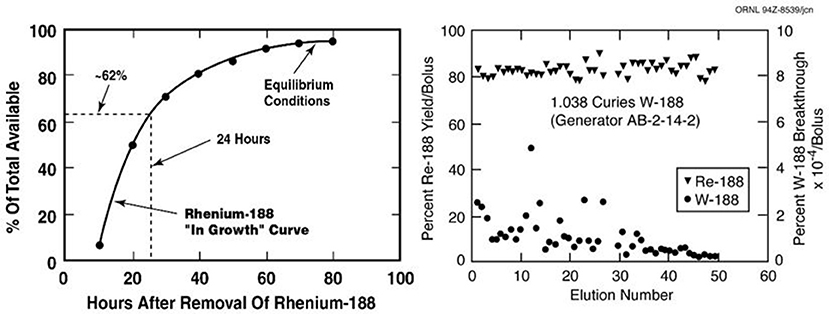
Figure 2. The useful shelf-life of the ORNL alumina-based 188W/188Re generator shows consistently high 188Re-perrhenate yields and low 188W breakthrough over at least 2 months (Image property of ORNL, courtesy of Dr. Russ Knapp, Oak Ridge, TN).
Reactor Production of 188W
The reactor production of 188W by double neutron capture of enriched 186W targets by the [186W(n,γ)187W(n,γ)188W] double neutron capture pathway has been demonstrated in some detail (34, 35). Naturally occurring stable tungsten isotopes are: 182W (26.5%), 183W (14.3%), 184W (20.64%), and 186W (28.43%). Since neutron capture will thus produce a variety of generally unwanted radioisotope products, isotopically enriched 186W (~ > 90%) is used for reactor production of 188W. Facilities in the U.S. (ORNL) and in the Russian Federation had traditionally enriched isotopes of high Z metallic elements such as 186W, and significant inventories of 186W are still available. However, the aging and expensive calutron enrichment facilities which had been operated at ORNL since the 1940's, were taken out of operation in 1998. Significant inventory levels of 186W are still available at ORNL, and the good news is that the ORNL isotope enrichment capability is now being re-established. A comprehensive detailed overview on the issues associated with reactor production of 188W was published by the International Atomic Energy Agency (IAEA) in 2010 (36). Although the availability and broad use of particle accelerators for the production of many medical radioisotopes would be expected to be considerably less expensive than reactor production, no methods have yet been described for the practical accelerator production of 188W.
The neutron cross sections (σ, probability of nuclear neutron capture) for 188W production from neutron irradiation of 186W have been well studied (37). Since the thermal neutron cross section values are a function of the square of the thermal flux for such a double neutron capture process, the 188W product yield, for instance, is essentially doubled by a two-fold increase in neutron flux. Thus, the thermal neutron flux is an important crucial issue for production of 188W. For this reason, high flux nuclear reactors with thermal neutron flux values of at least 1014 thermal neutrons/cm2 are generally felt to be required for effective 188W production (i.e., sufficient specific activity for generator use). The 188W yields at these thermal neutron flux values are about 5–10 mCi/mg 186W target, but depend on a variety of factors regarding the reactor used.
Important factors for reactor production which are beyond the scope of this discussion include the reactor neutron flux spectrum, thermal flux values, reactor cycle, target volume capabilities, shutdown between reactor cycles, etc. The saturation of 188W production and maximization of specific activity are important factors to optimize 188W production and processing costs. At the ORNL HFIR, for instance, two successive reactor cycles are optimal and practical for 188W saturation as a balance between specific activity increase and operation costs, since the down time between cycles is usually only 1 week. Another issue is the radioactive impurities which are produced as irradiation increase and which should be minimized. By many standards, these modest production activity yields and low specific activity may seem low, but in the case of the 188W/188Re generator, these factors are significantly and practically off-set by several attractive operational parameters (38). These factors include the long 188W 60-day physical half-life, the high routine daily 188Re generator elution yields of 60–80% and the very long useful 188W/188Re operational shelf-life of several months.
188W Target Material, Irradiation, and Processing
Because reactor irradiation costs are usually based on the target volume, the early use of low density encapsulated 186W targets was replaced at some institutions by use of high density pressed/sintered 186W targets (39), which greatly increases the 186W mass within the target capsule, thus significantly decreasing the costs per Ci of the 188W produced. Ergo, more target mass allows production of higher product activity levels. For this reason, the 188W has been usually produced at the following three institutions (33): High Flux Isotope Reactor in Oak Ridge, TN (1.8x1015/neutrons/cm/sec), the SM3 Reactor in Dimitrovgrad, Russian Federation (3x1015/neutrons/cm/sec), and the BR2 reactor in Mol, Belgium (1x1015/neutrons/cm/sec) (40). Traditional processing of reactor-irradiated enriched 186W metal oxide powder targets involved caustic dissolution (41, 42). Processing of the preferred pressed 186W metal targets, involves initial high temperature conversion of the irradiated metallic 188W/186W (i.e., only low percent of 186W atoms are activated) with the oxygen in atmospheric air using a quartz glass reaction apparatus (39). Subsequent dissolution of the [188W]WO2 product with caustic provides the 188W-tungstate ([188W]Na2WO4) stock solution which is then acidified to tungstic acid ([188W]HWO4) on an on-required basis for generator fabrication.
188W Target Material Recovery
Because only a small fraction of the enriched 186W target atoms are activated to 188W during the reactor irradiation, once the activity levels of eluted 188Re-perrhenate equilibrium from the generators reach activity levels which are too low and are impractical for radiopharmaceutical preparation, the non-activated 186W remaining on the generator matrix can be removed by basic elution and then reprocessed for subsequent activation (43).
188W/188Re Generator Fabrication and Use
Generator Fabrication
Similar to fabrication of the 99Mo/99mTc generator, activated alumina is currently the most widely used absorbent for fabrication of the 188W/188Re generator column (44, 45). Significant R&D has been devoted over the last three decades to the development of 188W/188Re generator prototypes, most notably in studies supported by the IAEA. A variety of other methods have been evaluated for separation of 188Re from 188W, although detailed discussion of these strategies is beyond the scope of this overview and has been reviewed elsewhere (32). As a brief summary, in addition to the use of alumina, other metal oxides, such as zirconium and titanium tungstates, nanocrystalline titania, polymeric titanium oxychloride sorbets and hydroxyapatite, have also been evaluated, and alternative methods which have been investigated for separation of 188Re from 188W include solvent extraction and electrochemistry. Evidently, these methods have not progressed further since the alumina-based 188W/188Re adsorbent has been extensively evaluated in the clinical setting with excellent performance.
For the alumina-based generator, the processed basic sodium tungstate stock solution ([188W]Na2WO4) is then converted to tungstic acid by acidification with HCl to pH 2–3 and then slowly percolated through the saline-washed alumina column which is then washed thoroughly with additional saline solution.
188W/188Re Generator Elution
The standard alumina-based generator is eluted with saline at a slow flow-rate of typically 1–2 mL/min., with the volume based on the size of the generator (i.e., “void volume”) to insure complete removal of the 188Re bolus. Some institutions have instituted the use of semi- or totally-automated elution systems (46–48). These methods have helped move use of the generator forward, and are important to insure reproducible results and reduce the user radiation burden. Microprocessor-controlled detector systems have also been often incorporated for selection of only the peak 188Re activity volume, in order to optimize the bolus 188Re volume. The potential importance for use of these methods is dependent on the particular clinical application and thus the total 188Re activity and specific activity requirements.
188Re Eluent Concentration
Because of the relatively low specific activity of reactor-produced 188W (typically 5–10 Ci/g 186W), the mass of alumina to bind the tungstic acid solution ([188W]HWO4) must be sufficient for irreversible 188W-tungstic acid binding, typically 10 grams alumina/Ci of 188W. In contrast, because of the very high specific activity of fission-produced 99Mo, only very low amounts of alumina are required for the 99Mo/99mTc generator system, resulting in very high specific volume of the saline bolus eluents (mCi/mL saline). Because of the much lower specific activity of 188W, higher volumes of saline are thus required for elution of 188Re eluents, resulting in relatively low specific volumes. With high activity (5–10 Ci) 188W/188Re generators, especially for initial use, bolus concentration is often unnecessary since the 188Re specific volume is adequate. However, use of bolus concentration is very important to extend generator shelf-life almost indefinitely and for use of generators fabricated with lower specific activity 188W.
Thus, a convenient and useful strategy for extending the 188W/188Re generator half-life involves post-elution concentration of the 188Re bolus solution. Generally, all methods which have been evaluated are based on a similar strategy, focused on the separation of the eluent anions for subsequent specific trapping of the eluted 188Re-perrhenate. The first and currently most widely used convenient method involves a simple two-column tandem flow-through system based on the specific separation of the macroscopic levels of the chloride anions (Cl−) from the saline eluting solution from the microscopic levels of the eluted perrhenate anions ([188Re]Re) (49, 50). The system, which was first described by Blower for concentrating 99mTc generator eluates (51), is based on the specific trapping of the chloride anions on a silver-nitrate-based anion trapping column through which the perrhenate anions flow through and then are subsequently retained in a second anion trapping column. The perrhenate is then obtained by low volume elution of the second column, providing very high 188Re specific volume solutions. The increase in 188Re specific volume from elution of the initial of the generator column can be at least 8–10-fold. An effective similar system uses salts of weak acids such as ammonium acetate for generator elution with subsequent trapping of [188Re]-perrhenate (52). Subsequently, a variety of potentially useful alternative methods have also been described (53–57).
Availability of GMP/Pharmaceutical-Grade Generators
Of course, for both early stage through routine clinical applications of 188Re-labeled therapeutic radiopharmaceuticals, GMP-manufactured generators are required, with subsequent GMP preparation of specific therapeutic agents. One previously widely used 188W/188Re generator had been available for several years form the Oak Ridge National Laboratory (ORNL) in the U.S., which were manufactured and distributed throughout the world as a non-sterile GMP-generator. Over about a 20-year period, several hundreds of these generators had been use in both pre-clinical and for a variety of clinical applications. The GMP generators are no longer available from ORNL. More recently, IRE in Fleurus, Belgium, has begun routine production and distribution of the “Rheni Eo” 188W/188Re generator system equipped with a GMP remote-controlled bolus concentration system. Because the reactor-production/processing/cGMP costs are not insignificant, the radiopharmacy use of the generator system and use of the eluted 188Re must be optimized to amortize the initial generator investment costs. In many cases through the last decades, the radiopharmacy/clinical use of these generators had not been optimized, thus resulting in unacceptably high unit 188Re costs.
188Re-Labeled Small Molecules
[188Re]-perrhenate, due to its structural analogy with iodide (near ionic radii, identical charge), has been tested in models of cancers expressing the sodium/iodide transporter (NIS). NIS is a plasma membrane protein that mediates active iodide transport into the thyroid gland and several extra-thyroidal tissues, and notably breast cancer, which naturally expresses NIS in more than 80% of cases. Beside, NIS can be used both as a reporter and as a therapeutic gene, making it possible to image and treat the tumor with radioiodide (131I), just as in differentiated thyroid cancer (58, 59). Using 188Re instead of 131I seems to be a potential alternative (60), and has been investigated in NIS-expressing mammary tumors (61, 62), as well as prostate (63), liver (64) and cervical cancers (65), after NIS gene transfection with adenoviruses or lentiviruses. This use of a virally-directed radioisotope therapy, called radiovirotherapy, seems particularly attractive (66), but it needs to be demonstrated in patients.
Apart from this above example, to be able to deliver its therapeutic activity to the tumor cells, rhenium-188 needs to be attached to a tumor-seeking agent, either based on specific site affinity or a particular mechanism (67).
188Re-DMSA for Medullary Carcinoma
DMSA (meso-2,3-dimercaptosuccinic acid) is a small molecule which exists in two forms labeled with technetium-99 m. Tc(III)-DMSA is a routinely used radiopharmaceutical useful for renal imaging, to evaluate renal structure and morphology, particularly in pediatric imaging for detection of scarring and pyelonephritis (68), while 99mTc(V)-DMSA is useful for imaging medullary carcinoma of thyroid, head and neck tumors and metastasis from breast carcinoma to liver, brain and skeleton (69). It was thus logical that 188Re(V)-DMSA was envisaged to be useful for the treatment of the above cancers. Three isomers (syn-endo, syn-exo and anti) are formed (Figure 3). The isomeric composition may vary depending on the conditions of preparation. The complex is synthesized from the commercial kit for 99mTc. Bolzati et al. have proposed a new approach (70) for the synthesis of 188Re(V)-DMSA, requiring less stringent conditions. The biological properties of 188Re(V)-DMSA have been studied in animals and humans (71, 72). The results in patients showed a selective attachment to tumor tissues, particularly to metastatic bone cancer originating from prostatic carcinoma, similar to that of the technetium analog (73). The limiting factor for the use of 188Re(V)-DMSA may be its high renal accumulation, higher than the 99mTc-counterpart (74), though, according to Blower et al. (73), this potential kidney irradiation should not be precluding a therapeutic or palliative use of 188Re(V)-DMSA.
Bone Pain Palliation Agents
Skeletal metastases occur in ~50% of women with breast cancer, the most common cancer in women, and in 80% of patients with prostate carcinoma, the second most common cancer in men, as well as some other tumors, such as myeloma or lung cancer (75). Medullary infiltration and matrix involvement are usually associated. Tumor infiltration is directly responsible for the pain phenomenon. Approximately half of the patients will continue to have substantial bone pain after the standard surgical and/or non-radiologic treatment options are exhausted. Metabolic radiotherapy offers a therapeutic alternative that is particularly noteworthy (76–78). All localizations are treated immediately by means of a single intravenous injection. Peptide receptor radionuclide therapy (PRRT) with somatostatin analogs (177Lu-octreotate) and PSMA ligands has also demonstrated its potential clinical usefulness for bone metastases arising from neuroendocrine tumors and metastatic castration-resistant prostate cancers (mCRPC), respectively, (79, 80). The idea of using therapeutic radioisotopes to treat the pain of bone metastases dates back to the 1940s. The first tests were due to Lawrence (81) who used phosphorus-32 as an orthophosphate. However, the major disadvantage of 32P is its high hematological toxicity related to the importance of the activity delivered to the bone marrow. For over 20 years, a wide variety of radiopharmaceuticals that can be used to deliver radiation to metastatic bone sites have been developed (82–87). Currently, four are commercially available: 89SrCl2 (Metastron®), 223RaCl2 (Xofigo®) 153Sm-EDTMP (Quadramet®), and 186Re-HEDP (186Re-etidronate®). 89Sr and 223Ra are used as such because of their natural tropism for bone, mimicking the Ca2+ cation, whereas 153Sm and 186Re are used as phosphonates (EDTMP = ethylenediaminetetramethylene phosphonate and HEDP = hydroxyethylidene diphosphonate), which are molecules having a very strong affinity toward calcium present in the actively growing bone. To date, 223RaCl2 is the only one with a proven benefit on overall survival (86, 88).
In a recent review on new radionuclides for bone pain palliation, 188Re appears to be one of the most promising candidates (89). The first example of the use of 188Re-HEDP to treat patients was reported by Maxon et al. (90). The cost and availability of 188Re make it a radioisotope more interesting than 186Re. In addition, it is expected that the maximum tolerated dose by the patient is more important for 188Re than for 186Re (91) and the shorter life of 188Re allows to fractionate the injected doses (92–94). The comparison of the biodistribution of 186Re-HEDP and 188Re-HEDP showed an identical behavior for the two molecules (95, 96). 188Re-HEDP also demonstrated similar efficacy in comparative studies with 186Re-HEDP, 153Sm-EDTMP and 89SrCl2 (97, 98). A Phase III trial has recently started to compare its efficacy to 223RaCl2, in patients with castration-resistant prostate cancer metastatic to bone (RaRe trial, NCT03458559). The maximum tolerated dose (MTD) of 188Re-HEDP was established to be 3.3 GBq in a dose escalation study by Palmedo et al. (91). Two other dosimetry-based studies demonstrated treatment was safe with an acceptable radiation-absorbed dose to the normal bone-marrow and no limiting hematological toxicity (92, 99). In a study with 15 patients suffering from breast or prostate cancer bone metastases (100), Liepe et al. reported pain relief in 80% of the patients, with 20% patients who were pain-free and could discontinue their analgesics. The same team later reported similar results in 27 prostate cancer patients (101). In a study on patients with lung cancer bone metastases (102), 46% of the patients were able to suspend their analgesics intake. As can be seen, tolerance and efficacy are highly dependent on the primary tumor site. In a study with 61 patients with skeletal metastases from lung, prostate, breast, renal, rhinopharingeal and bladder cancers, pain reliefs were achieved for, respectively, 77, 80, 83, 50, 50, and 100% of the patients (103), while in another study with 64 patients with prostate, breast, lung and liver cancer (104), pain relief was reported for 84.62, 78.57, 62.50, and 55.56%, respectively. In a very recent study by Shinto et al. (105), overall response rate was 89.5% in 48 patients with metastases from different types of cancers. Results were not detailed according to the primary tumor (Figure 4). Lange et al. specifically studied the impact on quality of life, proving the routine clinical benefit of 188Re-HEDP therapy (106). A small study by Sabet et al. on 6 patients, failed to demonstrate the usefulness of salvage therapy with 188Re-HEDP for patients with progressive bone metastases after 177Lu-octreotate therapy (107). It has been demonstrated that combination with a radiosensitizer, like capecitabine or taxane, could prove useful and lead to increased efficacy (108, 109). There is also evidence that, compared to single injection, multiple injection could lead to improved overall survival (88, 93, 94). In their retrospective analysis, Biersack et al. reported overall survivals increasing with the number of injections (from 1 to 3), from 4.50 to 15.66 months. The ongoing RaRe trial should answer this question.
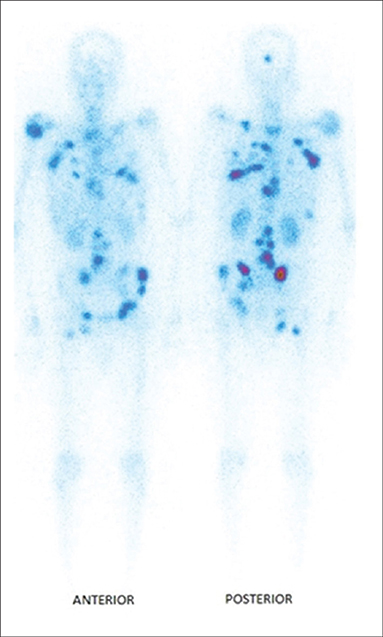
Figure 4. Typical distribution of 188Re-HEDP, 24 h post-injection [from Shinto et al. (105), available under the terms of the Creative Commons Attribution-NonCommercial-ShareAlike License (CC BY-NC-SA)].
An important point in the preparation of 188Re-HEDP is the necessity of decreasing the specific activity by adding “cold” rhenium (aka carrier) in order to have good bone fixation. Several studies have investigated the influence of the reaction conditions and kit composition on final product's stability and in vivo behavior (110–118). All of them pointed out that the addition of carrier was crucial. A GMP grade kit for the preparation of 188Re-HEDP has recently been described (119) and a standard procedure following the ICH Q8 guideline, and investigating the critical step parameters, has been reported by the same team (118).
Another bisphosphonate has recently been investigated in patients (120). In a Phase I/II trial including 63 patients, 188Re-zoledronic acid was compared with 89SrCl2, and demonstrated similar safety profile. In terms of survival, it seems treatment was more beneficial to breast cancer patients than prostate cancer ones, although the difference was not significant. Several other bisphosphonates and aminophosphonates derivatives have been the subject of development, but have not reach the clinic yet (121). As noted above, 188Re(V)-DMSA exhibited a high affinity for bone metastases from prostate cancer, but no further study was ever carried out following the one by Blower et al. (73).
188Re-Labeled Peptides and Antibodies for Hematological and Solid Tumors
Tumor cells overexpress a large range of cellular receptors not or poorly expressed by normal tissues. It is, in consequence, possible to selectively target these receptors through the use of targeting moieties with high affinity and selectivity for these receptors. For instance, antibodies targeting antigens expressed on the surface of the tumor or peptides acting as agonist or antagonist to those receptors. Radioimmunotherapy (RIT) and peptide receptor radionuclide therapy (PRRT) have demonstrated their clinical effectiveness, with some radiopharmaceuticals currently approved and a many more under clinical investigation (122–126). Best responses to RIT have been obtained with hematopoietic malignancies, in contrast to solid tumors, in spite of the delivery of somewhat low doses. This can be explained by a better vascularization, more homogenous tumor cell population and the contribution of apoptotic and immune mechanisms (127).
RIT With 188Re-Labeled Antibodies
Antibodies have long circulating times, so 188Re, with its short half-life, might not be the best suited radionuclide for antibody labeling, for which 186Re, with its 3.8-day half-life, could be more appropriate (Table 1). Nonetheless, several antibodies or antibody fragments have been labeled with 188Re, by direct or indirect methods (128), and investigated preclinically in a wide variety of tumors, like anti-CD52 and anti-CD66 in leukemia (129, 130), anti-CD20 (rituximab) in lymphoma (131), trastuzumab derivatives in breast, nasopharyngeal or prostate carcinomas (123–134), bevacizumab in non-small-cell lung cancer (135), cetuximab in lung cancer (136), anti-EGF-R antibody h-R3 (nimotuzumab) in glioma (137), anti-CEA MN-14 antibody in gastrointestinal cancers (138), C595 (anti-MUC1) in transitional cell bladder carcinoma (139), MEM238 (IGF2R-specific) in osteosarcoma (140), mAbCx-99 (anti-Ck19 antigen) and C1P5 (targeting E6 viral oncoprotein in human papillomavirus positive cervical cancers) in cervical cancers (141, 142), Listeria-binding antibodies in metastatic pancreatic cancer (143) or melanin-binding IgG or IgM in melanoma (144). Some of them have made their way to the clinics.
BW 250/183 [anti-CD66 (a, b, c, e) antibody], of murine origin and of IgG1 isotype, has a high affinity for the CD-66 antigen present on the cells of the granulocyte line. It is non-specifically directed against a surface glycoprotein, NCA-95, overexpressed on the membrane surface of human myelocytes and metamyelocytes. Radiolabeled with 188Re, it has been tested as an adjunct in marrow transplant packaging in 12 patients with acute leukemia (145) and in 36 patients with acute myeloid leukemia or myelodysplastic syndrome (146). Initial results suggest delivery of a significant radiation dose to bone marrow and minimal toxicity, demonstrating its potential clinical interest prior to bone marrow transplantation. Indeed, injection of radiolabeled antibodies maximizes immunosuppresion in the marrow while avoiding extra-medullary adverse effects (147). A phase I/II study was of particular interest in patients over 55 years of age with a high risk of acute leukemia (148, 149). Nevertheless, one of the main complications is the appearance of transplantation-related toxicity (150) and particularly nephropathies (151). To minimize its adverse effects, the use of ACE inhibitors, angiotensin receptor blockers or forced diuresis is recommended (152). A Phase II study demonstrated that combination of 188Re-radioimmunotherapy with reduced-intensity conditioning was feasible and effective (149), but that dose-reduction of alemtuzumab did not impact overall and disease-free survival (152). 188Re-RIT has also been investigated in patients with non-Hodgkin's lymphoma, using 188Re-rituximab (131). Preliminary dosimetric results indicate it could compare favorably with 131I-rituximab.
A study by Juweid et al. investigated the use of 188Re-labeled antibodies in solid tumors such as gastrointestinal or pancreatic cancer (138). They used an antibody of murine origin, MN 14, directed against the specific CEA epitope. Their results showed that the stability of the selected antibody was not the most suitable especially in patients with weak CEA expression and low tumor burden. The presence of a tumor that is too large and poorly vascularized decreases the therapeutic efficacy given the slow biodistribution of the antibodies. The authors proposed to develop more stable compounds in vivo using multi-step delivery system, to use bivalent antibodies or antibody fragments. However, the use of antibody fragments could increase the dose delivered to the kidneys. It would then be advisable to use cationic amino acid infusions to prevent these adverse effects. Another way to maximize the dose to the tumor while sparing healthy tissue is to administer radiolabeled antibodies locoregionally, or directly into the tumor cavity (153). This is the case of nimotuzumab radiolabeled with rhenium-188 in the management of high-grade gliomas in adults (154, 155). Indeed, some patients are not eligible for complete surgical resection or irradiation of lesions by conventional radiotherapy. Therefore, an uncontrolled, open-label, clinical phase I study was conducted to evaluate the safety and maximum tolerated dose of single intracavitary administration of radiolabeled nimotuzumab with 188Re, in 3 patients with anaplastic astrocytoma and 8 with glioblastoma multiforme. It is a humanized monoclonal antibody of IgG1 isotype that recognizes an epitope located in the extracellular domain of EGF-R receptors. Administration of a maximum activity of 10 mCi in brain tissue showed a high tumoricidal dose with acceptable irradiation of the kidneys, liver and bladder.
In consecutive Phase Ia and Phase Ib studies (156), Klein et al. demonstrated that 188Re-6D2, a radiolabeled IgM targeting melanin, was well tolerated, localized in melanoma metastases (Figure 5), and had antitumor activity, with a median overall survival of 13 months and no dose-limiting toxicities. The advantage of targeting melanin instead of ordinary antigens is that, in rapidly growing melanoma tumors, cell necrosis releases melanin into the extracellular space where it can easily be targeted (157). Moreover, melanin is insoluble, resistant to degradation, and can be expected to accumulate in targeted tissues.
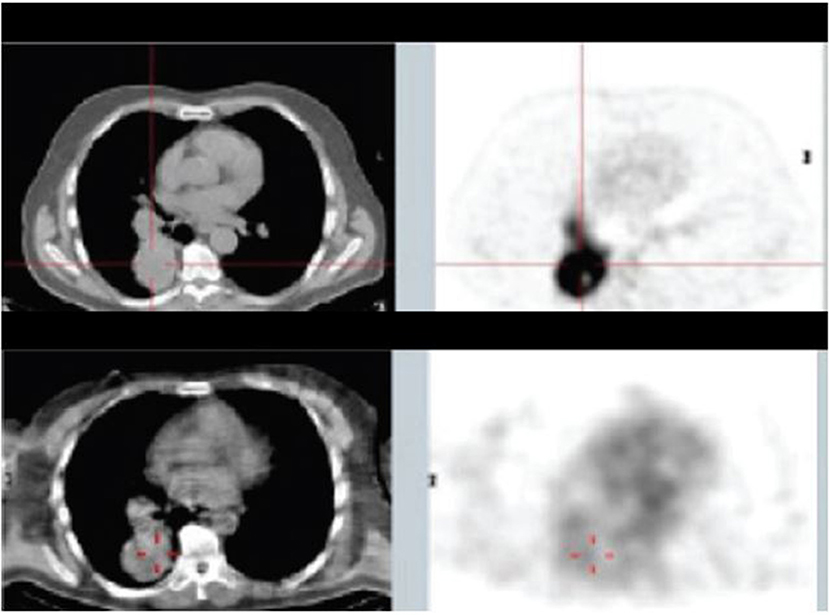
Figure 5. Patient from Phase Ia study with mediastinal and lung metastases: top panel—18FDG PET/CT 10 days before the study, lower panel—SPECT/CT of 188Re-6D2 mAb at 2 h after injection [from Klein et al. (156), available under the terms of the Creative Commons Attribution (CC BY)].
Some other really intriguing potential applications of 188Re-labeled antibodies, but falling out of the scope of this review, have been proposed by Dadachova's team. They aim at treating infectious diseases, such as microbial or fungal infection (158, 159) or HIV (160).
PRRT With 188Re
Peptides have several advantages over antibodies such as low immunogenicity, rapid penetration in the target tissue and clearance from plasma and non-target tissues. Moreover, due to the relatively short half-life of 188Re and the long circulating time of antibodies, radiolabeling peptides might be more suitable. Research on the labeling of peptides with 188Re has been very active, either on the search for the ideal chelating system (161) or on the quest for the analog having the highest affinity and stability (162, 163). A number of peptides have been radiolabeled with 188Re, mainly somatostatin derivatives (164–168). Other considered targets include gastrin releasing peptide receptor (GRPr) with bombesin (169) or GRPr-antagonist RM26 (170), αVβ3 integrin (169), NK1 receptors, with Substance P (171), HCC with SP94 peptide (172), VEGFR (173) or GRP78, a specific cancer cell-surface marker (174). Much work has also been done on targeting melanoma, either through melanin or melanocortin-1 receptor (MC1-R) (162, 175, 176).
There is, to date, however only one 188Re-labeled peptide that has been clinically investigated. It is 188Re-P2045 (Figure 6), an 11-amino acid peptide derived from 99mTc-P829 (depreotide) targeting SST receptors, which has been studied in patients with advanced pulmonary cancer (177). 5 of the 8 patients had stabilized disease for at least 8 weeks, and median overall survival was 11.5 months. Nevertheless, this study has shown a dose delivered to the kidneys that can cause irreversible damage, which prevented further escalation. This renal toxicity can occur in the long term without having early indicators of this failure. Future challenges for the development of radiolabeled antibodies and peptides will notably be to minimize these toxicities, in particular to minimize renal failure.
188Re Particulates
Radiolabeled Lipiodol and Microspheres for Liver Cancers
Primary and secondary liver tumors are a major cause of death, and their incidence is increasing. Among them, hepatocellular carcinoma (HCC), the major primary liver cancer, often appears on an underlying disease (fibrosis, cirrhosis) and is usually detected late, with a curative treatment which therefore can only be proposed to a small minority of patients. Taking advantage of the dual blood supply and rich vasculature of the liver, transarterial radioembolization (TARE) with radiolabeled Lipiodol or microspheres has demonstrated its interest for the management of HCCs at intermediate to advanced stages and intra-hepatic metastases (178–180). Notably, two 90Y-microspheres devices (SIR-Sphere® and TheraSphere®) have been successfully used for ~2 decades, and have been recently FDA-approved. Thanks to its on-site availability, and to its low-energy gamma-emission authorizing imaging, 188Re represents a potential alternative to 90Y.
Radiolabeled Lipiodol
There has been very active research on radiolabeling of Lipiodol with rhenium-188 (181). Three different 188Re-chelates are currently evaluated for the preparation of clinical 188Re-labeled Lipiodol, i.e., 188Re-HDD (182), 188ReN-DEDC (183) and 188Re-SSS (184), most clinical studies being carried out with the first one (185–198). 188Re-Lipiodol has been investigated in several early phase feasibility studies in non-operable HCC, with patients with advanced cirrhosis (189), or with extensive portal vein thrombosis (191), in second-line therapy to manage recurrences after a curative treatment (192, 193) and to stabilize patients on the liver transplant waiting list (190). To assess the maximum tolerated dose, several dose-escalation studies have been carried out (183, 186, 194, 199). The main at-risk organs are the lungs and healthy liver. In the frame of a Coordinated Research Project funded by the IAEA (200), Phase I (186) then Phase II (196) trials were undertaken in several countries. The overall results demonstrated favorable responses and potential usefulness of 188Re-Lipiodol for the therapy of HCC, which is now almost routinely used in several centers in India. One limitation of these studies is that, except the IAEA-sponsored trials, all of them included a very small number of patients, making it difficult to be conclusive. More trials, including larger cohorts of patients, are warranted. Another limitation, specifically with 188Re-HDD, is the low labeling yields and high urinary excretion (more than 40% at 72 h) (198). The next generation compounds, such as 188ReN-DEDC and 188Re-SSS, demonstrated higher yields and higher in vivo stabilities (183, 199) (Figure 7). A newly developed HDD complex (201) is expected to solve the problems encountered with the previous HDD, but no clinical data are available yet.
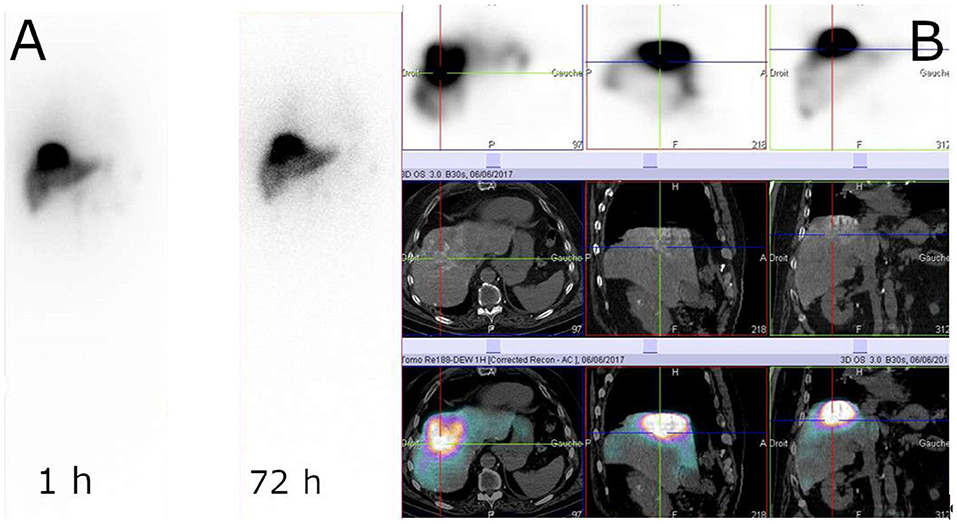
Figure 7. Example of 188Re-SSS biodistribution profile. Whole-body scintigraphy at 1 and 72 h (A) and SPECT/CT at 1 h (B) (Courtesy of Prof. Etienne Garin, Rennes, France).
Radiolabeled Microspheres
Different materials have been investigated for the preparation of 188Re-microspheres (202–205), but, to date, only human serum albumin (HSA) microspheres have made their way to the clinic. One advantage of HSA is that it is an approved drug, with 99mTc-HSA routinely used in nuclear medicine centers. Two feasibility studies, with patients suffering from HCC or metastatic tumors from various origin, have been published (206, 207). Both studies demonstrated a high product stability, with a low urinary excretion (208), and good tolerance, with acceptable toxicity. In the first study, 2 patients out of 10 demonstrated a partial response (PR) at 3 months, while, in the second one, 5 out of 13 had a PR (Figure 8). These encouraging studies included a small number of patients, with heterogeneous tumors. Larger cohorts are mandatory to be able to conclude on the usefulness of this device.
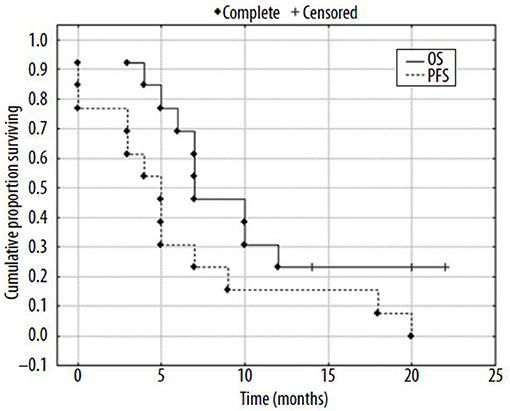
Figure 8. Kaplan-Mayer surviving curves for patients (N = 13) after radioembolization of liver tumors with 188Re-HSA microspheres [from Nowicki et al. (207), available under the terms of the Creative Commons Attribution Non Commercial-No Derivs 3.0 (CC BY-NC-ND 3.0)].
Radiocolloids and Liposomes
An alternative route to target and deliver radioactivity into close contact with tumors that are spread out over the serous membrane of cavities and to tumor cells present in the malignant effusions, is to inject the radiopharmaceutical directly into these cavities, as exemplified above with RIT. Intracavitary radionuclide therapy can be applied to the pleural, pericardial and peritoneal cavities, intrathecally and also into cystic tumors. For this purpose, radiolabeled colloids have been proven safe and effective (209), but most of the research conducted with 188Re has been preclinical. Melanoma-bearing mice have been treated with intra-peritoneal injection of 188Re-colloids, leading to an increased survival of the treated animals compared to control group (210). 188Re-microspheres embedded in a fibrin glue gel have been proposed as a potential adjuvant treatment to be applied in the tumor bed immediately after resection of glioblastomas (211). 188Re-loaded lipid nanocapsules demonstrated outstanding efficacy in rat glioblastoma models, after convection-enhanced delivery into the tumor, with a significant increase in the survival and induction of an immune response (212–214) (Figure 9). A Phase I/II study is expected to start soon. A radiobiological study by Hrycushko et al. aimed at demonstrating the potential usefulness of 188Re-loaded liposomes to prevent recurrence after surgical resection of breast tumors. Based on biodistribution results in rats, dose distributions were modeled and radiobiological indexes determined, following direct injection of 188Re-liposomes into the lumpectomy cavity (215, 216). The same group also carried out a similar work with head and neck squamous cell carcinoma, following direct intratumoral infusion of 99mTc-labeled liposomes (217, 218). These theoretical results would need to be confirmed in vivo. There is currently a clinical trial running in Taiwan, on 188Re-liposomes in patients with primary solid tumor in advanced or metastatic stage (NCT02271516). To date, only preliminary results have been published (219). One patient with advanced serous ovarian adenocarcinoma and one patient with endometrioid ovarian adenocarcinoma were treated twice with intraperitoneal injection of 188Re-BMEDA-liposome, leading to a decrease of cancer antigen 125 in serum, used as a biomarker of treatment response, and a longer than expected survival. The completion of the trial is thus expected to confirm these results.
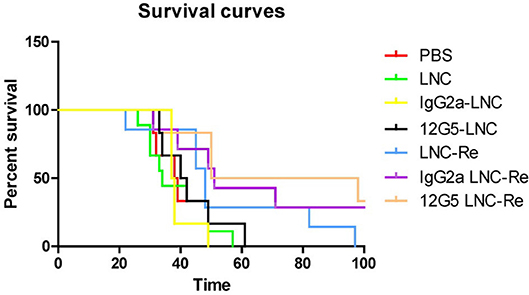
Figure 9. Kaplan Meier curves of mice treated with saline solution (PBS), blank LNC, immuno-LNCs (12G5-LNCs and IgG2a-LNCs) and internal radiation therapies (LNC188Re, IgG2a-LNC188Re, and 12G5-LNC188Re) after single infusion through convection-enhanced delivery into CXCR4-positive brain tumors [from Séhédic et al. (214), available under the terms of the Creative Commons Attribution Non Commercial 4.0 (CC BY-NC 4.0)].
Another intracavitary application of 188Re and other β-emitter-labeled radiocolloids is the radionuclide treatment of benign diseases by intra-articular injection in cases of persistent synovial effusions due to rheumatoid arthritis and other inflammatory joint disease (220).
Brachytherapy of Skin Cancers
A particularly original and attractive treatment modality using 188Re-particulates is the use of 188Re-colloids within a brachytherapy device for skin cancer treatment. Radioactive patches made of nitrocellulose filter paper loaded with 188Re-tin colloids were developed by Jeong et al. (221). This method was successfully used in patients with keloids, a benign dermal fibro proliferative tumor, and non-melanoma skin cancers (222, 223).
An alternative device embeds radiocolloids inside a mix of synthetic acrylic co-polymers inert matrix, and tensioactives, and has been investigated in patients with basal and squamous cell carcinomas (224). Fifty-three patients with histologically confirmed basal cell carcinoma (BCC) or squamous cell carcinoma (SCC) were treated. Three months later, complete healing was obtained in 100% of the treated patients; even after a single application in 82% of the cases. After a mean follow-up of 51 months, no clinical relapses were observed in the treated patients, and histological examination confirmed complete tumor regression. The inert matrix containing the 188Re is able to adapt to every skin surface without contamination, imparting an accurate distribution of dose and sparing the healthy tissue. The technology was further improved, and, in a more recent study (225), 29 BCC and 14 SCC patients were treated. One patient was lost to follow-up before wound closing, but wound healing was complete for all other 42 patients (average 65 days), with no side effects to be reported. During the period of follow-up (average 288 days), no single recurrence occurred. This 188Re-cream can be deposited through a CE-labeled applicator (Figure 10), now commercially available under tradename Rhenium-SCT® (Skin Cancer Therapy), from OncoBeta® GmbH (Garching, Germany) and this system is routinely used in Italy and South-Africa, where it is an approved therapy for the treatment of BCC and SCC, including Bowen's disease, in patients with comorbidities, when surgical intervention is not possible or conventional therapies cannot be expected to provide a satisfactory cosmetic result due to the anatomical location. This treatment modality is particularly interesting when surgery is not desirable, as in the case of SCC of the penis (226). In that study, 15 patients, ranging in age from 31 to 92 years, were treated with the Re-SCT® brachytherapy kit. After one to seven different previous treatments (for multifocal lesions), 12 patients were in complete remission, 2 did not respond, and one patient was lost to follow-up, with a mean follow-up of 51 months. Most importantly, this technique was painless and spared the anatomical integrity of the organ. In addition to BCC and SCC, this method was investigated in patients suffering from extramammary Paget's disease (EMPD) (227). Five patients with primary or secondary EMPD were successfully treated, in one or two sessions, with a mean follow-up of 34 months. All patients showed complete remission at the end of the treatments. Four patients later had relapse, either inside or at the periphery of the treated area.
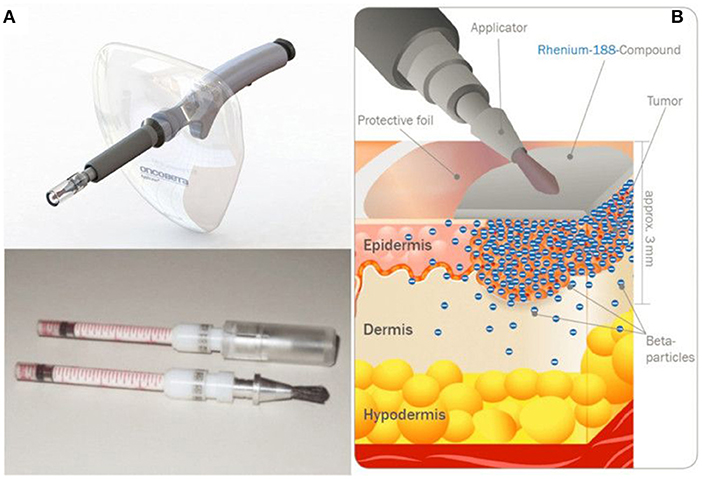
Figure 10. Rhenium-SCT device developed by OncoBeta® GmbH (Garching, Germany). Applicator and dispensing carpoules filled with 188Re-cream (A) and illustration of the principle (B) (Courtesy of Dr. Shannon Brown III, OncoBeta®).
Conclusion
Many clinical trials, from feasibility studies to Phase II studies, have been carried out with Rhenium-188-labeled radiopharmaceuticals and have demonstrated the feasibility and clinical usefulness of 188Re-labeled radiopharmaceuticals for a wide range of pathologies, especially in oncology, but also for benign diseases. Despite the advent of more “user-friendly” radionuclides such as 90Y and 177Lu, 188Re still holds great promise with compounds like 188Re-HEDP for bone pain palliation or 188Re-Lipiodol for liver cancers. Large cohorts of patients are now needed for these agents to find their place within a very competitive environment, with therapies already in use. Brachytherapy of skin cancers also appears particularly attractive, with no direct concurrent for these pathologies. Besides, the development of new 188Re radiotracers, with novel, more stable, cores like tricarbonyl, HYNIC, or nitrido, should lead to molecules with more favorable pharmacokinetic characteristics. More widespread use of 188Re-radiopharmaceuticals will now rely on availability of fully pharmaceutical grade generators and wide clinical proofs of its interest in radionuclide therapy, particularly with the possibility of having a matched theranostic pair with 99mTc.
Author Contributions
All authors listed have made a substantial, direct and intellectual contribution to the work, and approved it for publication.
Funding
This work has been supported in part by grants from the French National Agency for Research called Investissements d'Avenir Labex IRON (Grant no. ANR-11-LABX-0018).
Conflict of Interest Statement
The authors declare that the research was conducted in the absence of any commercial or financial relationships that could be construed as a potential conflict of interest.
Acknowledgments
The authors thank Dr. Shannon Brown III for providing pictures and for his critical reading of the manuscript.
References
1. Volkert WA, Hoffman TJ. Therapeutic radiopharmaceuticals. Chem Rev. (1999) 99:2269–92. doi: 10.1021/cr9804386
2. Chatal JF, Hoefnagel CA. Radionuclide therapy. Lancet. (1999) 354:931–5. doi: 10.1016/S0140-6736(99)06002-X
3. Srivastava S, Dadachova E. Recent advances in radionuclide therapy. Semin Nucl Med. (2001) 31:330–41. doi: 10.1053/snuc.2001.27043
4. Oyen WJ, Bodei L, Giammarile F, Maecke HR, Tennvall J, Luster M, et al. Targeted therapy in nuclear medicine–current status and future prospects. Ann Oncol. (2007) 18:1782–92. doi: 10.1093/annonc/mdm111
5. Elgqvist J, Frost S, Pouget JP, Albertsson P. The potential and hurdles of targeted alpha therapy - clinical trials and beyond. Front Oncol. (2014) 3:324. doi: 10.3389/fonc.2013.00324
6. Müller C, van der Meulen NP, Benešová M, Schibli R. Therapeutic radiometals beyond 177Lu and 90Y: production and application of promising α-Particle, β-Particle, and auger electron emitters. J Nucl Med. (2017) 58: 91S−6S. doi: 10.2967/jnumed.116.186825
7. Lacœuille F, Arlicot N, Faivre-Chauvet A. Targeted alpha and beta radiotherapy: an overview of radiopharmaceutical and clinical aspects. Med Nucl. (2018) 42:32–44. doi: 10.1016/j.mednuc.2017.12.002
8. Makvandi M, Dupis E, Engle JW, Nortier FM, Fassbender ME, Simon S, et al. Alpha-emitters and targeted alpha therapy in oncology: from basic science to clinical investigations. Target Oncol. (2018) 13:189–203. doi: 10.1007/s11523-018-0550-9
9. Arano Y. Recent advances in 99mTc radiopharmaceuticals. Ann Nucl Med. (2002) 16:79–93. doi: 10.1007/BF02993710
10. Zolle I. Technetium-99m Pharmaceuticals. Berlin: Springer-Verlag. (2007). 345 p. doi: 10.1007/978-3-540-33990-8
11. Bartholomä MD, Louie AS, Valliant JF, Zubieta J. Technetium and gallium derived radiopharmaceuticals: comparing and contrasting the chemistry of two important radiometals for the molecular imaging era. Chem Rev. (2010) 110:2903–20. doi: 10.1021/cr1000755
12. Vites JC, Lynam MM. Rhenium 1996. Coord Chem Rev. (1998) 172:357–88. doi: 10.1016/S0010-8545(98)00095-2
13. Alberto R, Schibli R, Waibel R, Abram U, Schubiger AP. Basic aqueous chemistry of [M(OH2)3(CO)3]+. (M = Re, Tc) directed towards radiopharmaceutical application. Coord Chem Rev. (1999) 190–2:901–19. doi: 10.1016/S0010-8545(99)00128-9
14. Bandoli G, Tisato F, Refosco F, Gerber TIA. Rhenium(V) Complexes: from pure hard donors to mixed soft/hard functionalized phosphine ligands. Rev Inorg Chem. (1999) 19:187–210. doi: 10.1515/REVIC.1999.19.3.187
15. Liu G, Hnatowich DJ. Labeling biomolecules with radiorhenium - a review of the bifunctional chelators. Anticancer Agents Med Chem. (2007) 7:367–77. doi: 10.2174/187152007780618144
16. Hahn EM, Casini A, Kühn FE. Re(VII) and Tc(VII) trioxo complexes stabilized by tridentate ligands and their potential use as radiopharmaceuticals. Coord Chem Rev. (2014) 276:97–111. doi: 10.1016/j.ccr.2014.05.021
17. Guertin A, Duchemin C, Haddad F, Michel N, Métivier V. Measurements of 186Re production cross section induced by deuterons on natW target at ARRONAX facility. Nucl Med Biol. (2014) 41:e16–8. doi: 10.1016/j.nucmedbio.2013.11.003
18. Moustapha ME, Ehrhardt GJ, Smith CJ, Szajek LP, Eckelman WC, Jurisson SS. Preparation of cyclotron-produced 186Re and comparison with reactor-produced 186Re and generator-produced 188Re for the labeling of bombesin. Nucl Med Biol. (2006) 33:81–9. doi: 10.1016/j.nucmedbio.2005.09.006
19. Dilworth JR, Parrott SJ. The biomedical chemistry of technetium and rhenium. Chem Soc Rev. (1998) 27:43–55. doi: 10.1039/a827043z
20. Mévellec F, Lepareur N, Roucoux A, Noiret N, Patin H, Bandoli G, et al. Chelated hydrazido(3-)rhenium(V) complexes: on the way to the nitrido-M(V) core (M = Tc, Re). Inorg Chem. (2002) 41:1591–7. doi: 10.1021/ic0110979
21. Wang JH, Eychenne R, Wolff M, Mallet-Ladeira S, Lepareur N, Benoist E. Design, synthesis, and reactivity of multidentate ligands with Rhenium(I) and Rhenium(V) Cores. Eur J Inorg Chem. (2017) 3908–18. doi: 10.1002/ejic.201700632
22. Deutsch E, Libson K, Vanderheyden JL, Ketring AR, Maxon HR. The chemistry of rhenium and technetium as related to the use of isotopes of these elements in therapeutic and diagnostic nuclear medicine. Nucl Med Biol. (1986) 13:465–77. doi: 10.1016/0883-2897(86)90027-9
23. De Rosales RTM, Blower P. Role of 99mTc in the development of rhenium radiopharmaceuticals. In: Technetium-99m Radiopharmaeuticals: Status and Trends. Vienna: IAEA Radioisotopes and Radiopharmaceuticals Series 1 (2010). Available online at: https://www-pub.iaea.org/MTCD/Publications/PDF/Pub1405_web.pdf
24. Helm L. Ligand exchange and complex formation kinetics studied by NMR exemplified on fac-[(CO)3M(H2O)]+. (M = Mn, Tc, Re). Coord Chem Rev. (2008) 252:2346–61. doi: 10.1016/j.ccr.2008.01.009
25. Donnelly PS. The role of coordination chemistry in the development of copper and rhenium radiopharmaceuticals. Dalton Trans. (2011) 40:999–1010. doi: 10.1039/c0dt01075h
26. Blower PJ. Rhenium-188 radiochemistry: challenges and prospects. Int J Nucl Med Res. (2017) 39–53. doi: 10.15379/2408-9788.2017.04
27. Knapp FF (Russ) Jr. Rhenium-188 - a generator-derived radioisotope for cancer therapy cancer. Cancer Biother Radiopharm. (1998) 13:337–49. doi: 10.1089/cbr.1998.13.337
28. Pillai MRA, Dash A, Knapp FF Jr. Rhenium-188: availability from the 188W/188Re generator and status of current applications. Curr Radiopharm. (2012) 5:228–3. doi: 10.2174/1874471011205030228
29. Knapp FF (Russ), Kropp J, Liepe K. (2012) Rhenium-188 generator-based radiopharmaceuticals for therapy. In: Baum R, ed. Therapeutic Nuclear Medicine. Medical Radiology. Berlin; Heidelberg: Springer. doi: 10.1007/174_2012_669
30. Argyrou M, Valassi A, Andreou M, Lyra M. Rhenium-188 production in hospitals, by w-188/re-188 generator, for easy use in radionuclide therapy. Int J Mol Imaging. (2013) 3:290750. doi: 10.1155/2013/290750
31. Dash A, Knapp FF (Russ) Jr. An overview of radioisotope separation technologies for development of 188W/188Re radionuclide generators providing 188Re to meet future research and clinical demands. RSC Adv. (2015) 5:39012–36. doi: 10.1039/C5RA03890A
32. Knapp FF (Russ). Continued availability of the tungsten-188/rhenium-188 generator to enhance therapeutic utility of 188Re Int J Nucl Med Res. (2017) 3–15. doi: 10.15379/2408-9788.2017.02
33. Shinto A, Pillai AMR. Rhenium-188 as a therapeutic radionuclide in low-income and middle-income countries. Nucl Med Commun. (2018) 39:1–2. doi: 10.1097/MNM.0000000000000755
34. Knapp FF (Russ) Jr, Mirzadeh S, Beets AL. Reactor production and processing of therapeutic radioisotopes for applications in nuclear medicine. J Radioanal Nucl Chem. (1996) 205:93–100. doi: 10.1007/BF02040554
35. Knapp FF (Russ) Jr, Mirzadeh S, Beets AL, Du M. Production of therapeutic radioisotopes in the ORNL High Flux Isotope Reactor. (HFIR) for applications in nuclear medicine, oncology and interventional cardiology J Radioanal Nucl Chem. (2005) 263:503–9. doi: 10.1007/s10967-005-0083-4
36. Knapp FF (Russ) Jr, Mirzadeh S, Garland M, Kuznetsov R. Reactor Production and Processing of 188W. In: Production of Long Lived Parent Radionuclides for Generators: 68Ge, 82Sr, 90Sr and 188W. International Atomic Energy Agency. Vienna: (IAEA) Radioisotopes and Radiopharmaceutical Series No. 2, IAEA (2010)
37. Mirzadeh S, Knapp FF (Russ) Jr, Lambrecht RM. Burn-up Cross Section of 188W. Radiochim Acta. (1997) 77:99–102. doi: 10.1524/ract.1997.77.12.99
38. Knapp FF (Russ) Jr, Turner JH, Jeong JM, Padhy AK. Issues associated with the use of the Tungsten-188/Rhenium188 generator and concentrator system and preparation of Re-188 HDD: A report. World J Nucl Med. (2004) 3:137–143.
39. Mirzadeh S, Du M, Beets AL, Knapp FF (Russ) Jr. Thermoseparation of neutron-irradiated tungsten from Re and Os. Indust Engineer Chem Res. (2000) 39:3169–72. doi: 10.1021/ie990582x
40. Ponsard B, Hiltunen J, Penttilla P, Vera-Ruiz H, Beets AL, Mirzadeh S, et al. The tungsten-188/rhenium-188 generator: effective coordination of tungsten-188 production between the HFIR and BR2 Reactors. J Radioanal Nucl Chem. (2003) 257:169–174. doi: 10.1023/A:1024730301381
41. Lewis RE, Eldridge JS. Production of 70-day W-188 and development of a 17-hour Re-188 radioisotope generator J Nucl Med. (1966) 7:804–5.
42. Knapp FF (Russ) Jr, Callahan AP, Beets AL, Mirzadeh S, Hsieh. BT. Processing of reactor-produced tungsten-188 for fabrication of clinical scale alumina-based tungsten-188/Rhenium-188 generators Appl Radiat Isot. (1994) 45:1123–8. doi: 10.1016/0969-8043(94)90026-4
43. Mushtaq A. Recovery of enriched 186W from spent 188W/188Re generators. Appl Radiat Isot. (1996) 47:727–9. doi: 10.1016/0969-8043(96)00032-2
44. Kamioki H, Mirzadeh S, Lambrecht RM, Knapp FF (Russ) Jr, Dadachova E. Tungsten-188/Rhenium-188 generator for biomedical applications Radiochim Acta. (1994) 65:39–46.
45. Lee JS, Lee JS, Park UJ, Son KJ, Han HS. Development of a high performance 188W/188Re generator using synthetic alumina. Appl Radiat Isot. (2009) 67:1162–6. doi: 10.1016/j.apradiso.2009.02.062
46. Jäckel B, Cripps R, Güntay S, Bruchertseifer H. Development of semi-automated system for preparation of 188Re aqueous solutions of high and reproducible activity concentrations. Appl Radiat Isot. (2005) 63:299–304. doi: 10.1016/j.apradiso.2005.04.009
47. Luo TY, Lo AR, Hsieh BT, Lin WJ. A design for automatic preparation of highly concentrated 188Re-perrhenate solutions. Appl Radiat Isot. (2007) 65:21–5. doi: 10.1016/j.apradiso.2006.03.016
48. Wunderlich G, Hartmann H, Andreef M, Kotzerke J. A semi-automated system for concentration of rhenium-188 for radiopharmaceutical applications. Appl Radiat Isot. (2008) 66:1876–80. doi: 10.1016/j.apradiso.2008.04.015
49. Singh J, Reghebi K, Lazarus CR, Clarke SEM, Callahan AP, Knapp FF Jr, et al. Studies on the preparation and isomeric composition of [186Re]- and [188Re]-pentavalent rhenium dimercaptosuccinic acid complex. Nucl Med Commun. (1993) 14:197–203. doi: 10.1097/00006231-199303000-00009
50. Knapp FF (Russ) Jr., Guhlke S, Weinberger J, Beets AL, Amols H, Palmedo H, et al. High specific volume rhenium-188 - clinical potential of a readily available therapeutic radioisotope Nuklearmedizin. (1997) 36:A38.
51. Blower PJ. Extending the life of a 99mTc generator: a simple and convenient method for concentrating generator eluate for clinical use. Nucl Med Commun. (1993) 14:995–7. doi: 10.1097/00006231-199311000-00010
52. Guhlke S, Beet al, Oetjen K, Mirzadeh S, Biersack HJ, Knapp FF (Russ) Jr. Simple new method for effective concentration of 188Re solutions from alumina-based 188W—188Re Generator. J Nucl Med. (2000) 41:1271–1278.
53. Mushtaq A. Concentration of 99m/188 by a single, compact, anion exchange cartridge Nucl Med Commun. (2004) 25:957–62. doi: 10.1097/00006231-200409000-00014
54. Sarkar SK, Venkatesh M, Ramammorthy N. Evaluation of two methods for concentrating perrhenate. (188Re) eluates obtained from 188W-188Re generator. Appl Radiat Isot. (2009) 67:234–9. doi: 10.1016/j.apradiso.2008.09.011
55. Chakravarty R, Dash A, Pillai MRA, Venkatesh M. Post-elution concentration of 188Re by an electrochemical method. Appl Radiat Isot. (2010) 68:2302–5. doi: 10.1016/j.apradiso.2010.06.022
56. Le VS, Morcos N, Bogulski Z. Development of multiple-elution cartridge-based radioisotope concentrator device for increasing the 99mTc and 188Re concentration and the effectiveness of 99mTc/99Mo utilisation. J Radioanal Nucl Chem. (2015) 303:1173–8. doi: 10.1007/s10967-014-3439-9
57. Chhabra A, Rathore Y, Bhusari P, Vatsa R, Mittal BR, Shukla J. Concentration protocol of rhenium-188 perrhenate eluted from tungsten-188/rhenium-188 generator for the preparation of high-yield rhenium-188-labelled radiopharmaceuticals. Nucl Med Commun. (2018) 39:957–9. doi: 10.1097/MNM.0000000000000893
58. Dadachova E, Carrasco N. The Na/I symporter. (NIS): imaging and therapeutic applications. Semin Nucl Med. (2004) 34:23–31. doi: 10.1053/j.semnuclmed.2003.09.004
59. Riesco-Eizaguirre G, Santisteban P. A perspective view of sodium iodide symporter research and its clinical implications. Eur J Endocrinol. (2006) 155:495–512. doi: 10.1530/eje.1.02257
60. Zuckier LS, Dohan O, Li Y, Chang CJ, Carrasco N, Dadachova E. Kinetics of perrhenate uptake and comparative biodistribution of perrhenate, pertechnetate, and iodide by NaI symporter-expressing tissues in vivo. J Nucl Med. (2004) 45:500–7.
61. Dadachova E, Bouzahzah B, Zuckier LS, Pestell RG. Rhenium-188 as an alternative to Iodine-131 for treatment of breast tumors expressing the sodium/iodide symporter. (NIS). Nucl Med Biol. (2002) 29:13–8. doi: 10.1016/S0969-8051(01)00279-7
62. Dadachova E, Nguyen A, Lin EY, Gnatovskiy L, Lu P, Pollard JW. Treatment with rhenium-188-perrhenate and iodine-131 of NIS-expressing mammary cancer in a mouse model remarkably inhibited tumor growth. Nucl Med Biol. (2005) 32:695–700. doi: 10.1016/j.nucmedbio.2005.05.007
63. Willhauck MJ, Sharif Samani BR, Gildehaus FJ, Wolf I, Senekowitsch-Schmidtke R, Stark HJ, et al. Application of 188rhenium as an alternative radionuclide for treatment of prostate cancer after tumor-specific sodium iodide symporter gene expression. J Clin Endocrinol Metab. (2007) 92:4451–8. doi: 10.1210/jc.2007-0402
64. Klutz K, Willhauck MJ, Wunderlich N, Zach C, Anton M, Senekowitsch-Schmidtke R, et al. Sodium iodide symporter. (NIS)-mediated radionuclide. (131I, 188Re) therapy of liver cancer after transcriptionally targeted intratumoral in vivo NIS gene delivery. Hum Gene Ther. (2011) 22:1403–12. doi: 10.1089/hum.2010.158
65. Zhang M, Shi S, Guo R, Miao Y, Li B. Use of rhenium-188 for in vivo imaging and treatment of human cervical cancer cells transfected with lentivirus expressing sodium iodide symporter. Oncol Rep. (2016) 36:2289–97. doi: 10.3892/or.2016.5034
66. Touchefeu Y, Franken P, Harrington KJ. Radiovirotherapy: principles and prospects in oncology. Curr Pharm Des. (2012) 18:3313–20. doi: 10.2174/1381612811209023313
67. Jürgens S, Herrmann WA, Kühn FE. Rhenium and technetium based radiopharmaceuticals: Development and recent advances. J Organomet Chem. (2014) 751:83–9. doi: 10.1016/j.jorganchem.2013.07.042
68. Piepsz A, Colarinha P, Gordon I, Hahn K, Olivier P, Roca I, et al. Guidelines for 99mTc-DMSA scintigraphy in children. Eur J Nucl Med. (2001) 28: BP37–41.
69. Clarke SE, Lazarus CR, Wraight P, Sampson C, Maisey MN. Pentavalent [99mTc]DMSA, [131I]MIBG, and [99mTc]MDP–an evaluation of three imaging techniques in patients with medullary carcinoma of the thyroid. J Nucl Med. (1988) 29:33–8.
70. Bolzati C, Boschi A, Uccelli L, Duatti A, Franceschini R, Piffanelli A. An alternative approach to the preparation of 188Re radiopharmaceuticals from generator-produced [188ReO4]−: efficient synthesis of 188Re(V)-meso-2,3-dimercaptosuccinic acid. Nucl Med Biol. (2000) 27:309–14. doi: 10.1016/S0969-8051(00)00079-2
71. Blower PJ, Lam AS, O'Doherty MJ, Kettle AG, Coakley AJ, Knapp FF Jr. Pentavalent rhenium-188 dimercaptosuccinic acid for targeted radiotherapy: synthesis and preliminary animal and human studies. Eur J Nucl Med. (1998) 25:613–21. doi: 10.1007/s002590050263
72. García-Salinas L, Ferro-Flores G, Arteaga-Murphy C, Pedraza-López M, Hernández-Gutiérrez S, Azorin-Nieto J. Uptake of the 188Re(V)-DMSA complex by cervical carcinoma cells in nude mice: pharmacokinetics and dosimetry. Appl Radiat Isot. (2001) 54:413–8. doi: 10.1016/S0969-8043(00)00278-5
73. Blower PJ, Kettle AG, O'Doherty MJ, Coakley AJ, Knapp FF Jr. 99mTc(V)DMSA quantitatively predicts 188Re(V)DMSA distribution in patients with prostate cancer metastatic to bone. Eur J Nucl Med. (2000) 27:1405–9. doi: 10.1007/s002590000307
74. Dadachova E, Chapman J. 188Re(V)-DMSA revisited: preparation and biodistribution of a potential radiotherapeutic agent with low kidney uptake. Nucl Med Commun. (1998) 19:173–81. doi: 10.1097/00006231-199802000-00012
75. Gdowski AS, Ranjan A, Vishwanatha JK. Current concepts in bone metastasis, contemporary therapeutic strategies and ongoing clinical trials. J Exp Clin Cancer Res. (2017) 36:108. doi: 10.1186/s13046-017-0578-1
76. Damerla V, Packianathan S, Boerner PS, Jani AB, Vijayakumar S, Vijayakumar V. Recent developments in nuclear medicine in the management of bone metastases: a review and perspective. Am J Clin Oncol. (2005) 28:513–20. doi: 10.1097/01.coc.0000162425.55457.10
77. Liepe K, Kotzerke J. Internal radiotherapy of painful bone metastases. Methods. (2011) 55:258–70. doi: 10.1016/j.ymeth.2011.07.001
78. Rubini G, Nicoletti A, Rubini D, Asabella AN. Radiometabolic treatment of bone-metastasizing cancer: from 186rhenium to 223radium. Cancer Biother Radiopharm. (2014) 29:1–11. doi: 10.1089/cbr.2013.1549
79. Sabet A, Khalaf F, Haslerud T, Al-Zreiqat A, Sabet A, Simon B, et al. Bone metastases in GEP-NET: response and long-term outcome after PRRT from a follow-up analysis. Am J Nucl Med Mol Imaging. (2013) 3:437–45.
80. Heck MM, Retz M, D'Alessandria C, Rauscher I, Scheidhauer K, Maurer T, et al. Systemic Radioligand Therapy with 177Lu labeled prostate specific membrane antigen ligand for imaging and therapy in patients with metastatic castration resistant prostate cancer. J Urol. (2016) 196:382–91. doi: 10.1016/j.juro.2016.02.2969
81. Lawrence JH, Tuttle LW, Scott KG, Connor CL. Studies on neoplasms with the aid of radioactive phosphorus. I. The total phosphorus metabolism of normal and leukemic mice. J Clin Invest. (1940) 19:267–71. doi: 10.1172/JCI101129
82. Mathieu L, Chevalier P, Galy G, Berger M. Preparation of rhenium-186 labelled EHDP and its possible use in the treatment of osseous neoplasms. Int J Appl Radiat Isot. (1979) 30:725–7. doi: 10.1016/0020-708X(79)90150-9
83. Hosain F, Spencer RP. Radiopharmaceuticals for palliation of metastatic osseous lesions: biologic and physical background. Semin Nucl Med. (1992) 22:11–6. doi: 10.1016/S0001-2998(05)80152-7
84. Bouchet LG, Bolch WE, Goddu SM, Howell RW, Rao DV. Considerations in the selection of radiopharmaceuticals for palliation of bone pain from metastatic osseous lesions. J Nucl Med. (2000) 41:682–7.
85. Pauwels EK, Stokkel MP. Radiopharmaceuticals for bone lesions. Imaging and therapy in clinical practice. Q J Nucl Med. (2001) 45:18–26.
86. Liepe K, Shinto A. From palliative therapy to prolongation of survival: 223RaCl2 in the treatment of bone metastases. Ther Adv Med Oncol. (2016) 8:294–304. doi: 10.1177/1758834016640494
87. Das T, Banerjee S. Radiopharmaceuticals for metastatic bone pain palliation: available options in the clinical domain and their comparisons. Clin Exp Metastasis. (2017) 34:1–10. doi: 10.1007/s10585-016-9831-9
88. Bouman-Wammes EW, de Klerk JMH, Bloemendal HJ, Van Dodewaard-de Jong JM, Lange R, Ter Heine R, et al. Bone-targeting radiopharmaceuticals as monotherapy or combined with chemotherapy in patients with castration-resistant prostate cancer metastatic to bone. Clin Genitourin Cancer. (2018) 17:e281–92. doi: 10.1016/j.clgc.2018.11.014
89. Guerra-Liberal FDC, Tavares AAS, Tavares JMRS. Palliative treatment of metastatic bone pain with radiopharmaceuticals: a perspective beyond Strontium-89 and Samarium-153. Appl Radiat Isot. (2016) 110:87–99. doi: 10.1016/j.apradiso.2016.01.003
90. Maxon HR 3rd, Schroder LE, Washburn LC, Thomas SR, Samaratunga RC, Biniakiewicz D, et al. Rhenium-188(Sn)HEDP for treatment of osseous metastases. J Nucl Med. (1998) 39:659–63.
91. Palmedo H, Guhlke S, Bender H, Sartor J, Schoeneich G, Risse J, et al. Dose escalation study with rhenium-188 hydroxyethylidene diphosphonate in prostate cancer patients with osseous metastases. Eur J Nucl Med. (2000) 27:123–30. doi: 10.1007/s002590050017
92. Savio E, Gaudiano J, Robles AM, Balter H, Paolino A, López A, et al. Re-HEDP: pharmacokinetic characterization, clinical and dosimetric evaluation in osseous metastatic patients with two levels of radiopharmaceutical dose. BMC Nucl Med. (2001) 1: 2. doi: 10.1186/1471-2385-1-2
93. Palmedo H, Manka-Waluch A, Albers P, Schmidt-Wolf IG, Reinhardt M, Ezziddin S, et al. Repeated bone-targeted therapy for hormone-refractory prostate carcinoma: tandomized phase II trial with the new, high-energy radiopharmaceutical rhenium-188 hydroxyethylidenediphosphonate. J Clin Oncol. (2003) 21:2869–75. doi: 10.1200/JCO.2003.12.060
94. Biersack HJ, Palmedo H, Andris A, Rogenhofer S, Knapp FF (Russ), Guhlke S, et al. Palliation and survival after repeated 188Re-HEDP therapy of hormone-refractory bone metastases of prostate cancer: a retrospective analysis. J Nucl Med. (2011) 52:1721–6. doi: 10.2967/jnumed.111.093674
95. Liepe K, Kropp J, Hliscs R, Franke WG. Significant reduction of the mass of bone metastasis 1 year after rhenium-186 HEDP pain palliation therapy. Clin Nucl Med. (2000) 25:901–4. doi: 10.1097/00003072-200011000-00009
96. Liepe K, Franke WG, Kropp J, Koch R, Runge R, Hliscs R. Comparison of Rhenium-188, Rhenium-186-HEDP and Strontium-89 in palliation of painful bone metastases. Nuklearmedizin. (2000) 39:146–51. doi: 10.1055/s-0038-1632262
97. Liepe K, Runge R, Kotzerke J. The benefit of bone-seeking radiopharmaceuticals in the treatment of metastatic bone pain. J Cancer Res Clin Oncol. (2005) 131:60–6. doi: 10.1007/s00432-004-0625-0
98. Liepe K, Kotzerke J. A comparative study of 188Re-HEDP, 186Re-HEDP, 153Sm-EDTMP and 89Sr in the treatment of painful skeletal metastases. Nucl Med Commun. (2007) 28:623–30. doi: 10.1097/MNM.0b013e32825a6adc
99. Liepe K, Hliscs R, Kropp J, Runge R, Knapp FF (Russ) Jr, Franke WG. Dosimetry of 188Re-hydroxyethylidene diphosphonate in human prostate cancer skeletal metastases. J Nucl Med. (2003) 44:953–60.
100. Liepe K, Hliscs R, Kropp J, Grüning T, Runge R, Koch R, et al. Rhenium-188-HEDP in the palliative treatment of bone metastases. Cancer Biother Radiopharm. (2000) 15:261–5. doi: 10.1089/108497800414356
101. Liepe K, Kropp J, Runge R, Kotzerke J. Therapeutic efficiency of rhenium-188-HEDP in human prostate cancer skeletal metastases. Br J Cancer. (2003) 89:625–9. doi: 10.1038/sj.bjc.6601158
102. Zhang H, Tian M, Li S, Liu J, Tanada S, Endo K. Rhenium-188-HEDP therapy for the palliation of pain due to osseous metastases in lung cancer patients. Cancer Biother Radiopharm. (2003) 18:719–26. doi: 10.1089/108497803770418265
103. Li S, Liu J, Zhang H, Tian M, Wang J, Zheng X. Rhenium-188 HEDP to treat painful bone metastases. Clin Nucl Med. (2001) 26:919–22. doi: 10.1097/00003072-200111000-00006
104. Cheng A, Chen S, Zhang Y, Yin D, Dong M. The tolerance and therapeutic efficacy of rhenium-188 hydroxyethylidene diphosphonate in advanced cancer patients with painful osseous metastases. Cancer Biother Radiopharm. (2011) 26:237–44. doi: 10.1089/cbr.2010.0873
105. Shinto AS, Mallia MB, Kameswaran M, Kamaleshwaran KK, Joseph J, Radhakrishnan ER, et al. Clinical utility of 188Rhenium-hydroxyethylidene-1,1-diphosphonate as a bone pain palliative in multiple malignancies. World J Nucl Med. (2018) 17:228–35. doi: 10.4103/wjnm.WJNM_68_17
106. Lange R, Overbeek F, de Klerk JM, Pasker-de Jong PC, van den Berk AM, Ter Heine R, et al. Treatment of painful bone metastases in prostate and breast cancer patients with the therapeutic radiopharmaceutical rhenium-188-HEDP. Clinical benefit in a real-world study. Nuklearmedizin. (2016) 55:188–95. doi: 10.3413/Nukmed-0828-16-05
107. Sabet A, Khalaf F, Mahjoob S, Al-Zreiqat A, Biersack HJ, Ezziddin S. May bone-targeted radionuclide therapy overcome PRRT-refractory osseous disease in NET? A pilot report on 188Re-HEDP treatment in progressive bone metastases after 177Lu-octreotate Am J Nucl Med Mol Imaging. (2014) 4:80–8.
108. Lam M, Bosma TB, van Rijk PP, Zonnenberg BA. 188Re-HEDP combined with capecitabine in hormone-refractory prostate cancer patients with bone metastases: a phase I safety and toxicity study. Eur J Nucl Med Mol Imaging. (2009) 369:1425–33. doi: 10.1007/s00259-009-1119-8
109. Lange R, ter Heine R, van Wieringen WN, Tromp AM, Paap M, Bloemendal HJ, et al. Cytotoxic effects of the therapeutic radionuclide rhenium-188 combined with taxanes in human prostate carcinoma cell lines. Cancer Biother Radiopharm. (2017) 32:16–23. doi: 10.1089/cbr.2016.2129
110. Verdera ES, Gaudiano J, Leon A, Martinez G, Robles A, Savio E, et al. Rhenium-188-HEDP-kit formulation and quality control. Radiochim Acta. (1997) 2:113–7. doi: 10.1524/ract.1997.79.2.113
111. Hashimoto K. Synthesis of a 188Re-HEDP complex using carrier-free 188Re, and a study of its stability. Appl Radiat Isot. (1998) 49:351–6. doi: 10.1016/S0969-8043(97)00284-4
112. Hsieh BT, Hsieh JF, Tsai SC, Lin WY, Wang SJ, Ting G. Comparison of various rhenium-188-labeled diphosphonates for the treatment of bone metastases. Nucl Med Biol. (1999) 26:973–6. doi: 10.1016/S0969-8051(99)00075-X
113. Lin WY, Hsieh JF, Lin CP, Hsieh BT, Ting G, Wang SJ, et al. Effect of reaction conditions on preparations of rhenium-188 hydroxyethylidene diphosphonate complexes. Nucl Med Biol. (1999) 26:455–9. doi: 10.1016/S0969-8051(99)00007-4
114. Petriev VM. Influence of reactant concentrations and solution acidity on the complexation of 188Re with 1-hydroxyethane-1,1-diphosphonic acid. Radiochemistry. (2008) 50:203–7. doi: 10.1134/S1066362208020227
115. Nassar MY, El-Kolaly MT, Mahran MRH. Synthesis of a 188Re-HEDP complex using carrier-free 188Re and a study of its stability and biological distribution. Radiochemistry. (2011) 53:352–6. doi: 10.1134/S1066362211040151
116. Shiryaeva VK, Petriev VM, Bryukhanova AA, Smoryzanova OA, Skvortsov VG, Shvert OE. Evaluation of the influence of preparation conditions on pharmacokinetics of bone-seeking radiopharmaceutical 188Re-labeled hydroxyethylidenediphosphonic acid monopotassium salt in rats. Pharm Chem J. (2012) 46:443–8. doi: 10.1007/s11094-012-0818-9
117. Lange R, de Klerk JMH, Bloemendal HJ, Ramakers RM, Beekman FJ, van der Westerlaken MML, et al. Drug composition matters: the influence of carrier concentration on the radiochemical purity, hydroxyapatite affinity and in-vivo bone accumulation of the therapeutic radiopharmaceutical 188Rhenium-HEDP. Nucl Med Biol. (2015) 42:465–9. doi: 10.1016/j.nucmedbio.2015.01.007
118. Lange R, Ter Heine R, van der Gronde T, Selles S, de Klerk J, Bloemendal H, et al. Applying quality by design principles to the small-scale preparation of the bone-targeting therapeutic radiopharmaceutical rhenium-188-HEDP. Eur J Pharm Sci. (2016) 30: 90:96–101. doi: 10.1016/j.ejps.2016.01.008
119. ter Heine R, Lange R, Breukels OB, Bloemendal HJ, Rummenie RG, Wakker AM, et al. Bench to bedside development of GMP grade Rhenium-188-HEDP, a radiopharmaceutical for targeted treatment of painful bone metastases. Int J Pharm. (2014) 465:317–24. doi: 10.1016/j.ijpharm.2014.01.034
120. Kochetova T, Krylov V, Smolyarchuk M, Sokov D, Lunev A, Shiryaev S, et al. 188Re zoledronic acid in the palliative treatment of painful bone metastases. Int J Nucl Med Res. (2017) 92–100. doi: 10.15379/2408-9788.2017.08
121. Lange R, Ter Heine R, Knapp FF (Russ), de Klerk JM, Bloemendal HJ, Hendrikse NH. Pharmaceutical and clinical development of phosphonate-based radiopharmaceuticals for the targeted treatment of bone metastases. Bone. (2016) 91:159–79. doi: 10.1016/j.bone.2016.08.002
122. Ansquer C, Kraeber-Bodéré F, Chatal JF. Current status and perspectives in peptide receptor radiation therapy. Curr Pharm Des. (2009) 15:2453–62. doi: 10.2174/138161209788682262
123. Lozza C, Navarro-Teulon I, Pèlegrin A, Pouget JP, Vivès E. Peptides in receptor-mediated radiotherapy: from design to the clinical application in cancers. Front Oncol. (2013) 3:247. doi: 10.3389/fonc.2013.00247
124. Dash A, Chakraborty S, Pillai MR, Knapp FF (Russ) Jr. Peptide receptor radionuclide therapy: an overview. Cancer Biother Radiopharm. (2015) 30:47–71. doi: 10.1089/cbr.2014.1741
125. Larson SM, Carrasquillo JA, Cheung NK, Press OW. Radioimmunotherapy of human tumours. Nat Rev Cancer. (2015) 15:347–60. doi: 10.1038/nrc3925
126. Kraeber-Bodéré F, Barbet J, Chatal JF. Radioimmunotherapy: from current clinical success to future industrial breakthrough? J Nucl Med. (2016) 57:329–31. doi: 10.2967/jnumed.115.167247
127. Goldenberg DM, Sharkey RM. Advances in cancer therapy with radiolabeled monoclonal antibodies. Q J Nucl Med Mol Imaging. (2006) 50:248–64.
128. Uccelli L, Martini P, Pasquali M, Boschi A. Monoclonal Antibodies Radiolabeling with Rhenium-188 for Radioimmunotherapy. Biomed Res Int. (2017) 2017:5923609. doi: 10.1155/2017/5923609
129. Bunjes D. 188Re-labeled anti-CD66 monoclonal antibody in stem cell transplantation for patients with high-risk acute myeloid leukemia. Leukemia Lymphoma. (2002) 43:2125–31. doi: 10.1080/1042819021000033015
130. De Decker M, Bacher K, Thierens H, Slegers G, Dierckx RA, De Vos F. In vitro and in vivo evaluation of direct rhenium-188-labeled anti-CD52 monoclonal antibody alemtuzumab for radioimmunotherapy of B-cell chronic lymphocytic leukemia. Nucl Med Biol. (2008) 35:599–604. doi: 10.1016/j.nucmedbio.2008.03.001
131. Torres-García E, Ferro-Flores G, Arteaga de Murphy C, Correa-González L, Pichardo-Romero PA. Biokinetics and dosimetry of 188Re-anti-CD20 in patients with non-Hodgkin's lymphoma: preliminary experience. Arch Med Res. (2008) 39:100–9. doi: 10.1016/j.arcmed.2007.06.023
132. Li G, Wang Y, Huang K, Zhang H, Peng W, Zhang C. The experimental study on the radioimmunotherapy of the nasopharyngeal carcinoma overexpressing HER2/neu in nude mice model with intratumoral injection of 188Re-herceptin. Nucl Med Biol. (2005) 32:59–65. doi: 10.1016/j.nucmedbio.2004.09.003
133. Luo TY, Tang IC, Wu YL, Hsu KL, Liu SW, Kung HC, et al. Evaluating the potential of 188Re-SOCTA-trastuzumab as a new radioimmunoagent for breast cancer treatment. Nucl Med Biol. (2009) 36:81–8. doi: 10.1016/j.nucmedbio.2008.10.014
134. Wang HY, Lin WY, Chen MC, Lin T, Chao CH, Hsu FN, et al. Inhibitory effects of Rhenium-188-labeled Herceptin on prostate cancer cell growth: a possible radioimmunotherapy to prostate carcinoma. Int J Radiat Biol. (2013) 89:346–55. doi: 10.3109/09553002.2013.762136
135. Xiao J, Xu X, Li X, Li Y, Liu G, Tan H, et al. Re-188 enhances the inhibitory effect of bevacizumab in non-small-cell lung cancer. Molecules. (2016) 21:E1308 doi: 10.3390/molecules21101308
136. Kuo WI, Cheng KH, Chang YJ, Wu TT, Hsu WC, Chen LC, et al. Radiolabeling, Characteristics and NanoSPECT/CT Imaging of 188Re-cetuximab in NCI-H292 Human Lung Cancer Xenografts. Anticancer Res. (2019) 39:183–90. doi: 10.21873/anticanres.13096
137. González-Navarro BO, Casaco A, León M, Leyva R, León A, Santana E, et al. High dose of hR-3, an anti-epidermal growth factor receptor monoclonal antibody labeled with 188rhenium following intravenous injection into rats. J Appl Res. (2006) 6:77–88.
138. Juweid M, Sharkey RM, Swayne LC, Griffiths GL, Dunn R, Goldenberg DM. Pharmacokinetics, dosimetry and toxicity of rhenium-188-labeled anti-carcinoembryonic antigen monoclonal antibody, MN-14, in gastrointestinal cancer. J Nucl Med. (1998) 39:34–42.
139. Murray A, Simms MS, Scholfield DP, Vincent RM, Denton G, Bishop MC, et al. Production and characterization of 188Re-C595 antibody for radioimmunotherapy of transitional cell bladder cancer. J Nucl Med. (2001) 42:726–32.
140. Geller DS, Morris J, Revskaya E, Kahn M, Zhang W, Piperdi S, et al. Targeted therapy of osteosarcoma with radiolabeled monoclonal antibody to an insulin-like growth factor-2 receptor (IGF2R). Nucl Med Biol. (2016) 43:812–7. doi: 10.1016/j.nucmedbio.2016.07.008
141. Chang CH, Tsai LC, Chen ST, Yuan CC, Hung MW, Hsieh BT, et al. Radioimmunotherapy and apoptotic induction on Ck19-overexpressing human cervical carcinoma cells with Re-188-mAbCx-99 Anticancer Res. (2005) 25:2719–28.
142. Phaeton R, Jiang Z, Revskaya E, Fisher DR, Goldberg GL, Dadachova E. Beta emitters rhenium-188 and lutetium-177 are equally effective in radioimmunotherapy of HPV-positive experimental cervical cancer. Cancer Med. (2016) 5:9–16. doi: 10.1002/cam4.562
143. Quispe-Tintaya W, Chandra D, Jahangir A, Harris M, Casadevall A, Dadachova E, et al. Nontoxic radioactive Listeria(at) is a highly effective therapy against metastatic pancreatic cancer. Proc Natl Acad Sci USA. (2013) 110:8668–73. doi: 10.1073/pnas.1211287110
144. Nosanchuk JD, Jeyakumar A, Ray A, Revskaya E, Jiang Z, Bryan RA, et al. Structure-function analysis and therapeutic efficacy of antibodies to fungal melanin for melanoma radioimmunotherapy. Sci Rep. (2018) 8:5466. doi: 10.1038/s41598-018-23889-z
145. Seitz U, Neumaier B, Glatting G, Kotzerke J, Bunjes D, Reske SN. Preparation and evaluation of the rhenium-188-labelled anti-NCA antigen monoclonal antibody BW 250/183 for radioimmunotherapy of leukaemia. Eur J Nucl Med. (1999) 26:1265–73. doi: 10.1007/s002590050582
146. Bunjes D, Buchmann I, Duncker C, Seitz U, Kotzerke J, Wiesneth M, et al. Rhenium 188-labeled anti-CD66. (a, b, c, e) monoclonal antibody to intensify the conditioning regimen prior to stem cell transplantation for patients with high-risk acute myeloid leukemia or myelodysplastic syndrome: results of a phase I-II study. Blood. (2001) 98:565–72. doi: 10.1182/blood.V98.3.565
147. Buchmann I, Bunjes D, Kotzerke J, Martin H, Glatting G, Seitz U, et al. Myeloablative radioimmunotherapy with Re-188-anti-CD66-antibody for conditioning of high-risk leukemia patients prior to stem cell transplantation: biodistribution, biokinetics and immediate toxicities. Cancer Biother Radiopharm. (2002) 17:151–63. doi: 10.1089/108497802753773775
148. Ringhoffer M, Blumstein N, Neumaier B, Glatting G, von Harsdorf S, Buchmann I, et al. 188Re or 90Y-labelled anti-CD66 antibody as part of a dose-reduced conditioning regimen for patients with acute leukaemia or myelodysplastic syndrome over the age of 55: results of a phase I-II study. Br J Haematol. (2005) 130:604–13. doi: 10.1111/j.1365-2141.2005.05663.x
149. Lauter A, Strumpf A, Platzbecker U, Schetelig J, Wermke M, Radke J, et al. 188Re anti-CD66 radioimmunotherapy combined with reduced-intensity conditioning and in-vivo T cell depletion in elderly patients undergoing allogeneic haematopoietic cell transplantation. Br J Haematol. (2010) 148:910–7. doi: 10.1111/j.1365-2141.2009.08025.x
150. Klein SA, Hermann S, Dietrich JW, Hoelzer D, Martin H. Transplantation-related toxicity and acute intestinal graft-versus-host disease after conditioning regimens intensified with Rhenium 188-labeled anti-CD66 monoclonal antibodies. Blood. (2002) 99:2270–1. doi: 10.1182/blood.V99.6.2270
151. Zenz T, Schlenk RF, Glatting G, Neumaier B, Blumstein N, Buchmann I, et al. Bone marrow transplantation nephropathy after an intensified conditioning regimen with radioimmunotherapy and allogeneic stem cell transplantation. J Nucl Med. (2006) 47:278–86.
152. Schneider S, Strumpf A, Schetelig J, Wunderlich G, Ehninger G, Kotzerke J, et al. Reduced-intensity conditioning combined with 188Rhenium radioimmunotherapy before allogeneic hematopoietic stem cell transplantation in elderly patients with acute myeloid leukemia: the role of in vivo t cell depletion. Biol Blood Marrow Transplant. (2015) 21:1754–60. doi: 10.1016/j.bbmt.2015.05.012
153. Zhi Y, Meiying Z, Baohe L, Changying Z, Yan H, Aping M, et al. 188Re-labelled McAb 3H11 used as preventive for the peritoneal micro-metastasis of gastric cancer. Nuclear Science and Techniques. (1999) 10:203–6.
154. Torres LA, Coca MA, Batista JF, Casaco A, Lopez G, García I, et al. Biodistribution and internal dosimetry of the 188Re-labelled humanized monoclonal antibody anti-epidermal growth factor receptor, nimotuzumab, in the locoregional treatment of malignant gliomas. Nucl Med Commun. (2008) 29:66–75. doi: 10.1097/MNM.0b013e3282f1bbce
155. Casacó A, López G, García I, Rodríguez JA, Fernández R, Figueredo J, et al. Phase I single-dose study of intracavitary-administered Nimotuzumab labeled with 188Re in adult recurrent high-grade glioma. Cancer Biol Ther. (2008) 7:333–9. doi: 10.4161/cbt.7.3.5414
156. Klein M, Lotem M, Peretz T, Zwas ST, Mizrachi S, Liberman Y, et al. Safety and efficacy of 188-rhenium-labeled antibody to melanin in patients with metastatic melanoma. J Skin Cancer. (2013) 2013:828329. doi: 10.1155/2013/828329
157. Dadachova E, Nosanchuk JD, Shi L, Schweitzer AD, Frenkel A, Nosanchuk JS, et al. Dead cells in melanoma tumors provide abundant antigen for targeted delivery of ionizing radiation by a mAb to melanin. Proc Natl Acad Sci USA. (2004) 101:14865–70. doi: 10.1073/pnas.0406180101
158. Dadachova E, Nakouzi A, Bryan RA, Casadevall A. Ionizing radiation delivered by specific antibody is therapeutic against a fungal infection. Proc Natl Acad Sci USA. (2003) 100:10942–7. doi: 10.1073/pnas.1731272100
159. Rivera J, Nakouzi AS, Morgenstern A, Bruchertseifer F, Dadachova E, Casadevall A. Radiolabeled antibodies to Bacillus anthracis toxins are bactericidal and partially therapeutic in experimental murine anthrax. Antimicrob Agents Chemother. (2009) 53:4860–8. doi: 10.1128/AAC.01269-08
160. Dadachova E, Patel MC, Toussi S, Apostolidis C, Morgenstern A, Brechbiel MW, et al. Targeted killing of virally infected cells by radiolabeled antibodies to viral proteins. PLoS Med. (2006) 3:e427. doi: 10.1371/journal.pmed.0030427
161. Bolzati C, Carta D, Salvarese N, Refosco F. Chelating systems for 99mTc/188Re in the development of radiolabeled peptide pharmaceuticals. Anticancer Agents Med Chem. (2012) 12:428–61. doi: 10.2174/187152012800617821
162. Howell RC, Revskaya E, Pazo V, Nosanchuk JD, Casadevall A, Dadachova E. Phage display library derived peptides that bind to human tumor melanin as potential vehicles for targeted radionuclide therapy of metastatic melanoma. Bioconjug Chem. (2007) 18:1739–48. doi: 10.1021/bc060330u
163. Li Y, Ma L, Gaddam V, Gallazzi F, Hennkens HM, Harmata M, et al. Synthesis, characterization, and in vitro evaluation of new 99mTc/Re(V)-cyclized octreotide analogues: an experimental and computational approach. Inorg Chem. (2016) 55:1124–33. doi: 10.1021/acs.inorgchem.5b02306
164. Zamora PO, Marek MJ, Knapp FF (Russ) Jr. Preparation of 188Re-RC-160 somatostatin analog: a peptide for local/regional radiotherapy. Appl Radiat Isot. (1997) 48:305–9. doi: 10.1016/S0969-8043(96)00226-6
165. Arteaga de Murphy C, Pedraza-López M, Ferro-Flores G, Murphy-Stack E, Chávez-Mercado L, Ascencio JA, et al. Uptake of 188Re-beta-naphthyl-peptide in cervical carcinoma tumours in athymic mice. Nucl Med Biol. (2001) 28:319–26. doi: 10.1016/S0969-8051(00)00174-8
166. Molina-Trinidad EM, de Murphy CA, Ferro-Flores G, Murphy-Stack E, Jung-Cook H. Radiopharmacokinetic and dosimetric parameters of 188Re-lanreotide in athymic mice with induced human cancer tumors. Int J Pharm. (2006) 310:125–30. doi: 10.1016/j.ijpharm.2005.11.043
167. Cyr JE, Pearson DA, Wilson DM, Nelson CA, Guaraldi M, Azure MT, et al. Somatostatin receptor-binding peptides suitable for tumor radiotherapy with Re-188 or Re-186. Chemistry and initial biological studies. J Med Chem. (2007) 50:1354–64. doi: 10.1021/jm061290i
168. Yu F, Lv M, Cai H, Li D, Yuan X, Lv Z. Therapeutic effect of 188Re-MAG3-depreotide on non-small cell lung cancer in vivo and in vitro. Int J Clin Exp Pathol. (2013) 6:421–30.
169. Cui L, Liu Z, Jin X, Jia B, Li F, Wang F. Evaluation of 188Re-MAG2-RGD-bombesin for potential prostate cancer therapy. Nucl Med Biol. (2013) 40:182–9. doi: 10.1016/j.nucmedbio.2012.11.002
170. Kan W, Zhou Z, Wei H, Zhong Z. Effective way to radiolabel the peptide of MAG3-RM26 with 188Re and the study on its coordination chemistry. J Radioanal Nucl Chem. (2017) 314:2087–90. doi: 10.1007/s10967-017-5580-8
171. Smilkov K, Janevik E, Guerrini R, Pasquali M, Boschi A, Uccelli L, et al. Preparation and first biological evaluation of novel Re-188/Tc-99m peptide conjugates with Substance-P. Appl Radiat Isot. (2014) 92:25–31. doi: 10.1016/j.apradiso.2014.06.003
172. Li Y, Hu Y, Xiao J, Liu G, Li X, Zhao Y, et al. Investigation of SP94 Peptide as a specific probe for hepatocellular carcinoma imaging and therapy. Sci Rep. (2016) 6:33511. doi: 10.1038/srep33511
173. Zhang X, Feng S, Liu J, Li Q, Zheng L, Xie L, et al. Novel small peptides derived from VEGF125−136: potential drugs for radioactive diagnosis and therapy in A549 tumor-bearing nude mice. Sci Rep. (2017) 7:4278. doi: 10.1038/s41598-017-04513-y
174. Wang SH, Lee AC, Chen IJ, Chang NC, Wu HC, Yu HM, et al. Structure-based optimization of GRP78-binding peptides that enhances efficacy in cancer imaging and therapy. Biomaterials. (2016) 94:31–44. doi: 10.1016/j.biomaterials.2016.03.050
175. Miao Y, Quinn TP. Peptide-targeted radionuclide therapy for melanoma. Crit Rev Oncol Hematol. (2008) 67:213–28. doi: 10.1016/j.critrevonc.2008.02.006
176. Carta D, Salvarese N, Morellato N, Gao F, Sihver W, Pietzsch HJ, et al. Melanoma targeting with [99mTc(N)(PNP3)]-labeled α-melanocyte stimulating hormone peptide analogs: effects of cyclization on the radiopharmaceutical properties. Nucl Med Biol. (2016) 43:788–801. doi: 10.1016/j.nucmedbio.2016.08.014
177. Edelman MJ, Clamon G, Kahn D, Magram M, Lister-James J, Line BR. Targeted radiopharmaceutical therapy for advanced lung cancer: phase I trial of rhenium Re188 P2045, a somatostatin analog. J Thorac Oncol. (2009) 4:1550–4. doi: 10.1097/JTO.0b013e3181bf1070
178. Khajornjiraphan N, Thu NA, Chow PK. Yttrium-90 microspheres: a review of its emerging clinical indications. Liver Cancer. (2015) 4:6–15. doi: 10.1159/000343876
179. Bozkurt MF, Salanci BV, Ugur Ö. Intra-arterial radionuclide therapies for liver tumors. Semin Nucl Med. (2016) 46:324–39. doi: 10.1053/j.semnuclmed.2016.01.008
180. Bouvry C, Palard X, Edeline J, Ardisson V, Loyer P, Garin E, et al. Transarterial radioembolisation (TARE) agents beyond 90Y-microspheres. Biomed Res Int. (2018) 2018:1435302. doi: 10.1155/2018/1435302
181. Lepareur N, Garin E. Transarterial radionuclide therapy with 188Re-labelled lipiodol. Int J Nucl Med Res. (2017) 79–91. doi: 10.15379/2408-9788.2017.07
182. Paeng JC, Jeong JM, Yoon CJ, Lee YS, Suh YG, Chung JW, et al. Lipiodol solution of 188Re-HDD as a new therapeutic agent for transhepatic arterial embolization in liver cancer: preclinical study in a rabbit liver cancer model. J Nucl Med. (2003) 44:2033–8.
183. Boschi A, Uccelli L, Duatti A, Colamussi P, Cittanti C, Filice A, et al. A kit formulation for the preparation of 188Re-lipiodol: preclinical studies and preliminary therapeutic evaluation in patients with unresectable hepatocellular carcinoma. Nucl Med Commun. (2004) 25:691–9. doi: 10.1097/01.mnm.0000130241.22068.45
184. Lepareur N, Ardisson V, Noiret N, Garin E. 188Re-SSS/Lipiodol: development of a potential treatment for HCC from bench to bedside. Int J Mol Imaging. (2012) 2012:278306. doi: 10.1155/2012/278306
185. Keng GH, Sundram FX, Yu SW, Somanesan S, Premaraj J, Oon CJ, et al. Preliminary experience in radionuclide therapy of hepatocellular carcinoma using hepatic intra-arterial radioconjugates. Ann Acad Med Singapore. (2002) 31:382–6.
186. Sundram F, Chau TCM, Onkhuudai P, Bernal P, Padhy AK. Preliminary results of transarterial rhenium-188 HDD Lipiodol in the treatment of inoperable primary hepatocellular carcinoma. Eur J Nucl Med Mol Imaging. (2004) 31:250–7. doi: 10.1007/s00259-003-1363-2
187. De Ruyck K, Lambert B, Bacher K, Gemmel F, De Vos F, Vral A, et al. Biologic dosimetry of 188Re-HDD/Lipiodol versus 131I-Lipiodol therapy in patients with hepatocellular carcinoma. J Nucl Med. (2004) 45:612–8.
188. Lambert B, Bacher K, Defreyne L, Gemmel F, Van Vlierberghe H, Jeong JM, et al. 188Re-HDD/Lipiodol therapy for hepatocellular carcinoma: a phase I clinical trial. J Nucl Med. (2005) 46:60–6.
189. Lambert B, Bacher K, De Keukeleire K, Smeets P, Colle I, Jeong JM, et al. 188Re-HDD/Lipiodol for treatment of hepatocellular carcinoma: a feasibility study in patients with advanced cirrhosis. J Nucl Med. (2005) 46:1326–32.
190. Lambert B, Praet M, Vanlangenhove P, Troisi R, de Hemptinne B, Gemmel F, et al. Radiolabeled Lipiodol therapy for hepatocellular carcinoma in patients awaiting liver transplantation: pathology of the explant livers and clinical outcome. Cancer Biother Radiopharm. (2005) 20:209–14. doi: 10.1089/cbr.2005.20.209
191. Kumar A, Bal C, Srivastava DN, Acharya SK, Thulkar SP, Sharma S, et al. Transarterial radionuclide therapy with Re-188-HDD-lipiodol in case of unresectable hepatocellular carcinoma with extensive portal vein thrombosis. Eur J Radiology Extra. (2005) 56:55–9. doi: 10.1016/j.ejrex.2005.07.016
192. Kumar A, Srivastava DN, Bal C. Management of postsurgical recurrence of hepatocellular carcinoma with Rhenium 188–HDD labeled iodized oil. J Vasc Interv Radiol. (2006) 17:157–161. doi: 10.1097/01.RVI.0000195321.20579.F2
193. Kumar A, Bal C, Srivastava DN, Thulkar SP, Sharma S, Acharya SK, et al. Management of multiple intrahepatic recurrences after radiofrequency ablation of hepatocellular carcinoma with rhenium-188–HDD-Lipiodol. Eur J Gastroenterol Hepatol. (2006) 18:219–3. doi: 10.1097/00042737-200602000-00016
194. Lambert B, Bacher K, Defreyne L, Van Vlierberghe H, Jeong JM, Wang RF, et al. 188Re-HDD/lipiodol therapy for hepatocellular carcinoma: an activity escalation study. Eur J Nucl Med Mol Imaging. (2006) 33:344–52. doi: 10.1007/s00259-005-1954-1
195. Kumar A, Srivastava DN, Chau TTM, Long HD, Bal C, Chandra P, et al. Inoperable hepatocellular carcinoma: transarterial 188Re HDD-labeled iodized oil for treatment prospective multicenter clinical trial. Radiology. (2007) 243:509–19. doi: 10.1148/radiol.2432051246
196. Bernal P, Raoul JL, Vidmar G, Sereegotov E, Sundram FX, Kumar A, et al. Intra-arterial Rhenium-188 Lipiodol in the treatment of inoperable hepatocellular carcinoma: results of an IAEA-sponsored multination study. Int J Radiat Oncol Biol Phys. (2007) 69:1448–55. doi: 10.1016/j.ijrobp.2007.05.009
197. Bernal P, Raoul JL, Stare J, Sereegotov E, Sundram FX, Kumar A, et al. International Atomic Energy Agency sponsored multination study of intra-arterial rhenium-188-labeled Lipiodol in the treatment of inoperable hepatocellular carcinoma: results with special emphasis on prognostic value of dosimetric study. Sem Nucl Med. (2008) 38:S40–5. doi: 10.1053/j.semnuclmed.2007.10.006
198. Esquinas PL, Shinto A, Kamaleshwaran KK, Joseph J, Celler A. Biodistribution, pharmacokinetics, and organ-level dosimetry for 188Re-AHDDLipiodol radioembolization based on quantitative post-treatment SPECT/CT scans. EJNMMI Physics. (2018) 5:30. doi: 10.1186/s40658-018-0227-6
199. Delaunay K, Garin E, Rolland Y, Lepareur N, Laffont S, Ardisson V, et al. Biodistribution and dosimetry assessments for hepatocellular carcinoma treated with 188Re-SSS Lipiodol: Preliminary results of the phase 1 trial Lip Re 1. J Nucl Med. (2018) 59:602. doi: 10.1007/s00259-019-04277-9
200. Padhy AK, Dondi M. A report on the implementation aspects of the International Atomic Energy Agency's first doctoral coordinated research project, “Management of liver cancer using radionuclide methods with special emphasis on trans-arterial radio-conjugate therapy and internal dosimetry”. Semin Nucl Med. (2008) 38:S5–12. doi: 10.1053/j.semnuclmed.2007.10.002
201. Banka VK, Moon SH, Jeong JM, Seelam SR, Lee YS, Kim YJ, et al. Development of 4-hexadecyl-4,7-diaza-1,10-decanedithiol. (HDD) kit for the preparation of the liver cancer therapeutic agent Re-188-HDD/lipiodol. Nucl Med Biol. (2015) 42:317–322. doi: 10.1016/j.nucmedbio.2014.11.013
202. Conzone SD, Häfeli UO, Day DE, Ehrhardt GJ. Preparation and properties of radioactive rhenium glass microspheres intended for in vivo radioembolization therapy. J Biomed Mater Res. (1998) 42:617–25.
203. Wang SJ, Lin WY, Chen MN, Chi CS, Chen JT, Ho WL, et al. Intratumoral injection of rhenium-188 microspheres into an animal model of hepatoma. J Nucl Med. (1998) 39:1752–7.
204. Wunderlich G, Pinkert J, Stintz M, Kotzerke J. Labeling and biodistribution of different particle materials for radioembolization therapy with 188Re. Appl Radiat Isot. (2005) 62:745–50. doi: 10.1016/j.apradiso.2004.11.003
205. Verger E, Drion P, Meffre G, Bernard C, Duwez L, Lepareur N, et al. 68Ga and 188Re starch-based microparticles as theranostic tool for the hepatocellular carcinoma: radiolabeling and preliminary in vivo rat studies. PLoS ONE. (2016) 11:e0164626. doi: 10.1371/journal.pone.0164626
206. Liepe K, Brogsitter C, Leonhard J, Wunderlich G, Hliscs R, Pinkert J, et al. Feasibility of high activity rhenium-188-microsphere in hepatic radioembolization. Jpn J Clin Oncol. (2007) 37:942–50. doi: 10.1093/jjco/hym137
207. Nowicki ML, Cwikla JB, Sankowski AJ, Shcherbinin S, Grimmes J, Celler A, et al. Initial study of radiological and clinical efficacy radioembolization using 188Re-human serum albumin. (HSA) microspheres in patients with progressive, unresectable primary or secondary liver cancers. Med Sci Monit. (2014) 20:1353–62. doi: 10.12659/MSM.890480
208. Liepe K, Kotzerke J, Lambert B. Advantage of 188Re-radiopharmaceuticals in hepatocellular cancer and liver metastases. J Nucl Med. (2005) 46:1407–8.
209. Hoefnagel CA. Radionuclide therapy revisited. Eur J Nucl Med. (1991) 18:408–31. doi: 10.1007/BF02258432
210. Chen FD, Hsieh BT, Wang HE, Ou YH, Yang WK, Whang-Peng J, et al. Efficacy of Re-188-labelled sulphur colloid on prolongation of survival time in melanoma-bearing animals. Nucl Med Biol. (2001) 28:835–44. doi: 10.1016/S0969-8051(01)00244-X
211. Häfeli UO, Pauer GJ, Unnithan J, Prayson RA. Fibrin glue system for adjuvant brachytherapy of brain tumors with 188Re and 186Re-labeled microspheres. Eur J Pharm Biopharm. (2007) 65:282–8. doi: 10.1016/j.ejpb.2006.10.016
212. Vanpouille-Box C, Lacoeuille F, Belloche C, Lepareur N, Lemaire L, LeJeune JJ, et al. Tumor eradication in rat glioma and bypass of immunosuppressive barriers using internal radiation with 188Re-lipid nanocapsules. Biomaterials. (2011) 32:6781–90. doi: 10.1016/j.biomaterials.2011.05.067
213. Cikankowitz A, Clavreul A, Tétaud C, Lemaire L, Rousseau A, Lepareur N, et al. Characterization of the distribution, retention, and efficacy of internal radiation of 188Re-lipid nanocapsules in an immunocompromised human glioblastoma model. J Neurooncol. (2017) 131:49–58. doi: 10.1007/s11060-016-2289-4
214. Séhédic D, Chourpa I, Tétaud C, Griveau A, Loussouarn C, Avril S, et al. Locoregional confinement and major clinical benefit of 188Re-Loaded CXCR4-targeted nanocarriers in an orthotopic human to mouse model of glioblastoma. Theranostics. (2017) 7:4517–36. doi: 10.7150/thno.19403
215. Hrycushko BA, Gutierrez AN, Goins B, Yan W, Phillips WT, Otto PM, et al. Radiobiological characterization of post-lumpectomy focal brachytherapy with lipid nanoparticle-carried radionuclides. Phys Med Biol. (2011) 56:703–19. doi: 10.1088/0031-9155/56/3/011
216. Hrycushko BA, Li S, Shi C, Goins B, Liu Y, Phillips WT, et al. Postlumpectomy focal brachytherapy for simultaneous treatment of surgical cavity and draining lymph nodes. Int J Radiat Oncol Biol Phys. (2011) 79:948–55. doi: 10.1016/j.ijrobp.2010.05.062
217. Hrycushko BA, Li S, Goins B, Otto RA, Bao A. Direct intratumoral infusion of liposome encapsulated rhenium radionuclides for cancer therapy: effects of nonuniform intratumoral dose distribution. Med Phys. (2011) 38:1339–47. doi: 10.1118/1.3552923
218. Hrycushko BA, Ware S, Li S, Bao A. Improved tumour response prediction with equivalent uniform dose in pre-clinical study using direct intratumoural infusion of liposome-encapsulated 86Re radionuclides. Phys Med Biol. (2011) 56:5721–34. doi: 10.1088/0031-9155/56/17/016
219. Chang CM, Lan KL, Huang WS, Lee YJ, Lee TW, Chang CH, et al. 188Re-liposome can induce mitochondrialautophagy and reverse drug resistance for ovarian cancer: from bench evidence to preliminary clinical proof-of-concept. Int J Mol Sci. (2017) 18:903. doi: 10.3390/ijms18050903
220. Liepe K, Zaknun JJ, Padhy A, Barrenechea E, Soroa V, Shrikant S, et al. Radiosynovectomy using yttrium-90, phosphorus-32 or rhenium-188 radiocolloids versus corticoid instillation for rheumatoid arthritis of the knee. Ann Nucl Med. (2011) 25:317–23. doi: 10.1007/s12149-011-0467-1
221. Jeong JM, Lee YJ, Kim EH, Chang YS, Kim YJ, Son M, et al. Preparation of 188Re-labeled paper for treating skin cancer. Appl Radiat Isot. (2003) 58:551–5. doi: 10.1016/S0969-8043(03)00063-0
222. Bhusari P, Shukla J, Kumar M, Vatsa R, Chhabra A, Palarwar K, et al. Noninvasive treatment of keloid using customized Re-188 skin patch. Dermatol Ther. (2017) 30:e12515. doi: 10.1111/dth.12515
223. Shukla J, Mittal BR. 188Re Tailor Made Skin Patch for the treatment of skin cancers and keloid: overview and technical considerations. Int J Nucl Med Res. (2017) 107–113. doi: 10.15379/2408-9788.2017.10
224. Sedda AF, Rossi G, Cipriani C, Carrozzo AM, Donati P. Dermatological high-dose-rate brachytherapy for the treatment of basal and squamous cell carcinoma. Clin Exp Dermatol. (2008) 33:745–9. doi: 10.1111/j.1365-2230.2008.02852.x
225. Cipriani C, Frisch B, Scheidhauer K, Desantis M. Personalized high-dose-rate brachytherapy with non-sealed rhenium-188 in non-melanoma skin cancer. Int J Nucl Med Res. (2017) 114–22. doi: 10.15379/2408-9788.2017.11
226. Carrozzo AM, Sedda AF, Muscardin L, Donati P, Cipriani C. Dermo beta brachytherapy with 188Re in squamous cell carcinoma of the penis: a new therapy. Eur J Dermatol. (2013) 23:183–8. doi: 10.1684/ejd.2013.1927
Keywords: bone pain palliation, oncology, peptides, radioembolization, radionuclide therapy, radiopharmaceuticals, Rhenium-188
Citation: Lepareur N, Lacœuille F, Bouvry C, Hindré F, Garcion E, Chérel M, Noiret N, Garin E and Knapp FFR Jr (2019) Rhenium-188 Labeled Radiopharmaceuticals: Current Clinical Applications in Oncology and Promising Perspectives. Front. Med. 6:132. doi: 10.3389/fmed.2019.00132
Received: 31 January 2019; Accepted: 29 May 2019;
Published: 14 June 2019.
Edited by:
Francesco Cicone, Lausanne University Hospital (CHUV), SwitzerlandReviewed by:
Ekaterina Dadachova, University of Saskatchewan, CanadaAdriano Duatti, University of Ferrara, Italy
Copyright © 2019 Lepareur, Lacœuille, Bouvry, Hindré, Garcion, Chérel, Noiret, Garin and Knapp. This is an open-access article distributed under the terms of the Creative Commons Attribution License (CC BY). The use, distribution or reproduction in other forums is permitted, provided the original author(s) and the copyright owner(s) are credited and that the original publication in this journal is cited, in accordance with accepted academic practice. No use, distribution or reproduction is permitted which does not comply with these terms.
*Correspondence: Nicolas Lepareur, n.lepareur@rennes.unicancer.fr