- 1School of Pharmacy and Biomedical Sciences, Faculty of Health Sciences, Curtin University, Perth, WA, Australia
- 2Curtin Health and Innovation Research Institute, Curtin University, Perth, WA, Australia
Aging is associated with an increased incidence of cancer. One contributing factor could be modulation of immune cells responsible for anti-tumor responses, such as dendritic cells (DCs) and T cells. These immunological changes may also impact the efficacy of cancer immunotherapies in the elderly. The effects of healthy aging on DCs and T cells, and their impact on anti-mesothelioma immune responses, had not been reported. This study examined DCs and T cells in young (2–5 months; equivalent to 16–26 human years) and elderly (20–24 months; equivalent to 60–70 human years) healthy and mesothelioma-bearing C57BL/6J mice. During healthy aging, elderly lymph nodes adopted a regulatory profile, characterized by: (i) increased plasmacytoid DCs, (ii) increased expression of the adenosine-producing enzyme CD73 on CD11c+ cells, and (iii) increased expression of multiple regulatory markers (including CD73, the adenosine A2B receptor, CTLA-4, PD-1, ICOS, LAG-3, and IL-10) on CD8+ and CD4+ T cells, compared to lymph nodes from young mice. Although mesotheliomas grew faster in elderly mice, the increased regulatory status observed in healthy elderly lymph node DCs and T cells was not further exacerbated. However, elderly tumor-bearing mice demonstrated reduced MHC-I, MHC-II and CD80 on CD11c+ cells, and decreased IFN-γ by CD8+ and CD4+ T cells within tumors, compared to young counterparts, implying loss of function. An agonist CD40 antibody based immunotherapy was less efficient at promoting tumor regression in elderly mice, which may be due to: (i) failure of elderly CD8+ T cells to up-regulate perforin, and (ii) increased expression of multiple regulatory markers on CD11c+ cells and T cells in elderly tumor-draining lymph nodes (including CD73, PD-1, ICOS, LAG-3, and TGF-β). Our findings suggest that checkpoint blockade may improve responses to immunotherapy in elderly hosts with mesothelioma, and warrants further investigation.
Introduction
There is an increased incidence of cancer with aging, and one contributing factor could be age-induced modulation of immune cells that mediate anti-tumor immune responses, such as dendritic cells (DCs) and T cells. DCs are antigen-presenting cells that play an important role in initiating anti-tumor immune responses, on account of their ability to activate tumor-specific CD8+ cytotoxic T cells, which can directly kill tumor cells and mediate tumor regression (1–5). There is some evidence that aging influences DC anti-tumor function, with DCs from elderly mice shown to have a reduced ability to stimulate tumor-specific cytotoxic CD8+ T cells in vivo (6, 7). Furthermore, administration of DC vaccines to elderly tumor-bearing mice leads to generation of weak cytotoxic T cell activity, and does not slow tumor growth, resulting in a shorter survival time (8, 9). Age-related defects in murine T cell anti-tumor function have also been reported, these include; reduced numbers of tumor-antigen-specific T cells, decreased proliferative capacity, impaired cytotoxic activity, and reduced production of effector cytokines, such as interferon (IFN)-γ and IL-2, in elderly tumor-bearing mice (10–18). However, the effects of healthy aging on DCs and T cells, and the potential impact on generation of anti-tumor immune responses in mesothelioma, an asbestos-induced cancer which occurs predominantly in elderly populations aged 60 years and above (19, 20), have not yet been reported.
Furthermore, age-related changes in DCs and T cells may impact on the efficacy of cancer immunotherapies in the elderly. The few studies performed to-date that have considered aging indicate that cancer immunotherapies are less effective in elderly hosts (6, 8, 9, 11, 21–25). Little is known about the effects of aging on responses to immunotherapy in mesothelioma. Our previous studies, using young mice (1.5–2 months of age, equivalent to 16–26 human years), have shown that intra-tumoral administration of IL-2 in combination with agonist anti-CD40 antibody (IL-2/CD40) induces permanent regression of large AE17 mesothelioma tumors mediated by CD8+ T cells, neutrophils (26), B cells (27) and pro-inflammatory M1 macrophages (28). Cured mice remained tumor-free for the remainder of their natural lives and were protected from tumor re-challenge by CD8+ and CD4+ T cells and natural killer cells (29, 30). Studies from our laboratory have also shown that elderly macrophages activated with in vitro IL-2 and agonist anti-CD40 antibody restore the capacity of elderly CD8+ T cells to produce IFN-γ and perforin (31, 32). Here, we extend these studies to investigate the influence of aging on DC and T cell function during treatment with IL-2/CD40 in vivo.
The aim of this study was to examine the influence of aging on DC and T cell function in healthy mice vs. mice with mesothelioma, and to examine responses to IL-2/CD40 immunotherapy in elderly and young mesothelioma-bearing mice. We compared DCs and T cells from young (2–5 months; equivalent to 16–26 human years) (33) and elderly C57BL/6J mice (20–24 months; equivalent to 60–70 human years) (33, 34) in: (i) lymph nodes of healthy mice, and (ii) tumor-draining lymph nodes and tumors of mesothelioma-bearing mice, with and without IL-2/CD40 immunotherapy. We examined the effects of aging on DC and T cell subset proportions, as well as expression of activation/effector and regulatory markers.
Materials and Methods
Mice
This project was approved by the Curtin University Animal Ethics Committee (approval number AEC-2012-21), and all experiments were performed according to the Australian Code of Practice for the care and use of animals for scientific purposes. Young (2–5 months; equivalent to 16–26 human years) (33) and elderly (20–24 months; equivalent to 60–70 human years) (33, 34) female C57BL/6J mice were obtained from the Animal Resources Center (Western Australia, Australia) and maintained under specific pathogen-free conditions in the Curtin University animal facility. For studies involving healthy mice, any mice that had a palpable mass, enlarged lymph nodes, enlarged spleen, or enlarged liver were excluded to ensure that only healthy, non-tumor-bearing mice were examined.
Murine Mesothelioma Cell Line Culture
AE17 is a murine mesothelioma cell line derived from the peritoneal cavity of C57BL/6J mice injected with asbestos fibers, as previously described (35). AE17sOVA was developed by transfecting the AE17 parental cell line with cDNA coding for secretory ovalbumin (OVA), hence ovalbumin, which contains the SIINFEKL peptide, functions as a marker tumor antigen, as previously described (35). AE17 cells were cultured in complete medium, consisting of RPMI 1640 media (Invitrogen, California, USA), supplemented with 10% fetal calf serum (FCS; ThermoScientific, Victoria, Australia), 100 units/ml of penicillin and 100 μg/ml streptomycin (Penicillin-Streptomycin; Life Technologies, Victoria, Australia) and 2 mM L-glutamax (Life Technologies). AE17sOVA cells were maintained in complete medium supplemented with 400 μg/L of the neomycin analog G418 (Geneticin; Life Technologies, Grand Island, USA). All cells were cultured at 37°C in a 5% CO2 atmosphere.
Murine Tumor Growth and Treatment With IL-2/CD40 Immunotherapy
For tumor inoculation, mice were injected subcutaneously in the right flank with 5 x 105 AE17 or AE17sOVA tumor cells per mouse, in 100 μl of PBS. Tumor growth was monitored and tumor size was measured daily using microcallipers. The maximum tumor size allowed was 140 mm2 in accordance with ethics approval from the Curtin University Animal Ethics Committee.
For immunotherapy, AE17 or AE17sOVA tumor-bearing mice were treated intra-tumorally once every 2-3 days with PBS diluent (100 μl/dose) or interleukin-2 (IL-2, 20 μg/dose; Cetus Corporation, California, USA) and agonist anti-CD40 antibody (FGK45, 40 μg/dose; Absolutions, Western Australia, Australia) as previously described (26), for 5 doses in total.
Processing and Staining Murine Tissues for Flow Cytometric Analysis
Mice were euthanized using methoxyflurane (Medical Developments International, Victoria, Australia). Spleens and lymph nodes were collected from healthy (non-tumor-bearing) mice, and tumors and tumor-draining lymph nodes were collected from AE17 tumor-bearing mice for flow cytometric analysis. For samples that required intracellular cytokine staining, murine tissues were collected into FACS buffer [1 × PBS/1% newborn calf serum (NCS)/1% bovine serum albumin (BSA)] + 2.5 μg/ml brefeldin A (Sigma), and incubated for 4 h prior to staining. Lymph nodes and tumors were disaggregated into single-cell suspensions by gentle dispersion between two frosted glass slides.
Single-cell suspensions of murine lymph nodes and tumors were stained with fluorescently-labeled antibodies to identify DC subsets (CD11c, CD11b, CD8, CD4, B220, and GR-1; Table 1), T cell subsets (CD3, CD8, CD4, CD25, and FoxP3; Table 1), and activation (MHC-I, MHC-II, CD40, CD80, CD86, IFN-γ, IL-12, TNF-α, and/or perforin; Table 1) and regulatory markers (CD39, CD73, A2AR, A2BR, PD-L1, GAL-9, IL-10, TGF-β latency-associated peptide, ICOS, LAG-3, CTLA-4, and/or PD-1; Table 1).
Samples were incubated with surface primary antibodies (diluted in FACS buffer + 2.5 μg/ml brefeldin A) for 30 min at 4°C in the dark, and washed twice in FACS buffer + 2.5 μg/ml brefeldin A by centrifuging at 1,200 rpm for 2 min. Samples stained with purified A2BR antibody or biotinylated primary antibodies (B220, CD11c, CD39, and PD-1) were incubated with goat anti-rabbit IgG-AF488 (Invitrogen) or streptavidin-V500 (BD), respectively (diluted in FACS buffer + 2.5 μg/ml brefeldin A), for 30 min at 4°C in the dark. Samples were washed twice in FACS buffer + 2.5 μg/ml brefeldin A, followed by two PBS washes, then stained with Zombie green or Zombie NIR viability dyes (both from Biolegend) for 15 min at 4°C in the dark, washed twice in FACS buffer, then twice in PBS. Cells were fixed in 1% paraformaldehyde (Sigma) diluted in PBS by incubating for 20 min at 4°C in the dark, washed twice with FACS buffer, and permeabilised with FACS buffer + 0.1% saponin (Sigma) for 15 min at 4°C in the dark. For samples requiring FoxP3 staining, cells were fixed using FoxP3 Fix/Perm buffer (Biolegend), permeabilised using FoxP3 Perm buffer (Biolegend), then stained with FoxP3 antibody (1 μl/well) for 30 min at 4°C in the dark, washed twice in FACS buffer + 0.1% saponin, then stained with other intracellular antibodies. Cells were stained with intracellular primary antibodies (diluted in FACS buffer + 0.1% saponin) for 30 min at 4°C in the dark, then washed twice in FACS buffer. Samples stained with TGF-β-biotin were incubated with streptavidin-V500 (diluted in FACS buffer + 0.1% saponin) for 30 min at 4°C in the dark, followed by two washes using FACS buffer, and resuspended in 200 μl of FACS buffer per well. Samples were stored at 4°C in the dark (up to a maximum of 1 week) before analysis on a FACSCanto II using FACSDiva software (BD) or FlowJo software (TreeStar, USA).
In vivo Cytotoxic T Lymphocyte (CTL) Assay for Analysis of CTL Function
The cytotoxic activity of tumor-specific CD8+ T cells was assessed via an in vivo CTL assay, as previously described (27). Briefly, target cells for this assay were derived from spleen and lymph node cells from healthy young C57BL/6J mice. Spleen and lymph node cell suspensions were RBC-lysed, washed and divided into two populations. One population was pulsed with 10−6 M SIINFEKL peptide for 90 min at 37°C, washed with PBS, and labeled with a high concentration (5 μm) of carboxyfluorescein succinimidyl ester (CFSE; Molecular Probes, Oregon, USA). Control target cells (i.e., not pulsed with peptide) were labeled with a low concentration of CFSE (0.5 μm). 107 cells from each population were pooled in 200 μl PBS and intravenously injected into each recipient AE17sOVA-bearing young or elderly mouse. Tumor-draining lymph nodes and tumors were collected from young and elderly recipient mice 24 h after target cell injection, and the number of cells in each target cell population in each tissue measured by flow cytometry. The ratio between the percentages of unpulsed vs. SIINFEKL-pulsed cells (CFSElo/CFSEhigh) was calculated to obtain a numerical value of cytotoxicity. To normalize data, allowing inter-experimental comparisons, ratios between the percentages of peptide-pulsed cells in control (tumor-free mice and tumor-bearing PBS-treated mice) vs. age-matched tumor-bearing mice were calculated.
Data Analysis
Statistical differences between young and elderly populations were calculated using a Mann-Whitney U-test, using GraphPad PRISM v7 (GraphPad Software Inc, USA). P < 0.05 were considered statistically significant.
Results
AE17 Mesothelioma Tumors Grow Faster in Elderly Mice
We first investigated whether aging influenced AE17 mesothelioma tumor growth in young (2–5 months) vs. elderly (20–24 months) C57BL/6J mice, and found that tumors grew faster in elderly mice (Figure 1A) (36). One possibility to account for faster tumor growth is that aging programs a more suppressive immune environment that is reflected by DCs and T cells.
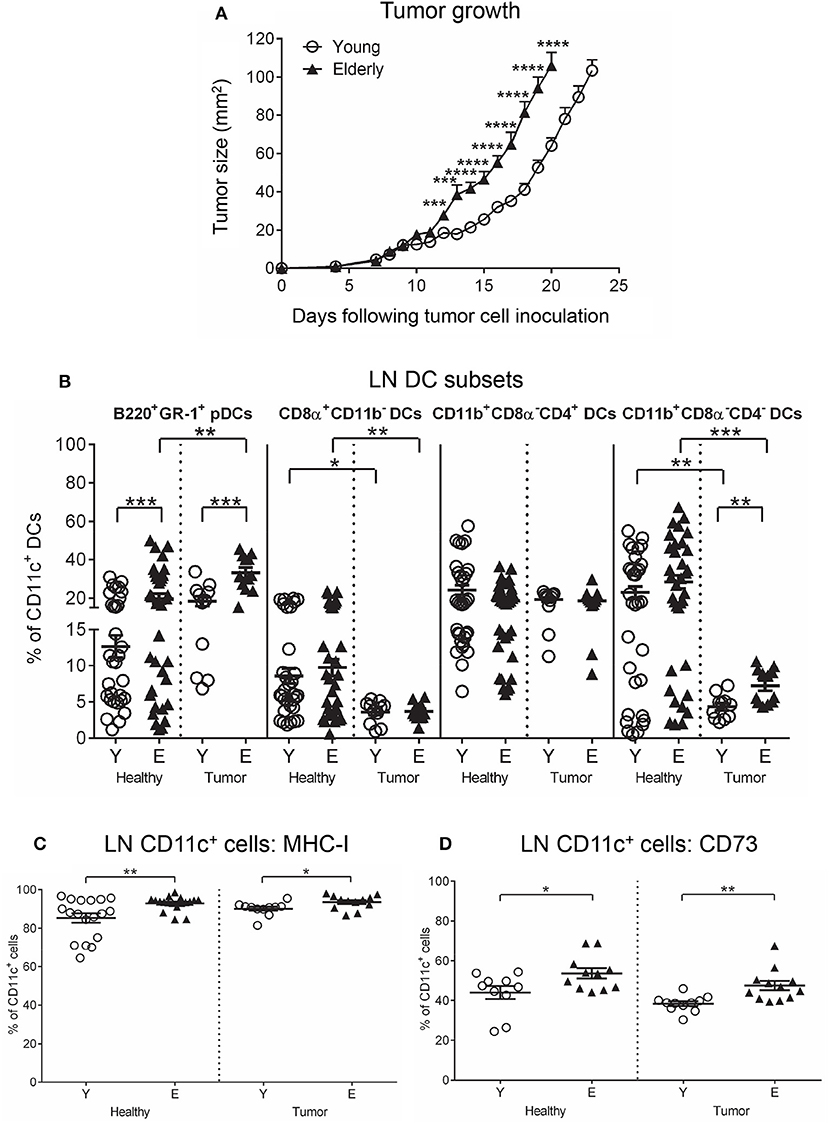
Figure 1. AE17 mesothelioma tumors grow faster in elderly mice. Young (n = 13) and elderly (n = 15) mice were inoculated subcutaneously with 5 × 105 AE17 tumor cells per mouse, and tumor size (mm2) measured daily (A); data shown as mean ± SEM. At end point, TDLNs from the same tumor-bearing mice and LNs from age-matched healthy mice (n = 32 young and 35 elderly mice) were analyzed for DC subsets (B), MHC-I+ CD11c+ cells (C) and CD73+ CD11c+ cells (D) using flow cytometry. Data are shown for individual mice as well as mean ± SEM. Mouse numbers for B-D differed on account of varying cell numbers available for analysis and ranged from n = 11–13 young and n = 12–15 elderly tumor-bearing mice, n = 10–32 young and n = 11–35 elderly healthy mice, *p < 0.05, **p < 0.005, ***p < 0.0005, ****p < 0.0001 comparing (i) young to elderly mice, or (ii) tumor-bearing mice to age-matched healthy mice.
Cross-Presenting DCs Decrease and pDCs Increase in Elderly Tumor-Bearing Lymph Nodes
To determine if aging reprograms suppressive DCs, we compared overall CD11c+ cells (as these antigen presenting cells represent DCs) and well-described DC subsets in lymph nodes (LNs) and tumor-draining LNs (TDLNs) from young to elderly healthy and AE17 mesothelioma-bearing mice using flow cytometry (Supplementary Figures S1A–S1F).
Neither age nor the presence of a tumor significantly affected TDLN/LN CD11c+ cell proportions (Supplementary Figure S1G). However, examination of DC subsets showed that mesothelioma significantly decreased cross presenting CD8α+CD11b− cDC (Figure 1B) and T helper 2 (Th2) cell-activating CD11b+CD8α−CD4− cDC proportions (Figure 1B) in TDLNs compared to LNs from age-matched healthy mice. In contrast, pDC proportions already increased in healthy elderly LNs were further elevated in the presence of a tumor (Figure 1B). No age-related or tumor-induced changes were observed in CD11b+CD8α−CD4+ cDC proportions (Figure 1B). These data imply increased suppression due to loss of cross-presenting DCs alongside increased pDCs that are suppressive in cancer (37).
Regulatory CD73+CD11C+ DCs Increase in Elderly Healthy and Tumor-Bearing Lymph Nodes
To investigate whether antigen-presenting cell function is altered with aging and mesothelioma, markers of antigen presentation (MHC-I and II), activation (CD40, CD80, CD86, intracellular IFN-γ, tumor necrosis factor (TNF)-α and IL-12) and regulation (CD39, CD73, A2A receptor (A2AR), A2B receptor (A2BR), programmed cell death ligand-1 (PD-L1), galectin-9 (GAL-9), intracellular IL-10, and transforming growth factor (TGF)-β latency-associated peptide) were compared on CD11c+ cells from young and elderly mice using flow cytometry.
Healthy elderly LNs contained elevated proportions of MHC-I+CD11c+ cells and CD11c+ cells co-expressing the suppressive adenosine-producing enzyme CD73, compared to their younger counterparts (Figures 1C,D) that remained unchanged in the presence of a tumor in TDLNs (Figures 1C,D). However, mesothelioma induced age-related differences in TDLNs, including elevated proportions of IL-12+ and IFN-γ+ CD11c+ cells in elderly TDLNs (Supplementary Figure S1H); this is despite the observation that, regardless of age, mesothelioma reduced IL-12+, IFN-γ+, and TNF-α+ CD11c+ cell proportions in young and elderly TDLNs, compared to age-matched healthy LNs (Supplementary Figure S1H). These data imply overall loss of pro-inflammatory function with progressing mesothelioma. In contrast, mesothelioma increased MHC-II+ and CD80+CD11c+ cells in TDLNs, relative to age-matched healthy LNs (Supplementary Figure S2A). Expression of CD80 can be a dual-edged sword as it can ligate CD28 on T cells leading to T cell activation, or it can ligate cytotoxic T lymphocyte antigen-4 (CTLA-4) leading to T cell tolerance. Mesothelioma increased PD-L1 expression on young CD11c+ cells to the same levels as seen in healthy and tumor-bearing elderly DCs (Supplementary Figure S2B). No other age- or tumor-induced changes were seen on LN/TDLN CD11c+ cells (Supplementary Figures S2A–C).
Elderly T Cells Increase Expression of Suppressive CD73, A2BR, CTLA-4, PD-1, ICOS, LAG-3, and IL-10
As a key function of DCs is activation or tolerization of T cell responses, we examined the effects of aging and mesothelioma on T cells in LNs/TDLNs from young and elderly mice (Supplementary Figures S3A–E). CD4+ T cell, CD8+ T cell and regulatory T cell (Treg) proportions reduced in elderly healthy LNs and TDLNs, compared to young LNs/TDLNs (Supplementary Figure S3F). Tumors reduced TDLN CD4+ T cell proportions in both age groups (Supplementary Figure S3F), compared to healthy LNs, yet did not affect TDLN CD8+ T cell and Treg proportions (Supplementary Figure S3F).
To investigate function, T cells were stained for markers of activation (CD25, intracellular IFN-γ and perforin), and regulation (CTLA-4, programmed cell death protein-1 (PD-1), inducible T cell co-stimulator (ICOS), lymphocyte activation gene-3 (LAG-3), CD39, CD73, A2AR, A2BR, and intracellular IL-10 and TGF-β latency-associated peptide) and analyzed via flow cytometry. Elderly healthy LN and TDLN CD8+ and CD4+ T cells significantly increased expression of several regulatory markers, including CD39, CD73, A2BR, CTLA-4, PD-1, ICOS, LAG-3, IL-10, and TGF-β, compared to their younger counterparts (Figures 2A,B, 3A,B). Note that the presence of a tumor did not alter healthy age-related increases in regulatory markers on elderly LN/TDLN T cells (Figures 2A,B, 3A,B). This suggests that the aging environment induces LN T cells with suppressive, rather than effector, function.
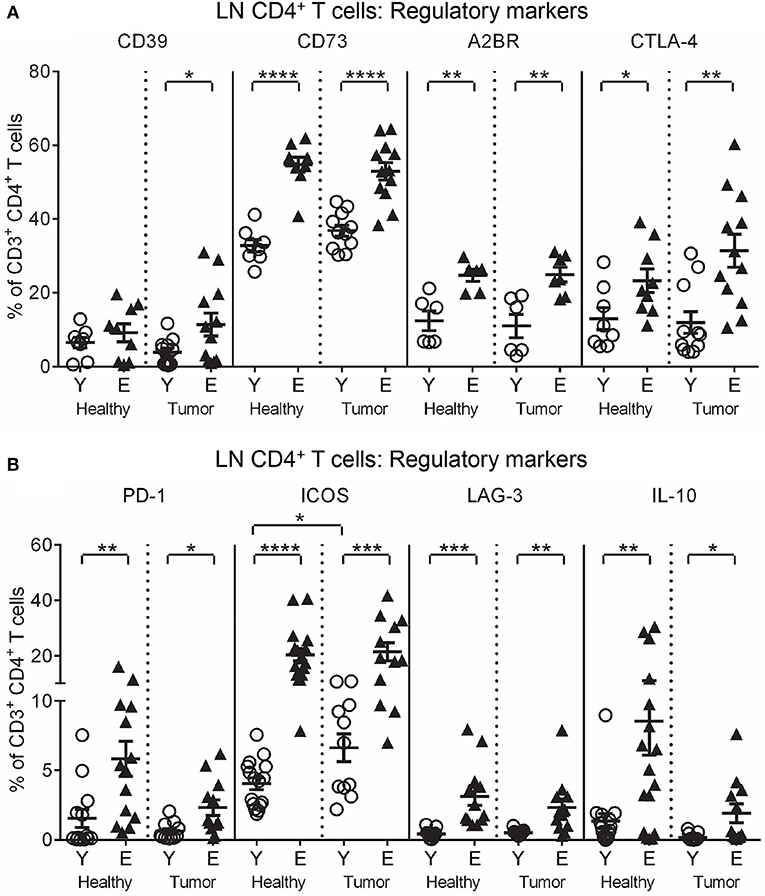
Figure 2. Increased regulatory markers on elderly LN/TDLN CD4+ T cells. Percentages of CD4+ T cells positive for regulatory markers were measured in TDLNs from the same mice shown in Figures 1A–D young and elderly tumor-bearing mice, and LNs from age-matched healthy young and elderly mice (A,B), via flow cytometry. Data are shown for individual mice as well as as mean ± SEM, n = 6–11 young and n = 7–12 elderly tumor-bearing mice, n = 6–17 young and n = 6–17 elderly healthy mice, *p < 0.05, **p < 0.005, ***p < 0.0005, ****p < 0.0001 comparing (i) young to elderly mice, or (ii) tumor-bearing mice to age-matched healthy mice.
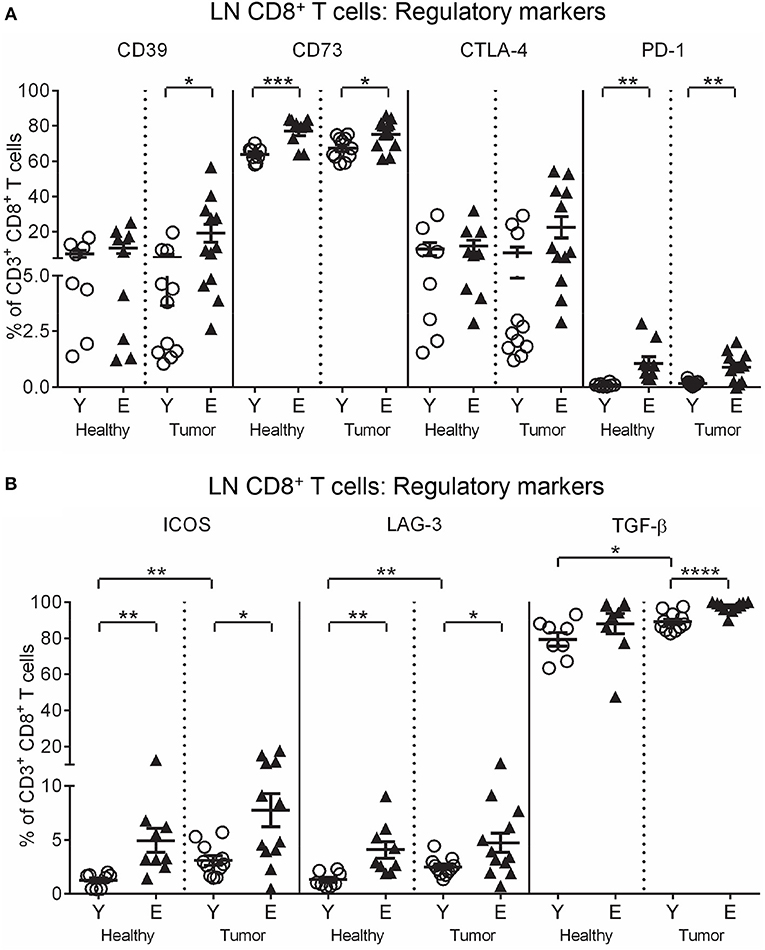
Figure 3. Increased regulatory markers on elderly LN/TDLN CD8+ T cells. Percentages of CD8+ T cells positive for regulatory markers were measured in TDLNs from young and elderly tumor-bearing mice, and LNs from age-matched healthy young and elderly mice, as per Figures 1, 2 (A, B), via flow cytometry. Data are shown for individual mice as well as mean ± SEM, n = 11 young and n = 12 elderly tumor-bearing mice, n = 8 young and n = 9 elderly healthy mice, *p < 0.05, **p < 0.005, ***p < 0.0005, ****p < 0.0001 comparing (i) young to elderly mice, or (ii) tumor-bearing mice to age-matched healthy mice.
The data above and summarized in Table 2 shows that LNs adopt a regulatory profile with healthy aging, characterized by increased: (i) pDCs, (ii) CD73 on CD11c+ DCs, and (iii) multiple regulatory markers on T cells. Furthermore, although pro-inflammatory CD11b+CD8α−CD4− cDCs and IFN-γ+ and IL-12+ CD11c+ DC proportions were elevated in elderly TDLNs, suggesting increased T cell-activating potential, this is undermined by concomitant maintenance of age-related increases in suppressive DCs and T cells.
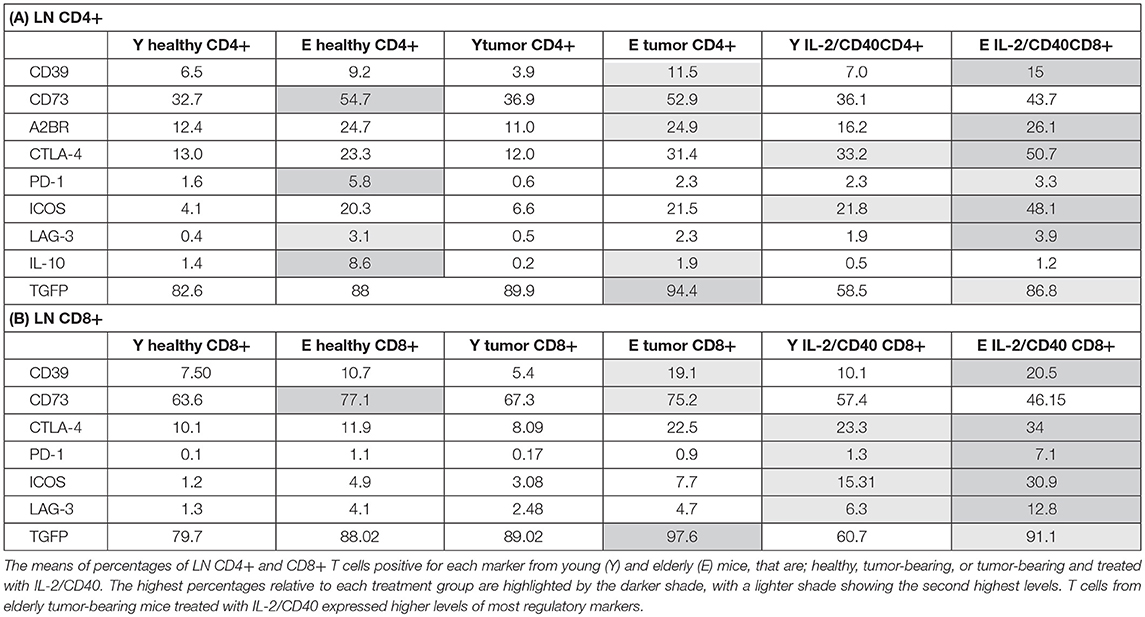
Table 2. Changes to regulatory molecules on LN CD4+ (A) and LN CD8+ (B) T cells with age and IL-2/CD40.
The Elderly Tumor Microenvironment Reduces MHC-I/II and CD80 on DCs and IFN-γ in T Cells.
We next investigated the tumor microenvironment and found that elderly tumors contained significantly lower levels of Th1-activating CD11b+CD8α−CD4+ cDCs compared to young tumors (Figure 4A). No age-related differences were seen in the other DC subsets (Supplementary Figure S4A). Elderly tumor-associated CD11c+ cells significantly reduced MHC-II and CD80 (Figure 4B), with a trend for reduced MHC-I (p = 0.08; Figure 4B) relative to their younger counterparts. In contrast, TNF-α+CD11c+ cells increased in elderly, compared to young, tumors (Supplementary Figure S4B). Examination of regulatory markers showed that IL-10+CD11c+ cells increased (Supplementary Figure S4C), whilst CD39+, PD-L1+, and TGF-β+ CD11c+ cells decreased in elderly tumors (Supplementary Figure S4C). These data suggest that aging induces deficits in elderly tumor-infiltrating CD11c+ cells leading to reduced antigen-presenting capacity and increased IL-10-mediated suppression.
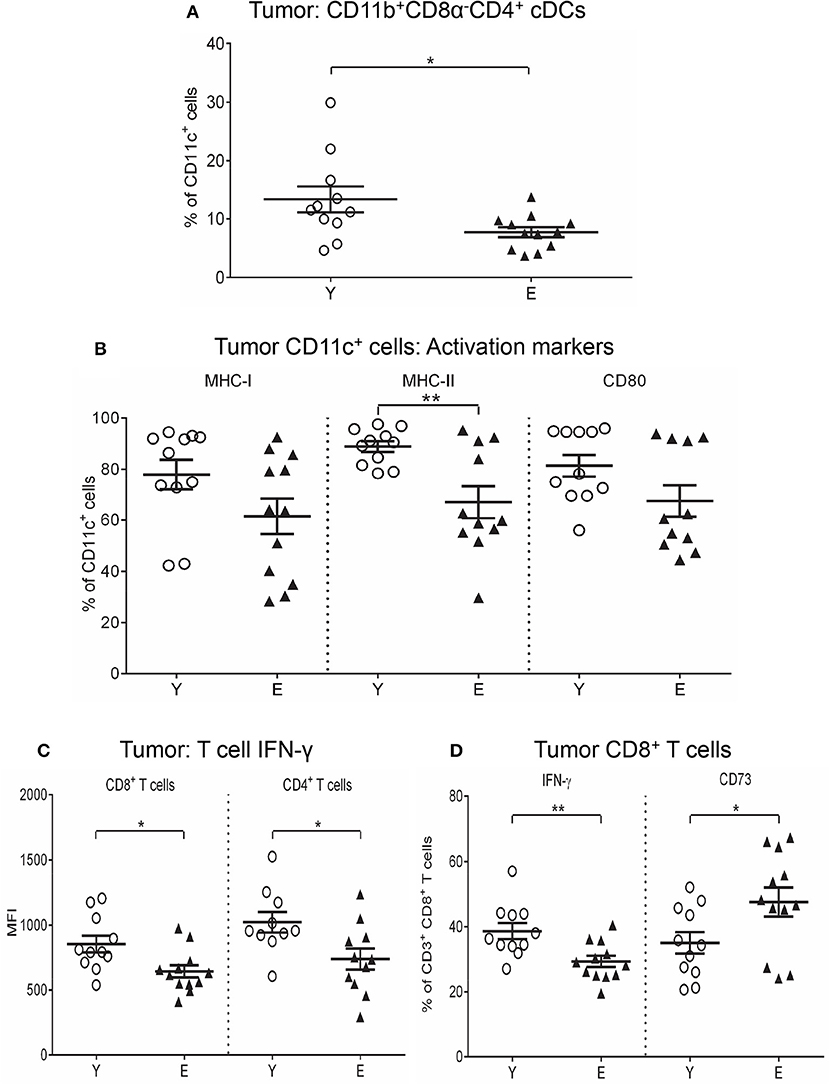
Figure 4. Reduced CD11b+CD8α−CD4+ cDCs, MHC-I/II, and CD80 on CD11c+ cells, and IFN-γ on T cells in elderly tumors. Young and elderly tumors as per Figures 1, 2 were analyzed using flow cytometry for percentages of CD11b+CD8α−CD4+ cDCs (A), percentages of CD11c+ cells positive for MHC-I/II and CD80 (B), IFN-γ expression (shown as geometric mean fluorescence intensity; MFI) on CD8+ and CD4+ T cells (C), and percentages of CD8+ T cells positive for IFN-γ and CD73 (D). Data are shown for individual mice as well as mean ± SEM, n = 11 young and n = 12 elderly tumor-bearing mice, *p < 0.05, **p < 0.005 comparing young to elderly mice.
An investigation of tumor-infiltrating T cells showed that CD4+ T cells and Tregs were significantly decreased in elderly mice (Supplementary Figure S5A), whilst CD8+ T cells were similar with age (Supplementary Figure S5A). Reduced IFN-γ was seen in CD8+ (Figures 4C,D) and CD4+ T cells (Figure 4C) in elderly tumors compared to young tumors implying reduced anti-tumor effector T cell activity with age. Examination of regulatory markers showed increased CD73+CD8+ T cells (Figure 4D), yet reduced TGF-β+CD8+ T cells (Supplementary Figure S5B), ICOS+CD4+ T cells (Supplementary Figure S5C) and TGF-β+CD4+ T cells (Supplementary Figure S5C) in elderly, compared to young tumors (data also summarized in Table 2), implying different regulatory age-related mechanisms.
IL-2/Agonist Anti-CD40 Immunotherapy Is Less Effective in Elderly Mice
Our previous studies demonstrated that IL-2/CD40 immunotherapy induces complete and permanent tumor regression in young mice (26, 29, 30). Herein, we investigated the efficacy of IL-2/CD40 treatment in elderly tumor-bearing mice. Young and elderly AE17 mesothelioma-bearing C57BL/6J mice were treated with IL-2/CD40, using previously described doses (26). A complete schedule of IL-2/CD40 (i.e., 5 doses) was less effective in elderly mice (45% tumor regression; Figure 5A), compared to young mice (100% tumor regression; Figure 5A) (36). Tumors and TDLNs were sampled after mice received one-third of the treatment schedule (i.e., two doses of IL-2/CD40; Figure 5A), to enable identification of tumors responding to treatment and still have enough tumor sample to collect for analysis, as young mice given the full regimen demonstrate complete resolution of tumors which therefore cannot be sampled (26, 29, 30). After two doses, IL-2/CD40 slowed tumor growth rates in both age groups, although the effects in terms of tumor size (Figure 5B) and weights (Figure 5C) were more pronounced in young mice, relative to PBS diluent controls.
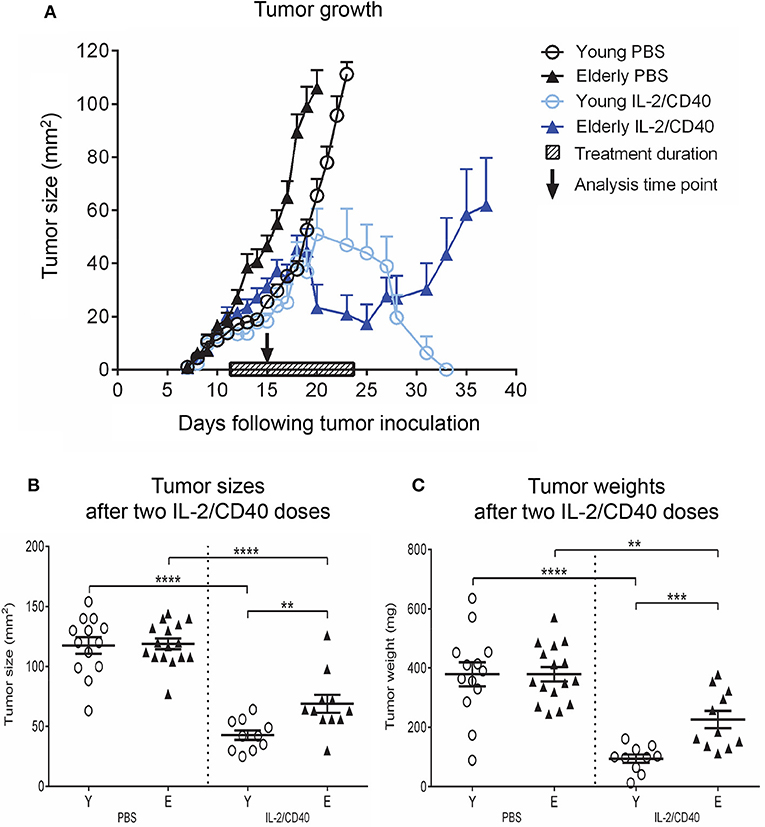
Figure 5. IL-2/CD40 is less efficient at inducing tumor regression in elderly mice. Young (n = 24) and elderly (n = 27) mice were inoculated subcutaneously with 5 × 105 AE17 cells, and tumors left to develop before treatment with intra-tumoral IL-2 (20 μg/dose) and agonist anti-CD40 antibody, 40 μg/dose; n = 10 young and 11 elderly mice or PBS diluent (n = 14 young and 16 elderly mice; 100 μl/dose) for 5 doses, each 2–3 days apart. The shaded bar in (A) represents treatment duration. Tumor size (in mm2) was measured to determine tumor growth rates and response to IL-2/CD40 (A); data shown as mean ± SEM. In a second experiment TDLNs and tumors were collected for analysis 2–3 days after the second dose, indicated by the black arrow in (A), and tumor sizes (B) and tumor weights (C) in n = 10 young and 11 elderly mice given IL-2/CD40 and n = 14 young and 16 elderly mice given PBS measured; data are shown for individual mice as well as mean ± SEM, mouse numbers for C differed on account of varying cell numbers available for analysis and ranged from n = 10–13 young and n = 8–16 elderly PBS control mice, **p < 0.005, ***p < 0.0005, ****p < 0.0001 comparing (i) young to elderly mice, or (ii) IL-2/CD40-treated mice to age-matched PBS control mice.
IL-2/CD40 Reduces Potential Licensing of Tumor-Specific T Cells by Increasing CD73 and TGF-β and Reducing CD40 on Elderly but not Young TDLN DCs
Examination of DCs in IL-2/CD40-treated mice showed that regardless of age CD11b+CD8α−CD4+ cDCs and CD11b+CD8α−CD4− cDCs (Supplementary Figure S6A) increased relative to controls, suggesting increased capacity to stimulate Th1 and Th2 responses. No significant changes were seen in the other DC subsets (Supplementary Figure S6A).
In both age groups, IL-2/CD40 exerted positive and negative effects on activation/maturation markers on TDLN CD11c+ cells: i.e., increased CD80 (Figure 6A) and CD86 (Supplementary Figure S6B), yet reduced IFN-γ, TNF-α and IL-12 (Supplementary Figure S6B), relative to age-matched PBS controls. As a result of these changes, elderly IL-2/CD40-treated mice expressed lower levels of CD80 on CD11c+ cells compared to their younger counterparts (Figure 6A), whilst CD86, IFN-γ, TNF-α, and IL-12 expression levels were similar for the two age groups (Supplementary Figure S6B). Importantly, IL-2/CD40 reduced expression of a number of regulatory markers on young and elderly TDLN CD11c+ cells including: CD73 (Figure 6A), TGF-β (Figure 6A), CD39 (Supplementary Figure S6C) and IL-10 (Supplementary Figure S6C), relative to age-matched untreated tumor-bearing mice. Nonetheless, CD73 (Figure 6A) and TGF-β (Figure 6A) remained higher on elderly CD11c+ cells in IL-2/CD40-treated mice. This suggests that whilst IL-2/CD40 reduces tumor-induced suppressive TDLN CD11c+ cells, it does not overcome the age-related increase in suppressive CD11c+ cells, which may be a contributing factor to the reduced efficacy of IL-2/CD40 in elderly mice.
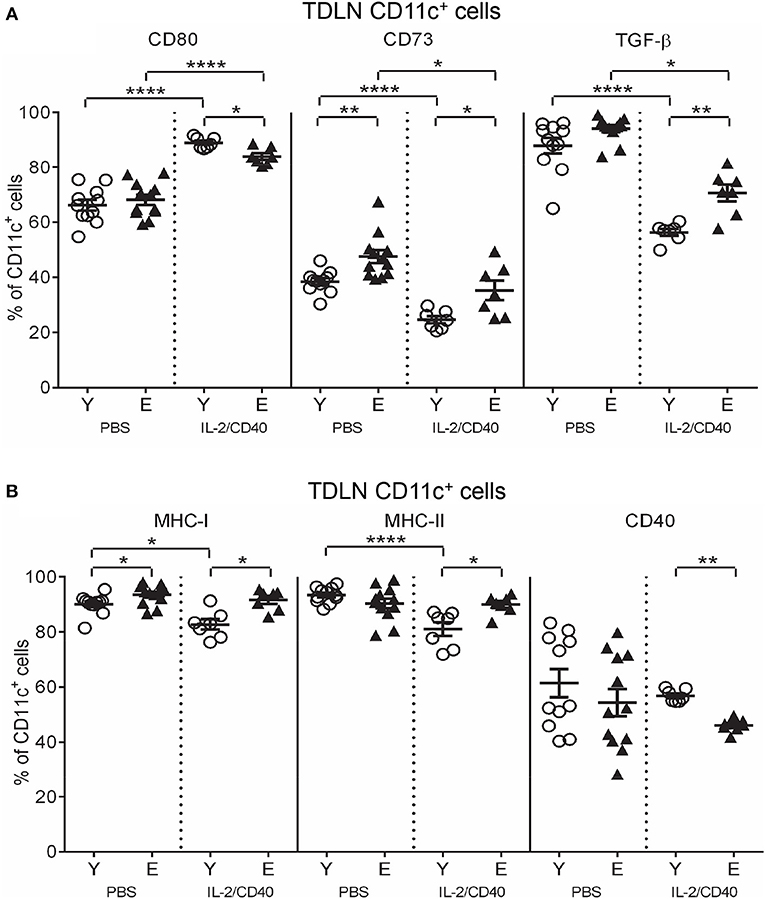
Figure 6. IL-2/CD40 increases MHC-I/II, CD80, CD73, and TGF-β on TDLN CD11c+ cells. After two doses of IL-2/CD40 or PBS diluent, percentages of CD11c+ cells positive for CD80, CD73, and TGF-β (A) and MHC-I, MHC-II,. and CD40 (B) were measured in TDLNs from the young and elderly tumor-bearing mice described in Figures 5B,C using flow cytometry. Data are shown as mean ± SEM, n = 7 young and n = 7 elderly IL-2/CD40-treated mice, n = 11 young and n = 12 elderly PBS control mice, *p < 0.05, **p < 0.005, ****p < 0.0001 comparing (i) young to elderly mice, or (ii) IL-2/CD40-treated mice to age-matched PBS control mice.
Some age-specific effects were also seen. Young, but not elderly, IL-2/CD40-treated mice down-regulated MHC-I (Figure 6B) and MHC-II (Figure 6B) on DCs, relative to age-matched PBS controls resulting in an increased age-related differential (Figure 6B). In contrast, CD40 on TDLN CD11c+ cells reduced (Figure 6B) in elderly, compared to young, IL-2/CD40-treated mice. Taken together, whilst CD11c+ cells from elderly IL-2/CD40-treated mice have increased capacity to present antigens to T cells, their ability to license tumor-specific T cells appears compromised, relative to young counterparts.
IL-2/CD40 Further Exacerbates the Regulatory Status of Elderly TDLN T Cells
The effects of IL-2/CD40 on CD8+ and CD4+ T cells and Tregs in TDLNs and tumors were also examined. IL-2/CD40 reduced TDLN CD4+ T cell proportions in young and elderly mice, relative to age-matched PBS controls (Supplementary Figure S7A). Unexpectedly, young IL-2/CD40-treated mice showed a reduction in TDLN CD8+ T cell proportions relative to their PBS controls (Supplementary Figure S7A).
IL-2/CD40 exerted positive and negative effects on regulatory marker expression on TDLN CD8+ T cells in both age groups, including increased PD-1, ICOS, and LAG-3 (Figure 7A), compared to age-matched PBS controls. Nonetheless, age-related differences in PD-1, ICOS, and LAG-3 were maintained, whereby elderly CD8+ T cells had higher expression (Figure 7A). In contrast, IL-2/CD40 induced reduced CD73 and TGF-β expression on CD8+ T cells (Figure 7B) in young and elderly TDLNs, relative to age-matched PBS controls. However, IL-2/CD40-treated young mice demonstrated a striking reduction in TGF-β, meaning elderly mice still expressed significantly higher TGF-β (Figure 7B). Overall, elderly TDLN CD8+ T cells maintain increased suppressive function with IL-2/CD40 treatment.
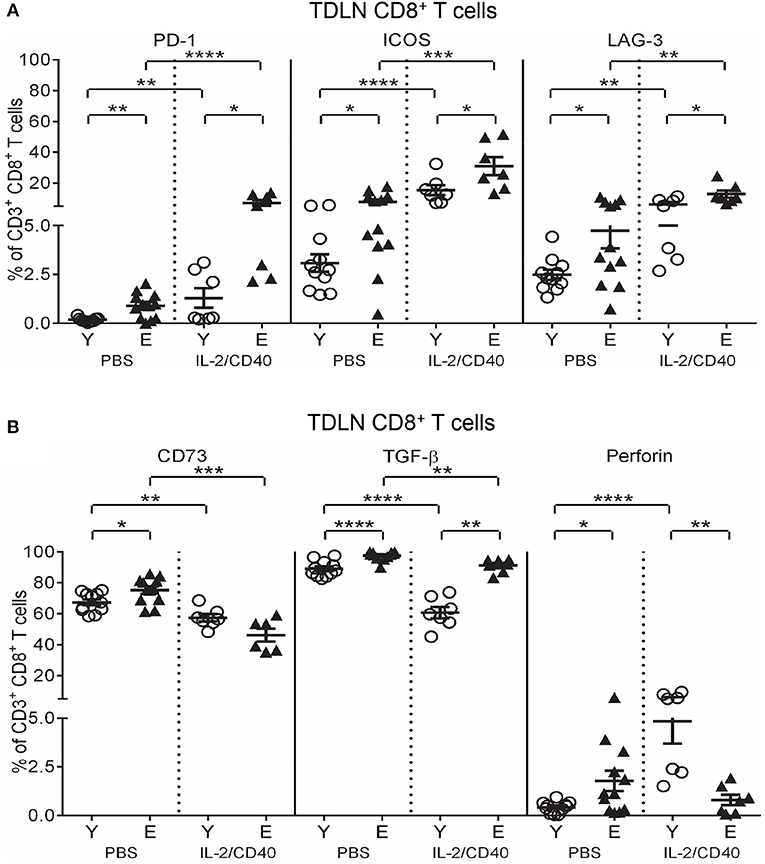
Figure 7. IL-2/CD40 increases PD-1, ICOS, LAG-3, CD73, and TGF-β on TDLN CD8+ T cells. After two doses of IL-2/CD40 or PBS diluent, percentages of CD8+ T cells positive for PD-1, ICOS, and LAG-3 (A) and CD73, TGF-β and perforin (B) were measured in TDLNs from the young and elderly tumor-bearing mice described in Figures 5B and C using flow cytometry. Data are shown as mean ± SEM, n = 7 young and n = 6–7 elderly IL-2/CD40-treated mice, n = 11 young and n = 12 elderly PBS control mice, *p < 0.05, **p < 0.005, ***p < 0.0005, ****p < 0.0001 comparing (i) young to elderly mice, or (ii) IL-2/CD40-treated mice to age-matched PBS control mice.
Interestingly, IL-2/CD40 exerted age-specific effects on TDLN CD8+ T cell perforin expression, with young mice up-regulating perforin expression (Figure 7B), resulting in higher expression compared to elderly IL-2/CD40-treated mice (Figure 7B). This suggests that in addition to their increased regulatory status, elderly TDLN CD8+ T cells have reduced cytotoxic effector activity due to a failure to up-regulate perforin in response to IL-2/CD40.
IL-2/CD40 also exerted common effects on several regulatory markers on TDLN CD4+ T cells from both age groups including increased CTLA-4 and ICOS and decreased TGF-β expression (Figure 8A), compared to age-matched PBS controls. Despite these changes, expression of CTLA-4, ICOS, and TGF-β remained higher on elderly TDLN CD4+ T cells compared to young IL-2/CD40-treated mice (Figure 8A).
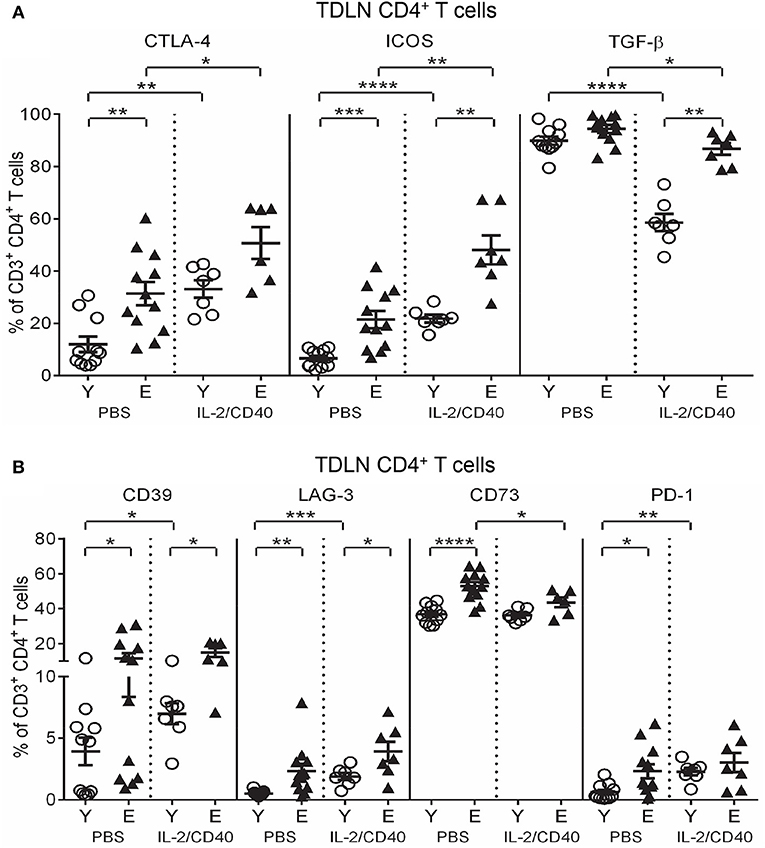
Figure 8. IL-2/CD40 increases CTLA-4, ICOS, TGF-β, CD39, and LAG-3 on young and elderly TDLN CD4+ T cells. After two doses of IL-2/CD40 or PBS diluent, percentages of CD4+ T cells positive for CTLA-4, ICOS and TGF-β (A) and CD39, LAG-3, CD73, and PD-1 (B) were measured in TDLNs from the young and elderly tumor-bearing mice described in Figures 5B,C using flow cytometry. Data are shown as mean ± SEM, n = 7 young and n = 6–7 elderly IL-2/CD40-treated mice, n = 11 young and n = 12 elderly PBS control mice, *p < 0.05, **p < 0.005, ***p < 0.0005, ****p < 0.0001 comparing (i) young to elderly mice, or (ii) IL-2/CD40-treated mice to age-matched PBS control mice.
Age-specific effects of IL-2/CD40 on TDLN CD4+ T cells were also seen. Elderly, but not young, IL-2/CD40-treated mice reduced CD4+ T cells expressing CD73 (Figure 8B) relative to controls. CD4+ T cells from young, but not elderly, IL-2/CD40-treated mice up-regulated CD39, LAG-3, and PD-1 (Figure 8B), compared to their PBS controls however, this did not alter the age differentials seen in CD39 and LAG-3 in untreated mice, with expression of these markers remaining higher on elderly CD4+ T cells (Figure 8B); data summarized in Tables 2. Taken together, elderly TDLN CD4+ T cells also demonstrate an enhanced regulatory status during IL-2/CD40 treatment, which may contribute to the reduced efficacy of IL-2/CD40 in elderly mice.
IL-2/CD40 Increases Suppressive TGF-β and A2BR and Reduces CD40 on DCs in Elderly Tumors
IL-2/CD40 mostly did not alter young or elderly tumor-associated DC subset proportions, relative to untreated mice, with the exception of a small, but significant, increase in cross-presenting CD8α+CD11b− cDCs in elderly tumors (Supplementary Figure S7B). The only common effect of IL-2/CD40 on tumor-associated CD11c+ cells seen in both age groups was up-regulation of IFN-γ (Figure 9A).
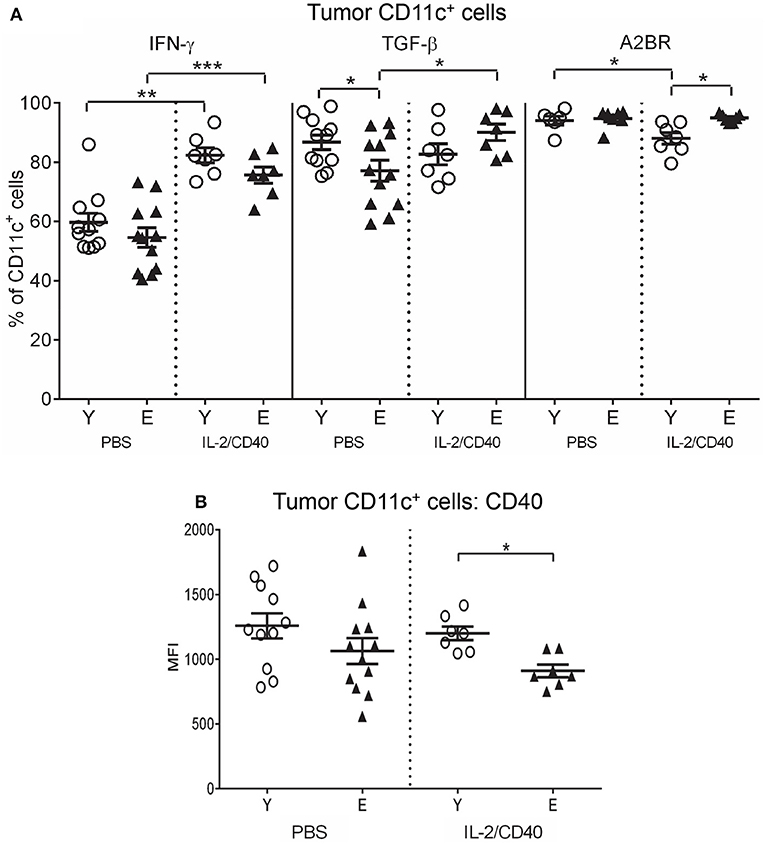
Figure 9. Increased IFN-γ, TGF-β, and A2BR, and reduced CD40 on CD11c+ cells from elderly IL-2/CD40-treated tumors. After two doses of IL-2/CD40 or PBS diluent, percentages of CD11c+ cells positive for IFN-γ, TGF-β and A2BR (A) and expression levels of CD40 (shown as geometric mean fluorescence intensity; MFI) on CD11c+ cells (B) were measured from the young and elderly tumor-bearing mice described in Figures 5B,C using flow cytometry. Data are shown as mean ± SEM, n = 7 young and n = 7 elderly IL-2/CD40-treated mice, n = 6–11 young and n = 7–12 elderly PBS control mice, *p < 0.05, **p < 0.005, ***p < 0.0005 comparing (i) young to elderly mice, or (ii) IL-2/CD40-treated mice to age-matched PBS control mice.
IL-2/CD40 induced several age-specific changes on tumor-associated CD11c+ cells including increased TGF-β on elderly DCs (Figure 9A). In contrast, IL-2/CD40 induced reduced MHC-I and CD80 (Supplementary Figure S7C) in young, but not elderly, tumor-associated CD11c+ cells. Concurrently, IL-2/CD40 reduced expression of several regulatory markers on young CD11c+ cells including: CD39 (Supplementary Figure S7C), A2AR (Supplementary Figure S7D), A2BR (Figure 9A) and PD-L1 (Supplementary Figure S7D), yet expression of CD73 increased (Supplementary Figure S7D), relative to PBS controls. However, elderly CD11c+ cells demonstrated reduced CD40 (Figure 9B) and increased A2BR (Figure 9A) relative to young CD11c+ cells. Taken together, this suggests that IL-2/CD40 alleviates suppressive CD11c+ cells in young, but not elderly tumors, likely contributing to reduced tumor responses to IL-2/CD40 by elderly mice.
IL-2/CD40 Reduces IFN-γ and Perforin on Elderly Tumor-Infiltrating CD8+ T Cells
Examination of tumor-associated T cells showed that IL-2/CD40 reduced young, but not elderly, CD4+ T cell (Supplementary Figure S8A) and Treg proportions (Supplementary Figure S8A), leaving elevated Treg proportions in elderly IL-2/CD40-treated tumors (Supplementary Figure S8A), implying increased suppressive activity in elderly tumors.
Regardless of age, IL-2/CD40 up-regulated CD25 on tumor-associated CD8+ T cells (Figure 10A) relative to PBS controls, this increase was more striking in young mice (Figure 10A).
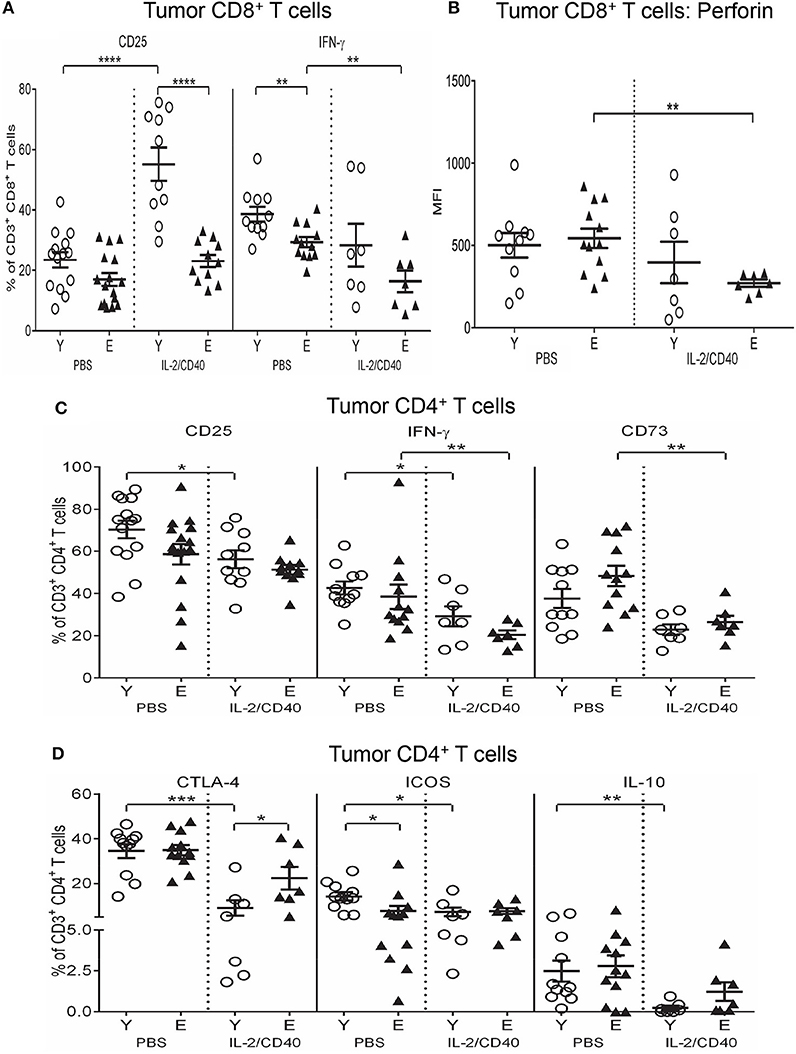
Figure 10. Reduced CD25, IFN-γ and perforin on CD8+ T cells and increased CTLA-4 on CD4+ T cells in elderly IL-2/CD40-treated tumors. After two doses of IL-2/CD40 or PBS diluent, percentages of CD8+ T cells positive for CD25 and IFN-γ (A), expression levels of perforin (shown as geometric mean fluorescence intensity; MFI) on CD8+ T cells (B), and percentages of CD4+ T cells positive for CD25, IFN-γ and CD73 (C) and CTLA-4, ICOS and IL-10 (D) were measured in tumors from the young and elderly tumor-bearing mice described in Figures 5B,C using flow cytometry. Data are shown as mean ± SEM, n = 7–10 young and n = 7–11 elderly IL-2/CD40-treated mice, n = 11–14 young and n = 12–16 elderly PBS control mice, *p < 0.05, **p < 0.005, ***p < 0.0005, ****p < 0.0001 comparing (i) young to elderly mice, or (ii) IL-2/CD40-treated mice to age-matched PBS control mice.
Several age-specific effects of IL-2/CD40 were also observed. In elderly IL-2/CD40-treated tumors, IFN-γ+CD8+ T cells (Figure 10A) and perforin levels on CD8+ T cells (Figure 10B) reduced compared to controls. This was accompanied by reductions in tumor-associated CD8+ T cells expressing CD73 (Supplementary Figure S8B) and IL-10 (Supplementary Figure S8B) in elderly, but not young, IL-2/CD40-treated mice, relative to PBS controls. Overall, despite the observation that IL-2/CD40 alleviates certain suppressive functions in elderly tumor-infiltrating CD8+ T cells, these cells also demonstrated reduced anti-tumor effector function.
Unexpectedly, IL-2/CD40 promoted a more regulatory phenotype in young tumor-associated CD8+ T cells, on account of increases in several regulatory markers, relative to PBS controls, specifically: CD39, A2AR, A2BR, ICOS, LAG-3, and PD-1 (Supplementary Figures S8B,C). As a result, expression of CD39, A2AR, A2BR, ICOS and LAG-3 (Supplementary Figures S8B,C) was higher on tumor-associated CD8+ T cells from young, compared to elderly, IL-2/CD40-treated mice. These data may reflect transition of young CD8+ T cells to the attenuation phase of the immune response following IL-2/CD40 stimulation.
IL-2/CD40 Reduces CTLA-4, ICOS and IL-10-Mediated Suppressive Potential in Young, but not Elderly, Tumor-Infiltrating CD4+ T Cells
IL-2/CD40 reduced CD25 (Figure 10C) and IFN-γ (Figure 10C) in tumor-associated CD4+ T cells in both age groups alongside reductions in the regulatory markers CD73 (Figure 10C) and CTLA-4 (Figure 10D). The reduction in CTLA-4 was more striking for young mice (Figure 10D). Furthermore, young, but not elderly, IL-2/CD40-treated mice demonstrated additional reductions in other regulatory markers expressed by tumor-associated CD4+ T cells, including ICOS and IL-10 (Figure 10D). Reduction of several layers of suppression in young tumor-associated CD4+ T cells may help account for the improved tumor response seen in young mice to IL-2/CD40 treatment.
Reduced Tumor-Specific CTL Activity in Elderly TDLNs and Tumors Is Not Restored by IL-2/CD40
The data above suggests elderly CD8+ T cells have reduced effector function and increased suppressive function, therefore we assessed the cytolytic capacity of young vs. elderly tumor-specific CD8+ T cells in TDLNs and tumors after two doses of IL-2/CD40 using an in vivo CTL assay. This assay uses AE17sOVA-bearing young and elderly mice, where ovalbumin acts as a marker tumor antigen, enabling measurement of antigen-specific CTL responses against SIINFEKL, the dominant peptide of ovalbumin. SIINFEKL-pulsed and unpulsed control target cells were injected into young and elderly AE17sOVA-bearing mice treated with PBS diluent or IL-2/CD40. 24 h later LNs and tumors were removed and lysis of SIINFEKL-labeled target cells measured to provide an indication of tumor-specific CTL activity. Significantly reduced SIINFEKL-specific lytic activity was observed in TDLNs (Figure 11A) and tumors (Figure 11B) of elderly IL-2/CD40-treated and PBS control mice, relative to their younger counterparts (36). IL-2/CD40 induced increased trends for CTL activity in young TDLNs (p = 0.08; Figure 11A) and tumors (p = 0.1; Figure 11B) whilst no increase in CTL activity was observed in elderly IL-2/CD40-treated mice, relative to PBS controls (Figures 11A,B). The age-related reduction in tumor-specific CTL function in untreated (PBS) elderly tumor-bearing mice may account for the faster tumor growth rate seen in elderly mice. Poorer CTL responses in elderly mice is likely to contribute to their reduced tumor responses to IL-2/CD40-treatment.
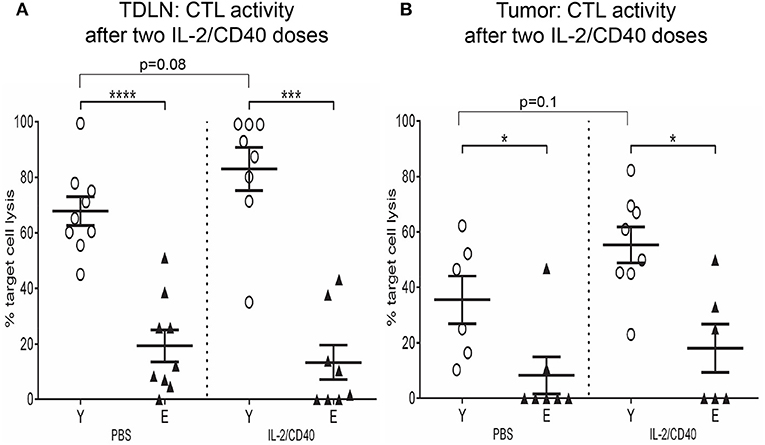
Figure 11. Reduced tumor-specific CTL activity in elderly TDLNs and tumors. In a further experiment the cytotoxic activity of tumor-specific CD8+ CTLs was assessed after two doses of IL-2/CD40 or PBS diluent in TDLNs (A) and tumors (B) of young (n = 17) and elderly (n = 17) AE17sOVA-bearing mice using an in vivo CTL assay. SIINFEKL-pulsed and unpulsed target cells were injected into tumor-bearing mice, and ratios between percentages of the two target cell populations in TDLNs and tumors measured 18 h later via flow cytometry. Data are shown for individual mice as well as mean ± SEM, mouse numbers differed on account of varying cell numbers available for analysis and ranged from n = 8 young and n = 6–8 elderly IL-2/CD40-treated mice, n = 6–9 young and n = 7–9 elderly PBS control mice, *p < 0.05, ***p < 0.0005, ****p < 0.0001 comparing young to elderly mice.
Discussion
Our study shows that aging programs development of a suppressive immune environment, which is reflected by DCs and T cells, and this may compromise generation of anti-tumor immune responses, influence mesothelioma progression and responses to IL-2/CD40 immunotherapy. We observed establishment of a suppressive environment within healthy elderly LNs, evidenced by increased regulatory pathways on DCs and T cells. This regulatory LN environment was maintained in TDLNs of elderly mesothelioma-bearing mice. As TDLNs are the main site of DC/T cell interactions leading to generation of anti-tumor effector T cell responses, increased suppressive mechanisms in elderly TDLNs likely led to compromised generation of anti-mesothelioma effector T cell responses, thereby contributing to the faster tumor growth rate observed in the elderly.
Elevated pDCs in elderly LNs/TDLNs represents one suppressive pathway that is likely to negatively affect elderly anti-tumor immune responses, as pDCs have been associated with immune suppression in cancer (37). There are several mechanisms by which pDCs induce immune suppression, including: (i) inducing Th1 cell anergy and apoptosis via indoleamine 2,3-dioxygenase (IDO) (38), (ii) inhibiting Th1 cell proliferation via granzyme B (39), and (iii) promoting suppressive Treg expansion via IDO (40, 41) and/or expression of ICOS ligand (ICOS-L), which binds ICOS expressed on Tregs, leading to Treg activation and IL-10 secretion (42–45). In turn, Tregs mediate suppression within LNs, by inhibiting effector CD8+ and CD4+ T cells, and promoting regulatory function in DCs (46). Thus, the age-related increase in LN/TDLN pDCs likely compromises the development of effective anti-mesothelioma immune responses in the elderly.
Age-related increases in several regulatory markers on LN/TDLN CD11c+ cells and T cells represents a further mechanism that contributes to a suppressive environment in the elderly. As the outcome of a T cell response depends on the summation of positive and negative signals it receives (47), age-related increases in regulatory molecules will skew the balance in favor of negative signals, leading to elderly T cell suppression, rather than activation. LN/TDLN CD11c+ cells demonstrated simultaneous increases in antigen-presenting (MHC-I), co-stimulatory (CD80, IL-12, and IFN-γ) and regulatory (CD73 and PD-L1) molecules with aging. Therefore, whilst antigen presentation to T cells may be maintained with aging, it is likely to be accompanied by an increased breadth of negative signals. The strength of a signal delivered by a molecule is determined by interaction with its ligand, and although we observed increased CD80 expression on elderly LN CD11c+ cells, others have reported that expression of its ligand, CD28, is reduced on elderly T cells (48–51), suggesting reduced T cell activation in the elderly. In this scenario, CD80 may instead play a role in inhibiting T cell responses during aging. This study, and others (52, 53), showed increased CTLA-4 expression on elderly T cells; ligation of CD80 and CTLA-4 negatively regulates T cell activation (54). Furthermore, CD80 on DCs can also bind PD-L1 on T cells, leading to T cell inhibition (55, 56), and others have shown that PD-L1 expression is increased on aged CD8+ T cells (57, 58). Thus, elevated CD80 on elderly CD11c+ cells may lead to T cell suppression.
The PD-L1/PD-1 pathway represents another mechanism by which T cell responses may be suppressed during aging. In agreement with others, this study observed increased PD-L1 expression on elderly LN CD11c+ cells (57), and increased PD-1 expression on elderly LN/TDLN T cells (52, 53, 57, 59–63), suggesting that the likelihood of inhibitory PD-L1/PD-1 interactions is increased with aging. The outcomes of this include inhibition of T cell proliferation, reduced effector T cell differentiation, reduced cytokine production and cytotoxic function, as well as increased T cell apoptosis (64–67), thereby compromising elderly effector T cell responses.
In addition, the age-related increase in LAG-3 expression on T cells, also observed in another study (62), may lead to increased negative signaling through the MHC-II/T cell receptor complex (68), and could contribute to the impaired ability of elderly DCs to prime CD4+ T cells and Th1 responses, described by others (7, 69, 70).
Increased CD73 on elderly CD11c+ cells represents another mechanism of T cell suppression as it indicates increased capacity to produce immunosuppressive adenosine, particularly if neighboring cells express CD39 (71), such as the concomitant increase in CD39 expression we observed on elderly TDLN T cells. Elderly T cells also had increased CD73, suggesting they contribute to the generation of adenosine. Moreover, elderly CD4+ T cells in LNs/TDLNs have an increased capacity to respond to adenosine due to elevated expression of the adenosine-binding A2B receptor. Adenosine-mediated signaling deactivates T cells by impairing IL-2 production (72, 73) and inducing Tregs (74, 75). Taken together, our data suggests that with aging, negative signals outweigh positive signals during DC/T cell interactions in elderly LN/TDLNs, leading to suppression of tumor-specific effector T cells. This is supported by our observation that CTLs in elderly TDLNs displayed reduced tumor antigen-specific cytolytic activity relative to their younger counterparts. Taken together, these changes suggest diminished anti-mesothelioma immune responses in the elderly, contributing to faster tumor progression.
The age-induced up-regulation of several inhibitory markers on LN/TDLN T cells not only suggests an increased capacity to respond to negative signals from DCs, but also implies that elderly T cells are functionally exhausted. In agreement with others, we observed that elderly CD8+ and CD4+ T cells had increased PD-1, CTLA-4, ICOS, LAG-3, and CD39 expression (52, 53, 57, 59–63, 76, 77), with simultaneous up-regulation of checkpoint inhibitory markers (particularly PD-1, CTLA-4, LAG-3, TIM-3, and CD39) being a hallmark of exhausted T cells (78, 79). This regulatory phenotype was accompanied by reduced lytic effector function in elderly CD8+ T cells. T cell exhaustion has been described in cancer (80–82) and is a state in which T cells are unable to proliferate, produce lower levels of cytokines such as IL-2 and IFN-γ, have diminished cytotoxic activity and effector function, and cannot generate memory responses (78, 83). The development of an exhausted phenotype in aged T cells is supported by studies showing that PD-1-expressing elderly T cells are dysfunctional, as they have reduced proliferative capacity (53, 57, 59, 63). Thus, aging-induced development of functionally exhausted T cells with diminished anti-tumor effector activity represents another potential mechanism contributing to faster mesothelioma tumor growth in the elderly.
Examination of CD11c+ cells and T cells within tumors provided further evidence that a suppressive immune environment develops during aging. CD11c+ cells in elderly tumors may have reduced ability to present antigens and stimulate CD8+ and CD4+ T cells on account of decreased MHC-I and II, and CD80. Generation of anti-tumor Th1 responses within elderly tumors may be further compromised due to reduced CD4+ T cells and CD11b+CD8α−CD4+ cDCs whose main role is activation of Th1 responses (84, 85). A reduction in the ability of elderly CD11c+ cells to stimulate anti-tumor effector T cells is consistent with other studies showing that elderly bone marrow-derived DCs are less efficient in stimulating tumor antigen-specific CD8+ and CD4+ T cells and mediating tumor regression (6, 7), and that administration of DC vaccines to elderly mice results in weak cytotoxic CD8+ T cell activity and does not slow tumor growth (8, 9). In addition, elderly tumor-associated CD11c+ cells may be further skewed toward suppressive function due to increased IL-10 and TNF-α. Production of IL-10 by DCs can lead to T cell inhibition and induction of Tregs (86, 87), and TNF has recently been shown to promote CD4+ T cell exhaustion in chronic viral infection (88). Changes in elderly tumor-associated CD11c+ cells suggest that the outcome of cross-talk between CD11c+ cells and T cells in tumors is likely to be skewed toward generation of suppressive T cells. This is supported by this study showing that elderly tumor-associated CD8+ and CD4+ T cells had reduced IFN-γ, implying reduced effector activity. Furthermore, elderly tumor-associated CD8+ CTLs also demonstrated compromised capacity to mediate tumor antigen-specific cell lysis and killing. Our observations agree with other studies showing that T cells in elderly tumor-bearing hosts are dysfunctional due to impaired cytotoxic activity and reduced production of IFN-γ and IL-2 (10–18). Additionally, our data suggest that elderly tumor-infiltrating CD8+ T cells are skewed toward suppressive function, due to increased CD73, suggesting increased potential to generate adenosine. Our parallel studies have also shown that elderly mesothelioma tumors contain increased tumor-associated macrophages (Duong et al. under review) which represent another layer of immune suppression. Thus, the elderly tumor microenvironment represents another site of increased immune suppression, which may disable local anti-tumor immune responses contributing to faster mesothelioma growth.
The increased regulatory status of elderly DCs and T cells may not only affect tumor progression, but also the efficacy of anti-tumor immunotherapies, which is supported by our data showing that IL-2/CD40 immunotherapy is less effective in inducing mesothelioma regression in elderly mice. IL-2/CD40 slowed tumor growth in both age groups (completely in young, partially in elderly) and this may be explained by our observations that IL-2/CD40 induced several common effects on tumor-infiltrating CD11c+ cells, CD8+ T cells, and CD4+ T cells from both age groups. Elevated IFN-γ expression by CD11c+ cells, and increased CD25 expression by CD8+ T cells suggests these cells are more activated with increased effector function and contribute to IL-2/CD40-induced slowing of tumor growth. This is supported by studies from our laboratory showing that CD8+ T cells are important anti-tumor effector cells during IL-2/CD40 treatment (26, 29), and other studies showing that DCs, CD8+ T cells and IFN-γ are required for IL-2/CD40-mediated tumor regression (89–92). Additionally, reduced CD25, CD73, and CTLA-4 on tumor-infiltrating CD4+ T cells in both age groups suggest reduced Treg-mediated suppression within IL-2/CD40-treated tumors. This is supported by other studies showing that IL-2/CD40 alleviates Treg suppression in murine tumors (89, 93). Importantly, IL-2/CD40 also reduced expression of several regulatory markers on young and elderly TDLN CD11c+ cells (CD39, CD73, TGF-β, and IL-10), suggesting alleviation of adenosine, TGF-β and IL-10-mediated suppression in TDLNs, which may create a more permissive environment for generation of IL-2/CD40-induced effector T cell responses. Collectively, these changes may contribute to the IL-2/CD40-induced slowing of tumor growth observed in both age groups.
Nonetheless, IL-2/CD40 was less effective in elderly tumor-bearing mice, which could be attributed to an exacerbated suppressive environment in elderly TDLNs. Although MHC-I and II increased on TDLN CD11c+ cells in elderly IL-2/CD40-treated mice, suggesting increased antigen presentation to T cells, this is likely to be accompanied by negative co-stimulatory signals, due to concomitant reductions in CD40 and CD80, and the observation that IL-2/CD40 did not overcome age-related increases in suppressive CD73 and TGF-β on elderly CD11c+ cells. Moreover, elderly TDLN CD8+ and CD4+ T cells may be more suppressive/exhausted and more responsive to negative signals from antigen-presenting cells, as age-related increases in CTLA-4, ICOS, TGF-β, A2AR, CD39, LAG-3, and/or PD-1 were further enhanced during IL-2/CD40 treatment. Further evidence for reduced generation of effector T cells in TDLNs of IL-2/CD40-treated elderly mice comes from the observation that elderly TDLN CD8+ T cells failed to up-regulate perforin and antigen-specific cytolytic effector function in response to IL-2/CD40, unlike their younger counterparts; this shows that anti-tumor cytotoxic effector T cell responses are compromised in the elderly. As previous studies from our laboratory have shown that CD8+ T cells and perforin-associated effector function are important for IL-2/CD40-mediated tumor regression in young mice (26), this likely contributes to poorer tumor responses by elderly mice to IL-2/CD40.
IL-2/CD40 alleviated suppression in young, but not elderly tumors, which also accounts for the better responses of young mice to IL-2/CD40 immunotherapy. Specifically, IL-2/CD40 reduced expression of several regulatory molecules (CD39, A2AR, A2BR, PD-L1, ICOS, and/or IL-10) on young tumor-infiltrating CD11c+ cells and CD4+ T cells, suggesting reduced suppressive activity. In contrast, CD11c+ cells and T cells infiltrating elderly IL-2/CD40-treated tumors were characterized by an increased regulatory and reduced effector status. Elderly CD11c+ cells displayed reduced CD40 expression, suggesting a reduced ability to license T cells (94, 95). This was associated with: (i) increased A2BR, implying an increased capacity of CD11c+ cells to respond to adenosine, leading to induction of tolerogenic/inhibitory DCs (96–98), and (ii) increased TGF-β, via which DCs suppress effector T cells and promote Tregs (99, 100); the latter is supported by elevations in Treg proportions and CTLA-4 expression on CD4+ T cells in elderly IL-2/CD40-treated tumors. Moreover, elderly tumor-associated CD8+ T cells demonstrated decreased perforin and IFN-γ implying reduced effector function; this is supported by our observation that elderly tumor-associated CTLs failed to up-regulate lytic function in response to IL-2/CD40, and represents another mechanism which reduces the efficacy of IL-2/CD40-mediated tumor regression in the elderly (26, 89, 90, 92). As the age-related differences in TDLN and tumor-infiltrating CD11c+ cells and T cells reported in this study were observed after one-third of the usual IL-2/CD40 schedule, further studies are required to examine CD11c+ cells/DCs and T cells after a complete schedule of IL-2/CD40.
Collectively, our data suggests that checkpoint blockade of inhibitory molecules may improve responses to IL-2/CD40 immunotherapy in elderly hosts with mesothelioma. In particular, blockade of multiple inhibitory molecules (CD39, CD73, A2A, and A2B receptors, PD-L1, PD-1, CTLA-4, LAG-3, ICOS, and TGF-β) will help reduce the likelihood of negative cross-talk during DC/T cell interactions in elderly TDLNs and tumors, thereby permitting activation of anti-tumor effector T cells. A few recent studies have reported promising results for elderly melanoma, non-small cell lung cancer and renal cancer patients treated with CTLA-4 or PD-1 blockade, with elderly patients demonstrating similar survival benefits and ability to tolerate treatments, compared to younger patients (101–104), this supports further investigation of checkpoint blockade as a therapeutic avenue for elderly mesothelioma patients.
In conclusion, our study has shown that aging programs a suppressive immune environment in healthy elderly LNs, characterized by increased suppressive pDCs, and elevated expression of several regulatory markers on CD11c+ cells and T cells. This regulatory environment is maintained in TDLNs of elderly mesothelioma-bearing mice, and is accompanied by increased suppression in the elderly tumor microenvironment. Thus, there is a greater summation of negative signals during elderly DC/T cell interactions, leading to effector T cell suppression and/or generation of Tregs, resulting in reduced anti-mesothelioma immune responses, that altogether contribute to faster tumor growth. Furthermore, the age-related suppressive status of elderly TDLN CD11c+ cells and T cells was exacerbated during IL-2/CD40 immunotherapy, which may have contributed to its reduced efficacy in elderly mice with mesothelioma. These data suggest that combining IL-2/CD40 with checkpoint blockade targeting multiple regulatory molecules could improve the efficacy of anti-cancer therapies in elderly hosts with mesothelioma.
Ethics Statement
This study was carried out in accordance with the recommendations of the Australian Code of Practice for the care and use of animals for scientific purposes. The protocol was approved by the Curtin University Animal Ethics Committee (approval number AEC-2012-21).
Data Availability
All datasets generated and analyzed for this study are included in the manuscript and the supplementary files.
Author Contributions
DN and CJ conceptualized and designed the project and experiments, were responsible for management and co-ordination of research activity and acquired financial support for the project. DN, CJ, and CM provided supervision for research activity planning and study execution. CJ and JG performed the experiments. JG, CJ, and DN analyzed the data. All authors contributed to writing, reviewing and editing the manuscript.
Conflict of Interest Statement
DN acts as a non-salaried Chief Scientific Officer for Selvax. Selvax did not fund or contribute to this study in any way.
The remaining authors declare that the research was conducted in the absence of any commercial or financial relationships that could be construed as a potential conflict of interest.
Acknowledgments
The authors acknowledge the Curtin Health Innovation Research Institute and the School of Pharmacy and Biomedical Sciences, Curtin University for provision of research facilities and technology platforms utilized in this study. The authors gratefully acknowledge the School of Pharmacy and Biomedical Sciences, Curtin University, and the Cancer Council Western Australia for the financial support to conduct this study. JG was supported by a Cancer Council Western Australia PhD Top-up Scholarship.
The content in this manuscript first appeared in JG PhD thesis, which is available online via the Curtin University Library.
Supplementary Material
The Supplementary Material for this article can be found online at: https://www.frontiersin.org/articles/10.3389/fmed.2018.00337/full#supplementary-material
References
1. Hadrup S, Donia M, Thor Straten P. Effector CD4 and CD8 T cells and their role in the tumor microenvironment. Cancer Microenviron. (2013) 6:123–33. doi: 10.1007/s12307-012-0127-6
2. Spel L, Boelens J-J, Nierkens S, Boes M. Antitumor immune responses mediated by dendritic cells: how signals derived from dying cancer cells drive antigen cross-presentation. Oncoimmunology (2013) 2:e26403. doi: 10.4161/onci.26403
3. Palucka K, Banchereau J. Cancer immunotherapy via dendritic cells. Nat Rev Cancer (2012) 12:265–77. doi: 10.1038/nrc3258
4. McDonnell AM, Robinson BWS, Currie AJ. Tumor antigen cross-presentation and the dendritic cell: Where it all begins? Clin Dev Immunol. (2010) 2010:539519. doi: 10.1155/2010/539519
5. Melief CJM. Cancer immunotherapy by dendritic cells. Immunity (2008) 29:372–83. doi: 10.1016/j.immuni.2008.08.004
6. Grolleau-Julius A, Harning EK, Abernathy LM, Yung RL. Impaired dendritic cell function in aging leads to defective antitumor immunity. Cancer Res. (2008) 68:6341–9. doi: 10.1158/0008-5472.can-07-5769
7. Grolleau-Julius A, Garg MR, Mo R, Stoolman LL, Yung RL. Effect of aging on bone marrow-derived murine CD11c+CD4–CD8α- dendritic cell function. J Gerontol A Biol Sci Med Sci. (2006) 61:1039–47. doi: 10.1093/gerona/61.10.1039
8. Sharma S, Lucia-Dominguez A, Lustgarten J. Aging affect the anti-tumor potential of dendritic cell vaccination, but it can be overcome by co-stimulation with anti-OX40 or anti-4-1BB. Exp Gerontol. (2006) 41:78–84. doi: 10.1016/j.exger.2005.10.002
9. Shi M, Bi X, Xu S, He Y, Guo X, Xiang J. Increased susceptibility of tumorigenicity and decreased anti-tumor effect of DC vaccination in aged mice are potentially associated with increased number of NK1.1+CD3+ NKT cells. Exp Oncol. (2005) 27:125–9.
10. Gravekamp C, Kim SH, Castro F. Cancer vaccination: manipulation of immune responses at old age. Mech Age Dev. (2009) 130:67–75. doi: 10.1016/j.mad.2008.05.003
11. Ruby CE, Weinberg AD. OX40-enhanced tumor rejection and effector T cell differentiation decreases with age. J Immunol. (2009) 182:1481–9. doi: 10.4049/jimmunol.182.3.1481
12. Provinciali M, Smorlesi A, Donnini A, Bartozzi B, Amici A. Low effectiveness of DNA vaccination against HER-2/neu in ageing. Vaccine (2003) 21:843–8. doi: 10.1016/S0264-410X(02)00530-3
13. Win S, Uenaka A, Nakayama E. Immune responses against allogeneic and syngeneic tumors in aged C57BL/6 mice. Microbiol Immunol. (2002) 46:513–9. doi: 10.1111/j.1348-0421.2002.tb02728.x
14. Young MR, Kolesiak K, Achille NJ, Meisinger J, Gonzalez E, Liu SW, et al. Impact of aging on immune modulation by tumor. Cancer Immunol Immunother. (2001) 50:315–20. doi: 10.1007/s002620100203
15. Provinciali M, Argentati K, Tibaldi A. Efficacy of cancer gene therapy in aging: adenocarcinoma cells engineered to release IL-2 are rejected but do not induce tumor specific immune memory in old mice. Gene Ther. (2000) 7:624–32. doi: 10.1038/sj.gt.3301131
16. Flood PM, Liu X, Alexander R, Schreiber H, Haque S. Loss of resistance to a highly immunogenic tumor with age corresponds to the decline of CD8 T cell activity. J Immunother. (1998) 21:307–16.
17. Flood PM, Urban JL, Kripke ML, Schreiber H. Loss of tumor-specific and idiotype-specific immunity with age. J Exp Med. (1981) 154:275–90.
18. Urban JL, Schreiber H. Rescue of the tumor-specific immune response of aged mice in vitro. J Immunol. (1984) 133:527–34.
19. Robinson BM. Malignant pleural mesothelioma: an epidemiological perspective. Ann Cardiothorac Surg. (2012) 1:491–6. doi: 10.3978/j.issn.2225-319X.2012.11.04
20. Grégoire M. What's the place of immunotherapy in malignant mesothelioma treatments? Cell Adhesion Migrat. (2010) 4:153–61.
21. Hurez V, Daniel BJ, Sun L, Liu A-J, Ludwig SM, Kious MJ, et al. Mitigating age-related immune dysfunction heightens the efficacy of tumor immunotherapy in aged mice. Cancer Res. (2012) 72:2089–99. doi: 10.1158/0008-5472.can-11-3019
22. Sharma S, Dominguez A, Hoelzinger D, Lustgarten J. CpG-ODN but not other TLR-ligands restore the antitumor responses in old mice: the implications for vaccinations in the aged. Cancer Immunol Immunother. (2008) 57:549–61. doi: 10.1007/s00262-007-0393-1
23. Lustgarten J, Dominguez AL, Thoman M. Aged mice develop protective antitumor immune responses with appropriate costimulation. J Immunol. (2004) 173:4510–5. doi: 10.4049/jimmunol.173.7.4510
24. Lustgarten J. Cancer immunotherapy: focusing on the young, neglecting the old. Future Oncol. (2010) 6:873–6. doi: 10.2217/fon.10.64
25. Lustgarten J. Cancer, aging and immunotherapy: lessons learned from animal models. Cancer Immunol Immunother. (2009) 58:1979–89. doi: 10.1007/s00262-009-0677-8
26. Jackaman C, Lew AM, Zhan Y, Allan JE, Koloska B, Graham PT, et al. Deliberately provoking local inflammation drives tumors to become their own protective vaccine site. Int Immunol. (2008) 20:1467–79. doi: 10.1093/intimm/dxn104
27. Jackaman C, Cornwall S, Graham PT, Nelson DJ. CD40-activated B cells contribute to mesothelioma tumor regression. Immunol Cell Biol. (2011) 89:255–67. doi: 10.1038/icb.2010.88
28. Jackaman C, Yeoh TL, Acuil ML, Gardner JK, Nelson DJ. Murine mesothelioma induces locally-proliferating IL-10+TNF-α+CD206–CX3CR1+ M3 macrophages that can be selectively depleted by chemotherapy or immunotherapy. OncoImmunology (2016) 5:e1173299. doi: 10.1080/2162402X.2016.1173299
29. Jackaman C, Nelson DJ. Intratumoral interleukin-2/agonist CD40 antibody drives CD4+ -independent resolution of treated-tumors and CD4+ -dependent systemic and memory responses. Cancer Immunol Immunother. (2012) 61:549–60. doi: 10.1007/s00262-011-1120-5
30. Jackaman C, Lansley S, Allan JE, Robinson BW, Nelson DJ. IL-2/CD40-driven NK cells install and maintain potency in the anti-mesothelioma effector/memory phase. Int Immunol. (2012) 24:357–68. doi: 10.1093/intimm/dxs005
31. Jackaman C, Dye DE, Nelson DJ. IL-2/CD40-activated macrophages rescue age and tumor-induced T cell dysfunction in elderly mice. Age (2014) 36:9655. doi: 10.1007/s11357-014-9655-y
32. Jackaman C, Radley-Crabb HG, Soffe Z, Shavlakadze T, Grounds MD, Nelson DJ. Targeting macrophages rescues age-related immune deficiencies in C57BL/6J geriatric mice. Aging Cell (2013) 12:345–57. doi: 10.1111/acel.12062
33. Yuan R, Tsaih SW, Petkova SB, Marin de Evsikova C, Xing S, Marion MA, et al. Aging in inbred strains of mice: study design and interim report on median lifespans and circulating IGF1 levels. Aging Cell (2009) 8:277–87. doi: 10.1111/j.1474-9726.2009.00478.x
34. Shavlakadze T, McGeachie J, Grounds MD. Delayed but excellent myogenic stem cell response of regenerating geriatric skeletal muscles in mice. Biogerontology (2010) 11:363–76. doi: 10.1007/s10522-009-9260-0
35. Jackaman C, Bundell CS, Kinnear BF, Smith AM, Filion P, van Hagen D, et al. IL-2 intratumoral immunotherapy enhances CD8+ T cells that mediate destruction of tumor cells and tumor-associated vasculature: a novel mechanism for IL-2. J Immunol. (2003) 171:5051–63. doi: 10.4049/jimmunol.171.10.5051
36. Duong L, Radley-Crabb HG, Gardner JK, Tomay F, Dye DE, Grounds MD, et al. Macrophage Depletion in Elderly Mice Improves Response to Tumor Immunotherapy, Increases Anti-tumor T Cell Activity and Reduces Treatment-Induced Cachexia. Front Genet. (2018) 9:526. doi: 10.3389/fgene.2018.00526
37. Demoulin S, Herfs M, Delvenne P, Hubert P. Tumor microenvironment converts plasmacytoid dendritic cells into immunosuppressive/tolerogenic cells: insight into the molecular mechanisms. J Leuk Biol. (2013) 93:343–52. doi: 10.1189/jlb.0812397
38. Munn DH, Sharma MD, Baban B, Harding HP, Zhang Y, Ron D, et al. GCN2 kinase in T cells mediates proliferative arrest and anergy induction in response to indoleamine 2,3-dioxygenase. Immunity (2005) 22:633–42. doi: 10.1016/j.immuni.2005.03.013
39. Jahrsdorfer B, Vollmer A, Blackwell SE, Maier J, Sontheimer K, Beyer T, et al. Granzyme B produced by human plasmacytoid dendritic cells suppresses T-cell expansion. Blood (2010) 115:1156–65. doi: 10.1182/blood-2009-07-235382
40. Sharma MD, Baban B, Chandler P, Hou D-Y, Singh N, Yagita H, et al. Plasmacytoid dendritic cells from mouse tumor-draining lymph nodes directly activate mature Tregs via indoleamine 2,3-dioxygenase. J Clin Invest. (2007) 117:2570–82. doi: 10.1172/JCI31911
41. Munn DH, Sharma MD, Hou D, Baban B, Lee JR, Antonia SJ, et al. Expression of indoleamine 2,3-dioxygenase by plasmacytoid dendritic cells in tumor-draining lymph nodes. J Clin Invest. (2004) 114:280–90. doi: 10.1172/JCI200421583
42. Ogata M, Ito T, Shimamoto K, Nakanishi T, Satsutani N, Miyamoto R, et al. Plasmacytoid dendritic cells have a cytokine-producing capacity to enhance ICOS ligand-mediated IL-10 production during T-cell priming. Int Immunol. (2013) 25:171–82. doi: 10.1093/intimm/dxs103
43. Conrad C, Gregorio J, Wang Y-H, Ito T, Meller S, Hanabuchi S, et al. Plasmacytoid dendritic cells promote immunosuppression in ovarian cancer via ICOS costimulation of Foxp3+ T-regulatory cells. Cancer Res. (2012) 72:5240–9. doi: 10.1158/0008-5472.can-12-2271
44. Faget J, Bendriss-Vermare N, Gobert M, Durand I, Olive D, Biota C, et al. ICOS-ligand expression on plasmacytoid dendritic cells supports breast cancer progression by promoting the accumulation of immunosuppressive CD4+ T cells. Cancer Res. (2012) 72:6130–41. doi: 10.1158/0008-5472.can-12-2409
45. Ito T, Yang M, Wang Y-H, Lande R, Gregorio J, Perng OA, et al. Plasmacytoid dendritic cells prime IL-10–producing T regulatory cells by inducible costimulator ligand. J Exp Med. (2007) 204:105–15. doi: 10.1084/jem.20061660
46. Munn DH, Mellor AL. The tumor-draining lymph node as an immune-privileged site. Immunol Rev. (2006) 213:146–58. doi: 10.1111/j.1600-065X.2006.00444.x
47. Emens LA. Breast cancer immunobiology driving immunotherapy: vaccines and immune checkpoint blockade. Expert Rev Anticancer Ther. (2012) 12:1597–611. doi: 10.1586/era.12.147
48. Weng NP, Akbar AN, Goronzy J. CD28- T cells: their role in the age-associated decline of immune function. Trends Immunol. (2009) 30:306–12. doi: 10.1016/j.it.2009.03.013
49. Warrington KJ, Vallejo AN, Weyand CM, Goronzy JJ. CD28 loss in senescent CD4+ T cells: reversal by interleukin-12 stimulation. Blood (2003) 101:3543–9. doi: 10.1182/blood-2002-08-2574
50. Vallejo AN, Nestel AR, Schirmer M, Weyand CM, Goronzy JJ. Aging-related deficiency of CD28 expression in CD4+ T cells is associated with the loss of gene-specific nuclear factor binding activity. J Biol Chem. (1998) 273:8119–29
51. Effros RB. Loss of CD28 expression on T lymphocytes: A marker of replicative senescence. Dev Comp Immunol. (1997) 21:471–8. doi: 10.1016/S0145-305X(97)00027-X
52. Channappanavar R, Twardy BS, Krishna P, Suvas S. Advancing age leads to predominance of inhibitory receptor expressing CD4 T cells. Mech Ageing Dev. (2009) 130:709–12. doi: 10.1016/j.mad.2009.08.006
53. Shimada Y, Hayashi M, Nagasaka Y, Ohno-Iwashita Y, Inomata M. Age-associated up-regulation of a negative co-stimulatory receptor PD-1 in mouse CD4+ T cells. Exp Gerontol. (2009) 44:517–22. doi: 10.1016/j.exger.2009.05.003
54. Krummel MF, Allison JP. CD28 and CTLA-4 have opposing effects on the response of T cells to stimulation. J Exp Med. (1995) 182:459–65. doi: 10.1084/jem.182.2.459
55. Butte MJ, Peña-Cruz V, Kim M-J, Freeman GJ, Sharpe AH. Interaction of human PD-L1 and B7-1. Mol Immunol. (2008) 45:3567–72. doi: 10.1016/j.molimm.2008.05.014
56. Butte MJ, Keir ME, Phamduy TB, Sharpe AH, Freeman GJ. Programmed death-1 ligand 1 interacts specifically with the B7-1 costimulatory molecule to inhibit T cell responses. Immunity (2007) 27:111–22. doi: 10.1016/j.immuni.2007.05.016
57. Lages CS, Lewkowich I, Sproles A, Wills-Karp M, Chougnet C. Partial restoration of T-cell function in aged mice by in vitro blockade of the PD-1/ PD-L1 pathway. Aging Cell (2010) 9:785–98. doi: 10.1111/j.1474-9726.2010.00611.x
58. Mirza N, Duque MA, Dominguez AL, Schrum AG, Dong H, Lustgarten J. B7-H1 expression on old CD8+ T cells negatively regulates the activation of immune responses in aged animals. J Immunol. (2010) 184:5466–74. doi: 10.4049/jimmunol.0903561
59. Lee KA, Shin KS, Kim GY, Song YC, Bae EA, Kim IK, et al. Characterization of age-associated exhausted CD8(+) T cells defined by increased expression of Tim-3 and PD-1. Aging Cell (2016) 15:291–300. doi: 10.1111/acel.12435
60. McClanahan F, Riches JC, Miller S, Day WP, Kotsiou E, Neuberg D, et al. Mechanisms of PD-L1/PD-1–mediated CD8 T-cell dysfunction in the context of aging-related immune defects in the Eμ-TCL1 CLL mouse model. Blood (2015) 126:212–21. doi: 10.1182/blood-2015-02-626754
61. Norrie IC, Ohlsson E, Nielsen O, Hasemann MS, Porse BT. C/EBPα is dispensable for the ontogeny of PD-1+ CD4+ memory T cells but restricts their expansion in an age-dependent manner. PLoS ONE (2014) 9:e84728. doi: 10.1371/journal.pone.0084728
62. Decman V, Laidlaw BJ, Doering TA, Leng J, Ertl HCJ, Goldstein DR, et al. Defective CD8 T cell responses in aged mice are due to quantitative and qualitative changes in virus-specific precursors. J Immunol. (2012) 188:1933–41. doi: 10.4049/jimmunol.1101098
63. Shimatani K, Nakashima Y, Hattori M, Hamazaki Y, Minato N. PD-1+ memory phenotype CD4+ T cells expressing C/EBPalpha underlie T cell immunodepression in senescence and leukemia. Proc Natl Acad Sci USA. (2009) 106:15807–12. doi: 10.1073/pnas.0908805106
64. Patsoukis N, Bardhan K, Chatterjee P, Sari D, Liu B, Bell LN, et al. PD-1 alters T-cell metabolic reprogramming by inhibiting glycolysis and promoting lipolysis and fatty acid oxidation. Nat Commun. (2015) 6:6692. doi: 10.1038/ncomms7692
65. Francisco LM, Sage PT, Sharpe AH. The PD-1 pathway in tolerance and autoimmunity. Immunol Rev. (2010) 236:219–42. doi: 10.1111/j.1600-065X.2010.00923.x
66. Riley JL. PD-1 signaling in primary T cells. Immunol Rev. (2009) 229:114–25. doi: 10.1111/j.1600-065X.2009.00767.x
67. Carter LL, Fouser LA, Jussif J, Fitz L, Deng B, Wood CR, et al. PD-1:PD-L inhibitory pathway affects both CD4+ and CD8+ T cells and is overcome by IL-2. Eur J Immunol. (2002) 32:634–43. doi: 10.1002/1521-4141(200203)32:3<634::AID-IMMU634>3.0.CO;2-9
68. Workman CJ, Vignali DA. Negative regulation of T cell homeostasis by lymphocyte activation gene-3 (CD223). J Immunol. (2005) 174:688–95. doi: 10.4049/jimmunol.174.2.688
69. Farazi M, Cohn Z, Nguyen J, Weinberg AD, Ruby CE. Early-onset age-related changes in dendritic cell subsets can impair antigen-specific T helper 1 (Th1) CD4 T cell priming. J Leukocyte Biol. (2014) 96:245–54. doi: 10.1189/jlb.1A0114-066R
70. Pereira LF, Duarte de Souza AP, Borges TJ, Bonorino C. Impaired in vivo CD4+ T cell expansion and differentiation in aged mice is not solely due to T cell defects: decreased stimulation by aged dendritic cells. Mech Age Dev. (2011) 132:187–94. doi: 10.1016/j.mad.2011.03.005
71. Antonioli L, Pacher P, Vizi ES, Hasko G. CD39 and CD73 in immunity and inflammation. Trends Mol Med. (2013) 19:355–67. doi: 10.1016/j.molmed.2013.03.005
72. Zhang H, Conrad DM, Butler JJ, Zhao C, Blay J, Hoskin DW. Adenosine acts through A2 receptors to inhibit IL-2-induced tyrosine phosphorylation of STAT5 in T lymphocytes: role of cyclic adenosine 3',5'-monophosphate and phosphatases. J Immunol. (2004) 173:932–44. doi: 10.4049/jimmunol.173.2.932
73. Mirabet M, Herrera C, Cordero OJ, Mallol J, Lluis C, Franco R. Expression of A2B adenosine receptors in human lymphocytes: their role in T cell activation. J Cell Sci. (1999) 112:491–502.
74. Ehrentraut H, Westrich JA, Eltzschig HK, Clambey ET. Adora2b adenosine receptor engagement enhances regulatory T cell abundance during endotoxin-induced pulmonary inflammation. PLoS ONE (2012) 7:e32416. doi: 10.1371/journal.pone.0032416
75. Nakatsukasa H, Tsukimoto M, Harada H, Kojima S. Adenosine A2B receptor antagonist suppresses differentiation to regulatory T cells without suppressing activation of T cells. Biochem Biophys Res Commun. (2011) 409:114–9. doi: 10.1016/j.bbrc.2011.04.125
76. Inoue S, Suzuki K, Komori Y, Morishita Y, Suzuki-Utsunomiya K, Hozumi K, et al. Persistent inflammation and T cell exhaustion in severe sepsis in the elderly. Crit Care (2014) 18:R130. doi: 10.1186/cc13941
77. Gardner JK. An Increase in Suppressive Dendritic Cells and T Cells is a Hallmark of Healthy Aging, A Phenomenon Exacerbated by Cancer and Modulated by Chemo- and Immunotherapy [PhD thesis]: Curtin University (2016).
79. Gupta PK, Godec J, Wolski D, Adland E, Yates K, Pauken KE, et al. CD39 expression identifies terminally exhausted CD8+ T cells. PLoS Pathog. (2015) 11:e1005177. doi: 10.1371/journal.ppat.1005177
80. Jiang Y, Li Y, Zhu B. T-cell exhaustion in the tumor microenvironment. Cell Death Dis. (2015) 6:e1792. doi: 10.1038/cddis.2015.162
81. Baitsch L, Baumgaertner P, Dev xEa, vre E, Raghav SK, et al. Exhaustion of tumor-specific CD8+ T cells in metastases from melanoma patients. J Clin Invest. (2011) 121:2350–60. doi: 10.1172/JCI46102
82. Sakuishi K, Apetoh L, Sullivan JM, Blazar BR, Kuchroo VK, Anderson AC. Targeting Tim-3 and PD-1 pathways to reverse T cell exhaustion and restore anti-tumor immunity. J Exp Med. (2010) 207:2187–94. doi: 10.1084/jem.20100643
83. Wherry EJ, Kurachi M. Molecular and cellular insights into T cell exhaustion. Nat Rev Immunol. (2015) 15:486–99. doi: 10.1038/nri3862
84. Pooley JL, Heath WR, Shortman K. Intravenous soluble antigen is presented to CD4 T cells by CD8- dendritic cells but cross-presented to CD8 T cells by CD8+ dendritic cells. J Immunol. (2001) 166:5327–30. doi: 10.4049/jimmunol.166.9.5327
85. Maldonado-Lopez R, De Smedt T, Michel P, Godfroid J, Pajak B, Heirman C, et al. CD8α+ and CD8α- subclasses of dendritic cells direct the development of distinct T helper cells in vivo. J Exp Med. (1999) 189:587–92. doi: 10.1084/jem.189.3.587
86. Hsu P, Santner-Nanan B, Hu M, Skarratt K, Lee CH, Stormon M, et al. IL-10 potentiates differentiation of human induced regulatory T cells via STAT3 and Foxo1. J Immunol. (2015) 195:3665–74. doi: 10.4049/jimmunol.1402898
87. Akbari O, DeKruyff RH, Umetsu DT. Pulmonary dendritic cells producing IL-10 mediate tolerance induced by respiratory exposure to antigen. Nat Immunol. (2001) 2:725–31. doi: 10.1038/90667
88. Beyer M, Abdullah Z, Chemnitz JM, Maisel D, Sander J, Lehmann C, et al. Tumor-necrosis factor impairs CD4+ T cell-mediated immunological control in chronic viral infection. Nat Immunol. (2016) 17:593–603. doi: 10.1038/ni.3399
89. Weiss JM, Back TC, Scarzello AJ, Subleski JJ, Hall VL, Stauffer JK, et al. Successful immunotherapy with IL-2/anti-CD40 induces the chemokine-mediated mitigation of an immunosuppressive tumor microenvironment. Proc Natl Acad Sci USA. (2009) 106:19455–60. doi: 10.1073/pnas.0909474106
90. Berner V, Liu H, Zhou Q, Alderson KL, Sun K, Weiss JM, et al. IFN-gamma mediates CD4+ T-cell loss and impairs secondary antitumor responses after successful initial immunotherapy. Nat Med. (2007) 13:354–60. doi: 10.1038/nm1554
91. Welniak LA, Shorts L, Subleski J, Blazar BR, Wiltrout RH, Murphy WJ. Tumor regression by anti-CD40 and interleukin-2: role of CD40 in hematopoietic cells and organ-specific effects. Biol Blood Bone Marrow Trans. (2004) 10:534–9. doi: 10.1016/j.bbmt.2004.05.006
92. Murphy WJ, Welniak L, Back T, Hixon J, Subleski J, Seki N, et al. Synergistic anti-tumor responses after administration of agonistic antibodies to CD40 and IL-2: coordination of dendritic and CD8+ cell responses. J Immunol. (2003) 170:2727–33. doi: 10.4049/jimmunol.170.5.2727
93. Weiss JM, Subleski JJ, Back T, Chen X, Watkins SK, Yagita H, et al. Regulatory T cells and myeloid-derived suppressor cells in the tumor microenvironment undergo Fas-dependent cell death during IL-2/αCD40 therapy. J Immunol. (2014) 192:5821–9. doi: 10.4049/jimmunol.1400404
94. Fransen MF, Sluijter M, Morreau H, Arens R, Melief CJ. Local activation of CD8 T cells and systemic tumor eradication without toxicity via slow release and local delivery of agonistic CD40 antibody. Clin Cancer Res. (2011) 17:2270–80. doi: 10.1158/1078-0432.ccr-10-2888
95. Stumbles PA, Himbeck R, Frelinger JA, Collins EJ, Lake RA, Robinson BWS. Cutting edge: Tumor-specific CTL are constitutively cross-armed in draining lymph nodes and transiently disseminate to mediate tumor regression following systemic CD40 activation. J Immunol. (2004) 173:5923–8. doi: 10.4049/jimmunol.173.10.5923
96. Wilson JM, Ross WG, Agbai ON, Frazier R, Figler RA, Rieger J, et al. The A2B adenosine receptor impairs the maturation and immunogenicity of dendritic cells. J Immunol. (2009) 182:4616–23. doi: 10.4049/jimmunol.0801279
97. Addi AB, Lefort A, Hua X, Libert F, Communi D, Ledent C, et al. Modulation of murine dendritic cell function by adenine nucleotides and adenosine: involvement of the A(2B) receptor. Eur J Immunol. (2008) 38:1610–20. doi: 10.1002/eji.200737781
98. Novitskiy SV, Ryzhov S, Zaynagetdinov R, Goldstein AE, Huang Y, Tikhomirov OY, et al. Adenosine receptors in regulation of dendritic cell differentiation and function. Blood (2008) 112:1822–31. doi: 10.1182/blood-2008-02-136325
99. Kushwah R, Hu J. Role of dendritic cells in the induction of regulatory T cells. Cell Biosci. (2011) 1:1–10. doi: 10.1186/2045-3701-1-20
100. Coombes JL, Siddiqui KR, Arancibia-Carcamo CV, Hall J, Sun CM, Belkaid Y, et al. A functionally specialized population of mucosal CD103+ DCs induces Foxp3+ regulatory T cells via a TGF-beta and retinoic acid-dependent mechanism. J Exp Med. (2007) 204:1757–64. doi: 10.1084/jem.20070590
101. Sgambato A, Casaluce F, Gridelli C. The role of checkpoint inhibitors immunotherapy in advanced non-small cell lung cancer in the elderly. Expert Opin Biol Ther. (2017) 17:565–71. doi: 10.1080/14712598.2017.1294157
102. Chiarion Sileni V, Pigozzo J, Ascierto PA, Grimaldi AM, Maio M, Di Guardo L, et al. Efficacy and safety of ipilimumab in elderly patients with pretreated advanced melanoma treated at Italian centres through the expanded access programme. J Exp Clin Cancer Res. (2014) 33:30. doi: 10.1186/1756-9966-33-30
103. Freeman M, Weber J. Subset analysis of the safety and efficacy of nivolumab in elderly patients with metastatic melanoma. J Immunother Cancer (2015) 3(Suppl. 2):P133. doi: 10.1186/2051-1426-3-S2-P133
Keywords: dendritic cells, aging, cancer, immunotherapy, immunosuppression
Citation: Gardner JK, Jackaman C, Mamotte CDS and Nelson DJ (2018) The Regulatory Status Adopted by Lymph Node Dendritic Cells and T Cells During Healthy Aging Is Maintained During Cancer and May Contribute to Reduced Responses to Immunotherapy. Front. Med. 5:337. doi: 10.3389/fmed.2018.00337
Received: 22 May 2018; Accepted: 15 November 2018;
Published: 30 November 2018.
Edited by:
Anis Larbi, Singapore Immunology Network (A*STAR), SingaporeReviewed by:
Anshu Agrawal, University of California, Irvine, United StatesSara De Biasi, Università degli Studi di Modena e Reggio Emilia, Italy
Copyright © 2018 Gardner, Jackaman, Mamotte and Nelson. This is an open-access article distributed under the terms of the Creative Commons Attribution License (CC BY). The use, distribution or reproduction in other forums is permitted, provided the original author(s) and the copyright owner(s) are credited and that the original publication in this journal is cited, in accordance with accepted academic practice. No use, distribution or reproduction is permitted which does not comply with these terms.
*Correspondence: Delia J. Nelson, ZGVsaWEubmVsc29uQGN1cnRpbi5lZHUuYXU=