- Materials Science and Engineering Department, University of Texas at Arlington, Arlington, TX, United States
A continuing quest exists to develop high-performance grease capable of meeting extreme demands of high energy efficiency and durability of modern mechanical systems. In this study, multi-walled carbon nanotubes (MWCNT) and ionic liquids (IL) either singly or as a binary additive system were prepared to study the tribological performance of lithium greases under boundary lubrication condition. The combination of MWCNT and IL can benefit from their respective lubricating mechanism to minimize friction and wear. To test this hypothesis, MWCNT and IL are tested individually and compared with combined MWCNT-IL additive mixture within lithium-based greases. MWCNT-IL gelatinous hybrids were prepared by inducing physical non-covalent interactions between MWCNTs and phosphorous based ILs through ultrasonication and magnetic stirring. Four different phosphorous based ILs, Choline bis (2-ethylhexyl)-phosphate, Choline dibutyl-dithiophosphate, Methyl-tributyl-phosphonium dimethyl-phosphate, Tetra-n-butyl-phosphonium O,O-diethyl-dithiophosphate were used. Four ball tribological test configuration was used to assess wear and friction properties of fabricated greases. The lubrication mechanism of these additives was elucidated through analysis of worn surfaces using surface characterization techniques like SEM, EDS, and stereo optical microscopy. All greases that contain ionic liquid exhibited better tribological performance compared to grease with ZDDP. The maximum reduction of the coefficient of friction (COF) of 60% and wear scar diameter (WSD) reduction of 25% was observed for greases containing MWCNTs and phosphonium cation based ILs hybrids as an additive. Results indicated either worse or comparable anti-wear behavior in greases with MWCNT-IL hybrids as compared to greases that only contained IL or MWCNT. The antagonistic interaction between MWCNT and IL has been postulated to occur when MWCNT adversely interacts with IL formed tribofilms, negating the benefit from IL resulting in increased wear in greases with binary mixtures of MWCNT-IL.
Introduction
The advent of nanotechnology and nanotribology has expanded the realms of research toward the use of nanoparticles or nanomaterials as additives in lubricating media like oil or grease. Nanomaterials offer unique physical, chemical, and mechanical properties due to their size effect, surface and interface effects, thermal stability, and variety of particle chemistries. In literature, various nanoparticles such as graphite (Lee et al., 2009), polytetrafluoroethylene (PTFE) (Sharma et al., 2017), MoS2 (An et al., 2014; Bagi and Aswath, 2015b), TiO2 (Ilie and Covaliu, 2016), La(OH)3 (Hao et al., 2010), PbS (Chen and Liu, 2006), LaF3 (Zhou et al., 2001), titanium borate (Hu and Dong, 1998), zinc borate (Dong and Hu, 1998), ferric oxide (Hu et al., 1998), calcium borate (Zhao et al., 2014), Ni (Qiu et al., 2001), and CaCO3 (Sunqing et al., 2000; Ji et al., 2011) have been used as lubricant additives with potentially interesting friction and wear properties. Some of the suggested mechanisms behind the excellent tribological performance of nanomaterials are rolling effect (Wu et al., 2007), protective film by tribochemical reactions (Liu and Chen, 2000), ball bearing effect (Tarasov et al., 2002), adsorption on surface to compensate for material loss, mending effect (Liu et al., 2004), and third body material transfer (Huang et al., 2006; Guo and Zhang, 2016).
Recently, carbon nanotubes (CNTs) have emerged as a promising candidate for lubricant additive. Addition of CNT nanoparticles is well-known to improve anti-wear, extreme pressure, and load carrying capability of lubricating oil/grease. The superior tribological properties of CNTs have been attributed to their unique structure and dimension, which allows them to be easily active in the contact area and reduce interfacial friction. Kobayashi et al. compared the tribological properties of greases containing carbon materials like graphite, cluster diamond with the grease having carbon nanohorn as extreme pressure additive and found that all carbon material containing greases resulted in improved friction and wear behavior of lithium-based grease (Kobayashi et al., 2005). Cursaru et al. reported that Co-based single-walled carbon nanotubes (SWCNTs) could be used as an additive for mineral base oils with friction reduction, and a synergistic effect can be observed with SWCNTs and commercial lubricant additives (Cursaru et al., 2012). Mohamed et al. added electric arc discharged CNTs in lithium grease and reported that 1 wt% of SWCNT improves antifriction and antiwear properties of grease (Mohamed et al., 2014). Most recently, Kamel et al. examined tribological behavior of calcium grease containing different concentrations of MWCNTs and suggested MWCNTs formed lubricating films on the worn surfaces preventing direct metal-metal contact; minimizing wear of the interacting surfaces (Kamel et al., 2016). However, the practical tribological applications of CNTs are hindered due to problems like agglomerations and poor dispersibility in oil or grease.
Ionic liquids (ILs) have gained considerable attention in the lubrication industry because of their remarkable chemical and physical properties. ILs are molten salts with melting points below 100°C. They offer several advantages such as low vapor pressure, non-flammability, high thermal stability, low melting point, non-volatility, and good electrochemical properties. Many studies have shown the potential of ILs as a base lubricant (Somers et al., 2010; Otero et al., 2014; López-Sánchez et al., 2017) or a lubricant additive (Jiménez et al., 2006; Qu et al., 2009a; Blanco et al., 2017). ILs undergo tribochemical reactions with interacting metal surfaces and form antiwear tribofilms which help to improve the antiwear and antifriction properties of the lubricant. Furthermore, ILs are known as designer's liquid because various characteristics can be created with different combinations of cation and anionic moieties and their properties like dissolving ability and solvent miscibility can be tuned by altering cation, anion, and attached substituents. The alteration of hydrophobicity and hydrophilicity of ILs can be helpful to control the properties of nanomaterials. Most recently, ILs have been used to covalently or non-covalently modify CNTs to improve their dispersion, electrical conductivity and charge transfer activity (Mukai et al., 2009; Hong et al., 2010; Tunckol et al., 2012). Fukushima et al. prepared CNT-IL hybrid by grinding a suspension of SWCNTs in imidazolium-based IL with an agate mortar. They reported the formation of gelatinous material (commonly known as Bucky gels) due to non-covalent interaction between the cation of IL and π-electrons of the nanotube surface (Fukushima et al., 2003). Wang et al. demonstrated ILs interact with CNTs through weak van der Waals forces without influencing electronic structure of CNTs (Wang et al., 2008). Gelation formation can take place with a variety of CNTs (Mukai et al., 2009; Hong et al., 2010) and different ILs like pyridinium and pyrrolidinium-based ILs (Chun et al., 2010; de Castro et al., 2010). Bucky gels are reported to form stable dispersions in desired solvents. Covalent modification of CNTs by ILs is achieved through extensive chemical methods of grafting IL component on the activated CNT surface. Imidazolium-based ILs was used to covalently modify CNTs (Carrión et al., 2010). Covalent modification of CNTs enables a stable dispersion of CNTs in water or organic solvents, however, the process requires a lot of steps which make it expensive and difficult to control and not suitable for large scale industrial applications. Also, covalent functionalization disrupts extended π networks of CNTs, impairing their mechanical and electrical properties.
CNT/IL hybrids have potential applications as supports for metal nanoparticles, additives in polymer nanocomposites, in fabrication of electrochemical sensors, actuators, supercapacitors, dye-sensitized solar cells (Fukushima et al., 2006; Fukushima and Aida, 2007; Mukai et al., 2009; Zhao et al., 2010; Subramaniam et al., 2011; Tunckol et al., 2012). Recently, CNT/IL hybrids have found prospective application as a lubricant and antiwear additive. Until now, limited research has been reported on tribological properties of CNT-IL hybrids. Yu et al. used MWCNT chemically modified by imidazolium based IL as an antiwear additive for IL lubricants in steel-steel contacts (Yu et al., 2015). Wang et al. prepared IL based nanofluids by adding MWCNTs covalently functionalized by hydroxyl/amine terminated IL in hexaflurophosphate based IL and compared tribological characteristics of IL nanofluids with pure IL using a ball on disk configuration in steel-steel contact (Wang et al., 2010). Authors attributed the better antiwear and antifriction performance of nanofluids to the uniform dispersion of functionalized MWCNTs and their intrinsic mechanical properties. To the best our knowledge, preparation of MWCNT-Phosphorous based IL hybrids and tribological studies of these hybrids as an additive in lubricating mediums like oil and greases has not been attempted.
In this research work, tribological properties of lithium complex grease containing MWCNT-phosphorous based IL hybrids was examined. Selection of a proper combination of cation and anion of ILs was critical to form hybrids and achieve important lubrication performance. Many studies have used ILs with cations like imidazolium (Lu et al., 2004; Mu et al., 2005; Jiménez et al., 2006; Jiménez and Bermúdez, 2007), ammonium (Qu et al., 2006, 2009b), pyridium (Kinzig et al., 2005; Qu et al., 2009a) along with halogen anion counterparts like BF (Ye et al., 2001; Phillips and Zabinski, 2004; Mu et al., 2008) and PF (Phillips and Zabinski, 2004; Yu et al., 2008) and have reported problems of tribocorrosion and poor/incomplete miscibility of these ILs in non-polar oils. Most novel choline cation (Gabler et al., 2011; Pisarova et al., 2012; Sharma et al., 2015, 2016) and phosphonium cation (Liu et al., 2006; Qu et al., 2009a, 2011, 2012, 2014; Minami et al., 2010; Yu et al., 2012; Sharma et al., 2015, 2016) based ILs are described to have excellent oil miscibility, thermal stability, non-corrosiveness and antiwear/antiscuffing capabilities when used as additives in mineral base oil or PAO oil. Motivated from this, the present goal of this study is to assess the potential use of choline and phosphonium cation ILs as additives in lubricating greases. Four ionic liquids with either phosphonium/choline cation or phosphate/thiophosphate anion were selected. Initially, experiments were conducted using four ball tribometer to assess the antifriction and antiwear behavior of MWCNT and ILs additive separately in the boundary lubrication regime. Their tribological mechanism is discussed based on the results procured from optical microscopy, scanning electron microscope (SEM) and energy dispersive spectroscopy (EDS) techniques. Tribological performance of MWCNTs and ILs was individually compared with commercial additive, ZDDP at the same P treat rate. Secondly, four MWCNT-IL hybrids were prepared by making formulations with 1 wt% of MWCNT and ILs at 0.2 wt% of P. Physical non-covalent interaction between MWCNTs and ILs was induced through ultrasonication and magnetic stirring. Tribological performance and wear mechanism of these MWCNT-IL hybrid additives is examined through the understanding of morphology and tribochemistry of worn surfaces.
Materials and Methods
Details of Base Grease and Additive Chemistries
Base grease used for this study was commercially purchased lithium complex thickener. This grease was obtained from the supplier by removing a pail during the grease making process before adding additives, which assists in correlating the role of additives directly with the tribological test results. As the base grease was pulled out early in the process, remaining base oil had to be added manually to achieve the desired consistency of NLGI 2 grade of medium viscosity. Base oil used was Shell 46 Rotella T Triple Protection 15W-40.
Hydroxyl functionalized MWCNTs used as antiwear and antifriction additives for grease preparation were commercially purchased. Both the type and diameter are essential for MWCNTs selection. The wider the diameter of the carbon nanotube (CNTs), the more it behaves like graphite. The narrower the diameter of the Carbon Nanotubes (CNTs), the more its intrinsic properties depends upon its specific type. The MWCNTs selected for the study had an outer diameter of 20–40 nm and length of 10–30 μm. Along with the MWCNTs, the second additive was an ionic liquid. To check the effect of different cation and anion moieties on the non-covalent interaction of MWCNT-IL, four different ionic liquids with P and S containing anion or cation were used. ILs were provided from AC2T research GmbH Austria. Table 1 details the chemical structure and coded name of the antiwear additives used including ZDDP and the four ionic liquids. Tetrabutyl-phosphonium O,O-diethyl dithiophosphate (P IL1), and methyl-tributyl-phosphonium dimethyl-phosphate (P IL2) have similar (not same) cations while the anions have either a thiophosphate or phosphate moiety. Choline dibutyl-dithiophosphate (N IL1) and choline bis (2-ethylhexyl)-phosphate (N IL2) have the same cation while one anion has a thiophosphate structure, and another has a phosphate structure. The as purchased zinc dialkyl dithiophosphate (ZDDP) used in this study is secondary alcohol derived ZDDP with approximately 70% neutral and 30% basic characteristic.
Grease Blend Formulation
As no studies were available on the optimal concentration of MWCNTs and ILs, it was very crucial to prepare a mixing protocol with the optimum concentration. Table 2 gives an overview of all the 12 grease blends prepared for this study. Initial grease blends were formulated by adding MWCNTs and ILs separately to understand the tribological mechanism of these additives. For the comparison purpose, baseline grease blend without any additives was prepared by maintaining 100:30 ratio of thickener and synthetic base oil. The blends were formulated using a Kitchen-Aid Mixer. The electrical specifications of the mixer were, 120 Volts, power rating of 250 W. Initially, base oil and MWCNTs additive was mixed with a hand-held spatula in kitchen aid steel container; this process allows dispersion of MWCNTs in the base oil. The Li-based grease was then added to the mixture and blended for 2 h at a speed of 100 rpm with conjunction hand mixing after each 20-min interval, to ensure homogeneity and adequate mixing of greases. A similar approach was used to prepare greases with four phosphorous containing ILs additive.
Additionally, grease blends with an additive mixture of MWCNTs and ILs were prepared. At first, both additives were weighed and mixed with the base oil. To achieve adequate mixing, the weighed mixture was subjected to ultrasonication for 30 min. Ionic liquids are known to be immiscible in the non-polar solvents like synthetic base oil. Therefore, the mixture was subjected to the proper mixing by using pulse ultrasonicator with D.I water bath. Ultrasonication heat is not sufficient to trigger the interaction between MWCNT and Ionic liquid. So, the mixing was carried out using the magnetic stirrer at a constant 150 rpm for 2 h at 50°C. Bucky gel formation due to physical non-covalent interactions between MWCNTs and ILs was ensured after the magnetic stirring of additives mixture. Later, additives mixture was added into lithium based thickener and was blended using kitchen aid mixer for about 2 h with manual mixing by hand-held spatula after each 20-min interval.
Tribological Test Procedure
The tribological tests were performed using rotary four ball tribometer. Tests were carried out by following ASTM D2266 test conditions of 392 N normal load, 1,200 rpm, 75°C temperature and 60 min time to ensure the continuous sliding mode under boundary lubrication regime. The steel balls used in the tests were made up of bearing quality aircraft steel grade alloy, AISI standard E52100 with a ½″ diameter. The testing assembly consists of three balls placed in a chuck which is locked in a cage and fourth ball rotated against stationary three balls with the lubricating grease in between; thus, evaluating its tribological properties. All tests were repeated three times to ensure repeatability and minimize external errors. The coefficient of friction (COF) was calculated using a formula as described in the ASTM D5183, the formula uses torque and normal load values of the test and a normalization factor to calculate friction coefficient.
The absolute torque and load values were received during tests from the Compend X software assisting the four ball tribometer. After the termination of every test, the three stationary steel balls were retrieved and analyzed to determine the Wear Scar Diameter (WSD) using a stereo-optical microscope. Stereo-Optical microscope (model type: Nikon SMZ 1,500) was used to image the wear scars formed on the three stationary steel balls at 100X magnification. The images were analyzed using Image J software. The software comprised of measuring tools and Pixel-counter which was used to document the WSD for all the three steel balls and an average value was reported. WSD for each blend was determined using an average of 18 readings on 9 samples from a total of three repeat runs. Each reading represents the average value of the longest horizontal striation and longest vertical striation. Imaging all the samples ensured machine repeatability and normal loading of the samples by the spindle.
Worn Surface Characterization
To understand surface morphology and chemical makeup of the wear surfaces generated due to lubrication of test grease formulations, secondary electron microscope (SEM) (model type: Hitachi S-3000N) attached with energy dispersive X-ray unit (EDS analyses) was used. SEM imaging was carried out at an accelerating voltage of 15–20 kV. The samples were cleaned using hexane and were placed in sample holder using carbon tape, to maintain good electrical contact and ensure good imaging of wear scar. Wear surfaces showing distinct wear tracks and surface morphology were imaged at a magnification of 100X and 1,000X to elucidate wear mechanism in play. EDS spectrums were collected for the wear test samples analyzed by SEM. The spectrums were recorded for the magnification of the 1,000X at an accelerating voltage of 15 kV to reduce the penetration depth and collect elemental information from near surface region rather than the bulk. EDS mapping was carried out to substantiate findings from EDS spectrum data. EDS elemental mapping helps to understand tribochemistry and possible compound formation in tribofilms.
Results and Discussion
Tribological Behavior of MWCNTs and ILs as an Additive
Figure 1 exhibits the change in COF values during the test run of grease blends having only MWCNT and ILs as additives. COF values marked in the boxes are the averages of the COF values obtained from three runs performed for each grease blend. Observation of COF variation will help to understand the effectiveness of these additives as a friction modifier within the test duration. Base grease blend exhibits a high COF which increases gradually during the test. The larger COF of the base grease blend resulted from a real contacting area of the rubbing surface owing to wear. The grease blends with MWCNT resulted in a smaller and more stable COF than the base grease. At the beginning of the test, stable COF value is observed which is further followed by a slight increase. This pattern is continued until the end of the test. These changes in COF values indicate that initially thin layers of lubricating grease containing MWCNT are formed between the interfacial surfaces and with the continuation of the test these films are removed due to build up frictional force normally exerted by the top rotating steel ball. Slight increase and stability in COF values for MWCNT grease blend signify the effective antifriction behavior of MWCNTs. Additionally, all four ILs and ZDDP show an improvement in friction behavior compared to base grease at 0.2 wt% of the P treatment rate. Phosphonium cation based ILs has the lowest average COF. Choline cation based ILs also display lower COF in comparison to ZDDP for the duration of the test. Careful observation reveals all antiwear additives exhibit some level of variance in instantaneous friction with the progression of the test.
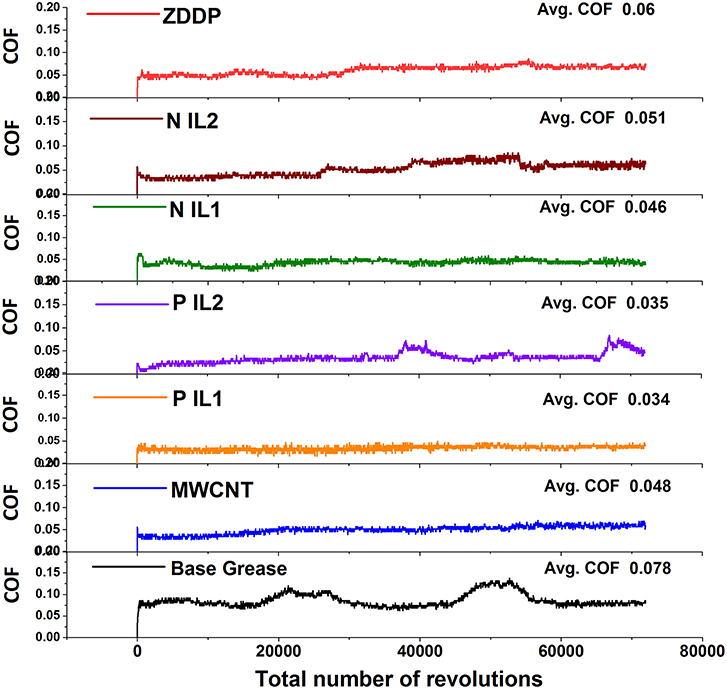
Figure 1. Coefficient of Friction from four ball wear tests for greases containing only MWCNT and only ILs additives compared with base grease and ZDDP grease blend.
From Figure 2, it can be seen that there is a significant decrease in the wear of the stationary steel ball surface when MWCNTs is used as an additive. Mohammed et al. research work on the tribological behavior of CNTs as an additive in lithium grease proposed that a threshold amount of 1 wt% results in need for improvement of extreme pressure, antiwear, and antifriction properties of lithium grease (Mohamed et al., 2014). MWCNTs can easily penetrate the tribological contact interface with the lubricating grease. They can get adsorbed and form a thin film on the rubbing surface. These layers minimize the sliding resistance and hence reduces the wear between contacting surfaces. In comparison with base grease and MWCNT, all ionic liquids exhibited significant improvement in wear protection in lithium base grease. ZDDP is a well-known antiwear additive, however, in this study addition of ZDDP at a concentration of 0.2 wt% P resulted in poor antiwear performance. Phosphonium cation based ILs exhibits better antiwear performance than the choline cation based ILs. The P IL1 and P IL2 structure have the same phosphonium cation; however, there is a difference in the anionic moiety, P IL1 has thiophosphate anion while P IL2 has phosphate anion. In addition, P IL1 and P IL2 can be differentiated based on their chain length. P IL1 has a longer chain length than the P IL2. When wear behavior of P IL1 and P IL2 are compared, P IL1 exhibited better wear protection. In the case of choline cation based N IL1 and N IL2. N IL1 exhibited better tribological performance. N IL1 has thiophosphate while N IL2 has phosphate anion. This indicates that the functional group in the ionic liquid molecules plays a vital role in increasing the antiwear and antifriction ability. Interestingly, both the anions and the length of the alkyl chains of the ionic liquid molecules have a significant effect on the tribological properties.
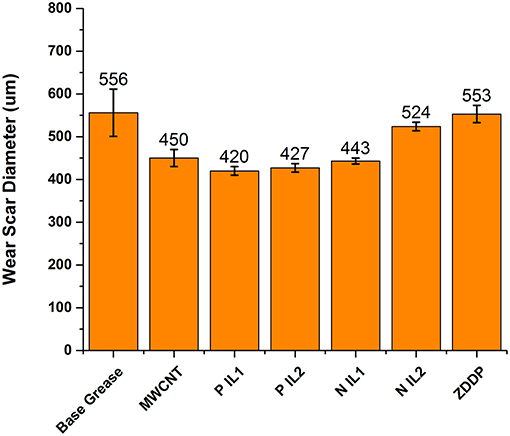
Figure 2. Wear scar diameters from four ball wear tests for greases containing only MWCNT and only ILs additives compared with base grease and ZDDP grease blend.
Analysis of Rubbed Surfaces for MWCNTs and ILs as an Additive
The morphology and characteristics of the wear tracks imaged using a scanning electron microscopy (SEM) provide significant information about the wear behavior of MWCNT and ILs based greases. Figures 3, 4 display wear scar surfaces magnified at 100X and 1,000X, respectively. SEM images of the wear scar at low magnification of 100X give an idea about the wear scar diameter and overall wear characteristic. While higher magnification images at 1,000X help to understand the nature of the wear/wear mechanism in play. The secondary electron SEM image [4(A)] of the wear scar for base grease exhibits severe plowing groves and adhesive wear surfaces. As no additives were present in the grease, direct metal to metal contact resulted in heavy wear. Greases with MWCNT has the smaller wear scar than base grease and ZDDP. Wear scar morphology exhibits deep abrasive grooves with some amount of polishing wear. In addition, the black color deposition can be seen near abrasive grooves and in deep scratches in the SEM image taken at 1,000X magnification [4(B)]. The worn surface lubricated by base grease blend has many wide and deep grooves and scuffing while the worn surface lubricated by grease with MWCNT at 1 wt% is relatively smoother and the grooves are not as deep. As detailed earlier MWCNTs tend to form thin, dry lubricant film covering the surface asperities and promoting smooth surfaces. It is possible that the MWCNT in contact with smooth surfaces may slip and slide and provide protection against high frictional forces. This explains the combined presence of abrasive grooves and polished smooth surfaces on the wear scars developed by grease blends containing MWCNT.
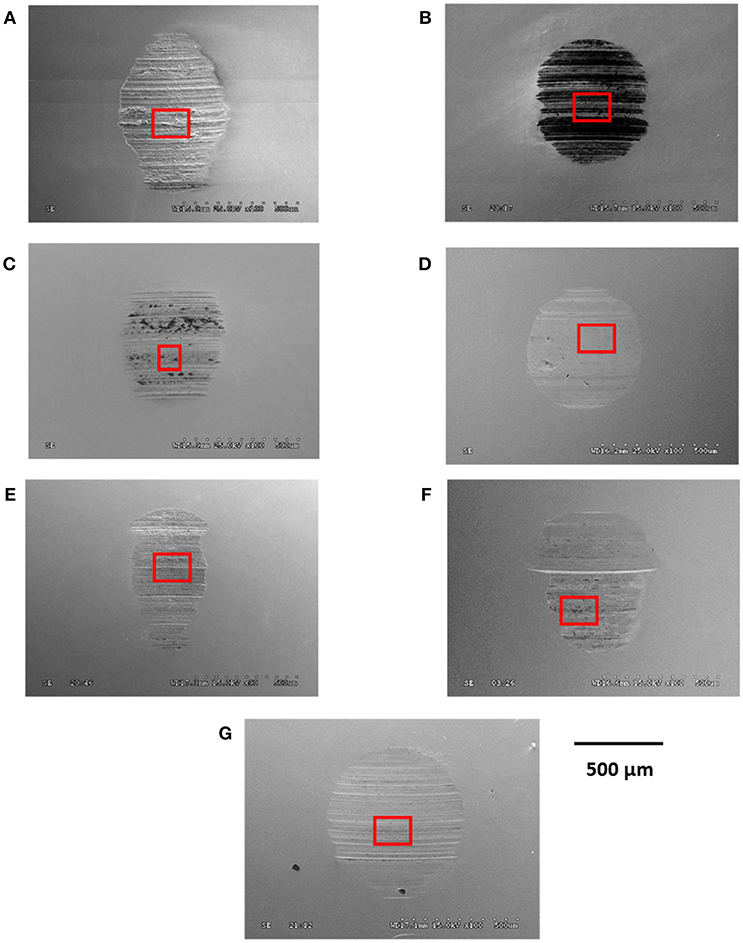
Figure 3. Secondary electron microscopy images at 100X magnification of the wear scars from four ball wear tests for grease blends. (A) Base grease (B) MWCNT (C) P IL1 (D) P IL2 (E) N IL1 (F) N IL2 (G) ZDDP.
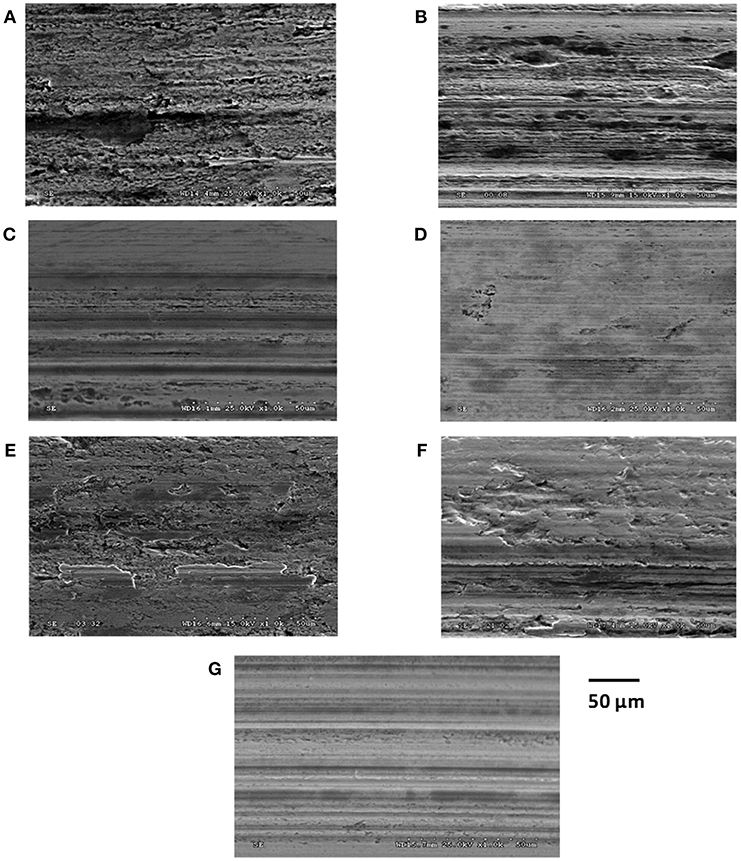
Figure 4. Secondary electron microscopy images at 1,000X magnification of the wear scars from four ball wear tests for grease blends. (A) Base grease (B) MWCNT (C) P IL1 (D) P IL2 (E) N IL1 (F) N IL2 (G) ZDDP.
Wear scars of P IL1 and ZDDP appears smooth as compared to P IL2, N IL1, and N IL2. The darker regions or patches in the high magnification SEM images possibly indicates the presence of non-conductive phosphates tribofilms. P IL1 SEM image [4(C)] shows dark patches throughout the wear scar which corresponds to the less conductive patchy tribofilms formation. The SEM image of P IL2 worn surface shows abrasive wear tracks and patchy tribofilm formation, with patch sizes that are larger than what is seen in P IL1. On the other hand, the wear surfaces observed in the case of N IL1 and N IL2 appears to be rougher than the P IL1 and P IL2. SEM image of ZDDP lubricated surface exhibits regions of abrasive wear with scratches aligned to the sliding direction together with the formation of patchy protective tribofilms. The analysis of the morphology of worn surfaces thus correlates to the wear results discussed earlier. P IL1 and P IL2 showed smaller WSD value than the N IL1 and N IL2.
Figure 5 represents the EDS spectrum obtained from the worn scar on the steel balls lubricated by base grease blend and grease blend containing 1 wt% MWCNTs, respectively. It can be seen that the element of C was present on the worn scar surface lubricated by lithium grease containing 1 wt% MWCNTs, and its atomic concentration was 18.56%. Comparison of spectrum carbon element data in Figure 5B with Figure 5A, indicates that the C present on the worn surfaces may be from the physical adsorption of MWCNTs during the process of friction. The higher concentration of C element suggests that a lubricating grease film must have formed and probably contained MWCNTs, which would have acted intermediary to prevent the direct metal-metal contact.
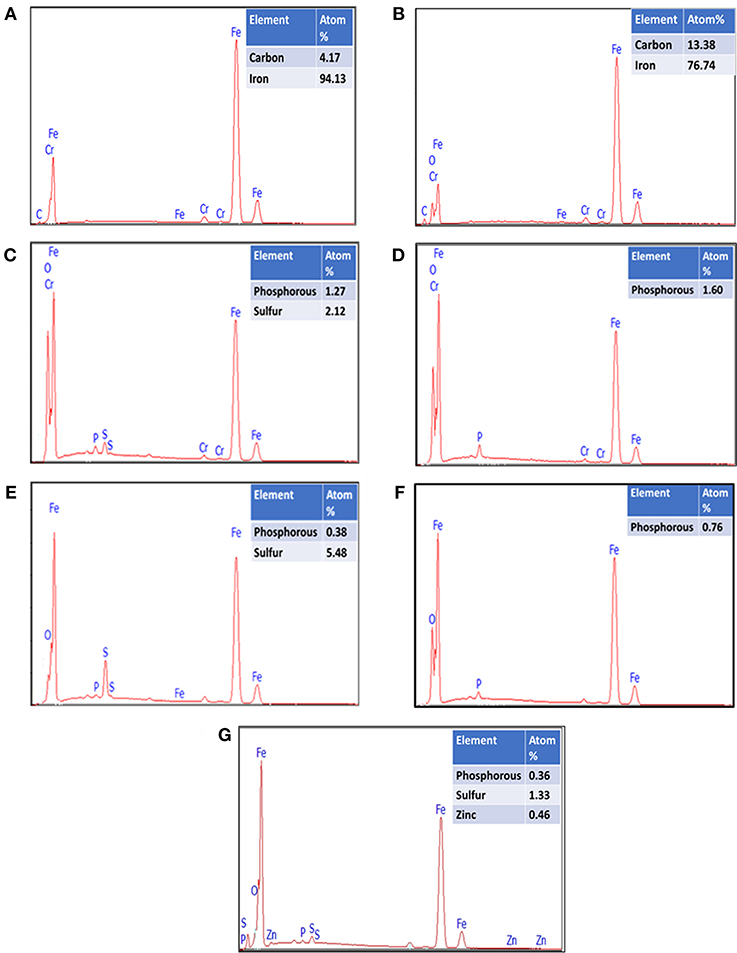
Figure 5. Energy dispersive spectroscopy spectrum of four ball wear scars for grease blends with antiwear additives (A) Base Grease (B) MWCNT (C) P IL1 (D) P IL2 (E) N IL1 (F) N IL2 (G) ZDDP.
Figure 5 also shows elemental spectrums for four ILs compared with ZDDP. All spectrums were obtained with the acquisition of approximately 120,000 net counts. Additionally, the table in Figure 5 details the amount of antiwear forming elements phosphorus and sulfur detected on the worn surface magnified at 1,000X. EDS spectra for P IL1 shows a large peak for S, Fe, O and low peak for P indicating the presence of a good amount of sulfur based films and smaller amounts of phosphorous based films. From Figures 5D,F it can be suggested that P IL2 and N IL2 results in the formation of iron phosphate based tribofilms. As the sulfur is not present in the original structure of P IL2 and N IL2, no sulfur peaks were determined from their lubricated worn surfaces. N IL2 EDS spectra demonstrate shorter peak of phosphorus than P IL2 and hence correlates well with the wear results of higher WSD than P IL2. N IL1 has the choline cation and dithiophosphate anion which results in detection of phosphorus and sulfur peaks (Note Figure 5E). Therefore, it can be postulated that the chemistry of the tribofilms formed on the worn surface is related to the original structure and anion moiety of the ILs. EDS spectra for ZDDP shows a strong signal for Zn, P, S, Fe, and O elements indicative of the formation of zinc phosphate, zinc sulfide/ sulfate, iron phosphate, iron sulfate/sulfide, and iron oxide compounds.
Tribological Mechanism of MWCNTs and ILs as an Additive
Many studies have investigated the lubricating mechanisms of nano-additives. The proposed suggestions behind the friction and wear reduction are mending effect (Liu et al., 2004), colloidal effect (Chiñas-Castillo and Spikes, 2003), rolling and ball bearing effect protective film (Tao et al., 1996), and third body material transfer (Tang and Li, 2014). In this work, several hypotheses can be made to explain the positive effect of MWCNT addition on tribological properties of the lithium grease. First, from the SEM-EDS mapping, it can be proposed that MWCNTs exhibits mending effect as the presence of carbon element was detected in the abrasive grooves developed on the worn surface. Second, EDS spectrum analysis suggests that MWCNT may get adsorbed on the rubbing surfaces and form thin films capable of preventing contact between the interacting surfaces. In addition to this, some MWCNTs can be ruptured or disintegrate under rigorous friction and extreme pressure condition to form small, planar graphene segments reflecting their origin, in effect resembling amorphous carbon. This newly formed lamella may strongly adhere to tribosurface and can further protect surfaces in motion. Such films are referred to as transfer films (Vander Wal et al., 2011). The role of the transfer film is to reduce the shear strength at the interface while maintaining the stiffness of the contact surfaces (Miyoshi et al., 2005). In all the cases, the benefit of MWCNT arises from their unique structure and properties. The high Young's modulus of MWCNT enables them to shear under high friction force. The rolling or sliding of MWCNTs between the interacting surfaces aid in reduced friction and improved wear resistance. In this study, though high C concentration was detected on worn surfaces, it is beyond the scope of this project to characterize the nature of the films. It may be transfer films or physically adsorbed films. However, it is safe to state that the MWCNTs can serve as effective spacers by getting adsorbed/accumulated on activated metal surfaces, prohibiting contact and wear of the metal under extreme pressure and load conditions.
This research study assessed the potential of phosphorous containing ILs as antiwear and antifriction additive under boundary lubrication regime. ILs exhibited better tribological performance than ZDDP. The good tribological performance of the ILs could be attributed to their unique dipolar structure. Owing to that ionic liquids could be very easily adsorbed on the friction contact pairs to form an effective boundary film. It is well-known that during the sliding process, low-energy electrons emit from contact convex points on the metal surface and the positive charge is formed at the surface of the tiny convex volume. The anion part of the ionic liquid can easily adsorb onto the positively charged sites of the metal worn surface. Under the boundary lubrication regime (used in this study), points at the surface asperity contacts generate very high temperature and pressure, which induces thermo-mechanical decomposition of the physiochemically adsorbed ionic liquid. These decomposition by-products react with the nascent metal surface to form a reaction film known as tribofilms (Martin et al., 1984; Fuller et al., 1997; Kim et al., 2010). The final byproduct of this reaction is deposited on the rubbing surface which ultimately protects the continued sliding condition. Therefore, it is safe to reason out that the ionic liquids undergo decomposition and tribo-chemically reacts with nascent Fe substrate to form tribofilms comprising of iron phosphate, iron sulfides/sulfates. The tribofilms act like a sacrificial film which periodically forms and break-down due to the shearing action during the process. Tribofilms formed on interacting surfaces provides low shearing strength, which leads to lower coefficient friction and excellent wear resistance (Fuller et al., 1997; Chen and Liu, 2001; Aktary et al., 2002; Qu et al., 2009a, 2014; Kim et al., 2010; Olomolehin et al., 2010; Somers et al., 2010; Otero et al., 2014; Sharma et al., 2015, 2016; Blanco et al., 2017). Many authors have proposed that the ionic liquids form tribofilms similar to ZDDP (Somers et al., 2013; Ghandi, 2014; Qu et al., 2014; Sharma et al., 2015). ZDDP forms tribofilms of zinc phosphates, zinc sulfides/sulfates, iron phosphate, and iron sulfides/sulfates and imparts excellent antiwear, and antifriction protection of surfaces in contact. However, it is important to note that in this study ZDDP didn't exhibit the comparable tribological performance to that of ILs. There are several possibilities for this performance, first may be the rate of film break down was higher than the rate of film formation by ZDDP. Secondly, it is possible that the concentration of ZDDP was insufficient to maintain the film formation throughout the test. Our group's research work on developing extreme pressure additive chemistries for greases suggests that optimal concentration for ZDDP is approximately 3 wt% to achieve effective improvement in antifriction and antiwear performance of the grease (Bagi and Aswath, 2015a; Bagi et al., 2018).
Evaluation of Tribological Properties of MWCNT-IL Hybrids as an Additive
Figure 6 exhibits friction spectra collected throughout tribological testing of grease blends containing MWCNT-IL additives. As anticipated, the average COF for base grease is highest. All grease blends with MWCNT-IL additives show improvement in friction response compared to base grease without any additives. Their friction profiles are a representation of presence and exclusion of films formed by additives. Friction profile of MWCNT-P IL1 was the most stable one throughout the test compared to rest of the grease blends, resulting in the lowest average COF values. Grease blend with MWCNT-P IL2 additive exhibited unstable friction profile at the initial and final phase of the test and exhibited better average COF value than base grease. Grease blends with MWCNT-N IL1 and MWCNT-N IL2 display low and stable COF, however, their friction profiles show sudden changes at certain points of the test. Grease blend with MWCNT-ZDDP additive performed better than base grease in terms of friction outcomes but displayed higher COF value as compared to other greases with MWCNT-IL additive mixture. Friction profile of MWCNT-ZDDP exhibited the most significant number of changes in COF till 30,000 revolutions. Comparison of Figure 6 with Figure 2 suggests improved antifriction behavior of MWCNT-IL greases than only MWCNT and only IL greases.
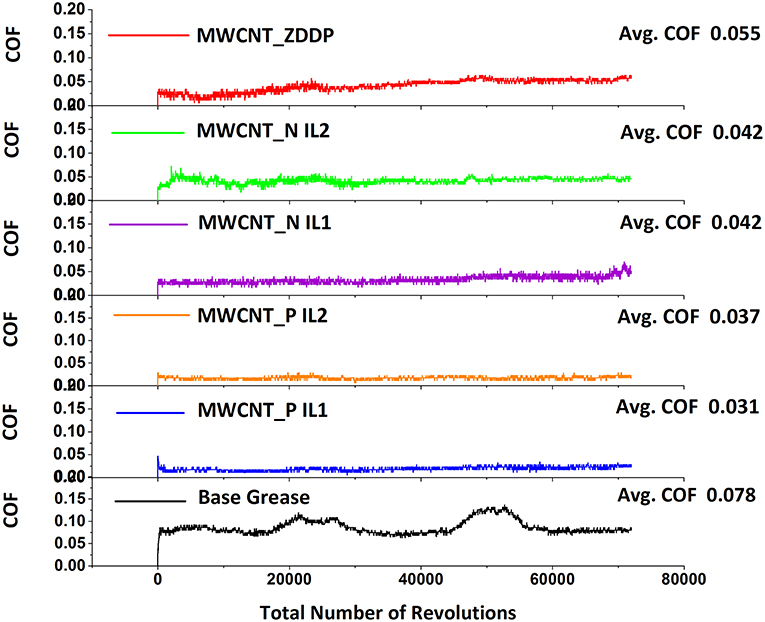
Figure 6. Coefficient of friction outcomes from four ball wear tests for greases with MWCNT and ILs additives compared with base grease and MWCNT_ZDDP grease blends.
Figure 7 provides a comparative representation of WSD values plotted in the form of a bar chart for greases containing either MWCNT-IL or IL. Wear behavior of base grease complimented the friction findings by displaying the highest wear outcomes. Grease blend with MWCNT-P IL1 and MWCNT-P IL2 additive showed small and almost comparable WSD values. Considering statistical significance, we can state that wear results with lubrication containing only P IL1 and P IL2 are almost similar to that of MWCNT- P IL1 and MWCNT-P IL2. Wear performance of MWCNT-N IL1 based grease is better than MWCNT-N IL2 but poorer than MWCNT-P IL1 and MWCNT-P IL2. This indicates that wear performance of phosphonium cation based ILs and MWCNT additive mixture is better than the choline cation based ILs and MWCNT additives. Additionally, MWCNT-ZDDP grease exhibited the highest WSD value of 620 μm and even worse wear behavior than the base grease. It is important to note that MWCNT-N IL greases resulted in higher wear as compared to greases containing only N IL. These observations clearly indicate that MWCNT in this binary additive system is not contributing positively toward enhancing wear preventive characteristics of lithium grease. It was hypothesized that non-covalent interaction of IL with MWCNTs will help to maintain a stable dispersion of MWCNTs in the grease system and hinder the formation of bundles of MWCNTs. Better distribution of the MWCNT will provide a larger solid-phase contribution to the lubrication system. At the same time, the potential of ILs to form protective tribofilms was expected to enhance the tribological properties of MWCNT-IL additive mixture. However, it appears that MWCNT and IL are not acting synergistically. To comprehend this behavior, the study of the worn surface morphology and tribo-chemistry is essential.
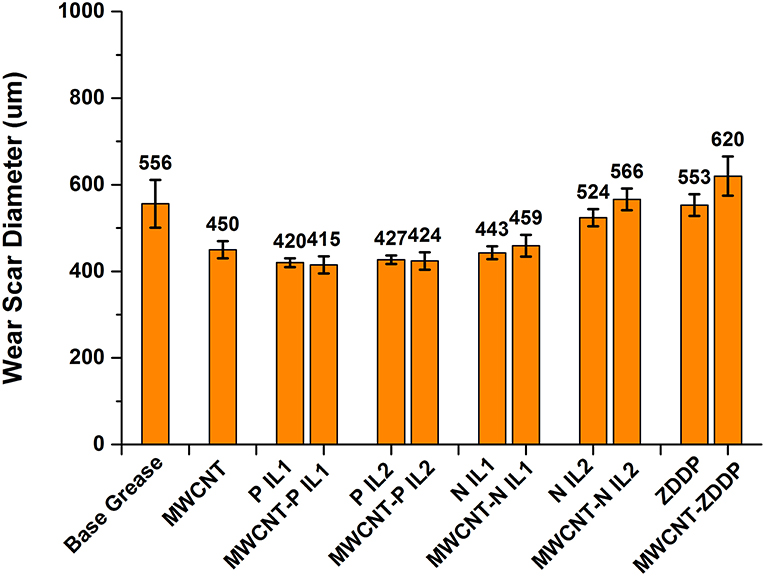
Figure 7. Wear scar diameter from four ball wear tests for greases with MWCNT and ILs additives compared with base grease and MWCNT_ZDDP grease blends.
Characterization of Worn Surfaces for MWCNT-IL Hybrids as an Additive
Secondary electron SEM images of rubbed surfaces formed on stationary balls were imaged using S-3000N variable pressure SEM. Figure 8 displays secondary emission images of worn surfaces for all four MWCNT-IL grease formulations taken at 100X and 1,000X. Low magnification SEM images of all grease blends display smooth polished wear surfaces. Higher magnification image at 1,000X details the nature of wear mechanism taking place. Deposition of the MWCNTs in the plowing grooves can be seen in the low magnification image of MWCNT-P IL1. Dark patches seen on the magnified wear surface indicates the presence of continuous thin layer tribofilms. This explains the lower COF and WSD value for grease blend containing MWCNT-P IL1 as an additive. Higher magnification image of grease blend containing MWCNT-P IL2 as an additive shows severe delamination and adhesive wear. Sharma et al. studied the tribological performance of phosphonium cation based ILs in mineral base oil and reported a similar type of wear tracks with uneven distribution of formed antiwear films and the roughness oriented in the direction of rubbing (Sharma et al., 2015). The lubricating grease containing N IL1 and MWCNTs exhibits the smearing type of adhesive wear with deep scratches all over the worn surface. Abrasive wear along with some polishing wear tracks and smooth patches of tribofilms can be observed in the direction of sliding for the rubbed surfaces generated by grease formulations containing MWCNT-N IL2. Also, black color deposition can be seen in grooves on wear tracks.
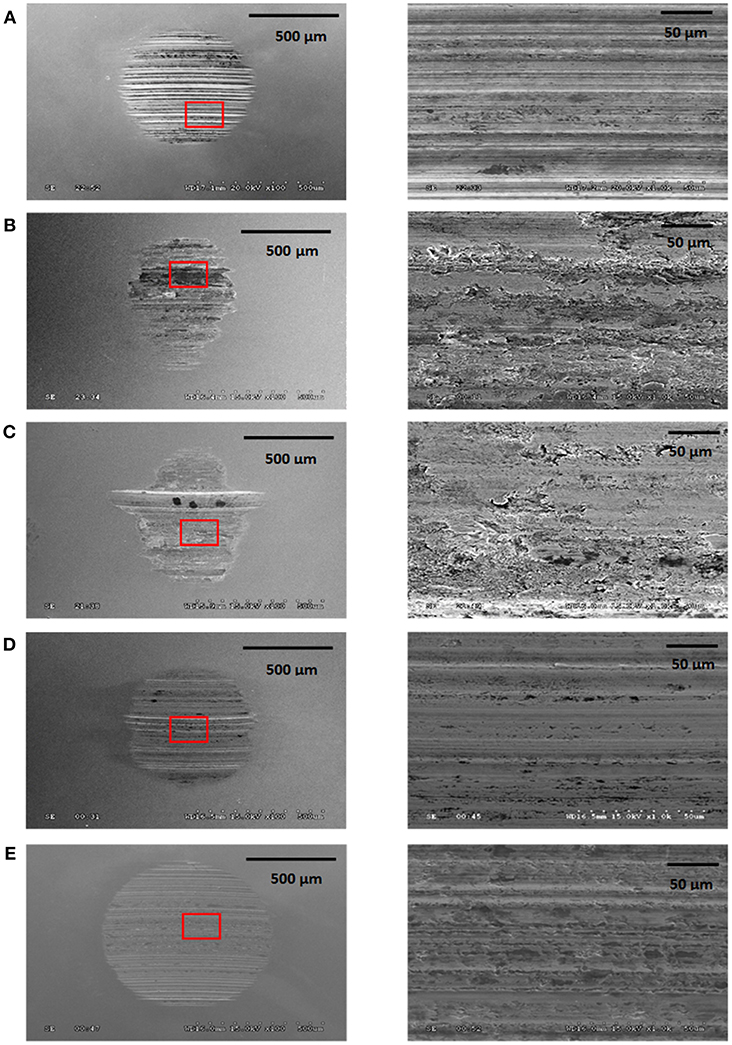
Figure 8. Secondary scanning electron microscopy images of four ball wear scars at 100X and 1,000X for grease blends (A) MWCNT_P IL1 (B) MWCNT_P IL2 (C) MWCNT_N IL1 (D) MWCNT_N IL2 (E) MWCNT_ZDDP.
The wear surface of MWCNT-N IL2 blend appears to be rougher than MWCNT-N IL1. Morphological analysis of worn surfaces thus correlates to the wear results discussed earlier. MWCNT-N IL2 showed higher WSD value than the MWCNT-N IL1. Additionally, wear surface lubricated by ZDDP and MWCNTs based grease formulation appears to be more abrasive as opposed to samples from all MWCNT-IL based grease formulations. Unevenly distributed dark patches covering scratches on the SEM image indicates the presence of non-conductive ZDDP antiwear tribofilm (Figure 8D).
Wear scar areas magnified at 1,000X shown in Figure 9 were subjected to EDS analysis to determine elemental distribution in the worn surface. Figure 9 exhibits elemental spectrums for four ILs compared with ZDDP. All spectrums were obtained with the acquisition of approximately 120,000 total counts. EDS spectrum of wear surface lubricated by grease blend MWCNT_P IL1 shows the presence of sulfur, phosphorous, oxygen, carbon, calcium, and iron. It has been reported in the literature that inherent polar molecules of ILs tribo-chemically react with metallic surfaces to form lubricative boundary surface film (Qu et al., 2014). Therefore, detection of antiwear tribofilm elements like P, S, and Fe suggest the formation of iron phosphate, iron sulfates and iron sulfides films on wear surface due to the chemical interaction of decomposed P IL1 with the steel surface. The presence of 21.37 atom % of carbon in the wear surface of MWCNT_P IL1 suggests that MWCNTs are physically adsorbed or accumulated on wear track's cracks and grooves. Identification of P, O, and Fe elements on the worn surfaces developed by MWCNT-P IL2 based grease formulation indicates that P IL2 formed tribofilms are comprised of only iron phosphates. Similar assessments of the presence of iron phosphates and MWCNTs on wear surface can be made for grease blend containing MWCNT-N IL1 additives. EDS spectra for MWCNT-N IL1 based grease formulation exhibits a low intensity peak of phosphorous with a high intensity peak of the sulfur element. This suggests the likely presence of iron sulfates, iron sulfides, and iron phosphates films on wear surface. These observations are in accordance with the findings of an extensive study by Sharma et al. on ILs, who reported using X-ray absorption near edge spectroscopy (XANES) that phosphorus and sulfur based ILs form pad like glassy tribofilms very similar to ZDDP with only one difference of presence of Fe cation coordinated compounds instead of Zn cations (Sharma et al., 2015, 2016). Analysis of data from EDS does not predict the coordination of elements like carbon and but one can make a rational conclusion based on possible reaction pathways. Recorded atom % of carbon can be from multiple sources like MWCNTs, and lithium grease. Comparison of SEM images and EDS elemental mapping can provide better evidence about the location of detected elements on wear surfaces.
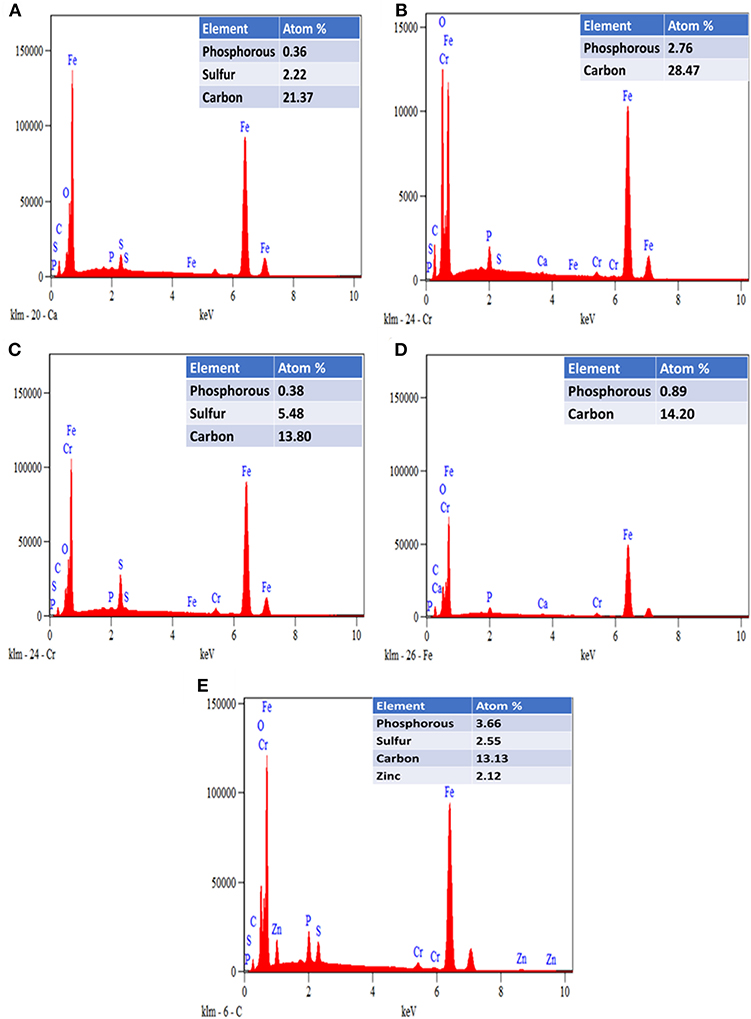
Figure 9. Energy dispersive spectroscopy spectra of four ball wear scars for grease blends with (A) MWCNT_P IL1 (B) MWCNT_P IL2 (C) MWCNT_N IL1 (D) MWCNT_N IL2 (E) MWCNT_ZDDP.
EDS mapping was carried out to understand tribo-chemistry of interacting surfaces lubricated with MWCNT-IL additive mixture. EDS map of the rubbed surface generated through lubrication of grease blend having MWCNTs and P IL1 as additives were selected for discussion because it showed best tribological performance among all grease formulations. Figure 10 represents EDS mapping with a corresponding SEM image for the rubbed surface. The box marked on the SEM image is the representative of the area under consideration. Bright colorful patches on O and P map indicates the presence of phosphates surface films. The smooth surface area in the marked box corresponding to strong intensity patches in S map suggests the presence of thick sulfates/sulfides films protecting steel substrate against frictional forces in the direction of sliding. Also, traces of carbon element on rough surfaces can be seen; however, it appears like MWCNTs are simply accumulated in grooves of wear track, no adsorbed film of MWCNTs is observed. As mentioned before EDS analysis can't give the definite source of carbon element and advanced characterization techniques like XPS, Raman and XANES should be used in correlation to EDS data to comment on the identification of adsorbed MWCNTs films on worn surfaces.
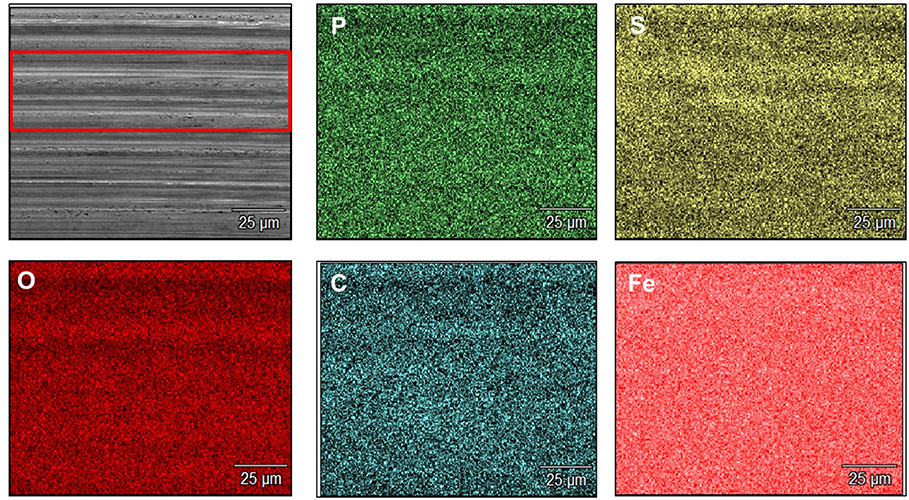
Figure 10. Energy dispersive spectroscopy mapping for wear scar from four ball wear tests of grease blend with MWCNT_P IL1 at a magnification of 1,000X.
Tribological Mechanism of MWCNT-IL Hybrids as an Additive
Interacting surfaces in boundary lubrication regime move against each other under high pressure and low speed, resulting in severe collision of contacting surface asperities. These interactions of asperities lead to both plastic and elastic deformation of surfaces and under such circumstances, physical and chemical properties of lubricating medium play a critical role in providing enhanced surface protection against friction and wear. In this research work, a mixed solid-liquid additive system of MWCNT-IL was prepared to enhance the tribological performance of lithium greases, and an effort was made to understand lubrication and wear mechanism of MWCNT-IL additives.
Examination of frictional and wear behavior of MWCNT-IL based greases and SEM-EDS analysis suggests that both MWCNT and IL, are specifically antagonistic in terms of wear. Findings of the SEM-EDS indicates the presence of MWCNTs nanoparticles in the wear tracks as physically adsorbed on nascent surfaces or as accumulated in rough surface grooves. Residual MWCNTs in wear tracks can minimize shear strength and surface energy of interface and minimize COF and wear of surfaces in contact (Zhang et al., 2017). Frictional resistance can be minimized due to rolling or sliding motion of cylindrical MWCNTs present at the interface (Ni and Sinnott, 2001; Peng et al., 2007). Additionally, excellent mechanical properties of MWCNTs like high Young's modulus and tensile strength can minimize the real area of contact, which can results in lower friction coefficient at surface asperity tips.
EDS spectrum analysis of MWCNT-ILs lubricated worn surface evidently detects the presence of P, S, Fe, and O rich tribofilms and suggests that ILs form protective antiwear tribofilms under boundary lubrication regime by reacting with nascent Fe substrate. However, comparison of EDS P elemental data in Figures 6, 10 indicates that less phosphate based antiwear films were present on MWCNT-IL lubricated worn surfaces than that of only ILs. There are two possible inferences from this observation, (i) MWCNTs in grease additive system are acting as a third body and directly abrading off IL formed tribofilms by mechanical action or (ii) MWCNTs disrupts the formation of tribofilms after initial removal by accumulating/physically adsorbing on the active nascent steel substrate. Tribofilms are reported to have less hardness than the steel substrate (Aktary et al., 2002; Olomolehin et al., 2010) and hence, can get easily removed by hard CNTs. As tribofilms are strongly adhered and assimilated with the nascent steel surface, removal of the antiwear films also results in the removal of the substrate. Determining exact modes of interaction is beyond the scope of this project. It is important to note that MWCNT does not appear to directly abrade steel ball surface as evident from the tribological results of greases containing only MWCNT. However, a significant decrease in P element (Figures 6, 10) and increase in WSD values (Figure 7) for MWCNT- ILs greases as opposed to only IL greases confirms that MWCNT is antagonistically interacting with ILs/IL formed tribofilms and is possibly responsible for the poor tribological performance of the MWCNT-IL hybrid greases.
Conclusion
1. Grease chemistries with MWCNTs and four different phosphorous based ILs hybrids were successfully prepared. Physical non-covalent interaction of IL and MWCNTs assisted in maintaining a stable dispersion of MWCNTs in grease mixture.
2. Initial tribological experiments helped to understand the lubricating mechanism of MWCNTs and ILs under boundary lubrication condition. MWCNTs effectively improved tribological behavior of lithium base greases by physically adsorbing and/ accumulating on wear-prone interacting surfaces.
3. Four phosphorous based ILs used in this study showed superior antiwear characteristics compared with the conventional ZDDP. Phosphonium cation based ILs exhibit better tribological performance than choline based ILs. Friction and wear results indicate that tribological performance depends upon the type of anion moiety and alkyl chain length of ILs. SEM-EDS analysis suggests that IL do participate in the tribochemical reaction during the rubbing action and form antiwear phosphate and sulfide/sulfate based films on interacting surfaces by tribo-chemically interacting with Fe.
4. The potential of MWCNT-IL hybrids as an antiwear and antifriction additive for lithium complex greases was evaluated. Hybrids of MWCNTs and phosphonium cation based ILs successfully improved lithium base grease friction coefficient by 60% and wear scar diameter by 25%. Tribological mechanism of MWCNT-IL additive based greases elucidated through SEM-EDS analysis indicates that IL phase in MWCNT-IL additive mixture experienced tribochemical reaction in the sliding of steel against steel, with the formation of a surface protective tribofilm. Whereas, MWCNTs solid additive phase contribution is attributed to its intrinsic physical and mechanical property.
5. Wear performance of the MWCNT-IL based greases was poor/comparable to that of the greases containing only ILs or MWCNT. SEM-EDS results suggest possible antagonistic interaction between MWCNT and IL. EDS spectrum comparison of only IL lubricated worn surfaces with MWCNT-IL indicate less protective phosphorous based IL tribofilms were formed when MWCNT is present in the grease mixture. It is hypothesized that MWCNTs act as a third body and interacts with ILs active species/IL tribofilms to hinder the antiwear performance of ILs.
Author Contributions
KV designed this research work, conducted the experiments, and wrote the manuscript. PA supervised all aspects of this research work and reviewed the paper.
Conflict of Interest Statement
The authors declare that the research was conducted in the absence of any commercial or financial relationships that could be construed as a potential conflict of interest.
Acknowledgments
The authors appreciate AC2T research GmbH for providing ionic liquids used in this study. Assistance provided by Dr. Nicole Doerr is gratefully acknowledged. Experimental facilities for SEM and EDS were conducted at the Center of characterization for materials and biology (CCMB), University of Texas at Arlington.
References
Aktary, M., McDermott, M. T., and McAlpine, G. A. (2002). Morphology and nanomechanical properties of ZDDP antiwear films as a function of tribological contact time. Tribol. Lett. 12, 155–162. doi: 10.1023/A:1014755123184
An, V., Irtegov, Y., and de Izarra, C. (2014). Study of Tribological properties of nanolamellar WS 2 and MoS 2 as additives to lubricants. J. Nanomater. 2014:865839. doi: 10.1155/2014/865839
Bagi, S., Vyavhare, K., and Aswath, P. B. (2018). Tribological characteristics of greases with and without metallo-organic friction-modifiers. Tribol. Mater. Surfaces Interfaces 12, 223–236. doi: 10.1080/17515831.2018.1542790
Bagi, S. D., and Aswath, P. (2015a). Mechanism of friction and wear in MoS2 and ZDDP/F-PTFE greases under spectrum loading conditions. Lubricants 3, 687–711. doi: 10.3390/lubricants3040687
Bagi, S. D., and Aswath, P. B. (2015b). Role of MoS2 morphology on wear and friction under spectrum loading conditions. Lubr. Sci. 27, 429–449. doi: 10.1002/ls.1296
Blanco, D., González, R., Viesca, J. L., Fernández-González, A., Bartolomé, M., and Hernández Battez, A. (2017). Antifriction and antiwear properties of an ionic liquid with fluorine-containing anion used as lubricant additive. Tribol. Lett. 65:66. doi: 10.1007/s11249-017-0846-4
Carrión, F. J., Espejo, C., Sanes, J., and Bermúdez, M. D. (2010). Single-walled carbon nanotubes modified by ionic liquid as antiwear additives of thermoplastics. Compos. Sci. Technol. 70, 2160–2167. doi: 10.1016/j.compscitech.2010.08.018
Chen, S., and Liu, W. (2001). Characterization and antiwear ability of non-coated ZnS nanoparticles and DDP-coated ZnS nanoparticles. Mater. Res. Bull. 36, 137–143. doi: 10.1016/S0025-5408(00)00477-3
Chen, S., and Liu, W. (2006). Oleic acid capped PbS nanoparticles: synthesis, characterization and tribological properties. Mater. Chem. Phys. 98, 183–189. doi: 10.1016/j.matchemphys.2005.09.043
Chiñas-Castillo, F., and Spikes, H. A. (2003). Mechanism of action of colloidal solid dispersions. J. Tribol. 125, 552–557. doi: 10.1115/1.1537752
Chun, K.-Y., Oh, Y., Rho, J., Ahn, J.-H., Kim, Y.-J., Choi, H. R., et al. (2010). Highly conductive, printable and stretchable composite films of carbon nanotubes and silver. Nat. Nanotechnol. 5, 853–857. doi: 10.1038/nnano.2010.232
Cursaru, D.-L., Andronescu, C., Pirvu, C., and Ripeanu, R. (2012). The efficiency of Co-based single-wall carbon nanotubes (SWNTs) as an AW/EP additive for mineral base oils. Wear 290–291, 133–139. doi: 10.1016/j.wear.2012.04.019
de Castro, C. A., Lourenço, M. J. V., Ribeiro, A. P. C., Langa, E., Vieira, S. I. C., Goodrich, P., et al. (2010). Thermal properties of ionic liquids and ionanofluids of imidazolium and pyrrolidinium liquids. J. Chem. Eng. Data 55, 653–661. doi: 10.1021/je900648p
Dong, J., and Hu, Z. (1998). A study of the anti-wear and friction-reducing properties of the lubricant additive, nanometer zinc borate. Tribol. Int. 31, 219–223. doi: 10.1016/S0301-679X(98)00017-6
Fukushima, T., and Aida, T. (2007). Ionic liquids for soft functional materials with carbon nanotubes. Chem. A Eur. J. 13, 5048–5058. doi: 10.1002/chem.200700554
Fukushima, T., Kosaka, A., Ishimura, Y., Yamamoto, T., Takigawa, T., Ishii, N., et al. (2003). Molecular ordering of organic molten salts trigged by single-walled carbon nanotubes. Science 300, 2072–2074. doi: 10.1126/science.1082289
Fukushima, T., Kosaka, A., Yamamoto, Y., Aimiya, T., Notazawa, S., Takigawa, T., et al. (2006). Dramatic effect of dispersed carbon nanotubes on the mechanical and electroconductive properties of polymers derived from ionic liquids. Small 2, 554–560. doi: 10.1002/smll.200500404
Fuller, M., Yin, Z., Kasrai, M., Bancroft, G. M., Yamaguchi, E. S., Ryason, P. R., et al. (1997). Chemical characterization of tribochemical and thermal films generated from neutral and basic ZDDPs using X-ray absorption spectroscopy. Tribol. Int. 30, 305–315. doi: 10.1016/S0301-679X(96)00059-X
Gabler, C., Tomastik, C., Brenner, J., Pisarova, L., Doerr, N., and Allmaier, G. (2011). Corrosion properties of ammonium based ionic liquids evaluated by SEM-EDX, XPS and ICP-OES. Green Chem. 13, 2869–2877. doi: 10.1039/c1gc15148g
Ghandi, K. (2014). A review of ionic liquids, their limits and applications. Green Sustain. Chem. 4, 44–53. doi: 10.4236/gsc.2014.41008
Guo, Y.-B., and Zhang, S.-W. (2016). The Tribological properties of multi-layered graphene as additives of PAO2 oil in steel–steel contacts. Lubricants 4:30. doi: 10.3390/lubricants4030030
Hao, L., Li, J., Xu, X., and Ren, T. (2010). Preparation, Characterization, and tribological evaluation of triethanolamine monooleate-modified lanthanum borate nanoparticles. Proc. Inst. Mech. Eng. Part J J. Eng. Tribol. 224, 1163–1171. doi: 10.1243/13506501JET817
Hong, S. H., Tung, T. T., Huyen Trang, L. K., Kim, T. Y., and Suh, K. S. (2010). Preparation of single-walled carbon nanotube (SWNT) gel composites using poly(ionic liquids). Colloid Polym. Sci. 288, 1013–1018. doi: 10.1007/s00396-010-2229-3
Hu, Z., Dong, J., and Chen, G. (1998). Study on antiwear and reducing friction additive of nanometer ferric oxide. Tribol. Int. 31, 355–360. doi: 10.1016/S0301-679X(98)00042-5
Hu, Z. S., and Dong, J. X. (1998). Study on antiwear and reducing friction additive of nanometer titanium borate. Wear 216, 87–91. doi: 10.1016/S0043-1648(97)00249-4
Huang, H. D., Tu, J. P., Gan, L. P., and Li, C. Z. (2006). An investigation on tribological properties of graphite nanosheets as oil additive. Wear 261, 140–144. doi: 10.1016/j.wear.2005.09.010
Ilie, F., and Covaliu, C. (2016). Tribological properties of the lubricant containing titanium dioxide nanoparticles as an additive. Lubricants 4:12. doi: 10.3390/lubricants4020012
Ji, X., Chen, Y., Zhao, G., Wang, X., and Liu, W. (2011). Tribological properties of CaCO 3 nanoparticles as an additive in lithium grease. Tribol. Lett. 41, 113–119. doi: 10.1007/s11249-010-9688-z
Jiménez, A. E., and Bermúdez, M. D. (2007). Ionic liquids as lubricants for steel-aluminum contacts at low and elevated temperatures. Tribol. Lett. 26, 53–60. doi: 10.1007/s11249-006-9182-9
Jiménez, A. E., Bermúdez, M. D., Carrión, F. J., and Martínez-Nicolás, G. (2006). Room temperature ionic liquids as lubricant additives in steel-aluminium contacts: influence of sliding velocity, normal load and temperature. Wear 261, 347–359. doi: 10.1016/j.wear.2005.11.004
Kamel, B. M., Mohamed, A., EL Sherbiny, M., and Abed, K. A. (2016). Tribological behaviour of calcium grease containing carbon nanotubes additives. Indust. Lubr. Tribol. 68, 723–728. doi: 10.1108/ILT-12-2015-0193
Kim, B., Mourhatch, R., and Aswath, P. B. (2010). Properties of tribofilms formed with ashless dithiophosphate and zinc dialkyl dithiophosphate under extreme pressure conditions. Wear 268, 579–591. doi: 10.1016/j.wear.2009.10.004
Kinzig, B. J., Sutor, P., Sawyer, G. W., Rennie, A., Dickrell, P., and Gresham, J. (2005). “Novel ionic liquid lubricants for aerospace and MEMS,” in Proceedings of World Tribology Congress, World Tribology Congress III (Washington, DC), 509–510. doi: 10.1115/WTC2005-63744
Kobayashi, K., Hironaka, S., Tanaka, A., Umeda, K., Iijima, S., Yudasaka, M., et al. (2005). Additive effect of carbon nanohorn on grease lubrication properties. J. Japan Pet. Inst. 48, 121–126. doi: 10.1627/jpi.48.121
Lee, C.-G., Hwang, Y.-J., Choi, Y.-M., Lee, J.-K., Choi, C., and Oh, J.-M. (2009). A study on the tribological characteristics of graphite nano lubricants. Int. J. Precis. Eng. Manuf. 10, 85–90. doi: 10.1007/s12541-009-0013-4
Liu, G., Li, X., Qin, B., Xing, D., Guo, Y., and Fan, R. (2004). Investigation of the mending effect and mechanism of copper nano-particles on a tribologically stressed surface. Tribol. Lett. 17, 961–966. doi: 10.1007/s11249-004-8109-6
Liu, W., and Chen, S. (2000). An investigation of the tribological behaviour of surface-modified ZnS nanoparticles in liquid paraffin. Wear 238, 120–124. doi: 10.1016/S0043-1648(99)00344-0
Liu, X. Q., Zhou, F., Liang, Y. M., and Liu, W. M. (2006). Tribological performance of phosphonium based ionic liquids for an aluminum-on-steel system and opinions on lubrication mechanism. Wear 261, 1174–1179. doi: 10.1016/j.wear.2006.03.018
López-Sánchez, F., Otero, I., López, E. R., and Fernández, J. (2017). Tribological Behavior of Two 1-Ethyl-3-methylimidazolium Alkyl Sulfates as Neat Lubricants for a Steel–Steel Contact. Tribol. Trans. 60, 729–738. doi: 10.1080/10402004.2016.1207829
Lu, Q., Wang, H., Ye, C., Liu, W., and Xue, Q. (2004). Room temperature ionic liquid 1-ethyl-3-hexylimidazolium- bis(trifluoromethylsulfonyl)-imide as lubricant for steel-steel contact. Tribol. Int. 37, 547–552. doi: 10.1016/j.triboint.2003.12.003
Martin, J. M., Mansot, J. L., Berbezier, I., and Dexpert, H. (1984). The nature and origin of wear particles from boundary lubrication with a zinc dialkyl dithiophosphate. Wear 93, 117–126. doi: 10.1016/0043-1648(84)90064-4
Minami, I., Inada, T., Sasaki, R., and Nanao, H. (2010). Tribo-chemistry of phosphonium-derived ionic liquids. Tribol. Lett. 40, 225–235. doi: 10.1007/s11249-010-9626-0
Miyoshi, K., Street, K. W., Vander Wal, R. L., Andrews, R., and Sayir, A. (2005). Solid lubrication by multiwalled carbon nanotubes in air and in vacuum. Tribol. Lett. 19, 191–201. doi: 10.1007/s11249-005-6146-4
Mohamed, A., Osman, T. A., Khattab, A., and Zaki, M. (2014). Tribological behavior of carbon nanotubes as an additive on lithium grease. J. Tribol. 137, 011801. doi: 10.1115/1.4028225
Mu, Z., Wang, X., Zhang, S., Liang, Y., Bao, M., and Liu, W. (2008). Investigation of tribological behavior of Al–Si alloy against steel lubricated with ionic liquids of 1-diethylphosphonyl-n-propyl-3- alkylimidazolium tetrafluoroborate. J. Tribol. 130:034501. doi: 10.1093/her/cyn014
Mu, Z., Zhou, F., Zhang, S., Liang, Y., and Liu, W. (2005). Effect of the functional groups in ionic liquid molecules on the friction and wear behavior of aluminum alloy in lubricated aluminum-on-steel contact. Tribol. Int. 38, 725–731. doi: 10.1016/j.triboint.2004.10.003
Mukai, K., Asaka, K., Sugino, T., Kiyohara, K., Takeuchi, I., Terasawa, N., et al. (2009). Highly conductive sheets from millimeter-long single-walled carbon nanotubes and ionic liquids: application to fast-moving, low-voltage electromechanical actuators operable in air. Adv. Mater. Weinheim. 21, 1582–1585. doi: 10.1002/adma.200802817
Ni, B., and Sinnott, S. B. (2001). Tribological properties of carbon nanotube bundles predicted from atomistic simulations. Surf. Sci. 487, 87–96. doi: 10.1016/S0039-6028(01)01073-1
Olomolehin, Y., Kapadia, R., and Spikes, H. (2010). Antagonistic interaction of antiwear additives and carbon black. Tribol. Lett. 37, 49–58. doi: 10.1007/s11249-009-9489-4
Otero, I., López, E. R., Reichelt, M., Villanueva, M., Salgado, J., and Fernández, J. (2014). Ionic liquids based on phosphonium cations as neat lubricants or lubricant additives for a steel/steel contact. ACS Appl. Mater. Interfaces 6, 13115–13128. doi: 10.1021/am502980m
Peng, Y., Hu, Y., and Wang, H. (2007). Tribological behaviors of surfactant-functionalized carbon nanotubes as lubricant additive in water. Tribol. Lett. 25, 247–253. doi: 10.1007/s11249-006-9176-7
Phillips, B. S., and Zabinski, J. S. (2004). Ionic liquid lubrication effects on ceramics in a water environment. Tribol. Lett. 17, 533–541. doi: 10.1023/B:TRIL.0000044501.64351.68
Pisarova, L., Gabler, C., Dörr, N., Pittenauer, E., and Allmaier, G. (2012). Thermo-oxidative stability and corrosion properties of ammonium based ionic liquids. Tribol. Int. 46, 73–83. doi: 10.1016/j.triboint.2011.03.014
Qiu, S., Zhou, Z., Dong, J., and Chen, G. (2001). Preparation of Ni nanoparticles and evaluation of their tribological performance as potential additives in oils. J. Tribol. 123, 441–443. doi: 10.1115/1.1286152
Qu, J., Bansal, D. G., Yu, B., Howe, J. Y., Luo, H., Dai, S., et al. (2012). Antiwear performance and mechanism of an oil-miscible ionic liquid as a lubricant additive. ACS Appl. Mater. Interfaces 4, 997–1002. doi: 10.1021/am201646k
Qu, J., Blau, P. J., Dai, S., Luo, H., and Meyer, H. M. (2009a). Ionic liquids as novel lubricants and additives for diesel engine applications. Tribol. Lett. 35, 181–189. doi: 10.1007/s11249-009-9447-1
Qu, J., Blau, P. J., Dai, S., Luo, H., Meyer, H. M., and Truhan, J. J. (2009b). Tribological characteristics of aluminum alloys sliding against steel lubricated by ammonium and imidazolium ionic liquids. Wear 267, 1226–1231. doi: 10.1016/j.wear.2008.12.038
Qu, J., Chi, M., Meyer, H. M., Blau, P. J., Dai, S., and Luo, H. (2011). Nanostructure and composition of tribo-boundary films formed in ionic liquid lubrication. Tribol. Lett. 43, 205–211. doi: 10.1007/s11249-011-9800-z
Qu, J., Luo, H., Chi, M., Ma, C., Blau, P. J., Dai, S., et al. (2014). Comparison of an oil-miscible ionic liquid and ZDDP as a lubricant anti-wear additive. Tribol. Int. 71, 88–97. doi: 10.1016/j.triboint.2013.11.010
Qu, J., Truhan, J. J., Dai, S., Luo, H., and Blau, P. J. (2006). Ionic liquids with ammonium cations as lubricants or additives. Tribol. Lett. 22, 207–214. doi: 10.1007/s11249-006-9081-0
Sharma, V., Doerr, N., and Aswath, P. B. (2016). Chemical–mechanical properties of tribofilms and their relationship to ionic liquid chemistry. RSC Adv. 6, 22341–22356. doi: 10.1039/C6RA01915C
Sharma, V., Gabler, C., Doerr, N., and Aswath, P. B. (2015). Mechanism of tribofilm formation with P and S containing ionic liquids. Tribol. Int. 92, 353–364. doi: 10.1016/j.triboint.2015.07.009
Sharma, V., Timmons, R., Erdemir, A., and Aswath, P. B. (2017). Plasma-Functionalized Polytetrafluoroethylene Nanoparticles for Improved Wear in Lubricated Contact. ACS Appl. Mater. Interfaces 9, 25631–25641. doi: 10.1021/acsami.7b06453
Somers, A., Howlett, P., MacFarlane, D., and Forsyth, M. (2013). A review of ionic liquid lubricants. Lubricants 1, 3–21. doi: 10.3390/lubricants1010003
Somers, A. E., Howlett, P. C., Sun, J., MacFarlane, D. R., and Forsyth, M. (2010). Phosphonium ionic liquids as lubricants for aluminium-steel. WIT Trans. Eng. Sci. 66, 273–283. doi: 10.2495/TD100231
Subramaniam, K., Das, A., and Heinrich, G. (2011). Development of conducting polychloroprene rubber using imidazolium based ionic liquid modified multi-walled carbon nanotubes. Compos. Sci. Technol. 71, 1441–1449. doi: 10.1016/j.compscitech.2011.05.018
Sunqing, Q., Junxiu, D., and Guoxu, C. (2000). Wear and friction behaviour of CaCO3 nanoparticles used as additives in lubricating oils. Lubr. Sci. 12, 205–212. doi: 10.1002/ls.3010120207
Tang, Z., and Li, S. (2014). A review of recent developments of friction modifiers for liquid lubricants (2007-present). Curr. Opin. Solid State Mater. Sci. 18, 119–139. doi: 10.1016/j.cossms.2014.02.002
Tao, X., Jiazheng, Z., and Kang, X. (1996). The ball-bearing effect of diamond nanoparticles as an oil additive. J. Phys. D Appl. Phys. 29, 2932–2937. doi: 10.1088/0022-3727/29/11/029
Tarasov, S., Kolubaev, A., Belyaev, S., Lerner, M., and Tepper, F. (2002). Study of friction reduction by nanocopper additives to motor oil. Wear 252, 63–69. doi: 10.1016/S0043-1648(01)00860-2
Tunckol, M., Durand, J., and Serp, P. (2012). Carbon nanomaterial–ionic liquid hybrids. Carbon N. Y. 50, 4303–4334. doi: 10.1016/j.carbon.2012.05.017
Vander Wal, R. L., Street, K. W., and Miyoshi, K. (2011). Transfer layers: a comparison across SWNTs, DWNTs, graphite, and an ionic fluid. Adv. Tribol. 2011:929642. doi: 10.1155/2011/929642
Wang, B. G., Wang, X. B., Lou, W. J., and Hao, J. C. (2010). Rheological and tribological properties of ionic liquid-based nanofluids containing functionalized multi-walled carbon nanotubes. J. Phys. Chem. C 114, 8749–8754. doi: 10.1021/jp1005346
Wang, J., Chu, H., and Li, Y. (2008). Why single-walled carbon nanotubes can be dispersed in imidazolium-based ionic liquids. ACS Nano 2, 2540–2546. doi: 10.1021/nn800510g
Wu, Y. Y., Tsui, W. C., and Liu, T. C. (2007). Experimental analysis of tribological properties of lubricating oils with nanoparticle additives. Wear 262, 819–825. doi: 10.1016/j.wear.2006.08.021
Ye, C., Liu, W., Chen, Y., and Yu, L. (2001). Room-temperature ionic liquids: a novel versatile lubricant. Chem. Commun. 21, 2244–2245. doi: 10.1039/b106935g
Yu, B., Bansal, D. G., Qu, J., Sun, X., Luo, H., Dai, S., et al. (2012). Oil-miscible and non-corrosive phosphonium-based ionic liquids as candidate lubricant additives. Wear 289, 58–64. doi: 10.1016/j.wear.2012.04.015
Yu, B., Liu, Z., Ma, C., Sun, J., Liu, W., and Zhou, F. (2015). Ionic liquid modified multi-walled carbon nanotubes as lubricant additive. Tribol. Int. 81, 38–42. doi: 10.1016/j.triboint.2014.07.019
Yu, B., Zhou, F., Pang, C., Wang, B., Liang, Y., and Liu, W. (2008). Tribological evaluation of α, over(ω,')-diimidazoliumalkylene hexafluorophosphate ionic liquid and benzotriazole as additive. Tribol. Int. 41, 797–801. doi: 10.1016/j.triboint.2008.02.004
Zhang, Z., Liu, J., Wu, T., and Xie, Y. (2017). Effect of carbon nanotubes on friction and wear of a piston ring and cylinder liner system under dry and lubricated conditions. Friction 5, 147–154. doi: 10.1007/s40544-016-0126-6
Zhao, G., Zhao, Q., Li, W., Wang, X., and Liu, W. (2014). Tribological properties of nano-calcium borate as lithium grease additive. Lubr. Sci. 26, 43–53. doi: 10.1002/ls.1227
Zhao, L., Li, Y., Liu, Z., and Shimizu, H. (2010). Carbon nanotube-conducting polymer core–shell hybrid using an imidazolium-salt-based ionic liquid as a linker: designed as a potential platinum electrode alternative material for large-scale solution processing. Chem. Mater. 22, 5949–5956. doi: 10.1021/cm102087e
Keywords: multi-walled carbon nanotubes, phosphonium ionic liquids, nano-additives, tribofilms, lithium grease, four-ball tribometer
Citation: Vyavhare K and Aswath PB (2019) Tribological Properties of Novel Multi-Walled Carbon Nanotubes and Phosphorus Containing Ionic Liquid Hybrids in Grease. Front. Mech. Eng. 5:15. doi: 10.3389/fmech.2019.00015
Received: 01 December 2018; Accepted: 26 March 2019;
Published: 16 April 2019.
Edited by:
Ashlie Martini, University of California, Merced, United StatesReviewed by:
Erik Kuhn, Hamburg University of Applied Sciences, GermanyXiaoqing Jin, Chongqing University, China
Copyright © 2019 Vyavhare and Aswath. This is an open-access article distributed under the terms of the Creative Commons Attribution License (CC BY). The use, distribution or reproduction in other forums is permitted, provided the original author(s) and the copyright owner(s) are credited and that the original publication in this journal is cited, in accordance with accepted academic practice. No use, distribution or reproduction is permitted which does not comply with these terms.
*Correspondence: Pranesh B. Aswath, aswath@uta.edu