- 1Faculty of Mechanical and Electrical Engineering, Kunming University of Science and Technology, Kunming, China
- 2Department of Physics, Chongqing University of Arts and Sciences, Chongqing, China
This work focuses on the electronic, magnetic, and half-metallic behaviors and interesting surface state of a hypothetical CsCrCl3 ferromagnet with a P63/mmc space group, investigated using the spin-polarized generalized gradient approach (GGA) and spin-polarized GGA plus Hubbard U correction within the framework of density functional theory. The calculated total magnetic moment of P63/mmc CsCrCl3 is ∼7.397 μB, and the main contribution to the total magnetism is from the Cr atoms. Based on the obtained spin-polarized band structures, we confirm that this hypothetical CsCrCl3 ferromagnet is a half-metal with 100% spin-polarization. Without spin-orbit coupling, i.e., when the spin and orbit degrees of freedom are dependent, we found an interesting nodal surface state at the kz = π plane due to the P63/mmc type lattice structure having a twofold screw axis S2z. Our study provides strong evidence that a protected nodal surface state can be achieved in magnetic materials.
Introduction
The search for half-metallic materials (De Groot et al., 1983; Pickett and Moodera, 2001; Elfimov et al., 2002; Kusakabe et al., 2004; Zhang et al., 2018) with 100% spin-polarization (P) (Žutic et al., 2004) is a hot research topic in next-generation spintronics (Li and Yang, 2016). Half-metallic materials (Ding and Wang, 2016; Hu et al., 2017; Wang et al., 2017; Wu et al., 2017; Bhattacharyya et al., 2018; Du et al., 2019; Huang et al., 2019; Wang et al., 2019; Zhang et al., 2019) are so called owing to their unique electronic band structures in both spin channels: the bands in one spin channel exhibit a metallic property, whereas those in the other spin channel have semiconducting or insulating behaviors. Therefore, based on the formula: , where (n↑(EF) and n↓(EF) are the spin-up density of states (DOS) and the spin-down DOS at the Fermi level (EF), respectively, the half-metallic materials should have 100% P in theory. Notably, half-metallic materials are good spin injectors into semiconductors, with maximum efficiency in spintronics devices. Various families of materials, including Heusler alloys (Kandpal et al., 2007; Wang et al., 2015; Cui et al., 2019; Han et al., 2019; Singh and Gupta, 2019), metallic oxides (Szotek et al., 2004), perovskite compounds (Li et al., 2015), wurtzite compounds (Wu et al., 2006), nanowires (Li et al., 2017), nanoribbons (Son et al., 2006), and some two-dimensional (2D) monolayers (Gao et al., 2016; Ashton et al., 2017; Feng et al., 2018; Liu et al., 2019), have been predicted to be half-metallic materials. Half-metals can be used for pure spin generation and injection. To develop practicable spintronic devices using half-metals, the spin-flip (half-metallic gap) should be wide enough to prevent thermally agitated spin-flip transition and preserve half-metallicity.
Wu et al. (2018) proposed a new class of topological bulk materials, 3D nodal surface semimetals. They are topological materials with 2D nodal surface states, which are different from topological semimetals with a 0D nodal point (Soluyanov et al., 2015; Young and Kane, 2015; Kumar et al., 2017; Jin K. et al., 2019) and 1D nodal line (Chen et al., 2018; Liu et al., 2018; Zhou et al., 2018; He et al., 2019; Jin L. et al., 2019; Pham et al., 2019; Yan et al., 2019; Yi et al., 2019; Zou et al., 2019; Zhao et al., 2020). To date, only a few nodal surface semimetals (Wu et al., 2018) have been the subject of theoretical investigations. Nodal surface semimetals have a 2D nodal surface state composed of numerous band-crossing points. That is, each point on the surface is an intersection point between the two bands, and its dispersion is linear along the surface normal direction. Coarse-grained quasiparticles excited from a nodal surface effectively behave as 1D massless Dirac fermions along the surface normal direction and may show novel physical behaviors.
In this work, we use density functional theory (DFT) calculations to systematically investigate the electronic, magnetic, and half-metallic properties of the hypothetical CsCrCl3 ferromagnet, as well as a topological nodal surface signature, with respect to its potential applications in next-generation spintronics and electronics devices. We predict that the hypothetical CsCrCl3 ferromagnet is a novel material co-featuring a half-metallic property and nodal surface state at the kz = π plane. The formation energy of the ferromagnetic type CsCrCl3 with P63/mmc space group and 194 space number was calculated to be -1.736 eV in https://www.materialsproject.org/materials/mp-570326/, indicating that this hexagonal ferromagnet is theoretically stable.
Computational Details
All atomic and electronic structure calculations were performed using the Vienna ab initio simulation package (Sun et al., 2003) with generalized gradient approximation (GGA) (Perdew et al., 1996) using the Perdew–Burke–Ernzerhof (Perdew et al., 1998) exchange-correlation functional. The projector augmented wave pseudo-potential was employed, with a cutoff energy of 600 eV for plane-wave expansions and a Monkhorst–Pack special 5 × 5 × 9 k-point mesh. The convergence criteria for energy and force were set to 10–6 eV per atom and 0.0005 eV/Ǻ, respectively. For the CsCrCl3 material, we considered strong correlation effects in the transition-metal Cr atoms. The Hubbard U correction was also used in the rotationally invariant DFT + U approach to further confirm the band structure.
Results and Discussion
The crystal structure of CsCrCl3 from different perspectives is given in Figures 1A,B, respectively. CsCrCl3 has a BaVS3 type hexagonal structure, with a P63/mmc space group and a space number of 194. As shown in Figure 1, the Cr atoms are surrounded by octahedrons of Cl atoms, which form 1D chains along the z-axis and share common faces. These chains are arranged in a trigonal lattice in the x-y plane, with Cs atoms inserted between the chains. We fully optimized the crystal model (Figure 1), and the obtained equilibrium lattice constants for the CsCrCl3 ferromagnet were a = b = 7.40130 Ǻ and c = 6.1870 Ǻ. Our calculated results were in good agreement with those in the materials project database1 (a = b = 7.395 Ǻ, c = 6.173 Ǻ) and topological materials database2 (a = b = 7.249 Ǻ, c = 6.228 Ǻ).
Based on the obtained equilibrium lattice constants, we studied the electronic band structures of the CsCrCl3 ferromagnet. The Γ-M-K-Γ-A-L-H-A high-symmetry points (Figure 2) in the first Brillouin zone were selected to describe the spin-polarized band structures of the CsCrCl3 ferromagnet. The spin-up and spin-down band structures were calculated using the GGA method and the results are shown in Figures 3A,B, respectively. One can see that the spin-up electronic bands and the EF overlapped with each other, reflecting the metallic property. For the spin-down channel, a clear band gap appeared, indicating the semiconducting behavior. Moreover, the values of the band gap and half-metallic gap (Guo et al., 2016; Wang et al., 2016) were calculated to be 3.78 and 0.7 eV, respectively, indicating that the half-metallic state of the CsCrCl3 system is very robust. To properly account for the strong correlation effects in the transition-metal Cr atoms, a Hubbard U correction was adopted in the rotationally invariant DFT + U approach. In Figure 4, the spin-polarized band structures obtained via the DFT + U method (U = 3 eV for Cr-d orbitals) are shown. One can see that the half-metallic state for this CsCrCl3 system was retained. That is, the metallic state and semiconducting state, in the spin-up and spin-down directions, respectively, could still be observed. However, the band gap and the half-metallic band gap in the spin-down channel increased to values of 4.496 and 2.375 eV, respectively.
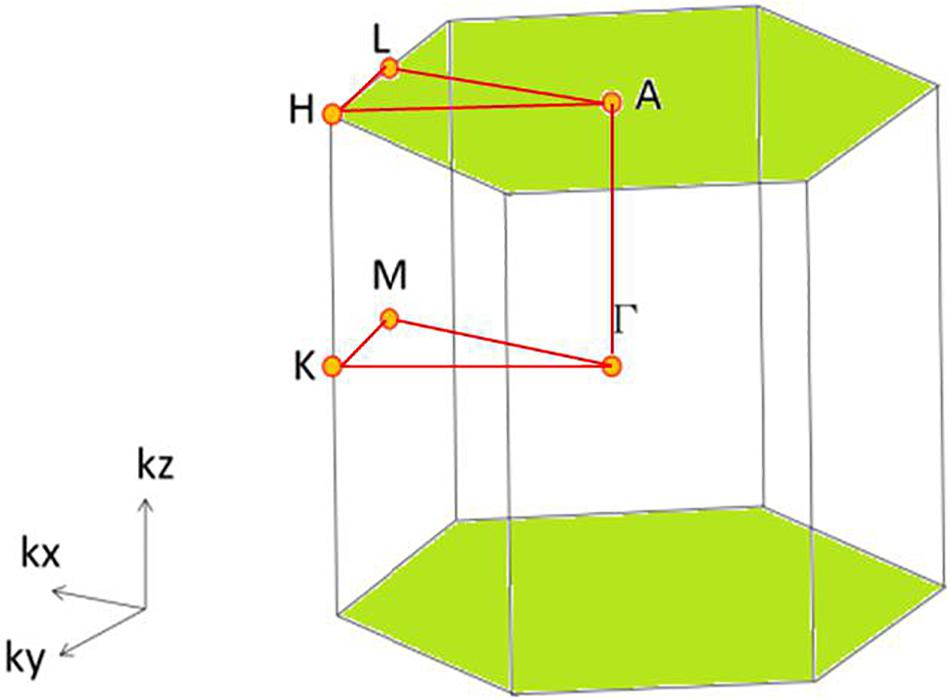
Figure 2. Selected high-symmetry points in the first Brillouin zone. The kz = π plane is shown in green.
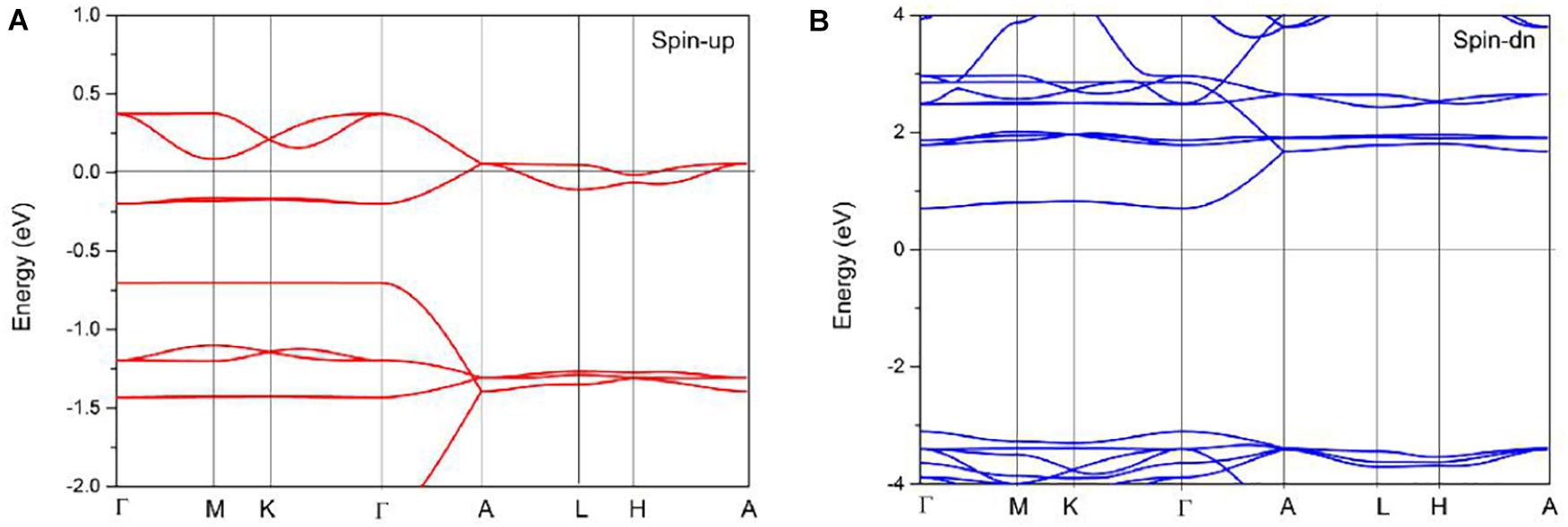
Figure 3. Spin-polarized band structures of CsCrCl3 system via GGA method. (A) for spin-up and (B) for spin-down.
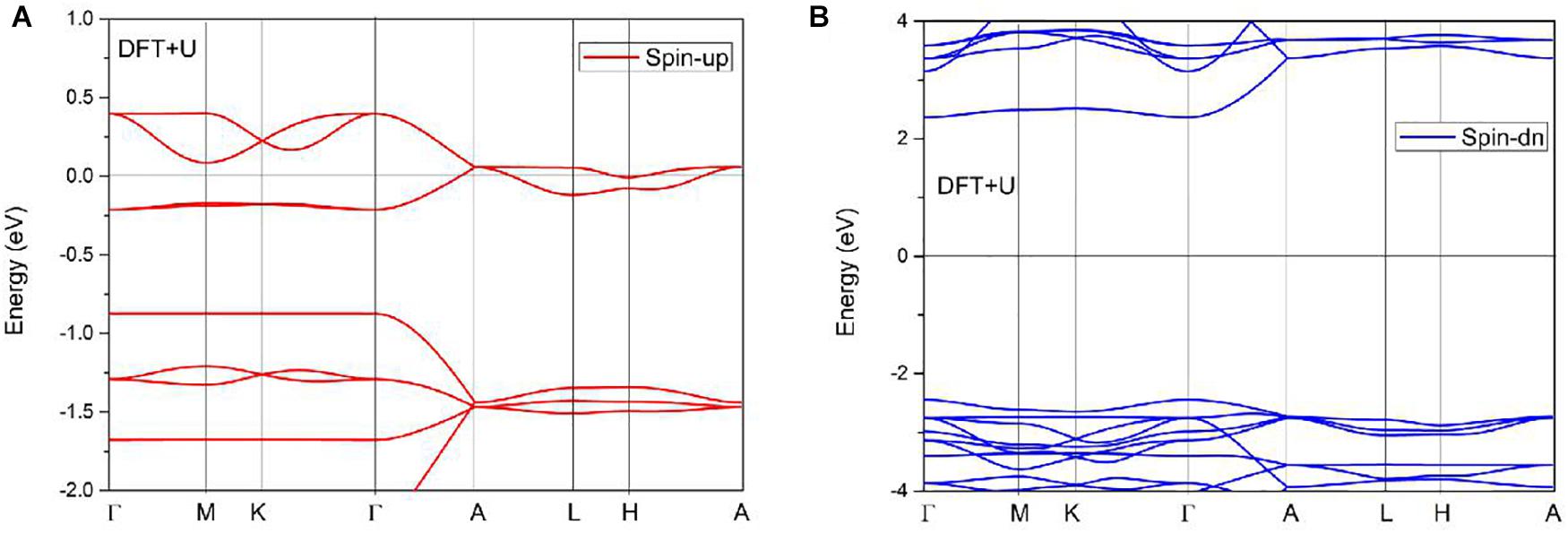
Figure 4. Spin-polarized band structures of CsCrCl3 system via DFT + U method. (A) for spin-up and (B) for spin-down.
Furthermore, we would like to point out that the half-metallic states of the CsCrCl3 ferromagnet are very robust to the Hubbard U correction and the uniform strain. In Figure 5, the band structures of the CsCrCl3 ferromagnet with U = 5 eV for Cr-d orbitals and with 5 GPa uniform strain, respectively, are given. One can see that the CsCrCl3 ferromagnet still hosts half-metallic properties under the above-mentioned situations.
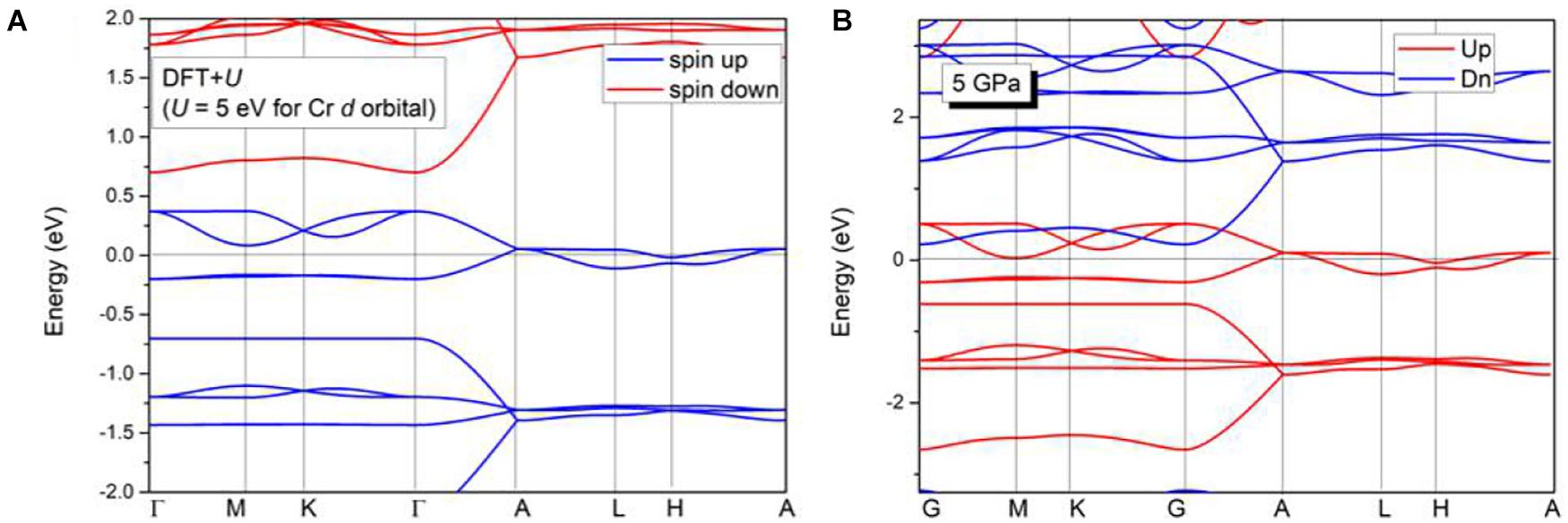
Figure 5. Spin-polarized band structures of CsCrCl3 system with DFT + U method (A) and with uniform strain (B), respectively.
The primitive cell of the CsCrCl3 ferromagnet contained eight atoms, i.e., two Cr atoms, two Cs atoms, and six Cl atoms. The total magnetic moment for this system was 7.397 μB, and the atomic magnetic moments of Cr atoms (∼3.693 μB) dominated the total magnetism. To further examine the magnetism of this system, the spin-density in both spin channels was determined (Figure 6). The spin-density was mainly located around the Cr atoms, indicating that they have large atomic magnetic moments. The contributions of other atoms, including Cs and Cl atoms, to the total magnetism were almost negligible.
Note that the spin-orbit coupling (SOC) effect was not considered in this work. Without SOC, the spin and orbit degrees of freedom are independent; therefore, the spin degree and the orbit degree can be regarded as different subspaces (Wu et al., 2018). With a selected spin-polarization axis, the spin-up and spin-down directions are decoupled; therefore, the bands for each spin species can be effectively seen as for a spin-free system. Thus, the protected nodal surface state can be realized in one spin of a ferromagnet when the SOC effect is neglected. As shown in Figure 3, along the A-L-H-A direction, two bands (in the range of -0.1 to 0.1 eV) were totally degenerate with each other. The lattice structure of the CsCrCl3 ferromagnetic system features a 2-fold screw axis S2z. As discussed by Wu et al. (2018), a nodal surface state (at the kz = π plane) must occur in the ferromagnetic material if the crystal structure of the ferromagnetic material hosts a 2-fold screw axis S2z. To further investigate whether there was a nodal surface state in this CsCrCl3 system, the 3D band structure (A-point-centered) in the spin-up channel was examined from different perspectives. In Figures 7A,B, one can see that a nodal surface state existed in the kz = π plane near the EF. Furthermore, one can see that the nodal surface state (at the kz = π plane and in the spin-up channel) was relatively flat in energy, that is, the energy variation was less than 0.2 eV. Finally, in Figure 8, the calculated orbital-resolved band structures of the Cl-p and Cr-d orbitals states are shown. The orbital-resolved band structure of the Cs-s orbitals is not shown, as it had little effect on the nodal surface state near the EF in the spin-up channel. The main contribution to the nodal surface state was from the Cr-d orbits; however, the contribution of the Cl-p orbitals was not negligible.
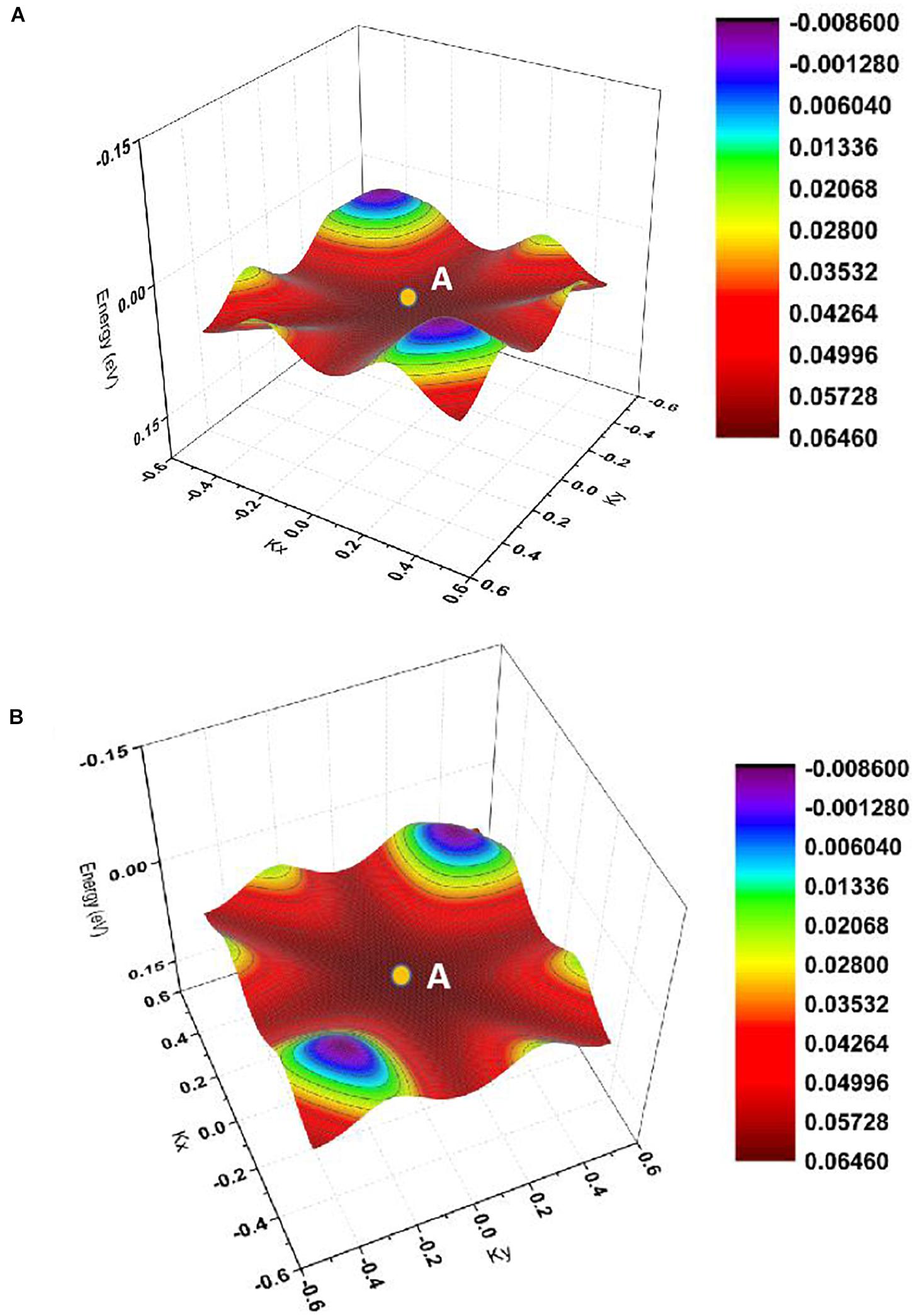
Figure 7. A-point centered 3D band structures of CsCrCl3 ferromagnet at kz = π plane in spin-up channel (without SOC effect). (A,B) are from different view sides.
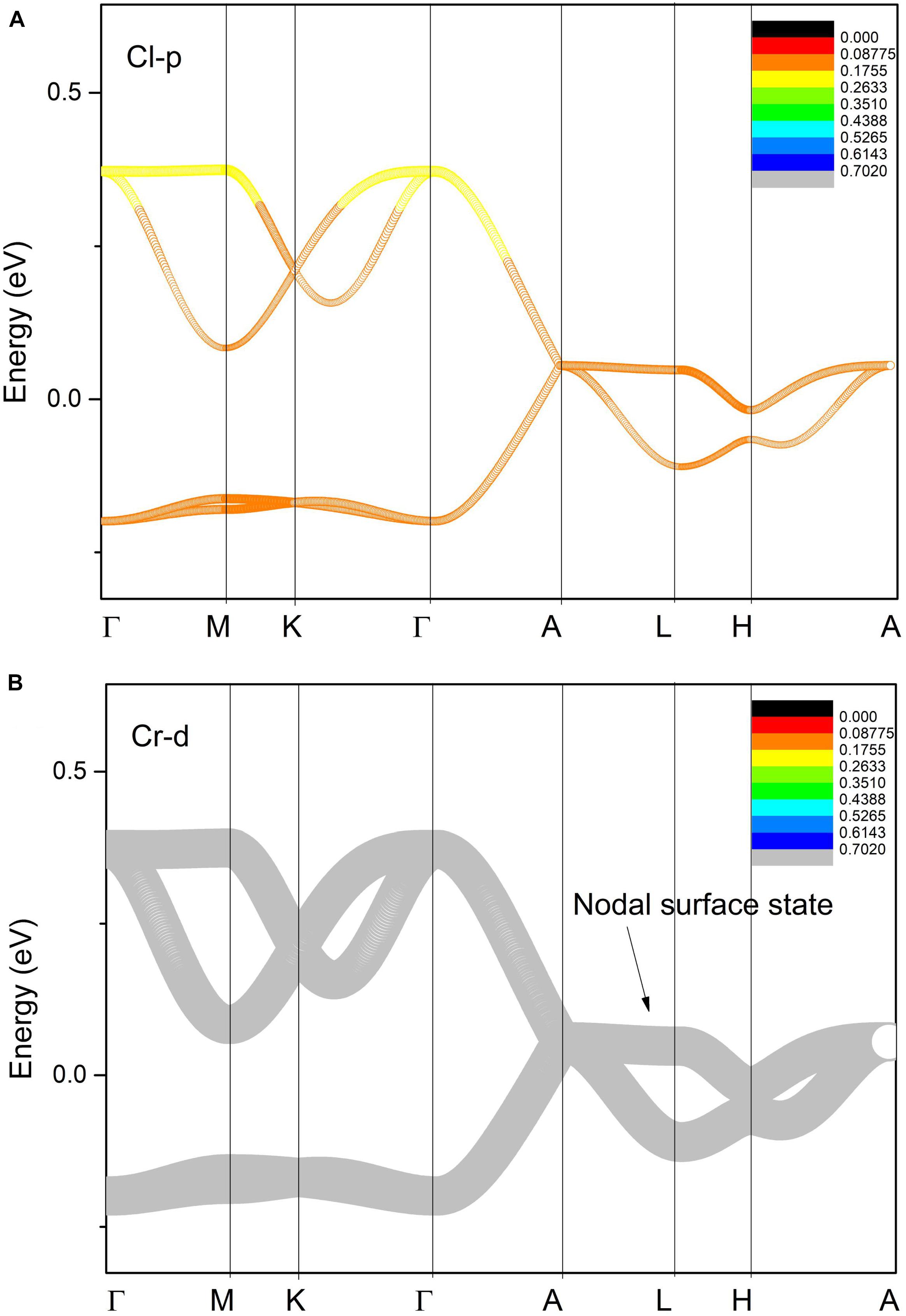
Figure 8. Orbital-resolved band structures of Cl-p (A) and Cr-d (B) orbitals of CsCrCl3 in the spin-up channel. The nodal surface state, formed by the crossing of two bands near the EF, is highlighted by an arrow along the A-L-H-A direction.
Conclusion
In conclusion, in this study, a hypothetical CsCrCl3 ferromagnetic system with a P63/mmc space group and a space number of 197 was investigated in detail with respect to its electronic structures, magnetism, half-metallic behavior, and topological signature via DFT and DFT + U. We predict that the CsCrCl3 ferromagnet is an excellent half-metal with a large band gap and a half-metallic gap. Moreover, this CsCrCl3 ferromagnet has a nodal surface state (at the kz = π plane) in the spin-up channel when the SOC effect is not taken into consideration. The nodal surface state in the spin-up channel is due to the P63/mmc lattice structure, which features a twofold screw axis S2z. Based on the spin-density and the orbital-resolved band structure, we confirmed that the main contribution to the total magnetism and the surface state near the EF was from the Cr-d orbitals. It is hoped that our theoretical work will inspire further research to explore the half-metallic behaviors and the nodal surface states of many other materials in the future.
Data Availability Statement
All datasets presented in this study are included in the article/supplementary material.
Author Contributions
The author confirms being the sole contributor of this work and has approved it for publication.
Funding
This work was supported by the first batch of post-doctoral research fund research projects in Yunnan Province (Serial No. 9), the Science and Technology Research Program of Chongqing Municipal Education Commission (Grant No. KJQN201801346), and the Chongqing University of Arts and Sciences Foundation (Grant No. Z2011Rcyj05).
Conflict of Interest
The authors declare that the research was conducted in the absence of any commercial or financial relationships that could be construed as a potential conflict of interest.
Footnotes
- ^ https://www.materialsproject.org/materials/mp-570326/
- ^ https://www.topologicalquantumchemistry.org/#/detail/36132
References
Ashton, M., Gluhovic, D., Sinnott, S. B., Guo, J., Stewart, D., and Hennig, R. G. (2017). Two-dimensional intrinsic half-metals with large spin gaps. Nano Lett. 17, 5251–5257. doi: 10.1021/acs.nanolett.7b01367
Bhattacharyya, G., Choudhuri, I., and Pathak, B. (2018). High Curie temperature and half-metallicity in an atomically thin main group-based boron phosphide system: long range ferromagnetism. Phys. Chem. Chem. Phys. 20, 22877–22889. doi: 10.1039/c8cp03440k
Chen, H., Zhang, S., Jiang, W., Zhang, C., Guo, H., Liu, Z., et al. (2018). Prediction of two-dimensional nodal-line semimetals in a carbon nitride covalent network. J. Mater. Chem. A 6, 11252–11259. doi: 10.1039/c8ta02555j
Cui, Z., Wu, B., Ruan, X., Zhou, Q., Liu, Z., Fu, X., et al. (2019). Enhancing the half-metallicity of equiatomic quaternary Heusler compound CoFeCrGe via atomic doping. Results Phys. 15:102533. doi: 10.1016/j.rinp.2019.102533
De Groot, R., Mueller, F. M., Van Engen, P. G., and Buschow, K. H. (1983). New class of materials: half-metallic ferromagnets. Phys. Rev. Lett. 50, 2024–2027. doi: 10.1103/physrevlett.50.2024
Ding, Y., and Wang, Y. (2016). Enhanced piezoelectricity and half-metallicity of fluorinated AlN nanosheets and nanoribbons: a first-principles study. J. Mater. Chem. C 4, 1517–1526. doi: 10.1039/c5tc03910j
Du, Y., Bu, H., Ji, C., Zhang, X., Li, C., and Fang, X. (2019). Tunable magnetic and half-metallic properties of the two-dimensional electron gas in LaAlO3/SrTiO3(111) heterostructures. Phys. Chem. Chem. Phys. 21, 18170–18178. doi: 10.1039/c9cp00746f
Elfimov, I. S., Yunoki, S., and Sawatzky, G. A. (2002). Possible path to a new class of ferromagnetic and half-metallic ferromagnetic materials. Phys. Rev. Lett. 89:216403.
Feng, Y., Wu, X., Han, J., and Gao, G. (2018). Robust half-metallicities and perfect spin transport properties in 2D transition metal dichlorides. J. Mater. Chem. C 6, 4087–4094. doi: 10.1039/c8tc00443a
Gao, G., Ding, G., Li, J., Yao, K., Wu, M., and Qian, M. (2016). Monolayer MXenes: promising half-metals and spin gapless semiconductors. Nanoscale 8, 8986–8994. doi: 10.1039/c6nr01333c
Guo, R., Liu, G., Wang, X., Rozale, H., Wang, L., Khenata, R., et al. (2016). First-principles study on quaternary Heusler compounds ZrFeVZ (Z = Al, Ga, In) with large spin-flip gap. RSC Adv. 6, 109394–109400. doi: 10.1039/c6ra18873g
Han, Y., Chen, Z., Kuang, M., Liu, Z., Wang, X., and Wang, X. (2019). 171 Scandium-based full Heusler compounds: a comprehensive study of competition between XA and L21 atomic ordering. Results Phys. 12, 435–446. doi: 10.1016/j.rinp.2018.11.079
He, T., Zhang, X., Meng, W., Jin, L., Dai, X., and Liu, G. (2019). Topological nodal lines and nodal points in the antiferromagnetic material β-Fe2PO5. J. Mater. Chem. C 7, 12657–12663. doi: 10.1039/c9tc04046c
Hu, R., Zhang, Z. H., and Fan, Z. Q. (2017). BN nanoflake quantum-dot arrays: structural stability, and electronic and half-metallic properties. Phys. Chem. Chem. Phys. 19, 20137–20146. doi: 10.1039/c7cp02391j
Huang, H. M., Cao, M., Jiang, Z., Xiong, Y., Zhang, X., Luo, S., et al. (2019). High spin polarization in formamidinium transition metal iodides: first principles prediction of novel half-metals and spin gapless semiconductors. Phys. Chem. Chem. Phys. 21, 16213–16222. doi: 10.1039/c9cp00958b
Jin, K., Huang, H., Wang, Z., and Liu, F. (2019). A 2D nonsymmorphic Dirac semimetal in a chemically modified group-VA monolayer with a black phosphorene structure. Nanoscale 11, 7256–7262. doi: 10.1039/c9nr00906j
Jin, L., Zhang, X., He, T., Meng, W., Dai, X., and Liu, G. (2019). Topological nodal line state in superconducting NaAlSi compound. J. Mater. Chem. C 7, 10694–10699. doi: 10.1039/c9tc03464a
Kandpal, H. C., Fecher, G. H., and Felser, C. (2007). Calculated electronic and magnetic properties of the half-metallic, transition metal based Heusler compounds. J. Phys. D Appl. Phys. 40, 1507–1523. doi: 10.1088/0022-3727/40/6/s01
Kumar, N., Sun, Y., Xu, N., Manna, K., Yao, M., Suss, V., et al. (2017). Extremely high magnetoresistance and conductivity in the type-II Weyl semimetals WP2 and MoP2. Nat. Commun. 8, 1–8.
Kusakabe, K., Geshi, M., Tsukamoto, H., and Suzuki, N. (2004). New half-metallic materials with an alkaline earth element. J. Phys. Condens. Matter. 16:S5639.
Li, M., Retuerto, M., Deng, Z., Stephens, P. W., Croft, M., Huang, Q., et al. (2015). Giant magnetoresistance in the half-metallic double-perovskite ferrimagnet Mn2FeReO6. Angew. Chem. 54, 12069–12073. doi: 10.1002/anie.201506456
Li, X., Lv, H., Dai, J., Ma, L., Zeng, X. C., Wu, X., et al. (2017). Half-metallicity in one-dimensional metal trihydride molecular nanowires. J. Am. Chem. Soc. 139, 6290–6293. doi: 10.1021/jacs.7b01369
Li, X., and Yang, J. (2016). First-principles design of spintronics materials. Natl. Sci. Rev. 3, 365–381. doi: 10.1093/nsr/nww026
Liu, J., Li, X., Wang, Q., Kawazoe, Y., and Jena, P. (2018). A new 3D Dirac nodal-line semi-metallic graphene monolith for lithium ion battery anode materials. J. Mater. Chem. 6, 13816–13824. doi: 10.1039/c8ta04428g
Liu, Y., Guo, S., Yu, J., and Zhong, H. (2019). Large magnetoresistance and perfect spin filter effect in Fe doped SnS2 half-metallic monolayers: a first principles study. Phys. Lett. A 383, 674–679. doi: 10.1016/j.physleta.2019.01.008
Perdew, J. P., Burke, K., and Ernzerhof, M. (1996). Generalized gradient approximation made simple. Phys. Rev. Lett. 77:3865. doi: 10.1103/physrevlett.77.3865
Perdew, J. P., Burke, K., and Ernzerhof, M. (1998). Perdew, burke, and ernzerhof reply. Phys. Rev. Lett. 80:891. doi: 10.1103/physrevlett.80.891
Pham, A., Klose, F., and Li, S. (2019). Robust topological nodal lines in halide carbides. Phys. Chem. Chem. Phys. 21, 20262–20268. doi: 10.1039/c9cp04330f
Pickett, W. E., and Moodera, J. S. (2001). Half metallic magnets. Phys. Today 54, 39–44. doi: 10.1063/1.1381101
Singh, S., and Gupta, D. C. (2019). Lanthanum based quaternary Heusler alloys LaCoCrX (X = Al, Ga): hunt for half-metallicity and high thermoelectric efficiency. Results Phys. 13:102300. doi: 10.1016/j.rinp.2019.102300
Soluyanov, A. A., Gresch, D., Wang, Z., Wu, Q., Troyer, M., Dai, X., et al. (2015). Type-II Weyl semimetals. Nature 527, 495–498.
Son, Y., Cohen, M. L., and Louie, S. G. (2006). Half-metallic graphene nanoribbons. Nature 444, 347–349. doi: 10.1038/nature05180
Sun, G., Kurti, J., Rajczy, P., Kertesz, M., Hafner, J., and Kresse, G. (2003). Performance of the Vienna ab initio simulation package (VASP) in chemical applications. J. Mol. Struct. Theochem. 624, 37–45. doi: 10.1016/s0166-1280(02)00733-9
Szotek, Z., Temmerman, W. M., Svane, A., Petit, L., Stocks, G. M., and Winter, H. (2004). Half-metallic transition metal oxides. J. Magn. Magn. Mater. 272-276, 1816–1817. doi: 10.1016/j.jmmm.2003.12.818
Wang, X., Cheng, Z., Liu, G., Dai, X., Khenata, R., Wang, L., et al. (2017). Rare earth-based quaternary Heusler compounds MCoVZ (M = Lu, Y; Z = Si, Ge) with tunable band characteristics for potential spintronic applications. IUCrJ 4, 758–768. doi: 10.1107/s2052252517013264
Wang, X., Cheng, Z., Wang, J., Wang, L., Yu, Z., Fang, C., et al. (2016). Origin of the half-metallic band-gap in newly designed quaternary Heusler compounds ZrVTiZ (Z = Al, Ga). RSC Adv. 6, 57041–57047. doi: 10.1039/c6ra08600d
Wang, X., Dai, X. F., Wang, L., Liu, X., Wang, W., Wu, G. H., et al. (2015). Electronic structures and magnetism of Rh3Z (Z = Al, Ga, In, Si, Ge, Sn, Pb, Sb) with DO3 structures. J. Magn. Magn. Mater. 378, 16–23. doi: 10.1016/j.jmmm.2014.10.161
Wang, X., Ding, G., Cheng, Z., Yuan, H., Wang, X., Yang, T., et al. (2019). R3c-type LnNiO3 (Ln = La, Ce, Nd, Pm, Gd, Tb, Dy, Ho, Er, Lu) half-metals with multiple Dirac cones: a potential class of advanced spintronic materials. IUCrJ 6, 990–995. doi: 10.1107/s2052252519012570
Wu, R. Q., Peng, G., Liu, L., and Feng, Y. P. (2006). Wurtzite NiO: a potential half-metal for wide gap semiconductors. Appl. Phys. Lett. 89:082504. doi: 10.1063/1.2335970
Wu, W., Liu, Y., Li, S., Zhong, C., Yu, Z., Sheng, X., et al. (2018). Nodal surface semimetals: theory and material realization. Phys. Rev. B 97:115125.
Wu, X., Han, J., Feng, Y., Li, G. Q., Wang, C., Ding, G., et al. (2017). Half-metals and half-semiconductors in a transition metal doped SnSe2 monolayer: a first-principles study. RSC Adv. 7, 44499–44504. doi: 10.1039/c7ra07648g
Yan, L., Liu, P., Bo, T., Zhang, J., Tang, M., Xiao, Y., et al. (2019). Emergence of superconductivity in a Dirac nodal-line Cu2Si monolayer: ab initio calculations. J. Mater. Chem. C 7, 10926–10932. doi: 10.1039/c9tc03740c
Yi, X., Li, W. Q., Li, Z. H., Zhou, P., Ma, Z., and Sun, L. Z. (2019). Topological dual double node-line semimetals NaAlSi(Ge) and their potential as cathode material for sodium ion batteries. J. Mater. Chem. C 7, 15375–15381. doi: 10.1039/c9tc04096j
Young, S. M., and Kane, C. L. (2015). Dirac semimetals in two dimensions. Phys. Rev. Lett. 115, 126803–126803.
Zhang, M., Zhang, C., Wang, P., and Li, S. (2018). Prediction of high-temperature Chern insulator with half-metallic edge states in asymmetry-functionalized stanene. Nanoscale 10, 20226–20233. doi: 10.1039/c8nr07503d
Zhang, Y., Liu, Z. H., Wu, Z., and Ma, X. Q. (2019). Prediction of fully compensated ferrimagnetic spin-gapless semiconducting FeMnGa/Al/In half Heusler alloys. IUCrJ 6, 610–618. doi: 10.1107/s2052252519005062
Zhao, Z., Zhang, Z., and Guo, W. (2020). A family of all sp2-bonded carbon allotropes of topological semimetals with strain-robust nodal-lines. J. Mater. Chem. C 8, 1548–1555. doi: 10.1039/c9tc05470g
Zhou, P., Ma, Z., and Sun, L. Z. (2018). Coexistence of open and closed type nodal line topological semimetals in two dimensional B2C. J. Mater. Chem. C 6, 1206–1214. doi: 10.1039/c7tc05095j
Zou, Z. C., Zhou, P., Ma, Z., and Sun, L. Z. (2019). Strong anisotropic nodal lines in the TiBe family. Phys. Chem. Chem. Phys. 21, 8402–8407. doi: 10.1039/c9cp00508k
Keywords: DFT, half-metallic materials, band gap, nodal surface properties, electronic structure
Citation: Li Y (2020) Hypothetical P63/mmc-Type CsCrCl3 Ferromagnet: Half-Metallic Property and Nodal Surface State. Front. Mater. 7:262. doi: 10.3389/fmats.2020.00262
Received: 06 June 2020; Accepted: 17 July 2020;
Published: 24 September 2020.
Edited by:
Zhenxiang Cheng, University of Wollongong, AustraliaReviewed by:
Biao Wang, Southwest University, ChinaGuangqian Ding, Chongqing University of Posts and Telecommunications, China
Xiaoming Zhang, Hebei University of Technology, China
Copyright © 2020 Li. This is an open-access article distributed under the terms of the Creative Commons Attribution License (CC BY). The use, distribution or reproduction in other forums is permitted, provided the original author(s) and the copyright owner(s) are credited and that the original publication in this journal is cited, in accordance with accepted academic practice. No use, distribution or reproduction is permitted which does not comply with these terms.
*Correspondence: Yang Li, bGl5YW5nQGNxd3UuZWR1LmNu; bGl5YW5nX3BoeXNpY3NAMTI2LmNvbQ==