- 1School of Civil Engineering and Transportation, Beijing University of Civil Engineering and Architecture, Beijing, China
- 2Beijing Advanced Innovation Center for Future Urban Design, Beijing University of Civil Engineering and Architecture, Beijing, China
- 3Beijing Key Laboratory of Traffic Engineering, College of Metropolitan Transportation, Beijing University of Technology, Beijing, China
- 4Department of Civil and Environmental Engineering, Michigan Technological University, Houghton, MI, United States
The main objective of this research is to investigate the permanent deformation of asphalt mixtures containing direct coal liquefaction residue (DCLR) under various tire pressures and temperatures. Three types of asphalt mixtures, including control/DCLR/composite-DCLR modified asphalt mixture, were prepared by the Marshall design method. The rutting test was conducted under a tire pressure range of 0.7–1.0 MPa with a 0.1-MPa interval and at a temperature range of 55–70°C with a 5°C interval. Moreover, the dynamic stability and rutting depth of three asphalt mixtures were obtained to evaluate their resistance of permanent deformation. It was found that the rutting resistance of three asphalt mixtures declines with the increased tire pressures and temperatures. The asphalt mixture containing DCLR has a higher dynamic stability and lower rutting depth compared to the control asphalt mixture under the same conditions. Furthermore, the rutting resistance of composite-DCLR modified asphalt mixture is better than that of DCLR modified asphalt mixture. It indicates that the composite-DCLR is favorable for the improvement of rutting resistance of asphalt mixture. Moreover, the analysis of variance was applied, which analysis results showed that the rutting resistance of asphalt mixture is more sensitive to temperature than tire pressure. Based on the least-squares procedure, the relationship between dynamic stability and rutting depth was obtained, and the accuracy of the prediction is acceptable.
Introduction
Direct coal liquefaction residue (DCLR), the main by-product from the direct liquefaction process of coal, contains 30% by weight raw coal. It has been found that the DCLR accounts for 30–50% asphaltene materials and heavy oil, which has been considered as a construction material. Based on its performance characteristics, such as high softening point and asphaltene percentage, DCLR has been utilized to modify virgin asphalt binder to improve its high-temperature performance and has the potential to apply as an asphalt modifier (Shu, 2003; Gu, 2012). Because the properties and component of DCLR are similar to Trinidad Lake Asphalt (TLA), it can potentially be a substitution of TLA (He, 2013; Zhao, 2015). Ji et al. (2014), Ji J. et al. (2015) studied the properties of asphalt binders containing DCLR and found that the DCLR additives improve the high-temperature properties but lower the low-temperature properties of asphalt binders. Meanwhile, the performance of DCLR modified asphalt mixture was studied. The results illustrated that the addition of DCLR into virgin asphalt binder could be a powerful approach to enhance the rutting resistance of asphalt mixture. The rutting resistance and moisture susceptibility of DCLR and composite-DCLR modified asphalt mixture are even better than those of asphalt mixture modified by styrene–butadiene–styrene (SBS) (Moghaddam et al., 2011; Zhao and Ji, 2014).
As one of main pavement distresses, the rutting often leads to the large-scale maintenance, as a result of bringing high economic consumption (Ma et al., 2017; Zhang et al., 2019). Therefore, the research on rutting of asphalt mixture is necessary and imminent. The performance of pavement is affected by many factors, such as tire pressure, materials, layer thickness, and environmental conditions (Saevarsdottir and Erlingsson, 2015; Ding et al., 2019). High temperature and slow-speed vehicle are more likely to cause the rutting of pavement (Moghaddam et al., 2011, 2014; Al-Humeidawi, 2016). In most areas of China, the air temperature can reach 40°C in summer, and the actual road surface temperature can even reach up to 70°C, which dramatically increases the probability of rutting. Khan et al. (2013) predicted the rutting of the pavement by the accelerated test and found that the rutting potential is sensitive to repeated loads and high temperatures. The correlation equation relating to the progression of rutting depth versus number of passes was established (Chen et al., 2020).
The relationship on pavement strains between tire pressure and speed of loading was investigated by Chatti et al. (1996). They pointed out that at the bottom of the layer the horizontal strains increased with the tire pressure increases. The parameters of the temperature and loading were used as the major factors to predict the asphalt mixture permanent deformation (Ji X. P. et al., 2015). Alkhateeb et al. (2011) found that the rutting depth increased with the increase in temperature and tire pressure and with the decrease in subgrade strength by the finite element models. Park (2006) used a triaxial repetitive load test to obtain temperature conversion factor (TCF) parameters and revealed the variation in pavement temperature during full-scale trafficking. The test results showed that TCF could convert the traffic loading at multiple temperatures to its equivalent traffic at a specification temperature. Abed and Al-Azzawi (2012) noted that because of the change of material properties, the rutting depth increased in the leveling and base courses, but the stress level decreased in those layers. Salama and Chatti (2008) investigated the relative rutting damage of different axle types and truck configurations to the hot asphalt mixture. The trucks with wide single-wheel axles increase causing the pavement sensitive to the surface layers’ creep, and wide single axles are more combative than dual wheels. Corte et al. (1994) and Attia and Ahmed (2014) studied the impact of tire pressure on overlay performance by the mechanistic-empirical pavement design method. It was noted that the tire pressure plays a serious role on the longitudinal cracks, fatigue cracks, and asphalt pavement rutting and has no significant effect on rutting in base and subgrade layers.
From previous studies, it was summarized that the different axle loading and test temperature have various influences on the rutting resistance of HMA, but few studies were engaged in the permanent deformation of DCLR modified asphalt mixtures. As the DCLR is a relatively new and intricate material, the influence of tire pressures and temperatures on the permanent deformation of DCLR asphalt mixtures is worth being studied. The motivation of this study is to validate the possibility of using DCLR in the highway constructions and to evaluate the high-temperature performance of DCLR modified asphalt mixtures. It is of great scientific significance for the development and application of new environmental-friendly materials in pavement engineering.
Objectives and Scopes
The main purpose of this research is to evaluate the permanent deformation of DCLR and composite-DCLR modified asphalt mixtures under various tire pressures and temperatures. The following tasks were listed for analysis:
• The three kinds of asphalt mixtures are the control asphalt mixtures, DCLR modified asphalt mixture, and composite-DCLR modified asphalt mixture. It is noted that three asphalt mixtures have the equal gradation and optimum asphalt content (OAC).
• Permanent deformation of three kinds of asphalt mixtures was evaluated using the rutting test under multiple tire pressures varying from 0.7 to 1.0 MPa with a 0.1-MPa interval and at temperatures ranging from 55 to 70°C with a 5°C interval.
• The permanent deformations of three kinds of asphalt mixtures related to different tire pressures and test temperatures were explored through the statistical analysis.
Materials
Asphalt Binders
Virgin asphalt binder was produced in South Korea with 80/100 penetration. Two types of modified asphalt binders were prepared through adding DCLR and composite-DCLR into the base asphalt binder. The specific preparation processes of DCLR modified asphalt and composite-DCLR modified asphalt were determined based on experimental experience and previous studies in the research group (Ji et al., 2019, 2020), shown in Figures 1, 2, respectively.
According to the Superpave performance grade (PG) specifications, the physical properties of the three kinds of asphalt binders were measured and are shown in Tables 1, 2.
Aggregates
Limestone was chosen as an aggregate material, and it includes coarse aggregates with the size of 9.5–19.0 mm, 4.75–9.5 mm, 2.36–4.75 mm, and fine aggregates with the size of 0–2.36 mm. The powder left from the limestone was recycled as the mineral powder. The physical properties of the aggregates were measured according to the “Test Methods of Aggregate for Highway Engineering” (JTG E42-2005, 2005), and the results meet the specification requirement in China.
Mixture Design
The type of the mixture was a recommended AC-20, which is popularly used in China. Its gradation is shown in Figure 3 and met the technical requirements specified in China. The target air void of all specimens was 4 ± 1.0%. The OAC of the mixture was determined in accordance with the ASTM D1559, and the OAC was 4.2%.
The performances of the mixture also meet the technical requirements specified in China. In order to indicate the effect of three asphalts on the permanent deformation of three mixtures, the same gradation and OAC were used. The other testing conditions are the same as that of the control mixture. The performances of the three asphalt mixtures are shown in Figure 4.
Test Methods
Test Conditions
Three types of asphalt mixtures, the control asphalt mixture, DCLR modified asphalt mixture, and composite-DCLR modified asphalt mixture, were prepared for the rutting test. During the test, the test temperatures were selected to be from 55 to 70°C with a 5°C interval. And the tire pressures were determined to be from 0.7 to 1.0 MPa with a 0.1-MPa interval. The increase in tire pressure would be caused by the increased axle loading of vehicles. With the tire pressure increases, the contact area of the tire pavement is induced to a reduction. It may prompt an increase in tire-pavement contact stress, which deteriorates the pavement service conditions (Jamy and Najeeb, 1991; ASTM D1559, 2006; Wang and Machemehl, 2006; Abdel-Motaleb, 2007; Homsi et al., 2011). In China, the standard axle loading is specified as a single axle with the dual tires at 100 kN, and the standard tire pressure is 0.7 MPa. However, several surveys conducted in China revealed the traffic volumes of trucks and their tire pressures had increased steadily in the last several decades. The truck axle loading was always larger than 100 kN, and tire pressure was higher than 0.7 MPa. Therefore, in this study, a tire pressure range of 0.7–1.0 MPa with a 0.1-MPa interval was selected in order to simulate the impact of high tire pressure on the pavement response. Additionally, the standard test temperature is 60°C during the process of the rutting test in China. However, the actual pavement temperature in summer months is also far higher than 60°C. Sometimes, the actual pavement temperature can achieve a peak at 70°C. In this study, to simulate the impact of temperature on pavement response, the test temperature range was selected to be from 55 to 70°C with a 5°C interval.
Specimen Preparation
At least three specimens, with each sample the length is 300 mm, the width is 300 mm, and the depth is 50 mm, were tested to measure their resistance to rutting. Before testing, the specimens of the control mixture were cured at the room temperature for 12 h, and the specimens of the DCLR and composite-DCLR modified asphalt mixtures were cooled at room temperature for 48 h, as required by the China Specification “Test Methods of Asphalt and Asphalt Mixtures for Highway Engineering” (JTGE20-2011).
Rutting Test
As reported by Test Methods of Asphalt and Asphalt Mixtures for Highway Engineering (JTGE20-2011), the standard experiment was supervised to apply a smooth rubber wheel load of 0.7 MPa on each wheel traveling at a speed of 42 ± 1 passes/min on specimens at 60°C. The test device and samples are shown in Figure 5.
The two indexes of dynamic stability and rutting depth were obtained for three asphalt mixtures. The dynamic stability refers to the passes of each 1-mm deformation between 45 and 60 min, and the rutting depth reflects the total deformation during the 60 min in the test. High dynamic stability and low rutting depth indicates a stiff mixture, which is likely to be less sensitive to the temperature and loads.
Results and Discussion
Properties of Three Asphalt Binders and Mixtures
The high-temperature PG (AASHTO T 315, 2012) and low-temperature PG (AASHTO T 313, 2012) of the asphalt binders were measured. The physical properties of three kinds of asphalt binders are presented in Tables 1, 2. The performances of the three asphalt mixtures are shown in Figure 5.
The test outcomes in Tables 1, 2 demonstrated that the composite-DCLR added asphalt binder has the highest high-temperature PG value and lowest low-temperature PG value. It indicated that the composite-DCLR significantly improved the high-temperature and low-temperature properties. From Figure 5, it is seen that the DCLR increased the dynamic stability, residual stability, and TSR of asphalt mixture while decreasing its rutting depth. This demonstrated that the addition of DCLR contributes to the improvement of high-temperature performance and moisture susceptibility of asphalt mixture. However, the DCLR decreased the low-temperature performance of asphalt mixture to some extent, which can be reflected by the test results of failure strain at −10°C. This is the main reason why the composite-DCLR was used and investigated in this study. The composite-DCLR significantly improved the high-temperature performance and low-temperature performance of asphalt mixture. The reason may be that the composite-DCLR, which includes SBS and rubber powder, increases the bonding strength with the matrix of the asphalt binders and reinforces the network of modified asphalt binders. This possible effect causes the asphalt binder stiffer and leads to an improvement of properties of asphalt binder and mixture, especially for the high-temperature performance (Ji et al., 2017).
Rutting Resistance of Three Mixtures
The permanent deformation of three asphalt mixtures was conducted using the rutting test under a test temperature range from 55 to 70°C with a 5°C interval and at a tire pressure range from 0.7 to 1.0 MPa with a 0.1-MPa interval. The rutting resistance of three asphalt mixtures was characterized in terms of the rutting depths and dynamic stabilities. The results are shown in Figures 6, 7.
In Figure 6, the dynamic stabilities of asphalt mixtures decrease with the increase in tire pressures and test temperatures, but the change amplitudes are different. The rise in tire pressure and test temperature led to a degeneration in dynamic stability and a deterioration in rutting potential. Moreover, when the tire pressure increased from 0.7 to 1.0 MPa under 55–70°C, the dynamic stabilities of asphalt mixtures decreased by 12.3, 25.0, and 20.6%, respectively. Furthermore, when test temperature increased from 55 to 70°C under the condition of 0.7- to 1.0-MPa tire pressures, the dynamic stabilities of asphalt mixtures decreased by 16.2, 66.4, and 49.6%, respectively. It can be deduced that the temperature has a more positive effect than the tire pressure on the permanent deformation. Although the dynamic stabilities of the DCLR modified asphalt mixture and composite-DCLR modified asphalt mixture decreased at a high rate, which were still higher compared with the control asphalt mixture. The DCLR and composite-DCLR had significant effects on the advancement of the rutting resistance. The composite-DCLR mixture had the highest dynamic stability compared to the other two asphalt mixtures under identical tire pressures and test temperatures, which indicated that the composite-DCLR mixture has the least sensitivity to the temperature and tire pressure. The composite-DCLR modified asphalt mixture has higher elasticity, stiffness (Ji et al., 2016), and viscosity compared to the control asphalt mixture and DCLR modified asphalt mixture.
Figure 7 displays that the increases in test temperature and tire pressure introduce an increase in rutting depth of asphalt mixtures. An increase in tire pressures or test temperatures yielded an increase in rutting potential. When tire pressure increased from 0.7 to 1.0 MPa, the rutting depths of asphalt mixtures increased by 95.8, 34.4, and 17.9%, respectively. When the test temperature increased from 55 to 70°C, the rut depths of asphalt mixtures increased by 307, 139, and 93.1%, respectively. The influence of the test temperature on the rutting resistance of asphalt mixtures is more noticeable than that of tire pressures, and the rutting depths of control asphalt mixture decreased at the highest rate. The base asphalt binder has the lowest high-temperature properties. The composite-DCLR modified asphalt mixture had the lowest rutting depth compared to the other two asphalt mixtures under the same tire pressures and test temperatures. The composite-DCLR modified asphalt mixture had a good resistance to rutting. As a result, it can be concluded that the composite-DCLR modified asphalt mixture is identified as a good mixture for application in pavement construction.
Analysis of Variance
Analysis of variance (ANOVA) is used for isolating the relevant factors and estimating the effects of these factors on the total deviation. In ANOVA, the F-value is defined as the ratio of the variance between treatments and variance in treatments. An enormous F-value represents more significant effects of the factor on the sample. In this article, the ANOVA was applied to detect the effects of multiple tire pressures and test temperatures on the permanent deformation of asphalt mixtures by using the ORIGIN software, and the confidence level was set as 0.95. The significant degree of variances can be detected using the range value in the value range analysis approach. The F-value is used to detect the significant degree of variances.
Tables 3, 4 list the range value and ANOVA of permanent deformation affected by different tire pressures and test temperatures.
According to the results of ORIGIN software, there was no interaction between temperatures and tire pressures. The test results in Tables 3, 4 illustrated that the temperature was a significant factor in the rutting resistance of asphalt mixtures. The rutting resistance of asphalt mixtures is more sensitive to temperature than tire pressure. The conclusion is coincident with the above outcomes of the rutting test.
Development of the Prediction Model for Dynamic Stability and Rutting Depths
The prediction model of rutting resistance was developed to verify the correlation between the predicted value and the measured value of dynamic stability and rutting depth under different tire pressures and temperatures. In the model, the temperatures and tire pressures were independent variables. The constitution of the model was regressed based on the least-square procedure, shown in Eq. 1.
where, RDS,RD(T, P) is the prediction results (dynamic stability and rutting depths) of mixtures; T is the temperature, °C; P is the tire pressure, MPa; a1, a2, and a3 are regression coefficients.
The regression coefficients a1, a2, and a3 and coefficient R2 value for three mixtures are listed in Table 5 and Figure 8.
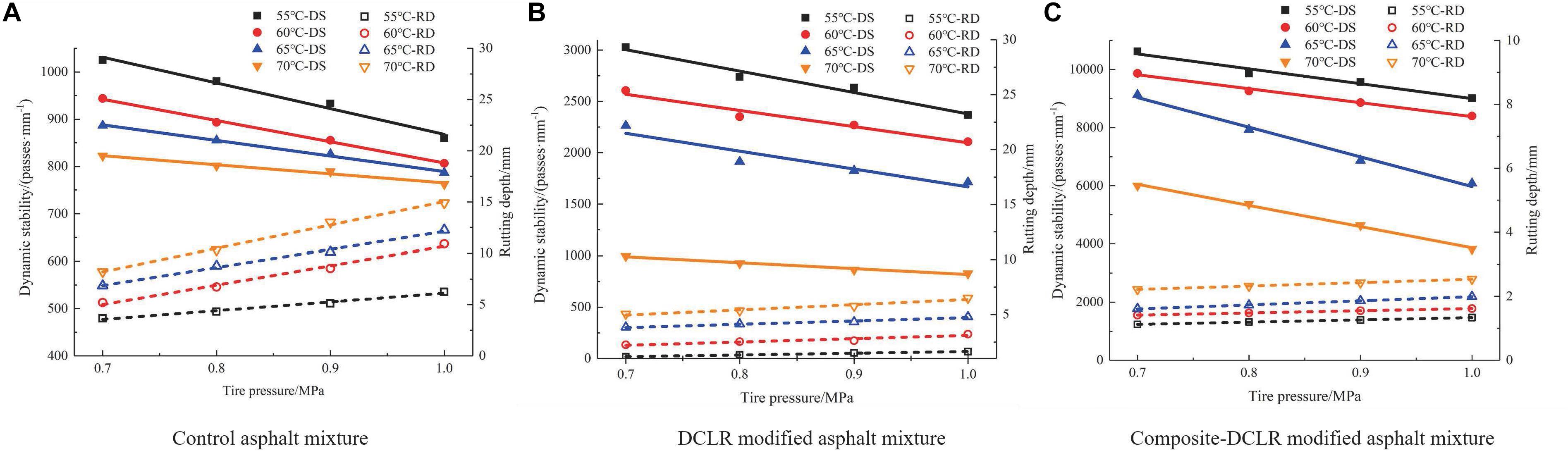
Figure 8. Dynamic stabilities/rutting depths of three asphalt mixtures. (A) control asphalt mixture, (B) DCLR modified asphalt mixture, and (C) composite DCLR asphalt mixture.
The coefficient R2 value of the prediction model ranges from 0.91 to 0.99. The test results in Table 5 and Figure 8 show that the dynamic stabilities and rutting depths of three asphalt mixtures have good linear correlations with the tire pressure or test temperature. Two parameters with three coefficients were used to predict the high-temperature performance of asphalt mixtures according to the dynamic stabilities and rutting depths, and the prediction is acceptable according to the accuracy. Furthermore, the model of dynamic stability and rutting depth is fitted by the laboratory test data, and the linear model better reflects the dynamic stability and rutting change under the laboratory conditions. The future study will be extended to the real road fields. The rutting reaction of the pavement under real load and temperature will show significant non-linear characteristics, and the non-linear model for correlation analysis will be applied in the future study.
Conclusion
The DCLR and composite-DCLR were applied as additives to modify the virgin asphalt binder, and the corresponding preparation procedures of modified asphalt binders were illustrated. Meanwhile, the properties of modified asphalt binders were evaluated and estimated. Moreover, three asphalt mixtures, including the control mixture, DCLR modified asphalt mixture, and composite-DCLR modified asphalt mixture, were designed. The permanent deformation of asphalt mixtures under different tire pressures and test temperatures were explored. Based on the above results and analysis, the conclusions are drawn below:
• The addition of DCLR or composite-DCLR improves the resistance to permanent deformation of asphalt mixtures. The composite-DCLR modified asphalt mixture has a better resistance to rutting compared to the control asphalt mixture and DCLR modified asphalt mixture. As a result, it is recommended that the composite-DCLR modified asphalt mixture is a better mixture for roads in tropical regions, where the rutting is one of the main distresses of pavement.
• The ANOVA was adopted to evaluate the development of tire pressures and test temperatures on the permanent deformation of three asphalt mixtures. The analysis results demonstrated that the rutting resistance of asphalt mixtures is more sensitive to the temperature than the tire pressure.
• The test temperatures and tire pressures have influence on the rutting resistance of asphalt mixtures. The prediction model was established to predict the dynamic stabilities and rutting depths of the asphalt mixtures based on the least-squares procedure, and the accuracy of the prediction is acceptable. However, the further improvement of accuracy of the model and the application of more parameters in the model are worth to be studied in the future.
Data Availability Statement
All datasets presented in this study are included in the article/supplementary material.
Author Contributions
All authors contributed to the article and approved the submitted version.
Funding
This study was sponsored by the National Natural Science Foundation of China (51778038), Beijing Natural Science Foundation and Beijing Municipal Education Commission (grant number: KZ201910016017), the Fundamental Research Funds for Beijing Universities (ZC05), and Program for Changjiang Scholars and Innovative Research Team in University (IRT-17R06).
Conflict of Interest
The authors declare that the research was conducted in the absence of any commercial or financial relationships that could be construed as a potential conflict of interest.
Acknowledgments
The authors appreciate Mr. Gao Jinqi for his help in the experiments.
References
AASHTO T 313 (2012). Standard Test Method for Determining Flexural Creep Stiffness of Asphalt Binder Using the Bending Beam Rheometer. Washington, DC: American Association of State Highway and Transportation Officials.
AASHTO T 315 (2012). Standard Test Method for Determining The Rheological Properties of Asphalt Binder Using a Dynamic Shear Rheometer. Washington, DC: American Association of State Highway and Transportation Officials.
Abdel-Motaleb, M. E. (2007). Impact of high-pressure truck tires on pavement design in Egypt. Emirates J. Eng. Res. 12, 65–73.
Abed, A. H., and Al-Azzawi, A. A. (2012). Evaluation of rutting depth in flexible pavements using finite element analysis and local empirical model. Am. J. Eng. Appl. Sci. 5, 163–169. doi: 10.3844/ajeassp.2012.163.169
Al-Humeidawi, B. H. (2016). Experimental characterization of rutting performance of HMA designed with aggregate gradations according to Superpave and Marshall methods. World J. Eng. Technol. 4, 477–487. doi: 10.4236/wjet.2016.43048
Alkhateeb, L. A., Saoud, A., and Al-Msouti, M. F. (2011). Rutting prediction of flexible pavements using finite element modeling. Jordan J. Civil Eng. 5, 173–190.
ASTM D1559 (2006). Standard Method of Test for Resistance to Plastic Flow of Bituminous Mixtures Using Marshall Apparatus. West Conshohocken, PA: American Society of Testing and Materials.
Attia, M. I. E., and Ahmed, M. A. (2014). Impact of vehicle class and tire pressure on pavement performance in MEPDG. J. Eng. Res. Appl. 4, 45–57. doi: 10.1055/s-0037-1604350
Chatti, K., Kim, H. B., Yun, K. K., Mahoney, J. P., and Monismith, C. L. (1996). Field investigation into effects of vehicle speed and tire pressure on asphalt concrete pavement strains. J. Trans. Res. Rec. 1539, 66–71. doi: 10.1177/0361198196153900109
Chen, T., Luan, Y., Ma, T., Zhu, J., Huang, X., and Ma, S. (2020). Mechanical and microstructural characteristics of different interfaces in cold recycled mixture containing cement and asphalt emulsion. J. Clean. Prod. 258:120674. doi: 10.1016/j.jclepro.2020.120674
Corte, J. F., Brosseaud, Y., Simoncelli, J. P., and Caroff, G. (1994). Investigation of rutting of asphalt surface layers: influence of binder and axle loading configuration. Trans. Res. Rec. 1436, 28–37.
Ding, Y., and Zhao, X. P. (2019). Research on the influence of anti-rutting agent on the performance of AC-20C SBS composite modified asphalt mixture. Highways Transp. Inner Mongolia 3, 34–38.
Gu, X. H. (2012). Properties and utilization of coal direct liquefaction residue. Clean Coal Technol. 18, 24–31.
He, L. (2013). Study on the Preparation and Performance of Asphalt Modified by Coal Liquefaction Residue. M.Sc. Thesis, Chang’an University, Beilin.
Homsi, F., Bodin, D., Yotte, S., Breysse, D., and Balay, J. M. (2011). Multiple-axle loadings: shape parameters and their effect on the fatigue life. Eur. J. Environ. Civil Eng. 15, 743–758. doi: 10.3166/ejece.15.743-758
Jamy, D., and Najeeb, A. (1991). Evaluation of Tire Pressure, Tire Construction, Axle Configuration, and Axle Load on Flexible Pavement Performance. M.Sc. Thesis, Texas A & M University, College Station, TX.
Ji, J., Shi, Y. F., Li, P. F., Yang, S., Suo, Z., and Xu, S. F. (2015). Analysis of high-and-low temperature properties of asphalt mortar affected by DCLR and filler-asphalt ratio. J.Xi’an Univ. Arch. Tech. 8, 511–516.
Ji, J., Shi, Y. F., Suo, Z., Xu, S. F., Yang, S., and Li, P. F. (2016). Comparison on properties of modified asphalt blended with DCLR and TLA. J. Fuel Chem. Technol. 43, 1061–1067.
Ji, J., Wang, D., Suo, Z., Xu, Y., and Xu, S. F. (2017). Study on direct coal liquefaction residue influence on mechanical properties of flexible pavement. Int. J. Pavement Res. Technol. 11, 355–362. doi: 10.1016/j.ijprt.2017.09.006
Ji, J., Wang, Z., Yao, H., Wang, D., Zhang, R., Diab, A., et al. (2019). A numerical study on rutting behaviour of direct coal liquefaction residue modified asphalt mixture. Road Mater. Pavement Des. 16, 1–15. doi: 10.1080/14680629.2019.1690029
Ji, J., Wang, Z., Zhang, R., Wei, J. M., Suo, Z., You, Z. P., et al. (2020). Rutting resistance of direct coal liquefaction residue (DCLR) modified asphalt mixture under variable loads over a wide temperauture range. Constr. Building Mater. 257:119489. doi: 10.1016/j.conbuildmat.2020.119489
Ji, J., Zhao, Y. S., and Xu, S. F. (2014). Study on properties of the blends with Direct Coal Liquefaction Residue and asphalt. Appl. Mech. Mater. 488-489, 316–321. doi: 10.4028/www.scientific.net/amm.488-489.316
Ji, X. P., Zheng, N. X., Niu, S. S., Meng, S., and Xu, Q. L. (2015). Development of a rutting prediction model for asphalt pavements with the use of an accelerated loading facility. Road Mater. Pavement Des. 17, 15–31. doi: 10.1080/14680629.2015.1055337
JTG E20-2011 (2011). Standard Test Material of Bitumen and Bituminous Mixtures for Highway Engineering. Beijing: China Ministry of Transport.
JTG E42-2005 (2005). Test Methods of Aggregate for Highway Engineering. Beijing: China Ministry of Transport.
Khan, S., Nagabhuashana, M. N., Tiwari, D., and Jain, P. K. (2013). Rutting in flexible pavement: an approach of evaluation with accelerate pavement. Proc. Soc. Behav. Sci. 104, 149–157. doi: 10.1016/j.sbspro.2013.11.107
Ma, J., Zhao, X. M., He, S. H., Song, H. X., Zhao, Y., Song, H. S., et al. (2017). Review of pavement detection technology. J. Traffic Transp. Eng. 17, 121–137.
Moghaddam, T. B., Karim, M. R., and Abdelaziz, M. (2011). A Review on fatigue and rutting performance of asphalt mixes. Sci. Res. Essays 6, 670–682. doi: 10.5897/SRE10.946
Moghaddam, T. B., Soltani, M., and Karim, M. R. (2014). Evaluation of permanent deformation characteristics of unmodified and polyethylene terephthalate modified asphalt mixtures using dynamic creep test. Mater. Des. 53, 317–324. doi: 10.1016/j.matdes.2013.07.015
Park, D. W. (2006). Traffic loadings considering temperature for pavement rutting life. KSCE J. Civil Eng. 10, 259–263. doi: 10.1007/bf02830780
Saevarsdottir, T., and Erlingsson, S. (2015). Modeling of responses and rutting profile of a flexible pavement structure in a heavy vehicle simulator test. Road Mater. Pavement Des. 16, 1–18. doi: 10.1080/14680629.2014.939698
Salama, H. S., and Chatti, K. (2008). A laboratory investigation of the effect of multiple axle and truck configurations on HMA mixture rutting. Road Mater. Pavement Des. 29, 589–613. doi: 10.1080/14680629.2008.9690140
Shu, G. P. (2003). Coal Liquefaction Technology. Beijing: China Coal Industry Publishing House, 115–117.
Wang, F., and Machemehl, R. (2006). Predicting Truck Tire Pressure Effects Upon Pavement Performance. Texas: Center for Transportation Research, University of Texas at Austin.
Zhang, R., You, Z. P., Wang, H. N., Ye, M. X., Yap, Y. K., and Si, C. (2019). The impact of bio-oil as rejuvenator for aged asphalt binder. Constr. Build. Mater. 196, 134–143. doi: 10.1016/j.conbuildmat.2018.10.168
Zhao, Y. S. (2015). Study on the Performances of DCLR Modified Asphalt and Asphalt Mortar. M.Sc. Thesis, Beijing University of Civil Engineering and Architecture, Xicheng.
Keywords: direct coal liquefaction residue, tire pressure and temperature, permanent deformation, analysis of variance, regression model
Citation: Zhi S, Jie J, Ran Z, Zhe W, Hui Y and Dongzhao J (2020) Effects of Tire Pressures and Test Temperatures on Permanent Deformation of Direct Coal Liquefaction Residue Mixture. Front. Mater. 7:246. doi: 10.3389/fmats.2020.00246
Received: 05 May 2020; Accepted: 07 July 2020;
Published: 04 September 2020.
Edited by:
Antonio Caggiano, Darmstadt University of Technology, GermanyCopyright © 2020 Zhi, Jie, Ran, Zhe, Hui and Dongzhao. This is an open-access article distributed under the terms of the Creative Commons Attribution License (CC BY). The use, distribution or reproduction in other forums is permitted, provided the original author(s) and the copyright owner(s) are credited and that the original publication in this journal is cited, in accordance with accepted academic practice. No use, distribution or reproduction is permitted which does not comply with these terms.
*Correspondence: Ji Jie, jijie@bucea.edu.cn