- 1National and Local Joint Engineering Laboratory of Traffic Civil Engineering Materials, Chongqing Jiaotong University, Chongqing, China
- 2School of Civil Engineering, Chongqing Jiaotong University, Chongqing, China
To explore the effect of emulsifier structure on mass transfer performance, and provide theoretical basis and ideas for the structure design of new asphalt emulsifier. The mass transfer process of the anionic surfactant sodium dodecyl benzene sulfonate (SDBS) and its four isomers on the solid surface of calcium carbonate, as well as the resulting main chemical component of its aggregates, were studied using molecular dynamics (MD) simulations. It was found that the SDBS and its isomers can be adsorbed on the calcium carbonate surface over a relatively short time and gradually form an aggregate structure during the mass transfer process. In this process, Na ions have no obvious aggregation behavior in the polar head of the emulsifier. The calculated interfacial interaction energy indicates that the adsorption performance and aggregation on the calcium carbonate surface are related to the degree of branching and steric hindrance of the emulsifier molecule. Both the lipophilic group and the hydrophilic group in the emulsifier promote its diffusion into the solution. The results show that molecular dynamics (MD) simulations can be used to supplement experiments to provide the necessary microscopic information and theoretical basis for further experimentation.
Introduction
It is of great importance to find a method for road construction that is environmentally friendly, resource-saving, and convenient in light of the increasingly significant environmental problems and diversification of construction in China. Traditional road asphalt pavement construction requires the asphalt to be heated to 160–170°C, the mineral materials to 170–180°C, and the mix asphalt mixture to 150–160°C. There are corresponding temperature requirements for the transportation, paving, and compaction of a mixture, especially when the mixture meets water, which makes paving roads difficult (Lissant, 1975). Moreover, the heating process of asphalt during road construction is time-consuming and produces harmful substances that cause serious pollution to the environment. Further, the organic solvents used to dilute asphalt volatilize into the air after road construction, which also pose a serious threat to environmental safety and endanger the health of construction workers (Jerzy and Bengt, 1990). Through long-term research and practice, researchers have shown that the development of emulsified asphalt is one of the most effective ways to solve many problems that exist in traditional road asphalt construction (Ouyang et al., 2017, 2018b).
The use of emulsified asphalt has important practical significance in the field of highway construction (Kong et al., 2017). Based on recent statistics, the apparent consumption of asphalt in China exceeded 32 million tons in 2018, which was distributed across road construction, housing construction, airport construction, and water conservancy projects. With the rapid growth of investment in China's highway construction, the asphalt used in road construction has exceeded 75% of the total national asphalt consumption. In current road construction methods, the heating temperature for the asphalt emulsification process only needs to reach 120°C, which is lower than the processing temperature of hot asphalt by about 50°C. Compared with hot asphalt, the production of emulsified asphalt of unit mass requires 50% less energy and allows a large reduction of the emission of harmful gases. Moreover, the construction environment for emulsified asphalt is largely unaffected by weather conditions; therefore, this production process is currently considered one of the most effective, economical, and environmentally friendly means of road construction and maintenance (Chu and Shen, 2016).
Emulsified asphalt was first used in the field of road construction in the early 1920s. Over the first 40 years of emulsified technology exploration, anionic emulsified asphalt was the primary method developed for construction. Anionic emulsified asphalt has the advantages of energy efficiency, convenient construction, and cost effectiveness, and enables the use of a wide range of emulsifiers. However, both anionic emulsified asphalt and the wet aggregate surface are negatively charged. The resulting homogeneous repulsion between the asphalt particles prevents rapid adsorption of the aggregate surface, which affects pavement formation early in the process and delays its availability for traffic use. With the recent development of surface and colloidal chemistry, cationic emulsified asphalt has gradually replaced anionic emulsified asphalt because of its opposite charge to the aggregate surface, which successfully compensates for the lack of adhesion from anionic emulsified asphalt and has gradually become the predominant technique. However, a wide range of inexpensive anionic emulsifiers are available; further, these emulsifiers can be synergized with cationic, non-ionic, and amphoteric emulsifiers to form a composite, which greatly improves its emulsifying performance. Therefore, the study of anionic emulsified asphalt remains of practical significance (Yang, 2004).
Background
Emulsifier and Emulsified Asphalt
Since the 1960s, the adsorption of emulsifier molecules on solid surfaces has gradually become a major focus of attention (Somasundaran et al., 1964). Methods for the study of emulsified asphalt have developed from a large number of emulsifying compatibility evaluation tests through a combination of Raman spectroscopy, surface free energy, fluorescence spectroscopy, interfacial viscoelasticity, neutron scattering, and other research techniques (Lu et al., 1995; Conboy et al., 1998; Kunieda et al., 1998; Penfold et al., 1998, 2000; Bumajdad et al., 1999; McKenna et al., 2000), which has provided abundant information on the structural design of emulsifiers. Jin et al. (2013) synthesized a composite asphalt emulsifier with an OP-10 cationic surfactant as the intermediate. The effects of the synthesis conditions and formulation on the emulsifying performance, surface activity, and service performance of the emulsifier were studied, and a new way to prepare composite asphalt emulsifiers was explored. Tan et al. (2013) found that emulsifier has significant retarding effect on cement hydration, which is relevant to the types of emulsifier and its dosages. Therefore, suitable emulsifier with little retarding effect on cement hydration and its appropriate dosage are recommended when producing asphalt emulsion for CA mortar. Han and Yu (2014) studied the pressure-sensitivity of cement mortar composites with different concentrations of multi-walled carbon nanotubes and different surfactants under repeated loading and impulsive loading, respectively. Experimental results indicate that the response of the electrical resistance of composites with SDBS to external force is more stable and sensitive than that of composites with Sodium dodecyl sulfate. Ouyang et al. (2016) studied the effect of emulsifier and superplasticizer on the rheology of cement paste and asphalt emulsion and the results show that the apparent viscosity and yield stress of asphalt emulsion increase with superplasticizer and emulsifier dosage. Wang et al. (2018) synthesized a new cationic surfactant containing non-ionic aliphatic alcohol polyoxyethylene ether using epichlorohydrin, octadecyl dimethylamine, and aliphatic alcohol polyoxyethylene ether as raw materials. It was concluded through experiments that the surfactant met the requirements for the asphalt emulsifier.
Emulsified asphalt is a kind of stable oil-in-water or water-in-oil emulsion formed by the action of the asphalt, emulsifier, and stabilizer, and offers the advantages of energy savings, reductions to environmental pollution, and convenient construction (Wu et al., 1992). Based on whether the hydrophilic group of the emulsifier molecule dissolves in water and what ions it dissociates into, asphalt emulsifiers can be divided into ionic and non-ionic types, among which the ionic type can be further divided into anionic, cationic, and amphoteric. The hydrophilic group of anionic asphalt emulsifiers consists of anions, which have been widely used since 1925. The common anionic asphalt emulsifiers are carboxylate (-COONa), sulfonate (-SO3Na), and sulfate (-OSO3Na). Cationic emulsified asphalt were developed after anionic types; however, owing to the excellent emulsifying performance and ability of the former to overcome the shortcomings of slow demulsification, long construction time, and poor ductility, the development of cationic emulsified asphalt progressed more quickly.
In recent years, Seref et al. (2006) studied the effect of Portland cement to improve the mechanical properties of dense graded emulsified asphalt mixtures for structural road layers. It was found that adding Portland cement to emulsified asphalt mixtures improves their mechanical properties, resilience modulus, temperature sensitivity, water damage, creep, and permanent deformation resistance. Yongjoo et al. (2011) studied the cold in-place recycling (CIR) mixture composed of foamed and emulsified asphalt, and found that the CIR-foam sample showed a higher tensile strength and lower water content than the more conventional CIR-latex sample. Zhao et al. (2012) studied the aging resistance of emulsified asphalt using the weightlessness coefficient method. It was found that the aging resistance of emulsified asphalt was better than that of the original asphalt. The aging kinetics equation was established based on the relationship between the n-pentane asphaltene and time. Ouyang et al. (2018a,b, 2019) found that temperature and Water greatly affects the mechanical properties of cement bitumen emulsion mixture.
Application of Molecular Dynamics Simulations for Research Into Road Materials
Molecular dynamics (MD) simulation technology is a comprehensive application that integrates multiple disciplines. As one of the most widely used simulation methods in recent years, MD simulations are mainly based on Newton's basic theory of kinematics and the corresponding force field to simulate the movement processes of molecular system. They can obtain trajectories for particles in a system in addition to predicting the micro-morphological changes during the movement process. At the same time, the structural characteristics and thermodynamic properties of some complex systems can be calculated and analyzed (Matthieu and Punit, 2019).
With the development of MD and of the force field, simulations have been more often and more widely applied while the computational efficiency has been greatly improved. To date, MD simulation technology has become an important method to study the mechanisms of molecular mass transfer. These simulations enable tracking of the dynamic evolution of complex systems over time, reveal the adsorption properties and mechanism of molecules at interfaces at small time scales, and provide kinetic information that is difficult to observe and detect directly through experiments (Han et al., 2016; Hou et al., 2018). Maiti et al. (2002) obtained the equilibrium conditions and surface coverage of surfactants in oil and water phases using symmetrical MD. Ding et al. (2014) studied the effect of styrene-butadiene-styrene (SBS) on the molecular aggregation behavior of asphalt binders via MD simulations. It was found that the influence of the modifier largely depends on the molecular structure of the asphaltene. Xu et al. (2016) developed a molecular simulation method based on thermodynamics to study the cohesion and adhesion of asphalt concrete for the first time. The results showed that the cohesion between the asphalt and aggregate depends mainly on the type of aggregate minerals (silica or calcite) under dry and wet surface conditions. In the case of low moisture content, the type of asphalt has a significant influence on the adhesion between asphalt and silica. Vekeman et al. (2019) studied the adsorption and selectivity of CH4/N2 mixtures on a flexible graphene layer by molecular simulation. It was found that the accuracy of the potentials guarantees a quantitative description of the interactions and trustable results for the dynamics, as long as the appropriate set of intramolecular and intermolecular force fields is chosen. And based on the MD simulations, Zhou et al. (2019) studied the Intermediate Phase in Calcium–Silicate–Hydrates: Mechanical, Structural, Rigidity, and Stress Signatures. It demonstrated that the intermediate phase observed in this system arises from a bifurcation between the rigidity and stress transitions. These features might be revealed to be generic to isostatic disordered networks.
Sodium dodecylbenzene sulfonate (SDBS) is a common anionic asphalt emulsifier that is suitable for slurry seals, viscous oil, and base maintenance. During the preparation of SDBS, a small amount of isomers are simultaneously generated. Owing to the similar properties and morphologies of SDBS and its isomers, it is difficult and expensive to purify its isomers. Therefore, the use of molecular dynamics simulation to study the mass transfer process of SDBS and its isomers on aggregate surface enables accurate prediction of their performance differences, saving manpower and material resources, and improving the efficiency of scientific research. Li et al. (2009) used MD simulations to study the role of SDBS for the oil-water separation of chloroform and water. It was found that the presence of SDBS significantly inhibited the separation of chloroform and water. Song et al. (2009) simulated the adsorption of SDBS on an amorphous silica solid surface using MD. It was found that SDBS can be adsorbed on solid surfaces from solution over a certain time. Liu et al. (2011) studied the adsorption behavior of SDBS and its isomers at the oil/water interface using the MD method, and proposed that changes in the adsorption from the simulations could be investigated by calculating the contact area. The results showed that the proper oil phase can make the interface SDBS and its isomers more compact and orderly, providing better interfacial activity. Ni et al. (2018) investigated the adsorption characteristics of anionic surfactant sodium dodecylbenzene sulfonate on the surface of montmorillonite minerals. It showed that the addition of H+ to the SDBS solution could reduce electrostatic repulsion and promote the adsorption of SDBS on montmorillonite.
Objective
In this paper, the interactions between SDBS, its isomers and calcium carbonate, which is the main chemical composition of the aggregate, were studied using MD simulations, and the effect of SDBS and its isomers on the mass transfer of the aggregate on the surface of the main chemical component was explored. The main purpose is as follows:
(i) To improve the understanding of mass transfer and condensation behavior of anionic asphalt emulsifier on the surface of main chemical components of aggregate.
(ii) The parameters of the radial distribution function (RDF), mean square displacement (MSD), and interfacial energy were used as indicators of molecular mass transfer and condensation behavior to assess the behavior changes of isomers on the surface of the main chemical components of aggregates.
(iii) To explore the effect of emulsifier structure on mass transfer performance, and provide theoretical basis and ideas for the structure design of new asphalt emulsifier.
Model and Method
Potential Function
At present, Condensed-phase Optimized Molecular Potentials for Atomistic Simulation Studies force field (Sun, 1998), Universal force field (Rappé et al., 1992) and consistent-valence force field (Jon et al., 1988) are widely used in the field of interface adsorption. Through a large number of trial calculations, the author found that the potential energy of Stretch-Torsion-Stretch, Separated-Stretch-Stretch, Torsion-Stretch, Bend-Bend, Torsion-Bend, Bend-Torsion-Bend, Long range correction in sodium dodecylbenzenesulfonate-water-calcium carbonate system is much smaller than that of Bond, Angle, Torsion, van der Waals, and Electrostatic interactions. To effectively utilize the limited computing resources, the simulation was performed using the FORCITE module in the Materials Studio (MS) 2017 software, for which the universal force field (Rappé et al., 1992) was selected. The force field includes six potential functions, including bond, angle, torsion, inversion, Van der Waals (VdW) and Electrostatic, which can save time and improve efficiency while ensuring simulation accuracy. The potential energy function has the following form:
The first four terms are Valence energy, which represent the potential energy of bond, angle, torsion and inversion respectively. They are obtained mainly by Fourier Cohen function expansion. Footmarks I, J, K, and L represent different atoms in the model. K_IJ, K_IJK and K_IJKL all represent force constants between different atoms in units of (kcal/mol)/Å2. r_IJ is the natural bond length between atoms in unit of Å. The coefficient C_n represents the minimum value satisfying the boundary conditions at the natural bond angle θ_0. θ, φ and ω represent the angles of bond angle, torsion, and inversion, respectively. The latter two terms are Non-bond energy, representing VdW, and Electrostatic, respectively. In Van der Waals, D_IJ denotes the depth of the potential well, and x denotes the distances of different atoms. In Electrostatic, Q denotes charge, R_ij denotes distance between Q_i and Q_j, and ϵ denotes dielectric constant.
Material Model
Based on the determined chemical composition of the aggregate (Kong, 2017), calcium carbonate is one of the main chemical components of the aggregate. Further, through a large number of trial calculations, it is found that calcium carbonate has higher adsorption performance than other chemical components in aggregate. Therefore, the calcium carbonate model was established in this study to replace aggregate for molecular simulation. The calcite structure model (Sitepu, 2009) was therefore established as the research object; its spatial group is R-3cH, and the spatial coordinates are given in Table 1.
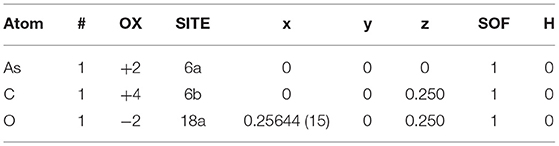
Table 1. Space coordinates for the calcite model (Sitepu, 2009).
The calcite supercell was constructed, and the SDBS (4-1ΦC12S) and its isomers (4-3ΦC12S, 4-5ΦC12S, 2-1ΦC12S, and 3-1ΦC12S) were established using the amorphous cell tool in MS. The model formed a layered structure with the calcite supercell (Liu et al., 2011). The chemical structural models of emulsifier are shown in Figure 1.
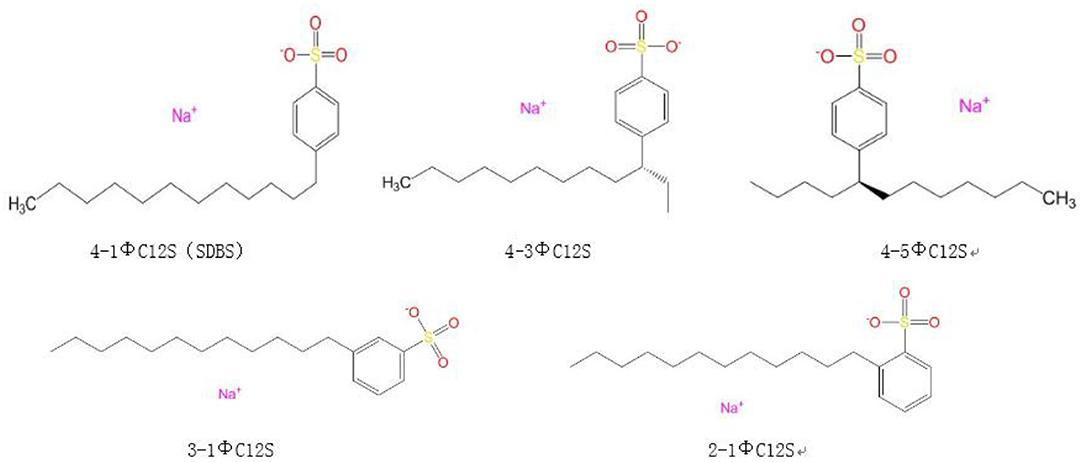
Figure 1. Sodium dodecyl benzene sulfonate (SDBS) and its isomer models. The leading digit 2, 3, or 4 indicates that the long alkyl chain is in the ortho, interposition, or para position of the benzene ring, respectively; Φ represents the benzene ring; 12 is the number of carbon atoms in the long alkyl chain; the digits 1, 3, or 5 before the Φ indicate that the benzene ring is at the position 1#, 3#, or 5# of the long alkyl chain; and S represents the sulfonate.
Structure Model
The supercell thickness for the calcium carbonate was 2.0 nm. An aqueous layer composed of 300 water molecules was placed above the emulsifier solution to prevent the influence of periodic boundary conditions on the emulsifier solution system, where the thickness of the vacuum layer was 3.0 nm. The emulsifier solution contained 10 SDBS molecules and 1,200 water molecules. SDBS molecules are often disassociated as Na+ ions and DBS− ions in solution; therefore, these ions were distributed randomly in a 1:1 ratio when constructing the amorphous emulsifier structure with the goal of maintaining electrical neutrality. Further, the net charge of the DBS− ions and water molecules were selected using the QEq method (Rappé and Goddard, 1991). Owing to the simple structure of the Na+ ions, their formal charge was distributed as a direct response to the force field, and the electrostatic interactions were calculated using the Ewald addition method (Allen and Tildesley, 1987). The van der Waals interactions were based on atomic calculations with a moderate simulation accuracy and a cutoff radius of 12.5 Å. The structural model discussed by Yan and Zhu was adopted for the water molecules (Yan and Zhu, 2013). The test value of the O-H bond length was 0.95718 Å and the H-O-H bond angle was 104.523°. The MD simulations used the canonical ensemble (NVT) approach to simulate a temperature of 298 K and the Nose method (Hoover, 1985) to control the temperature. The time step was 1 fs, the total simulation time was 1,000 ps, and the trace file was stored once every 0.5 ps for the subsequent structural and MD analyses. The component models and architecture are shown in Figure 2.
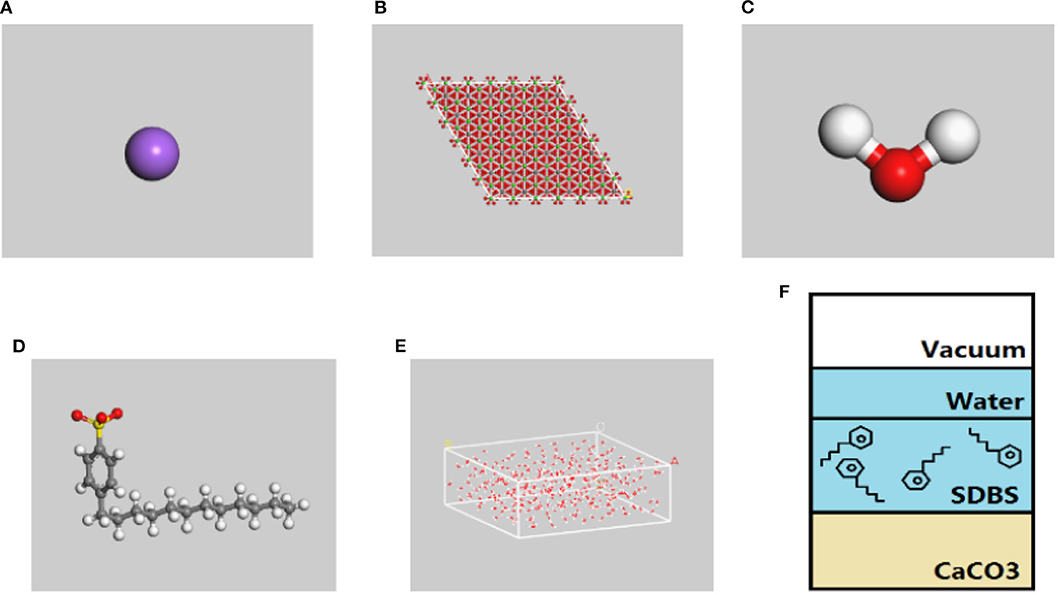
Figure 2. Representation of the simulation model. Obtained by Materials studio 2017. (A) Na+ ions, (B) the supercell o calcium carbonate, (C) H2O, (D) dodecylbenzene sulfonate ions (DBS), (E) water layer, and (F) test model.
Results and Discussion
Agglomeration Structure of the Amorphous Calcium Carbonate Surface
The SDBS MD simulation trace file was derived to clearly observe the adsorption process and improve the simulation efficiency. Figure 3A shows the random distribution model doe the DBS− with a negative charge and the anti-particle Na+ ions on the solid surface of the simulated system (at 0 ps). As the simulation progressed, the intermolecular interactions of the emulsifier were the first to occur. The DBS− ions aggregated in the water, the intermolecular space gradually reduced (200 ps; Figure 3B), and the degree of aggregation increased with time, which gradually formed half micelles (400 ps; Figure 3C). At the same time, because of the adsorption of calcium carbonate on the emulsifier molecules, the molecules close to the surface of the calcium carbonate gradually moved closer to the surface (600 ps; Figure 3D) where they were finally adsorbed (800 ps; Figure 3E). As a result of the difference in the concentrations between the upper water layer and the emulsifier solution, the upper water layer also had a certain adsorption effect on the emulsifier molecules. This eventually led to a separation of the upper and lower emulsifier molecules in the solution, which were adsorbed at the liquid–liquid and solid–liquid interfaces, respectively.
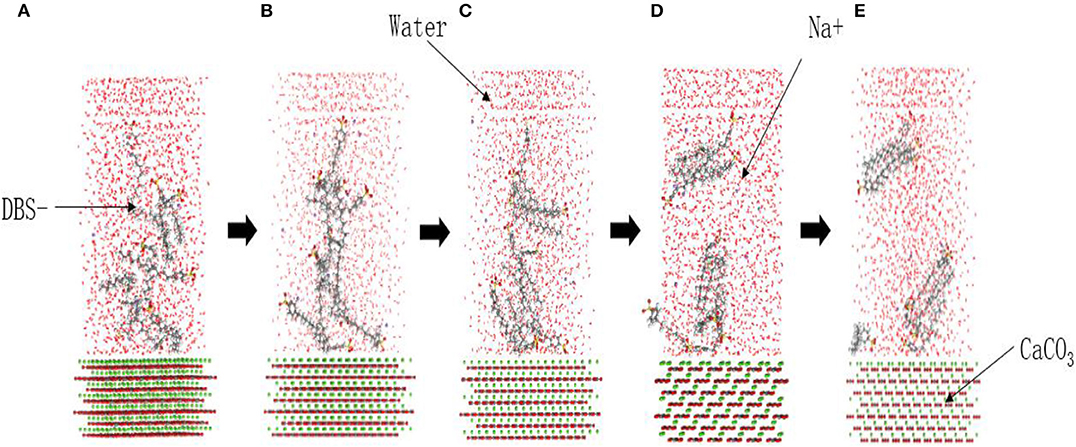
Figure 3. Adsorption process of the surfactant molecules at the solid–liquid interface in the SDBS system. (A) 0 ps, (B) 200 ps, (C) 400 ps, (D) 600 ps, and (E) 800 ps.
During the adsorption process, Na+ ions were distributed randomly in the emulsifier solution, and no obvious aggregation was observed around the polar head of the emulsifier (Figure 4). The authors reason that while the Na+ ions are positively charged, the oxygen atoms in the water and the polar head of the emulsifier molecule have a significant negative charge. The calculated charges given in Table 2 indicate that the charge of the oxygen atoms in the DBS− ion polarity head was significantly lower than that of the oxygen atoms in the water molecules. Thus, there were stronger electrostatic interactions between the Na+ ions and the water molecules based on the charge magnitudes.
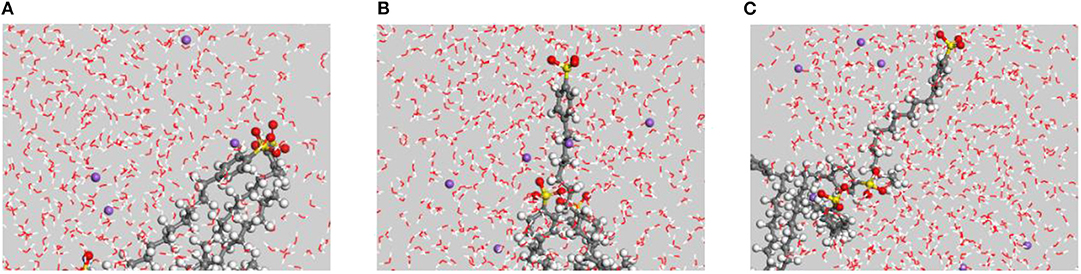
Figure 4. Na+ aggregation in the SDBS system. The purple sphere is Na+. (A) 0 ps, (B) 400 ps, and (C) 800 ps.
RDF of the sulfur atoms in the polar-head of the DBS− and Na+ ions indicates that there was no obvious aggregation in the polar-head of the DBS− or Na+ ions (Figure 5A). However, there were obvious aggregation peaks between (i) the sulfur and oxygen atoms in the water molecules and (ii) the Na+ ions and the oxygen atoms in the water molecules (Figures 5B,C, respectively). This indicates that a water layer between the Na+ ions and the polar head prevented relative proximity between them. For a Na+ ion and a polar-head to interact, the electrostatic interactions between (i) the water molecules and Na+ ions and (ii) the water molecules and the polar-heads must be broken down.
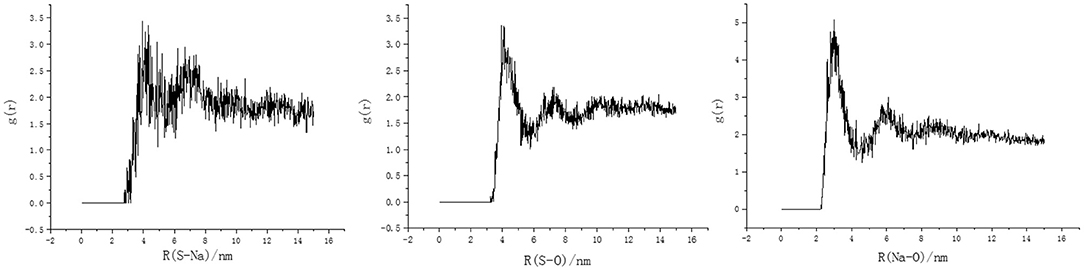
Figure 5. Radial distribution as a function of distance between the S atom in the polar-head, the O atom in water molecules, and the Na+ ions in the solution for the SDBS system. The obvious characteristic peak indicates that there is adsorption between them.
Adsorption of the SDBS and Its Isomers on the Calcium Carbonate Surface
Figure 6 shows the adsorption of SDBS and its isomers on the surface of calcium carbonate. It was observed that calcium carbonate had an adsorption effect on the SDBS and its isomers. Additionally, there were obvious aggregation peaks through the radial distribution function of the carbon atoms between the emulsifiers (Figure 7), indicating mutual aggregation between the emulsifiers at this concentration.
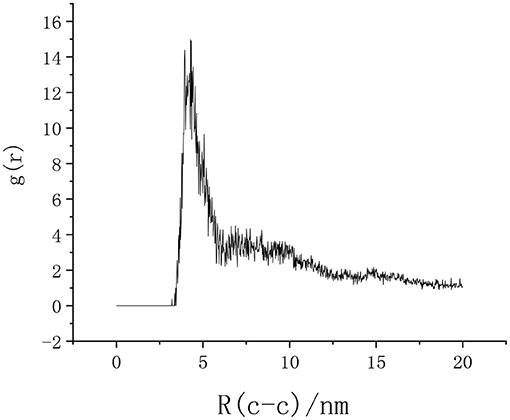
Figure 7. Radial distribution function of the emulsifier end-carbon atoms in the SDBS solution. The obvious characteristic peak indicates that there is adsorption between them.
In this system, the emulsifier molecules near the calcium carbonate surface aggregated on the solid surface to form a distinct semi-micelle structure. The emulsifier molecules far from the calcium carbonate surface also aggregated with each other because of their own electrostatic adsorption. The adsorption performances of the emulsifiers 4-3ΦC12S (Figure 6d) and 4-5ΦC12S (Figure 6e) on the calcium carbonate surface were significantly weaker than those of the other three. The calcium carbonate adsorbed only the lowermost layer of the 4-3ΦC12S and 4-5ΦC12S emulsifier molecules, and there was no adsorption effect on the half-micelle structure of the middle and upper layers of the system. This was attributed to the increased degree of branching as the aromatic hydrocarbons in the benzene sulfonate molecules moved toward the middle of the alkyl chain, which led to a higher coverage rate of the emulsifier molecules, enlarged the contact area between the emulsifier molecules and the water molecules, and led to decreased adsorption.
Further, as the benzene ring moved toward the middle of the alkyl chain, the degree of aggregation for the emulsifier in the solution gradually decreased, indicating that the critical micelle concentration increased with the degree of branching when the length of the alkyl chain remained the same. As the degree of intermolecular branching increased, the interactions between the emulsifier molecules gradually decreased. The spatial volume of the hydrophobic group increased as the benzene ring moved toward the middle of the alkyl chain, making it more difficult for the emulsifier to form a tight alignment, thereby increasing the critical micelle concentration.
Interface Energy Calculation and Analysis of the SDBS on the Calcium Carbonate Surface
The interfacial energy parameters characterize the bonding degree and adsorption performance of the solid-liquid phase. The adsorption model with the lowest energy can be obtained through MD simulations; furthermore, the energy parameters for each part could be obtained, whereupon the interface energy (Pradip, 2002) can be calculated according to
where E_total is the total energy of the system, E_surface is the energy of the underlying solid surface, E_“emulsifier” is the energy of the emulsifier solution, and ΔE is the interaction energy between the aggregate and emulsifier interface (Gao et al., 2013).
Xu et al. (2016) pointed out that the solid-liquid interface interaction energy ΔE, which characterizes the stability of the adsorption system, indicates that larger negative values give a greater interaction and stronger surface adsorption. In contrast, if ΔE is zero or positive, the emulsifier has little or no adsorption on the solid surface. The potential energies of the five emulsifier–calcium carbonate interface models were calculated using the FORCITE module in MS, and the interfacial interaction energy was calculated according to the interface energy formula.
The interfacial interaction energies of the SDBS and its isomers were consistently negative, indicating that the five emulsifiers had a certain adsorption on the calcium carbonate surface (Figure 8). Among them, the interfacial interaction energy of the 4-1ΦC12S, 4-3ΦC12S, and 4-5ΦC12S decreased as the benzene ring moved toward the middle of the alkyl chain. Furthermore, the interfacial interaction energies for the 4-3ΦC12S and 4-5ΦC12S were significantly lower than for the other three emulsifiers, indicating that their adsorption performances were weaker, which is consistent with the conclusions drawn in section Application of Molecular Dynamics Simulations for Research Into Road Materials. When the straight alkyl chain was in the ortho and meta positions of the benzene ring, the interfacial interaction energy was improved to some extent with respect to the 4-1ΦC12S, indicating that the 2-1ΦC12S and 3-1ΦC12S had better adsorption performances in the system. However, the benzenesulfonate ions of the 2-1ΦC12S were close to its own carbon chain, causing the outer electron clouds of different groups to overlap and repel each other. There was also a steric hindrance effect, making the adsorption performance lower than that of the 3-1ΦC12S.
Interfacial Diffusion of the Emulsifier (Solution)–Calcium Carbonate System
The diffusion coefficient refers to the ease by which a material passes through a unit area when the concentration gradient of the solution is constant. According to Einstein's analysis of Brownian motion, MSD has a linear relationship with time when the system is a liquid. Here, the diffusion coefficient of a particle in the emulsifier solution can be calculated from the slope of this linear relationship. Therefore, when an MD simulation is used to calculate the diffusion coefficient, the MSD curve of the particles is usually calculated from the Einstein equation (Allen and Tildesley, 1987). This relationship is given by
where D is the diffusion coefficient of the particles in the system, N is the number of diffusion molecules, and the differential term is the linear slope of the MSD vs. time. The MSD curves were obtained and statistically analyzed using the MD simulation of the post-phase trajectory files for each group model. The diffusion-coefficient relationships for the carbon atom at the end of the alkyl chain, the DBS− ion centroid, the S atom in the benzene sulfonate ions, the Na+ ions, and the emulsifier solution were obtained, as shown in Figure 9.
In the diffusion process, the diffusion coefficients of five layers were similar, which indicate that the molecular structure, degree of branching, and other factors had minimal effects on the diffusion ability of the emulsifier. However, the diffusion coefficients of the Na+ ions in different systems were significantly different. The authors believe that this is because the steric hindrance caused by different configurations of the emulsifiers was different. In the system, some atoms or groups were closer to each other with overlapping outer electron clouds. This resulted in repulsion between the atoms and groups and decreased the sensitivity of the soluble cations in the solution.
The diffusion-coefficient distributions of the carbon atom at the end of the alkyl chain and the S atom in the benzene sulfonate ions were higher than that of the DBS− ion centroid, indicating that both the oleophilic group and the hydrophilic group in the emulsifier system could promote the diffusion of the emulsifier in the solution. In the 3-1Φc12s, 4-1Φc12s, and 4-3Φc12s, the diffusion coefficient of the carbon atom at the end of the alkyl chain was higher than that of the sulfur atom in the benzene sulfonate ions, indicating that the activity of the oleophilic group was higher than that of the hydrophilic group in the system.
Experimental Study on Mass Transfer Performance of Emulsifier (Solution)–CaCO3 System
In the solution system of the emulsifier/aggregate, the mass transfer process was complete at the moment solid-liquid contact occurred. However, the relevant test parameters cannot be obtained directly using existing testing methods. Therefore, the conductivity method was used to characterize the mass transfer performance of the emulsifier onto the surface of CaCO3. This method can determine the mass transfer by measuring the conductivity of the supernatant after solid-liquid mixing and centrifugation. A higher conductivity and supernatant concentration of the emulsifier causes fewer emulsifier molecules to be adsorbed onto the CaCO3 surface in the original mixed solution, which leads to a weaker mass transfer performance.
The isomers of 12 alkylbenzene sulfonates are currently rarely sold in China because of their difficult purification process, poor biodegradability, and high price. Therefore, the reliability of the simulation data was verified using the relevant tests with the 4-1ΦC12S and 2-1ΦC12S emulsifier products due to their availability. The emulsifier reagents are shown in Figures 10a,b.
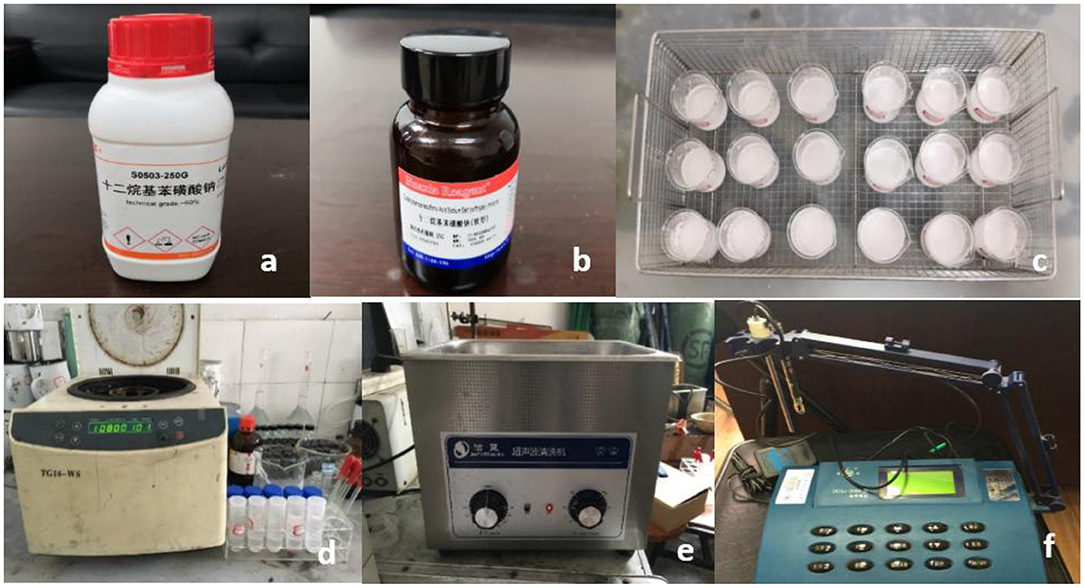
Figure 10. Test equipment. (a) 4-1ipmen, (b) 2-1ΦC12S, (c) samples, (d) TG16-WS high speed centrifuge, (e) ultrasonic cleaning machine, and (f) DDSJ-308A conductivity meter.
To verify the simulation results, the supernatants of the samples (Figure 10c) with CaCO3/emulsifier solution solid-liquid ratios of 1:1, 1:5, and 1:10 were obtained after ultrasonic oscillation and high-speed centrifugation (Figures 10d,e). The conductivity of the supernatant was measured using a DDSJ-308A conductivity meter (Figure 10f) where the test temperature was 28°C and the conductivity units were μS/cm. The results are shown in Figure 11.
It can be seen from Figure 11 that the conductivity of the 4-1ΦC12S solution was higher than that of the 2-1ΦC12S solution at the same concentration. It was shown that calcium carbonate adsorbs more 2-1ΦC12S emulsifier at the same concentration, which results in a relatively low conductivity for the upper supernatant as extracted from the experiment. This suggests that the mass transfer performance for the 2-1ΦC12S emulsifier is better than that of the 4-1ΦC12S emulsifier. The 2-1ΦC12S showed better adsorption performance on the surface of calcium carbonate, which is consistent with the calculation results for the interfacial energy in Interface Energy Calculation and Analysis of the SDBS on the calcium carbonate Surface. At the same time, it was found that a larger solid-liquid ratio of the calcium carbonate/emulsifier solution in the 4-1ΦC12S sample caused a gradually increasing conductivity. This indicates that the 4-1ΦC12S emulsifier had better adsorption performance at low solid-liquid ratios. In contrast, larger solid-liquid ratios for the 2-1ΦC12S sample showed a gradually decreasing conductivity, which indicates it had a better adsorption performance at higher solid-liquid ratios. Determining whether this phenomenon is related to interatomic repulsions caused by overlapping outer electron clouds due to the close group in the emulsifier requires further study.
Conclusions
The use of emulsified asphalt in highway construction has significant practical applications. The existing asphalt emulsifier qualities are intermingled, which leads to poor adaptability, low compatibility with asphalt, an incomplete formula design, and problems associated with quality control methods. Research on traditional asphalt emulsifiers and its formulation is often based on experience and relies on a large number of emulsifying compatibility selection experiments, which lacks systematic theoretical guidance. This study introduces a new method to examine the mass transfer of emulsifiers and their isomers on the surface of the main chemical components of aggregates through MD simulations regarding the structural design of asphalt emulsifiers for road use. Further, the mass transfer of emulsifier and its isomers on the surface of calcium carbonate, the main chemical composition of aggregate, was also investigated. The following conclusions are obtained through relevant studies:
1. During the mass transfer process, it was found that the five emulsifier molecules aggregated in a relatively short period and were adsorbed at the liquid–liquid and solid–liquid interfaces. Furthermore, the Na+ ions did not aggregate around the polar head of the emulsifier.
2. Owing to the influence of the steric hindrance, the branching degree, and other factors, the mass transfer performance of the 3-1Φc12s was the strongest while that of the 4-5Φc12s was the weakest. As the aromatics in the benzene sulfonate molecules moved to the middle of the alkyl chain, the branching degree of the molecules increased, and the mass transfer performance and degree of aggregation on the calcium carbonate surface decreased.
3. Factors such as the molecular structure and degree of branching had a limited effect on the diffusion capacity of the SDBS and its isomers. Both the lipophilic group and the hydrophilic group promoted the mass transfer performance of the emulsifier in the solution.
4. Conductivity tests showed that the 2-1ΦC12S emulsifier had an overall better mass transfer performance on the surface of the calcium carbonate than the 4-1ΦC12S emulsifier, which is consistent with the MD simulation results. At the same time, it was found that the 4-1ΦC12S emulsifier had a better mass transfer performance at lower solid-liquid ratios while the 2-1ΦC12S emulsifier was better at higher solid-liquid ratios.
Data Availability Statement
The datasets generated for this study are available on request to the corresponding author.
Author Contributions
LK proposed the research direction and provided the funds for the research. WL wrote the first draft, completed the molecular simulation, and participated in the subsequent experiments. BF and XQ participated in the experiment.
Conflict of Interest
The authors declare that the research was conducted in the absence of any commercial or financial relationships that could be construed as a potential conflict of interest.
References
Bumajdad, A., Eastoe, J., Griffiths, P., Steytler, D. C., Heenan, R. K., Lu, J. R., et al. (1999). Interfacial compositions and phase structures in mixed surfactant microemulsions. J. Langmuir 15, 5271–5278. doi: 10.1021/la990060m
Chu, J. J., and Shen, C. L. (2016). Application of emulsified asphalt in spraying rapid-hardening rubber-modified bitumen waterproof coating. J. Petroleum Asphalt 30, 55–56. doi: 10.3969/j.issn.1006-7450.2016.02.013
Conboy, J. C., Messmer, M. C., and Richmond, G. (1998). Effect of alkyl chain length on the conformation and order of simple ionic surfactants adsorbed at the d2o/ccl4 interface as studied by sum-frequency vibrational spectroscopy. J. Langmuir 14, 6722–6727. doi: 10.1021/la980132u
Ding, Y. J., Boming, T., Zhang, Y. Z., Wei, J. M., and Cao, X. J. (2014). Molecular dynamics simulation to investigate the influence of SBS on molecular agglomeration behavior of asphalt. J. Mater. Civ. Eng. 27:C4014004. doi: 10.1061/(asce)mt.1943-5533.0000998
Gao, Z. Y., Sun, W., Hu, Y. H., and Liu, X. W. (2013). Surface energies and appearances of commonly exposed surfaces of scheelite crystal. J. Trans. Nonferr. Metals Soc. China 23, 2147–2152. doi: 10.1016/S1003-6326(13)62710-7
Han, B., and Yu, X. (2014). Effect of surfactants on pressure-sensitivity of CNT filled cement mortar composites. J. Front. Mater. 1:27. doi: 10.3389/fmats.2014.00027
Han, Y., Qu, G. M., Xue, C. L., Liang, S., and Ding, W. (2016). Molecular dynamics simulation on the aggregation behavior of sodium dodecyl benzene sulfonate at air/water interface. J. Sci. Technol. Chem. Ind. 24, 22–26. doi: 10.3969/j.issn.1008-0511.2016.04.005
Hoover, W. G. (1985). Canonical dynamics: equilibrium phase-space distributions. J. Phys. Rev. A 31, 1695–1702. doi: 10.1103/PhysRevA.31.1695
Hou, Y. B., Ren, Q., Dai, Z. Y., and Zhou, H. (2018). Interaction mechanism of surfactant molecules with oil-water interfacial molecules. J. Acta Petrol. Sin. 34, 108–114. doi: 10.3969/j.issn.1001-8719.2018.01.015
Jerzy, K., and Bengt, K. (1990). On the formation and stability of concentrated water-in-oil emulsions, aphrons. J. Colloids Surf. 50, 131–140. doi: 10.1016/0166-6622(90)80258-6
Jin, S. R., Zhang, K., Pang, J. X., and Song, S. S. (2013). Synthesis and application research of op-10/ cationic surfactant composite asphalt emulsifier. Appl. Mech. Mater. 364, 664–668. doi: 10.4028/www.scientific.net/AMM.364.664
Jon, R. M., Uri, D., and Arnold, T. H. (1988). Derivation of force fields for molecular mechanics and dynamics from ab initio energy surfaces. J. Proc. Natl. Acad. Sci. U.S.A. 15, 5350–5354. doi: 10.1073/pnas.85.15.5350
Kong, L. Y. (2017). Study on Aggregate Properties and its Impact on the Mass-Transfer of Aggregate/Emulsifier System. D. Qingdao: China University of Petroleum.
Kong, L. Y., Tang, F. L., Xu, Y., Zhao, P. H., and Zhang, Y. Z. (2017). Evaluation of emulsified asphalt demulsification process by UV spectrum method. J. J. Chang'an Univ. 6, 17–23.
Kunieda, H., Ozawa, K., Aramaki, K., Nakano, A., and Solans, C. (1998). Formation of microemulsions in mixed ionic–nonionic surfactant systems. J. Langmuir 14, 260–263. doi: 10.1021/la9704112
Li, Z. Q., Guo, X. L., Wang, H. Y., Li, Q. H., Yuan, S. L., Xu, G. Y., et al. (2009). Molecular dynamics simulation of anionic surfactant aggregation at the oil/water interface. J. Acta Phys. Chim. Sin. 25, 6–12. doi: 10.3866/PKU.WHXB20090102
Lissant, K. J. (1975). Emulsions and emulsion technology. Soil. Sci. 120:160. doi: 10.1097/00010694-197508000-00017
Liu, G. Y., Gu, D. M., Ding, W., Yu, T., and Cheng, J. C. (2011). Molecular dynamics simulation of anionic surfactant aggregation at the interface. J. Acta Petrol. Sin. 27, 77–84. doi: 10.3969/j.issn.1001-8719.2011.01.013
Lu, J. R., Purcell, I. P., Lee, E. M., Simister, E. A., Thomas, R. K., Rennie, A. R., et al. (1995). The composition and structure of sodium dodecyl sulfate-dodecanol mixtures adsorbed at the air-water interface: a neutron reflection study. J. Colloid Interface Sci. 174, 441–455. doi: 10.1006/jcis.1995.1412
Maiti, P. K., Lasnsac, Y., and Glaser, M. A. (2002). Self-assembly in surfactant oligomers. J. Langmuir 18, 1908–1918. doi: 10.1021/la0111203
Matthieu, M., and Punit, B. (2019). Signature of coordination defects in the vibrational spectrum of amorphous chalcogenides. J. Front. Mater. 6:283. doi: 10.3389/fmats.2019.00283
McKenna, C. E., Knock, M. M., and Bain, C. D. (2000). First-Order phase transition in mixed monolayers of hexadecyltrimethylammonium bromide and tetradecane at the air–water interface. J. Langmuir 16, 5853–5855. doi: 10.1021/la000675f
Ni, X., Li, Z., and Wang, Y. (2018). Adsorption characteristics of anionic surfactant sodium dodecylbenzene sulfonate on the surface of montmorillonite minerals. J. Front. Chem. 6:390. doi: 10.3389/fchem.2018.00390
Ouyang, J., Han, B. G., Cao, Y., Zhou, W. J., Li, W. G., and Surendra, P. S. (2016). The role and interaction of superplasticizer and emulsifier in fresh cement asphalt emulsion paste through rheology study. J. Construct. Build. Mater. 125, 643–653. doi: 10.1016/j.conbuildmat.2016.08.085
Ouyang, J., Hu, L. J., Li, H. Y., and Han, B. G. (2018a). Effect of cement on the demulsifying behavior of over-stabilized asphalt emulsion during mixing. J. Construct. Build. Mater. 177, 252–260. doi: 10.1016/j.conbuildmat.2018.05.141
Ouyang, J., Li, H. Y., and Han, B. G. (2017). The rheological properties and mechanisms of cement asphalt emulsion paste with different charge types of emulsion. J. Construct. Build. Mater. 147, 566–575. doi: 10.1016/j.conbuildmat.2017.04.201
Ouyang, J., Pan, B. F., Xu, W., and Hu, L. J. (2019). Effect of water content on volumetric and mechanical properties of cement asphalt emulsion mixture. J. Mater. Civ. Eng. 31:04019085. doi: 10.1061/(ASCE)MT.1943-5533.0002736
Ouyang, J., Zhao, J. Y., and Tan, Y. Q. (2018b). Modelling the mechanical properties of cement asphalt emulsion mortar with different asphalt to cement ratio and temperature. J. Mater. Civ. Eng. 30:04018263. doi: 10.1061/(ASCE)MT.1943-5533.0002480
Penfold, J., Staples, E., Tucker, I., Soubiran, L., Creeth, A., and Hubbard, J. (2000). Adsorption of di-chain cationic and non-ionic surfactant mixtures at the air/water interface. J. Phys. Chem. Chem. Phys. 2, 5230–5234. doi: 10.1039/b003439h
Penfold, J., Staples, E., Tucker, I., Soubiran, L., Lodi, A. K., Thompson, L., et al. (1998). Structure and composition of the mixed monolayer of hexadecyltrimethylammonium bromide and benzyl alcohol adsorbed at the air/water interface. J. Langmuir 14, 2139–2144. doi: 10.1021/la9710740
Pradip, R. B. (2002). Design of tailor-made surfactants for industrial applications using a molecular modelling approach. J. Colloids Surf. A Physicochem. Eng. Asp. 205, 139–148. doi: 10.1016/S0927-7757(01)01153-0
Rappé, A. K., Casewit, C. J., Colwell, K. S., Goddard, W. A., and Skiff, W. M. (1992). UFF, a full periodic table force field for molecular mechanics and molecular dynamics simulations. J. Am. Chem. Soc. 114, 10024–10035. doi: 10.1021/ja00051a040
Rappé, A. K., and Goddard, W. A. (1991). Charge equilibration for molecular-dynamics simulations. J. Phys. Chem. 95, 3358–3363. doi: 10.1021/j100161a070
Seref, O., Fazil, C., and Akpinar, M. V. (2006). Effect of cement on emulsified asphalt mixtures. J. JMEPEG 16, 578–583. doi: 10.1007/s11665-007-9095-2
Sitepu, H. (2009). Texture and structural refinement using neutron diffraction data from molybdite (MoO3) and calcite (CaCO3) powders and a Ni-rich Ni50.7 Ti49.30 alloy. J. Am. Chem. Soc. 24, 315–326. doi: 10.1154/1.3257906
Somasundaran, P., Fuerstenau, D. W., and Healy, T. W. (1964). Surfactant adsorption at the solid—liquid interface—dependence of mechanism on chain length. J. Phys. Chem. 68, 3562–3566. doi: 10.1021/j100794a021
Song, Q. S., Guo, X. L., Yuan, S. L., and Liu, C. B. (2009). Molecular dynamics simulation of sodium dodecyl benzene sulfonate aggregation on silica surface. J. Acta Phys. Chim. Sin. 25, 1053–1058. doi: 10.3866/PKU.WHXB20090623
Sun, H. (1998). COMPASS: an ab initio force-field optimized for condensed-phase applications overview with details on alkane and benzene compounds. J. Am. Chem. Soc. 114, 10024–10039. doi: 10.1021/jp980939v
Tan, Y. Q., Ouyang, J., Lv, J. F., and Li, Y. L. (2013). Effect of emulsifier on cement hydration in cement asphalt mortar. J. Construct. Build. Mater. 47, 159–164. doi: 10.1016/j.conbuildmat.2013.04.044
Vekeman, J., Faginas-Lago, N., Lombardi, A., Sánchez de Merás, A., García Cuesta, I., and Rosi, M. (2019). Molecular dynamics of CH4/N2 mixtures on a flexible graphene layer: adsorption and selectivity case study. Front. Chem. 7:386. doi: 10.3389/fchem.2019.00386
Wang, Y. X., Gao, Y. Q., Zhang, Q., and Meng, Q. (2018). Novel cationic emulsifier used for preparing slow-cracking and rapid-setting asphalt: synthesis, surface activity and emulsification ability. J. Disper. Sci. Technol. 39, 478–483. doi: 10.1080/01932691.2015.1120676
Wu, B. G., Yang, Y. R., Pan, X. F., Wang, X. S., and Liu, G. P. (1992). Novel synthetic method of lingninamine-type asphalt emulsifier. J. Northeast For. Univ. 3, 89–94.
Xu, L. H., Dong, F. Q., Wu, H. Q., Liu, W., Wang, Z., and Wang, J. M. (2016). Flotation separation of anionic collectors—surface crystal chemistry of spondite and kaolinite. J. Acta Mineral. Sin. 36, 265–270. doi: 10.16461/j.cnki.1000-4734.2016.02.015
Yan, L. M., and Zhu, S. H. (2013). Theory and Practice of Molecular Dynamics Simulation[M]. Beijing: Science Press.
Yang, L. H. (2004). The Technology of Modified Asphalt and Modified Asphalt Emulsion [M]. Beijing: Communications Press.
Yongjoo, K., Soohyok, I., and Hosin, L. (2011). Impacts of curing time and moisture content on engineering properties of cold in-place recycling mixtures using foamed or emulsified asphalt. J. Mater. Civ. Eng. 23, 542–553. doi: 10.1061/(ASCE)MT.1943-5533002E0000209
Zhao, H., Li, H. P., Liao, K. J., and Li, Y. G. (2012). The anti-aging performance of emulsified asphalt. J. Pet. Sci. Technol. 30, 193–200. doi: 10.1080/10916461003792336
Keywords: solid–liquid interface, mass transfer, molecular dynamics simulation, anionic asphalt emulsifier, road engineering
Citation: Kong L, Luo W, Feng B and Quan X (2020) Influence of Emulsifier on Surface Mass Transfer Based on Molecular Dynamics Simulations. Front. Mater. 7:1. doi: 10.3389/fmats.2020.00001
Received: 02 December 2019; Accepted: 03 January 2020;
Published: 30 January 2020.
Edited by:
Jian Ouyang, Dalian University of Technology (DUT), ChinaReviewed by:
Pengfei Liu, RWTH Aachen University, GermanyBaoguo Han, Dalian University of Technology (DUT), China
Copyright © 2020 Kong, Luo, Feng and Quan. This is an open-access article distributed under the terms of the Creative Commons Attribution License (CC BY). The use, distribution or reproduction in other forums is permitted, provided the original author(s) and the copyright owner(s) are credited and that the original publication in this journal is cited, in accordance with accepted academic practice. No use, distribution or reproduction is permitted which does not comply with these terms.
*Correspondence: Lingyun Kong, 43112443@qq.com