- 1State Key Laboratory of Marine Resource Utilization in South China Sea, Hainan University, Haikou, China
- 2School of Materials Science and Engineering, Hainan University, Haikou, China
3D bare TiO2 nanotube arrays loaded with Au nanoparticles (3D Au-NPs/TNTAs) were prepared to effectively enhance the photoelectrocatalytic properties. A simple flow injection sensing system was proposed for the real-time on-line determination of chemical oxygen demand using the 3D Au-NPs/TNTAs electrode. The proposed photoelectrochemical oxidative degradation principle of the sensing system was analyzed and validated by the representative organic compounds with known COD values at a potential bias 1.5 V under UV illumination. A practical detection limit of the sensing system is 0.18 mg/L and the linear range is 1.92–3,360 mg/L under the optimum conditions. In the experimental process, the sensing system exhibits long-term stability and good reproducibility.
Introduction
Chemical oxygen demand (COD) is the oxygen equivalent on degradation of the organic compounds, which is a significant parameter of water quality assessment (Kondo et al., 2014). Even though potassium dichromate oxidation method is widely used, many inherent shortcomings such as time-consuming (2–4 h) reflux process, highly toxic chemicals, corrosiveness and high cost (Ag2SO4) were existing (Allan Moore et al., 1949; Zhang J. et al., 2009; Zhang S. et al., 2009). Photocatalytic methods can effectively solve these problems, but the resulting electron hole pairs are easy to recombine. Currently, an environmentally friendly and rapid photoelectrochemical COD determination way was considered a more promising approach to surmount the recombination of electron-hole pairs further to improve the degradation efficiency (Qu et al., 2011; Li et al., 2012).
However, the photoelectrocatalytic COD determination method implies a relatively narrow working range and poor reproducibility with a stop-flow operation mode in the quartz cell. Also, the shortcomings of conventional photoelectrochemical method are as follows: one is the complex three-electrode structure, the other is that it's hard to improve sensitivity because of inadequate degradation efficiency of working electrode (Liao et al., 2016).
TiO2 nanotube arrays (TNTAs) extending on the Ti foil have been used as sensing electrode to detect chemical oxygen demand (COD) in recent years, owning to its intrinsic nanotubular architecture and remarkable properties (Li et al., 2006; Grimes, 2007; Zheng et al., 2008). Ti foil could be entirely transformed into nanotubes but a few micrometers thickness of nanotubes are sufficient for performing efficient photocatalysis. In addition, it is easy to have scattering effects in the liquid (Paulose et al., 2007; Kar and Smith, 2009). It's wasteful for Ti foil since it has relatively much loss of photons (Zhuang et al., 2007; Beranek et al., 2009). However, three-dimensional (3D) TNTAs photoelectrode extending radically on a grid of titanium wires can avoid this wastage and because of absorbing the reflected and/or refracted light (Liao et al., 2012). Furthermore, the 3D TNTAs on Ti mesh possess larger surface area than TiO2 nanotube arrays on the Ti foil which means higher photocatalytic activity. Nevertheless, TiO2 nanotube arrays show shorter lifetime, poor stability, unsatisfactory photocatalytic activity in real application for COD analysis (Liang et al., 2019). Therefore, it is important to propose a new sensor further to improve the weakness of present TiO2 sensing.
To tackle these problems, we propose a flow injection photoelectrocatalytic COD determination method by combining continuous flow injection thin-cell reactor mode and Au nanoparticles (Au-NPs) loaded on 3D TNTAs. The influence of annealing temperature and anodizing time on the as-prepared bare 3D TNTAs were studied. The as-prepared 3D TiO2 nanotube arrays were characterized via material characterizations and photoelectrochemical testing, such as FE-SEM, EDS, XRD, photocurrent-density potential characteristics and chronoamperometry experiments, to investigate their morphology, elements, structure, and photoelectrocatalytic activities. A new COD determination analytical principle was proposed, which was experimentally validated with representative organic compounds. Because of the different adsorption position on the surface of TiO2, glucose, glutamic acid and GGA (glucose: glutamic acid = 1:1) were chosen as the representative organic compounds (Zhang et al., 2006; Han et al., 2010; Liu et al., 2014). The analytical signal generation of the proposed sensing system was optimized on the important experimental parameters such as supporting electrolyte and applied potential bias.
Experimental Section
Preparation of 3D Au-NPs/TNTAs/Ti
The working electrode was prepared by following steps. Firstly, titanium mesh (>99% purity, 50 meshes) was sliced rectangle pieces of 30 × 70 mm, which were fixed by titanium wire. Above treated titanium meshes were dipped into the mixture solution (HF: HNO3: H2O = 1:4:5 in v/v) about 30 s to polish, then treated with acetone, ethanol and ultrapure water by ultra-sonication in order. Subsequently, dried in air. Secondly, the treated titanium mesh was electrochemical anodization in ethylene glycol electrolyte solution (2 wt% H2O and 0.3 wt% NH4F) by two electrode configurations in different anodization time 10, 20, 30, and 60 min. With the rate of heating 2°C min−1, the obtained samples were calcinated at 350, 450, 550, and 650°C for 3 h in muffle furnace, respectively. Among them, the electrode material with the best oxidation ability was selected as the as-prepared working electrode. Thirdly, to improve photocatalytic activity and stability of the as-prepared working electrode, Au nanoparticles were deposited on the bare 3D TNTAs by the photo-reduction method. The as-prepared working electrode was dipped into the electrolyte consisted of mixture (HAuCl4 (0.1g/L): HCHO = 100:1 in v/v) in the quartz cell under UV irradiation for 40 s. Then the electrode was washed with ethanol, deionized water and dried in the air, which then was used as the working electrode. As for the counter electrode, Pt nanoparticles were electrodeposited on the titanium mesh treated above at −0.3V for 3 min in the mixed solution (H2PtCl6 (0.2g/L): HCHO = 100:1 in v/v) (Si et al., 2019).
Characterization
The morphologies and microstructure of the electrode material were studied by scanning electron microscopy (FE-SEM) equipped with an energy-dispersive spectrometer (EDS). Crystal phase of the working electrode was analyzed by X-ray diffraction (XRD, Bruker D8) with Cu Kα radiation. To choose a 3D TNTAs electrode material with best oxidation ability, linear sweep voltammetry (LSV) was performed in cube quartz reactor (50 × 50 × 50 mm) at room temperature using saturated Ag/AgCl (KCl) reference electrode, platinum foil counter electrode and the as-prepared 3D TiO2 nanotube arrays working electrode in all the experiments.
Fabrication of Flow Injection Sensing System and Measurement of Their Photoelectrocatalytic Real-Time On-Line COD Sensing Properties
Au modified 3D TiO2 Nanotube Arrays as sensing electrode was used for real-time on-line COD determination in our proposed thin-cell reactor which can be seen from our previous work (Si et al., 2019). The schematic diagram and digital image of the real-time on-line thin-cell reactor was shown in Figure S1. The application potential bias and the recording of response current signal were supplied by the electrochemical workstation (Zahner Zennium, Germany). A 5W UV lamp was used as the UV source in the experiment. A 0.2 mM Na2SO4 solution was as the supporting electrolyte in the photoelectrochemical measurements.
Results and Discussion
Characterization of the Electrode Materials
The effect of preparation parameters of anodic time and calcination temperature on the saturated photocurrent density of the as-prepared electrodes was obtained in LSV under the UV illumination (Zhang J. et al., 2009). As shown in Table S1, the electrode with anodic oxidation for 10 min and calcination temperature at 450°C possess the highest saturated photocurrent density. Therefore, the electrode which is prepared at 60 V for anodic time 10 min and calcination temperature at 450°C for 3 h (No. 2 in Table S1) was selected as the as-prepared working electrode in our proposed flow injection sensing system.
Figure 1 shows the surface morphology of the working electrode material where highly-ordered TNTAs are distributed uniformly and aligned vertically on the Ti meshes (Liao et al., 2012). The average inner diameter of the 3D TNTAs is 65 nm with length of 3 um, as depicted in Figures 1B,C. Figure 1D displays Au-NPs loaded on the 3D TNTAs and the EDS spectrum further identify the presence of Au-NPs on the bare 3D TNTAs where the weight of Au is 0.48% (Figure S2). The XPS results show the coexistence of Ti, O and Au elements in the prepared nanocomposite, as shown in Figure S3. From the XPS spectra, Ti 2p1/2 and Ti 2p3/2 were obtained at 464 eV and 458 eV while O 1s was at 530 eV, which are all consistent with the literature reports (Luo et al., 2016; Siavash et al., 2017). Furthermore, the existence of Au in the nanocomposite is confirmed by the Au 4f7/2 and Au 4f5/2 peaks at 83 and 86.7 eV, respectively. The role of Au in the Au/TiO2 composite enhances the electrical conductivity and raises the optical conductivity further to improve the photoelectrocatalytic properties (Rudra et al., 2018a,b).
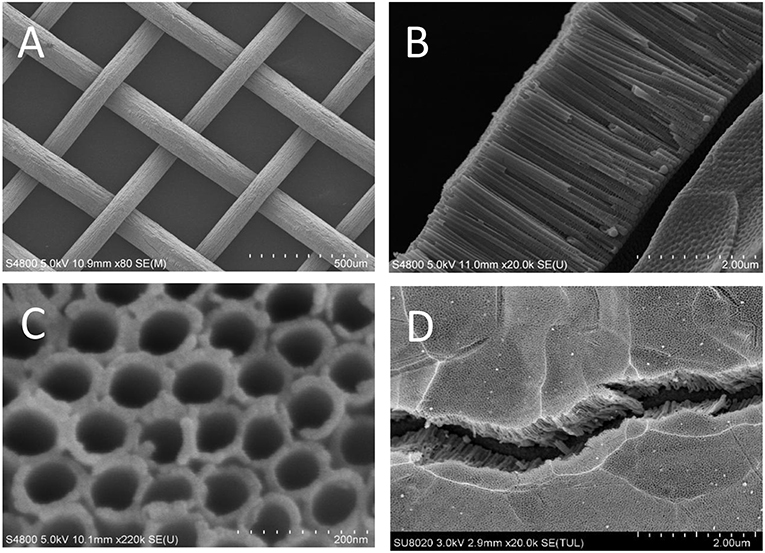
Figure 1. SEM images: (A) Ti meshes, (B) side view and (C) top view of the bare 3D TNTAs, (D) Au-NPs on the bare 3D TNTAs.
Figure 2 shows the XRD patterns of the 3D TNTAs before, and after calcinated. The diffraction peaks attributed to the formation of anatase TiO2 structure after annealing, which has higher photocatalytic activity (Zhang et al., 2016). There appears rutile when the annealing temperature reaches to 650°C. Furthermore, the photocurrent response of the samples annealed at different temperatures were measured in comparation to that of Au nanoparticles modified TiO2 nanotube arrays, as depicted in Figure S4. Among them, the sample annealed at 450°C has the optimal photocurrent density. The obtained working electrode possess the higher photocurrent density compared to pure TiO2 nanotube arrays, indicating the existence of Au nanoparticles can improve the sensing property.
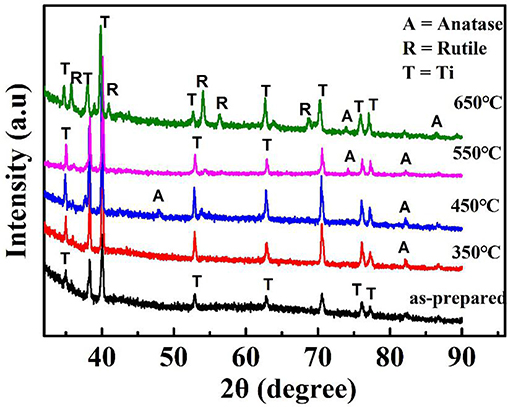
Figure 2. XRD patterns of the reduced at 350, 450, 550, and 650°C, respectively and non-reduced as-prepared 3D TNTAs electrode.
The Photoelectrocatalytic Properties of the Sensing System
Figure 3 shows photocurrent response of the sensing system as a function of time at the repeated on/off cycles with applied potential 1.5 V under UV illumination. The 0.2 mM Na2SO4 solution is as the supporting electrolyte. The photocurrent density of non-reduced bare 3D TNTAs was measured nearly to be 0 mA without UV light, but a significant increasing in current value was seen under UV illumination. The non-light current was measured to be low upon switch off the light, but the current quickly increased when turned on the UV light, indicating the sensing system responds quickly to UV source.
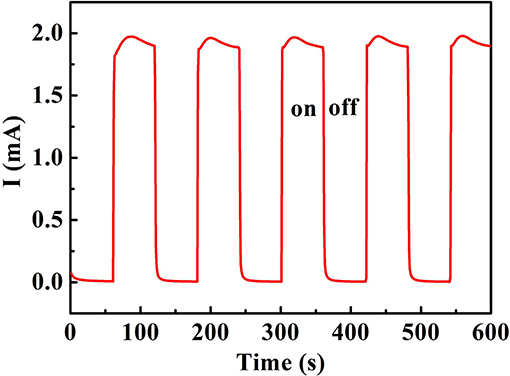
Figure 3. Photocurrent responses of the sensing system as a function of time at the repeated on/off cycles with an applied potential 1.5 V under UV illumination. 0.2 mM Na2SO4 solution as the supporting electrolyte.
Figure 4 shows linear sweep voltammograms of the Au-NPs/3D TNTAs electrode in 0.2 mM Na2SO4 and 1 mM glucose in 0.2 mM Na2SO4 solution with and without UV irradiation, respectively, in order to investigate the response of oxidation current produced by degradation of organic compounds. As we can see from Figure 4, there was almost no response to oxidation current when glucose was added in the non-UV light (Figure 4A). However, a significant oxidation current appears when ultraviolet light was applied (Figure 4B).
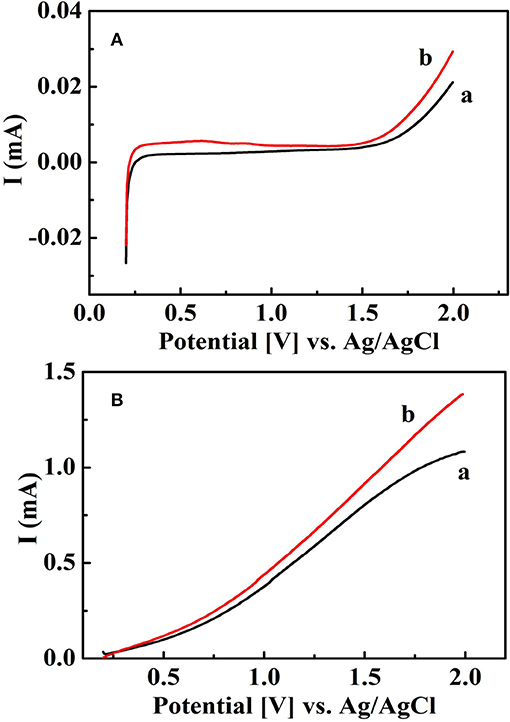
Figure 4. Linear voltammograms of the sensing system: (a) 0.2 mM Na2SO4 and (b) 0.2 mM Na2SO4 containing glucose organic using 3D Au-NPs/TNTAs sensing electrode at the potential scan rate of 10 mVs−1 without (A) and with (B) UV illumination.
COD Sensing Mechanism
A new detection principle was proposed based on the postulate that the potential of the counter electrode
The analog circuit diagram of the proposed COD sensor is as follows Figure 5:
According to the “Closed Circuit Ohm Law,” for the proposed COD sensor
Where E is the applied voltage; vreactor is the voltage on the photoelectrochemical reactor; I is the current in the closed circuit; R is the resistance of the closed circuit except reactor.
When ultraviolet light irradiates the sensing electrode, photoholes possess strong oxidizing capacities which can mineralize organic compounds. Assuming that the organic compounds are stoichiometrically mineralized on surface of the sensing electrode, the potential of sensing electrode can be given by Nernst equation
Where Cm, T, k, n, A are the molar concentration, temperature, Boltzmann constant, the electron transfer number of the individual organic compound and constant, respectively.
Therefore, the applied voltage on the reactor can be given as follows:
According to the relationship between molar concentration (Cm) and COD concentration [COD] = 8000 nCm (Wang et al., 2013), then Equation (4) can be given by:
Figure 6 shows the photocurrent-time profiles with the absence (iblank) and existence (itotal) of organic compounds using the proposed sensing system. When the sensing electrode is illuminated with UV light, iblank obtained in the supporting electrolyte mainly from the oxidation of water, while the observed photocurrent (itotal) in the sample organic solution originates from both the oxidation of water and the photoelectrocatalytic oxidation of organic compounds. As we know, inet (net steady state current) originates from itotal via subtracting the iblank, which is the step between the blank photocurrent and the total photocurrent in Figure 6 (Chen et al., 2012).
Inet can be transformed into the equivalent COD values according to Faraday's law.
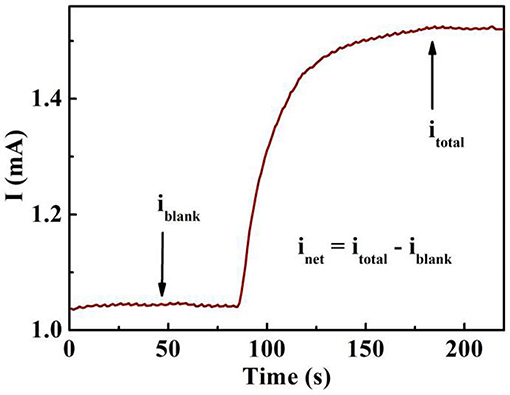
Figure 6. Photocurrent response of a 0.2 mM Na2SO4 blank solution (iblank) and the 0.2 mM Na2SO4 solution containing organic compounds (itotal). The step originates from the oxidation of the organic compounds.
According to Equations (2), (5), and (6), Inet can be given by:
Where both α and A are constant. The Inet possess linear relationship with the logarithm of the COD value.
COD Sensing Properties
Photogenerated hole is a strong oxidant for degradation of organic compounds during the process of photocatalytic oxidation. The combination of photogenerated electrons and holes often limits the degradation efficiency. However, the addition of a bias voltage can transfer photogenerated electrons to the internal circuit, thereby improving the degradation efficiency (Zheng et al., 2008). In order to find the proper applied bias, the effect of applied bias on net current is studied under different bias conditions (Li et al., 2014), as shown in Figure 7A. The determined Inet values are lower than that the applied bias value is above 1 V, however, the obtained Inet values are not fluctuation when the applied voltages are between 1.0 and 1.5 V. In all the experiments, the applied bias 1.5 V was chosen as the proper voltage. In addition, the current signal is influenced by the large solution resistance due to the solution channel in the thin-layer reactor. The influence of supporting electrolyte concentration on the generated photocurrent is also studied. In Figure 7B, the current rises with the increase of conductive ions at a certain concentration range. The current reaches its saturation value at higher electrolyte concentration. Thus, 0.2 mM Na2SO4 was selected as the optimal supporting electrolyte concentration.
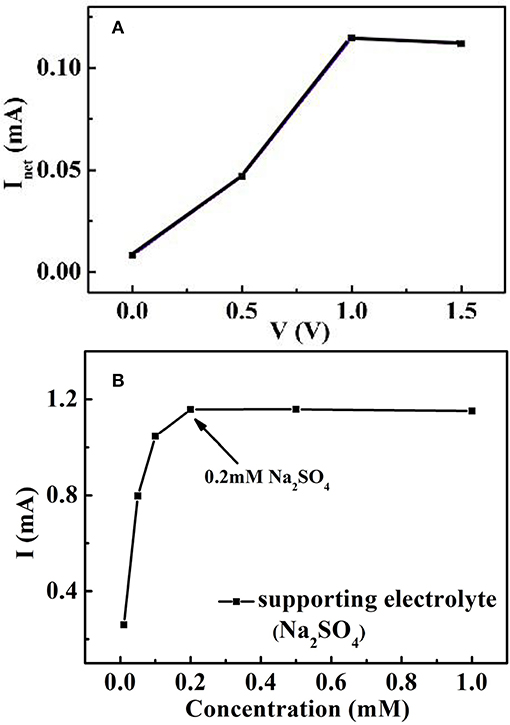
Figure 7. Optimization of the real-time on-line sensing system: effect of (A) applied voltage and (B) supporting electrolyte concentration on photocurrent.
In order to validate the proposed COD analysis principle, some pure organic compounds such as glucose and sucrose and synthetic sample (GGA) were chosen as the representative organic compounds. Chronoamperometric experiments were performed via injecting various molar concentration organic compounds at a constant applied bias V = 1.5 V with UV light. When various concentration organic compounds were added, the rapidly raising photocurrent responses were obtained and then recorded by the electrochemical workstation (Zahner zennium, Germany). The relationship between Inet and molar concentration of individual organic compounds with equal COD values of each is presented in Figure 8A. In addition, the relationship between Inet and the equivalent theoretical COD values converted from the molar concentrations of organic compounds ([COD] = 8000nCm) were deduced in Figure 8B. In the figure, Inet possess linear relationship with the logarithm of the organic compound concentrations studied in this paper. The plots display that the investigated organic compounds are fitted into a line y = 0.000887 log(x) – 3.22×10−4 (where y represents Inet obtained from organics oxidation, x represents the COD value of the organics) with R2 = 0.975, indicating the proportional relationship between the Inet and the COD value for the 3D Au-NPs/TNTAs COD sensing system. Furthermore, a wide working linear range from 1.92 to 3,360 mg L−1 were obtained. The proposed COD sensing system also possess a detection limit of 0.18 mg L−1 COD (S/N=3).
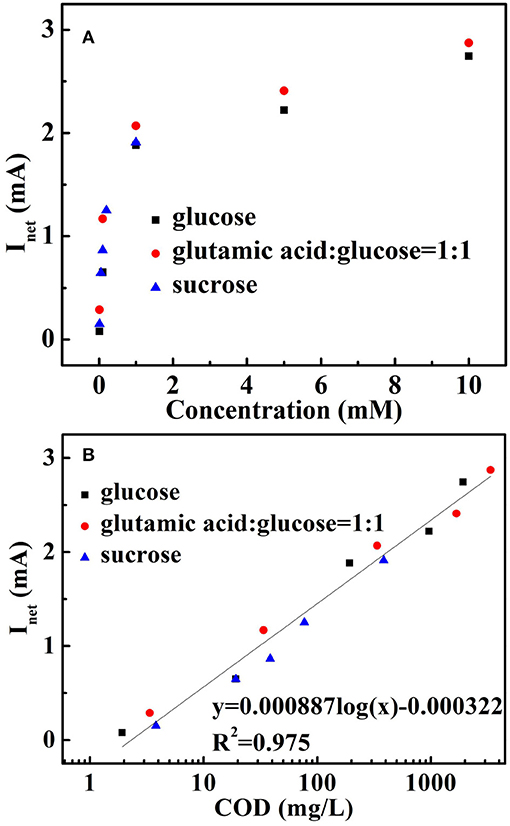
Figure 8. Verification of analysis principle: (A) the correlation of Inet and molar concentrations of selected organic compounds; (B) the relationship between Inet and the theoretical COD values of the selected organic compounds.
Reproducibility and Operational Stability of the Proposed Sensing System
The reproducibility of the photoelectrochemical sensor was assessed by measuring the current signal at 19.2 mg/L COD (Yao et al., 2015). The relative standard deviation was 2.5% for 11 determinations, suggesting excellent reproducibility. To study the long-term stability about the proposed sensing system, response photocurrent to 0.1 mM glucose (theoretical COD value 19.2 mg/L) was measured during 10 days period. The RSD is 3.7%, showing good stability of the sensing system.
Conclusions
A real-time on-line sensing system combining with 3D Au-NPs/TNTAs sensing electrode and photoelectrochemical reactor is proposed toward the determination of COD, which is on account of the relationship between the COD values and the quantity captured charge applying the photoelelctrocatalytic method. In the photoelectrochemical experiments, the optimum conditions such as applied bias and supporting electrolyte were investigated. Glucose, sucrose and GGA were chosen as the representative organic compounds to validated the proposed sensing principle. The sensing photocurrent presents a linear relationship with the logarithm of COD values. The proposed sensing system shows a detection limit of 0.18 mg/L with the linear range from 1.96 to 3,360 mg/L.
Data Availability Statement
All datasets generated for this study are included in the manuscript/Supplementary Files.
Author Contributions
HS designed and conducted the experiments and analyzed the results. XZ prepared the bare 3D TNTAs nanotube arrays. SL revised, read, and approved the submitted version.
Funding
This work was supported by the National Natural Science Foundation of China (Grant No. 61764003), Major Science and Technology Planning Project of Hainan Province (ZDKJ201810).
Conflict of Interest
The authors declare that the research was conducted in the absence of any commercial or financial relationships that could be construed as a potential conflict of interest.
Supplementary Material
The Supplementary Material for this article can be found online at: https://www.frontiersin.org/articles/10.3389/fmats.2019.00238/full#supplementary-material
References
Allan Moore, W., Kroner, R. C., and Ruchhoft, C. C. (1949). Dichromate reflux method for determination of oxygen consumed. Anal. Chem. 21, 953–957. doi: 10.1021/ac60032a020
Beranek, R., Macak, J. M., Gärtner, M., Meyer, K., and Schmuki, P. (2009). Enhanced visible light photocurrent generation at surface-modified TiO2 nanotubes. Electrochim. Acta 54, 2640–2646. doi: 10.1016/j.electacta.2008.10.063
Chen, H., Zhang, J., Chen, Q., Li, J., Li, D., Dong, C., et al. (2012). Assessment of a COD analytical method based on the photoelectrocatalysis of a TiO2 nanotube array sensor. Anal. Methods 4:1790. doi: 10.1039/c2ay05940a
Grimes, C. A. (2007). Synthesis and application of highly ordered arrays of TiO2 nanotubes. J. Mater. Chem. 17, 1451–1457. doi: 10.1039/b701168g
Han, Y., Zhang, S., Zhao, H., Wen, W., Zhang, H., Wang, H., et al. (2010). Photoelectrochemical characterization of a robust TiO2/BDD Heterojunction electrode for sensing application in aqueous solutions. Langmuir 26, 6033–6040. doi: 10.1021/la903706e
Kar, A., and Smith, Y. R. (2009). Improved photocatalytic degradation of textile dye using titanium dioxide nanotubes formed over titanium wires. Environ. Sci. Technol. 43, 3260–3265. doi: 10.1021/es8031049
Kondo, T., Tamura, Y., Hoshino, M., Watanabe, T., Aikawa, T., Yuasa, M., et al. (2014). Direct determination of chemical oxygen demand by anodic decomposition of organic compounds at a diamond electrode. Anal. Chem. 86, 8066–8072. doi: 10.1021/ac500919k
Li, J., Zheng, L., Li, L., Shi, G., Xian, Y., and Jin, L. (2006). Photoelectro-synergistic catalysis at Ti/TiO2/PbO2 electrode and its application on determination of chemical oxygen demand. Electroanalysis 18, 2251–2256. doi: 10.1002/elan.200603644
Li, L., Zhang, S., Li, G., and Zhao, H. (2012). Determination of chemical oxygen demand of nitrogenous organic compounds in wastewater using synergetic photoelectrocatalytic oxidation effect at TiO2 nanostructured electrode. Anal. Chim. Acta 754, 47–53. doi: 10.1016/j.aca.2012.10.008
Li, X., Bai, J., Liu, Q., Li, J., and Zhou, B. (2014). WO3/W nanopores sensor for Chemical Oxygen Demand (COD) determination under visible light. Sensors 14, 10680–10690. doi: 10.3390/s140610680
Liang, L., Yin, J., Bao, J., Cong, L., Huang, W., Lin, H., et al. (2019). Preparation of Au nanoparticles modified TiO2 nanotube array sensor and its application as chemical oxygen demand sensor. Chin. Chem. Lett. 30, 167–170. doi: 10.1016/j.cclet.2018.01.049
Liao, J., Lin, S., Zeng, M., and Yang, Y. (2016). A miniature photoelectrochemical sensor based on organic electrochemical transistor for sensitive determination of chemical oxygen demand in wastewaters. Water Res. 94, 296–304. doi: 10.1016/j.watres.2016.02.061
Liao, J., Lin, S., Zhang, L., Pan, N., Cao, X., and Li, J. (2012). Photocatalytic degradation of methyl orange using a TiO2/Ti mesh electrode with 3D nanotube arrays. ACS Appl. Mater. Interfaces 4, 171–177. doi: 10.1021/am201220e
Liu, C., Zhao, H., Ma, Z., An, T., Lau, C., Zhao, L., et al. (2014). Novel environmental analytical system based on combined biodegradation and photoelectrocatalytic detection principles for rapid determination of organic pollutants in wastewaters. Environ. Sci. Technol. 48, 1762–8. doi: 10.1021/es4031358
Luo, J., Chen, J., Wang, H., and Liu, H. (2016). Ligand-exchange assisted preparation of plasmonic Au / TiO2 nanotube arrays photoanodes for visible-light-driven photoelectrochemical water splitting. J. Power Sources 303, 287–293. doi: 10.1016/j.jpowsour.2015.11.016
Paulose, M., Prakasam, H. E., Varghese, O. K., Peng, L., Popat, K. C., Mor, G. K., et al. (2007). TiO2 nanotube arrays of 1000 μm length by anodization of titanium foil: phenol red diffusion. J. Phys. Chem. C 111, 14992–14997. doi: 10.1021/jp075258r
Qu, X., Tian, M., Chen, S., Liao, B., and Chen, A. (2011). Determination of chemical oxygen demand based on novel photoelectro-bifunctional electrodes. Electroanalysis 23, 1267–1275. doi: 10.1002/elan.201000641
Rudra, S., Nayak, A. K., Chakraborty, R., Maji, P. K., and Pradhan, M. (2018a). Synthesis of Au-V2O5 composite nanowires through the shape transformation of a vanadium(iii) metal complex for high-performance solid-state supercapacitors. Inorg. Chem. Front. 5, 1836–1843. doi: 10.1039/C8QI00325D
Rudra, S., Nayak, A. K., Koley, S., Chakraborty, R., Maji, P. K., and Pradhan, M. (2018b). Redox-mediated shape transformation of Fe3O4 nanoflakes to chemically stable Au–Fe2O3 composite nanorods for a high-performance asymmetric solid-state supercapacitor device. ACS Sustain. Chem. Eng. 7, 724–733. doi: 10.1021/acssuschemeng.8b04300
Si, H., Pan, N., Zhang, X., Liao, J., Rumyantseva, M. N., Gaskov, A. M., et al. (2019). A real-time on-line photoelectrochemical sensor toward chemical oxygen demand determination based on field-effect transistor using an extended gate with 3D TiO2 nanotube arrays. Sens. Actuators B Chem. 289, 106–113. doi: 10.1016/j.snb.2019.03.071
Siavash, R., Kia, G., Goh, L., Dolati, A., and Ghorbani, M. (2017). Applied catalysis B: environmental sunlight-driven photoelectrochemical sensor for direct determination of hexavalent chromium based on Au decorated rutile TiO2 nanorods. Appl. Catal. B Environ. 201, 411–418. doi: 10.1016/j.apcatb.2016.08.026
Wang, C., Wu, J., Wang, P., Ao, Y., Hou, J., and Qian, J. (2013). Photoelectrocatalytic determination of chemical oxygen demand under visible light using Cu2O-loaded TiO2 nanotube arrays electrode. Sens. Actua. B Chem. 181, 1–8. doi: 10.1016/j.snb.2013.02.011
Yao, N., Liu, Z., Chen, Y., Zhou, Y., and Xie, B. (2015). A novel thermal sensor for the sensitive measurement of chemical oxygen demand. Sensors 15, 20501–20510. doi: 10.3390/s150820501
Zhang, J., Zhou, B., Zheng, Q., Li, J., Bai, J., Liu, Y., et al. (2009). Photoelectrocatalytic COD determination method using highly ordered TiO2 nanotube array. Water Res. 43, 1986–1992. doi: 10.1016/j.watres.2009.01.035
Zhang, S., Jiang, D., and Zhao, H. (2006). Development of chemical oxygen demand on-line monitoring system based on a photoelectrochemical degradation principle. Environ. Sci. Technol. 40, 2363–2368. doi: 10.1021/es052018l
Zhang, S., Li, L., and Zhao, H. (2009). A portable photoelectrochemical probe for rapid determination of chemical oxygen demand in wastewaters. Environ. Sci. Technol. 43, 7810–7815. doi: 10.1021/es901320a
Zhang, Z., Chang, X., and Chen, A. (2016). Determination of chemical oxygen demand based on photoelectrocatalysis of nanoporous TiO2 electrodes. Sens. Actua. B Chem. 223, 664–670. doi: 10.1016/j.snb.2015.10.001
Zheng, Q., Zhou, B., Bai, J., Li, L., Jin, Z., Zhang, J., et al. (2008). Self-organized TiO2 nanotube array sensor for the determination of chemical oxygen demand. Adv. Mater. 20, 1044–1049. doi: 10.1002/adma.200701619
Keywords: 3D Au-NPs/TNTAs, photoelectrocatalytic, chemical oxygen demand (COD), flow injection sensing, real-time on-line COD determination
Citation: Si H, Zhang X and Lin S (2019) A Simple Flow Injection Sensing System for the Real-Time On-Line Determination of Chemical Oxygen Demand Based on 3D Au-NPs/TiO2 Nanotube Arrays. Front. Mater. 6:238. doi: 10.3389/fmats.2019.00238
Received: 14 May 2019; Accepted: 12 September 2019;
Published: 04 October 2019.
Edited by:
Girish Kale, University of Leeds, United KingdomReviewed by:
Mukul Pradhan, National Institute of Technology Meghalaya, IndiaRamendra Sundar Dey, Institute of Nano Science and Technology (INST), India
Copyright © 2019 Si, Zhang and Lin. This is an open-access article distributed under the terms of the Creative Commons Attribution License (CC BY). The use, distribution or reproduction in other forums is permitted, provided the original author(s) and the copyright owner(s) are credited and that the original publication in this journal is cited, in accordance with accepted academic practice. No use, distribution or reproduction is permitted which does not comply with these terms.
*Correspondence: Shiwei Lin, linsw@hainanu.edu.cn