- Key Laboratory of C&PC Structures of the Ministry of Education, Southeast University, Nanjing, China
Harmful vibrations can cause many engineering problems, such as disasters of civil engineering structures, failure of precision equipment, and the disruption of some spacecraft components. In most situations, the vibrations are usually three-dimensional excitations. Therefore, research on multi-dimensional vibration control has become a world-wide hotspot in engineering. This article presents a comprehensive assessment of recent developments of multi-dimensional vibration mitigation devices and the material applied in such devices. The vibration mitigation materials mainly include the passive vibration mitigation materials and smart vibration mitigation materials. Subsequently, the vibration mitigation devices in civil engineering, mechanical engineering, and aerospace engineering are reviewed and commented, respectively. Above all, the multi-dimensional vibration mitigation devices and materials are found to be effective for reducing multi-dimensional vibrations. However, enhancing the mitigation performance of the devices in each direction, including improving the mitigation capability of materials, is still a challenging issue.
Introduction
Vibration is a cyclical reciprocating motion that exists widely in nature and engineering. When vibration exceeds a certain limit, it will cause harm to equipment, infrastructure, the environment, and the human body. Therefore, more scholars have begun to study the field of vibration isolation and mitigation, which is important for the safety, reliability, and durability of infrastructure and equipment. In this field, the vibration mitigation materials, and devices are two key factors that affect the performance of the vibration control effect.
Over the past few decades, significant contributions have been made by researchers around the world toward research on vibration mitigation materials. Many different kinds of materials are widely concerned in this field, from traditional passive vibration mitigation materials (viscoelastic materials, rubber, etc.) to smart materials (magnetorheological fluid, magnetorheological elastomers, piezoelectric ceramics, etc.). However, it is still significant to continually investigate and improve the properties of the vibration mitigation materials.
On the other hand, conventional mitigation/isolation technology of vibration usually focused on one dimension, which was far from enough. Consequently, multi-dimensional vibration isolation technology was put forward in the middle of the last century and gradually became a hotspot of research. The multi-dimensional vibration isolator was proposed by Stewart and named after himself (Stewart, 1965), and represents the beginning of research on the passive vibration isolators working in multi-dimensions. Afterwards, more optimized theories and precise models were proposed for multi-dimensional vibration isolation devices. For example, as for the description of viscoelastic dampers, the traditional models based on one dimension such as the Kelvin model, the Maxwell model, and the standard linear solid model were developed into the fractional-derivative equivalent standard solid model and the integrated mathematical model to meet the demands of multi-dimensional vibration isolation (Xu et al., 2014, 2017b). The application of these devices has been expanded in a variety of fields, such as civil engineering, mechanical engineering and aerospace engineering.
Vibration Mitigation Materials
Research on multi-dimensional vibration mitigation devices is committed to achieving and improving multi-directional mitigation capacity, which requires finding and developing the most suitable materials to apply to multi-dimensional vibration mitigation devices, so as to make great progress in vibration mitigation and isolation technology.
Passive Vibration Mitigation Materials
Viscous damping material usually refers to viscous fluids, which makes viscous dampers widely used in the field of vibration mitigation. The fluid damping medium commonly used in engineering is high viscosity organic material, such as hydraulic oil, silicone oil, special suspension, etc. Hydraulic oil is not suitable as a viscous medium or viscous damper due to its high freezing point and poor viscosity-temperature performance. Among the silicone oils, dimethyl silicone oil is the most suitable material for viscous medium due to its excellent high/low temperature resistance, chemical stability, shearing resistance, viscosity-temperature characteristics, and high compressibility. Many scholars have studied the viscosity, shear properties and chemical stability of methyl silicone oil. The special suspension mainly refers to magnetorheological fluid, which will be introduced later as a smart vibration mitigation material.
Viscoelastic (VE) damping material is composed of high polymer and has the characteristics of both viscous fluid and elastic solid. Many polymeric materials having long-chain molecules exhibit viscoelastic behavior, such as plastics, rubbers, acrylics, silicones, vinyls, adhesives, urethanes, epoxies, etc. Rubber is the most widely developed vibration mitigation material. In order to broaden the range of working temperature and frequency, many researchers have studied techniques such as blending (Lu et al., 2017), copolymerization (Pukkate et al., 2007; Suksawad et al., 2011; Yamazaki et al., 2011), and interpenetrating polymer networks (Manoj et al., 2002; Patri et al., 2007; Xu et al., 2016a) to improve the damping performance of VE materials. Liu et al. (2017) investigated the beneficial effect of in-situ polymerization blends on rubber damping properties, which proves that compared with mechanical blending, the damping temperature range is obviously expanded, meanwhile the peak and valley of the damping temperature range are greatly promoted. Qin et al. (2018) investigated gradient laminating to prepare NR/ENR composites, which proves that the layered structure can effectively improve the damping performance and the mechanical properties. Xu et al. (2016c) studied several kinds of VE materials based on different matrix rubbers, conducted experiments on VE dampers based on nitrile butadiene rubber (NBR) matrix and silicone rubber (SR) matrix. The results demonstrate that the NBR matrix VE dampers possess higher energy dissipation capacity, while the performance of the SR matrix VE dampers under various working conditions is more stable. Xu et al. (2016b) investigated the effects of the micromolecular structures and fillers on the VE properties of the VE materials and established an equivalent fractional order microstructure standard linear solid model.
Metallic materials are widely used in passive control, including alloys, metal matrix composites, and foam metals. The researchers studied the influence of heat treatment (Pulino-Sagradi et al., 1998; Murakami et al., 2006; González-Martínez et al., 2007), processing technology (Nishiyama et al., 2003), and the addition of reinforcing phase (Luo et al., 2004; Lihua et al., 2007) on the damping and mechanical properties of the alloy. Metal matrix composite material is a composite material made by adding reinforcing phase into the metal matrix, which has both good damping performance and mechanical properties. Jia (2007) investigated the damping performance of FeAl3 reinforced composites and base alloy over the temperature range of 30–300°C, which proves that the Al/FeAl3 composites exhibit higher damping capacity compared to Al matrix composites. Cell aluminum foams are widely used to absorb impact energy in the aero and automotive industries. Raj et al. (2009) investigated the plateau stress and energy absorption of closed-cell aluminum foam under quasi-static and dynamic compression, which shows that the plateau stress of aluminum foam improves with the increase of relative density and strain rate.
Smart Vibration Mitigation Materials
As a new type of damping material, smart materials are widely used in the field of vibration mitigation and isolation. Magnetorheological fluid (MRF) has reversible and instantaneous rheological properties, and the viscosity and yield stress change with the magnetic field intensity. The general MRF is composed of ferromagnetic particles dispersed in the carrier fluid. The density difference between particles and the carrier fluid leads to clear sedimentation, which reduces the adjustability and seismic performance of the MR device. Researchers investigated the influence of particle shape (Bell et al., 2008; De Vicente et al., 2009), size (Bondi, 1956), multi-diameter ratio (Foister, 1997; Guo et al., 2017), and material (Thomas, 1966) on the performance of magnetorheological fluids. In addition, many researchers have found that different from conventional silicon oil carrier fluids, ionic liquids (Guerrero-Sanchez et al., 2007), magnetofluids (Ginder et al., 1996), polymers (Iyengar et al., 2004) and other carrier fluids improve the properties of magnetorheological fluids. At present, it has become a hot topic to coat magnetic particles with submicron filler or polymer such as multi-walled carbon nanotubes (Guo et al., 2018), single-walled carbon nanotubes (Fang et al., 2009), graphene oxide (Xu et al., 2018b), gas-phase silica (Liu et al., 2013), etc., to improve the stability of MRF deposition.
Magnetorheological elastomers (MREs) are composed of magnetic particles dispersed in non-magnetic polymers. Compared with MRF, MREs have obvious advantages of preventing particle deposition, environmental pollution, and leakage. Stepanov et al. (2007) studied and compared the mechanical property of isotropic and anisotropic “soft” MREs in homogeneous magnetic fields of different intensities: elongation, static, and dynamic shears. Kamath et al. (1999) and Padalka et al. (2010) studied MREs composites with Fe and Co microparticles and nanowires of the same weight fraction, and the result was that the composites based on nanowire have much higher dynamic stiffness and equivalent damping coefficient values compared to the composites based on microparticles. Additionally, the properties of the aligned MRE composites filled with nanowires synthesized from typical ferromagnetic metals (Fe, Co, and Ni) were investigated. Zhu et al. (2012, 2013) and Xu et al. (2018a) investigated the magnetoviscoelasticity properties of the MREs in the condition of different magnetic fields, displacement amplitudes, and frequencies under sinusoidal loadings, then proposed a parameter model to describe MREs performances.
Shape memory alloys (SMAs) are widely used in the design of structural vibration mitigation and isolation devices due to the unique shape memory effect and superelasticity. Ferromagnetic shape memory alloys (FSMAs) as an attractive category of SMAs can change their dimensions based on the external magnetic field. Compared with traditional temperature-controlled SMAs, it has the advantages of fast response. Kainuma et al. (2006) reported the magnetic-field-induced shape recovery of a compressively deformed Ni-Co-Mn-In alloy. Stress of over 100 MPa exists in the material in a magnetic field of 70 kOe, and 3 percent deformation then almost completely recover the original shape of the alloy. Wang et al. (2008) firstly revealed that the application of a magnetic field at the martensitic transition temperature results in redistributions of local strains of martensitic variants, and investigated the effect of inhomogeneous microstructures on the magnetic-field-induced reverse transformation in the polycrystalline Ni-Co-Mn-In alloy by X-ray tomography.
Magnetostrictive materials have the property of strain with the change of magnetization state (Magnetostriction effect), thus realizing the mutual conversion of electromagnetic energy and mechanical energy. Giant magnetostrictive materials (GMM) represented by TbDyFe have become a research hotspot due to their large displacement, large output power, and fast response. Duenas and Carman (2001) studied the particle size and range of size distribution in magnetostrictive composites with low volume percentage and its influence on magnetostrictive properties. Since Guruswamy et al. (2000) found that Fe-Ga alloys have more advantages than Terfenol-D alloys, it has become a new type of promising magnetostrictive material. Many scholars have investigated the influence of the third elements (Jin et al., 2014; He et al., 2016; Meng and Jiang, 2016), heat treatment (Clark et al., 2000; Lograsso and Summers, 2006), preparation process (Yang et al., 2006; Li et al., 2008) and other factors on the magnetostrictive property of Fe-Ga alloys.
Piezoelectric ceramics produce stress/strain under the action of electric current and electric charge under stress/strain. The active control can be realized by adjusting the current level. Being environmentally friendly and lead-free, piezoelectric ceramics have gradually become one of the hotspots in the research of piezoelectric ceramics, and the main research objects are KNN, BNT, and BT ceramics. Liu and Ren (2009) reported a non-Pb piezoelectric ceramic system BaO3-TiO3 which shows a surprisingly high piezoelectric coefficient of d33~620 Pc/N at optimal composition. This is the first time in more than 50 years that the performance of lead-free piezoelectric materials surpasses PZT piezoelectric ceramics. Leontsev and Eitel (2009) investigated dielectric, ferroelectric, and piezoelectric properties of Mn-modified (1-x) BF-xBT ceramics, which proves that the addition of Mn improves dielectric losses and increases DC resistivity by one to five orders of magnitude in bulk BF–BT.
Multi-Dimensional Vibration Mitigation/Isolation Devices Applied in Civil Engineering
Mitigation and Isolation Devices With Springs
The passive control device composed of horizontal and vertical components is the most widely used multi-dimensional mitigation and isolation device which can independently achieve isolation performance in all directions. This kind of device has strong carrying capacity under normal use, and energy dissipation capacity is provided by the springs. On the other hand, since the springs and horizontal components are usually combined in the vertical direction, the height of the device is difficult to control and the stability is relatively low. The application of springs, including air springs and metal springs, is a common vertical isolation method. Suhara et al. (2002) tested the workability of three-dimensional seismic isolation using the laminated rubber bearing and the rolling seal type air spring working in series, which demonstrates that this device combines excellent 3D seismic isolation performance with sufficient vertical load carrying capacity. Two parameters were considered in the design of the experiment, which decided the different contact of the horizontal and vertical loads. Kageyama et al. (2003) studied the feasibility of applying an isolation device using three-dimensional cable reinforcing air spring to actual nuclear power plants, which shows that the air spring enables isolation in three dimensions with a single device, achieving a cost reduction. Zhao et al. (2008) studied the valid performance of a newly multi-dimensional isolation using one main and eight auxiliary disk springs in parallel to modify the conventional rubber bearing in the vertical direction, which shows that this device can isolate three-dimensional earthquake energy remarkably. Mori et al. (2012) verified a new method to achieve three-dimensional isolation for building by exploiting a device which consists of the rubber bearing, the air spring and oil damping, which proves that both the three-direction seismic response and the rocking motion are successfully suppressed by the device. Cai et al. (2016) analyzed the influence of a three-dimensional isolation bearing using the combination of horizontal, vertical spring and sliding surface, “which indicates that the isolated lattice shell structures have better performance with respect to the absolute acceleration response to the axial force of components. Miyagawa et al. (2017) proposed a new combination system achieving three-dimensional seismic isolation by combing coned disc springs with rubber bearings,” which mitigates vertical motion and suppresses rocking without any suppression system to provide a reasonable aseismic design for a sodium-cooled fast reactor. By combining the vertical spring with the horizontal isolation, it is possible to reduce the vertical stiffness of the device while providing a large bearing capacity, which can provide good multi-dimensional isolation performance. Furthermore, through the friction of the spring system, the seismic energy can be dissipated and the mitigation effect can be achieved.
Mitigation and Isolation Devices With High Damping Materials
Another efficient multi-dimensional mitigation and isolation method uses a high damping material such as viscous fluid and viscoelastic materials, etc. Devices with high damping materials have an excellent energy dissipation effect at the time of isolation. The structure of these devices is generally simple, therefore the stability is improved and the overturn of a superstructure under a large earthquake can be prevented effectively. Shimada et al. (2004) evaluated the performance of a three-dimensional earthquake isolation and mitigation system adopting a novel viscous damper wall, which shows that the damper wall can dissipate horizontal and vertical earthquake energy simultaneously by displacing it out of the plane direction. Xu et al. (2014) proposed an innovative multi-dimensional high-damping passive control device, named the multi-dimensional high damping earthquake isolation device (MHEID), for large-span reticulate structures, as shown in Figure 1. From the tests on the properties of the device, including the effect of excitation frequency and amplitude, “it is observed that the device has good capability of energy dissipation in the vertical direction and perpendicular paper direction” (Xu et al., 2012). The device has been applied on the large pipelines of Luoyang petrochemical corporation in Henan Province, China. A correction model based on the equivalent standard solid model is presented and used during the design, and excitation amplitude is taken into account.
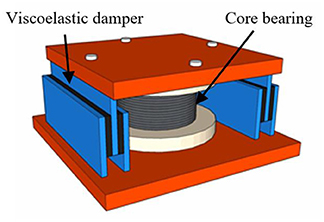
Figure 1. The structural diagram of the MHEID (Xu et al., 2014).
Xu et al. (2017b) studied the excellent performance of an innovative device using viscoelastic material named MEIMD for multi-dimensional earthquake isolation and mitigation, as shown in Figure 2. Based on the experimental results of property tests and shaking table tests, it proves that this device has good isolation ability and damping performance both horizontally and vertically (Xu, 2009). The device has been applied on Kunming planning museum in Yunnan province, China, and effectively reduces the displacement and vertical tensile stress of the isolated layer under rare earthquakes. This study provides a new avenue for building structures to achieve multi-dimensional vibration control by adopting the viscoelastic materials with high energy dissipation capacity.
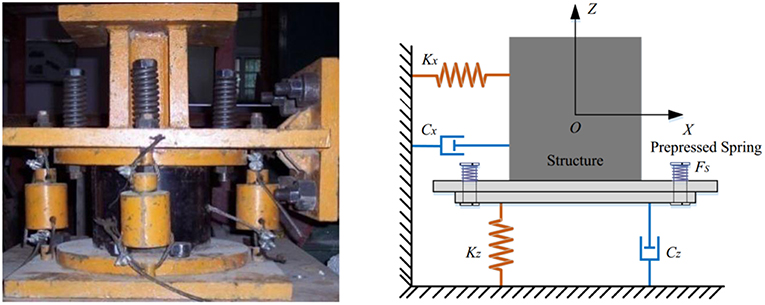
Figure 2. The structural diagram and the mechanics model of MEIMD (Xu et al., 2017b).
Due to the introduction of high energy-consuming materials, it not only exploits the advantages of the isolation bearing but also greatly increases the damping ratio of the whole system. Through the movement of the isolation layer and the additional damping device, it can mitigate a large amount of seismic energy to ensure safety and stability.
Other Mitigation and Isolation Devices
In addition to conceptual simplicity and moderate cost, good durability and temperature insensitivity are also basic requirements in the application of multi-dimensional vibration mitigation and devices in the field of civil engineering (Lu et al., 2018a). Shi and Huang (2013) verified the unique performance of an innovative three-dimensional seismic isolation, called periodic foundation, that was integrated with a high-density core, a soft coating and a concrete matrix, which shows that both horizontal and vertical seismic action can be greatly reduced after crossing the periodic foundation. He et al. (2014) verified the control effect of a multi-dimensional tuned mass damper named MDTMD, including tuned mass blocks, torsional blocks, and a rotation lever, which shows that the coupled responses decrease significantly by MDTMD and the vibration reduction ration is much higher compared to traditional TMD. Li et al. (2011) verified the effectiveness of a novel three-dimensional seismic isolation made up of martensitic shape memory alloy wires named 3-D SMAPRI, which proves that the 3-D isolator has effective mechanical properties as well as energy dissipation performance along both horizontal and vertical directions.
Hybrid Multi-Dimensional Mitigation and Isolation Device
The combination of various types of devices into a hybrid device is a novel multi-dimensional mitigation and isolation method with high control efficiency. One type of hybrid device is the passive hybrid multi-dimensional mitigation and isolation device. Ou and Jia (2010) validated the effectiveness of a three-dimensional isolation bearing consisting of a laminated rubber bearing and a combined coned disc spring with a vertical energy dissipation device, which shows that the 3D seismic isolation bearing is expected to be applied to the medium-to-short span bridges. Okamura et al. (2011) evaluated the effectiveness of an advanced seismic isolation system adopting thicker laminated rubber bearings and oil dampers, which confirms the possibility of isolating three-dimensional earthquake motion to protect nuclear power plants by increasing the thickness of the rubber layer of conventional rubber bearings. Yang and Niu (2012) studied the beneficial effect of a new three-dimensional isolation combining SMA strands and the disc spring with laminated rubber bearings, which indicates that the application of SMA causes clear seismic energy dissipation and reduces upper structure deformation in all directions. Jia et al. (2014) illustrated the advantages of a 3D isolation device composed of combined disk springs, rhombic steel plate damper and lead rubber bearing, which indicates that the isolator supplies appropriate vertical and horizontal stiffness and an energy dissipation mechanism to protect engineering structures in severe 3D earthquake motions. Kitayama et al. (2017) assessed the seismic performance of a three-dimensional isolator composed of a triple FP isolator in the horizontal direction and a spring-damper system in the vertical direction by incremental dynamic analysis, which is demonstrated to be a reliable way to seismically protect the electric power system. Liu et al. (2018a) analyzed the excellent property of a separation type three-dimensional isolation system with multiple guide rails to decouple horizontal and vertical motion, which indicates that the system not only displays desirable isolation but also contributes significantly to resist the overturning moment. Liu et al. (2018b) studied the property of a new multi-dimensional device using several inclined lead rubber bearings to decompose vertical seismic action, as shown in Figure 3. From the analysis of dynamic properties such as vertical acceleration, it is found that the seismic response is largely reduced compared with that of fixed-base structures. It is indicated that this device balances a high supporting capacity and low vertical stiffness to realize 3D isolation by optimizing the inclination angle of the lead rubber bearings.
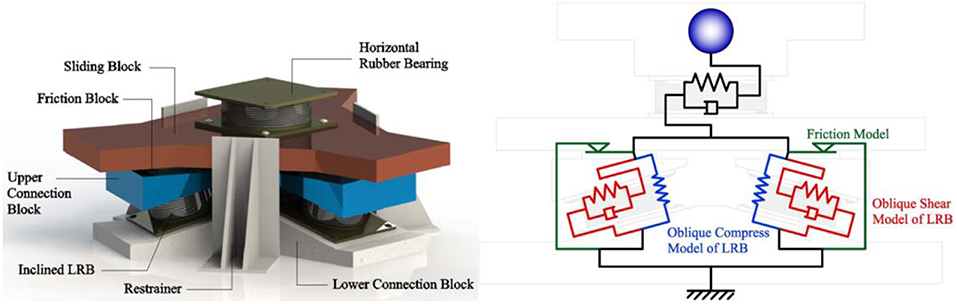
Figure 3. The 3D structural diagram and the mathematical model of the inclined lead rubber bearings (Liu et al., 2018b).
Cimellaro et al. (2018) investigated the performance of a novel three-dimensional base isolation system adopting an adoptive passive protection system called a negative stiffness device, as shown in Figure 4. From the numerical analyses of the isolated frame in strong earthquake, it can be observed that the vertical acceleration in the structure is apparently decreased. This study proposes a new method to reduce the multi-direction seismic responses of superstructures by way of combining the negative stiffness device with the conventional bearing systems.
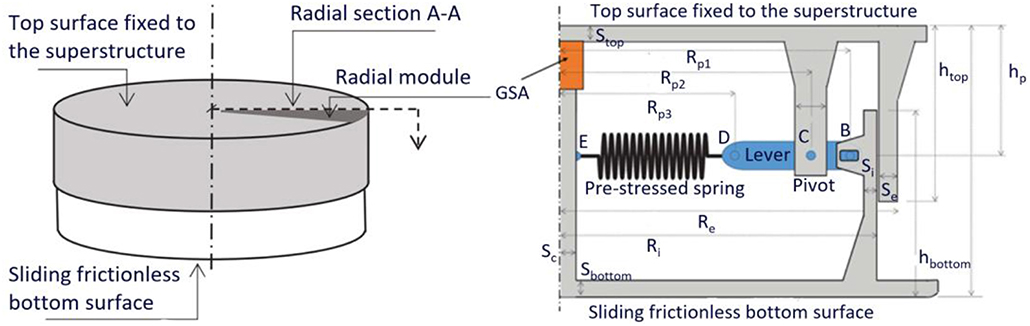
Figure 4. 3D view and detailed diagram of the Negative Stiffness Devices (Cimellaro et al., 2018).
Another type of hybrid device is the intelligent hybrid multi-dimensional mitigation and isolation device. Fujita et al. (2011) studied the basic property and performance of an intelligent three-dimensional isolation system encompassing air bearings and an earthquake early warning system, which indicates that this system is suitable for earthquakes in multiple directions and complex situations, even against near-field earthquakes. MR damper is also considered to be an effective intelligent control method. Xu et al. (2003) presented an on-line real-time control method for structures with MR dampers and proposed a novel design method for MR dampers (Xu et al., 2013), both of which promote the use of MR dampers in multi-dimensional mitigation and isolation devices. Cesmeci et al. (2018) demonstrated the superiority of a new device utilizing controllable MR damper and liquid springs in series with an elastomeric bearing for the three-dimensional isolation of building structures, as shown in Figure 5. From the experiments which reflect the device's real-life loading condition, it proves that the device operates well under the combined axial and shearing loading without any loss in performance. This study is the first time an MR damper has been tested in combination with horizontal and vertical seismic loads. Through semi-active control, the stability of the mitigation and isolation system during normal use can be ensured, while the horizontal and vertical performance can be fully exhibited under multi-dimensional earthquakes.
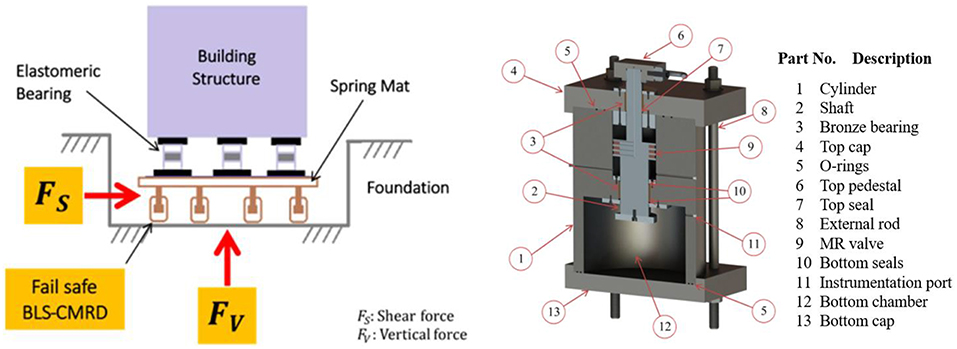
Figure 5. The installation of the liquid spring–magnetorheological damper in structure and its cutout view (Cesmeci et al., 2018).
The hybrid multi-dimensional mitigation and isolation device not only involves the advantages of each component, but also overcomes the deficiencies of the individual parts. Taking devices combining springs and high damping materials as an example, the springs arranged vertically isolate the earthquake efficiently, but the capability of energy dissipation is relatively weak. The addition of high damping materials enhances the energy dissipation performance during the earthquake; consequently, both the vibration isolation and vibration mitigation properties are realized in the device. Therefore, this has become the most mainstream research direction in the field of multi-dimensional mitigation and isolation device. On the other hand, these devices are currently in the theoretical research stage and are not yet applicable in practical engineering because of the immature technology.
Multi-Dimensional Vibration Mitigation/Isolation Devices Applied in Mechanical Engineering
Passive Control Devices
Passive control systems add an energy mitigation device or substructure system to the appropriate portion of the structure to optimize the dynamic characteristics of the structural system. Carrella et al. (2007) investigated the static characteristics of a quasi-zero-stiffness (QZS) mechanism consisting of a vertical spring and two oblique springs, which uses a negative stiffness component and realizes low stiffness without overlarge static deflection. Hoque et al. (2011) presented a module-type 3 degree of freedom (DOF) vibration isolation system using reformative zero-power control and parallel mechanism technique, whose reliability and efficiency has been verified by the analytical and experimental studies. Kim et al. (2013) presented an optimal design of a QZS isolator consisting of flexures, a vertical spring, a horizontal spring, and a vertical gravity compensator. The isolator has strong vertical stiffness to sustain a wide range of payloads because the properties of vertical springs lead to more gravity compensation within a given stroke. Sun and Jing (2015) presented an advanced vibration isolator with 3D QZS property, as shown in Figure 6. The isolator applies symmetrically scissor-like structures (SLS) in the horizontal directions and a conventional spring-mass-damper system in the vertical direction with positive stiffness. Due to the non-linear stiffness and damping properties produced by the non-linearity of geometric relations within the SLSs, the isolator has noticeable performance with structural non-linearity and only passive elements.
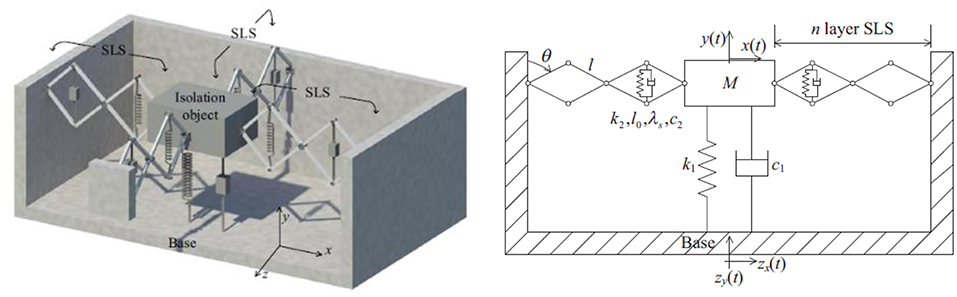
Figure 6. The structural diagram of the multi-direction isolation system (Sun and Jing, 2015).
Wu et al. (2016) studied on a novel 6-DOF passive vibration isolator which has been validated with experiments that the isolator has effective isolation behavior in all the six directions and takes the advantage of passive control on account of the combination of the Stewart platform and the X-shape structure. Zhou et al. (2017) extended the QZS vibration isolation method from one DOF to six DOFs by the method of applying a new-type QZS strut to establish a 6-DOF QZS vibration isolation platform, which possesses broader bandwidth and higher effectiveness compared with the linear counterpart. Ramesh et al. (2019) combined rigid body isolation and modal analysis principles to obtain the elastic and dissipative properties of the vibration isolator and analyzed several kinds of 3-DOF model isolation systems on the modal experiments and system characterization for validation purposes. Traditional linear energy dissipative methods and devices are greatly limited for wider application on account of the unsatisfactory properties under certain conditions, while non-linear vibration mitigation devices with broadband response and high robustness are being widely investigated and applied (Lu et al., 2018b). Non-linear stiffness is designed on the QZS vibration isolators, whose static stiffness is high while dynamic stiffness is low. The studies using passive control take advantage of non-linearity to enhance the performance of isolation, and the bandwidth of isolators has been broadened into both lower and higher frequencies.
Intelligent Control Devices
Different from passive control systems, intelligent control systems often require the input of external energy, while they have superior vibration control performance. Yoshioka et al. (2001) presented an active 6-DOF micro-vibration isolation system consisting of voice-coil linear motors and pneumatic and piezoelectric actuators with a feed-forward control link applied to the sway elements in each direction, which shows good vibration control performance in the vibration isolation table test. Liao and Wang (2003) investigated a semi-active secondary train suspension system with MR dampers which has been verified to be significantly effective in attenuating the vertical pitch and roll vibrations of the car body. Unsal et al. (2008) proposed a 6-DOF parallel platform model for which each leg is modeled as a 2-DOF system with a coil-over MR damper to adjust damping and an actuator for positioning, and it provides effective vibration isolation performance and precise positioning capability with reduced cost and reduced power requirements. Zareh et al. (2012) proposed an 11-DOF passenger car's suspension system which uses MR dampers with neuro-fuzzy (NF) control strategy as well as a novel intelligent semi-active control system. The inverse mapping model is proposed to estimate the force needed for a semi-active damper. Xu and Sun (2015) investigated the potential remarkable behavior of a multi-direction QZS vibration isolator using linear time-delayed active control, as shown in Figure 7. “From the research on the inherent property such as natural frequency, it can be observed that the performance of the isolator in different dimensions can be adjusted independently”. “The study provides an advanced insight into the design and analysis of multi-dimensional vibration isolation devices by utilizing potential non-linear superiority of the time delay in active vibration control”.
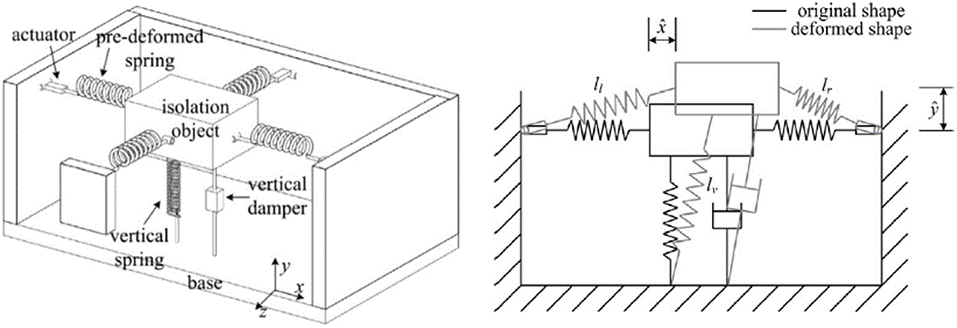
Figure 7. Structural diagram of the multi-direction QZS vibration isolator (Xu and Sun, 2015).
Zhou et al. (2015) demonstrated experimentally an ultra-low frequency active vibration isolation system which suppresses the vibration noise in three dimensions. The system is provided for atom gravimeters, especially for the gravimeter whose sensitivity is adversely affected by vibration couplings. The performance of multi-dimensional isolators using MR dampers is verified by the simulation and experiment and enhanced by a variety of control strategies (Xu et al., 2016d,f). In the field of mechanical engineering, the novel instruments put forward higher demands for precision and the rheological properties of the MR dampers meet the needs. For further research, it is imperative to establish a more accurate model of MR dampers to improve the control effect because of its highly non-linear nature.
Hybrid Control Devices
Aside from the passive control systems and intelligent control systems, hybrid systems combining active and passive devices are also one of the main development directions of multi-dimensional vibration isolation systems. Wang and Liu (2009) presented a hybrid control system comprising five air springs installed vertically in parallel and eight actuators installed in both vertically and horizontally, whose control performance against ground motion and direct disturbance is superior to that of the passive control system verified by the simulation. Hoque et al. (2010) presented a 6-DOF hybrid vibration isolation system composed of an active negative suspension, an active–passive positive suspension and a passive weight support mechanism, as shown in Figure 8. The system possesses outstanding behavior on direct disturbance suppression and payload supporting capabilities without diminishing transmissibility characteristics. Additionally, the influence of on-board generated direct disturbances of the six-axis motions in vertical and horizontal directions and ground vibrations could be isolated effectively. The mathematical model of the system has been developed and it is indicated that zero compliance to direct disturbance is provided by the system.
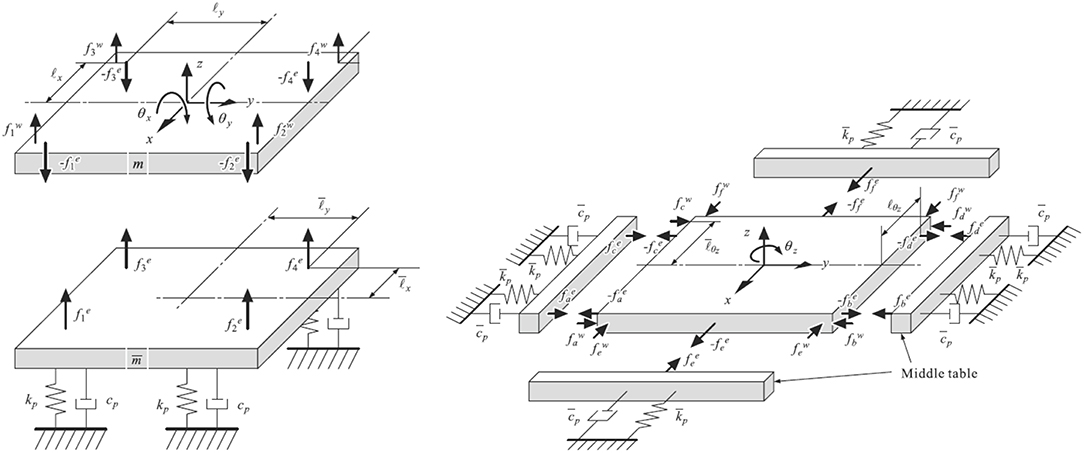
Figure 8. The structural diagram of the solation table for six-axis motions (Hoque et al., 2010).
Li et al. (2012) proposed a novel vibration isolator possessing three translational DOFs and two rotational DOFs, which widens the range of frequency by decoupling the translations and rotations of the manipulator. The systems reviewed above achieve a superior performance to passive control systems within a wide frequency range of vibrations. The dynamic characteristics of the systems are related to the control parameters as well as the physical parameters, hence, the optimal design of control and physical parameters is imperative to improve the dynamic performance of the systems.
Multi-Dimensional Vibration Mitigation/Isolation Devices Applied in Aerospace Engineering
Vibration Mitigation/Isolation of Whole-Spacecraft
Vibration control in aerospace engineering falls into two main categories—whole-spacecraft vibration isolation (WSVI) and micro-vibration control of satellites. WSVI is for the reduction of the vibration loads during launch, and it greatly reduces the risk of the spacecraft and its instruments being damaged before entering orbit. Jun et al. (2007) presented a whole-spacecraft passive isolation system with a demonstration unit integrated with all kinds of isolator components. The payload attach fitting is limited by the constrained viscoelastic layer on the outer surface, thus its damping is enhanced obviously. Li et al. (2016) designed and tested an active vibration isolator, which includes flexible piezoelectric sensors and actuators. The thin shell hypothesis and the converse piezoelectric effect are considered to obtain the modal control force during the design of the isolator.
Tang et al. (2018) designed, fabricated, and tested a novel active whole-spacecraft isolation system based on voice coil motors (VCMs), as shown in Figure 9. Vibration tests were conducted on the developed prototype for the verification purpose, and the experiment results indicate that the new system attenuates the resonant peak remarkably well. The study provides advanced insight into the analysis and design of whole-spacecraft isolator in active vibration control.
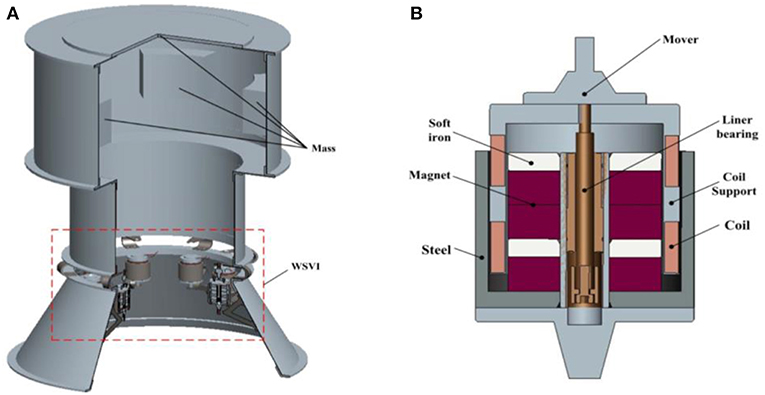
Figure 9. (A) WSVI system (B) Structure of the electromagnetic actuator (Tang et al., 2018).
ESA developed an active payload adaptor to take the place of the traditional passive payload adaptors whose framework is a truss structure with 24 active struts in the load path applying piezo-ceramics as actuator (Rittweger et al., 2005). The adaptor is designed to reduce the vibration below 100 Hz to a quarter for Ariane 5, and the result is obtained in the frame of the ESA GSP program. Liu et al. (2006) proposed an octo-strut passive vibration isolation platform for whole-spacecraft vibration isolation which introduces redundancy. Liu et al. (2010) presented a novel Circular Payload Adapter Fitting (CPAF) which integrates passive and active vibration control with the piezoelectric stack actuators based on passive control. Based on the CPAF, Chi et al. (2014) designed an active vibration isolation method applying the voice coil based actuator whose voice coil motor is designed and optimized as the active control actuator. The passive isolator is simpler and more convenient than the new adapter, but the new adapter has better vibration isolation performance. The active isolator proposed by Tang is in between. Both whole-space vibration isolator and new payload adaptors have great vibration isolation performance and as a result, more sensitive equipment is able to be launched into space.
Micro-Vibration Mitigation/Isolation of Satellite
The micro-vibration of satellites in orbit is an important factor that restricts the resolution of the payload. Therefore, it is necessary to control the micro-vibration of satellites in orbit. The James Webb Space Telescope employed dual-stage passive isolation (Bronowicki, 2006). The first stage of isolation is at the disturbance source in which individual reaction wheels use the flight-proven Chandra isolator while the second stage of isolation is a full isolator positioned between the top of the spacecraft bus and the pop-up thermal isolation tower which orientates the telescope. More than one kind of damping material are added, thus, the analysis methodology is extended to fit the multiple structural partitions. Kamesh et al. (2010) proposed a passive and active space frame platform integrated with four folded continuous beams arranged in three directions.
The Air Force Research Laboratory (AFRL) Space Vehicle Directorate and Honeywell Defense and Space designed MVIS-II for miniature hybrid (passive/active) vibration isolation of sensitive optical payloads (McMickell et al., 2007), as shown in Figure 10. Results of the experiment based on ground lead to the expectation of better on-orbit isolation properties, and the study provides a useful insight into the design of payload isolators in vibration hybrid control.
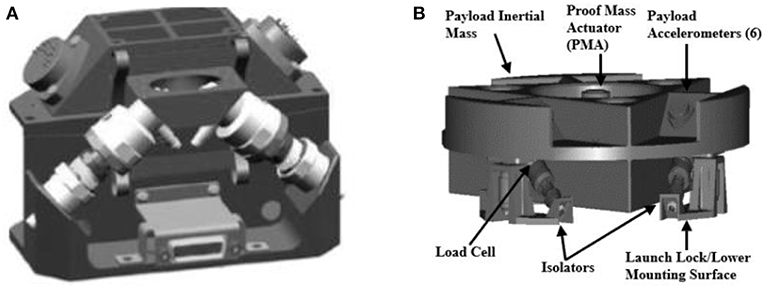
Figure 10. (A) Two hybrid MVIS-II Isolators (B) MVIS-II original flight demonstration with integral launch lock (McMickell et al., 2007).
Dai et al. (2018) proposed a bio-inspired quadrilateral shape (BIQS) structure inspired by the smooth movement of a running kangaroo to suppress the vibrations of a free-floating spacecraft suffered from periodic or impulsive forces, as shown in Figure 11. Numerical simulation shows that the BIQS system has superior behavior under conditions of either periodic or impulsive forces. The study provides a highly efficient passive way for vibration suppression in free-floating spacecraft.
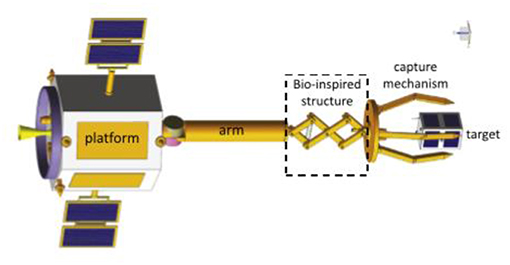
Figure 11. The schematic diagram of an on-orbit capture mission (Dai et al., 2018).
Compared to the vibration isolation method of the James Webb Space Telescope, the integrated attitude control and vibration isolation of MVIS-II is more convenient but more expensive. Dai's design includes the idea of simulation, which is a promising research direction. In addition, hybrid control is also worth studying. For example, Xu et al. (2016e) presented a platform combining MR and VE devices, which is able to effectively isolate 0–500 Hz vibrations. Above all, with the development of high-resolution satellites, the research of micro-vibration control methods will be further deepened.
Conclusions and Prospects
Using materials of high performance, including wide temperature domain and wide frequency domain, is supposed to be an efficient way to reduce the vibration, even for high-intensity vibrations. Meanwhile, there still exists promising prospects for the coupling application of various materials and promoting them to multi-dimensional vibration mitigation and isolation devices. The research will continuously focus on optimizing the performance of existing materials and establishing accurate constitutive models for describing the behavior of materials and in the future.
In the field of civil engineering, research started late on multi-dimensional mitigation and isolation devices. Most of the traditional research focuses on a single direction or a single function, while little research is concerned about mitigation and isolation in multiple directions or multiple functions. For example, most of the current isolation systems just focus on horizontal or vertical isolation, while the shocking effect after the combination of horizontal and vertical motion and the uplifting action caused by the swing of superstructure are less involved. Additionally, the existing multi-dimensional mitigation and isolation devices commonly adopt different combinations of springs and a variety of dampers. Few devices utilize high damping material or smart mitigation material. Therefore, research should accelerate when it comes to the development of hybrid multi-dimensional mitigation and isolation devices and the application of high damping and smart mitigation materials.
In the field of mechanical engineering, precision for instruments and instantaneity of mitigation are in great demand. Thus, intelligent control under different directions is a promising approach for mitigating multi-dimensional vibration responses. As for semi-active vibration control devices, the accuracy of the parametric dynamic modeling is of great importance due to the inherent non-linearity. Although the research on active control devices is still a small proportion of multi-dimensional isolators, it will be promoted in further research. Furthermore, the optimal design for intelligent control devices needs to be investigated and more work should look at the problems of control algorithms.
In the field of aerospace engineering, multi-dimensional vibration control is an important means to ensure the safety of satellites and their equipment in the launch stage, while improving the accuracy of satellite payloads in orbit. Meanwhile, the vibrations during launch are generated by a variety of flight events such as engine ignition, booster separation, and acoustic excitation, and the frequency domains of each excitation are different. Thus, the vibration mitigation and isolation of wide frequency domains in multiple dimensions will be the future development priority. For whole-spacecraft vibration isolation, passive control devices have been widely used, but their performance in vibration isolation is inferior to active control devices, which are more complex and expensive. Therefore, research on semi-active control devices deserves more attention because of relatively excellent performance and simple structure. For micro-vibration control of satellites in orbit, it has great prospects to combine attitude control and the isolation of micro-vibrations generated by the attitude control unit. Additionally, current research focuses on internal vibration sources such as the attitude control unit, while vibration caused by external sources deserves more attention.
At present, research on multi-dimensional mitigation and isolation devices is relatively scarce. Moreover, research on most devices is still in the theoretical and experimental stages, and there are few examples of using these multi-dimensional isolation devices in practical engineering. The authors aim to devote themselves to researching the wide temperature domain and wide frequency domain of vibration mitigation materials, meanwhile the application of the materials and devices is also an important research direction. However, there is still a long way to go to expand different multi-dimensional mitigation and isolation devices and promote applications in practical engineering.
Author Contributions
Z-DX identified the framework of the article and wrote the main content of the article. All other authors are responsible for the organization of literature and pictures.
Funding
This study was financially supported by the Program of Chang Jiang Scholars of Ministry of Education, National Science Fund for Distinguished Young Scholars with Grant No. 51625803, National Key R&D Programs of China with Grant Nos. 2016YFE0200500 and 2016YFE0119700, National Natural Science Foundation of China with Grant No. 11572088.
Conflict of Interest Statement
The authors declare that the research was conducted in the absence of any commercial or financial relationships that could be construed as a potential conflict of interest.
References
Bell, R. C., Karli, J. O., Vavreck, A. N., Zimmerman, D. T., Ngatu, G. T., and Wereley, N. M. (2008). Magnetorheology of submicron diameter iron microwires dispersed in silicone oil. Smart Mater. Struct. 17:015028. doi: 10.1088/0964-1726/17/01/015028
Bondi, A. A. (1956). Magnetic Fluids. U.S. Patent No. 2,751,352. Washington, DC: U.S. Patent and Trademark Office.
Bronowicki, A. J. (2006). Vibration isolator for large space telescopes. J Spacecraft Rockets 43, 45–53. doi: 10.2514/1.12036
Cai, Y., Li, X., and Xue, S. (2016). Application and design of 3D seismic isolation bearing in lattice shell structure. HKIE Transac. 23, 200–213. doi: 10.1080/1023697X.2016.1231016
Carrella, A., Brennan, M. J., and Waters, T. P. (2007). Static analysis of a passive vibration isolator with quasi-zero stiffness characteristic. J. Sound Vibration 301, 678–689. doi: 10.1016/j.jsv.2006.10.011
Cesmeci, S., Gordaninejad, F., Ryan, K. L., and Eltahawy, W. (2018). A liquid spring–magnetorheological damper system under combined axial and shear loading for three-dimensional seismic isolation of structures. J. Intell. Mater. Syst. Struct. 29, 3517–3532. doi: 10.1177/1045389X18783090
Chi, W., Cao, D., and Huang, W. (2014). “Design of active whole-spacecraft vibration isolation based on voice-coil motor,” in Sensors and Smart Structures Technologies for Civil, Mechanical, and Aerospace Systems, Vol. 9061. 90613X-1–90613X-7. doi: 10.1117/12.2044920
Cimellaro, G. P., Domaneschi, M., and Warn, G. (2018). Three-dimensional base isolation using vertical negative stiffness devices. J. Earthquake Eng. 1–29. doi: 10.1080/13632469.2018.1493004. [Epub ahead of print].
Clark, A. E., Restorff, J. B., Wun-Fogle, M., Lograsso, T. A., and Schlagel, D. L. (2000). Magnetostrictive properties of body-centered cubic Fe-Ga and Fe-Ga-Al alloys. IEEE Transac. Magnetics 36, 3238–3240. doi: 10.1109/20.908752
Dai, H., Jing, X., Wang, Y., Yue, X., and Yuan, J. (2018). Post-capture vibration suppression of spacecraft via a bio-inspired isolation system. Mech. Syst. Signal Proc. 105, 214–240. doi: 10.1016/j.ymssp.2017.12.015
De Vicente, J., Segovia-Gutiérrez, J. P., Andablo-Reyes, E., Vereda, F., and Hidalgo-Álvarez, R. (2009). Dynamic rheology of sphere-and rod-based magnetorheological fluids. J. Chem. Phys. 131:194902. doi: 10.1063/1.3259358
Duenas, T. A., and Carman, G. P. (2001). Particle distribution study for low-volume fraction magnetostrictive composites. J. Appl. Phys. 90, 2433–2439. doi: 10.1063/1.1389518
Fang, F. F., Choi, H. J., and Jhon, M. S. (2009). Magnetorheology of soft magnetic carbonyl iron suspension with single-walled carbon nanotube additive and its yield stress scaling function. Colloids Surfaces A Physicochem. Eng. Aspects 351, 46–51. doi: 10.1016/j.colsurfa.2009.09.032
Foister, R. T. (1997). U. S. Magneto-Rheological Fluid. Patent No. 5,667,715. Washington, DC: U.S. Patent and Trademark Office.
Fujita, S., Minagawa, K., Tanaka, G., and Shimosaka, H. (2011). Intelligent seismic isolation system using air bearings and earthquake early warning. Soil Dynam. Earthquake Eng. 31, 223–230. doi: 10.1016/j.soildyn.2010.06.006
Ginder, J. M., Davis, L. C., and Elie, L. D. (1996). Rheology of magnetorheological fluids: models and measurements. Int. J. Modern Phys. B 10, 3293–3303. doi: 10.1142/S0217979296001744
González-Martínez, R., Göken, J., Letzig, D., Steinhoff, K., and Kainer, K. U. (2007). Influence of aging on damping of the magnesium–aluminium–zinc series. J. Alloys Compounds 437, 127–132. doi: 10.1016/j.jallcom.2006.07.085
Guerrero-Sanchez, C., Lara-Ceniceros, T., Jimenez-Regalado, E., Raşa, M., and Schubert, U. S. (2007). Magnetorheological fluids based on ionic liquids. Adv. Mater. 19, 1740–1747. doi: 10.1002/adma.200700302
Guo, Y. Q., Sun, C. L., and Xu, Z. D. (2018). Preparation and tests of MR fluids with CI particles coated with MWNTs. Front. Mater. 5:50. doi: 10.3389/fmats.2018.00050
Guo, Y. Q., Xu, Z. D., Chen, B. B., Ran, C. S., and Guo, W. Y. (2017). Preparation and experimental study of magnetorheological fluids for vibration control. Int. J. Acoust. Vibration 22, 194–201. doi: 10.20855/ijav.2017.22.2464
Guruswamy, S., Srisukhumbowornchai, N., Clark, A. E., Restorff, J. B., and Wun-Fogle, M. (2000). Strong, ductile, and low-field-magnetostrictive alloys based on Fe-Ga. Scripta Mater. 43, 239–244. doi: 10.1016/S1359-6462(00)00397-3
He, H. X., Han, E. Z., and Lv, Y. W. (2014). Coupled vibration control of tuned mass damper in both horizontal and torsional direction. Appl. Mech. Mater. 578, 1000–1006. doi: 10.4028/www.scientific.net/AMM.578-579.1000
He, Y., Jiang, C., Wu, W., Wang, B., Duan, H., Wang, H., et al. (2016). Giant heterogeneous magnetostriction in Fe–Ga alloys: effect of trace element doping. Acta Mater. 109, 177–186. doi: 10.1016/j.actamat.2016.02.056
Hoque, M. E., Mizuno, T., Ishino, Y., and Takasaki, M. (2010). A six-axis hybrid vibration isolation system using active zero-power control supported by passive weight support mechanism. J. Sound Vibration 329, 3417–3430. doi: 10.1016/j.jsv.2010.03.003
Hoque, M. E., Mizuno, T., Ishino, Y., and Takasaki, M. (2011). A three-axis vibration isolation system using modified zero-power controller with parallel mechanism technique. Mechatronics 21, 1055–1062. doi: 10.1016/j.mechatronics.2011.05.002
Iyengar, V. R., Alexandridis, A. A., Tung, S. C., and Rule, D. S. (2004). Wear testing of seals in magneto-rheological fluids. Tribol. Transac. 47, 23–28. doi: 10.1080/05698190490279083
Jia, C. (2007). Study on damping behavior of FeAl3 reinforced commercial purity aluminum. Mater. Design 28, 1711–1713. doi: 10.1061/9780784413234.057
Jia, J. F., Dong, H. H., Zhang, T., and Du, X. L. (2014). “Mechanics performance test and seismic behavior of a novel 3D isolation bearing for bridges,” in International Efforts in Lifeline Earthquake Engineering (Chengdu), 441–448.
Jin, T., Wu, W., and Jiang, C. (2014). Improved magnetostriction of Dy-doped Fe83Ga17 melt-spun ribbons. Scripta Mater. 74, 100–103. doi: 10.1016/j.scriptamat.2013.11.010
Jun, Z., Hongxing, H., and Zhiyi, Z. (2007). An evaluation of the whole-spacecraft passive vibration isolation system. Proc. Inst. Mech. Engineers Part G J. Aerospace Eng. 221, 67–72. doi: 10.1243/09544100JAERO81
Kageyama, M., Iba, T., Umeki, K., Somaki, T., and Moro, S. (2003). “Development of three-dimensional base isolation system with cable reinforcing air spring,” in Proceedings of the 17th International Conference on Structural Mechanics in Reactor Technology (Prague: SMiRT 17).
Kainuma, R., Imano, Y., Ito, W., Sutou, Y., Morito, H., Okamoto, S., et al. (2006). Magnetic-field-induced shape recovery by reverse phase transformation. Nature 439:957. doi: 10.1038/nature04493
Kamath, G. M., Wereley, N. M., and Jolly, M. R. (1999). Characterization of magnetorheological helicopter lag dampers. J. Am. Helicopter Soc. 44, 234–248. doi: 10.4050/JAHS.44.234
Kamesh, D., Pandiyan, R., and Ghosal, A. (2010). Modeling, design and analysis of low frequency platform for attenuating micro-vibration in spacecraft. J. Sound Vibration 329, 3431–3450. doi: 10.1016/j.jsv.2010.03.008
Kim, K. R., You, Y. H., and Ahn, H. J. (2013). Optimal design of a QZS isolator using flexures for a wide range of payload. Int. J. Precision Eng. Manufacturing 14, 911–917. doi: 10.1007/s12541-013-0120-0
Kitayama, S., Lee, D., Constantinou, M. C., and Kempner, L. Jr. (2017). Probabilistic seismic assessment of seismically isolated electrical transformers considering vertical isolation and vertical ground motion. Eng. Struct. 152, 888–900. doi: 10.1016/j.engstruct.2017.10.009
Leontsev, S. O., and Eitel, R. E. (2009). Dielectric and piezoelectric properties in Mn-modified (1– x) BiFeO3–BaTiO3 ceramics. J. Am. Ceramic Soc. 92, 2957–2961. doi: 10.1111/j.1551-2916.2009.03313.x
Li, B., Zhao, W., and Deng, Z. (2012). Modeling and analysis of a multi-dimensional vibration isolator based on the parallel mechanism. J. Manufacturing Syst. 31, 50–58. doi: 10.1016/j.jmsy.2010.12.001
Li, H., Li, H. Y., Chen, Z. B., and Tzou, H. S. (2016). Experiments on active precision isolation with a smart conical adapter. J. Sound Vibration 374, 17–28. doi: 10.1016/j.jsv.2016.03.039
Li, J. H., Gao, X. X., Zhu, J., Zhang, M. C., and He, C. X. (2008). Texture and magnetostriction in rolled Fe-Ga alloy. Acta Metallurg. Sinica 44, 1031–1034.
Li, S., Mao, C., Li, H., and Zhao, Y. (2011). Mechanical properties and theoretical modeling of self-centering shape memory alloy pseudo-rubber. Smart Mater. Struct. 20:115008. doi: 10.1088/0964-1726/20/11/115008
Liao, W. H., and Wang, D. H. (2003). Semiactive vibration control of train suspension systems via magnetorheological dampers. J. Intell. Mater. Syst. Struct. 14, 161–172. doi: 10.1177/1045389X03014003004
Lihua, L., Xiuqin, Z., Xianfeng, L., Haowei, W., and Naiheng, M. (2007). Effect of silicon on damping capacities of pure magnesium and magnesium alloys. Mater. Lett. 61, 231–234. doi: 10.1016/j.matlet.2006.04.038
Liu, F., Fang, B., and Huang, W. H. (2010). “Vibration control of flexible satellites using a new isolator,” in 2010 3rd International Symposium on Systems and Control in Aeronautics and Astronautics Harbin: IEEE, 593–597.
Liu, K., Lv, Q., and Hua, J. (2017). Study on damping properties of HVBR/EVM blends prepared by in situ polymerization. Polymer Test. 60, 321–325. doi: 10.1016/j.polymertesting.2017.02.026
Liu, L. K., Zheng, G. T., and Huang, W. H. (2006). Octo-strut vibration isolation platform and its application to whole spacecraft vibration isolation. J. Sound Vibration 289, 726–744. doi: 10.1016/j.jsv.2005.02.040
Liu, W., and Ren, X. (2009). Large piezoelectric effect in Pb-free ceramics. Phys. Rev. Lett. 103:257602. doi: 10.1103/PhysRevLett.103.257602
Liu, W., Tian, K., Wei, L., He, W., and Yang, Q. (2018a). Earthquake response and isolation effect analysis for separation type three-dimensional isolated structure. Bull. Earthquake Eng. 16, 6335–6364. doi: 10.1007/s10518-018-0417-6
Liu, W., Xu, H., He, W., and Yang, Q. (2018b). Static test and seismic dynamic response of an innovative 3D seismic isolation system. J. Struct. Eng. ASCE 144:04018212. doi: 10.1061/(ASCE)ST.1943-541X.0002195
Liu, Y. D., Lee, J., Choi, S. B., and Choi, H. J. (2013). Silica-coated carbonyl iron microsphere based magnetorheological fluid and its damping force characteristics. Smart Mater. Struct. 22:065022. doi: 10.1088/0964-1726/22/6/065022
Lograsso, T. A., and Summers, E. M. (2006). Detection and quantification of D03 chemical order in Fe–Ga alloys using high resolution X-ray diffraction. Mater. Sci. Eng. A 416, 240–245. doi: 10.1016/j.msea.2005.10.035
Lu, X., Huang, R., Li, H., Long, J., and Jiang, Z. (2017). Preparation of an elastomer with broad damping temperature range based on EPDM/ENR blend. J. Elastomers Plastics 49, 758–773. doi: 10.1177/0095244317698737
Lu, Z., Wang, Z., Masri, S. F., and Lu, X. (2018a). Particle impact dampers: past, present, and future. Struct. Control Health Monitor. 25:e2058. doi: 10.1002/stc.2058
Lu, Z., Wang, Z., Zhou, Y., and Lu, X. (2018b). Nonlinear dissipative devices in structural vibration control: a review. J. Sound Vibration 423, 18–49. doi: 10.1016/j.jsv.2018.02.052
Luo, B. H., Bai, Z. H., and Xie, Y. Q. (2004). The effects of trace Sc and Zr on microstructure and internal friction of Zn–Al eutectoid alloy. Mater. Sci. Eng. A 370, 172–176. doi: 10.1016/j.msea.2002.12.007
Manoj, N. R., Chandrasekhar, L., Patri, M., Chakraborty, B. C., and Deb, P. C. (2002). Vibration damping materials based on interpenetrating polymer networks of carboxylated nitrile rubber and poly (methyl methacrylate). Polymers Adv. Technol. 13, 644–648. doi: 10.1002/pat.324
McMickell, M. B., Kreider, T., Hansen, E., Davis, T., and Gonzalez, M. (2007). “Optical payload isolation using the miniature vibration isolation system (MVIS-II),” in Industrial and Commercial Applications of Smart Structures Technologies 2007, eds L. P. Davis, B. K. Henderson, and M. B. McMickell (San Diego, CA: International Society for Optics and Photonics), 652703.
Meng, C., and Jiang, C. (2016). Magnetostriction of a Fe83Ga17 single crystal slightly doped with Tb. Scrip. Mater. 114, 9–12. doi: 10.1016/j.scriptamat.2015.11.022
Miyagawa, T., Watakabe, T., Yamamoto, T., Fukasawa, T., and Okamura, S. (2017). “Research and development of three dimensional seismic isolation system utilized coned-disc-springs with rubber bearings,” in ASME 2017 Pressure Vessels and Piping Conference (Waikoloa: American Society of Mechanical Engineers), V008T08A043.
Mori, S., Suhara, I., Saruta, M., Okada, K., Tomizawa, T., Tsuyuki, Y., et al. (2012). “Simulation analysis of free vibration test in a building “Chisuikan” using three-dimensional seismic base isolation system,” in 15th World Conference on Earthquake Engineering (Lisbon).
Murakami, T., Inoue, T., Shimura, H., Nakano, M., and Sasaki, S. (2006). Damping and tribological properties of Fe–Si–C cast iron prepared using various heat treatments. Mater. Sci. Eng. A 432, 113–119. doi: 10.1016/j.msea.2006.06.090
Nishiyama, K., Matsui, R., Ikeda, Y., Niwa, S., and Sakaguchi, T. (2003). Damping properties of a sintered Mg–Cu–Mn alloy. J. Alloys Comp. 355, 22–25. doi: 10.1016/S0925-8388(03)00256-1
Okamura, S., Kamishima, Y., Negishi, K., Sakamoto, Y., Kitamura, S., and Kotake, S. (2011). Seismic isolation design for JSFR. J. Nucl. Sci. Technol. 48, 688–692. doi: 10.1080/18811248.2011.9711750
Ou, J. P., and Jia, J. F. (2010). “Seismic performance of a novel 3D isolation system on continuous bridges,” in Active and Passive Smart Structures and Integrated Systems 2010, 7643 (San Diego, CA: International Society for Optics and Photonics), 764304. doi: 10.1117/12.847506
Padalka, O., Song, H. J., Wereley, N. M., Filer, I. I. J. A, and Bell, R. C. (2010). Stiffness and damping in Fe, Co, and Ni nanowire-based magnetorheological elastomeric composites. IEEE Trans. Magn. 46, 2275–2277. doi: 10.1109/TMAG.2010.2044759
Patri, M., Reddy, C. V., Narasimhan, C., and Samui, A. B. (2007). Sequential interpenetrating polymer network based on styrene butadiene rubber and polyalkyl methacrylates. J. Appl. Polymer Sci. 103, 1120–1126. doi: 10.1002/app.24338
Pukkate, N., Kitai, T., Yamamoto, Y., Kawazura, T., Sakdapipanich, J., and Kawahara, S. (2007). Nano-matrix structure formed by graft-copolymerization of styrene onto natural rubber. Eur. Polymer J. 43, 3208–3214. doi: 10.1016/j.eurpolymj.2007.04.037
Pulino-Sagradi, D., Sagradi, M., Karimi, A., and Martin, J. L. (1998). Damping capacity of Fe-Cr-X high-damping alloys and its dependence on magnetic domain structure. Scrip. Mater. 39. doi: 10.1016/S1359-6462(98)00157-2
Qin, R., Huang, R., and Lu, X. (2018). Use of gradient laminating to prepare NR/ENR composites with excellent damping performance. Mater. Design 149, 43–50. doi: 10.1016/j.matdes.2018.03.063
Raj, R. E., Parameswaran, V., and Daniel, B. S. S. (2009). Comparison of quasi-static and dynamic compression behavior of closed-cell aluminum foam. Mater. Sci. Eng. A 526, 11–15. doi: 10.1016/j.msea.2009.07.017
Ramesh, R. S., Fredette, L., and Singh, R. (2019). Identification of multi-dimensional elastic and dissipative properties of elastomeric vibration isolators. Mech. Syst. Signal Proc. 118, 696–715. doi: 10.1016/j.ymssp.2018.09.009
Rittweger, A., Beig, H. G., Konstanzer, P., and Bureo Dacal, R. (2005). Feasibility demonstration of an active payload adapter for Ariane 5. In Spacecraft Structures, Materials and Mechanical Testing 2005, 581.
Shi, Z., and Huang, J. (2013). Feasibility of reducing three-dimensional wave energy by introducing periodic foundations. Soil Dynam. Earthquake Eng. 50, 204–212. doi: 10.1016/j.soildyn.2013.03.009
Shimada, T., Fujiwaka, T., and Moro, S. (2004). “Study on three-dimensional seismic isolation system for next-generation nuclear power plant: hydraulic three-dimensional base isolation system,” in Proceedings of Pressure Vessels and Piping Conference (San Diego, CA: ASME) 486, 35–42. doi: 10.1115/PVP2004-2931
Stepanov, G. V., Abramchuk, S. S., Grishin, D. A., Nikitin, L. V., Kramarenko, E. Y., and Khokhlov, A. R. (2007). Effect of a homogeneous magnetic field on the viscoelastic behavior of magnetic elastomers. Polymer 48, 488–495. doi: 10.1016/j.polymer.2006.11.044
Suhara, J., Tamura, T., Okada, Y., and Umeki, K. (2002). “Development of three dimensional seismic isolation device with laminated rubber bearing and rolling seal type air spring,” in ASME 2002 Pressure Vessels and Piping Conference (Vancouver, BC: American Society of Mechanical Engineers), 43–48. doi: 10.1115/PVP2002-1430
Suksawad, P., Yamamoto, Y., and Kawahara, S. (2011). Preparation of thermoplastic elastomer from natural rubber grafted with polystyrene. Eur. Polymer J. 47, 330–337. doi: 10.1016/j.eurpolymj.2010.11.018
Sun, X., and Jing, X. (2015). Multi-direction vibration isolation with quasi-zero stiffness by employing geometrical nonlinearity. Mech. Syst. Signal Proc. 62, 149–163. doi: 10.1016/j.ymssp.2015.01.026
Tang, J., Cao, D., Ren, F., and Li, H. (2018). Design and experimental study of a VCM-based whole-spacecraft vibration isolation system. J. Aerospace Eng. ASCE 31:04018045. doi: 10.1061/(ASCE)AS.1943-5525.0000871
Thomas, J. R. (1966). Preparation and magnetic properties of colloidal cobalt particles. J. Appl. Phys. 37, 2914–2915. doi: 10.1063/1.1782154
Unsal, M., Niezrecki, C., and Crane, I. I. I. C. D. (2008). Multi-axis semi-active vibration control using magnetorheological technology. J. Intel. Mater. Syst. Struct. 19, 1463–1470. doi: 10.1177/1045389X07088287
Wang, Y., and Liu, H. J. (2009). Six degree-of-freedom microvibration hybrid control system for high technology facilities. Int. J. Struct. Stab. Dynam. 9, 437–460. doi: 10.1142/S0219455409003168
Wang, Y. D., Huang, E. W., Ren, Y., Nie, Z. H., Wang, G., Liu, Y. D., et al. (2008). In situ high-energy X-ray studies of magnetic-field-induced phase transition in a ferromagnetic shape memory Ni–Co–Mn–In alloy. Acta Mater. 56, 913–923. doi: 10.1016/j.actamat.2007.10.045
Wu, Z., Jing, X., Sun, B., and Li, F. (2016). A 6DOF passive vibration isolator using X-shape supporting structures. J. Sound Vibrat. 380, 90–111. doi: 10.1016/j.jsv.2016.06.004
Xu, C., Xu, Z. D., Ge, T., and Liao, Y. X. (2016b). Modeling and experimentation of a viscoelastic microvibration damper based on a chain network model. J. Mech. Mater. Struct. 11, 413–432. doi: 10.2140/jomms.2016.11.413
Xu, J., and Sun, X. (2015). A multi-directional vibration isolator based on Quasi-Zero-Stiffness structure and time-delayed active control. Int. J. Mech. Sci. 100, 126–135. doi: 10.1016/j.ijmecsci.2015.06.015
Xu, K., Chen, R., Wang, C., Sun, Y., Zhang, J., Liu, Y., et al. (2016a). Organomontmorillonite-modified soybean oil-based polyurethane/epoxy resin interpenetrating polymer networks (IPNs). J. Therm. Anal. Calorim. 126, 1253–1260. doi: 10.1007/s10973-016-5795-x
Xu, Z. D. (2009). Horizontal shaking table tests on structures using innovative earthquake mitigation devices. J. Sound Vibrat. 325, 34–48. doi: 10.1016/j.jsv.2009.03.019
Xu, Z. D., Gai, P. P., Zhao, H. Y., Huang, X. H., and Lu, L. Y. (2017b). Experimental and theoretical study on a building structure controlled by multi-dimensional earthquake isolation and mitigation devices. Nonlinear Dynam. 89, 723–740. doi: 10.1007/s11071-017-3482-5
Xu, Z. D., Liao, Y. X., Ge, T., and Xu, C. (2016c). Experimental and theoretical study of viscoelastic dampers with different matrix rubbers. J. Eng. Mech. ASCE, 142:04016051. doi: 10.1061/(ASCE)EM.1943-7889.0001101
Xu, Z. D., Sha, L. F., Zhang, X. C., and Ye, H. H. (2013). Design, performance test and analysis on magnetorheological damper for earthquake mitigation. Struct. Control Health Monit. 20, 956–970. doi: 10.1002/stc.1509
Xu, Z. D., Shen, Y. P., and Guo, Y. Q. (2003). Semi-active control of structures incorporated with magnetorheological dampers using neural networks. Smart Mater. Struct. 12:80. doi: 10.1088/0964-1726/12/1/309
Xu, Z. D., Suo, S., and Lu, Y. (2016d). Vibration control of platform structures with magnetorheological elastomer isolators based on an improved SAVS law. Smart Mater. Struct. 25:065002. doi: 10.1088/0964-1726/25/6/065002
Xu, Z. D., Suo, S., Zhu, J. T., and Guo, Y. Q. (2018a). Performance tests and modeling on high damping magnetorheological elastomers based on bromobutyl rubber. J. Intel. Mater. Syst. Struct. 29, 1025–1037. doi: 10.1177/1045389X17730909
Xu, Z. D., Tu, Q., and Guo, Y. F. (2012). Experimental study on vertical performance of multidimensional earthquake isolation and mitigation devices for long-span reticulated structures. J. Vibrat. Control 18, 1971–1985. doi: 10.1177/1077546311429338
Xu, Z. D., Wang, S. A., and Xu, C. (2014). Experimental and numerical study on long-span reticulate structure with multidimensional high-damping earthquake isolation devices. J. Sound Vibrat. 333, 3044–3057. doi: 10.1016/j.jsv.2014.02.013
Xu, Z. D., Xu, F. H., and Chen, X. (2016e). Intelligent vibration isolation and mitigation of a platform by using MR and VE devices. J. Aerospace Eng. ASCE, 29:04016010. doi: 10.1061/(ASCE)AS.1943-5525.0000604
Xu, Z. D., Xu, F. H., and Chen, X. (2016f). Vibration suppression on a platform by using vibration isolation and mitigation devices. Nonlinear Dynam. 83, 1341–1353. doi: 10.1007/s11071-015-2407-4
Xu, Z. D., Xu, M., and Sun, C. L. (2018b). Performance tests and microchain model validation of a novel kind of MR fluid with GO-coated iron particles. J. Mater. Civil Eng. ASCE, 30:04018072. doi: 10.1061/(ASCE)MT.1943-5533.0002253
Yamazaki, H., Takeda, M., Kohno, Y., Ando, H., Urayama, K., and Takigawa, T. (2011). Dynamic viscoelasticity of poly (butyl acrylate) elastomers containing dangling chains with controlled lengths. Macromolecules 44, 8829–8834. doi: 10.1021/ma201941v
Yang, C., Yang, G., Liu, F., Chen, Y., Liu, N., Chen, D., et al. (2006). Metastable phase formation in eutectic solidification of highly undercooled Fe83B17 alloy melt. Physica B Condensed Matter 373, 136–141. doi: 10.1016/j.physb.2005.11.112
Yang, S. Y., and Niu, J. (2012). “Numerical analysis on isolation effect of the SMA-disc spring three-dimensional composite bearings,” in Advanced Materials Research, eds H. Li, Y. F. Liu, M. Guo, R. Zhang, and Jing Du (Trans Tech Publications), 374, 2245–2249. doi: 10.4028/www.scientific.net/AMR.374-377.2245
Yoshioka, H., Takahashi, Y., Katayama, K., Imazawa, T., and Murai, N. (2001). An active microvibration isolation system for hi-tech manufacturing facilities. J. Vibrat. Acoust. 123, 269–275. doi: 10.1115/1.1350566
Zareh, S. H., Sarrafan, A., Khayyat, A. A. A., and Zabihollah, A. (2012). Intelligent semi-active vibration control of eleven degrees of freedom suspension system using magnetorheological dampers. J. Mechan. Sci. Technol. 26, 323–334. doi: 10.1007/s12206-011-1007-6
Zhao, Y., Su, J., Zhou, X., and Sui, Y. (2008). Shaking table test and numerical analysis of vertical-isolated building model with combined disk spring bearing. J. Build. Struct. 29, 99–106.
Zhou, J., Xiao, Q., Xu, D., Ouyang, H., and Li, Y. (2017). A novel quasi-zero-stiffness strut and its applications in six-degree-of-freedom vibration isolation platform. J. Sound Vibrat. 394, 59–74. doi: 10.1016/j.jsv.2017.01.021
Zhou, M. K., Xiong, X., Chen, L. L., Cui, J. F., Duan, X. C., and Hu, Z. K. (2015). Note: A three-dimension active vibration isolator for precision atom gravimeters. Rev. Sci. Instr. 86:046108. doi: 10.1063/1.4919292
Zhu, J. T., Xu, Z. D., and Guo, Y. Q. (2012). Magnetoviscoelasticity parametric model of an MR elastomer vibration mitigation device. Smart Mater. Struct. 21:075034. doi: 10.1088/0964-1726/21/7/075034
Keywords: multi-dimensional vibration isolator, vibration control, vibration mitigation material, civil engineering, mechanical engineering, aerospace engineering
Citation: Xu Z-D, Chen Z-H, Huang X-H, Zhou C-Y, Hu Z-W, Yang Q-H and Gai P-P (2019) Recent Advances in Multi-Dimensional Vibration Mitigation Materials and Devices. Front. Mater. 6:143. doi: 10.3389/fmats.2019.00143
Received: 15 March 2019; Accepted: 06 June 2019;
Published: 05 July 2019.
Edited by:
Yu-Fei Wu, RMIT University, AustraliaReviewed by:
Zheng Lu, Tongji University, ChinaAntonio Caggiano, Darmstadt University of Technology, Germany
Yi-Qing Ni, Hong Kong Polytechnic University, Hong Kong
Copyright © 2019 Xu, Chen, Huang, Zhou, Hu, Yang and Gai. This is an open-access article distributed under the terms of the Creative Commons Attribution License (CC BY). The use, distribution or reproduction in other forums is permitted, provided the original author(s) and the copyright owner(s) are credited and that the original publication in this journal is cited, in accordance with accepted academic practice. No use, distribution or reproduction is permitted which does not comply with these terms.
*Correspondence: Zhao-Dong Xu, eHV6aGRneXEmI3gwMDA0MDtzZXUuZWR1LmNu