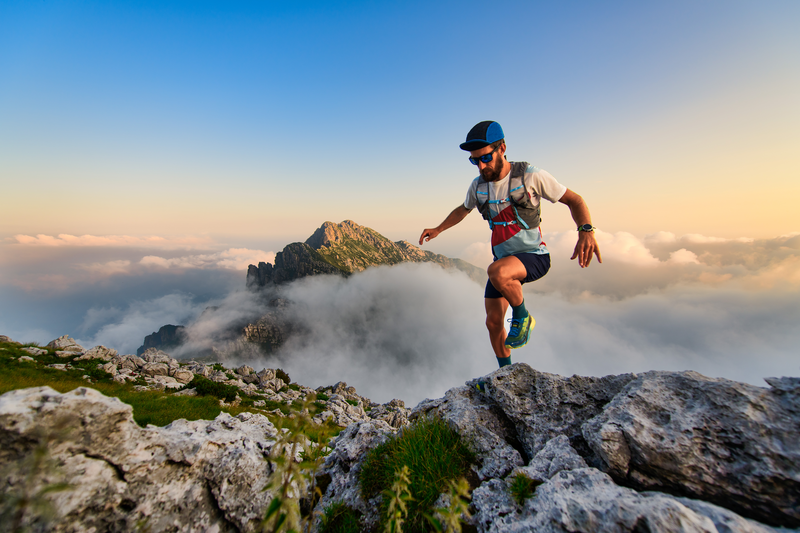
94% of researchers rate our articles as excellent or good
Learn more about the work of our research integrity team to safeguard the quality of each article we publish.
Find out more
ORIGINAL RESEARCH article
Front. Mater. , 30 April 2019
Sec. Structural Materials
Volume 6 - 2019 | https://doi.org/10.3389/fmats.2019.00090
This article is part of the Research Topic Innovation in Cements for Sustainability View all 11 articles
This paper presents the effect of nano alumina (NA) on compressive strength and microstructure of cement paste containing high volume blast furnace slag (HVBFS) contents of 70, 80, and 90% as partial replacement of cement and combined blast furnace slag (BFS) and class F fly ash (FA) contents of 70 and 80% as partial replacement of cement. FA is used at 30% as partial replacement of BFS. NA contents are varied from 1 to 4% as partial replacement of BFS and BFS-FA. Results show that the addition of NA improves the compressive strength of high volume BFS and BFS-FA pastes by 2 to 16%. The compressive strength of paste containing 69% BFS, 30% cement, and 1% NA exceeded the compressive strength of control cement paste while the compressive strength of paste containing 77% BFS, 20% cement, and 3% NA is 1% lower than control cement paste. NA significantly reduced the large capillary pores of >0.1 microns of high volume BFS and BFS-FA pastes. No evidence of reduction of Ca(OH)2 in high volume BFS pastes is observed due to addition of NA, however, in high volume BFS-FA paste the Ca(OH)2 is reduced due to addition of NA. Increase in intensity peaks of CAH, Ettringite and CSH in X-ray diffraction analysis is observed in high volume BFS and BFS-FA pastes due to addition of NA, which coincides with the observed more dense microstructure of high volume BFS and BFS-FA pastes containing NA than those without NA.
Concrete is the most widely used construction materials in the world. Its demand continues due to rapid urbanization and population growth in the world. As a result the demand of cement, the key ingredient of concrete, also increases. However, the manufacturing of cement is energy intensive and contributes about 5–7% of total CO2 release in to the atmosphere globally (Benhelal et al., 2013). Therefore, there is worldwide initiative to reduce the use of cement in concrete by partial replacement of cement using various industrial by-products such as fly ash, blast furnace slag (BFS), silica fume, rice husk ash, sugarcane bagasse ash, etc. Significant amount of research have been devoted worldwide to investigate various properties of concrete containing above by-products. Very good level of understanding of their use in concrete at low volume as well as at high volume already exists, which result their use in concrete as partial replacement of cement in many countries. However, their use in high volume in concrete often results in reduction in early age mechanical and durability properties due to slow pozzolanic reaction of these materials with cement hydration product (Oner and Akyuz, 2007; Aghaeipour and Madhkhan, 2017). Various ultrafine and nano materials are used to address the above limitation of slow pozzolanic reaction at early stage of concrete. In a number of studies the effect of various nano materials e.g., nano silica, nano CaCO3, nano TiO2, nano alumina, carbon nano tube, etc. on the properties of concrete containing fly ash at various volume fractions have been evaluated and promising results in terms of improvement in mechanical and durability properties of concrete containing fly ash are reported (Sato and Diallo, 2010; Hou et al., 2013; Shaikh and Supit, 2014, 2015; Shaikh et al., 2014).
Blast furnace slag is the by-product of iron/steel industry and is widely used in concrete as partial replacement of cement. Its use up to 60–70% as partial replacement of cement in concrete is researched (Elchalakani et al., 2014). It has been reported that above this limit the mechanical and durability properties are significantly affected (Oner and Akyuz, 2007). Despite the use of various nano materials in concrete containing high volume fly ash, not enough research is reported on the use of nano materials in concrete containing high volume slag above 60%, especially nano alumina which exhibited significant improvement in modulus of elasticity of concrete. Nano alumina, similar to other nano materials, provides synergistic effects in cement hydration process through seeding and filler effects and pozzolanic reaction. In a number of studies the effect of nano alumina on mechanical properties of concrete and cement composite is evaluated. Li et al. (2006) investigated the effect of various nano alumina contents of 3–7% on compressive strength and elastic modulus of cement mortar and reported about 143% improvement in 28 days elastic modulus of cement mortar due to addition of 5% nano alumina due to densification of cement matrix around interfacial transition zone of aggregates in mortar. However, at the same nano alumina content the improvement in compressive strength was not that great. In a separate study, Nazari and Riahi (2011) however, reported significant improvement (about 44%) in compressive strength of cement paste due to addition of 4% nano alumina. Reduction in harmful pores in cement paste due to addition of nano alumina is also observed in their study. On the other hand, Barbhuiya et al. (2014) reported no improvement in 7 days compressive strength of cement paste due to addition of nano alumina despite formation of dense microstructure of cement paste containing nano alumina. Improvement in compressive strength of cement pastes due to addition of nano alumina is also reported by others (Heikal et al., 2015; Gowda et al., 2017). The variation of results on properties of concrete due to addition of NA could be due to their different particle sizes e.g., the size of NA was ~150nm in the study by Li et al. (2006) whereas Nazari and Riahi (2011) use much smaller size NA particles of about 15 nm. On the other hand, the size of NA was 27–43 nm in Barbhuiya et al. (2014) study.
Mohseni and Tsavdaridis (2016) reported improvement in compressive strength and durability of fly ash mortar containing 25% fly ash due to addition of nano alumina. Reduction in gel and medium capillary pores of fly ash mortar is reported in this study due to addition of nano alumina. So far only one study reported by Nazari and Riahi (2011) evaluated the effect of nano alumina on mechanical and microstructure of self-compacting concrete containing various BFS contents of up to 60% as partial replacement of cement. While nano alumina exhibited improvement in mechanical and durability properties of cement paste and mortars and those containing fly ash and BFS, no study so far reported its effect on the concrete containing high volume slag content above 70% as partial replacement of cement. This paper presents the effect of various nano alumina contents on compressive strength and microstructure of cement pastes containing high volume blast furnace slag (HVBFS) and high volume blast furnace slag and fly ash (HVBFS-FA) as partial replacement of cement.
This study is consisted of five parts. In the first three parts, three high volume slag pastes containing 70, 80, and 90% BFS by weight as partial replacement of ordinary Portland cement (OPC) are considered. In the fourth and fifth parts, the effect of two hybrid combinations of BFS and class F fly ash (FA) at total contents of 70 and 80% by weight are considered, where FA is used at 30% by weight of BFS. In all parts the effect of nano alumina (NA) content of 1–4% by weight on the high volume BFS pastes and high volume BFS-FA pastes are evaluated. Therefore, total 26 mixes are considered in this study.
The cement used in this study was OPC. The fly ash (FA) was class F fly ash supplied by Eraring power station of New South Wales in Australia. The ground granulated BFS was supplied by BGC cement of Australia. The nano alumina (NA) powder was purchased from Nanostructured and Amorphous Materials, Inc. of USA. Table 1 shows the physical and chemical properties of OPC, FA, BFS, and NA, while Figures 1, 2 show the X-ray diffraction (XRD) analysis of FA, BFS and NA. It can be seen by comparing the results that NA was less amorphous compared to FA and BFS. Among FA and BFS, BFS is more amorphous with amorphous content of 95.7% compared to 67.8% amorphous content of FA based on quantitative XRD analysis. Detail mix proportions of high volume BFS pastes, high volume BFS-FA pastes and those containing NA are shown in Table 2. It can be seen that water/binder ratio of all mixes were kept constant at 0.4. In the case of mixes containing NA, a naphthalene sulfonate based superplasticizer was used to maintain the workability of pastes. All pastes were mixed in Hobart mixer, where cement, FA and BFS were mixed first for ~3 min. Water was added and mixed for further 3 min. In the case of high volume BFS and high volume BFS-FA pastes containing NA, the NA powder was first ultrasonically dispersed in water containing superplasticizer using ultrasonic mixer for 30 min shown in Figure 3 and then added with remaining water during mixing. The paste samples of 50 mm cubes were cast and demoulded after 24 h. The specimens were cured in water at room temperature for 28 days.
Table 2. Mix proportions of high volume slag and high volume slag-fly ash blend cement pastes containing nano alumina (NA).
Figure 3. Dispersion of nano alumina in water contining superplasticizer solution in ultrasonication method.
Compressive strength of the specimens were tested according to ASTM C109 (2012) using a loading rate of 0.7 MPa/s. In order to observe the changes in reaction phases in cement pastes containing high volume BFS and BFS-FA blends due to inclusion of NS thermogravimetric analysis (TGA), X-ray diffraction (XRD) analysis, Mercury intrusion porosimetry (MIP), scanning electron microscopy (SEM), and energy dispersive X-ray spectroscopy (EDS) were done on selected samples.
For the XRD analysis, the samples were measured with a D8 Advance Diffractometer (Bruker-AXS) using copper radiation and a Lynx Eye position sensitive detector. The diffractometer scanned the samples from 7° to 70° (2θ) in steps of 0.015° at a scanning rate of 0.5°/ min. XRD patterns were obtained using Cu Ka lines (k = 1.5406 Å). A knife edge collimator was fitted to reduce air scatter. SEM analyses were performed using a Zeiss EVO 40XVP microscope equipped with an energy dispersive X-ray analyser. The thermal stability of the samples was studied by thermogravimetric analysis (TGA). A Mettler Toledo TGA one star system analyser was used for all these measurements. Samples weighing 25 mg were placed in an alumina crucible and the tests were carried out in an Argon atmosphere at a heating rate of 10°C/min from 25 to 1,000°C.
Mercury intrusion porosimetry (MIP) was used to measure the pore volume and pore size distribution of pastes samples. The pore diameter and intruded mercury volume were recorded at each pressure point over a pressure range of 0.0083–207 MPa. The pressures values were converted into equivalent pore diameters using the Washburn expression 1921, as expressed in Equation (1):
where, d is the pore diameter (μm), γ is the surface tension (mN/m), θ is the contact angle between mercury and the pore wall (°), and P is the net pressure across the mercury meniscus at the time of the cumulative intrusion measurement (MPa).
The effects of various NA contents of 1–4% on compressive strength of high volume BFS pastes are shown in Figure 4. Figure 4A shows that due to addition of NA the compressive strength of high volume BFS paste containing 70% BFS is higher than that without NA. The compressive strength is increased by 2–16% due to addition of NA. In the case of high volume BFS pastes containing 80 and 90% BFS the improvement in compressive strength due to addition of NA is not better than 70% BFS content. It can be seen in Figures 4B,C about 2–8 and 1% improvement in compressive strength due to addition of various amount of NA in pastes containing 80 and 90% BFS, respectively. While the compressive strength of high volume BFS pastes containing 70% and NA is higher than that of control OPC paste, however, the compressive strength of paste containing 80 and 90% BFS is lower than OPC paste despite the addition of NA. This can be due to significantly lower level of Portlandite (Ca(OH)2) generation in these mixes due to very high volume of OPC replacement by BFS. It can also be seen no trend in compressive strength improvement with increase in NA contents in all high volume BFS pastes, however, beyond 3% no improvement in compressive strength is observed presumably due to agglomeration of NA as it has been reported by several researchers. Due to higher van der Waal's forces, the nano alumina particles in water causes agglomeration and hence, adversely affects their uniform dispersion in the matrix (Senff et al., 2009; Quercia et al., 2012).
Figure 4. Compressive strength of cement pastes containing (A) 70% slag, (B) 80% slag, (C) 90% slag, (D) 70% slag-fly ash blend and (E) 80% slag-fly ash blend and those containing various nano alumina contents.
The effect of NA on compressive strength of pastes containing combined BFS and FA contents of 70 and 80% is shown in Figures 4D,E. It can be seen that the addition of NA improves the compressive strength by up to 9% of paste containing total BFS and FA content of 70%, which is slightly lower than paste containing 70% BFS. In the case of combined BFS and FA content of 80% containing NA up to about 16% improvement in compressive strength is observed which is higher than those containing 80% BFS. However, the compressive strength of these two HVBFS-FA pastes for all NA contents is still lower than that of control OPC paste. The improved compressive strength due to addition of NA in pastes containing combined BFS and FA than the pastes containing BFS can be interpreted as the better dispersion of NA in those mixes due to spherical shape of the FA particles.
It can be seen in above compressive strength results that the high volume BFS paste containing 70% BFS and NA exhibited higher compressive strength than that of control OPC paste. High volume BFS paste containing 80% BFS and combined BFS and FA content of 70% showed about 1 and 7%, respectively, lower compressive strength then that of OPC paste. These mixes contain significantly less OPC hence much lower carbon footprint of concrete made using these blends. Therefore, in the microstructural analysis the changes of microstructures in terms of their pore sizes and volumes, conversion of Portlandite to secondary calcium silicate hydrate (CSH)/calcium aluminate hydrate (CAH) through pozzolanic reaction and formation of new mineral phases are studied due to addition of NA.
Figure 5 shows the cumulative pore volume and distribution of various pore sizes of high volume BFS and combined BFS and FA pastes with and without NA. It can be seen in Figure 5A that the volume of pores corresponding to pore diameter >0.1 microns increases in cement paste due to addition of high volume BFS contents of 70 and 80% as partial replacement of OPC. However, the volume of medium capillary pores and gel pores corresponding to pore diameters 0.05–0.01 micron and <0.01 microns, respectively, are decreased due to addition of 70% BFS compared to control OPC paste. In the case of paste containing 80% BFS the volume of medium capillary pores and gel pores are higher than OPC paste. By comparing the pastes containing two BFS contents, it can be seen that at 70% BFS content the total pore volume of all pores is less than at 80% BFS content, which might be the reason for observed higher compressive strength in the former paste than the latter as large pores might have caused stress concentration. On the other hand, in the paste containing combined BFS and FA with a total content of 70% the pore volume of larger capillary pores is reduced significantly compared to both high volume BFS pastes and control OPC paste. The distribution of pores having different diameters in all pastes is shown in Figure 5B. The region under the curves represent the concentration of pores and the peak point of the curve represents the critical pore diameter. By comparing different curves its can be seen that the maximum concentration of pores in OPC paste is in 0.03–0.2 microns range which is in lower side of large capillary pores. However, in the case of pastes containing 70 and 80% BFS and combined BFS and FA content of 70% the peak of their curves is shifted toward smaller pore diameter and they fall within medium capillary pores. This indicates that due to addition of BFS and combined BFS and FA the concentration of pores is shifted from large capillary pores to medium capillary pores.
Figure 5. Cumulative pore volume (A) and pore size distribution (B) of HVS and HVS-FA pastes with and without nano alumina.
The cumulative pore volume and pore size distribution of high volume BFS and combined BFS and FA pastes containing NA are also shown in Figure 5. It can be seen in Figure 5A that due to addition of NA the volume of pores corresponding to pore size >0.1 microns is significantly decreased in all high volume BFS and combined BFS and FA pastes. Surprisingly it can also be seen that volume of gel pores and medium capillary pores is increased due to addition of NA in high volume paste containing 70% BFS. The cumulative pore volume of paste containing 80% BFS is reduced from 0.164 to 0.155 cc/g due to addition of 3% NA. In the case of paste containing combined BFS and FA content of 70% the cumulative pore volume is reduced significantly from 0.155 to 0.14 cc/g due to addition of 1% NA. The formation of additional CSH and CAH gels due to enhanced pozzolanic reaction of NA with Portlandite in those high volume BFS and combined BFS and FA pastes could be the reasons for such reduction in volume of large capillary pores in those pastes. However, by looking into the pore size distribution shown in Figure 5B it can be seen that the concentration of pores is not significantly changed in high volume BFS and combined BFS and FA pastes due to addition of NA, but the volume of pores in the concentration of pore sizes in each paste containing NA is reduced.
TGA cures of high volume BFS and BFS-FA pastes and those containing NA are shown in Figure 6A. TGA curve shows the mass of a substance under control environment as a function of temperature. It has been reported that mass loss between 420 and 540°C corresponds to the dehydration of calcium hydroxide (Keattch and Dollimore, 1975). The amount of calcium hydroxide (CH) can be quantified according to Taylor (1990) formula shown as follows in Equation (2):
Where, WLCH is the weight loss during the dehydration of CH between 420 and 540°C as percentage of the ignited weight (%); MWCH is the molecular weight of CH; MWH2O is the molecular weight of H2O.
Figure 6. TGA analysis (A) and Ca(OH)2 contents (B) of HVS and HVS-FA pastes with and without nano alumina.
The calculated CH contents are shown in Figure 6B and it can be seen that CH contents of all high volume BFS and BFS-FA pastes and those containing NA are lower than that of OPC paste. This clearly indicate that due to pozzolanic reaction of SiO2 in BFS and FA with CH in those pastes CSH is formed. In Figure 6B it can also be seen slightly higher amount of CH content in high volume BFS pastes containing 77%BFS + 3%NA and 69%BFS + 1%NA pastes than that containing 80% BFS and 70%BFS pastes, respectively. This indicates that Al2O3 in NA did not produce any CSH in the matrix rather formed CAH which is confirmed in XRD and EDS analysis discussed in latter sections. Surprisingly, the CH content is much lower in paste containing 49%BFS + 20%FA + 1%NA then those of pastes containing 70% and 80% BFS. This can be interpreted due to reaction of SiO2 of FA with CH in that paste.
XRD patterns of high volume BFS and combined BFS and FA pastes with and without NA are shown in Figure 7. The horizontal scale is the diffraction angels measured in degrees and the vertical scale is the peaks height of the intensity of the diffractions measured in pulses/s. The diffraction spectra analysis of high volume BFS and combined BFA and FA pastes with and without NA indicate the predominance of calcium hydroxide (CH), calcium silicate hydrate (CSH), calcium aluminate hydrate (CAH), Ettringite (E), and Quartz (Q). By comparing the CH peaks of pastes with and without NA it can be seen that in most occasions intensity of CH peaks is not changed due to addition of NA except at 2θ angles 34.5° and 47° , where intensity of CH is reduced in pastes containing NA. Comparison also shows the formation of Ettringite in high volume BFS pastes containing NA at 2θ angles 43.5° and increase in intensity of Ettringite peaks at 2θ angles 19°, 25.6°, and 35°. The intensity of CAH peak at 2θ angle of 10.8° is also increased in high volume BFS and combined BFA and FA pastes due to addition of NA. In the case of high volume BFS-FA paste new mineral peaks of Mullite (M) and Gehlenite (G) can be seen at 2θ angles 16.5° and 20.8°, respectively, with increase of Gehlenite peak due to addition of NA.
SEM images and EDS spectra of high volume BFS and high volume BFS-FA pastes with and without NA are shown in Figure 8. It can be seen by comparing Figure 8B with Figure 8A that the microstructure of high volume BFS paste containing 69% BFS+1% NA is much denser than paste containing 70% BFS. By comparing both figures it can also be revealed less black spots indicative of pores/voids in high volume BFS paste containing NA than high volume BFS paste indicating formation of additional hydration products such as CSH/CAH as shown in the figure. Similar, improvement can also be seen in the high volume BFS paste containing 80% BFS and that containing NA in Figures 8C,D where paste containing 77% BFS and 3% NA in Figure 8D exhibited much denser microstructure than high volume BFS paste containing 80% BFS in Figure 8C. The EDS spectra in the same figure shows the formation of more CSH/CAH in the high volume BFS pastes containing NA than that of high volume BFS pastes without NA. The similar densification of microstructure of high volume BFS-FA paste due to addition of NA can also be seen by comparing Figure 8F with Figure 8E, where the peak of silica in EDS is much higher in paste containing NA than without NA indicating formation of more CSH/CAH in the former than the latter. The higher peak of silica in EDS is also evident in high volume BFS pastes containing NA than those without NA.
Figure 8. Backscattered scanning electron microscopic (SEM) images and EDS spectra of HVS and HVSFA pastes with and without nano alumina. (A) 70BFS paste. (B) 69BFS+1NA paste. (C) 80BFS paste. (D) 77BFS+3NA paste. (E) 49BFS21FA paste. (F) 48BFS21FA+1NA paste.
The effects of various nano alumina contents on compressive strength and microstructure of cement pastes containing HVBFS and high volume blast furnace slag-fly ash combination are reported. Based on limited study the following conclusion can be drawn:
• The addition of nano alumina (NA) improved the compressive strength of cement pastes containing 70, 80, and 90% BFS by about 16, 8, and 2%, respectively. In the case of combined BFS and FA content of 70 and 80% the improvements are 9 and 16%, respectively, due to addition of NA.
• The compressive strength of high volume BFS paste containing 70% BFS and NA exceeded the compressive strength of control OPC paste. However, not exceeded at 80 and 90% BFS contents and combined BFS and FA content of 70 and 80%.
• The addition of nano alumina significantly reduced the pore volume of large capillary pores of high volume BFS pastes, and increased the gel and medium capillary pores of paste containing 70% BFS. However, in the case of paste containing 80% BFS the volume of gel and medium capillary pores is reduced. The addition of nano alumina however, shifted the critical pore diameter of high volume BFS pastes from medium capillary pores to large capillary pores. However, in the case of high volume BFS-FA paste an opposite trend is observed.
• High volume BFS and combined BFS-FA pastes with and without NA exhibited significant reduction in Ca(OH)2 compared to control OPC paste. However, NA does not reduced the Ca(OH)2 in High volume BFS paste. Reduction in Ca(OH)2 is observed in high volume BFS-FA paste due to addition of NA than the control.
• Intensity of CAH, Ettringite and CSH peaks is increased in high volume BFS pastes due to addition of NA. New crystalline peaks for Mullite and Gehlenite are detected in high volume BFS-FA pastes containing NA.
• SEM images show denser microstructure of High volume BFS and combined BFS-FA pastes containing NA than those without NA. EDS analysis also shows increase in “Si” and “Al” peaks in EDS spectra around slag particles of High volume BFS and combined BFS-FA pastes due to addition of NA indicating formation of CSH/CAH in the matrix.
• The optimum mix could be that containing 69% slag and 1% NA whose compressive strength exceeded the control OPC paste's strength.
FS planned the experimental program, analyzed results, and wrote the manuscript. AH cast and tested all specimens in mechanical and microstructural studies and compiled and analyzed the results.
The authors declare that the research was conducted in the absence of any commercial or financial relationships that could be construed as a potential conflict of interest.
Aghaeipour, A., and Madhkhan, M. (2017). Effect of ground granulated blast furnace slag (GGBFS) on RCCP durability. Constr. Build. Mater. 141, 533–541. doi: 10.1016/j.conbuildmat.2017.03.019
ASTM C109 (2012). Standard Test Method for Compressive Strength of Hydraulic Cement Mortars Using 50mm cube Specimens. West Conshohocken, PA: ASTM.
Barbhuiya, S., Mukherjee, S., and Nikraz, H. (2014). Effects of nano-Al2O3 on early age microstructural properties of cement paste. Constr. Build. Mater. 52, 189–193. doi: 10.1016/j.conbuildmat.2013.11.010
Benhelal, E., Zahedi, G., Shamsaei, E., and Bahadori, A. (2013). Global strategies and potentials to curb CO2 emissions in cement industry. J. Cleaner Prod. 51, 142–161. doi: 10.1016/j.jclepro.2012.10.049
Elchalakani, M., Aly, T., and Abu-Aisheh, E. (2014). Sustainable concrete with high volume GGBFS to build Masdar City in the UAE. Case Stud. Constr. Mater. 1, 10–24. doi: 10.1016/j.cscm.2013.11.001
Gowda, R., Narendra, H., Rangappa, D., and Prabhakar, R. (2017). Effect of nano-alumina on workability, compressive strength and residual strength at elevated temperature of cement mortar. Mater. Today Proc. 4, 12152–12156. doi: 10.1016/j.matpr.2017.09.144
Heikal, M., Ismail, M. N., and Ibrahim, N. S. (2015). Physico-mechanical, microstructure characteristics and fire resistance of cement pastes containing Al2O3 nano-particles. Constr. Build. Mater. 91, 232–242. doi: 10.1016/j.conbuildmat.2015.05.036
Hou, P.-K., Kawashima, S., Wang, K. J., Corr, D. J., Qian, J. S., and Saha, S. P. (2013). Effects of colloidal nanosilica on rheological and mechanical properties of fly ash–cement mortar. Cem. Concr. Composites 35, 12–22. doi: 10.1016/j.cemconcomp.2012.08.027
Keattch, C. J., and Dollimore, D. (1975). Introduction to Thermogravimetry, Vol. 45. London: Heydon.
Li, Z., Wang, H., He, S., Lu, Y., and Wang, M. (2006). Investigations on the preparation and mechanical properties of the nano-alumina reinforced cement composite. Mater. Lett. 60, 356–359. doi: 10.1016/j.matlet.2005.08.061
Mohseni, E., and Tsavdaridis, K. D. (2016). Effect of nano-alumina on pore structure and durability of class F fly ash self-compacting mortar. Am. J. Eng. Appl. Sci. 9, 323–333. doi: 10.3844/ajeassp.2016.323.333
Nazari, A., and Riahi, S. (2011). Effects of Al2O3 nanoparticles on properties of self-compacting concrete with ground granulated blast furnace slag as binder. Sci. China Technol. Sci. 54, 2327–2338. doi: 10.1007/s11431-011-4440-y
Oner, A., and Akyuz, S. (2007). An experimental study on optimum usage of GGBS for the compressive strength of concrete. Cem. Concr. Composites 29, 505–514. doi: 10.1016/j.cemconcomp.2007.01.001
Quercia, G., Husken, G., and Brouwers, H. J. H. (2012). Water demand of amorphous nano silica and its impact on the workability of cement paste. Cem Concr Res.42, 344–357. doi: 10.1016/j.cemconres.2011.10.008
Sato, T., and Diallo, F. (2010). Seeding effect of nano-CaCO3 on the hydration of tri-calcium silicate. J. Transp. Res. Board 2141, 61–67. doi: 10.3141/2141-11
Senff, L., Hotza, D., Repette, W. L., Ferreira, V. M., and Labrincha, J. A. (2009). Influence of added nanosilica and/or silica fume on fresh and hardened properties of moratrs and cement pastes. Adv. Appl. Ceram.108, 418–428. doi: 10.1179/174367609X422108
Shaikh, F. U. A., and Supit, S. W. M (2014). Mechanical and durability properties of high volume fly ash (HVFA) concrete containing calcium carbonate (CaCO3) nanoparticles. Constr. Build. Mater. 70, 309–321. doi: 10.1016/j.conbuildmat.2014.07.099
Shaikh, F. U. A., and Supit, S. W. M. (2015). Chloride induced corrosion durability of high volume fly ash concretes containing nano particles. Constr. Build. Mater. 99, 208–225. doi: 10.1016/j.conbuildmat.2015.09.030
Shaikh, F. U. A., Supit, S. W. M., and Sarker, P. K. (2014). A study on the effect of nano silica on compressive strength of high volume fly ash mortars and concretes. Mater. Design. 60, 433–442. doi: 10.1016/j.matdes.2014.04.025
Keywords: nano alumina, blast furnace slag, fly ash, compressive strength, microstructure
Citation: Shaikh FUA and Hosan A (2019) Effect of Nano Alumina on Compressive Strength and Microstructure of High Volume Slag and Slag-Fly Ash Blended Pastes. Front. Mater. 6:90. doi: 10.3389/fmats.2019.00090
Received: 20 December 2018; Accepted: 10 April 2019;
Published: 30 April 2019.
Edited by:
Maria Juenger, University of Texas at Austin, United StatesReviewed by:
Denvid Lau, City University of Hong Kong, Hong KongCopyright © 2019 Shaikh and Hosan. This is an open-access article distributed under the terms of the Creative Commons Attribution License (CC BY). The use, distribution or reproduction in other forums is permitted, provided the original author(s) and the copyright owner(s) are credited and that the original publication in this journal is cited, in accordance with accepted academic practice. No use, distribution or reproduction is permitted which does not comply with these terms.
*Correspondence: Faiz Uddin Ahmed Shaikh, cy5haG1lZEBjdXJ0aW4uZWR1LmF1
Disclaimer: All claims expressed in this article are solely those of the authors and do not necessarily represent those of their affiliated organizations, or those of the publisher, the editors and the reviewers. Any product that may be evaluated in this article or claim that may be made by its manufacturer is not guaranteed or endorsed by the publisher.
Research integrity at Frontiers
Learn more about the work of our research integrity team to safeguard the quality of each article we publish.