- Department of Materials Science and Engineering, National Taiwan University of Science and Technology, Taipei, Taiwan
Among several active photothermal nanomaterials, tungsten-oxide-based materials have received considerable attention recently because of their ability to absorb near-infrared (NIR) light and their efficient light-to-heat conversion properties. In addition, tungsten-oxide-based materials have an unusual oxygen defect structure and strong local surface plasma resonance (LSPR), which offers strong photoabsorption in a broad wavelength range of the NIR region. In the past, several light-absorbing nanomaterials such as noble metals, polymeric materials, and other inorganic nanomaterials were of interest for their use in photothermal therapy for cancer treatment. In this study, we review the synthesis, properties, and applications of tungsten-oxide-based nanomaterials as a new type of photothermal material. The basic ideas behind photothermal nanomaterial development as well as the factors that influence their structural designs are also discussed in this study. In addition, recent progress in various fields such as NIR light-shielding, pyroelectric, water evaporation, photocatalysis, gas sensors, and energy-related applications for WO3−x- and MxWO3-based nanomaterials (including their hybrids) are highlighted. Finally, this review presents promising insights into this rapidly growing field that may inspire additional research leading to practical applications.
Introduction
Near-infrared (NIR) irradiation has a broad wavelength in the range of 780–2,500 nm. Fundamentally, nearly half of the energy available on the earth's surface is composed of sunlight that is near-infrared (i.e., greater than 780 nm). Maximizing NIR light for human use has been an interesting topic for scientists. NIR-absorbing photothermal materials (PTMs) have gained research interest because of their attractive light-to-heat behavior. Photothermal conversion is a process in which light energy of a specific wavelength is absorbed and is converted directly into heat. Through light-to-heat conversion, the heat generated can be applied to many fields such as photothermal therapy (Wang et al., 2017a), water evaporation (Wang, 2018), photocatalysis (Wang et al., 2017c), electrochromic devices (Liao et al., 2007), NIR shielding (Li et al., 2016b), and energy-related applications (Liu et al., 2018). PTMs have been used for photothermal ablation (PTA) therapy for the past few decades. Photothermal therapy is used in cancer treatment in which the target tissues are exposed to higher temperatures derived from photothermal properties to destroy abnormal cells (Chen et al., 2013). Yang et al. (2010) studied the in-vivo behavior of PEGylated nanographene sheets in tumor-bearing mice by in-vivo fluorescence imaging and determined that PEGylated nanographene sheets were extremely effective in in-vivo photothermal therapy (PTT).
In recent years, a variety of nanomaterials, noble metal nanomaterials, carbon-based materials, conductive polymers, and semiconductor nanoparticles have been studied for their NIR absorption properties (Lee et al., 2003; Marques, 2013; Zhang et al., 2015a; Wang et al., 2016a,b, 2017b; Riley and Day, 2017; Chen et al., 2018; Xu et al., 2018; Zhenzhen et al., 2018; Zeng et al., 2019).
Non-stoichiometric metal oxides such as WO3−x, MoO3−x, CuS, and TiOx are of particular interest, as their strong photoabsorption properties in the broad wavelength range of the NIR region make them suitable for various applications (Guo et al., 2011b; Chen et al., 2013; Hu et al., 2016; Yan et al., 2016; Ding et al., 2017). In semiconductor-based materials, light is absorbed to generate electron-hole pairs. In particular, tungsten-oxide-based materials are effective in their utilization of the NIR region. Of these, oxygen-deficient WO2.72 have been found to be useful in various applications such as smart windows, electrochromic devices, photothermal therapy, and NIR shielding. Other than photoabsorption properties, WOx-based materials with large band gaps have attracted a lot of research interest due to their potential uses in optical recording devices (Aoki et al., 2005), field-emission applications (Baek and Yong, 2007) and high-Tc superconductors (Reich et al., 2009). Moreover, tungsten-bronze-type compounds with the general structure of MxWO3 (, where M = Cs, Rb, K, Na, and NH4) were developed by doping an element that produces a tungsten bronze structure, which in turn exhibits broadband NIR absorption properties (Kim et al., 2012; Li et al., 2016c). The aim of this review is to discuss WO2.72, MxWO3, and their hybrid-based materials to promote further scientific investigations in this field. The utilization and preparation of common PTMs such as WO2.72 and MxWO3 are reported and the preparation techniques, photothermal conversion properties, and recent progress in their applications, including those in NIR shielding, water evaporation, pyroelectricity, photocatalysis, energy-related devices, and gas sensors are highlighted.
Photothermal Conversion Materials and their Properties
The first and foremost requirement of a PTM is that it must have a light-absorption capability. During the last few decades, various PTMs with strong light absorbance have been investigated for wide solar spectra. In the literature, various photothermal nanomaterials have been examined. Because of their NIR responsive properties, the photothermal conversion dynamics of these materials have not been fully explored.
Noble-based metals like Au, Ag, and Pd have been widely studied mainly for applications in cancer therapy. Among them, Au has been investigated for cancer therapy because of its chemical stability, facile synthesis, high quality, high yield, and nontoxicity (Huang and El-Sayed, 2010). The local surface plasma resonance (LSPR) band is much stronger, particularly for noble metals like Au and Ag. Chen et al. (2007) designed a relatively small gold nanocage with an 810-nm dimension using laser-driven PTT. They showed that immuno-gold nanocages strongly absorb at their LSPR peak and thus might be a major factor in reducing the thermal damage threshold. Despite the advantages related to the photothermal effects of noble metals, their relatively high cost and poor photostability at a prolonged laser irradiation have led the research community to explore other alternatives.
Carbon-based nanomaterials are primarily of two types: graphene- and carbon-nanotube (CNT)-based. These have been used in cancer therapy, solar evaporation, and sensor applications (Hashishin and Tamaki, 2008; Lou et al., 2016; Son et al., 2016; Hu et al., 2017). CNTs are best known for their photothermal conversion properties as well as their low cost, light weight, and high stability. Wang et al. (2016b) studied photothermal CNT-based materials and reported a solar thermal conversion efficiency of as high as 82% with bilayered CNT-silica materials. Polymers such as polydopamine was pioneered as a biodegradable PTM (Jiang et al., 2017). Organic polymers such as polypyrrole (Zhang et al., 2015a), polyaniline (PANI) (Huang et al., 2015a), and poly(1,3,5-hexahydro-1,3,5-triazine) (Chen et al., 2018) have been explored for their photothermal properties. Song et al. (2018) reported that hydrophobic Cu12Sb4S13 nanoparticles deposited on a porous cellulose acetate membrane form a photothermal film that could achieve photothermal heating for vapor generation and antibacterial activity simultaneously under light irradiation.
The research on semiconductor materials is rapidly expanding. In addition, studies are being conducted on noble and carbon-based PTMs, which includes copper chalcogenides, Ti2O3, MXene- (Ti3C2), MoO3−x, and WO3−x (Guo et al., 2012b; Ding et al., 2017; Lin et al., 2017; Wang et al., 2017b; Yan et al., 2017). These semiconductor-based materials are considered to be highly promising candidates, as they offer desirable photothermal conversion efficiencies. Copper chalcogenides are plasmonic-based metal-oxide-type semiconductors that are promising. Some researchers have investigated copper compounds, as they satisfy the efficiency requirement of strong vis-NIR absorption. Tian et al. (2011) synthesized hydrophilic flower-like CuS by using the hydrothermal method and achieved an enhanced absorption ability for a 980-nm laser. Similarly, nanocomposites like Cu9S5@SiO2 (Song et al., 2013), Cu7S4 (Song et al., 2014), and CuS (Zhou et al., 2010) nanoparticle have been investigated for potential use in 980-nm laser-driven PTA therapy (Hua et al., 2017). developed porous floating HCuPO-PDMS that exhibited a very high absorption in the vis-NIR band at 808 nm. A photothermal conversion effciency of 41.8% was achieved for solar evaporation by HCuPO due to d-d transition of Cu2+.
In this review, tungsten oxide materials are discussed in more detail with information on preparation and applications of WO2.72 and MxWO3. WO3 consists of perovskite units and is one of the most attractive candidates for photothermal conversion because of its suitable band gap (2.62 eV) (Huang et al., 2015b). It is also known for its good optical absorption characteristics under ultraviolet light. However, its photothermal conversion ability in NIR is inferior to that of WO2.72. As the NIR absorbent properties of WO2.72 have recently received greater attention, the photothermal performance of WO3 in other optical regions has also been investigated. The non-stoichiometric properties of WO2.72 have been an interesting topic over the past decades. Many forms of WO3−x such as WO2.9, WO2.83, and WO2.72, which generally are blue in color, have also been considered because of their unusual defects; these defects improve the electrical conductivity by a large degree. However, these materials are not soluble in any solvent. With a prolonged time, they can be oxidized to WO3. Moreover, the low crystallinity of WO2.72 may be a concern (Sun et al., 2018). Thus far, the photothermal capabilities of other forms of WO3−x are still unknown. Doping an element in WO3 produces MxWO3, which further enhances the optical absorption in the solar spectrum. MxWO3 has also attracted considerable attention because it offers a wide variety of crystal structures and has many interesting attributes such as electrochromic, optical-electrical, and superconductive properties.
Semiconductors such as WO3−x are interesting candidates as LSPR hosts because of their unique characteristic of having an outer d-valence electron. WO3−x is well-known for its non-stoichiometric properties derived from the presence of numerous oxygen vacancies that can narrow the band gap. The new discrete energy bands below the conduction band are created by the oxygen vacancies (Yan et al., 2015; Zheng et al., 2015). The strong NIR absorption properties are induced by LSPR with an intensity comparable to the bandgap absorption and is achieved either by creating oxygen vacancies or by inserting metal ions. The LSPR band in plasmonic nanoparticles is proportional to the square root of the free electron density in the particle. The LSPR is dependent on certain factors such as the particle size, shape, structure, and the dielectric properties of the metal (Huang and El-Sayed, 2008). WO3−x with a band gap of 2.4–3.0 eV are recognized as n-type semiconductors. Heat treatment in a reducing atmosphere is an effective approach to increase the concentration of oxygen vacancies. The dopants contribute electrons and increase the free-electron density in the conduction band. Finally, the LSPR creates an intensive local electric field, which is favorable for many practical applications (a topic to be discussed in the next section). The presence of mixed valence W5+ and W6+ sites are promising for harvesting NIR light through small polaron absorption and for increasing the electrical conductivity (Wang et al., 2017c). Among the non-stoichiometry tungsten oxides, monoclinic WO2.72 (expressed as W18O49) has received considerable interest due to its distinctive oxygen defect structure and intense near-infrared photoabsorption.
In our previous study, we demonstrated the photothermal conversion properties of WO2.72/polyurethane (PU) nanocomposites using a UV-Vis NIR spectrometer (Chala et al., 2017). The transmittance values of WO2.72/PU were found to be 75% in the visible region (400–780 nm). Transmittance of the WO2.72/PU nanocomposites in the range of 780–2500 nm was very low (8%), which suggested that the WO2.72/PU nanocomposites exhibited stronger NIR absorption (92%) compared to other nanocomposites. This was because of the presence of free electrons or oxygen-vacancy-induced small polarons formed during the reduction process. For comparison, the transmittance spectra of WO2.8/PU, WO3/PU, and WO2/PU nanocomposites are also shown (Figure 1A). The WO3−x phase went through phase transformations during reduction, under a CO atmosphere, and created oxygen vacancies that led to a non-stoichiometric structure. The color of the WO3−x powder varied after reduction at different temperatures (Figure 1B). The color changed from yellow to dark blue and to black for WO3, WO3−x, and WC, respectively. The temperature ΔT rapidly increased for WO2.72/PU, WO2.8/PU, WO3/PU, and pure PU to 58.9, 41.9, 30.9, and 9.9 °C after 30 s, respectively (see Figure 1C) (Chala et al., 2017). These results suggested that the WO2.72/PU nanocomposites exhibit a faster photothermal conversion rate than do WO2.8/PU, WO3/PU, and pure PU. As previously discussed, WO2.72 considerable instabilities is the result if a longer time passes in which WO2.72 can be oxidized to WO3. This problem can be overcome by MxWO3, as it has similar absorption properties. Chen and Chen (2013) reported the photothermal conversion property of Cs0.33WO3, which exhibited strong characteristic absorption in the NIR region because of free electrons or polarons. It was demonstrated that NIR absorption was considerably enhanced by reducing the particle size or by increasing the particle concentration. In addition, the study reported that it generated a photothermal conversion efficiency of approximately 73% while demonstrating excellent photothermal stability. An effective NIR absorption and photothermal conversion ability was proved, which revealed great potential for practical applications.
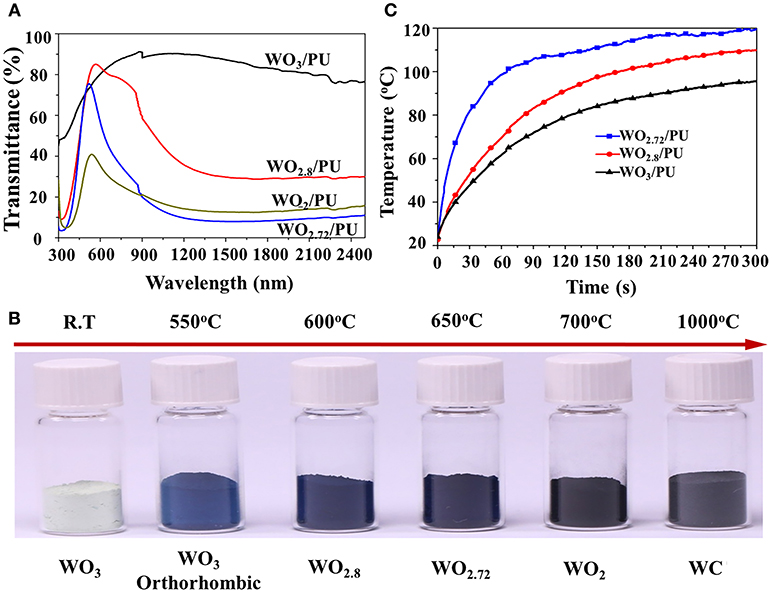
Figure 1. Photothermalconversion properties of WO2.72/PU nanocomposites by (A) UV-Vis-NIR transmittance spectra, (B) variation in the color observed for reduced tungsten oxide powder, and (C) the temperature difference WO3/PU, WO2.8/PU, and WO2.72/PU with 7 wt % as a function of time under infrared light irradiation. Reproduced with permission (Chala et al., 2017). Copyright 2017, MDPI.
Preparation of Tungsten-oxide-based Materials
In this section, we discuss various preparation methods and the pros and cons of synthesizing nanomaterials including MxWO3 and WO3−x. For MxWO3, these are summarized in Table 1. These include mechanochemical, vapor-phase synthesis, solid phase reaction, inductively coupled thermal plasma, and hydrothermal and solvothermal methods. Hydrothermal and solvothermal methods have been widely adopted because of their easy scalability.
Mechanochemical Method
Mechanochemical techniques like ball-milling or grinding are considered to be candidates for solvent-free synthesis. This method involves a chemical transformation which is induced by mechanical energies such as compression, shearing, or friction. Wang et al. (2003) prepared Na0.88WO3 nanocrystals by grinding the precursor Na pieces and WO3 powders having an average grain size of 17 nm. Electrical property tests showed that Na0.88WO3 exhibited semiconductor characteristics that might cause lattice distortion of the material as a result of high-energy ball-milling affecting its electrical conductivity. This process has many advantages including the use of low-cost raw materials, simplicity of process, and the ability to obtain fine particles. However, the main limitation is that the chemical reaction must be controlled for air- and moisture-sensitive substances.
Chemical Vapor Transport
Based on the principles of chemical vapor transport (CVT), volatilization of a solid in the presence of a gaseous reactant (the so-called “transport agent”) deposits the solid elsewhere and usually in crystalline form Schmidt et al. (2013). Hussain et al. (1997) employed this method and prepared crystal growth of alkali metal tungsten bronzes MxWO3−x (M = K, Rb, Cs). Several transport agents were used (HgCl2, HgBr2, Hgl2, Cl2, PtCl2), but HgCl2 and HgBr2 were found to be equally efficient as transport agents for growing large crystals of tungsten bronzes. The crystals were grown to 6 mm in length for hexagonal tungsten bronzes. However, the tetragonal tungsten bronzes (MxWO3) were prepared at a size of 0.1 mm by using HgCl2 and HgBr2, where x was 0.25. Yet, when x ≥ 0.35, very little or no transport occurred. The transport rate and crystal size were reduced with increasing alkali metal concentration. These were prepared under isothermal conditions both with and without adding transport agents, which showed nearly identical results. However, the results showed an appreciable transport effect when used as transport agents. Note also that the use of transport agents may cause environmental damage with high-energy consumption.
Solid-Phase Reaction
A solid-phase reaction refers to a process in which a solid reaction occurs between two solids, and a solid product is formed without chemical equilibrium. This technique is simple and requires low-cost equipment. However, it has a slow reaction rate at a high temperature. Preparation of tungsten bronze Cs, Rb, Na, and K have been reported when using the solid-phase reaction method (Lee et al., 2014b). Takeda and Adachi (2007) synthesized hexagonal tungsten bronze (HTB) Cs0.33WO3, Rb0.33WO3 powder and cubic tungsten bronze (CTB) Na0.75 WO3 powder using metal salts mixed with WO3·NH3 as a precursor that reacted at 550°C for 1 h with H2/N2 or H2/Ar. It was then annealed at 800 °C in an N2 atmosphere for 1 h. Cs0.33WO3, Rb0.33WO3 resulted in strong and broad NIR absorption peaking at approximately 1,500 nm. It was revealed that the HTB phase M0.33WO3 was quite suitable for solar filter applications because of its strong absorption in the NIR range. Moon et al. (2013) demonstrated that quaternary tungsten bronze has better NIR absorption properties in the range of 780 to 1,200 than does tungsten bronze because of the modulated optical response by the quaternary element of sodium.
Inductively Coupled Thermal Plasma Method
Thermal plasma is mainly used as a heat source for the evaporation of solid precursors or decomposition of gaseous precursors. Reactive gases are used as main constituents to form a plasma flame for synthesis of nano-sized materials. Thermal plasma synthesis of MxWO3 was reported by Mamak et al. (2010) in which a powder mixture containing a precursor of (NH4)10(H2W12O42). 4H2O and salt of HCOOCs, Na2CO3, and K3C6H5O7 in a varied ratio of W and M were used. However, Ar was used as the central gas along with a small amount of H2 to provide the reducing environment required for the synthesis of MxWO3. A mixture of tungsten and alkali salts with low decomposition temperature was used as the precursor. Thermal plasma synthesis has advantages in terms of material handling, raw material cost, and processing throughput. Inductively coupled thermal plasma (ICTP) synthesis has proven to be a unique method for the high throughput production of MxWO3, where M = Na, K, and Cs tungsten bronze nanopowders were synthesized at a high purity, using low-cost precursor materials. In general, materials produced by thermal plasma have a favorable optical absorption, high purity, and a tunable composition when using low-cost precursor materials. Major applications include coatings and heat shielding filters, as they exhibit a high extinction coefficient in the NIR region with little effect on transparency or visible color. ICTP is a fast reaction method for high production at low temperatures. It has high potential and can be used extensively in future research.
Hydrothermal Method
The hydrothermal method is simple and versatile for use in the synthesis of inorganic nanomaterials from aqueous solutions under high-temperature and high-pressure conditions. Temperature, pressure, and precursor concentration are the parameters that must be adjusted to the characteristics of nanomaterials. Water is the most commonly used solvent in the hydrothermal process. The water density and the dielectric constant are highly dependent on the temperature and pressure. A drop in the dielectric water constant is closely linked to an increase in temperature and a decrease in pressure. As the dielectric constant of water decreases, the reaction rate is enhanced considerably and thus the nucleation growth of crystals is facilitated. It offers many advantages such as a one-step synthetic procedure, environmental friendliness, production feasibility, good dispersion in solutions, and inexpensive instrumentation. Moreover, this method avoids the use of H2 and considerably improves safety. However, it induces high-temperature energy consumption. Tungsten bronze such as Cs0.33WO3, K0.26WO3 nanorods and (NH4)0.33WO3 nanorods have been reported by hydrothermal method. For the preparation of Cs0.33WO3, the precursor Cs2WO4, WO2, WO3, and distilled water were mixed and heated at 800°C for 24 h (Okusako et al., 2012). K0.26WO3 nanorods were synthesized with K2WO4, K2SO4, and distilled water at 200°C for 24 h and further post-calcined at 600°C for 2 h under an atmosphere of H2 (5 vol%)/N2 (Wu et al., 2017b). For the synthesis of (NH4)0.33WO3 nanorods, ammonium paratungstate, ethylene glycol, and acetic acid were mixed and heated at 200°C for 72 h (Guo et al., 2012a). Liu et al. (2013a) reported the synthesis of K0.26WO3 nanowires by an electrostatic-induced process using K2WO4 as a precursor along with ethylenediamine (EDA) and water. It was heated further in an electric oven at 250°C for 48 h. EDA served as a reducing agent, which is much milder compared to other reducing agents such as NaBH4 (Zhu and Manthiram, 1994) or N2H4 (Yang et al., 2003) used in the preparation of tungsten bronzes. In fact, it is important to note from these preparations that different nanoparticles have different reaction schemes and processing conditions. Therefore, determining the proper chemical reactions with suitable conditions for different nanoparticles is desirable.
Solvothermal Method
The solvothermal method uses ethanol and ethylene glycol instead of water as a solvent to achieve the dual role of solvent and reducing agent. The control variables can be varied by adjusting the solvent type, changing the reaction atmosphere, and using different surfactants, pH, reactant concentration, and filled volume of autoclave. The Cs0.3WO3 powder was synthesized by a normal solvothermal reaction with the precursor of WCl6, where their metal hydroxides (CsOH) were mixed with ethanol and heated at 200°C for 12 h and further annealed in the NH3 atmosphere at 500°C for 1 h (Liu et al., 2010). Cs0.32WO3 powder was also synthesized by a H2WO4 precursor heated at 220°C for 4 h (Yao et al., 2018). To control the water releasing process, which is based on an esterification reaction between ethanol and acetate acid, monodispersed nanorods of MxWO3 (M = Cs and Rb) were produced (Guo et al., 2010; Wu et al., 2017b). An ethanol solution of WCl6 and CsOH was mixed with CH3COOH and heated at 200°C for 20 h. In this method, the heat treatment temperature is reduced by replacing it with ethanol as compared to the hydrothermal method. Moreover, the ethanol solvent serves a multifunctional property to control the morphology and to decrease the heat treatment temperature. Thus, it overcomes the high temperature energy consumption using a low temperature. However, comparatively, the hydrothermal method has a reduced environmental impact.
Figure 2 demonstrates different morphologies of WO2.72 with the function of reaction time. This shows a morphological evolution from nanoparticles to urchin nanostructures (Su and Lin, 2009; Guo et al., 2012c; Moshofsky and Mokari, 2012). Table 2 presents a brief summary of the nanostructured WO2.72 types that were prepared by CVT and solid-phase reaction. W/WO2.72 heterostructures (Liu et al., 2013b) (WO2.72 nanowires grown on the side surface of the W whiskers along the radial direction), WO2.72 nanoneedles (Jin et al., 2004), WO2.72 sub-micro fibers (Liu et al., 2012), and WO2.72 tapered needles (Wang et al., 2007) were synthesized using WO3 as a precursor by a two-step CVT method. In general, the temperature of the furnace was increased from the room temperature to 800–1000°C. WO2.72 nanoparticles were reported by the solid-phase reaction method using WCl6 as a raw material with ethanol (Venables and Brown, 1996; Takeda and Adachi, 2007). Ma et al. (2017) prepared WO2.72 nanoparticles using WCl6 and ethanol with NH3 solution as a solvent, which was pyrolyzed under air atmosphere. Because ammonia molecules tend to chelate strongly with tungsten ions, they could work as capping agents to hinder particle growth.
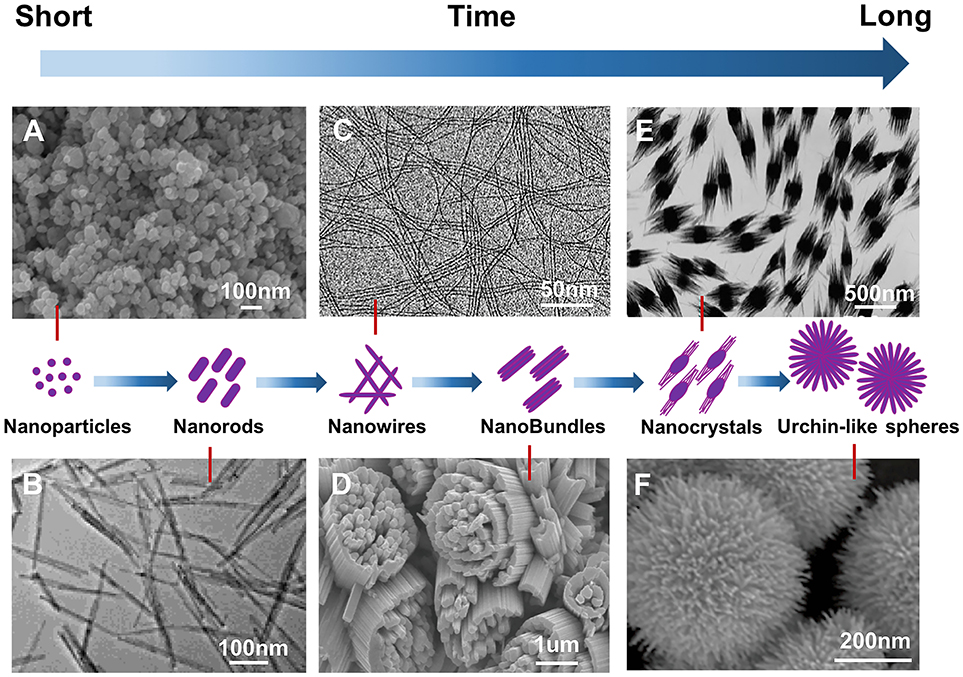
Figure 2. Morphologies of WO2.72 prepared with different reaction time. (A) nanoparticles, (B) nanorods, (C) nanowires. Reproduced with permission (Moshofsky and Mokari, 2012). Copyright 2012, Chemistry of Materials. (D) nanobundles. Reproduced with permission (Su and Lin, 2009). Copyright 2009, The Journal of Physical Chemistry C. (E) nanocrystals. Reproduced with permission (Guo et al., 2012c) Copyright 2012, American chemical society (ACS), and (F) urchin-like spheres.
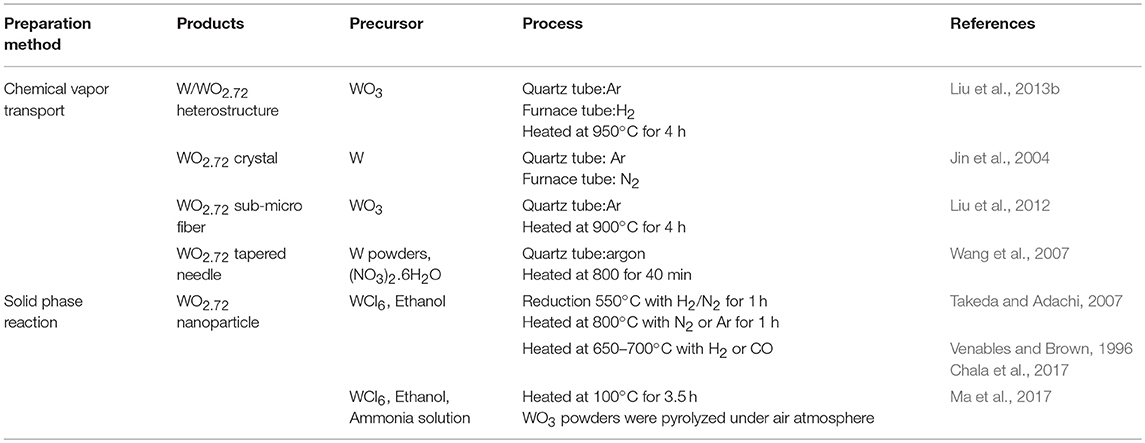
Table 2. Preparations of non-stoichiometric tungsten-oxide (WO2.72) by chemical vapor transport and solid phase reaction.
A brief summary of the preparation of WO2.72 nanostructures by hydrothermal and solvothermal methods are listed in Table 3. WO2.72 nanorods have been reported by the hydrothermal method using H2WO4 and Na2SO4 at 160°C for 24 h (Lou and Zeng, 2003). Guo et al. (2011b) reported WO2.72 nanorods synthesized hydrothermally by reducing the as-obtained (NH4)xWO3+x/2 and using sulfate as a capping agent in an atmosphere of H2 (5 vol%)/N2 at 500°C for 1 h. The solvothermal method was extensively studied for WO2.72 for different nanostructures. WO2.72 nanowires were synthesized by a simple solvothermal method using WCl6 and ethanol solution under 180°C for 10–24 h (Qin et al., 2011b; Xi et al., 2012; Guo et al., 2016). Cetyltrimethylammonium bromide has also been used as a growth-directing agent to fabricate WO2.72 nanowires (Li et al., 2016a). Huang et al. (2014a) synthesized WO2.72 nanowires by using WCl6/NaNO3 through -mediated assembly. With an increase in the concentration, the uniformity of the WO2.72 alignments was enhanced, clearly demonstrating the process of -medicated orientation. Li et al. (2016b) fabricated WO2.72 nanofibers by using WCl6 with oleic acid and 2.5 mL tri-n-octylamine under 350°C for 1 h. WO2.72 nanowire bundles were synthesized with WCl6 and propanol solvent at 200°C for 9 h, and the product was annealed in air at 250–450 °C for 2 h (Qin et al., 2011b). A different morphology was reported when varying the annealing temperature from 250 to 550°C. It was found that nanowires remain unchanged by the annealing treatment at 250 and 350°C. However, annealing at 450 and 550 °C induced WO3 nanobelt-like structures. The Fabrication of mesoporous 1D-WO2.72 nanobelts was conducted using a solvothermal method mixed with WO3, polyvinyl pyrrolidone, and EDA at 180°C for 12 h, calcined at Ar/H2 atmosphere at 580°C for 3 h (Sun et al., 2016). 3D WO2.72 networks were obtained by solvothermally treating WCl6 in ethanol at 160°C (Bai et al., 2013). The results showed that high precursor concentration contributed to the formation of WO2.72 networks. However, when the concentration was further increased, plate-like WO2.72 was found at a lower concentration. Sea-urchin-like structures composed of radial nanowires were obtained. WO2.72 nanocrystals were synthesized by treating the precursor of WCl6 and ethanol under 200°C for 24 h (Guo et al., 2012c). The effect of the tungsten precursor on the morphology was investigated in this case. Similar experiments were conducted using mixtures of WCl6 and tungsten (VI) ethoxide (W(EtO)6) with varied molar ratios, WO2.72 nanorods were obtained with sizes ranging from 300 to 600 nm when mixtures of 5 mM W(EtO)6 and 10 mM WCl6 were used as the tungsten source. However, when equimolar mixtures of W(EtO)6 and WCl6 (7.5 mM) were used, plate-like particles with sizes of 300–500 nm were obtained. As the concentration of W(EtO)6 was increased to 50 mM, well-defined monodispersed microspheres of 0.5–2 μm diameter were formed. It was suggested this might be because of the rate of water generation from different tungsten sources, and the difference in the hydrolysis behavior between W(EtO)6 and WCl6 resulted in different morphologies of WO3−x. Mesoporous sphere 3D WO2.72 was formed by using WCl6 as a precursor mixed with solvent ethanol and CH3COOH at 180°C for 16 h (Huang et al., 2014b; Zhao et al., 2017). At low precursor concentrations, only semi-closed spheres and disordered nanoparticles were formed. At higher concentration, hollow spheres were formed very easily that were bigger and had thicker shells. Only disordered nanoparticles were formed at a low temperature (120°C) even when sufficient WCl6 was provided. This demonstrated that the morphology can be fine-tuned by controlling variables such as time, precursor concentration, and temperature (Guo et al., 2016). synthesized WO2.72 urchin-like nanostructures and nanowires by treating WCl6 with ethanol under 180°C for 10 h. WO2.72 nanowires were obtained when the precursor concentration was 0.5 g L−1 and the reaction time was 10 h. Control of different morphologies by adjusting the concentration of precursor was discussed in this study. When the precursor concentration increased to 1 g L−1, the WO2.72 nanowires changed to bundle-like WO2.72. When the concentration was further increased to 3 g L−1, a mixture of urchin-like nanostructures and nanowires was obtained. Once the concentration reached 5 g L−1, nanospheres were obtained. The results showed that urchin-like WO2.72 nanostructures have a better light harvesting capacity in the IR region than nanowires. The mechanism of different nanostructures proposed as WO2.72 nanocrystals would preferably grow in the [010] direction as nanowires. Because the diameter of the nanowires was very small (with a large surface energy), the nanowires became bundle-like WO2.72 (thus reducing the surface energy). As a result, bundle-like WO2.72 nanostructures continued to be packaged and developed into urchin-like WO2.72 nanostructures. Urchins were thus formed and further developed into nanospheres. Zhao et.al reported that WO2.72 architectures including nanofibers, nanofiber bundles, and sea urchin-like microspheres were prepared by a template-free solvothermal method that tuned the WCl6 concentrations (Zhao et al., 2017). In fact, it is important to note from these reports that different nanoparticles have different reaction schemes and processing conditions. Therefore, the proper chemical reactions with suitable conditions for different nanoparticles must be determined. Compared with the methods previously mentioned, solvothermal treatment is a facile, cost-effective, and well-studied liquid-phase technique, which has the capability of producing WOx with different nanomorphologies.
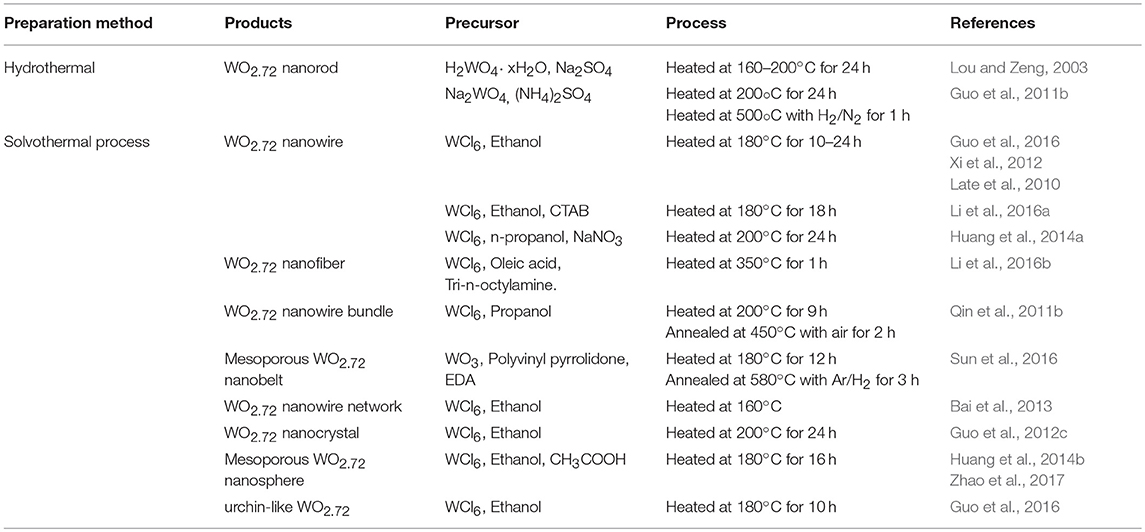
Table 3. Preparations of non-stoichiometric tungsten-oxide (WO2.72) by hydrothermal and solvothermal methods.
Applications of Tungsten-oxide-based Materials
Tungsten-oxide-based materials WO2.72, MxWO3, and their hybrids have attracted considerable attention in various fields such as heat generation, photocatalysis, and energy-related and gas-sensor applications. These applications are both important and interesting. They are discussed in more detail as follows.
Heat Generation
Heat generation is emerging as a promising technology and one of its important practical applications is in manufacturing polyethylene terephthalate (PET) bottles. An extruder PET preform is heated above its glass transition (Tg) point by IR irradiation so than it can be blown into the required shape. A small amount of WO3 is incorporated into the PET to reduce the IR irradiation time and thus speed up productivity. The photothermal conversion properties of WO2.7 show much greater potential in heat generation. A rise in temperature in a short span of time further reduces the IR irradiation time with WO2.72. This could considerably improve the productivity of PET bottles. Considering their potential in harvesting solar energy and in heat conversion, various applications such as thermo/pyroelectricity, water evaporation, and NIR shielding are discussed.
Thermo/Pyroelectricity
Thermoelectric technology has been widely used as a means to convert heat into electrical energy through the Seebeck effect. Pyroelectricity is one of the least-known properties of certain solids and condensed materials. This property pertains to temperature-dependent spontaneous polarization in certain anisotropic solids and refers to the ability of a certain class of materials to generate an electric charge when heated and cooled consecutively. The temperature variations slightly modify the position of atoms within the crystal structure causing effects such as polarization change. The change in polarization creates a voltage across the material. Thus, it can be used as a thermal-electric converter. However, achieving a high temperature difference in a non-conducted way is difficult. Therefore, it cannot be efficiently used to convert thermal energy into electric energy through a time-dependent temperature variation with spatial uniformity. To this end, PTM WO2.72 incorporated within an electrospun pyroelectric polyvinylidene difluoride (PVDF) fiber membrane were prepared. Schematics of the pyroelectric measurement are shown in Figure 3A. Here, a higher temperature variation was obtained by irradiating NIR light in an on-off sequence on WO2.72/PVDF fiber membranes. This resulted in higher output voltages compared to the fiber membranes without WO2.72 (Figure 3B) (Wu et al., 2017a). Steady reproducibility of temperature variation and higher output voltages are shown as well. The results demonstrate that the WO2.72/PVDF materials can be used for NIR sensing and solar energy harvester applications (Wu et al., 2017a). It is worth noting that hybrids of WO3 have recently gained attention in thermo/pyroelectric studies. However, WO2.72 and MxWO3 (their hybrids) are yet to be explored.
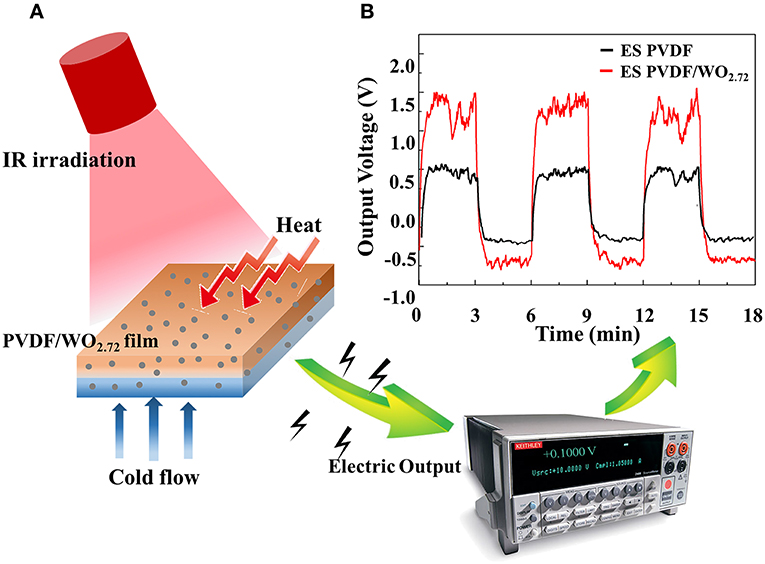
Figure 3. (A) Schematic illustrations of the pyroelectric measurement and (B) pyroelectric ability of electrospun PVDF and PVDF/WO2.72 in response to the corresponding rate of change in temperature (Wu et al., 2017a).
Water Evaporation
A solar-driven water evaporation process that utilizes sunlight as a renewable energy resource can be used in numerous practical applications. Such applications include freshwater production, desalination, and distillation (Hua et al., 2017; Shang et al., 2017; Awad et al., 2018; Kim et al., 2018). Solar heating designed as “air-water interface solar heating” has the ability to trap a wide solar spectrum selectively by strengthening the air-water interface (Wang et al., 2017d). However, heat transfer minimizes from interfacial to underlying bulk water. The photothermal layer that induces self-floating on the top of a water surface is deliberately designed as a heat barrier, that introduced interfacial heating in solar thermal applications (Liu et al., 2015; Lou et al., 2016). To include multifunctionality in a single-component material, multilayered materials offer researchers the opportunity to design more practical solutions, as demonstrated in our recent work. We had efficiently utilized WO2.72 photothermal materials with polylactic acid (PLA). The photoabsorption properties of these photothermal materials made them suitable for converting light energy to thermal energy (Chala et al., 2018a). These composites were designed as fiber membranes that have a self-floating ability and act as heat barriers at air-water interfaces for light-driven water evaporation. Figure 4A shows a schematic of the experimental setup used for this measurement. For WO2.72/PLA, a rapid rise of temperature ΔT can be seen from 19.4°C to 44.7°C and then to 75.3°C for over 5 min of irradiation containing 0, 4, and 7 wt% of WO2.72 nanoparticles, respectively, as shown in Figure 4B. The water evaporation efficiency of the WO2.72/PLA fiber membrane containing 7 wt% WO2.72 nanoparticles reached 81.39%, which is significantly higher than pure water 39.09% (Figure 4C) (Chala et al., 2018a). These distinct properties of WO2.72 make it feasible for commercial applications such as steam generation, desalination, and sterilization.
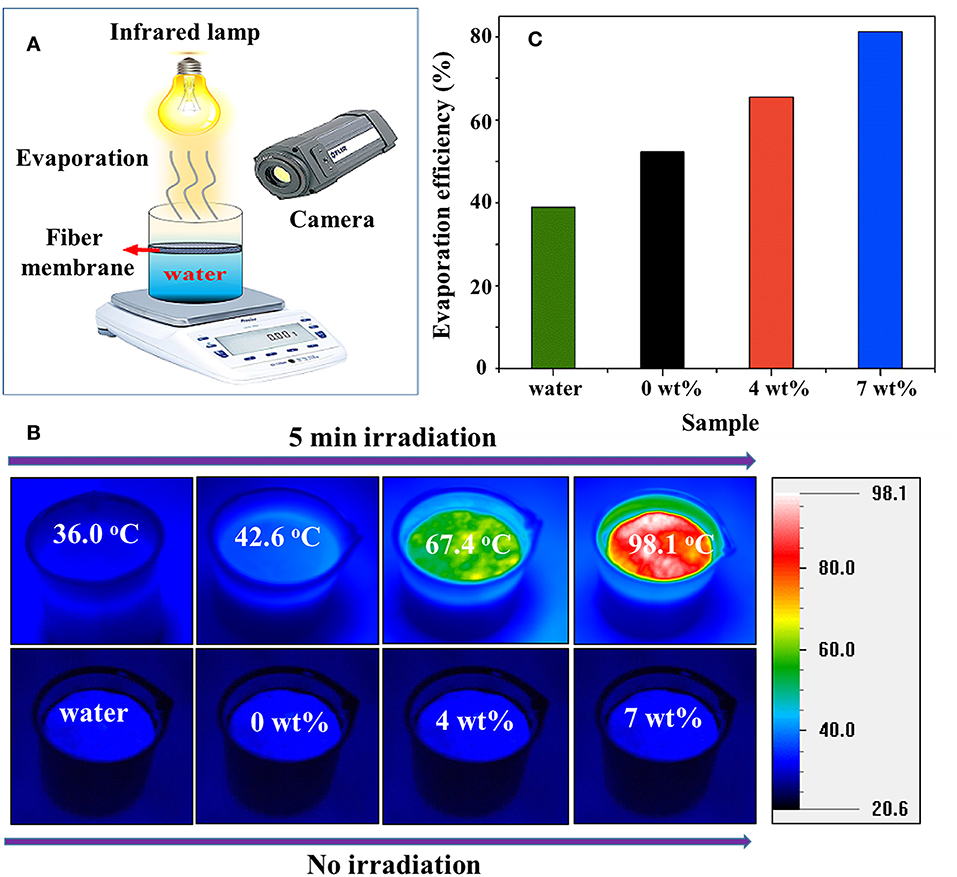
Figure 4. (A) Schematic of water evaporation measurement with WO2.72/PLA fiber membrane floating on air-water interface, (B) temperature of WO2.72/PLA fiber membrane containing 0, 4, and 7 wt% WO2.72 nanoparticles floating on water, and (C) evaporation efficiency for WO2.72/PLA fiber membrane containing 0, 4, and 7 wt% WO2.72 nanoparticles. Reproduced with permission (Chala et al., 2018a). Copyright 2018, ACS.
WOx photothermal materials have been investigated by (Ming et al., 2018) for direct steam generation. In their study, under 1 sun illumination, water and WOAr2 nanofluids reached 34.3°C and 41.0°C, respectively, after 1,800 s. The solar evaporation effciency of 2D defective WOx nanofluids reached 78.6% compared to that of the water (12.22%). WO2.72 and its hybrid recently gained interest for the study of water evaporation. It is worth noting that WO3 and MxWO3 (their hybrids) are still unknown in this field. Therefore, the development of hybrids of photothermal materials in full spectrum solar light has become important in terms of energy conservation and sustainability for photothermal water evaporation, desalination, and steam generation.
NIR Shielding
NIR-shielding materials have received considerable attention for their use in developing transparent solar heat-shielding filters, which can be used for solar control windows in automobiles and buildings. WO3−x and MxWO3, M = Na+ (Moon et al., 2013), K+ (Guo et al., 2011a), Rb+ (Guo et al., 2011a), Cs+ (Zeng et al., 2015), and (Huiyuan et al., 2018) are candidates for such applications. Among the many tungsten materials with a capability of shielding NIR light through absorption mechanism, cesium tungsten oxide (particularly Cs0.33WO3) nanoparticles are considered highly promising materials for transparent solar filter applications. Guo et al. developed WO2.72 nanorods coated on a quartz glass. Excellent heat-insulating performance was realized even after the WO2.72 nanorods on a quartz glass were irradiated for 1 h, when the inner temperature was increased to only 26.2 °C, which was much lower than the temperatures reached using the quartz glass and indium–tin oxide glass (Figure 5) (Guo et al., 2012c). CsxWO3/ZnO composite films were found to be highly efficient for heat insulation because of the excellent NIR shielding properties of CsxWO3 (Wu et al., 2015). These composite films also showed good capacity to block harmful UV lights. The hybrids of WO3 and MxWO3 for NIR shielding applications have been widely studied. However, to the best of our knowledge, WO2.72 and its hybrid have yet to be fully explored in this field.
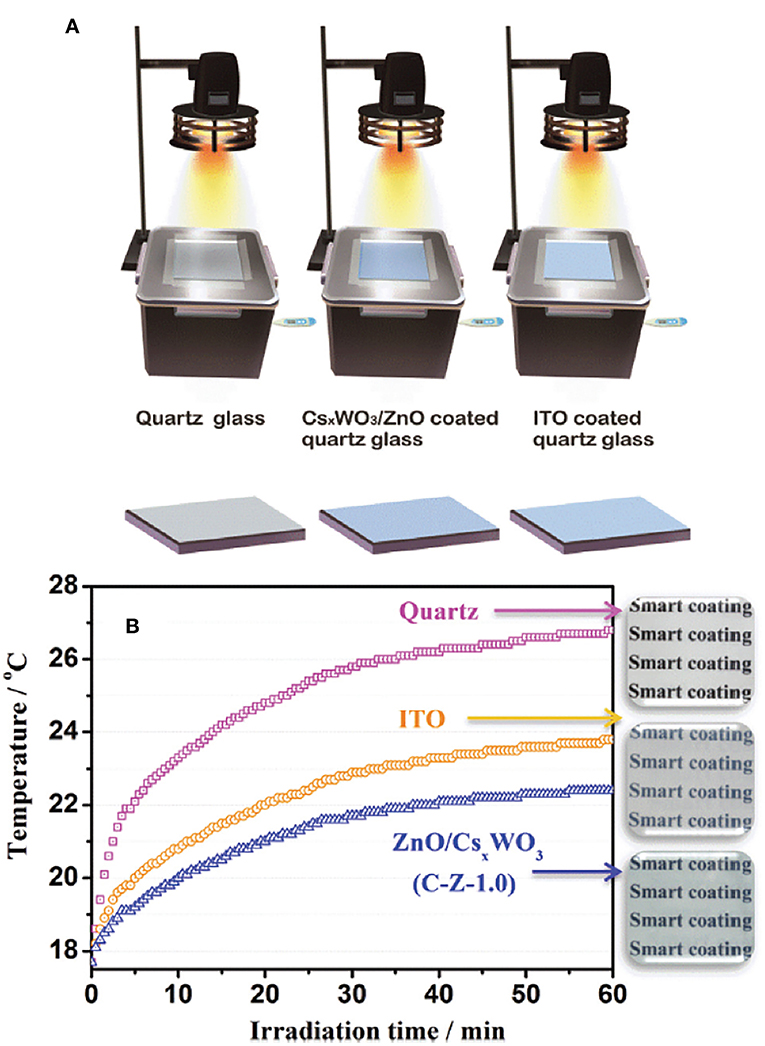
Figure 5. (A) Schematic illustration of the simulated heat ray shielding test using the boxes with facet covered by pure quartz glass, WO2.72 nanorods coated on quartz glass and ITO glass under the irradiation of a 50 W halogen lamp, and the temperature changes were determined by an electronic thermometer. (B) Time dependence of the temperature in the box. Reproduced with permission (Guo et al., 2012c) Copyright 2012, ACS.
Photocatalysts
Photochemical utilization of solar energy (i.e., photocatalytic degradation of organic pollutants, hydrogen production, photocatalytic reduction of CO2, and photocatalytic oxidation of alcohols) is being intensively studied (Wang et al., 2017c). Semiconductor materials such as for WO3−x and MxWO3 have been widely used in many fields of photocatalysis, including air purification, wastewater treatment, anti-virus sterilization, photolysis of hydrogen and oxygen production, nitrogen oxide fixation, and remediation of crude oil spills. Five photochemical uses of solar energy for WOx and MxWO3 materials are discussed as follows.
Water Oxidation
A water oxidation reaction acquires electrons from the earth's abundant water. An efficient water oxidation catalyst can provide the necessary electrons for proton reduction. However, in terms of effective utilization of solar light, the catalyst design concept requires light absorption characteristics in the visible light range. Water oxidation must meet one major thermodynamic criteria: the valence band (VB) level of a semiconductor should be more positive than the standard redox potential of H2O/O2 (1.23 eV). The VB potential of WO3−x is located at ca. 3.0 eV, and thus is a promising material for water oxidation application (Wang et al., 2012; Yan et al., 2015).
Reduction of CO2
Photocatalytic reduction of CO2 is also a catalyst that is excited in order to generate electrons and holes, which migrate to the surface of the catalyst. Molecules adsorbed on the surface of the catalyst trigger a series of chemical reactions and eventually produce various products such as CH4, HCOOH, HCHO, and CH3OH. However, the process of photoreduction of CO2 is complicated. In particular, the cleavage of C-O bonds and the formation of C-H bonds are complex processes. Xi reported that WO2.72 may also enable efficient reduction of CO2 to obtain CH4 (Xi et al., 2012).
Hydrogen Production
Photocatalysts may act as reducing and oxidizing agents. They may also decompose water molecules to produce H2 and O2. If photocatalysts are to be used to decompose water, their energy band gap (Eg) must be greater than 1.23 eV (<1,000 nm) and less than 3.0 eV (>400 nm) to respond in the visible region (Pihosh et al., 2015). In other words, the semiconductor photocatalyst must have a relatively small band gap (1.23 eV < Eg < 3.0 eV) to absorb as much light as possible for the purpose of photogenerated electrons/holes (Zhang et al., 2018). The results of hydrogen production by photolysis showed that the hydrogen production efficiency of g-C3N4 improved considerably after WO2.72 was incorporated. The WO2.72 (30 wt%)/g-C3N4 sample had the highest hydrogen production efficiency (3.69 μmol h−1), which was approximately 4.5 times that of pure g-C3N4 (Song et al., 2016).
Degradation of Organic Compounds
The conduction band of WO3 is slightly higher than the reduction potential of an H2/H2O reaction. In addition, its valence band is much higher than the oxidation potential of an H2O/O2 reaction, which enables the WO3 photocatalytic oxidation degradation of many organic compounds such as textile dyes and bacteria contaminants. In addition, WO3 offers strong stability in an acidic environment, making it promising for treating wastewater that contains organic acids. The oxygen vacancies in WO3−x are helpful for O2 reduction because of the electron transfer between mixed-valence states. WO2.72 exhibits a higher activity than WO3 in pollutant degradation, and is the major active species for the mineralization of pollutants (Bhuyan et al., 2017). Our research group recently reported on the outstanding photocatalytic activity of hybrid RbxWO3/Ag3VO4 degradation of methylene blue (MB) under a full spectrum (UV-VIS-NIR region). The photocatalytic performance was considerably enhanced because of the extended optical absorption in the entire UV-visible-NIR region, efficient separation of electron and hole pairs (e-/h+), and a synergetic effect between RbxWO3 and Ag3VO4 (Chala et al., 2018b).
Oxidation of Alcohols
The activated species in the photocatalytic reaction react with the alcohol, and the oxygen in the alcohol allows electrons to fill the holes in the valence band and finally obtain the corresponding aldehyde or ketone. The reaction has many advantages. For example, toxic and harmful metal salts do not need to be introduced as oxidants. In addition, it offers mild reaction conditions, minimal equipment requirements, a high conversion rate, and high selectivity. Thus, the photocatalytic oxidation of alcohols offers broad application prospects for the future. WO2.72 can be used for the photocatalytic dehydration of isopropanol to propylene (Bai et al., 2013). Three morphologies of WO2.72 including rods, urchins, and plates were studied. It was found that urchin-like products performed the best. WO3 and its hybrid have been widely explored for photocatalysis. Currently, hybrids of WO2.72- or MxWO3-based materials have received considerable attention for their use in developing solar absorbers capable of broadband absorption in the entire region. These materials must be further explored for photocatalysis.
Energy-Related Applications
WO3 and WO3−x have more efficient prospects for energy storage devices because of their multiple oxidation states, low price, high intrinsic density, high melting temperatures, and strong mechanical properties. These have been widely studied for supercapacitor electrodes, lithium ion batteries (LIB), and fuel cells. These are discussed in more detail as follows.
Supercapacitors
In the studies on supercapacitors, WO3 has been shown to suffer from low electrical conductivity because of the presence of oxides (Liu et al., 2018). Partial reduction of WO3−x enhanced the electrical conductivity because of multiple oxidation states (W+5 and W+6). It was found to have high capacity and faster performance than other tungsten oxides. Yoon et al. (2011b) synthesized mesoporous WO3−x (m-WO3−x) and found it had high rate capability and excellent capacitance of 366 μFcm−2 and 639 Fcm−3, respectively. Ordered mesoporous WO3−x showed high electrical conductivity of 1.76 S cm−1 and highly interconnected ordered pores with a large surface area, making them suitable for use in electrode materials of supercapacitors (Zhou et al., 2013). explored ordered mesoporous carbon/WO3−x nanocomposites. The results revealed excellent rate capability with high volumetric capacity (125 Fcm−1) (Tian et al., 2014). developed a novel smart supercapacitor electrode engraved with a pattern consisting of WO2.72 on a PANI background. Both WO2.72 and PANI displayed multiple color changes and they operated electrochemically with a widened potential window. The specific capacitance was found to be 302 Fg−1 at 10 Ag−1 current density, which might appear as a new feature of supercapacitors. Among the various , WO2.72 has attracted the most interest because it offers the highest oxygen deficiencies. Moreover, it is the most stable form and possesses good conductivity, making it a promising candidate for supercapacitors (Jung and Kim, 2018; Huang et al., 2019; Li et al., 2019a).
Lithium-Ion Batteries (LIB)
WO3 has been used as an anode material because it offers a high theoretical capacity, low cost, and environmental friendliness. The only major drawback is its low electrical conductivity, although this has been improved with WO3−x materials. Yoon et al. (2011a) focused on developing high performance anode mesoporous WO3−x using a hard template with high electrical conductivity. The developed material exhibited a high reversible capacity (748 mAh g−1) and a high volumetric capacity (1,500 mAh cm−3) compared to the bulk WO3−x. Lee et al. (2014a) reported that flexible reduced tungsten oxide–carbon composite nanofiber (WOx-C-NF) films used as anode materials in LIB exhibited a high reversible capacity (481 mA h g−1), stable cycle, and improved rate performance compared to WOx-C-nano and WOx-nano electrodes. These studies proved that WO3−x is one of the most promising anode materials for LIB. Recently, WO3−x composites as anode materials for high performance lithium-ion batteries were reported (Zhang et al., 2015b; Yue et al., 2017; Li et al., 2019b). Liu et al. (2017) reported that flower-like WO3/CoWO4/Co nanostructure electrodes could deliver discharge capacities of 2,435 and 1,074 mAhg−1 during the first cycle at current densities of 100 and 200 respectively. The pristine WO3 electrode without cobalt doping exhibited a capacity of 1,151 and 331 mAhg−1 at 100 and 200 respectively. The hybrids of WO3 (i.e., WO3/CoWO4/Co composites used as anode materials) have superior cycling performance as compared to that of WO3 particles.
Fuel Cells
H2 fuel has been investigated extensively. Improved catalytic activity and durability are highly desirable for this technology. Here, we focus on the use of WO3−x-based electrocatalysts in fuel cells. Lu et al. (2014) synthesized Pd tetrahedron–tungsten oxide nanosheet hybrids (Pd/WO2.72) that enhanced electrocatalytic activity and provided durability for fuel cells. As compared to Pd nanocrystal, Pd/WO2.72 hybrids demonstrated not only high activity but also superior stability for the oxygen reduction reaction (ORR) in alkaline solutions. The mass activity of Pd/WO2.72 at 0.90 V is 0.216 A mg −1, which is much higher than commercial Pt/C, Pd NPs, and Pd/C. Kang et al. (2010) prepared ordered mesoporous WO3−x by using a hard template and mesostructured WO3−x responsible for its high conductivity. Pt/mesoporous WO3−x exhibited a considerable tolerance to cycling between 0.6 and 1.3 VNHE. It could be used as an ORR catalyst support, thus offering long-term stability. In general, hybrids of WO3 have been explored widely for energy-related devices in the past few decades. Hybrids of WO2.72 in energy applications have also gained attention recently, but MxWO3 remain unnoticed.
Gas Sensors
WOx has oxygen defects in its crystal lattice, which cause the band to bend and enable conductivity. When the material is in contact with oxygen, oxygen absorbs electrons from the surface of the semiconductor to form negative ions, and the surface energy band is bent upward. This results in a decrease in surface electron concentration of the gas sensing material, a decrease in electrical conductivity, and an increase in resistance of the sensor. However, if the gas sensitive material is in contact with the reducing gas, desorption occurs, the surface energy band is lowered, both the electron concentration and electrical conductivity increase, and the resistance value of the sensor decreases.
WO3 and WO2.72 were reported as sensor materials to monitor flammable and toxic gases such as NH3 (Kim et al., 2005), NOx (Qin et al., 2011a), H2 (Boudiba et al., 2010), H2S (Rout et al., 2008), and SOx (Godbole et al., 2017). WOx can also reduce gases such as H2, CH4, COx (Stankova et al., 2005), and C2H5OH (Vallejos et al., 2015). Although WO2.72 has the largest oxygen deficiency, it has greater potential in this field (Wang et al., 2018). prepared WO3 nanorod/sulfonated reduced graphene oxides (WO3/S-rGO), which showed fast response recovery characteristics at all concentrations of NO2 gas, indicating its good response and recovery properties for sensor applications. However, the gas sensor of the WOx still has the disadvantages of poor stability, low selectivity, and a high operating temperature. Therefore, how to reduce the working temperature and improve the selectivity and sensitivity of the detection gas have become the focus of our current research. Composite and metal-hybrid doping have proven effective at improving the sensitivity of WOx for reducing gases. WO3 and its hybrid are widely explored for gas sensors and the properties of WO2.72 have recently gained attention. However, MxWO3 has yet to be fully studied.
Conclusion and Future Prospects
In this study, we summarized the comprehensive progress made in the last few years in the application of tungsten-oxide-based materials. WO3−x, MxWO3, and their hybrid materials as interesting research topics, particularly for morphology control and composite construction to enhance optical absorption, charge separation, redox capability, and electrical conductivity. The well-studied liquid-phase technique known as the solvothermal treatment is the most used method alongside the hydrothermal treatment, which is a facile and cost-effective method that can produce WOx with different nanomorphologies. The morphology can be fine-tuned by controlling variables such as time, precursor concentration, and temperature. A critical challenge is to enhance the utilization efficiency by extending the solar spectrum response from the UV to the NIR region. To meet these requirements, hybrids of WO2.72 and MxWO3 have become important because of their strong photoabsorption ability and intervalence charge properties. A major advantage of this material is the LSPR effect, which may encourage researchers to focus not only on the interesting properties for new applications but also to investigate the many opportunities it offers to improve the efficiency of current applications.
Data Availability
All datasets generated for this study are included in the manuscript and/or the supplementary files.
Author Contributions
C-MW performed initial literature search to identify papers, provided relevant information for the text, reviewed final manuscript, and conceptualization. SN contributed to writing the manuscript. M-HC, J-HW, and Y-QJ performed literature search and provided relevant information. All authors approved the final version to be published and agreed to be accountable for all aspects of the work.
Conflict of Interest Statement
The authors declare that the research was conducted in the absence of any commercial or financial relationships that could be construed as a potential conflict of interest.
Acknowledgments
The authors are thankful for the partial financial support received from the Ministry of Science and Technology of Taiwan, ROC, under contract numbers: MOST 107-2221-E-011-044.
References
Aoki, T., Matsushita, T., Suzuki, A., Tanabe, K., and Okuda, M. (2005). Optical recording characteristics of WO3 films grown by pulsed laser deposition method. J. Vacuum Sci. Technol. A 23, 1325–1330. doi: 10.1116/1.1978891
Awad, F. S., Kiriarachchi, H. D., Abouzeid, K. M., özgürü, and El-Shall, M. S. (2018). Plasmonic graphene polyurethane nanocomposites for efficient solar water desalination. ACS Appl. Energy Mater. 1, 976–985. doi: 10.1021/acsaem.8b00109
Baek, Y., and Yong, K. (2007). Controlled growth and characterization of tungsten oxide nanowires using thermal evaporation of WO3 powder. J. Phys. Chem. C 111, 1213–1218. doi: 10.1021/jp0659857
Bai, H., Su, N., Li, W., Zhang, X., Yan, Y., Li, P., et al. (2013). W18O49 nanowire networks for catalyzed dehydration of isopropyl alcohol to propylene under visible light. J. Mater. Chem. A 1, 6125–6129. doi: 10.1039/c3ta10835j
Bhuyan, B., Paul, B., Dhar, S. S., and Vadivel, S. (2017). Facile hydrothermal synthesis of ultrasmall W18O49 nanoparticles and studies of their photocatalytic activity towards degradation of methylene blue. Mater. Chem. Phys. 188, 1–7. doi: 10.1016/j.matchemphys.2016.12.035
Boudiba, A., Zhang, C., Navio, C., Bittencourt, C., Snyders, R., and Debliquy, M. (2010). Preparation of highly selective, sensitive and stable hydrogen sensors based on Pd-doped tungsten trioxide. Proc. Eng. 5, 180–183. doi: 10.1016/j.proeng.2010.09.077
Chala, T. F., Wu, C. -M., Chou, M. -H., Gebeyehu, M. B., and Cheng, K. -B. (2017). Highly efficient near infrared photothermal conversion properties of reduced tungsten oxide/polyurethane nanocomposites. Nanomaterials 7:91. doi: 10.3390/nano7070191
Chala, T. F., Wu, C. -M., Chou, M. -H., and Guo, Z. -L. (2018a). Melt electrospun reduced tungsten oxide /polylactic acid fiber membranes as a photothermal material for light-driven interfacial water evaporation. ACS Appl. Mater. Interfaces 10, 28955–28962. doi: 10.1021/acsami.8b07434
Chala, T. F., Wu, C. -M., and Motora, K. G. (2018b). RbxWO3/Ag3VO4 Nanocomposites Towards Efficient Full-Spectrum (UV, Visible, and Near Infrared) Photocatalysis (Taiwan Institute of Chemical Engineers).
Chen, C. -J., and Chen, D. -H. (2013). Preparation and near-infrared photothermal conversion property of cesium tungsten oxide nanoparticles. Nanoscale Res. Lett. 8, 57. doi: 10.1186/1556-276X-8-57
Chen, J., Wang, D., Xi, J., Au, L., Siekkinen, A., Warsen, A., et al. (2007). Immuno gold nanocages with tailored optical properties for targeted photothermal destruction of cancer cells. Nano Lett. 7, 1318–1322. doi: 10.1021/nl070345g
Chen, Q., Pei, Z., Xu, Y., Li, Z., Yang, Y., Wei, Y., et al. (2018). A durable monolithic polymer foam for efficient solar steam generation. Chem. Sci. 9, 623–628. doi: 10.1039/C7SC02967E
Chen, Z., Wang, Q., Wang, H., Zhang, L., Song, G., Song, L., et al. (2013). Ultrathin PEGylated W18O49 nanowires as a new 980 nm-laser-driven photothermal agent for efficient ablation of cancer cells in vivo. Adv. Mater. 25, 2095–2100. doi: 10.1002/adma.201204616
Ding, D., Huang, W., Song, C., Yan, M., Guo, C., and Liu, S. (2017). Non-stoichiometric MoO3−x quantum dots as a light-harvesting material for interfacial water evaporation. Chem. Commun. 53, 6744–6747. doi: 10.1039/C7CC01427A
Godbole, R., Godbole, V. P., and Bhagwat, S. (2017). Surface morphology dependent tungsten oxide thin films as toxic gas sensor. Mater. Sci. Semicond. Process. 63, 212–219. doi: 10.1016/j.mssp.2017.02.023
Guo, C., Yin, S., Dong, Q., and Sato, T. (2012a). Simple route to (NH4)xWO3 nanorods for near infrared absorption. Nanoscale 4, 3394–3398. doi: 10.1039/c2nr30612c
Guo, C., Yin, S., Huang, L., and Sato, T. (2011a). Synthesis of one-dimensional potassium tungsten bronze with excellent near-infrared absorption property. ACS Appl. Mater. Interfaces 3, 2794–2799. doi: 10.1021/am200631e
Guo, C., Yin, S., Huang, Y., Dong, Q., and Sato, T. (2011b). Synthesis of W18O49 nanorod via ammonium tungsten oxide and its interesting optical properties. Langmuir 27, 12172–12178. doi: 10.1021/la202513q
Guo, C., Yin, S., and Sato, T. (2012b). Tungsten oxide-based nanomaterials: morphological-control, properties, and novel applications. Rev. Adv. Sci. Eng. 1, 235–263. doi: 10.1166/rase.2012.1016
Guo, C., Yin, S., Yan, M., Kobayashi, M., Kakihana, M., and Sato, T. (2012c). Morphology-controlled synthesis of W18O49 nanostructures and their near-infrared absorption properties. Inorg. Chem. 51, 4763–4771. doi: 10.1021/ic300049j
Guo, C., Yin, S., Zhang, P., Yan, M., Adachi, K., Chonan, T., et al. (2010). Novel synthesis of homogenous CsxWO3 nanorods with excellent NIR shielding properties by a water controlled-release solvothermal process. J. Mater. Chem. 20, 8227–8229. doi: 10.1039/c0jm01972k
Guo, X., Qin, X., Xue, Z., Zhang, C., Sun, X., Hou, J., et al. (2016). Morphology-controlled synthesis of WO2.72 nanostructures and their photocatalytic properties. RSC Adv. 6, 48537–48542. doi: 10.1039/C6RA08551B
Hashishin, T., and Tamaki, J. (2008). Conductivity-type sensor based on CNT-WO3 composite for NO2 detection. J. Nanomater. 2008:31. doi: 10.1155/2008/352854
Hu, X., Xu, W., Zhou, L., Tan, Y., Wang, Y., Zhu, S., et al. (2017). Tailoring graphene oxide-based aerogels for efficient solar steam generation under one sun. Adv. Mater. 29:1604031. doi: 10.1002/adma.201604031
Hu, X. -S., Shen, Y., Xu, L. -H., Wang, L. -M., Lu, L. -S., and Zhang, Y. -T. (2016). Preparation of flower-like CuS by solvothermal method for photocatalytic, UV protection and EMI shielding applications. Appl. Surf. Sci. 385, 162–170. doi: 10.1016/j.apsusc.2016.05.089
Hua, Z., Li, B., Li, L., Yin, X., Chen, K., and Wang, W. (2017). Designing a novel photothermal material of hierarchical microstructured copper phosphate for solar evaporation enhancement. J. Phys. Chem. C 121, 60–69. doi: 10.1021/acs.jpcc.6b08975
Huang, S., He, Q., Xu, S., and Wang, L. (2015a). Polyaniline-based photothermal paper sensor for sensitive and selective detection of 2,4,6-trinitrotoluene. Anal. Chem. 87, 5451–5456. doi: 10.1021/acs.analchem.5b01078
Huang, W., and El-Sayed, M. A. (2008). Photothermally excited coherent lattice phonon oscillations in plasmonic nanoparticles. Eur. Phys. J.Spec. Top. 153, 325–333. doi: 10.1140/epjst/e2008-00456-x
Huang, X., and El-Sayed, M. A. (2010). Gold nanoparticles: optical properties and implementations in cancer diagnosis and photothermal therapy. J. Adv. Res. 1, 13–28. doi: 10.1016/j.jare.2010.02.002
Huang, X., Zhang, Z., Li, H., Wang, H., and Ma, T. (2019). In-situ growth of nanowire WO2.72 on carbon cloth as a binder-free electrode for flexible asymmetric supercapacitors with high performance. J. Energy Chem. 29, 58–64. doi: 10.1016/j.jechem.2018.01.024
Huang, Z. -F., Song, J., Pan, L., Jia, X., Li, Z., Zou, J. -J., et al. (2014a). W18O49 nanowire alignments with a BiOCl shell as an efficient photocatalyst. Nanoscale 6, 8865–8872. doi: 10.1039/C4NR00905C
Huang, Z. -F., Song, J., Pan, L., Lv, F., Wang, Q., Zou, J. -J., et al. (2014b). Mesoporous W18O49 hollow spheres as highly active photocatalysts. Chem. Commun. 50, 10959–10962. doi: 10.1039/C4CC02201G
Huang, Z. -F., Song, J., Pan, L., Zhang, X., Wang, L., and Zou, J. -J. (2015b). Tungsten oxides for photocatalysis, electrochemistry, and phototherapy. Adv. Mater. 27, 5309–5327. doi: 10.1002/adma.201501217
Huiyuan, L., Hua, L., Yujie, C., and Hezhou, L. (2018). A facile hydrothermal method to synthesize ammonium tungsten bronze nanoplatelets for nir absorption. IOP Conf. Series Mater. Sci. Eng. 382:022062. doi: 10.1088/1757-899X/382/2/022062
Hussain, A., Gruehn, R., and Rüscher, C. H. (1997). Crystal growth of alkali metal tungsten brozes MxWO3 (M = K, Rb, Cs), and their optical properties. J. Alloys Compd. 246, 51–61. doi: 10.1016/S0925-8388(96)02470-X
Jiang, Q., Gholami Derami, H., Ghim, D., Cao, S., Jun, Y. -S., and Singamaneni, S. (2017). Polydopamine-filled bacterial nanocellulose as a biodegradable interfacial photothermal evaporator for highly efficient solar steam generation. J. Mater. Chem. A 5, 18397–18402. doi: 10.1039/C7TA04834C
Jin, Y. Z., Zhu, Y. Q., Whitby, R. L., Yao, N., Ma, R., Watts, P. C., et al. (2004). Simple approaches to quality large-scale tungsten oxide nanoneedles. J. Phys. Chem. B 108, 15572–15577. doi: 10.1021/jp048596q
Jung, J., and Kim, D. H. (2018). W18O49 nanowires assembled on carbon felt for application to supercapacitors. Appl. Surf. Sci. 433, 750–755. doi: 10.1016/j.apsusc.2017.10.109
Kang, E., An, S., Yoon, S., Kim, J. K., and Lee, J. (2010). Ordered mesoporous WO3−x possessing electronically conductive framework comparable to carbon framework toward long-term stable cathode supports for fuel cells. J. Mater. Chem. 20, 7416–7421. doi: 10.1039/c0jm00227e
Kim, J. Y., Jeong, S. Y., Shin, G. J., Lee, S. K., and Choi, K. H. (2012). Near infrared cut-off characteristics of various perovskite-based composite films. Appl. Mech. Mater. 229–231, 2733–2736. doi: 10.4028/www.scientific.net/AMM.229-231.2733
Kim, K., Yu, S., An, C., Kim, S. -W., and Jang, J. -H. (2018). Mesoporous three-dimensional graphene networks for highly efficient solar desalination under 1 sun illumination. ACS Appl. Mater. Interfaces 10, 15602–15608. doi: 10.1021/acsami.7b19584
Kim, Y. S., Ha, S. -C., Kim, K., Yang, H., Choi, S. -Y., Kim, Y. T., et al. (2005). Room-temperature semiconductor gas sensor based on non-stoichiometric tungsten oxide nanorod film. Appl. Phys. Lett. 86:213105. doi: 10.1063/1.1929872
Late, D. J., Kashid, R. V., Sekhar rout, C., More, M. A., and Joag, D. S. (2010). Low threshold field electron emission from solvothermally synthesized WO2.72 nanowires. Appl. Phys. A 98, 751–756. doi: 10.1007/s00339-009-5536-0
Lee, J., Jo, C., Park, B., Hwang, W., Lee, H. I., Yoon, S., et al. (2014a). Simple fabrication of flexible electrodes with high metal-oxide content: electrospun reduced tungsten oxide/carbon nanofibers for lithium ion battery applications. Nanoscale 6, 10147–10155. doi: 10.1039/C4NR01033G
Lee, K., Seo, W. S., and Park, J. T. (2003). Synthesis and Optical properties of colloidal tungsten oxide nanorods. J. Am. Chem. Soc. 125, 3408–3409. doi: 10.1021/ja034011e
Lee, S. Y., Kim, J. Y., Lee, J. Y., Song, H. J., Lee, S., Choi, K. H., et al. (2014b). Facile fabrication of high-efficiency near-infrared absorption film with tungsten bronze nanoparticle dense layer. Nanoscale Res. Lett. 9:294. doi: 10.1186/1556-276X-9-294
Li, B., Shao, X., Liu, T., Shao, L., and Zhang, B. (2016a). Construction of metal/WO2.72/rGO ternary nanocomposites with optimized adsorption, photocatalytic and photoelectrochemical properties. Appl. Catal. B Environ. 198, 325–333. doi: 10.1016/j.apcatb.2016.06.001
Li, G., Gao, L., Li, L., and Guo, L. (2019a). An electrochromic and self-healing multi-functional supercapacitor based on PANI/nw-WO2.7/Au NPs electrode and hydrogel electrolyte. J. Alloys Comp. 786, 40–49. doi: 10.1016/j.jallcom.2018.12.142
Li, G., Wu, G., Guo, C., and Wang, B. (2016b). Fabrication of one-dimensional W18O49 nanomaterial for the near infrared shielding. Mater. Lett. 169, 227–230. doi: 10.1016/j.matlet.2016.01.094
Li, G., Zhang, S., Guo, C., and Liu, S. (2016c). Absorption and electrochromic modulation of near-infrared light: realized by tungsten suboxide. Nanoscale 8, 9861–9868. doi: 10.1039/C5NR09147K
Li, Y., Chang, K., Tang, H., Li, B., Qin, Y., Hou, Y., et al. (2019b). Preparation of oxygen-deficient WO3−x nanosheets and their characterization as anode materials for high-performance Li-ion batteries. Electrochim. Acta 298, 640–649. doi: 10.1016/j.electacta.2018.12.137
Liao, C. -C., Chen, F. -R., and Kai, J. -J. (2007). Electrochromic properties of nanocomposite WO3 films. Solar Energy Mater. Solar Cells 91, 1282–1288. doi: 10.1016/j.solmat.2006.11.020
Lin, H., Wang, X., Yu, L., Chen, Y., and Shi, J. (2017). Two-dimensional ultrathin Mxene ceramic nanosheets for photothermal conversion. Nano Lett. 17, 384–391. doi: 10.1021/acs.nanolett.6b04339
Liu, G., Wang, S., Nie, Y., Sun, X., Zhang, Y., and Tang, Y. (2013a). Electrostatic-induced synthesis of tungsten bronze nanostructures with excellent photo-to-thermal conversion behavior. J. Mater. Chem. A 1, 10120–10129. doi: 10.1039/c3ta11479a
Liu, J., Zhang, Z., Wang, Z., Tang, M., Li, J., Yi, J., et al. (2017). Flower-like WO3/CoWO4/Co nanostructures as high performance anode for lithium ion batteries. J. Alloys Compd. 727, 107–113. doi: 10.1016/j.jallcom.2017.08.057
Liu, J. -X., Ando, Y., Dong, X. -L., Shi, F., Yin, S., Adachi, K., et al. (2010). Microstructure and electrical–optical properties of cesium tungsten oxides synthesized by solvothermal reaction followed by ammonia annealing. J. Solid State Chem. 183, 2456–2460. doi: 10.1016/j.jssc.2010.08.017
Liu, X., He, Y., Wang, S., Zhang, Q., and Song, M. (2012). Controllable synthesis and tunable field-emission properties of tungsten oxide sub-micro fibers. Int. J. Refract. Metals Hard Mater. 34, 47–52. doi: 10.1016/j.ijrmhm.2012.04.002
Liu, X., Sheng, G., Zhong, M., and Zhou, X. (2018). Dispersed and size-selected WO3 nanoparticles in carbon aerogel for supercapacitor applications. Mater. Des. 141, 220–229. doi: 10.1016/j.matdes.2017.12.038
Liu, X., Song, M., Wang, S., and He, Y. (2013b). Structure and field-emission properties of W/WO2.72 heterostructures fabricated by vapor deposition. Phys. E 53, 260–265. doi: 10.1016/j.physe.2013.05.017
Liu, Y., Chen, J., Guo, D., Cao, M., and Jiang, L. (2015). Floatable, self-cleaning, and carbon-black-based superhydrophobic gauze for the solar evaporation enhancement at the air–water interface. ACS Appl. Mater. Interfaces 7, 13645–13652. doi: 10.1021/acsami.5b03435
Lou, J., Liu, Y., Wang, Z., Zhao, D., Song, C., Wu, J., et al. (2016). Bioinspired multifunctional paper-based rGO composites for solar-driven clean water generation. ACS Appl. Mater. Interfaces 8, 14628–14636. doi: 10.1021/acsami.6b04606
Lou, X. W., and Zeng, H. C. (2003). An inorganic route for controlled synthesis of W18O49 nanorods and nanofibers in solution. Inorg. Chem. 42, 6169–6171. doi: 10.1021/ic034771q
Lu, Y., Jiang, Y., Gao, X., Wang, X., and Chen, W. (2014). Strongly coupled Pd nanotetrahedron/tungsten oxide nanosheet hybrids with enhanced catalytic activity and stability as oxygen reduction electrocatalysts. J. Am. Chem. Soc. 136, 11687–11697. doi: 10.1021/ja5041094
Ma, B., Huang, E., Wu, G., Dai, W., Guan, N., and Li, L. (2017). Fabrication of WO2.72/RGO nano-composites for enhanced photocatalysis. RSC Adv. 7, 2606–2614. doi: 10.1039/C6RA26416F
Mamak, M., Choi, S. Y., Stadler, U., Dolbec, R., Boulos, M., and Petrov, S. (2010). Thermal plasma synthesis of tungsten bronze nanoparticles for near infra-red absorption applications. J. Mater. Chem. 20, 9855–9857. doi: 10.1039/c0jm02169e
Marques, M. P. M. (2013). Platinum and palladium polyamine complexes as anticancer agents: the structural factor. ISRN Spectrosc. 2013, 1–29. doi: 10.1155/2013/287353
Ming, X., Guo, A., Wang, G., and Wang, X. (2018). Two-dimensional defective tungsten oxide nanosheets as high performance photo-absorbers for efficient solar steam generation. Solar Energy Mater. Solar Cells 185, 333–341. doi: 10.1016/j.solmat.2018.05.049
Moon, K., Cho, J. -J., Lee, Y. -B., Yoo, P. J., Bark, C. W., and Park, J. (2013). Near infrared shielding properties of quaternary tungsten bronze nanoparticle Na0.11Cs0.22WO3. Bull. Korean Chem. Soc. 34, 731–734. doi: 10.5012/bkcs.2013.34.3.731
Moshofsky, B., and Mokari, T. (2012). Length and diameter control of ultrathin nanowires of substoichiometric tungsten oxide with insights into the growth mechanism. Chem. Mater. 25, 1384–1391. doi: 10.1021/cm302015z
Okusako, T., Tokuyama, T., Mikami, K., Nogami, Y., and Hanasaki, N. (2012). Thermoelectric effect in hexagonal tungsten oxides. J. Phys. Soc. Japan 81:SB028. doi: 10.1143/JPSJS.81SB.SB028
Pihosh, Y., Turkevych, I., Mawatari, K., Uemura, J., Kazoe, Y., Kosar, S., et al. (2015). Photocatalytic generation of hydrogen by core-shell WO3/BiVO4 nanorods with ultimate water splitting efficiency. Sci. Rep. 5:11141. doi: 10.1038/srep11141
Qin, Y., Li, X., Wang, F., and Hu, M. (2011a). Solvothermally synthesized tungsten oxide nanowires/nanorods for NO2 gas sensor applications. J. Alloys Compd. 509, 8401–8406. doi: 10.1016/j.jallcom.2011.05.100
Qin, Y., Shen, W., Li, X., and Hu, M. (2011b). Effect of annealing on microstructure and NO2-sensing properties of tungsten oxide nanowires synthesized by solvothermal method. Sens. Actuat. B Chem. 155, 646–652. doi: 10.1016/j.snb.2011.01.024
Reich, S., Leitus, G., Popovitz-Biro, R., Goldbourt, A., and Vega, S. (2009). A Possible 2D HxWO3 Superconductor with a T c of 120 K. J. Superconduct. Novel Magnet. 22, 343–346. doi: 10.1007/s10948-009-0443-3
Riley, R. S., and Day, E. S. (2017). Gold nanoparticle-mediated photothermal therapy: applications and opportunities for multimodal cancer treatment. Wiley Interdiscipl. Rev. Nanomed. Nanobiotechnol. 9:e1449. doi: 10.1002/wnan.1449
Rout, C. S., Hegde, M., and Rao, C. N. R. (2008). H2S sensors based on tungsten oxide nanostructures. Sens. Actuat. B Chem. 128, 488–493. doi: 10.1016/j.snb.2007.07.013
Schmidt, P., Binnewies, M., Glaum, R., and Schmidt, M. (2013). “Chemical vapor transport reactions–methods, materials, modeling,” in Advanced Topics on Crystal Growth (London: InTech), 227–305.
Shang, M., Li, N., Zhang, S., Zhao, T., Zhang, C., Liu, C., et al. (2017). Full-spectrum solar-to-heat conversion membrane with interfacial plasmonic heating ability for high-efficiency desalination of seawater. ACS Appl. Energy Mater. 1, 56–61. doi: 10.1021/acsaem.7b00135
Son, K. H., Hong, J. H., and Lee, J. W. (2016). Carbon nanotubes as cancer therapeutic carriers and mediators. Int. J. Nanomed. 11, 5163–5185. doi: 10.2147/IJN.S112660
Song, C., Li, T., Guo, W., Gao, Y., Yang, C., Zhang, Q., et al. (2018). Hydrophobic Cu12Sb4S13-deposited photothermal film for interfacial water evaporation and thermal antibacterial activity. New J. Chem. 42, 3175–3179. doi: 10.1039/C7NJ04545J
Song, G., Han, L., Zou, W., Xiao, Z., Huang, X., Qin, Z., et al. (2014). A novel photothermal nanocrystals of Cu7S4 hollow structure for efficient ablation of cancer cells. Nano Micro Lett. 6, 169–177. doi: 10.1007/BF03353781
Song, G., Wang, Q., Wang, Y., Lv, G., Li, C., Zou, R., et al. (2013). A low-toxic multifunctional nanoplatform based on Cu9S5@mSiO2 core-shell nanocomposites: combining photothermal- and chemotherapies with infrared thermal imaging for cancer treatment. Adv. Funct. Mater. 23, 4281–4292. doi: 10.1002/adfm.201203317
Song, K., Xiao, F., Zhang, L., Yue, F., Liang, X., Wang, J., et al. (2016). W18O49 nanowires grown on g-C3N4 sheets with enhanced photocatalytic hydrogen evolution activity under visible light. J. Mol. Catal. A Chem. 418, 95–102. doi: 10.1016/j.molcata.2016.03.029
Stankova, M., Vilanova, X., Llobet, E., Calderer, J., Bittencourt, C., Pireaux, J., et al. (2005). Influence of the annealing and operating temperatures on the gas-sensing properties of rf sputtered WO3 thin-film sensors. Sens. Actuat. B Chem. 105, 271–277. doi: 10.1016/j.snb.2004.06.009
Su, C. -Y., and Lin, H. -C. (2009). Direct route to tungsten oxide nanorod bundles: microstructures and electro-optical properties. J. Phys. Chem. C 113, 4042–4046. doi: 10.1021/jp809458j
Sun, L., Li, Z., Su, R., Wang, Y., Li, Z., Du, B., et al. (2018). Phase-Transition induced conversion into a photothermal material: quasi-metallic WO2.9 Nanorods for solar water evaporation and anticancer photothermal therapy. Angew. Chem. Int. Ed. 57, 10666–10671. doi: 10.1002/anie.201806611
Sun, Y., Wang, W., Qin, J., Zhao, D., Mao, B., Xiao, Y., et al. (2016). Oxygen vacancy-rich mesoporous W18O49 nanobelts with ultrahigh initial Coulombic efficiency toward high-performance lithium storage. Electrochim. Acta 187, 329–339. doi: 10.1016/j.electacta.2015.11.064
Takeda, H., and Adachi, K. (2007). Near infrared absorption of tungsten oxide nanoparticle dispersions. J. Am. Ceram. Soc. 90, 4059–4061. doi: 10.1111/j.1551-2916.2007.02065.x
Tian, Q., Tang, M., Sun, Y., Zou, R., Chen, Z., Zhu, M., et al. (2011). Hydrophilic flower-like CuS superstructures as an efficient 980 nm laser-driven photothermal agent for ablation of cancer cells. Adv. Mater. 23, 3542–3547. doi: 10.1002/adma.201101295
Tian, Y., Cong, S., Su, W., Chen, H., Li, Q., Geng, F., et al. (2014). Synergy of W18O49 and polyaniline for smart supercapacitor electrode integrated with energy level indicating functionality. Nano Lett. 14, 2150–2156. doi: 10.1021/nl5004448
Vallejos, S., Gràcia, I., Bravo, J., Figueras, E., Hubálek, J., and Cané, C. (2015). Detection of volatile organic compounds using flexible gas sensing devices based on tungsten oxide nanostructures functionalized with Au and Pt nanoparticles. Talanta 139, 27–34. doi: 10.1016/j.talanta.2015.02.034
Venables, D. S., and Brown, M. E. (1996). Reduction of tungsten oxides with carbon. part 1: thermal analyses. Thermochim. Acta 282, 251–264. doi: 10.1016/0040-6031(95)02814-5
Wang, G., Ling, Y., Wang, H., Yang, X., Wang, C., Zhang, J. Z., et al. (2012). Hydrogen-treated WO3 nanoflakes show enhanced photostability. Energy Environ. Sci. 5, 6180–6187. doi: 10.1039/c2ee03158b
Wang, H. -Q., Hu, P. -F., Zheng, Y., Zhao, Z., Zheng, B., Chang, J., et al. (2017a). Construction of ICG encapsulated W18O49@MSN as a fluorescence carrier for real-time tracked photothermal therapy. Mater. Sci. Eng. C 80, 102–109. doi: 10.1016/j.msec.2017.05.131
Wang, J., Li, Y., Deng, L., Wei, N., Weng, Y., Dong, S., et al. (2017b). High-performance photothermal conversion of narrow-bandgap Ti2O3 nanoparticles. Adv. Mater. 29:1603730. doi: 10.1002/adma.201603730
Wang, J., Liu, G., and Du, Y. (2003). Mechanochemical synthesis of sodium tungsten bronze nanocrystalline powders. Mater. Lett. 57, 3648–3652. doi: 10.1016/S0167-577X(03)00142-3
Wang, P. (2018). Emerging investigator series: the rise of nano-enabled photothermal materials for water evaporation and clean water production by sunlight. Environ. Sci. Nano 5, 1078–1089. doi: 10.1039/C8EN00156A
Wang, S., He, Y., Zou, J., Cao, P., Jiang, Y., Huang, B., et al. (2007). Synthesis of tungsten oxide tapered needles with nanotips. J. Cryst. Growth 303, 574–579. doi: 10.1016/j.jcrysgro.2006.11.342
Wang, T., Hao, J., Zheng, S., Sun, Q., Zhang, D., and Wang, Y. (2018). Highly sensitive and rapidly responding room-temperature NO2 gas sensors based on WO3 nanorods/sulfonated graphene nanocomposites. Nano Res. 11, 791–803. doi: 10.1007/s12274-017-1688-y
Wang, X., Ou, G., Wang, N., and Wu, H. (2016a). Graphene-based recyclable photo-absorbers for high-efficiency seawater desalination. ACS Appl. Mater. Interfaces 8, 9194–9199. doi: 10.1021/acsami.6b02071
Wang, X., Wang, F., Sang, Y., and Liu, H. (2017c). Full-spectrum solar-light-activated photocatalysts for light–chemical energy conversion. Adv. Energy Mater. 7:1700473. doi: 10.1002/aenm.201700473
Wang, Y., Zhang, L., and Wang, P. (2016b). Self-floating carbon nanotube membrane on macroporous silica substrate for highly efficient solar-driven interfacial water evaporation. ACS Sustain. Chem. Eng. 4, 1223–1230. doi: 10.1021/acssuschemeng.5b01274
Wang, Z., Ye, Q., Liang, X., Xu, J., Chang, C., Song, C., et al. (2017d). based membranes on silicone floaters for efficient and fast solar-driven interfacial evaporation under one sun. J. Mater. Chem. A 5, 16359–16368. doi: 10.1039/C7TA03262E
Wu, C. -M., Chou, M. -H., and Chala, T. F. (2017a). “Pyroelectricity of electrospun polyvinylidene fluoride/tungsten oxide nanofibrous membranes,” in 22nd International Conference on Advanced Materials and Nanotechnology (Osaka, Japan).
Wu, X., Wang, J., Zhang, G., Katsumata, K. -I., Yanagisawa, K., Sato, T., et al. (2017b). Series of MxWO3/ZnO (M= K, Rb, NH4) nanocomposites: combination of energy saving and environmental decontamination functions. Appl. Catal. B Environ. 201, 128–136. doi: 10.1016/j.apcatb.2016.08.030
Wu, X., Yin, S., Xue, D., Komarneni, S., and Sato, T. (2015). A CsxWO3/ZnO nanocomposite as a smart coating for photocatalytic environmental cleanup and heat insulation. Nanoscale 7, 17048–17054. doi: 10.1039/C5NR04452A
Xi, G., Ouyang, S., Li, P., Ye, J., Ma, Q., Su, N., et al. (2012). Ultrathin W18O49 nanowires with diameters below 1 nm: synthesis, near-infrared absorption, photoluminescence, and photochemical reduction of carbon dioxide. Angew. Chem. 124, 2445–2449. doi: 10.1002/ange.201107681
Xu, X. -Q., Wang, Z., Li, R., He, Y., and Wang, Y. (2018). A degradable and recyclable photothermal conversion polymer. Chem. A Eur. J. 24, 9769–9772. doi: 10.1002/chem.201801654
Yan, C., Tian, Q., and Yang, S. (2017). Recent advances in the rational design of copper chalcogenide to enhance the photothermal conversion efficiency for the photothermal ablation of cancer cells. RSC Adv. 7, 37887–37897. doi: 10.1039/C7RA05468H
Yan, J., Liu, P., Ma, C., Lin, Z., and Yang, G. (2016). Plasmonic near-touching titanium oxide nanoparticles to realize solar energy harvesting and effective local heating. Nanoscale 8, 8826–8838. doi: 10.1039/C6NR01295G
Yan, J., Wang, T., Wu, G., Dai, W., Guan, N., Li, L., et al. (2015). Tungsten oxide single crystal nanosheets for enhanced multichannel solar light harvesting. Adv. Mater. 27, 1580–1586. doi: 10.1002/adma.201404792
Yang, K., Zhang, S., Zhang, G., Sun, X., Lee, S. -T., and Liu, Z. (2010). Graphene in mice: ultrahigh in vivo tumor uptake and efficient photothermal therapy. Nano Lett. 10, 3318–3323. doi: 10.1021/nl100996u
Yang, X. -G., Li, C., Mo, M. -S., Zhan, J. -H., Yu, W. -C., Yan, Y., et al. (2003). Growth of K0.4WO3 whiskers via a pressure-relief-assisted hydrothermal process. J. Cryst. Growth 249, 594–599. doi: 10.1016/S0022-0248(02)02323-0
Yao, Y., Zhang, L., Chen, Z., Cao, C., Gao, Y., and Luo, H. (2018). Synthesis of CsxWO3 nanoparticles and their NIR shielding properties. Ceram. Int. 44, 13469–13475. doi: 10.1016/j.ceramint.2018.04.158
Yoon, S., Jo, C., Noh, S. Y., Lee, C. W., Song, J. H., and Lee, J. (2011a). Development of a high-performance anode for lithium ion batteries using novel ordered mesoporous tungsten oxide materials with high electrical conductivity. Phys. Chem. Chem. Phys. 13, 11060–11066. doi: 10.1039/c1cp20940j
Yoon, S., Kang, E., Kim, J. K., Lee, C. W., and Lee, J. (2011b). Development of high-performance supercapacitor electrodes using novel ordered mesoporous tungsten oxide materials with high electrical conductivity. Chem. Commun. 47, 1021–1023. doi: 10.1039/C0CC03594G
Yue, L., Tang, J., Li, F., Xu, N., Zhang, F., Zhang, Q., et al. (2017). Enhanced reversible lithium storage in ultrathin W18O49 nanowires entwined Si composite anode. Mater. Lett. 187, 118–122. doi: 10.1016/j.matlet.2016.10.093
Zeng, W., Suo, L., Zhang, C., Wu, D., and Zhu, H. (2019). AgI–Ag2S heterostructures for photothermal conversion and solar energy harvesting. J. Taiwan Inst. Chem. Eng. 95, 273–280. doi: 10.1016/j.jtice.2018.07.012
Zeng, X., Zhou, Y., Ji, S., Luo, H., Yao, H., Huang, X., et al. (2015). The preparation of a high performance near-infrared shielding CsxWO3/SiO2 composite resin coating and research on its optical stability under ultraviolet illumination. J. Mater. Chem. C 3, 8050–8060. doi: 10.1039/C5TC01411E
Zhang, L., Tang, B., Wu, J., Li, R., and Wang, P. (2015a). Hydrophobic light-to-heat conversion membranes with self-healing ability for interfacial solar heating. Adv. Mater.27, 4889–4894. doi: 10.1002/adma.201502362
Zhang, W., Yue, L., Zhang, F., Zhang, Q., Gui, X., Guan, R., et al. (2015b). One-step in situ synthesis of ultrathin tungsten oxide@ carbon nanowire webs as an anode material for high performance. J. Mater. Chem. A 3, 6102–6109. doi: 10.1039/C4TA06262K
Zhang, Z., Jiang, X., Liu, B., Guo, L., Lu, N., Wang, L., et al. (2018). IR-driven ultrafast transfer of plasmonic hot electrons in non-metallic branched heterostructures for enhanced H2 generation. Adv. Mater. 30:1705221. doi: 10.1002/adma.201705221
Zhao, Y., Tang, Q., Yang, P., and He, B. (2017). Robust electrocatalysts from metal doped W18O49 nanofibers for hydrogen evolution. Chem. Commun. 53, 4323–4326. doi: 10.1039/C7CC01249G
Zheng, J. Y., Haider, Z., Van, T. K., Pawar, A. U., Kang, M. J., Kim, C. W., et al. (2015). Tuning of the crystal engineering and photoelectrochemical properties of crystalline tungsten oxide for optoelectronic device applications. CrystEngComm 17, 6070–6093. doi: 10.1039/C5CE00900F
Zhenzhen, G., Xin, M., Gang, W., Baofei, H., Xinghang, L., Tao, M., et al. (2018). Super-hydrophilic copper sulfide films as light absorbers for efficient solar steam generation under one sun illumination. Semicond. Sci. Technol. 33:025008. doi: 10.1088/1361-6641/aaa323
Zhou, M., Zhang, R., Huang, M., Lu, W., Song, S., Melancon, M. P., et al. (2010). A chelator-free multifunctional [64Cu]CuS nanoparticle platform for simultaneous micro-PET/CT Imaging and photothermal ablation therapy. J. Am. Chem. Soc. 132, 15351–15358. doi: 10.1021/ja106855m
Zhou, Y., Ko, S., Lee, C. W., Pyo, S. G., Kim, S. -K., and Yoon, S. (2013). Enhanced charge storage by optimization of pore structure in nanocomposite between ordered mesoporous carbon and nanosized WO3−x. J. Pow. Sources 244, 777–782. doi: 10.1016/j.jpowsour.2013.04.054
Keywords: photothermal conversion, non-stoichiometric tungsten-oxides (WO2.72), tungsten bronze (MxWO3), water evaporation, photocatalyst
Citation: Wu C-M, Naseem S, Chou M-H, Wang J-H and Jian Y-Q (2019) Recent Advances in Tungsten-Oxide-Based Materials and Their Applications. Front. Mater. 6:49. doi: 10.3389/fmats.2019.00049
Received: 03 January 2019; Accepted: 04 March 2019;
Published: 27 March 2019.
Edited by:
Sheikh A. Akbar, The Ohio State University, United StatesReviewed by:
Rujia Zou, Donghua University, ChinaMukul Pradhan, National Institute of Technology Meghalaya, India
Copyright © 2019 Wu, Naseem, Chou, Wang and Jian. This is an open-access article distributed under the terms of the Creative Commons Attribution License (CC BY). The use, distribution or reproduction in other forums is permitted, provided the original author(s) and the copyright owner(s) are credited and that the original publication in this journal is cited, in accordance with accepted academic practice. No use, distribution or reproduction is permitted which does not comply with these terms.
*Correspondence: Chang-Mou Wu, cmwu@mail.ntust.edu.tw