- 1Department of Biomedical Sciences and Engineering, and Department of Chemical and Materials Engineering, National Central University, Taoyuan, Taiwan
- 2R&D Center for Membrane Technology, Chung Yuan Christian University, Taoyuan, Taiwan
- 3Genomics Research Center, Academia Sinica, Taipei, Taiwan
- 4Department of Chemical Engineering, Stanford University, Stanford, CA, United States
The cell membrane is an organized and fluid structure that modulates cellular activities in response to specific extracellular signals, and maintains the critical communication, integration, and homeostasis between the cytosol and the extracellular matrix (ECM). In recent years, tissue engineering and cell biology research has been rapidly progressed by a remarkable understanding of cell and ECM interfaces. In this review, the design of new biomimetic platforms based on the conjugation of ECM proteins on solid supported lipid bilayers (SLBs) will be summarized. The platforms provide a better system to evaluate cellular responses to specific recognition events, gradient, mechanical property, nanostructures, and inter- and intra-molecular interactions of ECM proteins on a non-fouling and fluid membrane. Moreover, the findings from the molecular interactions and cellular activities will be highlighted to look into the cell-materials mechanisms.
Extracellular Matrix
The interactions between extracellular matrix (ECM) proteins and cells play an important role in cytoskeletal organization, cell growth, cell migration, and tissue development (Zhong et al., 1998; Sternlicht and Werb, 2001; Stevens and George, 2005). The ECM contains secreted molecules that constitute the cell microenvironment. Main constituents of the ECM are a network of hydrophilic, extended gels of glycosaminoglycans (GAGs) and fibrous proteins, including elastin, collagens, laminin, and fibronectin, which connect through inter- and intra-molecularly specific binding domains. GAGs, such as heparan sulfate, chondroitin sulfate, and keratan sulfate are highly swollen carbohydrate polymers attached to ECM proteins to form proteoglycans. The GAGs can hold water via osmosis to keep the growth factors, ECM, and cells hydrated and active. GAG molecules can regulate a wide range of biological activities, including angiogenesis, developmental processes, tumor metastasis, and blood coagulation (Kim et al., 2011). For example, collagens, the most abundant protein in the ECM, comprise 90% of bone matrix protein content. Collagens are present in the ECM as fibrillar proteins and provide a structural scaffold for accommodation of cells. Structurally, collagen is assembled from procollagen, which composes of three left-handed helices. Fibrous procollagen is arranged to form an extended superhelix structure with roughly 1.5 nm in diameter and 300 nm in length. The procollagens can self-assemble spontaneously in vivo and in vitro into fibrils with up to several microns long and ranging from 10 to 500 nm wide and a periodicity of 67 nm. The aggregation states and spatial distribution of collagens have been found to modulate cellular development, and signaling through integrins recognition (Hui et al., 2008; Huang et al., 2010a).
Fibronectins are glycoproteins that attach cells with collagen scaffolds in the ECM, allowing cells to migrate through the ECM. Therefore, in the organization of the basal structure of the ECM network and the control of cell behaviors, the attachment of fibronectins on collagens is particularly crucial (roekelmann et al., 1991; Dalton et al., 1995). In healing wounds, for example, fibroblasts express high levels of smooth muscle actin, procollagen, and fibronectin. It has been shown that fibronectin is a mediator for cell attachment to collagen. Thus, cell adhesion to the ECM is important for subsequent behaviors of differentiation and proliferation (Ingber, 2003). In the in vitro environment, cells are seeded on culture surfaces and soon can adapt to fit their environment by secreting suitable ECM proteins, such as fibronectin, to remodel the surfaces for better spreading and adhesion. The intermolecular interactions in ECM dynamically change with the cellular activities. The complex process is related to the culture environment including culture medium, adsorbed proteins, underlying substrate, and cell types. It has been found that sufficient levels of fibronectin are required for cell adhesion and spreading on collagen-deposited surfaces (Grinnell and Minter, 1978; Dewez et al., 1999). Moreover, the surface properties of the underlying substrates dominate the adhesion of the proteins, leading to various surface contents, conformation, and concentration of the deposited proteins. It should be noted that tradition culture systems, such as glass, and tissue culture polystyrene, facilitating non-specific protein adsorption, are unable to investigate definitely the interactions between cells and a correspondent component/protein in the ECM.
Most mammalian cells can just grow in vitro when they are attached to surfaces with deposition of the ECM proteins. The ECM provides more than structural and mechanical support, implications in stem cell niches, developmental patterning, and cancer progress. The physical properties of the ECM may also serve a critical position in the signaling process. ECM structures can be extendable and flexible, and the various mechanical tension can impose on cryptic sites, which could further react with their receptors or growth factors. In regard to mechanical properties, the ECM can possess varying degrees of elasticity, and stiffness, from hard bone tissues to soft brain, according to their constitutions, concentrations, and crosslinking structures. The elasticity of the ECM can span over several orders of magnitude, which has been shown to play an important role in controlling numerous cell functions, such as cellular contraction, cell proliferation, migration, differentiation, and cell death (Discher et al., 2005; Engler et al., 2006). In addition, it was found that the nanostructures of ECM proteins can considerably affect a cell's activities (Koyama et al., 1996; Jones et al., 1997; Huang et al., 2010a). Type I collagen in nature spontaneously forms a helix structure. After the treatment of heat and acid, the regular three-dimensional structure of collagen is destroyed (denatured collagen or gelatin). Surprisingly, the denatured collagen promotes smooth muscle cell (SMC) cellular better spreading and proliferation with respect to wild type collagen (native collagen). A mechanistic study proposed that native collagen suppresses phosphorylation of cyclin-dependent kinase 2 (Cdk2) and cyclin E-associated kinase while increasing levels of the Cdk2 inhibitors p21Cip1/waf1, and p27Kip1 (Koyama et al., 1996). The effect appeared to control the growth of pulmonary stem cells in the primary culture system (Huang et al., 2010a). The system included stroma cells, pulmonary stem cells, and type I collagen. When the fibrillar size of collagen increases, the proliferation and spreading of mesenchymal stromal cells decrease, which leads to inhibition of the growth of primary neonatal pulmonary cells (Huang et al., 2010a). Therefore, mesenchymal stromal cells serve as niche cells to directly regulate the renewal ability of pulmonary epithelial stem cells.
Dynamic Functional Biointerfaces
To better understand the recognition and regulation mechanisms between the ECM and cells, several model systems have been established based on functional self-assembled monolayers (SAMs) (Roberts et al., 1998; Xiao et al., 1998; Rezania et al., 1999). The SAMs of alkanethiolates on gold surfaces can present mixtures of arginine-glycine-aspartate (RGD), and oligo(ethylene glycol) (OEG) moieties. RGD is a tripeptide, found to promote cell adhesion by binding to cell surface integrin receptors, and OEG is typically used to resist non-specific adsorption of proteins and cells. The fraction and structures of the RGD tripeptide moieties permitted the manipulation of cell adhesion behaviors. The systems allowed for the elucidation of the complex biological activities to a certain extent. However, as mentioned above, the mechanical properties and molecular interactions among ECM components (i.e., fibronectin-collagen and GAG-collagen) in the microenvironments can substantially determine the cellular activities. Moreover, the relatively static ligands on the surfaces cannot reflect the dynamics at cell-ECM contacts.
Because of the fact that protein deposition on the surface in a random manner will fail to define its interaction with cells, a well-characterized platform is required. In order to scrutinize the cellular activities in tissues, such a platform should exhibit multiple functions, such as capabilities to immobilize specific ligands, to prevent misleading cellular activities induced from adsorbed non-target proteins, and to present extended and hydrated ECM-like structure. Thus, the functionalizable and non-fouling properties of substrates become substantial as a platform for cell growth. Among the many non-biofouling materials that can strongly resist protein non-specific adsorption (Ishihara et al., 1998; Chen et al., 2010; Jiang and Cao, 2010), lipid-based materials are thought to be the best to biological systems because they create a cell membrane-like microenvironment for investigating cell-cell and cell-biomaterial interactions. Particularly, the complex cell-cell and cell-biomaterial interactions are highly dynamic with a wide range of biomolecules in a spatiotemporal controlled fashion, such as complex intracellular signaling processes and structural reorganization. They are not able to be conducted by static SAM systems. In order to monitor the complexity of the cellular membrane and associated processes in vitro, dynamic synthetic materials are developed where control over ligand characteristics such as mobility, density, and presentation are possible. The model cell membranes, supported lipid bilayers (SLBs), offer a bottom-up approach to construct dynamic functional biointerfaces to enable self-assembly and ligand mobility, presentation and distribution at given locations (Kocer and Jonkheijm, 2018). Therefore, the focus of this article lies on developing cell-ECM contacts using functional SLBs to elucidate the complex dynamic behaviors to exploit the key characteristics of the cell membranes and their interactions with ECMs. Based on SLBs, studies have constructed a functionalized SLB modified with small molecules, such as RGD peptide (Svedhem et al., 2003; Jensen et al., 2004; Ochsenhirt et al., 2006) and epidermal growth factor (Nam et al., 2006) for controls over cellular responses. However, to broaden the scope and complexity of the microenvironment, a biomimetic system constructed by conjugation of ECM proteins to a functionalized SLB can be more applicable to illustrate the interactions between ECM components and cells. The fluidity, gradient, mechanical properties, nanostructures, inter-molecular (e.g., collagen-fibronectin) and intra-molecular (e.g., collagen fibrillogenesis) interactions of ECM components on SLBs can be defined and evaluated to explore the actual cellular responses in the microenvironment, thus distinguishing this type of cell culture platform from other ones on solids, such as plastics, metals, oxides, and SAMs. Therefore, SLB offers a robust platform with unique properties similar to the cell membrane, such as non-fouling, hydration, lateral diffusion, and the ability to control the spatial location and density of ligands for great potential in medical science and engineering (Sackmann, 1996; Groves et al., 2001).
Extracellular Matrix-Supported Lipid Bilayer Systems
The pioneering work of the biomimetic cell culture system based on an ECM-conjugated SLB were conducted by Chang's group (Huang et al., 2010b). They constructed a biomimetic platform by functionalizing type I collagen on SLBs to serve as a substrate to verify cell culture and to investigate cell behaviors., The functionalizable lipid vesicles composed of 1,2-dipalmitoyl-sn-glycero-3-phosphoethanolamine-N-(glutaryl) (DP-NGPE) and 1-palmitoyl-2-oleoyl-sn-glycero-3-phosphocholine (POPC) were deposited onto an SiO2 substrate to form SLBs (Figure 1). The molar fraction of DP-NGPE, a carboxylic acid-functionalized phospholipid, in the SLBs was varied from 0 to 40%. To create the type I collagen-functionalized lipid bilayer assembly, native type I collagen molecules were brought into the chamber to react activated DP-NGPE lipids and to form stable collagen-lipid conjugates with amide linkages through amine coupling chemistry. The binding kinetics of the protein immobilization and the film characteristics of film thickness, viscoelasticity, conformation, and fluidity were monitored using a quartz crystal microbalance with dissipation (QCM-D), atomic force microscopy (AFM), and fluorescence recovery after photobleaching (FRAP). The results showed that the collagen that adsorbed onto functionalized SLBs that had a high percentage of DP-NGPE increased their surface mass, viscoelasticity, and self-assembly into fibrillar structures. The data from FRAP indicated that lateral lipid mobility was reduced by up to 20% after the coupling of type I collagen to the SLBs. Smooth muscle cells (A10) spread and grew on the collagen-functionalized platform in a regular fashion, unlike the POPC/DG-NGPE bilayer without collagen and the bare POPC lipid bilayer. The simple bottoms-up biomimetic system, containing key constituents of the plasma membrane and the ECM, offer the opportunity to reveal cellular recognition and response to ECM elements and physical clues.
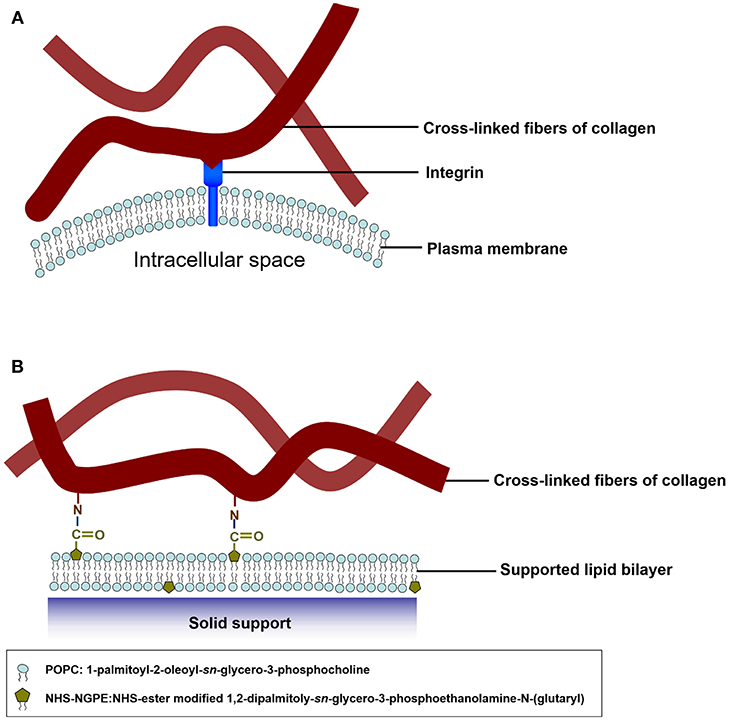
Figure 1. Schematic illustration of mimetic collagen modification strategy based on integrins in mammalian cells. (A) In the in vivo microenvironment of cells, they attach collagen fibers via integrins. (B) To mimic the microenvironment of the cells, collagen fibers are chemically modified onto the model SLB. The blue spheres symbolize POPC headgroups and the olive pentagons represent the NHS-ester groups of DP-NGPE. Reprinted with permission from Huang et al. (2010b). Copyright 2010 American Chemical Society.
In addition to the point-resolved study, evolutionary cellular responses on the ECM-SLB system were investigated by the same group (Huang et al., 2010c). In the study, the biomimetic ECM microenvironments were fabricated by conjugating type I collagen and/or fibronectin on the functionalizable SLBs containing activated DP-NGPE (Figure 2). The protein-conjugated systems were quantitatively characterized by QCM-D in terms of surface mass, viscoelasticity, and collagen-fibronectin interactions. The retardation of mobility of the SLB after protein conjugation and cell culture were revealed by FRAP. NIH 3T3 fibroblasts were cultured on the ECM-SLB constructs and on oxygen plasma treated polystyrene (PSo) for parallel comparison. On the ECM-SLB cultures, the largest cell number counts and spreading size of fibroblasts were found on the fibronectin deposited SLBs. Nevertheless, no such distinguishable differences on the ECM-coated PSo can be observed among all the protein contents. In addition, immunofluorescent staining data indicated that the adsorption level of fibronectin, endogenously produced by 3T3 cells, on PSo-based surfaces was obviously more than that on SLB-based platforms. The findings highlight the important role of the anti-fouling nature of the underlying SLBs in preventing 3T3 cells from remodeling effectively their microenvironment. On the contrary, cells can easily remodel by the nonspecific adsorption of ECM proteins on the PSo-based platforms. Consequently, the ECM-SLB platform can be utilized for monitoring and regulating cellular specific responses to ECM compositions and subsequent signaling events with extracellular environments.
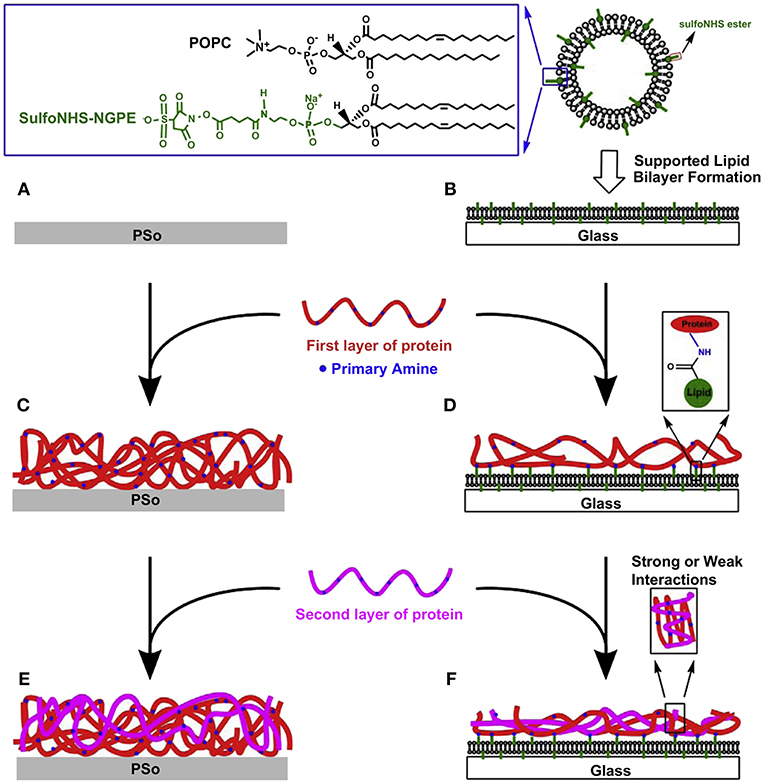
Figure 2. Fabrication processes of functionalized PSo- (A,C,E) and SLB-based (B,D,F) protein adsorbed films for investigating responses of NIH-3T3 fibroblasts. In serum-free culture medium, cells were incubated on six types of surfaces for 6 h. Reprinted with permission from Huang et al. (2010c) Copyright 2010 Elsevier Ltd.
Cho's group examined the behavior of cells on low rigidity SLB functionalized with either fibronectin or type I collagen via amine coupling chemistry (Vafaei et al., 2017a). Traditionally, plastic cell culture plates exhibit an extremely stiff substrate, which could not reflect the real mechanical stiffness experienced by cells in physiological microenvironments. Recently, elastomers, such as polydimethyl siloxane (PDMS), have been used to modulate wide stiffness regimes to provide many important insights into the role of mechanical properties of the ECM in cellular responses (Engler et al., 2006). Nevertheless, the SLB systems offer opportunities to access the softest and most fluid interfaces to monitor cell activities may in a physiological environment. The lipid vesicles composed of a zwitterionic 1,2-dioleoyl-sn-glycero-3-phosphatidylcholine (DOPC) and DP-NGPE, which then were deposited on SiO2 coverslips by the solvent assisted lipid bilayer (SALB) formation method (Tabaei et al., 2014; Vafaei et al., 2017b). Different from conventional vesicle fusion method, which involves the spontaneous adsorption and rupture of phospholipid vesicles, and is limited to only a subset of lipid compositions and solid supports (Huang et al., 2010a,b,c), the SALB formation method is based on the reverse-phase evaporation phenomenon and involves lipid deposition onto a hydrophilic solid surface in an alcohol (Tabaei et al., 2014). The SALB method has been applied successfully to a wide variety of substrates, such as Au, Al2O3, and graphene, which are intractable to the conventional method (Tabaei et al., 2014; Jackman et al., 2015). The ECM proteins of type I collagen and fibronectin were chemical conjugated on SALB-assisted SLBs. The QCM-D studies were conducted and found that ECMs on SLB are less dense and have higher structural flexibility than when adsorbed onto SiO2 (Vafaei et al., 2017b).
Furthermore, the SLB-based low rigidity substrate was functionalized with type I collagen or fibronectin for cell adhesion studies (Vafaei et al., 2017a). The lateral fluidity of the SLB was finely tuned by controlling the molar fraction of DP-NGPE and cholesterol in the SLB. Specific adhesion of cells on the bilayer, from the fluorescence images, was indicated by an enrichment of the ECM proteins and the appearance of a zone of depletion around the cells. On the high cholesterol-containing bilayers, >10% of cells showed a depletion of either fibronectin or collagen. This is ascribed by the reduced lateral movement of ECM proteins, which is controlled by the viscosity of the underlying bilayer. Therefore, the ECM-SLB platform with addition of cholesterol provides a range of substrate rigidity for potential biomedical applications that require cell adhesion to extremely low-rigidity surfaces such as neuronal tissues.
For the development of a platform that is more closely mimic the cell microenvironment, an SLB was functionalized with natural decellularized extracellular matrix (dECM), which retain 3D-ultrastructure and support the survival and attachment of human hepatocyte Huh 7.5 (Figure 3) (Vafaei et al., 2018). The dECM was extracted from cadavers of mouse and were decellularized using mixture of chloroform/methanol solution. The dECM contains GAGs, collagen, and fibronectin. The dECM components were chemically attached to the SLB containing DP-NGPE and DOPC by amine coupling chemistry. QCM-D was used to monitor the bilayer formation and the kinetics of the subsequent dECM deposition. Results from FRAP confirmed the fluidity of the membrane after functionalization with dECM. The platform supports the survival and attachment of the cells, and maintains the representative hepatocellular function. Strikingly, the lateral clustering and organization of growth factor receptors and cell-binding ligands in the presence of tissue-derived dECM components with the rich biochemical profile were observed on the platform.
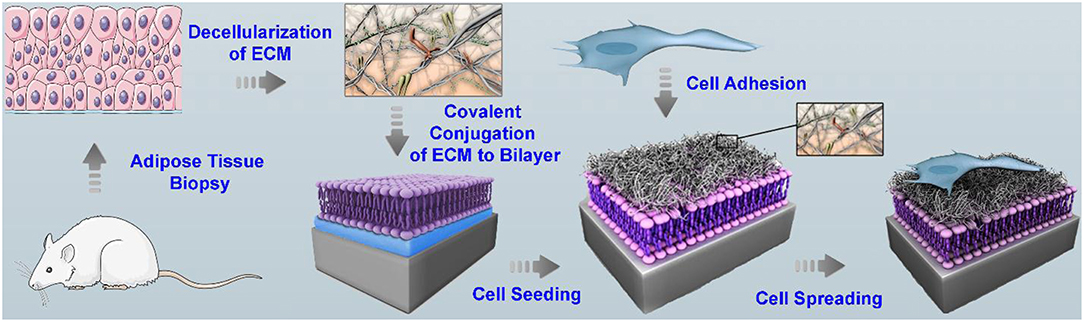
Figure 3. Scheme of the various steps involved in the preparation of SLB functionalized with adipose tissue dECM components for cell culture. The decellularized ECM derived from adipose tissue was solubilized in an acidic condition and was chemically attached via amine coupling chemistry to the surface of a supported bilayer containing lipids with a head group bearing a free carboxylic acid group. Subsequently, cells were cultured and their attachment, spreading, growth, and function were studied. Reprinted with permission from Vafaei et al. (2018). Copyright 2018 American Chemical Society.
Hao et al. reported the effect of the mechanical properties of an ECM-SLB on the differentiation of neural stem cells (NSCs) (Hao et al., 2018). SLBs were used to as a culture platform to mimic the dynamic characteristics of the fluid ECM. The fluidity of SLBs was finely varied by the surface properties of the substrates. The SLBs contain 1,2-dipalmitoyl-sn-glycero-3-phosphoethanolamine-N-(7-nitro-2-1, 3-benzoxadiazol-4-yl) (NBD-PE), 1,2-dimyristoyl-sn-glycero-3-phosphocholine (DMPC), and 1,2-dipalmitoyl-sn-glycero-3-phosphoethanolamine-N-(succinyl) (Succinyl-PE). From the FRAP measurements, the SLB on piranha-treated glass (P-SLB) exhibits greater fluidity compared with that on cholesteryl chloroformate tether-modified glass (CC-SLB) or chitosan-modified glass (Cs-SLB). Moreover, the fluidity of CC-SLB was slightly lower than Cs-SLB. Fibronectin was subsequently physically adsorbed on the SLBs on different substrates. The results indicated that the behavior of the NSCs was highly related to the fluidity of the SLBs. Enhanced focal adhesion, a stretched and elongated cellular morphology, a dense network of stress fibers, and increased neuronal differentiation were observed on the SLB with low fluidity. On the contrary, less focal adhesion formation, a round cellular morphology, immature stress fibers, and more astrocyte differentiation were found on the SLB with high fluidity. In mechanistic studies, they showed that activation of FAK-MEK/ERK signaling pathways is a key route to the enhanced focal adhesion formation and ultimately promoted neuronal differentiation of NSCs on the SLB with low fluidity. The work provided insight into the effect of the dynamic ECM on stem cell behavior as well as improves the efficacy of stem cell applications.
Tethered liposomes on SLBs have been established for DNA hybridization (Benkoski and Hook, 2005; Yoshina-Ishii et al., 2005), biotin-streptavidin binding (Patel et al., 2009), and drug delivery (Tseng and Chang, 2012). The tethered liposome systems were applied for the reconstitution of membrane proteins to study protein-lipid and protein-protein interactions, as well as the encapsulation of proteins for single biomolecule detection. A biomimetic construct containing ECM-SLBs was formed with polyethylene glycol (PEG), fibronectin, and cholesterol-containing liposomes (Tseng and Chang, 2012). The SLBs were made of functional 1,2-dipalmitoyl-sn-glycero-3-phosphoethanolamine-N-cap-biotinyl (b-PE) and zwitterionic POPC. The drug-encapsulated liposomes were immobilized by the biotin-streptavidin interaction (Figure 4). The liposome-SLB construct serves as a multifunctional platform for drug release and cell attachment. The formation of the fibronectin-liposome-SLB model platform was monitored in situ with QCM-D. The excellent stability of the surface-tethered liposomes on SLBs was accessed via an encapsulated fluorescent probe. It shows that < 20% of the fluorescent probe content was released in 8 days. HeLa cells were cultured on the fibronectin-liposome-SLB platform to investigate the cellular interactions. The fibronectin was found to be critical to enhance HeLa cell adhesion on the platforms. Therefore, upon cell adhesion, the liposomes were spatially reorganized and engulfed by the cells. The surface density of doxorubicin-loaded liposomes determines apoptosis of HeLa cell, confirming effectiveness of drug delivery by the liposomes. Therefore, the multifunctional model platform could be beneficial as pre-administered, controlled-release system for cell- and tissue-based assays.
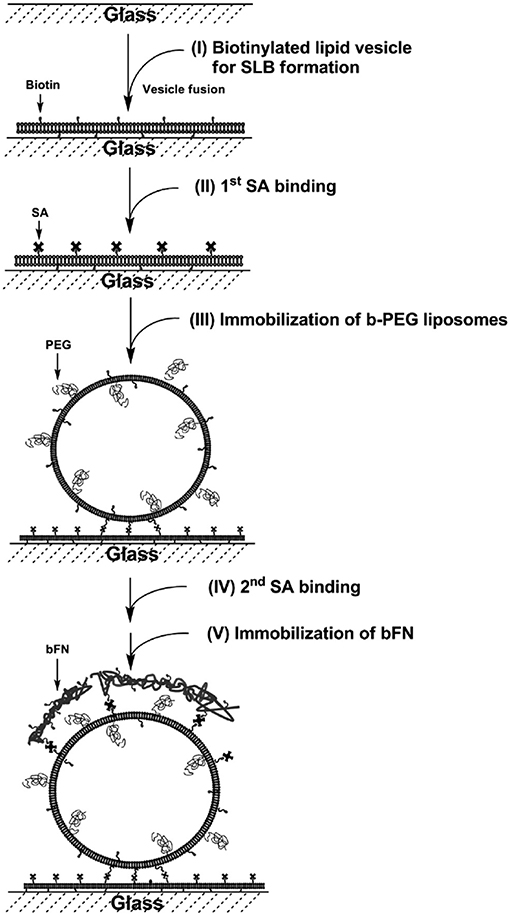
Figure 4. Fibronectin-liposome functionalized surfaces based on a biotinylated lipid bilayer (FN-liposome-SLB). Construction of the model surface involves five steps: (I) formation of SLB, (II) first layer of streptavidin binding, (III) immobilization of b-PEG liposomes, which may contain dye-labeled lipids, or encapsulate DOX/fluorescent dye, (IV) the second layer of streptavidin binding, and (V) immobilization of biotinylated-fibronectin (bFN). Reprinted with permission from Tseng and Chang (2012). Copyright 2012 American Chemical Society.
In addition to the ECM proteins, GAGs exhibit multiple functions in tissues including providing support, mediating cell differentiation and division, and taking part in important interactions with proteins. Understanding GAG-related interactions is inherently difficult and requires proper and defined platforms. Svedhem et al. showed two immobilization strategies to chemically couple the GAG chondroitin sulfate (CS) to fluid SLBs: 1. by activating carboxyl groups on CS prior to the addition of an amino-functionalized lipid, i.e., 1,2-dioleoyl-sn-glycero-3-phosphoethanolamine-N-(lauroylamine) (DOPE-NH2); 2. by activating carboxy-functionalized phospholipids, such as 1,2-dioleoyl-sn-glycero-3-phosphoethanolamine-N-(lauroyl) (DOPE-COOH), followed by the addition of hydrazide-functionalized CS (Altgarde et al., 2013). The formation of SLBs and subsequent conjugation was followed in situ using QCM-D. The results show that the two strategies yielded thin CS films with similar viscoelastic properties. The fluidity of the lipid bilayer was not affected by the conjugation of CS. Thus, the application of the developed CS platform on SLBs was exemplified for the bone inducing growth factor bone morphogenetic protein-2.
Summary and Outlook
The establishment of the microenvironments for growth and regulations of cells in vitro remains challenging. The complexity of ECMs was absent in the regular cell culture systems, which includes the fluidity, gradient, mechanical property, nanostructures, and inter- and intra-molecular interactions. The biomimetic SLB system conjugated with ECM components shed some light on the actual cellular responses in the microenvironment in vivo. The SLB provides unique characteristics of fluidity, fouling resistance, versatility, and biocompatibility. Up to now, we have not found any alternative to be used for the same purpose. The physical properties of SLB-ECM platforms can be clearly defined by applying advanced instruments, such as QCM-D, AFM, and FRAP, to correlate the cellular responses. Moreover, the dynamic behaviors of the cells on the SLB-ECM platforms should be worthwhile to follow because the ECM compositions and structures sustainably change with the growth of cells. On the SLB-ECM platform, researchers are allowed to observe the specific recognition interactions between cells and their microenvironment for direct translation of biological activities on the artificial culture system. Beyond the fundamental studies, the SLB-ECM platform is believed to be useful for various applications, such as drug screening, rare cell capture, regeneration medicine, and biosensing.
Author Contributions
C-JH is responsible for the literature search and writing. Y-CC is responsible for writing and revision.
Funding
MOST 105-2628-E-008-007-MY3; 106-2119-M-194-002; 107-0210-01-19-01; 107-2119-M-001-039 from the Ministry of Science and Technology (MOST), Taiwan, and Academia Sinica, Taiwan.
Conflict of Interest Statement
The authors declare that the research was conducted in the absence of any commercial or financial relationships that could be construed as a potential conflict of interest.
Acknowledgments
We acknowledge the Ministry of Science and Technology (MOST 105-2628-E-008-007-MY3; 106-2119-M-194-002; 107-2119-M-001-039) for financial support of this project.
References
Altgarde, N., Becher, J., Moller, S., Weber, F. E., Schnabelrauch, M., and Svedhem, S. (2013). Immobilization of chondroitin sulfate to lipid membranes and its interactions with ECM proteins. J. Colloid Interface Sci. 390, 258–266. doi: 10.1016/j.jcis.2012.07.063
Benkoski, J. J., and Hook, F. (2005). Lateral mobility of tethered vesicle - DNA assemblies. J. Phys. Chem. B 109, 9773–9779. doi: 10.1021/jp044947p
Chen, S. F., Li, L. Y., Zhao, C., and Zheng, J. (2010). Surface hydration: Principles and applications toward low-fouling/nonfouling biomaterials. Polymer 51, 5283–5293. doi: 10.1016/j.polymer.2010.08.022
Dalton, B. A., McFarland, C. D., Underwood, P. A., and Steele, J. G. (1995). Role of the heparin-binding domain of fibronectin in attachment and spreading of human bone-derived cells. J. Cell Sci. 108, 2083–2092.
Dewez, J. L., Doren, A., Schneider, Y. J., and Rouxhet, P. G. (1999). Competitive adsorption of proteins: Key of the relationship between substratum surface properties and adhesion of epithelial cells. Biomaterials 20, 547–559. doi: 10.1016/s0142-9612(98)00207-5
Discher, D. E., Janmey, P., and Wang, Y. L. (2005). Tissue cells feel and respond to the stiffness of their substrate. Science 310, 1139–1143. doi: 10.1126/science.1116995
Engler, A. J., Sen, S., Sweeney, H. L., and Discher, D. E. (2006). Matrix elasticity directs stem cell lineage specification. Cell 126, 677–689. doi: 10.1016/j.cell.2006.06.044
Grinnell, F., and Minter, D. (1978). Attachment and spreading of baby hamster kidney cells to collagen substrata: effects of cold-insoluble globulin. Proc Natl Acad Sci U S A. 75, 4408–4412.
Groves, J. T., Mahal, L. K., and Bertozzi, C. R. (2001). Control of cell adhesion and growth with micropatterned supported lipid membranes. Langmuir 17, 5129–5133. doi: 10.1021/la010481f
Hao, W., Han, J., Chu, Y., Huang, L., Sun, J., Zhuang, Y., et al. (2018). Lower fluidity of supported lipid bilayers promotes neuronal differentiation of neural stem cells by enhancing focal adhesion formation. Biomaterials 161, 106–116. doi: 10.1016/j.biomaterials.2018.01.034
Huang, C. J., Chien, Y. L., Ling, T. Y., Cho, H. C., Yu, J., and Chang, Y. C. (2010a). The influence of collagen film nanostructure on pulmonary stem cells and collagen-stromal cell interactions. Biomaterials 31, 8271–8280. doi: 10.1016/j.biomaterials.2010.07.038
Huang, C. J., Cho, N. J., Hsu, C. J., Tseng, P. Y., Frank, C. W., and Chang, Y. C. (2010b). Type I collagen-functionalized supported lipid bilayer as a cell culture platform. Biomacromolecules 11, 1231–1240. doi: 10.1021/bm901445r
Huang, C. J., Tseng, P. Y., and Chang, Y. C. (2010c). Effects of extracellular matrix protein functionalized fluid membrane on cell adhesion and matrix remodeling. Biomaterials 31, 7183–7195. doi: 10.1016/j.biomaterials.2010.05.076
Hui, T. Y., Cheung, K. M. C., Cheung, W. L., Chan, D., and Chan, B. P. (2008). In vitro chondrogenic differentiation of human mesenchymal stem cells in collagen microspheres: Influence of cell seeding density and collagen concentration. Biomaterials 29, 3201–3212. doi: 10.1016/j.biomaterials.2008.04.001
Ingber, D. E. (2003). Tensegrity II. How structural networks influence cellular information processing networks. J. Cell Sci. 116, 1397–1408. doi: 10.1242/jcs.00360
Ishihara, K., Nomura, H., Mihara, T., Kurita, K., Iwasaki, Y., and Nakabayashi, N. (1998). Why do phospholipid polymers reduce protein adsorption? J. Biomed. Mater. Res. 39, 323–330. doi: 10.1002/(SICI)1097-4636(199802)39:2%3C323::AID-JBM21%3E3.0.CO;2-C
Jackman, J. A., Tabaei, S. R., Zhao, Z. L., Yorulmaz, S., and Cho, N. J. (2015). Self-assembly formation of lipid bilayer coatings on bare Aluminum oxide: overcoming the force of interfacial water. Acs Appl. Mater. Interfaces 7, 959–968. doi: 10.1021/am507651h
Jensen, T. W., Hu, B. H., Delatore, S. M., Garcia, A. S., Messersmith, P. B., and Miller, W. M. (2004). Lipopeptides incorporated into supported phospholipid monolayers have high specific activity at low incorporation levels. J. Am. Chem. Soc. 126, 15223–15230. doi: 10.1021/ja048684o
Jiang, S., and Cao, Z. Q. (2010). Ultralow-fouling, functionalizable, and hydrolyzable zwitterionic materials and their derivatives for biological applications. Adv. Mater. 22, 920–932. doi: 10.1002/adma.200901407
Jones, P. L., Crack, J., and Rabinovitch, M. (1997). Regulation of tenascin-C, a vascular smooth muscle cell survival factor that interacts with the αvβ3 integrin to promote epidermal growth factor receptor phosphorylation and growth. J. Cell Biol. 139, 279–293. doi: 10.1083/jcb.139.1.279
Kim, S. H., Turnbull, J., and Guimond, S. (2011). Extracellular matrix and cell signalling: the dynamic cooperation of integrin, proteoglycan and growth factor receptor. J. Endocrinol. 209, 139–151. doi: 10.1530/joe-10-0377
Kocer, G., and Jonkheijm, P. (2018). About chemical strategies to fabricate cell-instructive biointerfaces with static and dynamic complexity. Adv. Healthcare Mater. 7:32. doi: 10.1002/adhm.201701192
Koyama, H., Raines, E. W., Bornfeldt, K. E., Roberts, J. M., and Ross, R. (1996). Fibrillar collagen inhibits arterial smooth muscle proliferation through regulation of Cdk2 inhibitors. Cell 87, 1069–1078. doi: 10.1016/s0092-8674(00)81801-2
Nam, J. M., Nair, P. M., Neve, R. M., Gray, J. W., and Groves, J. T. (2006). A fluid membrane-based soluble ligand-display system for live-cell assays. Chembiochem 7, 436–440. doi: 10.1002/cbic.200500479
Ochsenhirt, S. E., Kokkoli, E., McCarthy, J. B., and Tirrell, M. (2006). Effect of RGD secondary structure and the synergy site PHSRN on cell adhesion, spreading and specific integrin engagement. Biomaterials 27, 3863–3874. doi: 10.1016/j.biomaterials.2005.12.012
Patel, A. R., Kanazawa, K. K., and Frank, C. W. (2009). Antibody binding to a tethered vesicle assembly using QCM-D. Anal. Chem. 81, 6021–6029. doi: 10.1021/ac802756v
Rezania, A., Johnson, R., Lefkow, A. R., and Healy, K. E. (1999). Bioactivation of metal oxide surfaces. 1. Surface characterization and cell response. Langmuir 15, 6931–6939. doi: 10.1021/la990024n
Roberts, C., Chen, C. S., Mrksich, M., Martichonok, V., Ingber, D. E., and Whitesides, G. M. (1998). Using mixed self-assembled monolayers presenting RGD and (EG)(3)OH groups to characterize long-term attachment of bovine capillary endothelial cells to surfaces. J. Am. Chem. Soc. 120, 6548–6555. doi: 10.1021/ja972467o
roekelmann, T. J., Limper, A. H., Colby, T. V., and McDonald, J. A. (1991). Transforming growth factor-beta-1 is present at sites of extracellular-matrix gene-expression in human pulmonary Fibrosis. Proc. Natl. Acad. Sci. U S A. 88, 6642–6646. doi: 10.1073/pnas.88.15.6642
Sackmann, E. (1996). Supported membranes: Scientific and practical applications. Science 271, 43–48. doi: 10.1126/science.271.5245.43
Sternlicht, M. D., and Werb, Z. (2001). How matrix metalloproteinases regulate cell behavior. Ann. Rev. Cell Dev. Biol. 17, 463–516. doi: 10.1146/annurev.cellbio.17.1.463
Stevens, M. M., and George, J. H. (2005). Exploring and engineering the cell surface interface. Science 310, 1135–1138. doi: 10.1126/science.1106587
Svedhem, S., Dahlborg, D., Ekeroth, J., Kelly, J., Hook, F., and Gold, J. (2003). In situ peptide-modified supported lipid bilayers for controlled cell attachment. Langmuir 19, 6730–6736. doi: 10.1021/la034172w
Tabaei, S. R., Choi, J. H., Zan, G. H., Zhdanov, V. P., and Cho, N. J. (2014). Solvent-assisted lipid bilayer formation on silicon dioxide and gold. Langmuir 30, 10363–10373. doi: 10.1021/la501534f
Tseng, P. Y., and Chang, Y. C. (2012). Tethered fibronectin liposomes on supported lipid bilayers as a prepackaged controlled-release platform for cell-based assays. Biomacromolecules 13, 2254–2262. doi: 10.1021/bm300426u
Vafaei, S., Tabaei, S. R., Biswas, K. H., Groves, J. T., and Cho, N. J. (2017a). Dynamic cellular interactions with extracellular matrix triggered by biomechanical tuning of low-rigidity: supported lipid membranes. Adv. Healthcare Mater. 6, 1700243–1700250. doi: 10.1002/adhm.201700243
Vafaei, S., Tabaei, S. R., and Cho, N. J. (2017b). Optimizing the performance of supported lipid bilayers as cell culture platforms based on extracellular matrix functionalization. Acs Omega 2, 2395–2404. doi: 10.1021/acsomega.7b00158
Vafaei, S., Tabaei, S. R., Guneta, V., Choong, C., and Cho, N. J. (2018). Hybrid biomimetic interfaces integrating supported lipid bilayers with decellularized extracellular matrix components. Langmuir 34, 3507–3516. doi: 10.1021/acs.langmuir.7b03265
Xiao, S. J., Textor, M., Spencer, N. D., and Sigrist, H. (1998). Covalent attachment of cell-adhesive, (Arg-Gly-Asp)-containing peptides to titanium surfaces. Langmuir 14, 5507–5516. doi: 10.1021/la980257z
Yoshina-Ishii, C., Miller, G. P., Kraft, M. L., Kool, E. T., and Boxer, S. G. (2005). General method for modification of liposomes for encoded assembly on supported bilayers. J. Am. Chem. Soc. 127, 1356–1357. doi: 10.1021/ja043299k
Keywords: supported lipid bilayer, extracellular matrix, cell-materials interactions, biomimetic culture platform, bioconjugation
Citation: Huang C-J and Chang Y-C (2019) Construction of Cell–Extracellular Matrix Microenvironments by Conjugating ECM Proteins on Supported Lipid Bilayers. Front. Mater. 6:39. doi: 10.3389/fmats.2019.00039
Received: 31 August 2018; Accepted: 20 February 2019;
Published: 19 March 2019.
Edited by:
Nam-Joon Cho, Nanyang Technological University, SingaporeReviewed by:
Hitoshi Washizu, University of Hyogo, JapanHock Jin Quah, Universiti Sains Malaysia, Malaysia
Copyright © 2019 Huang and Chang. This is an open-access article distributed under the terms of the Creative Commons Attribution License (CC BY). The use, distribution or reproduction in other forums is permitted, provided the original author(s) and the copyright owner(s) are credited and that the original publication in this journal is cited, in accordance with accepted academic practice. No use, distribution or reproduction is permitted which does not comply with these terms.
*Correspondence: Chun-Jen Huang, cjhuang@ncu.edu.tw
Ying-Chih Chang, yingchih@gate.sinica.edu.tw