- State Key Laboratory of Heavy Oil Processing, College of Chemical Engineering, China University of Petroleum (East China), Qingdao, China
The transformation of ethanol to propylene (ETP) was investigated over H-ZSM-5 (40) and H-LEV (40) catalysts. For H-ZSM-5 (40), the propylene yield kept constant at about 20.0% during 8 h. For H-LEV (40), higher initial propylene yield reached 34.0%. However, there is almost no propylene obtained over H-LEV (40) catalyst after 2 h. H-ZSM-5 (40) catalyst exhibited higher stability than H-LEV (40). The lower stability of H-LEV (40) is probably due to coke deposition. The reactant and products adsorption performances in the ethanol conversion reaction over H-ZSM-5 (40) and H-LEV (40) catalysts were studied by Monte Carlo simulations. Results show that the higher adsorption amount of ethanol, ethylene and propylene in H-LEV (40) led to the more difficult desorption of products and higher content of coke deposition.
Introduction
Propylene is one of the most important chemicals, which could obtain from several routes such as steam cracking of naphtha, fluid catalytic cracking (Ren et al., 2006), and MTP (methanol to propylene). These processes depend on the fossil resources and confront environmental problems heavily. Bioethanol is considered to be one of the most prospective renewable resources for the production of ethylene and propylene (Parajuli et al., 2017). Thus, the conversion of ethanol to propylene (ETP) attracts broad attention in the academic and industrial fields (Huangfu et al., 2016; Xia et al., 2017a,b). The research work on the conversion of ETP over zeolite and transition metal oxide catalysts were reviewed by Li. The existing researches show that ZSM-5 is the best candidate for propylene production (Li et al., 2016). Levyne (LEV), which belongs to CHA type zeolite, exhibit the specific shape selectivity for the transformation of methanol or ethanol (Venkatathri and Yoo, 2008; Inoue et al., 2009; Bhawe et al., 2012). The optimum selectivity of ethylene (43.0%) and propylene (32.0%) from methanol over LEV catalysts was reported by Bhawe group (Bhawe et al., 2012). Venkatathri et al. synthesized LEV catalyst and investigated the conversion of methanol to olefin (MTO) over synthesized LEV catalysts. Higher selectivity of light olefins was obtained over LEV than that over SAPO-34 catalyst (Venkatathri and Yoo, 2008). Inoue et al. also reported that 35.8% of C2H4 and 34.4% of C3H6 were acquired over LEV catalyst for the transformation of ethanol to olefins (Inoue et al., 2009).
The adsorption of reactants and products could influence the catalytic selectivity and stability in the heterogeneous catalytic reaction. However, it is very difficult to interpret the adsorption processes of guest molecules in the host zeolites through only experiment. Molecular simulation is a valuable method to simulate the motion behavior of molecules by theoretical calculation. Much more particular properties, such as the energy and density distribution of components in zeolite, could also be achieved from the molecular simulation. To investigate the adsorption and diffusion of adsorbate in different porous materials, molecular simulation is widely used (Klemm et al., 1998; Hansen et al., 2005; Navarro et al., 2010). Many researchers have studied the adsorption behavior of different systems in zeolite. Zhang et al. studied gas permeation of propane and propylene across ZSM-5 membranes by a non-equilibrium molecular dynamics technique (Zhang et al., 2003). The adsorption and diffusion behavior of ethanol and water through silicalite membranes was also reported by Yang et al. using molecular simulation method (Yang et al., 2007). Phenol obtained from benzene catalyzed by ZSM-5 type zeolite was investigated by Klemm et al. The strong adsorption of phenol should be inhibited to prevent the formation of coke (Klemm et al., 1998). The adsorption and diffusion were examined regarding the conversion of methanol to ethylene in HZSM-5 (300) and HZSM-5 (30) by (Chatterjee and Chatterjee, 2008). Adsorption amount is the most important physical quantity in the adsorption study. In the process of ETP reaction, the adsorption of reactants and products play an important role. Thus, in order to understand the ZSM-5 and LEV catalytic performance for ETP reaction, it is of great significance to study the adsorption behavior of ethanol and main products. There are a few researches which combined the adsorption of light olefins in zeolite with the product distribution in the process of catalytic reaction (Yang et al., 2007).
Transformation of ETP was systematically investigated over H-ZSM-5 (40) and H-LEV (40) with time-on-stream. Monte Carlo simulations are used to shed light on the effects of zeolite on their adsorption performance in the ETP reaction. The adsorptions of ethanol and light olefins molecules in H-ZSM-5 (40) and H-LEV (40) are calculated by Monte Carlo simulations.
Materials and Methods
Catalytic Apparatus and Ethanol Transformation Reaction
The H-LEV and H-ZSM-5 catalysts (Si/Al ratio of 40) were obtained from Zeolyst International, which were calcined in air for 4 h at 873 K. The heating rate is 4.5 K/min. The calcined H-LEV and H-ZSM-5 samples were named H-LEV (40) and H-ZSM-5 (40), respectively. Ethanol (99.5%, Wako) was used without further purification. The ETP reactions were carried out in a fixed-bed quartz tubular reactor at atmospheric pressure. Details of the catalytic reaction process can be found in our previous report (Wang et al., 2018).
A TG/DTA apparatus (SSC/5200 Seiko Instruments) was used to measure the coke deposition (airflow 50 mL/min from 323 to 973 K with a heating rate of 10 K/min). The amounts of coke deposition were calculated as follows:
Where mfresh and mused were the mass of fresh catalyst and the mass of used catalyst, respectively.
Molecular Simulations
The structures of H-LEV (40) and H-ZSM-5 (40) are present in Figure 1, which are obtained from International Zeolite Association. Simulations were performed in a simulation box of 2*2*2 unit cell. For ZSM-5 and LEV catalysts, initial frameworks include oxygen and silicon atoms. In order to obtain the structure of Si/Al atomic ratio = 40, some silicon atoms are replaced by aluminum atoms basing on the Lowenstein rule (Jakobtorweihen et al., 2005). H+ extra-framework cations were used to compensate the negative charge, which was introduced by taking place of Si by Al. Generally, extra-framework cations are located around oxygen atoms neighboring aluminum atoms, therefore, their positions are determined by the location of Al atoms (Beerdsen et al., 2002; Mentzen et al., 2006). Forcite module of Materials Studio 8.0 package was firstly used to geometrically optimize the structure.
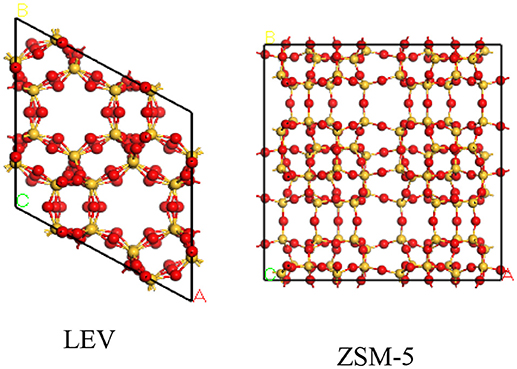
Figure 1. Structures of Lev and ZSM-5. The yellow and red balls denote Si and O atoms, respectively.
The GCMC method is carried out by the sorption module in Materials Studio 8.0 simulation package to simulate the adsorption process. The distribution of movements was 40% exchange, 20% rotation, 20% conformation, and 20% translation in each MC simulation. The COMPASS forcefield was used in this study. The electrostatic and van der Waals potential energy were calculated by the Ewald summation and the atom based technique, respectively. Lennard-Jones potentials were used to present the interactions between atoms. The production steps were set to 2 * 106 and the equilibration steps were set to 2 * 106 Monte Carlo steps. To build a homogeneous solid, periodic boundary conditions are adopted in three directions. A cut-off of 12.5 Å is used to the Lennard–Jones interactions. Ewald sum was used to calculate the long-range electrostatic interactions. The simulated value of heat of adsorption was compared with the experimental ones to check the accuracy of interaction parameters. The calculated isosteric heat of adsorption for ethylene in ZSM-5 is 31.8 KJ·mol−1 at 303 K; the available experimental data is 24.0–32.7 KJ·mol−1 (Jakobtorweihen et al., 2005). The adsorption isotherms and isosteric heat of ethylene on ZSM-5 zeolite at 303 K have been calculated to verify the rationality of model construction and simulation parameters.
Results and Discussion
Catalytic Performance
The transformation reaction of ETP over H-ZSM-5 (40) and H-LEV (40) catalysts were systematically investigated. Catalyst stability results are shown in Figure 2. For two catalyst samples, the conversions of ethanol were 100% during the whole time on stream. For H-ZSM-5 (40), the propylene yield gradually decreased from 20.0 to 17.8%. The C4 olefin yield also slowly decreased from 16.0 to 13.2% (as shown in Figure 2A). For H-LEV (40), the initial propylene yield reached 34.0% and sharply decreased to 4.0% after 2 h. There is almost no propylene produced after 2 h. The C4 olefins yield sharply decreased from 19.3 to 0.0%, ethylene yield quickly increased from 7.4 to 94.4% with time on stream from 0.5 to 2 h (as shown in Figure 2B). The formation of ethylene seems not influenced by catalyst deactivation. It could be explained that ethylene formed at weak acidic sites, and the formation of other products such as propylene requires stronger acidic sites (Aguayo et al., 2002). The production distribution could be strongly affected by the catalyst deactivation, especially coke deposition (Inoue et al., 2009). Figure 3 shows the different coke deposition content over the two catalysts. As shown in Figure 3, the coke deposition content on H-LEV (40) is much higher than that on H-ZSM-5 (40). The coke deposition content of H-LEV (40) increased sharply during 2 h, after that the trend of growth become gentle to steady state with time on stream. Due to the high content of coke deposition on H-LEV (40) at the beginning of reaction, the catalytic performance of H-LEV (40) decreased quickly. It is for this reason that the yield of propylene decreased sharply with time on stream.
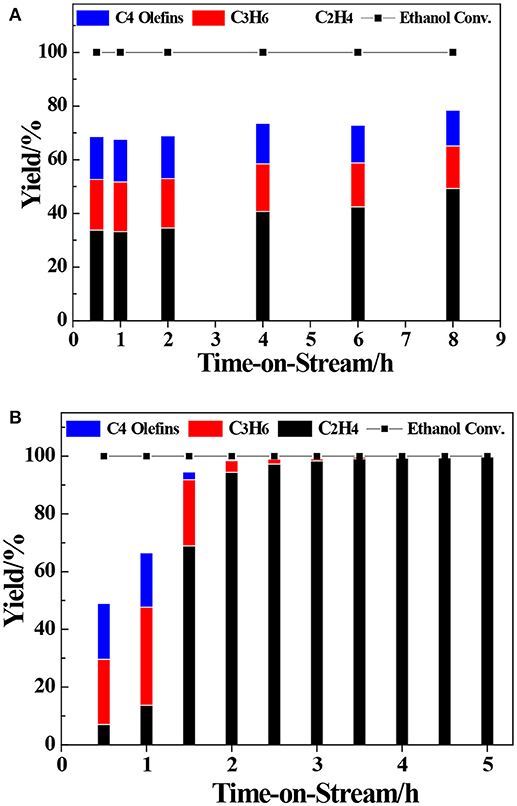
Figure 2. Product distributions of ethanol conversion over ZSM-5 and LEV with time on stream. (A) H-ZSM-5 (40), (B) H-LEV (40), Reaction conditions: 673 K, 0.1 MPa, W/F = 0.05 g.min.mL−1.
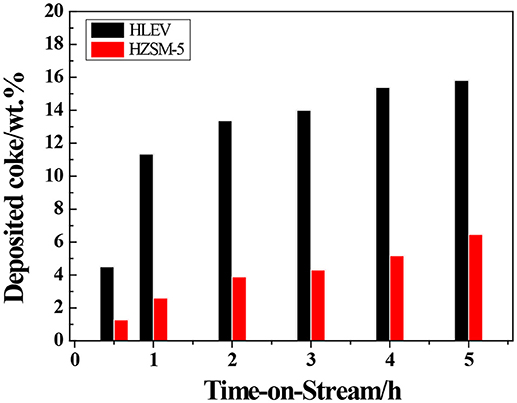
Figure 3. Evolution of coke deposited over H-ZSM-5 (40) and H-LEV (40) with time on stream. Reaction conditions: 673 K, 0.1 MPa, W/F = 0.05 g.min.mL−1.
Results of Molecular Simulation
The catalytic performance could be strongly affected by the adsorption of the guest molecule in the host zeolite. Under the same ETP reaction condition, reactant (ethanol) and products (ethylene and propylene) adsorptions in the H-ZSM-5 (40) and H-LEV (40) structure models were investigated through Monte Carlo simulations. The adsorption amounts of ethanol, ethylene, and propylene in zeolites at different temperatures were shown in Figures 4, 5, 6, respectively. Ethanol, ethylene, and propylene have obvious adsorption on H-ZSM-5 (40) and H-LEV (40) structure models. All of the ethanol, ethylene, and propylene adsorption amounts decreased with increasing of temperature for any one component. According to product of propylene, under certain conditions, higher reaction temperature favors the ETP reaction. As shown in Figures 4, 5, 6, all of the ethanol, ethylene, and propylene have higher adsorption amounts in H-LEV (40) than that in H-ZSM-5 (40). Under same conditions, the ethanol adsorption capacity on molecular sieves is obviously higher than those of ethylene and propylene. This is mainly due to the larger molecular weight of ethanol, the dipole distance is 1.68D, and the greater polarizability. The adsorption amounts of ethanol, ethylene and propylene obviously decreased with temperature increasing over H-ZSM-5 (40) and H-LEV (40). The adsorptive molecules easily break away from intramolecule bound with the increase of molecular energy, thus the amount of adsorption amounts decreased (Smit and Maesen, 2008). Under the same conditions, the order of adsorption amount of ethanol, ethylene, and propylene on molecular sieve is H-LEV (40) > H-ZSM-5 (40). It is favorable for the desorption of reactant and products from H-ZSM-5 (40). Propylene has larger adsorption amount in H-LEV (40) and H-ZSM-5 (40) than ethylene. It is favorable for the desorption of ethylene from H-LEV (40) and H-ZSM-5 (40) than propylene. Therefore, two kinds of zeolite catalysts are all helpful to improve selection of ethylene in ETP reaction. On the H-ZSM-5 (40), the adsorption amounts of products are less than that on H-LEV (40). These products could desorb quickly which restrain the polymerization and dimerization reactions, which is the main reason of coking deposition (Huang et al., 2015). The higher adsorption amount of products in the structure model of H-LEV (40) led to the higher amount of coke deposition, which is the main factor influencing the product distribution.
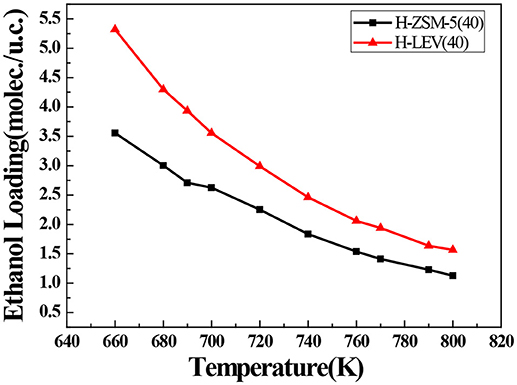
Figure 4. Ethanol adsorption amounts in H-ZSM-5 (40) and H-LEV (40) with different temperatures under 100 kPa.
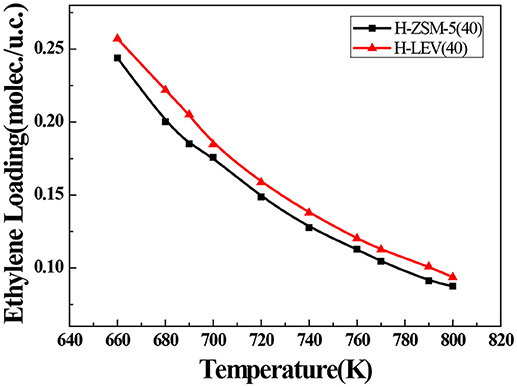
Figure 5. Ethylene adsorption amounts in H-ZSM-5 (40) and H-LEV (40) with different temperatures under 100 kPa.
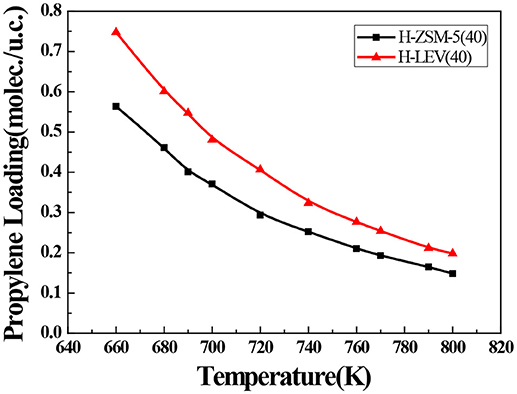
Figure 6. Propylene adsorption amounts in H-ZSM-5 (40) and H-LEV (40) with different temperatures under 100 kPa.
Figures 7, 8, 9 show the various density distributions of ethanol, ethylene, and propylene in the model at 680 K, 100 kPa over the two zeolite catalysts, respectively. As shown in Figures 7, 8, 9, ethanol, ethylene, and propylene mainly adsorbed in straight channel of H-ZSM-5 (40). Ethanol, ethylene, and propylene mainly adsorbed in the 8-membrane ring channels of H-LEV (40). The components are able to fully occupy most of the molecular sieve pore position in 10-membrane ring channel of H-ZSM-5 (40) and 8-membrane ring channel of H-LEV (40). For same component, the distribution probability density in the straight channel is higher than that in the curved channel, and the density is higher in the region closer to the center of channel. Higher adsorption amount of component, higher proportion of red region could be observed in the probability density map.
Conclusions
In this work, conversions of ETP were systematically carried out on H-ZSM-5 (40) and H-LEV (40). H-LEV (40) exhibited higher propylene yield and lower stability than H-ZSM5 (40) for ETP reaction. The molecular simulation results show that the adsorption of ethanol, ethylene and propylene in H-LEV (40) was stronger that in H-ZSM-5 (40), which led to the difficult desorption of products, high yields of by-products, and coke deposition. A strong adsorption of reactants and products in H-LEV (40) should be depressed to inhibit the coke formation.
Author Contributions
WX designed experiments. FW, LW, and JW carried out experiments. FW, XY, and KC analyzed experimental results. FW and WX wrote the manuscript.
Conflict of Interest Statement
The authors declare that the research was conducted in the absence of any commercial or financial relationships that could be construed as a potential conflict of interest.
Acknowledgments
This work is a project sponsored by the National Natural Science Foundation of China (Grant 21406269 k, 21502227), Shandong Provincial Natural Science Foundation, China (Grant ZR2014BQ012), Scientific Research Foundation for Returned Scholars, Ministry of Education of China (K1504051C), Shandong Provincial Key Research Program (Grant: 2015GSF121017), the Fundamental Research Funds for the Central Universities (15CX05013A), the International Cooperation and Exchange Fund, China University of Petroleum (East China) (UPCGJ2018010), and the Graduate Innovation Project, China University of Petroleum (East China) (YCX2018047).
References
Aguayo, A. T., Gayubo, A. G., Tarrío, A. M., Atutxa, A., and Bilbao, J. (2002). Study of operating variables in the transformation of aqueous ethanol into hydrocarbons on an HZSM-5 zeolite. J. Chem. Technol. Biotechnol. 77, 211–216. doi: 10.1002/jctb.540
Beerdsen, E., Smit, B., and Calero, S. (2002). The influence of non-framework sodium cations on the adsorption of alkanes in MFI- and MOR-type zeolites. J. Phys. Chem. B 106, 10659–10667. doi: 10.1021/jp026257w
Bhawe, Y., Moliner-Marin, M., Lunn, J. D., Liu, Y., Malek, A., and Davis, M. (2012). Effect of cage size on the selective conversion of methanol to light olefins. ACS Catal. 2, 2490–2495. doi: 10.1021/cs300558x
Chatterjee, A., and Chatterjee, M. (2008). Computational designing of gradient type catalytic membrane: application to the conversion of methanol to ethylene. Mol. Simulat. 34, 1091–1097. doi: 10.1080/08927020802225848
Hansen, N., Jakobtorweihen, S., and Keil, F. J. (2005). Reactive Monte Carlo and grand-canonical Monte Carlo simulations of the propene metathesis reaction system. J. Chem. Phys. 122:164705. doi: 10.1063/1.1884108
Huang, Y., Dong, X., Li, M., and Yu, Y. (2015). A density functional theory study on ethylene formation and conversion over P modified ZSM-5. Catal. Sci. Technol. 5, 1093–1105. doi: 10.1039/C4CY01205D
Huangfu, J., Mao, D., Zhai, X., and Guo, Q. (2016). Remarkably enhanced stability of HZSM-5 zeolite co-modified with alkaline and phosphorous for the selective conversion of bio-ethanol to propylene. Appl. Catal. A 520, 99–104. doi: 10.1016/j.apcata.2016.04.016
Inoue, T., Itakura, M., Jon, H., Oumi, Y., Takahashi, A., Fujitani, T., et al. (2009). Synthesis of LEV zeolite by interzeolite conversion method and its catalytic performance in ethanol to olefins reaction. Micropor. Mesopor. Mat. 122, 149–154. doi: 10.1016/j.micromeso.2009.02.027
Jakobtorweihen, S., Hansen, N., and Keil, F. J. (2005). Molecular simulation of alkene adsorption in zeolites. Mol. Phys. 103, 471–489. doi: 10.1080/00268970512331316021
Klemm, E., Wang, J., and Emig, G. (1998). A comparative study of the sorption of benzene and phenol in silicalite, HAlZSM-5 and NaAlZSM-5 by computer simulation. Micropor. Mesopor. Mat. 26, 11–21. doi: 10.1016/S1387-1811(98)00203-0
Li, X., Kant, A., He, Y., Thakkar, H. V., Atanga, M. A., Rezaei, F., et al. (2016). Light olefins from renewable resources: selective catalytic dehydration of bioethanol to propylene over zeolite and transition metal oxide catalysts. Catal. Today 276, 62–77. doi: 10.1016/j.cattod.2016.01.038
Mentzen, B. F., Bergeret, G., Emerich, H., and Weber, H. P. (2006). Dehydrated and Cs+-exchanged MFI zeolites: location and population of Cs+ FROM in situ diffraction data as a function of temperature and degree of exchange. J. Phys. Chem. B 110, 97–106. doi: 10.1021/jp055230k
Navarro, M. V., Puértolas, B., García, T., Murillo, R., Mastral, A. M., Varela-Gandía, F. J., et al. (2010). Experimental and simulated propene isotherms on porous solids. Appl. Surf. Sci. 256, 5292–5297. doi: 10.1016/j.apsusc.2009.12.120
Parajuli, R., Knudsen, M. T., Birkved, M., Djomo, S. N., Corona, A., and Dalgaard, T. (2017). Environmental impacts of producing bioethanol and biobased lactic acid from standalone and integrated biorefineries using a consequential and an attributional life cycle assessment approach. Sci. Total Environ. 598, 497–512. doi: 10.1016/j.scitotenv.2017.04.087
Ren, T., Patel, M., and Blok, K. (2006). Olefins from conventional and heavy feedstocks: Energy use in steam cracking and alternative processes. Energy 31, 425–451. doi: 10.1016/j.energy.2005.04.001
Smit, B., and Maesen, T. L. (2008). Molecular simulations of zeolites: adsorption, diffusion, and shape selectivity. Chem. Rev. 108, 4125–4184. doi: 10.1021/cr8002642
Venkatathri, N., and Yoo, J. W. (2008). Synthesis, characterization and catalytic properties of a LEV type silicoaluminophosphate molecular sieve, SAPO-35 from aqueous media using aluminium isopropoxide and hexamethyleneimine template. Appl. Catal. A 340, 265–270. doi: 10.1016/j.apcata.2008.02.026
Wang, F., Xia, W., Mu, X., and Chen, K. (2018). Experimental and molecular simulation studies on ethanol conversion to propylene over different zeolite catalyst. Catal. Lett. 148, 1768–1774. doi: 10.1007/s10562-018-2375-7
Xia, W., Wang, F., Mu, X., Chen, K., Takahashi, A., Nakamura, I., et al. (2017a). Catalytic performance of H-ZSM-5 zeolites for conversion of ethanol or ethylene to propylene: effect of reaction pressure and SiO2/Al2O3 ratio. Catal. Commun. 91, 62–66. doi: 10.1016/j.catcom.2016.12.010
Xia, W., Wang, F., Mu, X., Chen, K., Takahashi, A., Nakamura, I., et al. (2017b). Highly selective catalytic conversion of ethanol to propylene over yttrium-modified zirconia catalyst. Catal. Commun. 90, 10–13. doi: 10.1016/j.catcom.2016.11.011
Yang, J. Z., Liu, Q. L., and Wang, H. T. (2007). Analyzing adsorption and diffusion behaviors of ethanol/water through silicalite membranes by molecular simulation. Membr. Sci. 291, 1–9. doi: 10.1016/j.memsci.2006.12.025
Keywords: ethanol, propylene, adsorption, zeolite, Grand Canonical Monte Carlo
Citation: Wang F, Xia W, Wang L, Wang J, Yang X and Chen K (2018) Grand Canonical Monte Carlo Simulations of Ethanol Conversion to Propylene Over Zeolite Catalysts. Front. Mater. 5:64. doi: 10.3389/fmats.2018.00064
Received: 15 September 2018; Accepted: 12 October 2018;
Published: 31 October 2018.
Edited by:
Jie-Sheng Chen, Shanghai Jiao Tong University, ChinaReviewed by:
Baowang Lu, University of Toyama, Toyama, JapanShuyan Gao, Henan Normal University, China
Qiang Wu, Shanghai University of Electric Power, China
Copyright © 2018 Wang, Xia, Wang, Wang, Yang and Chen. This is an open-access article distributed under the terms of the Creative Commons Attribution License (CC BY). The use, distribution or reproduction in other forums is permitted, provided the original author(s) and the copyright owner(s) are credited and that the original publication in this journal is cited, in accordance with accepted academic practice. No use, distribution or reproduction is permitted which does not comply with these terms.
*Correspondence: Wei Xia, xiawei@upc.edu.cn