- 1Norwegian Polar Institute, Fram Centre, Tromsø, Norway
- 2Cornell Lab of Ornithology, Ithaca, NY, United States
- 3Littoral Environnement et Sociétés (LIENSs), UMR 7266 CNRS-La Rochelle Université, La Rochelle, France
- 4Institut Universitaire de France (IUF), Paris, France
- 5Centre d’Etudes Biologiques de Chizé (CEBC), UMR 7372 CNRS-La Rochelle Université, Villiers-en-Bois, France
- 6Centre for Ecology and Conservation, College of Life and Environmental Sciences, University of Exeter, Penryn, United Kingdom
- 7School of Environmental Sciences, University of Liverpool, Liverpool, United Kingdom
Individual heterogeneity in foraging behaviour determines how individuals and populations respond to changes in the availability and distribution of resources. Antarctic krill Euphausia superba is a pivotal species in Southern Ocean food webs and an important target for Southern Ocean fisheries. Changes in its abundance could dramatically impact marine predators, with effects depending on the extent to which all individuals rely on krill as prey. The Antarctic petrel Thalassoica antarctica is a high latitude seabird thought to be dependent on krill in part of its breeding range. Here, by combining fine-scale GPS tracking of petrel foraging trips with diet data, we examined the level and consistency of inter-individual variation in foraging strategies in breeding Antarctic petrels in Dronning Maud Land, Antarctica, and assessed whether all individuals share a similar reliance on Antarctic krill. We found that Antarctic petrels showed high levels of repeatability in their diet and foraging movements at sea, indicating consistent individual differences in foraging strategies. During consecutive foraging trips, petrels tend to make trips of similar lengths and durations to reach similar terminal locations and to feed on similar prey. These individual differences in diet were spatially structured, with individuals travelling towards the west consuming a more fish-based diet. These different foraging tactics did not appear to be associated with different costs and/or benefits as adult body mass, chick survival and chick growth were unrelated to birds’ foraging movements and diet. Our results show that, even if a large part of the population may be dependent on krill, some individuals specialize on fish. Such inter-individual variation in foraging suggests that this population could be more resilient to changes in the marine environment, such as a decline in krill abundance or a shift in krill distributions.
1 Introduction
Individual heterogeneity is ubiquitous in natural populations and can have a strong influence on population dynamics and ecosystem functioning (Benton et al., 2006; Bolnick et al., 2011; Kendall et al., 2011; Vindenes and Langangen, 2015). Variation among individuals in their foraging behaviour and/or resource use may, for example, lead to differences in demography (Morales et al., 2010), which can ultimately affect the population viability (Okuyama, 2008; Gibert and Brassil, 2014). Such heterogeneity in resource use can be driven by many different factors such as sex, age, and morphology, and can be consistent over various periods of time (Bolnick et al., 2003). It may be the consequence of individual resource specialization per se (i.e. individuals are specialized on specific resources among all those available) or of individual variation in foraging habitat in an environment where prey vary spatially (i.e. individuals are not specialist per se but the available resources vary among individuals). Whatever the mechanism, such inter-individual variation may affect the way populations respond to environmental perturbations and changes in resource availability (Bolnick et al., 2011; Phillips et al., 2017).
Individual heterogeneity in resource use has been described in many organisms such as marine and freshwater fish (Holbrook and Schmitt, 1992; Chavarie et al., 2021), amphibians (Lunghi et al., 2020), terrestrial and marine mammals (Estes et al., 2003; Edwards et al., 2011), and birds (Woo et al., 2008; Pagani-Núñez et al., 2015). In birds, evidence of inter-individual variation in foraging behaviour and diet exists in different taxonomic groups (e.g., waders, Van De Pol et al., 2010; passerines, De León et al., 2012; raptors, Navarro-López et al., 2014), including seabirds, where such variation seems widespread (Ceia and Ramos, 2015; Phillips et al., 2017). Seabirds, by definition, depend on the marine environment to forage. Marine food resources are generally patchily distributed, and both their composition and abundance show strong spatial variation (e.g., Godø et al., 2012; Tarling and Fielding, 2016; Bergstad et al., 2018). Such spatial heterogeneity may drive variation among individuals in their space use (Fauchald, 1999; Trevail et al., 2021) and then ultimately in their diet as a way to minimize intra-specific competition (Araújo et al., 2011). This may be especially pronounced during the breeding season when seabirds are central-place foragers and intra-specific competition for resources is intensified (Ashmole, 1963).
Understanding the extent to which individuals in a given population vary in how they use their environment is critical to evaluating the resilience of this population to environmental change. Indeed, the deterioration of a specific foraging habitat or the decline in the abundance of a specific prey will have stronger effects on a population if all individuals depend on this area or prey (Durell, 2000). Such problems are especially pertinent for populations living at high latitudes (i.e. Arctic and Antarctic) where environmental changes like climate warming, are, or are predicted to be, extremely pronounced (Intergovernmental Panel on Climate Change, 2013), with impacts on the distribution and abundance of many key prey species. For populations dependent on harvested resources, it is further necessary to address the degree of individual specialization within the population, in order to evaluate the potential population-level impacts of resource exploitation.
Here, by combining fine-scale GPS tracking with diet data, we assessed the level and consistency of inter-individual variation in foraging strategies of breeding Antarctic petrels Thalassoica antarctica in Dronning Maud Land, Antarctica. In this region, Antarctic krill Euphausia superba (hereafter krill) is the main prey for Antarctic petrels, at least during the breeding season (Lorentsen et al., 1998; Descamps et al., 2016a). Krill is a pivotal species in the Southern Ocean food webs (Trathan and Hill, 2016) and also an important target for Southern Ocean fisheries (Nicol et al., 2012). Though the status and trend of the Antarctic krill is debated (Cox et al., 2018), some studies suggest that it may be declining (Atkinson et al., 2004). It thus appears even more important to better understand the relationships between krill and Antarctic petrels, and to what extent the entire petrel population may be relying on krill during the breeding season. Antarctic petrels from this population exhibit a wide range of foraging behaviours (e.g., Tarroux et al., 2020), but it is unclear whether these differences remain consistent through time and thus represent individual foraging specialization (in terms of space use and diet). Our study aimed at evaluating the inter-individual variation in resource use in Antarctic petrels and the level of consistency (i.e. repeatability) in their foraging movements and diet. We then assessed whether individual differences in foraging were associated with fitness consequences, by looking at offspring mass and survival. The Antarctic petrel, as well as many polar seabirds (e.g., Ainley et al., 2010; Strøm et al., 2020; Trathan et al., 2020; Descamps and Strøm, 2021), has been declining (by more than 50% in the last 30 years, Descamps et al., 2016b and unpublished data) and to better understand the individual variation in their foraging ecology, and more specifically in their dependence on krill, is especially important in a context of increasing krill fisheries (CCAMLR, 2021).
2 Methods
2.1 Study System
The Antarctic petrel is a medium-sized petrel weighing ca. 600 g that lays one egg between late November and early December. The incubation is shared by both parents and each incubation shift lasts for one to three weeks (Lorentsen and Røv, 1995). After hatching (mid-January), the chick is guarded for another two weeks (Lorentsen and Røv, 1995). In this period, foraging trips gradually shorten until the chick is left unattended for the first time (end of January). From this point, both parents feed their chick until it fledges at 6-7 weeks of age (early March). During the breeding season, the most common prey is Antarctic krill that represents about two third of the diet by mass on average, but fish (e.g. Antarctic lanternfish Electrona antarctica, and Antarctic silverfish Pleuragramma antarcticum) are also important prey items and represent on average 30% of the diet (Lorentsen et al., 1998; Descamps et al., 2016a). Adult petrels were captured at the Svarthamaren colony (71°53’S, 5°10’E) in Dronning Maud Land, Antarctica (Mehlum et al., 1988). This colony is located ca. 200 km inland and hosts between 20,000 and 200,000 pairs of Antarctic petrels depending on years (Descamps et al., 2016b). All captured birds and their chick were weighed at logger deployment and retrieval. Nests were also monitored (i.e. presence/absence of the chick) from logger deployment (17th-22nd January) until 9th-11th February (i.e. last nest visit). Hatching dates of these nests, and thus chick age, were unknown. All captures, sampling and logger deployments were carried out in accordance with the permit provided by the Norwegian Animal Welfare Authority (Mattilsynet, permit no.7935).
2.2 Tracking Data
Breeding adults were captured on their nest during chick-rearing and equipped with GPS loggers (CatTrack 1, Catnip Technologies Ltd., Anderson, USA). Loggers were deployed in period 17th-22nd January 2016 and retrieved in period 21st January-7th February. The GPS units weighed approx. 20 g (ca. 3% of bird body mass) and were taped to tail feathers (using Tesa tape). Additional details about the procedure are given in Tarroux et al. (2016; 2020). Birds were recaptured upon return to their nests to retrieve the loggers and download the data. The loggers recorded the locations of the birds along their foraging trip at 30 min intervals. We deployed 91 loggers and retrieved 41 (5 to 20 days after deployment; median: 12 days); the remaining 50 loggers were either lost by the birds or birds could not be recaptured. Three loggers did not work properly and so did not provide data. One individual’s breeding attempt failed after logger deployment but before departure and was excluded from the analyses. In total, data from 37 loggers were included in this study.
We considered a potential foraging trip as a round trip starting or ending when the bird left or entered, respectively, a 5 km-buffer area around the colony (defined as a single point at coordinates 71°53’S, 5°10’E), and reached the sea ice edge (approx. 200 km from the colony). We consequently excluded three short inland trips where birds reached a maximum distance of <25 km from the colony, which likely constitute local flights around the colony. We considered five variables describing each foraging trip: the trip duration, the total distance travelled, the maximum distance from the colony and the coordinates (latitude/longitude) of the farthest point. One trip was incomplete, but the bird was returning towards the colony and its last recorded position was <50 km from the colony (after reaching a maximum distance of almost 600 km from the colony). We thus included this trip in our analyses of maximum distance from the colony and the latitude/longitude of the farthest point, but not in our analyses of trip duration and total distance travelled. In total we recorded 80 foraging trips from the 37 tracked individuals (11 individuals with 1 trip, 12 with 2 trips, 11 with 3 trips and 3 with 4 trips). Data are given in the Supplementary Table 1.
2.3 Stable Isotopes and Diet Data
We collected ca. 0.3 ml of blood from a subsample of 21 individuals when recovering their GPS unit. Samples were collected from the brachial vein (using heparinized syringes with a 26G needle) and stored in heparinized tubes. Blood samples were centrifuged within 8 hr after collection at 10,000 rpm for 10 minutes. Plasma and blood cell samples were then frozen until stable isotope analyses at the LIENSs laboratory, La Rochelle University (France). Samples were used to determine the stable isotope ratios of nitrogen (δ15N), a proxy of the trophic position with higher values indicating higher position (Newsome et al., 2007; Hussey et al., 2014). The stable isotope ratios of carbon (δ13C) were also analysed (data available in the Supplementary Table 1) but were not included in the present study. δ13C values are generally used to identify bird foraging areas but in our case, as birds were GPS-tracked, examining variation in δ13C values would not provide any significant additional information.
Stable isotopes integrate dietary information over different time scales depending on the tissue type (Bearhop et al., 2004). Stable isotopes in plasma reflect diet integrated several days prior to sampling (and thus, the diet during the last foraging trip before sampling) whereas in blood cells, they reflect longer term integrated diet (i.e., several weeks, Hobson and Clark, 1993). As plasma and red blood cells were extracted from the same blood sample for a given individual, their respective isotopic values were not completely independent. However, given that red cell isotopic values reflect the bird diet over the 3-4 weeks preceding sampling, while plasma isotopic values reflect diet over the 3-5 days prior to sampling (Hobson and Clark, 1993), the red cell isotopic values should only be marginally affected by what the bird ate in the few days before sampling, and the red cell and plasma isotopic values should thus be largely independent.
Subsamples (~0.3 mg) of plasma and blood cells were packed into tin cups and their relative abundance of N isotope were determined with a continuous flow mass spectrometer (Thermo Scientific Delta V Advantage) coupled to an elemental analyzer (Thermo Scientific Flash EA 1112). Stable isotope concentrations were expressed in conventional δ notation (δX = [Rsample/Rstandard) - 1] x 1000) where X is 15N and R is 15N/14N and Rstandard is the atmospheric N2. Replicate measurements of internal laboratory standards (acetanilide) indicated measurement errors <0.15 ‰.
Additionally, we collected in the same period (Jan/Feb 2016) the stomach content of 10 individuals by stomach lavage (Wilson, 1984), five of them being from tracked individuals when recovering their GPS unit. Collection took place immediately after the return of the bird to its nest from a foraging trip and before it started feeding its chick. Stomach contents were immediately frozen and later transferred to the laboratory for taxonomic analysis, following Cherel et al. (2002). Prey was identified using published keys and descriptions and by comparison with material held in our reference collections.
2.4 Statistical Analyses
All statistical analyses were done with R software version 4.0.2 (R Development Core Team, 2020).
2.4.1 Individual Consistency in Foraging Behaviour
We first quantified repeatability in movement metrics (trip duration, total distance travelled, maximum distance travelled, latitude and longitude of the farthest location), defined as the proportion of variation in a given trait that can be attributed to between-individual variation (Lessells and Boag, 1987; Nakagawa and Schielzeth, 2010). We used a linear mixed model approach using the rpt() function of the rptR package (Stoffel et al., 2017). We included logger deployment date as a fixed effect and thus estimated adjusted repeatabilities (Nakagawa and Schielzeth, 2010). All individuals, including those tracked during only one foraging trip, were included in the repeatability estimations in order to base these estimations on the best possible estimate of the among-individual variance in the traits considered. All metrics followed a Gaussian distribution, except the longitude of the farthest location which was left-skewed [and was thus transformed as (Longitude+30)2]. The 95% confidence intervals of repeatability coefficients were estimated using parametric bootstrapping with 1000 bootstraps (Stoffel et al., 2017).
In a second step, we estimated the consistency in individual diet (equivalent to the level of individual specialization in diet) by quantifying the correlation (Pearson’s r) between plasma and blood cell isotopic values. Indeed, as these tissues have different turn-over rates (several weeks for blood cells and several days for plasma), a strong correlation between stable isotopic ratios in blood cells and plasma would indicate some level of temporal consistency in individuals’ diet, or more exactly its trophic position (Bond et al., 2016; Cherel et al., 2018).
2.4.2 Relationships Between Spatial Distribution and Diet
We tested in a third step whether or not the spatial distribution of petrels (i.e. their farthest latitude and longitude) was associated with their diet. We first used a linear model with δ15Nplasma as the response variable and Longfarthest location and Latfarthest location as the explanatory variables. Visual inspection of model residuals indicated that they were normally distributed and homoscedastic. For each individual, we included only the foraging trip made immediately prior to blood sampling (i.e. only one trip per individual) and the δ15Nplasma value is expected to represent the diet during this single trip (i.e., these foraging trips averaged 4.6 days ± 1.6 SD, which corresponds to the expected plasma turn-over). Then, using the same data, we performed a cluster analysis based on the distance between the farthest locations of each foraging trip to group individuals into categories representing their space use, and compared the mean δ15Nplasma value among these groups. We used the distm function (from package geosphere, Hijmans et al., 2017) to generate a geodesic distance matrix between these farthest locations and the hclust and cutree functions to define clusters, using an arbitrary distance threshold (i.e. cut-of value for the height of the cluster dendrogram) of 400 km (which corresponds to scale of the foraging areas used by Antarctic petrels; Fauchald and Tveraa, 2006). This latter analysis was used to confirm the previous results based on a linear regression and to give a more explicit spatial description of the differences among individuals in their diet but did not intend to identify the optimal number of clusters.
Results from stomach samples were further used to aid interpretation of results from stable isotope analyses, but sample size was too low (n=5) to test for a link with the spatial distribution of birds.
2.4.3 Consequence of Individual Foraging Strategies on Adult Mass, Chick Mass and Chick Survival
To test for the effect of petrel foraging strategies (movement metrics and diet) on petrel body mass chick mass and survival, we used linear models with either adult mass, chick mass or chick survival as response variables. For both adult and chick mass we considered mass measurements on adults’ return to the nest. We used a Gaussian distribution for mass variables and a binomial distribution with a logit-link function for chick survival. The chick survival was defined based on the last nest visit done in period 9th-11th February. For the mass analyses, we included in the models the adult or chick mass at deployment, as well as the logger deployment date as covariates. For the chick survival analysis, we also included the deployment date as a covariate. Chick age was unknown and could thus not be included in models.
We first tested for the effect of the mean distance travelled per foraging trip and the mean coordinates of the farthest locations on these three dependent variables (adult mass, chick mass and chick survival). We did not include the mean trip duration and mean maximum distance from the colony as these two variables were highly correlated with the mean distance travelled (Pearson’s r=0.85 and 0.73, respectively). These analyses were based on all 37 tracked individuals. We then tested in separate models for the effect of δ15Ncells (i.e. a proxy of the average trophic position of the birds during the previous weeks) on the three previously described dependent variables. We used separate models to test for an effect of δ15Ncells because isotope data were only available for a subsample of tracked individuals (n=21 individuals).
3 Results
3.1 Individual Consistency in Foraging Behaviour
Antarctic petrel movement metrics were repeatable (Table 1 and Supplementary Figure 1) indicating that Antarctic petrels show substantial levels of individual consistency in their movements at sea when foraging. More specifically, individuals that travelled far from the colony and/or that had a long foraging trip tend to do the same during consecutive trips. The highest repeatability was obtained for the coordinates (latitude and longitude) of the farthest positions (Table 1) suggesting that birds were generally consistent in their most distant foraging areas.
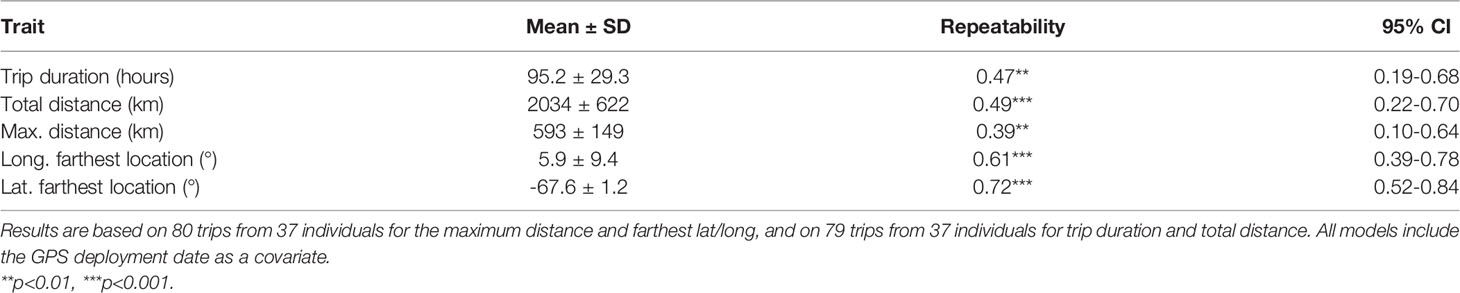
Table 1 Adjusted repeatability in movement metrics in the Antarctic petrel (data from Jan-Feb 2016, Svarthamaren, Dronning Maud Land).
Antarctic petrels did not all feed on the same prey (range of δ15N values in plasma and blood cells, respectively: 8.44-12.36 and 8.81-10.18 ‰; Fig.1) and our results suggest some level of individual specialization in diet. Individuals’ plasma (mean ± SD: 9.71 ± 1.27 ‰) and blood cell (mean ± SD: 9.46 ± 0.35 ‰) δ15N values were highly correlated (Pearson’s r=0.75, 95%CI=[0.47, 0.89], p<0.001]; Figure 1) indicating that individuals were consistently foraging on high, or low, trophic level prey. The relationship between δ15N in plasma and blood cells shows an increase in the δ15N values in the plasma of the individuals that already had higher δ15N values in their blood cells (slope higher than one, Figure 1). This suggests that those individuals were feeding on higher trophic levels during their last foraging trip as compared to the trips occurring in the previous weeks.
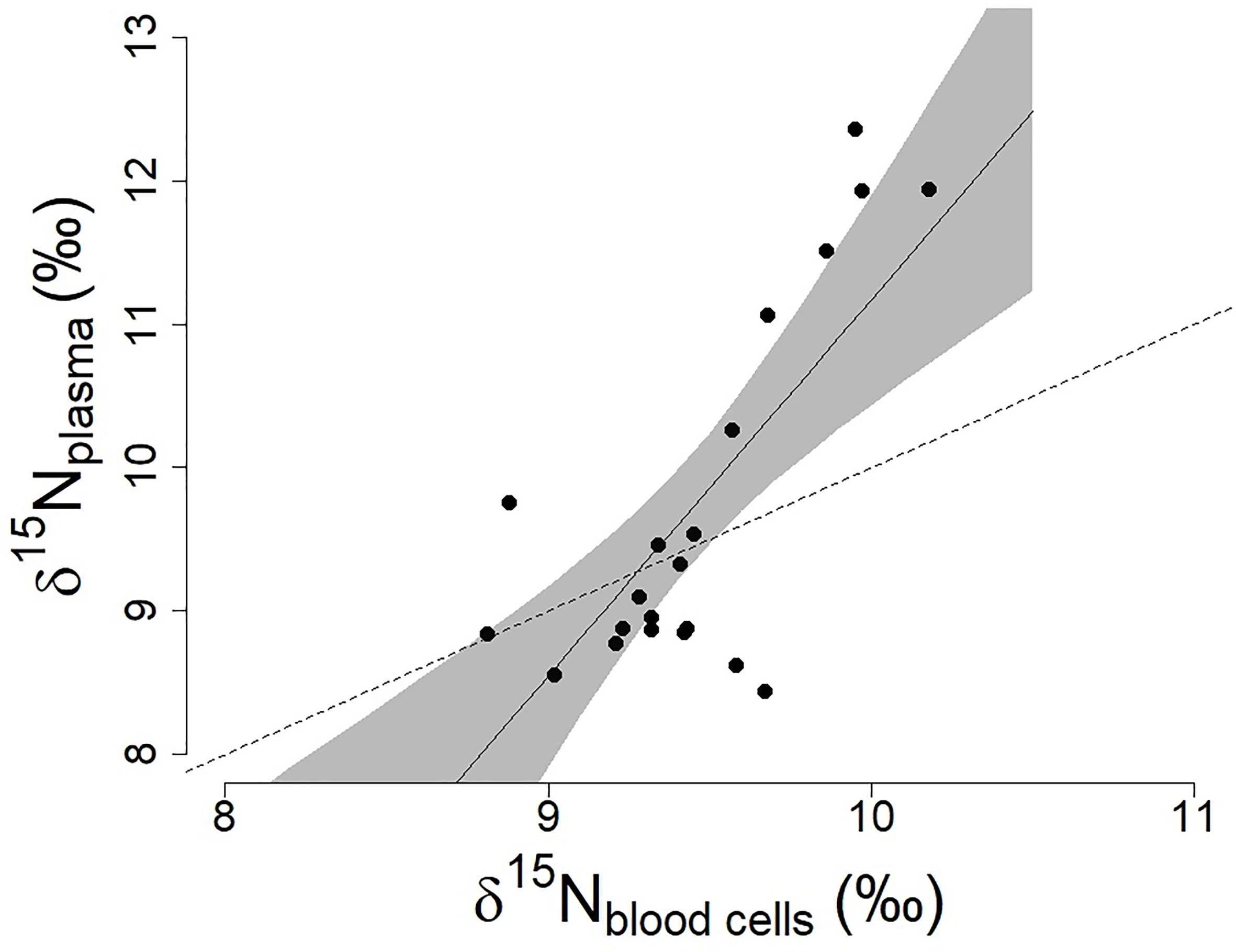
Figure 1 Relationships between δ15N values in plasma and blood cells in the Antarctic petrel (n=21; data from Jan-Feb 2016, Svarthamaren Dronning Maud Land). The regression line and associated 95% confidence interval (shaded area) are from the single regression model δ15Nplasma ~ δ15Nblood cells (β ± SE = 2.70 ± 0.55, t=4.90, p<0.001). The dotted line represents the regression with a slope equal to 1.
3.2 Relationships Between Spatial Distribution and Diet
The δ15N value of Antarctic petrel was significantly associated with their movements at sea and individuals travelling towards more eastern longitudes were characterized by lower δ15N values (effect of the longitude of the farthest position: β=-0.09 ± 0.03 SE, t=-3.58 p=0.002; Figure 2). The effect of the latitude was negative (lower δ15N values at higher latitudes) but only marginally significant once the longitude effect was taken into account (effect of the latitude of the farthest position: β=-0.41 ± 0.20 SE, t=-2.03, p=0.06).
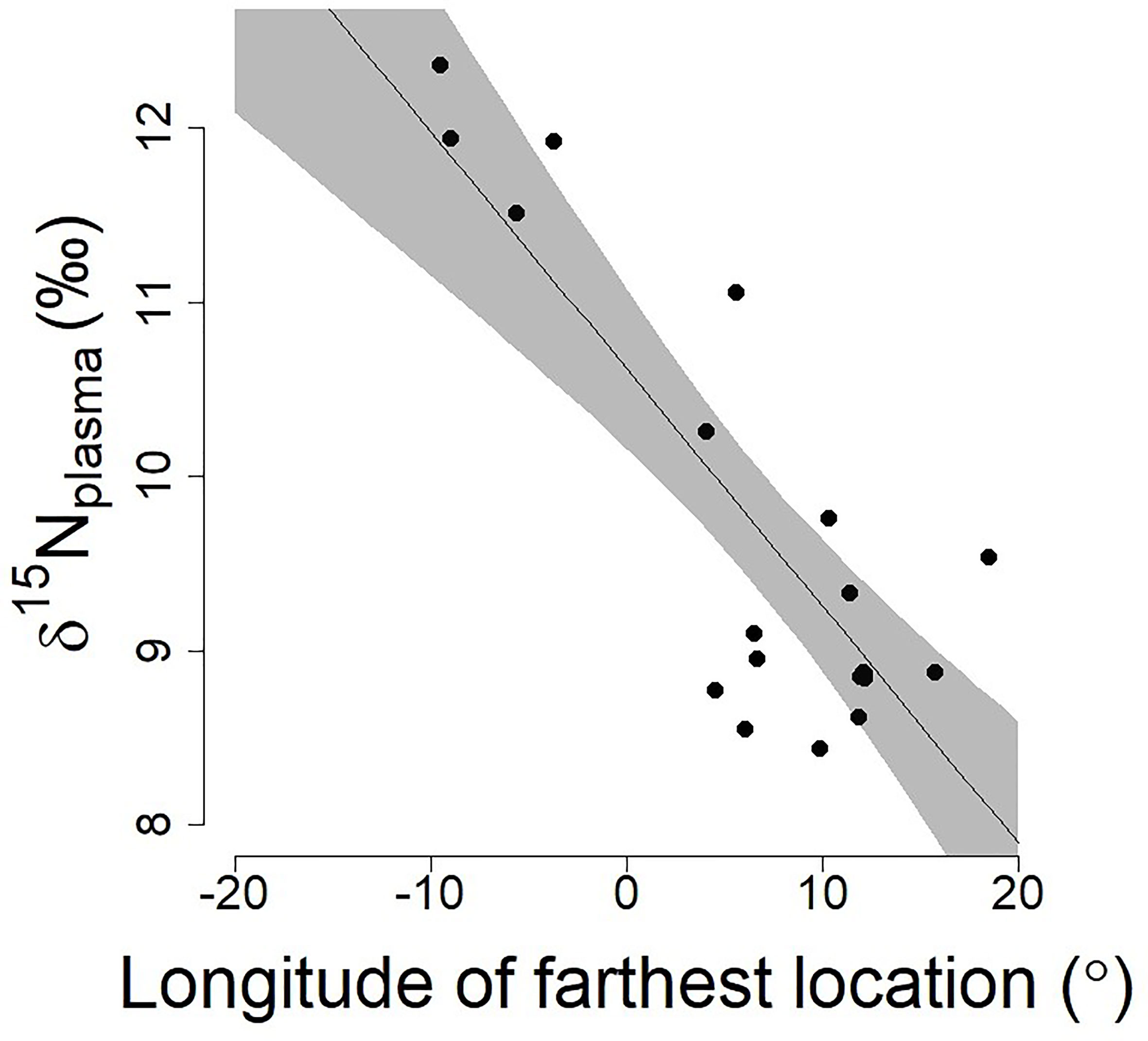
Figure 2 Relationships between the longitude of the farthest position during a foraging trip and the corresponding δ15N plasma values in chick-rearing Antarctic petrels (n=21; data from Jan-Feb 2016, Svarthamaren Dronning Maud Land). The regression line and associated 95% confidence interval (shaded areas) are from a single regression model: (δ15Nplasma ~ Long farthest location, β ± SE = -0.13 ± 0.02, t=-6.24, p<0.001; R2 = 0.67).
A cluster analysis based on the farthest locations from the colony indicated three different clusters (Figure 3). Petrels that travelled towards the “central” and “east” clusters (Figure 3) had similar mean δ15Nplasma values equal to 9.5 ‰ (95%CI=[8.7, 9.2]) and 9.0 ‰ (95%CI=[8.8, 9.3]), respectively. The mean δ15Nplasma value of those that travelled towards the west was higher and equal to 11.9 ‰ (95%CI=[11.6, 12.3]; Figure 2).
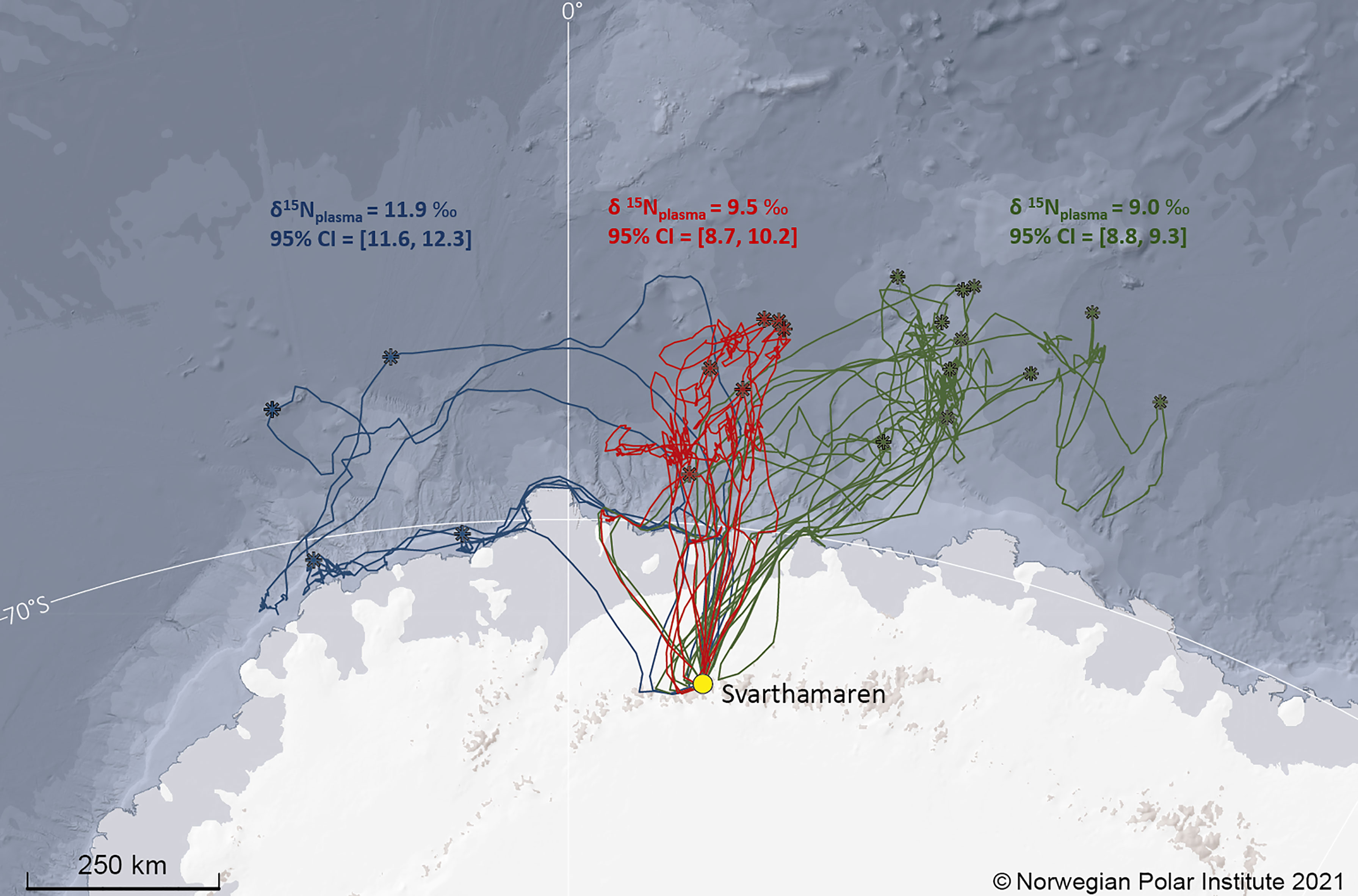
Figure 3 Foraging trips of Antarctic petrels in January-February 2016 (n=21 trips from 21 individuals for which blood was sampled at return to the colony for stable isotope analyses). The breeding colony, Svarthamaren, is indicated by a yellow dot. The farthest position from the colony for each foraging trip is indicated by a star. The three colours represent the three clusters of Antarctic petrels based on their farthest positions. The mean (and associated 95% confidence interval) δ15Nplasma value is indicated for each group using the same colour code.
Stomach samples revealed a high proportion of fish (72% on average of the wet biomass of the samples, mostly the neritic Pleuragramma antarcticum, and the oceanic Notolepis coatsi and Electrona antarctica) and a low proportion of crustaceans (dominated by krill; 26% of the wet biomass) in Jan/Feb 2016 (n=10). While fish appeared to be the dominant prey in 2016, results from stomach content of tracked individuals (representative of the diet of their chick) match results from stable isotopes (that are representative of adult diet) and indicate that the proportion of fish in the diet was higher and the proportion of krill lower in birds that travelled towards the west. If we consider the same areas as the ones depicted in Figure 2, we found that food samples of birds travelling towards the east were composed of 27% krill and 71% fish (n=3; range for the % of krill and fish respectively: [5, 50] and [50, 88]), whereas for birds travelling towards the west, stomach contents were comprised of 1% krill and 97% fish (n=2; range for the % of krill and fish respectively: [1, 2] and [95, 99]).
3.3 Consequence of Individual Foraging Strategies on Adult Mass, Chick Mass and Chick Survival
After adjusting for the logger deployment date and mass at deployment, we found that petrel movement and diet metrics were not significantly associated with the adult or chick mass at return, nor with the chick survival probability (Table 2).
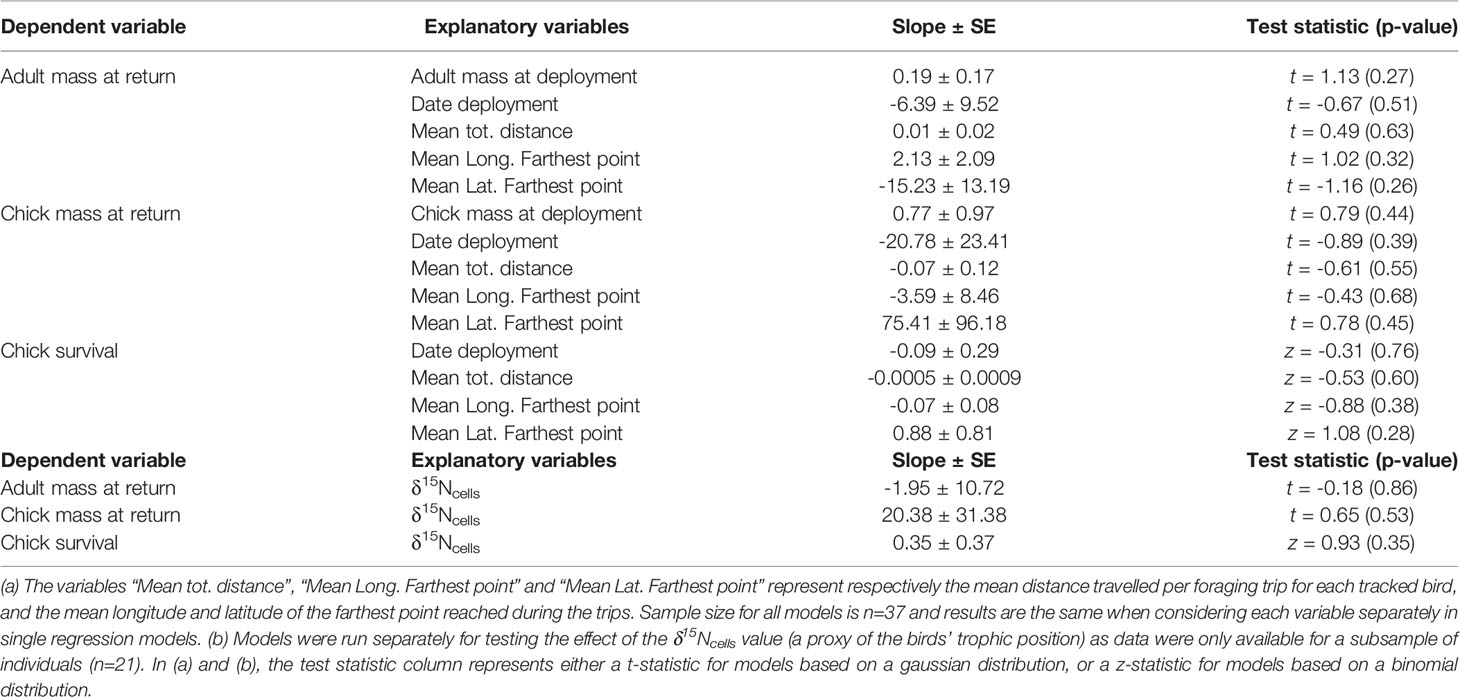
Table 2 Consequence of individual foraging strategies on adult mass, chick mass and chick survival in the Antarctic petrel (Svarthamaren Dronning Maud Land).
4 Discussion
Antarctic petrels from Svarthamaren show high levels of repeatability in their diet and movements at sea. During consecutive foraging trips, petrels tend to make trips of similar lengths and durations, reach similar terminal locations and feed on similar prey. These individual differences in foraging are spatially structured, with individuals travelling towards the west feeding more on fish than those travelling to the east.
4.1 Individual Specialization in the Antarctic Petrel?
Our results, based on GPS-tracking, stable isotope and stomach content data, support the hypothesis of individual specialization in foraging behaviour. However, our results are based on relatively short-term tracking of individuals during the chick-rearing period and the longer-term persistence of petrel specialization in foraging behaviour remains unknown. Studies during the other parts of the Antarctic petrel life-cycle (e.g., incubation, migration), and in consecutive years would be needed to get a more complete understanding of the level of individual specialization in this population.
Our results contrast with previous observations made in another Antarctic petrel colony in East Antarctica (Hop Island, Prydz Bay region). Dehnhard et al. (2020) reported that all tracked Antarctic petrels behaved like generalists and did not show any sign of specialization in terms of their foraging sites and habitat use, neither during incubation nor chick-rearing. This was explained by the high variability and low predictability of the petrels’ foraging environment surrounding Hop Island. Indeed, individual specialization in foraging is only expected when the environment shows some level of consistency and/or predictability. In a constantly changing foraging environment, being generalist (in terms of foraging habitat and/or diet) is expected to be the most beneficial strategy and there should be limited individual specialization (Futuyma and Moreno, 1988). The apparent individual specialization of Antarctic petrels breeding at Svarthamaren may thus be explained by a more predictable foraging environment than at Prydz Bay/East Antarctica. In support of this, recent observations found that some regions within the Svarthamaren Antarctic petrel foraging range are characterized by recurrent very high productivity (Descamps et al., 2021; Kauko et al., 2021). These studies identified a large late-summer phytoplankton bloom spanning 300 km east of the Weddell Gyre, associated with high krill densities. At-sea observations indicated that this area (that overlaps with the “central” and “East” clusters shown in Figure 3) is used intensively by Antarctic petrels. This bloom happens regularly in this area and such predictability may be linked to the individual specialization observed in petrels from Svarthamaren.
Based on previous satellite and GPS tracking, Fauchald et al. (2006; 2017) suggested that Antarctic petrels from Svarthamaren had no fixed foraging areas and adjust their behaviour and search for food based on real-time cues (i.e. during the ongoing foraging bout). However, our new results indicate that Antarctic petrels show some level of consistency in their movements and tend to have the same final destination between consecutive foraging trips. This does not necessarily mean that they follow the exact same routes and forage at the same locations along these trips. In fact, when looking at potential foraging areas along each foraging trip (see details in Supplementary Table 2), we found that foraging areas were distributed along the tracks and there was very little overlap in these potential foraging areas between consecutive trips. This supports Fauchald et al. (2006; 2017) results and indicates that, even if petrels generally travel to the same destination during consecutive trips, they show a flexible foraging strategy and opportunistically adjust their search areas based on cues they find along the way.
4.2 Antarctic Krill and Petrel Foraging
The differences in Antarctic petrel movements were linked to differences in diet. Birds travelling towards the East were characterized by a diet more based on lower trophic level-prey than birds travelling westward. This indicates spatial differences in prey composition or spatial variation in the isotopic value of the prey. Indeed, the isotope values of fish or krill can vary spatially, which could ultimately affect the isotope values in their predators (e.g., Jaeger et al., 2010; Moreno et al., 2011). More specifically, the δ15N values of krill vary with both its size and the chlorophyll-a concentration in their environment (Polito et al., 2019). Such intra-specific differences in krill (or fish) could in theory explain the differences in δ15N values observed in the different Antarctic petrel foraging areas. However, both the large δ15N difference (2.9 ‰, one full trophic level; Figure 2) and the results from food sample analyses do not support this interpretation and rather suggest different diets in these areas, with krill being more important in the eastern areas. These eastern “krill foraging areas” correspond to the phytoplankton bloom area described above and that is characterized in some years by very high primary productivity in late summer and high densities of Antarctic krill (Moreau et al. in prep.). On the contrary, Antarctic petrels foraging west of Svarthamaren had a diet dominated by fish, which fits with results from an earlier study (Lorentsen et al., 1998). In this study, ten stomach content samples were obtained from Antarctic petrels shot at sea along the coast, west of Svarthamaren (at 72°25’S, 16°43’W) in February 1994 and these samples were also dominated by fish (87% of the total stomach mass).
Although the sample size is small, our results also indicate that the proportion of krill in the diet in 2016 was much lower than observed in previous years (Lorentsen et al., 1998; Descamps et al., 2016b). Indeed, krill represented only 26% on average of the wet biomass of samples collected in January and February 2016 while it represented ≥60% in the 1992, 1994 and 2013 breeding seasons (Lorentsen et al., 1998; Descamps et al., 2016a). Surprisingly, primary production was very high in the “bloom area” in 2016 but not in 2013 (Moreau et al. in prep.), while the proportion of krill in the diet was high in 2013 but low in 2016. The relationships between primary production, krill availability and petrel diet in this area may be complex and additional years of data are needed to understand them.
The 2015/2016 breeding season had one of the fewest number of breeders since the work at this colony started in 1984/1985 and was characterized by the lowest number of breeding pairs (ca. 18,000 active nests at the end of January 2016, after the peak of hatching, as compared to 20,000-50,000 in period 2012-2014 and 50,000-200,000 in period 1985-2001, unpublished data). To determine whether this low colony size was linked to the generally low proportion of krill in the diet, which could reflect poor foraging conditions and low krill availability in the marine environment, warrants further investigation.
4.3 Demographic Consequences
Individual variation in habitat use, foraging behaviour and/or diet may lead to differences in demography (Morales et al., 2010). Such relationships have been observed in several seabirds like the wandering albatross Diomedea exulans (Weimerskirch, 2018), herring gull Larus argentatus (Pierotti and Annett, 1987; van den Bosch et al., 2019) or Adélie penguins Pygoscelis adeliae (Chapman et al., 2011; Ainley et al., 2018). In the Adélie penguin, there seems to be clear energetic, and potentially fitness, benefits for the chicks of having fish (more specifically Antarctic silverfish) in their diet as compared to a diet based on krill only (Chapman et al., 2011; Ainley et al., 2018). Our results do not support these findings in petrels, as the different foraging tactics and diet observed in Svarthamaren petrels were not associated with different adult body mass, or in chick survival or growth. Our results suggest that these costs and benefits associated to different foraging strategies were similar for all strategies, at least in the 2016 breeding season. Whether having a fish-based or krill-based diet may bear different costs and/or benefits in different years remain to be elucidated. Moreover, Antarctic petrels share parental care and both parents feed the chick. Consequently, to conclude more firmly that individual variation in foraging strategies does not affect chick growth or survival, information on both parents’ foraging behaviour would be needed as one parent could potentially compensate for a low foraging efficiency of its partner (Varpe et al., 2004). Such study would require the tracking of both parents simultaneously.
4.4 Conclusion
Variation among individuals in their foraging strategies and resource use has major implications for our understanding of animal ecology and of how populations can interact with their environment and respond to environmental change. The apparent inter-individual variation in foraging strategies and dependence on krill that we observed in Antarctic petrels from Dronning Maud Land may increase the resilience of this population to future changes in the krill population, and more generally to changes in their foraging environment. In this context, assessing whether the individual specialization we observed at an intra-seasonal scale also exists at an inter-annual scale, and assessing the proportion of the population adopting each different strategy, is a priority.
Data Availability Statement
The original contributions presented in the study are included in the article/Supplementary Material. Further inquiries can be directed to the corresponding author.
Ethics Statement
The animal study was reviewed and approved by Forsøksdyrutvalget/Mattilsynet (permit 7935).
Author Contributions
SD: conception and draft the first version of the manuscript. SD and SP: design of the study. SD, SH and JF: spatial and statistical analyses. PB and M-BF: isotope analyses. YC: stomach content analyses. SD and AT: data collection. All authors revised the manuscript critically and had substantial impact on the final draft of the manuscript.
Funding
The study was funded by the Norwegian Research council (NARE program).
Conflict of Interest
The authors declare that the research was conducted in the absence of any commercial or financial relationships that could be construed as a potential conflict of interest.
Publisher’s Note
All claims expressed in this article are solely those of the authors and do not necessarily represent those of their affiliated organizations, or those of the publisher, the editors and the reviewers. Any product that may be evaluated in this article, or claim that may be made by its manufacturer, is not guaranteed or endorsed by the publisher.
Acknowledgments
The authors thank Andrew Lowther for helpful comments on a first draft, Emmannuelle Grimaud for her help in food sample analysis at the CEBC laboratory, Oddveig Ørvoll for her help with Figure 3 and they are grateful to G. Guillou from the Plateforme Analyses Isotopiques of LIENSs laboratory for running isotope analyses. The authors also thank the CPER (Contrat de Projet Etat-Région) and FEDER (European regional Development Fund) for funding the IR-MS of LIENSs, and the Institut Universitaire de France (IUF) for its support to PB as a Senior Member.
Supplementary Material
The Supplementary Material for this article can be found online at: https://www.frontiersin.org/articles/10.3389/fmars.2022.809852/full#supplementary-material
References
Ainley D. G., Dugger K. M., La Mesa M., Ballard G., Barton K. J., Jennings S., et al. (2018). Post-Fledging Survival of Adélie Penguins at Multiple Colonies: Chicks Raised on Fish do Well. Marine Ecol. Prog. Ser. 601, 239–251. doi: 10.3354/meps12687
Ainley D. G., O’connor E. F., Boekelheide R. J. (1984). The Marine Ecology of Birds in the Ross Sea, Antarctica. (Washington, D.C: The American Ornithologist Union).
Ainley D. G., Ribic C. A., Fraser W. R. (1992). Does Prey Preference Affect Habitat Choice in Antarctic Seabirds? Marine Ecol. Prog. Ser. 90, 207–221. doi: 10.3354/meps090207
Ainley D., Russell J., Jenouvrier S., Woehler E., Lyver P. O. B., Fraser W. R., et al. (2010). Antarctic Penguin Response to Habitat Change as Earth’s Troposphere Reaches 2 C Above Preindustrial Levels. Ecol. Monogr. 80, 49–66. doi: 10.1890/08-2289.1
Araújo M. S., Bolnick D. I., Layman C. A. (2011). The Ecological Causes of Individual Specialisation. Ecol. Lett. 14, 948–958. doi: 10.1111/j.1461-0248.2011.01662.x
Ashmole N. (1963). The Regulation of Numbers of Oceanic Tropical Seabirds. Ibis 458, 473. doi: 10.1111/j.1474-919X.1963.tb06766.x
Atkinson A., Siegel V., Pakhomov E., Rothery P. (2004). Long-Term Decline in Krill Stock and Increase in Salps Within the Southern Ocean. Nature 432, 100–103. doi: 10.1038/nature02996
Bard S. M. (1999). Global Transport of Anthropogenic Contaminants and the Consequences for the Arctic Marine Ecosystem. Marine Pollut. Bull. 38, 356–379. doi: 10.1016/S0025-326X(99)00041-7
Bearhop S., Adams C. E., Waldron S., Fuller R. A., Macleod H. (2004). Determining Trophic Niche Width: A Novel Approach Using Stable Isotope Analysis. J. Anim. Ecol. 73, 1007–1012. doi: 10.1111/j.0021-8790.2004.00861.x
Benton T. G., Plaistow S. J., Coulson T. N. (2006). Complex Population Dynamics and Complex Causation: Devils, Details and Demography. Proc. R. Soc. B-Biol. Sci. 273, 1173–1181. doi: 10.1098/rspb.2006.3495
Bergstad O. A., Johannesen E., Høines Å., Ellingsen K. E., Lien V. S., Byrkjedal I., et al. (2018). Demersal Fish Assemblages in the Boreo-Arctic Shelf Waters Around Svalbard During the Warm Period 2007–2014. Polar. Biol. 41, 125–142. doi: 10.1007/s00300-017-2176-2
Bolnick D. I., Amarasekare P., Araújo M. S., Bürger R., Levine J. M., Novak M., et al. (2011). Why Intraspecific Trait Variation Matters in Community Ecology. Trends Ecol. Evol. 26, 183–192. doi: 10.1016/j.tree.2011.01.009
Bolnick D. I., Svanback R., Fordyce J. A., Yang L. H., Davis J. M., Hulsey C. D., et al. (2003). The Ecology of Individuals: Incidence and Implications of Individual Specialization. Am. Nat. 161, 1–28. doi: 10.1086/343878
Bond A. L., Jardine T. D., Hobson K. A. (2016). Multi-Tissue Stable-Isotope Analyses can Identify Dietary Specialization. Methods Ecol. Evol. 7, 1428–1437. doi: 10.1111/2041-210X.12620
Carravieri A., Fort J., Tarroux A., Cherel Y., Love O. P., Prieur S., et al. (2018). Mercury Exposure and Short-Term Consequences on Physiology and Reproduction in Antarctic Petrels. Environ. Pollut. 237, 824–831. doi: 10.1016/j.envpol.2017.11.004
Carravieri A., Warner N. A., Herzke D., Brault-Favrou M., Tarroux A., Fort J., et al. (2021). Trophic and Fitness Correlates of Mercury and Organochlorine Compound Residues in Egg-Laying Antarctic Petrels. Environ. Res. 193, 110518. doi: 10.1016/j.envres.2020.110518
CCAMLR (2021). Fishery Report 2020: Euphausia Superba in Area 48. Hobart, Australia: CCAMLR Secretariat
Ceia F. R., Ramos J. A. (2015). Individual Specialization in the Foraging and Feeding Strategies of Seabirds: A Review. Marine Biol. 162, 1923–1938. doi: 10.1007/s00227-015-2735-4
Chapman E. W., Hofmann E. E., Patterson D. L., Ribic C. A., Fraser W. R. (2011). Marine and Terrestrial Factors Affecting Adélie Penguin Pygoscelis Adeliae Chick Growth and Recruitment Off the Western Antarctic Peninsula. Marine Ecol. Prog. Ser. 436, 273–289. doi: 10.3354/meps09242
Chavarie L., Howland K. L., Harris L. N., Gallagher C. P., Hansen M. J., Tonn W. M., et al. (2021). Among-Individual Diet Variation Within a Lake Trout Ecotype: Lack of Stability of Niche Use. Ecol. Evol. 11, 1457–1475. doi: 10.1002/ece3.7158
Chen C., Amirbahman A., Fisher N., Harding G., Lamborg C., Nacci D., et al. (2008). Methylmercury in Marine Ecosystems: Spatial Patterns and Processes of Production, Bioaccumulation, and Biomagnification. EcoHealth 5, 399–408. doi: 10.1007/s10393-008-0201-1
Cherel Y., Bocher P., De Broyer C., Hobson K. A. (2002). Food and Feeding Ecology of the Sympatric Thin-Billed Pachyptila Belcheri and Antarctic P-Desolata Prions at Iles Kerguelen, Southern Indian Ocean. Marine Ecol. Prog. Ser. 228, 263–281. doi: 10.3354/meps228263
Cherel Y., Parenteau C., Bustamante P., Bost C. A. (2018). Stable Isotopes Document the Winter Foraging Ecology of King Penguins and Highlight Connectivity Between Subantarctic and Antarctic Ecosystems. Ecol. Evol. 8, 2752–2765. doi: 10.1002/ece3.3883
Cipro C. V., Cherel Y., Bocher P., Caurant F., Miramand P., Bustamante P. (2018). Trace Elements in Invertebrates and Fish From Kerguelen Waters, Southern Indian Ocean. Polar. Biol. 41, 175–191. doi: 10.1007/s00300-017-2180-6
Cox M. J., Candy S., de la Mare W. K., Nicol S., Kawaguchi S., Gales N. (2018). No Evidence for a Decline in the Density of Antarctic Krill Euphausia Superba Danin the Southwest Atlantic Sector Between 1976 and 2016. J. Crustacean Biol. 38, 656–661. doi: 10.1093/jcbiol/ruy072
Dehnhard N., Achurch H., Clarke J., Michel L. N., Southwell C., Sumner M. D., et al. (2020). High Inter-and Intraspecific Niche Overlap Among Three Sympatrically Breeding, Closely Related Seabird Species: Generalist Foraging as an Adaptation to a Highly Variable Environment? J. Anim. Ecol. 89, 104–119. doi: 10.1111/1365-2656.13078
De León L. F., Rolshausen G., Bermingham E., Podos J., Hendry A. P. (2012). Individual Specialization and the Seeds of Adaptive Radiation in Darwin’s Finches. Evol. Ecol. Res. 14, 365–380.
Descamps S., Moreau S., Tarroux A., Lorentsen S.-H., Bustnes J.-O., Yoccoz N. G., et al. (2021). “Svarthamaren seabirds, Sentinels of the Southern Ocean,” in Fram Forum (Tromsø, Norway: FRAM – High North Research Center for Climate and Environment) 102.
Descamps S., Strøm H. (2021). As the Arctic Becomes Boreal: Ongoing Shifts in a High-Arctic Seabird Community. Ecology 102, e03485. doi: 10.1002/ecy.3485
Descamps S., Tarroux A., Cherel Y., Delord K., Godø O. R., Kato A., et al. (2016a). At-Sea Distribution and Prey Selection of Antarctic Petrels and Commercial Krill Fisheries. PloS One 11, e0156968. doi: 10.1371/journal.pone.0156968
Descamps S., Tarroux A., Lorentsen S. H., Love O. P., Varpe O., Yoccoz N. G. (2016b). Large-Scale Oceanographic Fluctuations Drive Antarctic Petrel Survival and Reproduction. Ecography 39, 496–505. doi: 10.1111/ecog.01659
Durell S.E.L.V.D. (2000). Individual Feeding Specialisation in Shorebirds: Population Consequences and Conservation Implications. Biol. Rev. 75, 503–518. doi: 10.1111/j.1469-185X.2000.tb00053.x
Edwards M. A., Derocher A. E., Hobson K. A., Branigan M., Nagy J. A. (2011). Fast Carnivores and Slow Herbivores: Differential Foraging Strategies Among Grizzly Bears in the Canadian Arctic. Oecologia 165, 877–889. doi: 10.1007/s00442-010-1869-9
Estes J., Riedman M., Staedler M., Tinker M., Lyon B. (2003). Individual Variation in Prey Selection by Sea Otters: Patterns, Causes and Implications. J. Anim. Ecol. 72, 144–155. doi: 10.1046/j.1365-2656.2003.00690.x
Fauchald P. (1999). Foraging in a Hierarchical Patch System. Am. Nat. 153, 603–613. doi: 10.1086/303203
Fauchald P., Tarroux A., Tveraa T., Cherel Y., Ropert-Coudert Y., Kato A., et al. (2017). Spring Phenology Shapes the Spatial Foraging Behavior of Antarctic Petrels. Marine Ecol. Prog. Ser. 568, 203–215. doi: 10.3354/meps12082
Fauchald P., Tveraa T. (2006). Hierarchical Patch Dynamics and Animal Movement Pattern. Oecologia 149, 383–395. doi: 10.1007/s00442-006-0463-7
Futuyma D. J., Moreno G. (1988). The Evolution of Ecological Specialization. Annu. Rev. Ecol. Systematics 19, 207–233. doi: 10.1146/annurev.es.19.110188.001231
Gibert J. P., Brassil C. E. (2014). Individual Phenotypic Variation Reduces Interaction Strengths in a Consumer–Resource System. Ecol. Evol. 4, 3703–3713. doi: 10.1002/ece3.1212
Godø O. R., Samuelsen A., Macaulay G. J., Patel R., Hjøllo S. S., Horne J., et al. (2012). Mesoscale Eddies Are Oases for Higher Trophic Marine Life. PloS One 7, e30161. doi: 10.1371/journal.pone.0030161
Goutte A., Barbraud C., Meillère A., Carravieri A., Bustamante P., Labadie P., et al. (2014a). Demographic Consequences of Heavy Metals and Persistent Organic Pollutants in a Vulnerable Long-Lived Bird, the Wandering Albatross. Proc. R. Soc. B.: Biol. Sci. 281, 20133313. doi: 10.1098/rspb.2013.3313
Goutte A., Bustamante P., Barbraud C., Delord K., Weimerskirch H., Chastel O. (2014b). Demographic Responses to Mercury Exposure in Two Closely Related Antarctic Top Predators. Ecology 95, 1075–1086. doi: 10.1890/13-1229.1
Hijmans R. J., Williams E., Vennes C. (2017). Geosphere: Spherical Trigonometry. R Package Version 1.5-14. Available at: https://cran.r-project.org/web/packages/geosphere/index.html.
Hobson K. A., Clark R. (1993). Turnover of 13 C in Cellular and Plasma Fractions of Blood: Implications for Nondestructive Sampling in Avian Dietary Studies. Auk. 110, 638–641. doi: 10.2307/4088430
Holbrook S. J., Schmitt R. J. (1992). Causes and Consequences of Dietary Specialization in Surfperches: Patch Choice and Intraspecific Competition. Ecology 73, 402–412. doi: 10.2307/1940748
Hussey N. E., Macneil M. A., Mcmeans B. C., Olin J. A., Dudley S. F., Cliff G., et al. (2014). Rescaling the Trophic Structure of Marine Food Webs. Ecol. Lett. 17, 239–250. doi: 10.1111/ele.12226
Intergovernmental Panel on Climate Change (2013) Climate Change 2013: The Physical Science Basis. Working Group II Contribution to the IPCC 5th Assessment Report. Available at: http://www.ipcc.ch/report/ar5/wg2/.
Jaeger A., Lecomte V. J., Weimerskirch H., Richard P., Cherel Y. (2010). Seabird Satellite Tracking Validates the Use of Latitudinal Isoscapes to Depict Predators’ Foraging Areas in the Southern Ocean. Rapid Commun. Mass Spectrometry 24, 3456–3460. doi: 10.1002/rcm.4792
Kauko H. M., Hattermann T., Ryan-Keogh T., Singh A., De Steur L., Fransson A., et al. (2021). Phenology and Environmental Control of Phytoplankton Blooms in the Kong Håkon VII Hav in the Southern Ocean. Front. Marine Sci. 8, 287. doi: 10.3389/fmars.2021.623856
Kendall B. E., Fox G. A., Fujiwara M., Nogeire T. M. (2011). Demographic Heterogeneity, Cohort Selection, and Population Growth. Ecology 92, 1985–1993. doi: 10.1890/11-0079.1
Lessells C. M., Boag P. T. (1987). Unrepeatable Repeatabilities: A Common Mistake. Auk. 104, 116–121. doi: 10.2307/4087240
Lorentsen S. H., Klages N., Røv N. (1998). Diet and Prey Consumption of Antarctic Petrels Thalassoica Antarctica at Svarthamaren, Dronning Maud Land, and at Sea Outside the Colony. Polar. Biol. 19, 414–420. doi: 10.1007/s003000050267
Lorentsen S. H., Røv N. (1995). Incubation and Brooding Performance of the Antarctic Petrel Thalassoica Antarctica at Svarthamaren, Dronning Maud Land. Ibis 137, 345–351. doi: 10.1111/j.1474-919X.1995.tb08031.x
Lunghi E., Manenti R., Cianferoni F., Ceccolini F., Veith M., Corti C., et al. (2020). Interspecific and Interpopulation Variation in Individual Diet Specialization: Do Environmental Factors Have a Role? Ecology 101, e03088. doi: 10.1002/ecy.3088
Mehlum F., Gjessing Y., Haftorn S., Bech C. (1988). Census of Breeding Antarctic Petrels Thalassoica Antarctica and Physical Features of the Breeding Colony at Svarthamaren, Dronning Maud Land, With Notes on Breeding Snow Petrels Pagodroma Nivea and South Polar Skuas Catharacta Maccormicki. Polar. Res. 6, 1–9. doi: 10.1111/j.1751-8369.1988.tb00576.x
Morales J. M., Moorcroft P. R., Matthiopoulos J., Frair J. L., Kie J. G., Powell R. A., et al. (2010). Building the Bridge Between Animal Movement and Population Dynamics. Philos. Trans. R. Soc. B.: Biol. Sci. 365, 2289–2301. doi: 10.1098/rstb.2010.0082
Moreau T., Hattermann L., de Steur H. M., Kauko H., Steen H., Ahonen M., et al. Wind-Driven Upwelling Unveils a Large Phytoplankton Bloom and Rich Ecosystem in the Open Southern Ocean.
Moreno R., Jover L., Velando A., Munilla I., Sanpera C. (2011). Influence of Trophic Ecology and Spatial Variation on the Isotopic Fingerprints of Seabirds. Marine Ecol. Prog. Ser. 442, 229–239. doi: 10.3354/meps09420
Nakagawa S., Schielzeth H. (2010). Repeatability for Gaussian and Non-Gaussian Data: A Practical Guide for Biologists. Biol. Rev. 85, 935–956. doi: 10.1111/j.1469-185X.2010.00141.x
Navarro-López J., Vergara P., Fargallo J. A. (2014). Trophic Niche Width, Offspring Condition and Immunity in a Raptor Species. Oecologia 174, 1215–1224. doi: 10.1007/s00442-013-2855-9
Newsome S. D., Martinez Del Rio C., Bearhop S., Phillips D. L. (2007). A Niche for Isotopic Ecology. Front. Ecol. Environ. 5, 429–436. doi: 10.1890/1540-9295(2007)5[429:ANFIE]2.0.CO;2
Nicol S., Foster J., Kawaguchi S. (2012). The Fishery for Antarctic Krill–Recent Developments. Fish. Fish. 13, 30–40. doi: 10.1111/j.1467-2979.2011.00406.x
Okuyama T. (2008). Individual Behavioral Variation in Predator–Prey Models. Ecol. Res. 23, 665–671. doi: 10.1007/s11284-007-0425-5
Pagani-Núñez E., Valls M., Senar J. (2015). Diet Specialization in a Generalist Population: The Case of Breeding Great Tits Parus Major in the Mediterranean Area. Oecologia 179, 629–640. doi: 10.1007/s00442-015-3334-2
Phillips R. A., Lewis S., González-Solís J., Daunt F.J.M.E.P.S. (2017). Causes and Consequences of Individual Variability and Specialization in Foraging and Migration Strategies of Seabirds. J. Marine Ecol. Prog. Series 578, 117–150. doi: 10.3354/meps12217
Pierotti R., Annett C. (1987). “Reproductive Consequences of Dietary Specialization and Switching in an Ecological Generalist,” in Foraging Behavior (Boston, MA, USA: Springer), 417–442.
Polito M. J., Trivelpiece W. Z., Reiss C. S., Trivelpiece S. G., Hinke J. T., Patterson W. P., et al. (2019). Intraspecific Variation in a Dominant Prey Species can Bias Marine Predator Dietary Estimates Derived From Stable Isotope Analysis. Limnol. Oceanography: Methods 17, 292–303. doi: 10.1002/lom3.10314
R Development Core Team (2020). R: A Language and Environment for Statistical Computing (Vienna, Austria: R Foundation for Statistical Computing). Available at: http://www.R-project.org/.
Stoffel M. A., Nakagawa S., Schielzeth H. (2017). Rptr: Repeatability Estimation and Variance Decomposition by Generalized Linear Mixed-Effects Models. Methods Ecol. Evol. 8, 1639–1644. doi: 10.1111/2041-210X.12797
Strøm H., Bakken V., Skoglund A., Descamps S., Fjeldheim V. B., Steen H. (2020). Population Status and Trend of the Threatened Ivory Gull Pagophila Eburnea in Svalbard. Endangered Species Res. 43, 435–445. doi: 10.3354/esr01081
Tarling G. A., Fielding S. (2016). “Swarming and Behaviour in Antarctic Krill,” in Biology and Ecology of Antarctic Krill (Switzerland: Springer), 279–319.
Tarroux A., Cherel Y., Fauchald P., Kato A., Love O. P., Ropert-Coudert Y., et al. (2020). Foraging Tactics in Dynamic Sea-Ice Habitats Affect Individual State in a Long-Ranging Seabird. Funct. Ecol. 34, 1839–1856. doi: 10.1111/1365-2435.13632
Tarroux A., Weimerskirch H., Wang S.-H., Bromwich D. H., Cherel Y., Kato A., et al. (2016). Flexible Flight Response to Challenging Wind Conditions in a Commuting Antarctic Seabird: Do You Catch the Drift? Anim. Behav. 113, 99–112. doi: 10.1016/j.anbehav.2015.12.021
Trathan P. N., Hill S. L. (2016). “The Importance of Krill Predation in the Southern Ocean,” in Biology and Ecology of Antarctic Krill (Switzerland: Springer), 321–350.
Trathan P. N., Wienecke B., Barbraud C., Jenouvrier S., Kooyman G., Le Bohec C., et al. (2020). The Emperor Penguin-Vulnerable to Projected Rates of Warming and Sea Ice Loss. Biol. Conserv. 241, 108216. doi: 10.1016/j.biocon.2019.108216
Trevail A. M., Green J. A., Bolton M., Daunt F., Harris S. M., Miller P. I., et al (2021). Environmental Heterogeneity Promotes Individual Specialisation in Habitat Selection in a Widely Distributed Seabird. J. Anim. Ecol. 90, 2875–2887. doi: 10.1111/1365-2656.13588
Van Den Bosch M., Baert J. M., Müller W., Lens L., Stienen E. W. (2019). Specialization Reduces Foraging Effort and Improves Breeding Performance in a Generalist Bird. Behav. Ecol. 30, 792–800. doi: 10.1093/beheco/arz016
Van De Pol M., Brouwer L., Ens B. J., Oosterbeek K., Tinbergen J. M. (2010). Fluctuating Selection and the Maintenance of Individual and Sex-Specific Diet Specialization in Free-Living Oystercatchers. Evol.: Int. J. Organic Evol. 64, 836–851. doi: 10.1111/j.1558-5646.2009.00859.x
Varpe O., Tveraa T., Folstad I. (2004). State-Dependent Parental Care in the Antarctic Petrel: Responses to Manipulated Chick Age During Early Chick Rearing. Oikos 106, 479–488. doi: 10.1111/j.0030-1299.2004.13212.x
Vindenes Y., Langangen Ø. (2015). Individual Heterogeneity in Life Histories and Eco-Evolutionary Dynamics. Ecol. Lett. 18, 417–432. doi: 10.1111/ele.12421
Weimerskirch H. (2018). Linking Demographic Processes and Foraging Ecology in Wandering Albatross—Conservation Implications. J. Anim. Ecol. 87, 945–955. doi: 10.1111/1365-2656.12817
Wilson R. P. (1984). An Improved Stomach Pump for Penguins and other Seabirds. J. Field Ornithol. 55, 109–112.
Keywords: diet, foraging, seabird, Antarctic, krill (Euphausia superba)
Citation: Descamps S, Harris SM, Fluhr J, Bustamante P, Cherel Y, Trevail AM, Brault-Favrou M and Patrick SC (2022) Variation in Antarctic Petrel Foraging Ecology: Not All Individuals Specialize on Krill. Front. Mar. Sci. 9:809852. doi: 10.3389/fmars.2022.809852
Received: 05 November 2021; Accepted: 04 March 2022;
Published: 23 March 2022.
Edited by:
Jorge M. Pereira, University of Coimbra, PortugalReviewed by:
Vitor H. Paiva, University of Coimbra, PortugalDavid Ainley, H.T. Harvey & Associates, United States
Copyright © 2022 Descamps, Harris, Fluhr, Bustamante, Cherel, Trevail, Brault-Favrou and Patrick. This is an open-access article distributed under the terms of the Creative Commons Attribution License (CC BY). The use, distribution or reproduction in other forums is permitted, provided the original author(s) and the copyright owner(s) are credited and that the original publication in this journal is cited, in accordance with accepted academic practice. No use, distribution or reproduction is permitted which does not comply with these terms.
*Correspondence: S. Descamps, sebastien.descamps@npolar.no
†ORCID: S. Descamps, orcid.org/0000-0003-0590-9013
S. M. Harris, orcid.org/0000-0002-8580-9444
P. Bustamante, orcid.org/0000-0003-3877-9390
Y. Cherel, orcid.org/0000-0001-9469-9489
A. M. Trevail, orcid.org/0000-0002-6459-5213
S. C. Patrick, orcid.org/0000-0003-4498-944X