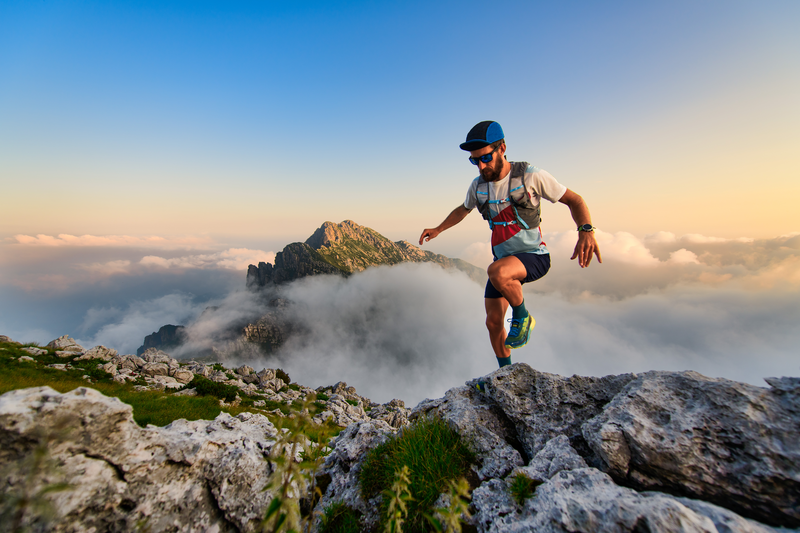
94% of researchers rate our articles as excellent or good
Learn more about the work of our research integrity team to safeguard the quality of each article we publish.
Find out more
ORIGINAL RESEARCH article
Front. Mar. Sci. , 07 October 2020
Sec. Marine Fisheries, Aquaculture and Living Resources
Volume 7 - 2020 | https://doi.org/10.3389/fmars.2020.578976
Using breeding programs to improve feed efficiency, the ratio between fish body weight gain (BWG) and feed intake (FI), could increase aquaculture sustainability through reduced feed costs and environmental impact. To this end, individual phenotypic information is required. Individual FI can be measured by isolating each fish. Under these conditions, restricting the feeding rate has proved relevant to improve feed efficiency indirectly by selecting faster-growing animals. Moreover, a restricted feeding rate reduces the work load of collecting uneaten pellets after each meal. The approach assumes the most efficient fish at high and low feeding rates are the same, but this assumption remains untested. In European sea bass (Dicentrarchus labrax), feed efficiency is likely to be impacted also by population, temperature, and their interaction, as already demonstrated for growth in this species. To investigate these issues, 200 European sea bass from three wild populations, Atlantic (AT), West Mediterranean (WM) and East Mediterranean (EM), were reared individually at two temperatures, 18°C and 24°C. Their BWG and FI were measured at six different feeding rates, from ad libitum (100% ADL) down to fasting. A trade-off between performance at 100% ADL and at fasting was observed: more efficient fish at 100% ADL showed a stronger decrease in BWG (standardized to metabolic weight) when the feeding rate was progressively lowered and lost more weight at fasting. The most efficient fish were not the same depending on the feeding rate, suggesting the feeding rate used to phenotype fish in selective breeding programs must be the same as that used in commercial practices. The slope in the linear relationship between BWG and FI (both standardized to metabolic weight) was similar among populations and temperatures. However, EM fish had a higher intercept than others, suggesting this population grew more and thus was more efficient for an equal feeding rate. Similarly, fish reared at 18°C were more efficient for an equal feeding rate. When feed efficiency was studied in fish fed at 100% ADL, the temperature effect disappeared but the population effect remained. This highlights the complex interplay between population, temperature and feeding rate when evaluating individual feed efficiency.
In order to feed the increasing world’s population, including more fish in future diets could help provide a solution because it would spare lands and feed crops when comparing with livestock production (Froehlich et al., 2018). Fisheries production has not increased over the last two decades, thus meeting the future demand for aquatic products will rely on aquaculture (FAO, 2018). However, to reach the fish production required, aquaculture needs to address some sustainability issues. The main issue is linked to fish feed which accounts for 30% to 60% of total costs in an intensive fish farm (Goddard, 1996). Furthermore, feed production will have to compete with both agriculture and human consumption for access to ingredients (Troell et al., 2014), and is responsible for a high proportion of the environmental impact of aquaculture (Aubin et al., 2009; Besson et al., 2016a).
Improved use of feed by fish may involve fish nutrition (NRC, 2011), husbandry (De Silva and Anderson, 1995) and genetics (de Verdal et al., 2018a,b; Besson et al., 2019). Nutrition and husbandry have already been widely addressed, but genetic studies are scarce. Selective breeding could reduce feed use in aquaculture and improve sustainability by improving feed efficiency of farmed fish, i.e., the ratio between fish body weight gain (BWG) and feed intake (FI) (Besson et al., 2014, 2016a). Improving feed efficiency means using less feed to produce the same amount of fish, or producing more fish with the same amount of feed. However, to perform a selective breeding program, accurate individual phenotypic information is required. Measuring individual BWG is easy when fish are individually tagged, but measuring the individual FI of a large number of fish is challenging as fish are reared in large groups. Individual FI can be measured using individual rearing (Silverstein, 2006; Martins et al., 2011; Besson et al., 2019). This implies managing the exact number of pellets eaten by each individual. This method has the major advantage of being exhaustive: FI can be measured for each meal over several months and thus the temporal variability of FI is fully considered (Rodde et al., 2020). Moreover, the method gives immediate results (FI can be determined only a few hours after feeding). Besson et al. (2019) already managed to assess the feed efficiency of 588 European sea bass Dicentrarchus labrax in 194 days using this methodology. However, this method is tedious because of the need to collect all the uneaten pellets in all the individual aquariums. In their study, Besson et al. (2019) restricted the feeding rate to 50% of the optimal feeding rate. Using this methodology, it was demonstrated that selecting faster-growing individuals under a restricted feeding rate improved feed efficiency of the progenies in pigs (Nguyen et al., 2005) and in rabbits (Drouilhet et al., 2016), no matter if those progenies were then fed at a restricted or an ad libitum (abbreviated as “100% ADL” in the present study) feeding rate. Advantageously, using a restricted feeding rate reduces the workload. The reduced labor costs from lowering fish feeding rate make the transfer of this phenotyping method to practical selective breeding programs more likely and economically viable (Besson et al., 2019).
However, to our knowledge, there is no existing evidence that the most efficient fish at high feeding rates are also the most efficient at low feeding rates, and this issue needs to be addressed prior to starting breeding programs. As changing the feeding rate may impact the accuracy of feed efficiency estimates, it is useful to study individual variation in feed efficiency according to the feeding rate. For this study, three populations of European sea bass identified in the wild were used, Atlantic (AT), West Mediterranean (WM), and East Mediterranean (EM) (Guinand et al., 2017). The Atlantic and Mediterranean lineages of European sea bass started to diverge around 300,000 years before present following spatial separation during this glacial period. While the differentiation between the EM and the AT European sea bass was maintained, the secondary contact between these two lineages led to an admixed population in West Mediterranean area (Duranton et al., 2018, 2020). This evolutionary process occurred in environments whose average temperatures differed, since a North-West to South-East temperature gradient exists in European waters (Lindgren and Håkanson, 2011). Feed efficiency performance in European sea bass may thus be impacted by population, rearing temperature, and their interaction. Population by rearing temperature interactions on growth have been demonstrated already in this species (Vandeputte et al., 2014) suggesting similar effects could be found in feed efficiency. If such differences exist, there is a potential to choose more efficient source populations to start a selective breeding program, and to favor some specific rearing sites according to the temperature gradient existing in European waters.
In the present study, individual fish BWG and FI were measured at six different feeding rates, from 100% ADL down to fasting (0% ADL) to test whether feed efficiency at low feeding rates reflected feed efficiency at high feeding rates. A total of 200 European sea bass from AT, WM, and EM were reared individually at two temperatures: 18°C and 24°C, corresponding to the average and optimal temperatures, respectively, for European sea bass growth in the West Mediterranean area (Person-Le Ruyet et al., 2004; Besson et al., 2016b). Moreover, 18°C and 24°C reflect well the coldest and warmest average temperatures at which European sea bass is reared across Europe (Vandeputte et al., 2014). The objectives of the present study were (1) to assess the impact of the feed ration on the relationship between BWG and FI at individual level, and (2) to determine whether the relationship between BWG and FI varies with population and/or temperature.
This study was carried out in accordance with the recommendations of Directive 2010-63-EU on the protection of animals used for scientific purposes. The protocols were approved by C2EA–36 (“Comité d’éthique en expérimentation animale Languedoc-Roussillon”) under authorization APAFiS n° 2018032109435819 (version 2).
The 200 European sea bass used in the present study were produced by artificial fertilization on the 5th of February 2018 at the Ifremer Experimental Aquaculture Research Station (Palavas-les-Flots, France, 43°31′13°N; 3°54′37°E), with a similar protocol to Doan et al. (2017). The Atlantic (AT) and West Mediterranean (WM) groups were produced by mating wild sires and wild dams from each of the populations in a full factorial mating design, thus producing pure AT and WM offspring. Wild AT and WM fish were initially captured in the English Channel and the Gulf of Lions (France), respectively. Equal numbers of eggs from 22 WM dams, fertilized with sperm from 40 WM sires, and eggs from 9 AT dams, fertilized by 26 AT sires, were used. The East Mediterranean (EM) group was produced by mating 39 wild EM sires and 13 F1 EM × WM dams thus producing 75% EM-25% WM backcross progenies, hereafter called EM. Only wild EM males were available, which were initially captured in Turkey and Egypt (Vandeputte et al., 2014), and this is why only 75%EM-25%WM progenies could be produced. Hatching occurred on the 9th of February 2018. Each fish population was reared in separated tanks until 188 days post-hatching (dph). Fish (62 AT, 66 WM, and 72 EM) were then gathered in a single 1000 L holding tank after the injection of PIT-tags (Biolog-id, France). At 213 dph, fish were fasted until the beginning of the experiment at 221 dph to stimulate their appetite.
The rearing system consisted of two independent recirculating water systems placed in the same room. Each rearing system was comprised of 100 aquariums (10 L each), a sand filter, a biological filter and a UV filter. Water renewal rate was 300% per hour in each aquarium. Water temperature was set at 18 and 24°C in first and second rearing systems, respectively. For the first and second systems, mean oxygen saturation was, respectively, 114.1% (8.64 mg O2/L) and 107.1% (7.28 mg O2/L), mean water salinity was, respectively, 37.2‰ and 37.4‰ and mean water pH was 8.3 in both cases. Photoperiod was artificial: 12 h light/12 h dark.
The experimental procedure consisted of three distinct periods: acclimation, reaching 100% ADL and phenotyping (Figure 1). Acclimation lasted 4 weeks. During the first 2 weeks, five fish were reared per aquarium. Groups were then split to acclimate one fish per aquarium for another 2 weeks, as described by Besson et al. (2019). Feed, manufactured by Le Gouessant Aquaculture, was from the commercial diet called “Neo Start 3” with: 47% of crude protein, 18% of crude fat, 1.5% of crude fiber, 8% of ash, 1% of phosphorus, 19 MJ/kg for digestible energy content, 23 g/MJ for digestible protein/digestible energy ratio. The diet used remained the same over the whole experiment. From the beginning of acclimation onward, feed was supplied once a day in the morning (9 a.m.) by automatic feeders. During acclimation, fish were fed 50% of the feeding ration recommended by the feed provider with a single daily meal, and the number of uneaten pellets per aquarium was recorded as soon as fish were reared individually.
Figure 1. Experimental schedule applied to each fish. Fish age (in days post hatching) at each step is indicated by the numbers. ADL, ad libitum feeding rate; BW, body weight measurement.
During the second period, three rounds of 1 week were needed to reach 100% ADL for each fish (Figure 1). Over each round, the number of pellets uneaten by each fish was manually counted 2 h after each meal. The last week of acclimation was used as a starting point to estimate which fish were already fed at 100% ADL. At the end of each round, different choices were made according to the number of pellets wasted by each fish over the week:
(a) if the fish left a relatively low number of uneaten pellets (less than 30 pellets per day), that fish was considered to have reached 100% ADL and the FI of the fish was considered equal to its 100% ADL;
(b) if the fish left a large number of uneaten pellets (higher than 30 pellets per day), feed ration was decreased the week after by 10% of the feeding ration recommended by the feed provider to ease the wasted pellets counting;
(c) if the fish did not waste any pellets, its feed ration was increased the week after by 20% of the feeding ration recommended by the feed provider to reach 100% ADL.
At 270 dph, the end of round 3, there were 99 fish reared at 18°C (28 AT, 34 WM and 37 EM) and 95 fish reared at 24°C (28 AT, 32 WM and 35 EM). Six fish died before 270 dph because they jumped out of their aquarium (five AT at 18°C and one AT at 24°C).
The third period of the experiment was the phenotyping period, fish were first fed for 22 days (from 270 to 292 dph) with an individual feeding rate taking into account the various choices previously made to reach 100% ADL (Figure 1). Fish were weighed at 270, 281, and 292 dph (beginning, half and end of the 22 days) to update the individual feeding ration according to their body weight (BW) and to determine individual BWG (final BW – initial BW). Fish were anaesthetized with benzocaine (37.5 g per m3 of seawater) before weight measurements. Uneaten pellets were counted in each aquarium and removed daily. The uneaten feed weight was estimated every day, considering that all the pellets had the same weight (14.6 ± 1.6 mg with CV = 11.0%) and cumulated over the 100% ADL step. Fish were fasted the day of weight measurements and the day before. Feed intake of each fish during this 100% ADL step was calculated as: weight of feed given – weight of feed uneaten, and converted to a% of BW per day to estimate 100% ADL.
After estimation of 100% ADL, fish were successively fed 80%, 60%, 40%, 20%, and 0% ADL for 10 to 11 days at each step (Figure 1). Individual BWG and FI were measured over each step as previously defined, with fish being weighed on the days indicated in Figure 1. For ethical reasons, if a fish had lost weight both between 270 and 281 dph and between 281 and 292 dph when fed at 100% ADL (Figure 1), or if a fish had lost weight when fed at 80%, 60%, or 40% ADL, the next step was directly 0% ADL and then the fish was removed from the experiment. Moreover, five fish (two AT fish at 18°C, two AT fish at 24°C, and one WM fish at 24°C) did not eat at all at 100% ADL (<1% of their BW over the 22 days) and were directly removed from the experiment, without going through a 0% ADL step.
All statistical analyses were done using R software (R Core Team, 2018). The normality of residuals was checked using the quantile-quantile method (comparing residuals quantiles with theoretical normal quantiles). The homoscedasticity and independence of the residuals were checked by comparing the residuals with the fitted values from the models. Linear mixed models and tests associated to these models were performed using R packages “lme4” (Bates et al., 2015), “lmerTest” (Kuznetsova et al., 2017) and “lsmeans” (Lenth, 2016).
Individual metabolic weight (MBW) was calculated for each step (100% ADL to 0% ADL) as with Wi and Wf the initial and final BW of each specific feeding rate step (Lupatsch et al., 2003; Saravanan et al., 2012). In order to allow a more accurate comparison between fish with heterogeneous BW, individual BWG and FI were standardized to MBW (respectively, named StdBWG and StdFI) at each feeding rate step as StdBWG = 100*BWG/MBW and StdFI = 100*FI/MBW and expressed in % of MBW.day–1. Metabolic body weight was used instead of BW as BWG and FI in fish are more closely related to MBW than to BW (Paloheimo and Dickie, 1966; Warren and Davis, 1967; Fonds et al., 1992).
To study individual feed efficiency at 100% ADL, feed efficiency ratio (FER) was calculated as FER = BWG/FI. The impact of temperature and population on StdBWG, StdFI and FER during the 100% ADL step was determined using the following linear model:
where Yijk is the phenotype (StdBWG, StdFI or FER) at temperature i (18 or 24°C), for population j (AT, WM or EM) and animal k; μ is the general mean; T is the fixed effect of temperature i (18 or 24°C); P is the fixed effect of population j (AT, WM or EM); TP is the interaction of these two effects, and εijk is the residual [εijk ∼ N(0;σe2)]. Fixed effects significance was determined with Fisher test and then pairwise differences between temperature by population combinations were determined with Tukey post hoc test.
The individual StdBWG and StdFI data of every step (100% ADL to 0% ADL) were then analyzed all together. Focus was firstly made on the variability between temperatures and populations in the relationship between StdBWG and StdFI. The following repeated measures linear mixed model was used to analyze this relationship:
where StdBWGijkl and StdFIijkl are, respectively, StdBWG and StdFI at temperature i (18 or 24°C) for population j (AT, WM or EM), step k (k between 1 for 100% ADL and 6 for 0% ADL) and animal l; μ is the general mean, T is the fixed effect of temperature i (18 or 24°C), P is the fixed effect of population j (AT, WM or EM), and TP is the interaction of temperature by population: all those effects are the “intercept” part of the relationship since they do not depend on StdFI; β is the fixed effect of StdFI, βT and βP are the interactions of StdFI by temperature and population, respectively, and βTP is the triple interaction of StdFI by temperature and population: all those effects are the “slope” part of the relationship since they depend on StdFI. Finally, Al and Bl are the random effects of the animal l, respectively, associated to intercept and slope, with Al ∼ N(0;σ2a) and Bl ∼ N(0;σ2b), and εijkl is the residual [εijkl ∼ N(0;σe2)]. Fixed effects significance was determined with Fisher test and then pairwise differences between populations or temperatures were determined with Tukey post hoc test using R packages “lme4” (Bates et al., 2015), “lmerTest” (Kuznetsova et al., 2017) and “lsmeans” (Lenth, 2016). Data were considered as outliers and discarded from the analyses when their Cook’s distance (i.e., their influence) in the model linear mixed model was higher than 4/n (Algur and Biradar, 2017), with n the total number of StdBWG and StdFI measurements. The residuals of the model, i.e., εijkl, were extracted for data collected at 100% ADL (res100%ADL) and 0% ADL (res0%ADL). From a biological point of view, the higher the individual res100%ADL, the more efficient the fish at 100% ADL, and the lower the individual res0%ADL, the higher the body weight loss at fasting.
To assess individual variability within each temperature by population combination, parameters of the linear relationship between StdBWG and StdFI, i.e., intercept and slope, were calculated for each individual using the following model:
where StdBWGk and StdFIk are, respectively, the StdBWG and StdFI of the individual at step k (k between 1 for 100% ADL and 6 for 0% ADL) and εk the residual [εk ∼ N(0;σe2)]. This calculation was done exclusively for individuals with data available for at least 100% ADL, 80% ADL, 60% ADL, and 0% ADL (114 individuals out of 194). The coefficient of variation (CV = 100*standarddeviation/mean, expressed in %) was then estimated for intercepts and slopes within each population by temperature combination.
Finally, Pearson’s correlations were estimated between res100%ADL, res0%ADL, intercepts and slopes. Since the number of data for individual intercepts and slopes within each combination of temperature by population was too low (from 9 to 28) to ensure robust correlation analysis at combination level, it was decided to merge the data from the different combinations to estimate Pearson’s correlation. To avoid a bias in correlation estimations due to potential population and temperature effects on individual intercepts and slopes, intercepts and slopes were corrected by these fixed effects before merging the data. To correct for these effects, the following linear model was used:
where Yijk is the intercept or slope at temperature i (18 or 24°C), for population j (AT, WM, or EM) and animal k; μ is the general mean; T is the fixed effect of temperature i (18 or 24°C); P is the fixed effect of population j (AT, WM, or EM); TP is the interaction of these two effects, and εijk is the residual [εijk ∼ N(0;σe2)]. In the present model, residuals are the individual intercepts and slopes corrected for potential temperature, population, and interaction effects. Thus, these residuals were extracted to estimate the correlations.
All fish left some pellets uneaten and thus reached 100% ADL during the 22-day period: 95.9% of the fish left feed uneaten over at least nine meals out of 18 and 83.5% of the fish did so over at least 15 meals out of 18. Among the 194 fish that were successfully evaluated at 100% ADL, 46 (23.7%) lost weight and were consequently discarded from the analyses. Over the 22 days, fish grew from 26.5 ± 10.1 g (CV = 38.1%) to 29.6 ± 11.4 g (CV = 38.4%). Individual BWG, StdBWG, FI and StdFI were all significantly different according to temperature. This effect was driven by WM and EM fish which grew faster and consumed more feed at 24°C than at 18°C (Table 1). Individual FI was also significantly different according to population (Table 1), with EM fish eating on average 15 and 17% less than WM and AT fish, respectively. Feed efficiency ratio was significantly different according to population but not according to temperature (Table 1), with EM fish being on average 18 and 41% more efficient than WM and AT fish, respectively.
Table 1. Mean ± standard deviation of body weight gain (BWG), body weight gain standardized to metabolic body weight (StdBWG), feed intake (FI), feed intake standardized to metabolic body weight (StdFI) and feed efficiency ratio (FER) recorded for 22 days with fish fed at 100% of ad libitum feeding rate.
The data from 100% ADL to 0% ADL were merged. Using Cook’s distance to detect outlier data, 30 data out of 729 were rejected (4.1% of the total dataset). The proportion of variance explained by the models with StdBWG as a linear function of StdFI ranged from R2 = 0.80 to R2 = 0.90 according to the population by temperature combination (Figure 2). No difference was seen in slopes between temperatures or populations, as well as no temperature by population interaction (P > 0.05 in all cases with Fisher test). The intercept, i.e., the part of StdBWG variance that does not depend on StdFI, was significantly different among populations and temperatures (P < 0.001 in both cases with Fisher test), but the interaction was not significant (P > 0.05, Fisher test). The intercept was higher for EM than for WM (P < 0.001, Tukey test) which was higher than for AT (P < 0.001, Tukey test). The intercept was also significantly higher at 18°C than at 24°C (P < 0.001, Tukey test). This result means that for an equal StdFI, StdBWG was higher for EM fish than for WM and AT fish, and thus EM fish were the most efficient. Similarly, fish reared at 18°C were more efficient than fish reared at 24°C for an equal StdFI.
Figure 2. Linear relationships between body weight gain and feed intake, standardized to metabolic body weight (MBW), for the different population by temperature combinations. The three populations are from Atlantic Ocean (AT), West Mediterranean sea (WM), and East Mediterranean sea (EM). Standardized body weight gain and feed intake are expressed in % of MBW per day.
Modeling the individual relationship between StdBWG and StdFI with a linear function appeared suitable since 101 individuals out of 114 had a corresponding R2 higher than 0.80. The coefficients of variation were between 14.5% and 38.8% for intercepts and between 14.4% and 34.0% for slopes among the six different temperature by population combinations (Figure 3). No significant correlation was found between res100%ADL and res0%ADL. A significant and positive correlation was found between intercept and res0%ADL as well as between res100%ADL and slope. A significant and negative correlation was found between intercept and res100%ADL, between intercept and slope, as well as between res0%ADL and slope (Table 2).
Figure 3. Linear relationships between body weight gain and feed intake, standardized to metabolic body weight (MBW), at individual level within the different population by temperature combinations. The three populations are from Atlantic Ocean (AT), West Mediterranean sea (WM), and East Mediterranean sea (EM). Standardized body weight gain and feed intake are expressed in % of MBW per day. Parameters presented within each combination are the minimum, maximum and average of the R-squared (R2) of the various linear relationships, the number of individuals (n) and the coefficients of variation of intercept (CVint) and slope (CVslope).
Table 2. Correlation matrix with phenotypic correlations above diagonal and statistical significance (given as p-values) of the correlations below diagonal.
The objective of the present study was firstly to determine whether individual feed efficiency at low feeding rates reflected feed efficiency at high feeding rate. This aspect is key to give new insights on the potential development of selective breeding programs for feed efficiency in European sea bass. Secondly, the present study aimed at providing a better understanding of the variations in the relationship between BWG and FI at population and temperature levels, across a range of feeding rates. Such variations are of major interest to determine the impact of temperature and population on feed efficiency.
At the individual level, there appeared to be a trade-off between performance observed at 100% ADL and at fasting. Due to the high and significant correlation between res100%ADL and the slope of the linear relationship between StdBWG and StdFI, it seemed that more efficient fish at 100% ADL were showing a stronger decrease in StdBWG when the feeding rate was progressively lowered. Moreover, these fish were losing more weight at fasting (lower intercept). Surprisingly, no significant correlation was found between res100%ADL and res0%ADL. Actually, both res0%ADL and intercept are an estimation of body weight loss at fasting. These two parameters are not perfectly equivalent (r = 0.47) and the intercept of the linear relationship may better reflect body weight loss at fasting. Indeed, the intercept integrates all data from 100% down to 0% ADL (four to six measurement periods) whereas res0%ADL is based only on one measurement period.
Trade-offs in growth performance between high and low feeding rates have been reported in European sea bass (Dupont-Prinet et al., 2010; Grima et al., 2010), but not specifically for feed efficiency. These authors identified two profiles of fish: those exploiting the available feed as much as possible, growing faster and losing more weight during a fasting period (“boom and bust”) versus those with less capacity to exploit the available feed, growing slower and losing less weight during feed deprivation (Dupont-Prinet et al., 2010). McKenzie et al. (2014) provided evidence that such variation in weight loss at fasting was linked to metabolic costs, but also to the composition of the reserves used. They concluded that fish tolerant to feed deprivation rely more on lipids whereas fish sensitive to feed deprivation rely more on proteins. Variation in feed efficiency at 100% ADL might be linked to the same factors as variation in weight loss at fasting, i.e., differences in metabolic costs or in the nature of the energy reserves used, but also to differences in the digestive process as observed by Dupont-Prinet et al. (2010). Further investigation is required to determine the physiological processes underlying these observations, but present results strengthen the previously noted hypothesis that some individuals are more adapted to feed abundance whereas others are more adapted to feed deprivation. In contrast, measuring feed efficiency both in isolated aquariums and in groups, Besson et al. (2019) highlighted that fish with lower weight loss at fasting were more efficient. This difference might be due to the fact that Besson et al. (2019) used genetic information whereas present data are only phenotypic. For instance, de Verdal et al. (2018b) found no phenotypic correlation between feed efficiency and weight loss at fasting in Nile tilapia Oreochromis niloticus but found a strong genetic correlation between the same traits.
At the individual level, the most efficient fish at high feeding rates are not the most efficient at low feeding rates. Present results suggest that the feeding rate used to phenotype fish in selective breeding programs for feed efficiency must be the same as that used in commercial practices. However, the present results are only phenotypic and it is required to describe the genetic correlations between these traits before adding such characters in selective breeding programs.
In this study, WM and EM fish grew more at 24°C than at 18°C when fed at 100% ADL, but no population effect was significant. This contrasts with previous results (Vandeputte et al., 2014) showing EM had higher growth rates compared to other populations when reared at an average of 24.4°C. This difference may be due to the fact that Vandeputte et al. (2014) used EM×AT and EM×WM hybrids to extract additive effects of the EM population, whereas in the present study EM fish were in fact 75% EM-25% WM. Furthermore, in the present study, WM and EM fish showed an increase of FI and StdFI with temperature, similar to evidence of increase of FI with temperature reported in larger European sea bass (Lanari et al., 2002). The fact that AT fish did not exhibit a lower BWG and FI at 18°C than at 24°C could be explained by evolutionary differences between AT and the two Mediterranean populations (WM and EM). In particular, one possible explanation is a potential specific adaptation of AT population to lower temperatures, since the Atlantic Ocean is colder than the Mediterranean sea. The differentiation between the populations has been widely reported at the genomic level (Duranton et al., 2018) and associated with phenotypic variation in sex ratio, muscle fat or resistance to viral nervous necrosis (Doan et al., 2017; Guinand et al., 2017). Duranton et al. (2020) demonstrated that the maintenance of the genomic differentiation between the AT and Mediterranean populations was due to reproductive isolation barriers established after the ancient admixture of the Atlantic European sea bass with the closely related Dicentrarchus punctatus. In addition, the subsequent rapid fixation of some D. punctatus alleles in the Atlantic D. labrax could have provided a selective advantage in the Atlantic environment compared to ancestral D. labrax alleles (Duranton et al., 2020).
Results from the six different feeding rates (from 100% ADL to 0% ADL) showed that for an equal value of StdFI, fish from the EM population and fish reared at 18°C had the highest StdBWG, and thus were the most efficient. To explain these differences, it can be hypothesized that metabolic costs are not similar between the different temperatures and populations. Fish obtain energy from feed and invest this energy both into metabolism (to ensure routine requirements) and growth (Warren and Davis, 1967; Bureau et al., 2003). The use of energy could be differently balanced between metabolism and growth among the various populations and temperatures. In the case of temperature, the fact that higher temperatures increase fish metabolic costs is well known in various species (meta-analysis by Clarke and Johnston, 1999), including European sea bass (Claireaux and Lagardère, 1999). This supports the idea that fish reared at 24°C in the present study had higher metabolic costs and so were less efficient than fish reared at 18°C for an equal value of StdFI. Similarly, AT fish might have higher metabolic costs because their StdBWG was lower than other populations for an equal value of StdFI. However, differences in metabolic costs among populations have never been studied in European sea bass to our knowledge. Further investigation is required to assess these aspects. Alternatively, the observation that AT fish were less efficient may be linked to their muscle fat content, higher than in the Mediterranean populations (Vandeputte et al., 2014; F. Allal, personal communication, 2020). Indeed, it was demonstrated in several terrestrial and aquatic species that the most efficient animals had the lowest muscle fat content (Knap and Kause, 2018). As detailed by Knap and Kause (2018), deposition of 1 g of lipid leads to 1.1 g of weight gain, including 0.1 g of water in the associated adipose tissue. Conversely, deposition of 1 g of protein leads to 4–5 g of weight gain, including 3–4 g of water. Even if protein deposition is energetically more expensive than lipid deposition (59.9 kJ/g vs. 43.5–55.3 kJ/g), this higher energetic cost is small compared to the four to fivefold increase in weight gain associated to protein deposition (Knap and Kause, 2018). Considering an equal value of StdFI, fish reared at 18°C were more efficient than fish reared at 24°C, which was not the case when fish were fed at 100% ADL, i.e., with different values of StdFI. When fed at 100% ADL, fish reared at 24°C had a higher FI, permitting them to compensate for probably higher metabolic costs, and increasing the proportion of dietary energy allocated to growth.
Present results indicate that whatever the rearing temperature (18°C or 24°C), and for an equal feeding rate, the EM population had better individual feed efficiency than the AT and WM populations. This population effect remained when fish were fed at 100% ADL. In contrast, the impact of temperature on growth and feed efficiency was different whether fish were restricted or fed at 100% ADL. However, investigating a broader range of temperatures could give more generic results. Furthermore, these results still need to be validated in group rearing systems.
The need to obtain individual data regarding European sea bass FI implied the use of an individual rearing system. However, whether performance exhibited in individual rearing reflects what would be observed in group rearing is debatable. The level of 100% ADL ranged from 0.53 to 0.73% and from 0.85 to 1.12% of BW.day–1 at 18°C and 24°C, respectively, which is low compared to group rearing. With the model developed by Lanari et al. (2002) for European sea bass, 100% ADL was estimated to be around 1.1% and 1.7% of BW.day–1 at 18°C and 24°C, respectively, for fish weighing 26.5 g (mean weight at the beginning of the 100% ADL step). Feed provider tables were advising an even higher feeding rate: about 1.90% and 2.75% of BW.day–1 at 18°C and 24°C, respectively. It can be hypothesized that fish performance was degraded because of stress due to isolation, as it has been demonstrated that chronic stress lowers BWG and FI performance in European sea bass (Leal et al., 2011). Present results may also reflect individual variation in stress resistance because stress reaction is known to be highly variable among individuals. For instance, Volckaert et al. (2012) estimated a CV of 44.4% in plasma cortisol (stress marker) when an acute stress is applied in European sea bass. It is important to note that almost one quarter of the fish lost weight at 100% ADL in the present study, which can be considered as a non-adaptation to this isolation rearing system. In particular, at 24°C, more AT and WM than EM fish lost weight at 100% ADL. It suggests an interaction between population and temperature may exist in the ability to adapt to the isolation rearing system. This ability to adapt to the individual rearing system, whatever the temperature, may also be linked to the coping style of European sea bass. Indeed, we showed in a preliminary (unpublished) experiment that shy (reactive) fish adapted better to the individual rearing system than bold (proactive) fish. Moreover, the proportion of bold and shy fish might be different from a population to another, as a genetic basis to coping style has been evidenced in European sea bass (Ferrari et al., 2016). Further investigation is required to confirm this hypothesis. Nevertheless, it is still unknown whether stress or coping style affect the ranking of the fish based on the feed efficiency performance. Although individual rearing certainly has lowered fish performance, its relevance for selective breeding was supported by Besson et al. (2019) who demonstrated a link between individual feed efficiency, measured using the same isolated rearing system, and subsequent group feed efficiency. Thus, it can be suggested that the most efficient fish in the present study would still be the most efficient in “classical” group rearing.
At the individual level, the phenotypic variability reported here in the relationship between StdBWG and StdFI suggests opportunities to develop genetic breeding programs for feed efficiency. Whether this variability is genetically determined or not still needs to be addressed. However, the most efficient fish at high feeding rates were not the most efficient at low feeding rates, stressing the fact that feeding rate must be chosen carefully before phenotyping fish for selective breeding. Besides, it was observed that for an equal feeding rate, whatever the temperature, EM fish were the most efficient. Furthermore, for an equal feeding rate and whatever the population, fish were more efficient when reared at 18°C than at 24°C, but this effect disappeared when fish were fed at 100% ADL while the population effect remained. These results were measured on fish reared in isolation and need to be validated in group rearing, as well as at other development stages. Nevertheless, investigating the interactions between populations and temperatures seems a promising pathway to improve on-farm feed efficiency.
The datasets presented in this study can be found in online repositories. The names of the repository/repositories and accession number(s) can be found below: The data are available in the following link: https://doi.org/10.7910/DVN/1HTKPJ.
The animal study was reviewed and approved by Comité d’éthique en expérimentation animale Languedoc-Roussillon (authorization APAFiS n° 2018032109435819, version 2).
MV, FA, HdV, and JB got the funding to conduct the experiments. MV, FA, MB, HdV, and CR conceptualized the experiment. MV, FA, FC, and AV produced the biological material. CR conducted the experiment with support of MB, FC, AV, and HdV. CR, HdV, MV, FA, and MB analyzed the data. CR and HdV wrote the manuscript with substantial help from MV, FA, MB, and JB as well as corrections from FC and AV. All authors contributed to the article and approved the submitted version.
The authors declare that the research was conducted in the absence of any commercial or financial relationships that could be construed as a potential conflict of interest.
This publication was made possible through support provided by CIRAD and the CGIAR Research Program on Fish Agrifood Systems (FISH) and the International Fund for Agricultural Development (IFAD). The authors are grateful to the Ifremer Experimental Aquaculture Research Station staff and facilities and to H2020 AQUAEXCEL2020 (No. 652831).
Algur, S. P., and Biradar, J. G. (2017). Cooks distance and Mahanabolis distance outlier detection methods to identify review spam. Int. J. Eng. Comput. Sci. 6, 21638–21649. doi: 10.18535/ijecs/v6i6.16
Aubin, J., Papatryphon, E., van der Werf, H. M. G., and Chatzifotis, S. (2009). Assessment of the environmental impact of carnivorous finfish production systems using life cycle assessment. J. Clean. Prod. 17, 354–361. doi: 10.1016/j.jclepro.2008.08.008
Bates, D., Mächler, M., Bolker, B., and Walker, S. (2015). Fitting linear mixed-effects models using lme4. J. Stat. Softw. 67, 1–48. doi: 10.18637/jss.v067.i01
Besson, M., Allal, F., Chatain, B., Vergnet, A., Clota, F., and Vandeputte, M. (2019). Combining individual phenotypes of feed intake with genomic data to improve feed efficiency in sea bass. Front. Genet. 10:219. doi: 10.3389/fgene.2019.00219
Besson, M., Aubin, J., Komen, H., Poelman, M., Quillet, E., Vandeputte, M., et al. (2016a). Environmental impacts of genetic improvement of growth rate and feed conversion ratio in fish farming under rearing density and nitrogen output limitations. J. Clean. Prod. 116, 100–109. doi: 10.1016/j.jclepro.2015.12.084
Besson, M., Komen, H., Aubin, J., de Boer, I. J. M., Poelman, M., Quillet, E., et al. (2014). Economic values of growth and feed efficiency for fish farming in recirculating aquaculture system with density and nitrogen output limitations: a case study with African catfish (Clarias gariepinus). J. Anim. Sci. 92, 5394–5405. doi: 10.2527/jas.2014-8266
Besson, M., Vandeputte, M., van Arendonk, J. A. M., Aubin, J., de Boer, I. J. M., Quillet, E., et al. (2016b). Influence of water temperature on the economic value of growth rate in fish farming: the case of sea bass (Dicentrarchus labrax) cage farming in the Mediterranean. Aquaculture 462, 47–55. doi: 10.1016/j.aquaculture.2016.04.030
Bureau, D. P., Kaushik, S. J., and Cho, C. Y. (2003). “Bioenergetics,” in Fish Nutrition, eds J. E. Halver and R. W. Hardy (San Diego, CA: Academic Press), 1–59.
Claireaux, G., and Lagardère, J.-P. (1999). Influence of temperature, oxygen and salinity on the metabolism of the European sea bass. J. Sea Res. 42, 157–168. doi: 10.1016/S1385-1101(99)00019-2
Clarke, A., and Johnston, N. M. (1999). Scaling of metabolic rate with body mass and temperature in teleost fish. J. Anim. Ecol. 68, 893–905. doi: 10.1046/j.1365-2656.1999.00337.x
De Silva, S. S., and Anderson, T. A. (1995). Fish Nutrition in Aquaculture. London: Chapman and Hall.
de Verdal, H., Komen, H., Quillet, E., Chatain, B., Allal, F., Benzie, J. A. H., et al. (2018a). Improving feed efficiency in fish using selective breeding: a review. Rev. Aquacult. 10, 833–851. doi: 10.1111/raq.12202
de Verdal, H., Vandeputte, M., Mekkawy, W., Chatain, B., and Benzie, J. A. H. (2018b). Quantifying the genetic parameters of feed efficiency in juvenile Nile tilapia Oreochromis niloticus. BMC Genet. 19:105. doi: 10.1186/s12863-018-0691-y
Doan, Q. K., Vandeputte, M., Chatain, B., Haffray, P., Vergnet, A., Breuil, G., et al. (2017). Genetic variation of resistance to Viral Nervous Necrosis and genetic correlations with production traits in wild populations of the European sea bass (Dicentrarchus labrax). Aquaculture 478, 1–8. doi: 10.1016/j.aquaculture.2017.05.011
Drouilhet, L., Achard, C. S., Zemb, O., Molette, C., Gidenne, T., Larzul, C., et al. (2016). Direct and correlated responses to selection in two lines of rabbits selected for feed efficiency under ad libitum and restricted feeding: I. Production traits and gut microbiota characteristics. J. Anim. Sci. 94, 38–48. doi: 10.2527/jas.2015-9402
Dupont-Prinet, A., Chatain, B., Grima, L., Vandeputte, M., Claireaux, G., and McKenzie, D. J. (2010). Physiological mechanisms underlying a trade-off between growth rate and tolerance of feed deprivation in the European sea bass (Dicentrarchus labrax). J. Exp. Biol. 213, 1143–1152. doi: 10.1242/jeb.037812
Duranton, M., Allal, F., Fraïsse, C., Bierne, N., Bonhomme, F., and Gagnaire, P.-A. (2018). The origin and remolding of genomic islands of differentiation in the European sea bass. Nat. Commun. 9:2518. doi: 10.1038/s41467-018-04963-6
Duranton, M., Allal, F., Valière, S., Bouchez, O., Bonhomme, F., and Gagnaire, P.-A. (2020). The contribution of ancient admixture to reproductive isolation between European sea bass lineages. Evol. Lett. 4, 226–242. doi: 10.1002/evl3.169
FAO (2018). The State of World Fisheries and Aquaculture 2018 - Meeting the Sustainable Development Goals. Rome: Food and Agriculture Organization of the United Nations.
Ferrari, S., Horri, K., Allal, F., Vergnet, A., Benhaim, D., Vandeputte, M., et al. (2016). Heritability of boldness and hypoxia avoidance in European seabass, Dicentrarchus labrax. PLoS One 11:e0168506. doi: 10.1371/journal.pone.0168506
Fonds, M., Cronie, R., Vethaak, A. D., and Van Der Puyl, P. (1992). Metabolism, food consumption and growth of plaice (Pleuronectes platessa) and flounder (Platichthys flesus) in relation to fish size and temperature. Neth. J. Sea Res. 29, 127–143. doi: 10.1016/0077-7579(92)90014-6
Froehlich, H. E., Runge, C. A., Gentry, R. R., Gaines, S. D., and Halpern, B. S. (2018). Comparative terrestrial feed and land use of an aquaculture-dominant world. Proc. Natl. Acad. Sci. U.S.A. 115, 5295–5300. doi: 10.1073/pnas.1801692115
Grima, L., Chatain, B., Ruelle, F., Vergnet, A., Launay, A., Mambrini, M., et al. (2010). In search for indirect criteria to improve feed utilization efficiency in sea bass (Dicentrarchus labrax): part II: heritability of weight loss during feed deprivation and weight gain during re-feeding periods. Aquaculture 302, 169–174. doi: 10.1016/j.aquaculture.2010.02.016
Guinand, B., Vandeputte, M., Dupont-Nivet, M., Vergnet, A., Haffray, P., Chavanne, H., et al. (2017). Metapopulation patterns of additive and nonadditive genetic variance in the sea bass (Dicentrarchus labrax). Ecol. Evol. 7, 2777–2790. doi: 10.1002/ece3.2832
Knap, P. W., and Kause, A. (2018). Phenotyping for genetic improvement of feed efficiency in fish: lessons from pig breeding. Front. Genet. 9:184. doi: 10.3389/fgene.2018.00184
Kuznetsova, A., Brockhoff, P. B., and Christensen, R. H. B. (2017). lmerTest package: tests in linear mixed effects models. J. Stat. Softw. 82, 1–26. doi: 10.18637/jss.v082.i13
Lanari, D., D’Agaro, E., and Ballestrazzi, R. (2002). Growth parameters in European sea bass (Dicentrarchus labrax L.): effects of live weight and water temperature. Ital. J. Anim. Sci. 1, 181–185. doi: 10.4081/ijas.2002.181
Leal, E., Fernández-Durán, B., Guillot, R., Ríos, D., and Cerdá-Reverter, J. M. (2011). Stress-induced effects on feeding behavior and growth performance of the sea bass (Dicentrarchus labrax): a self-feeding approach. J. Comp. Physiol. B Biochem. Syst. Environ. Physiol. 181, 1035–1044. doi: 10.1007/s00360-011-0585-z
Lenth, R. V. (2016). Least-squares means: the R package lsmeans. J. Stat. Softw. 69, 1–33. doi: 10.18637/jss.v069.i01
Lindgren, D., and Håkanson, L. (2011). Morphometric classification and GIS-based data analysis in coastal modeling and management. Open Environ. Sci. 5, 1–17. doi: 10.2174/1876325101105010001
Lupatsch, I., Kissil, G. W., and Sklan, D. (2003). Comparison of energy and protein efficiency among three fish species gilthead sea bream (Sparus aurata), European sea bass (Dicentrarchus labrax) and white grouper (Epinephelus aeneus): energy expenditure for protein and lipid deposition. Aquaculture 225, 175–189. doi: 10.1016/S0044-8486(03)00288-6
Martins, C. I. M., Conceição, L. E. C., and Schrama, J. W. (2011). Feeding behavior and stress response explain individual differences in feed efficiency in juveniles of Nile tilapia Oreochromis niloticus. Aquaculture 312, 192–197. doi: 10.1016/j.aquaculture.2010.12.035
McKenzie, D. J., Vergnet, A., Chatain, B., Vandeputte, M., Desmarais, E., Steffensen, J. F., et al. (2014). Physiological mechanisms underlying individual variation in tolerance of food deprivation in juvenile European sea bass, Dicentrarchus labrax. J. Exp. Biol. 217, 3283–3292. doi: 10.1242/jeb.101857
Nguyen, N. H., McPhee, C. P., and Wade, C. M. (2005). Responses in residual feed intake in lines of Large White pigs selected for growth rate on restricted feeding (measured on ad libitum individual feeding). J. Anim. Breed. Genet. 122, 264–270. doi: 10.1111/j.1439-0388.2005.00531.x
NRC (2011). Nutrient Requirements of Fish and Shrimp. Animal Nutrition Series. National Research Council of the National Academies. Washington, DC: The National Academies Press.
Paloheimo, J. E., and Dickie, L. M. (1966). Food and growth of fishes. II. Effects of food and temperature on the relation between metabolism and body weight. J. Fish. Res. Board Can. 23, 869–908. doi: 10.1139/f66-077
Person-Le Ruyet, J., Mahé, K., Le Bayon, N., and Le Delliou, H. (2004). Effects of temperature on growth and metabolism in a Mediterranean population of European sea bass, Dicentrarchus labrax. Aquaculture 237, 269–280. doi: 10.1016/j.aquaculture.2004.04.021
R Core Team (2018). R: A Language and Environment for Statistical Computing. Vienna: R Foundation for Statistical Computing.
Rodde, C., Chatain, B., Vandeputte, M., Quoc Trinh, T., Benzie, J. A. H., and de Verdal, H. (2020). Can individual feed conversion ratio at commercial size be predicted from juvenile performance in individually reared Nile tilapia Oreochromis niloticus? Aquac. Rep. 17:100349. doi: 10.1016/j.aqrep.2020.100349
Saravanan, S., Schrama, J. W., Figueiredo-Silva, A. C., Kaushik, S. J., Verreth, J. A. J., and Geurden, I. (2012). Constraints on energy intake in fish: the link between diet composition, energy metabolism, and energy intake in rainbow trout. PLoS One 7:e34743. doi: 10.1371/journal.pone.0034743
Silverstein, J. T. (2006). Relationships among feed intake, feed efficiency, and growth in juvenile rainbow trout. N. Am. J. Aqu. 68, 168–175. doi: 10.1577/A05-010.1
Troell, M., Naylor, R. L., Metian, M., Beveridge, M., Tyedmers, P. H., Folke, C., et al. (2014). Does aquaculture add resilience to the global food system? Proc. Natl. Acad. Sci. U.S.A. 111, 13257–13263. doi: 10.1073/pnas.1404067111
Vandeputte, M., Garouste, R., Dupont-Nivet, M., Haffray, P., Vergnet, A., Chavanne, H., et al. (2014). Multi-site evaluation of the rearing performances of 5 wild populations of European sea bass (Dicentrarchus labrax). Aquaculture 424–425, 239–248. doi: 10.1016/j.aquaculture.2014.01.005
Volckaert, F. A. M., Hellemans, B., Batargias, C., Louro, B., Massault, C., Van Houdt, J. K. J., et al. (2012). Heritability of cortisol response to confinement stress in European sea bass Dicentrarchus labrax. Genet. Sel. Evol. 44:15. doi: 10.1186/1297-9686-44-15
Keywords: aquaculture, individual rearing, feed efficiency, feeding rate, fasting tolerance, sea bass
Citation: Rodde C, Vandeputte M, Allal F, Besson M, Clota F, Vergnet A, Benzie JAH and de Verdal H (2020) Population, Temperature and Feeding Rate Effects on Individual Feed Efficiency in European Sea Bass (Dicentrarchus labrax). Front. Mar. Sci. 7:578976. doi: 10.3389/fmars.2020.578976
Received: 01 July 2020; Accepted: 18 September 2020;
Published: 07 October 2020.
Edited by:
Ivan Viegas, University of Coimbra, PortugalReviewed by:
Leonardo Julián Magnoni, University of Porto, PortugalCopyright © 2020 Rodde, Vandeputte, Allal, Besson, Clota, Vergnet, Benzie and de Verdal. This is an open-access article distributed under the terms of the Creative Commons Attribution License (CC BY). The use, distribution or reproduction in other forums is permitted, provided the original author(s) and the copyright owner(s) are credited and that the original publication in this journal is cited, in accordance with accepted academic practice. No use, distribution or reproduction is permitted which does not comply with these terms.
*Correspondence: Hugues de Verdal, aHVndWVzLmRlX3ZlcmRhbEBjaXJhZC5mcg==
Disclaimer: All claims expressed in this article are solely those of the authors and do not necessarily represent those of their affiliated organizations, or those of the publisher, the editors and the reviewers. Any product that may be evaluated in this article or claim that may be made by its manufacturer is not guaranteed or endorsed by the publisher.
Research integrity at Frontiers
Learn more about the work of our research integrity team to safeguard the quality of each article we publish.