- 1Institute of Marine Environment and Ecology, National Taiwan Ocean University, Keelung, Taiwan
- 2Center of Excellence for the Oceans, National Taiwan Ocean University, Keelung, Taiwan
Oligotrich ciliates play a key role in linking microbial food webs to the traditional grazing food chain. Hence, the numerical (growth) and functional (grazing) responses of oligotrich ciliates are very important issues in studies of marine ecosystems. Most oligotrich ciliates feed mainly on nanoflagellates, while some of them also have the ability to consume bacteria. Up until now, studies of ciliates grazing on algae have not specifically excluded the effects of bacteria. In the present study, we found that the presence of bacteria in the algal culture medium affected the growth and grazing rates of ciliates grazing on nanoflagellates, resulting in an overestimate of gross growth efficiency at low relative algal concentrations. Strombidium sp. is prey selective mainly grazing on bacteria at low relative algal concentrations, but on algae at high relative algal concentrations. Carbon obtained from ciliate grazing on bacteria should be taken into account in the coastal zone surveys and especially in culture experiments to avoid unreasonable results of carbon flow.
Introduction
Oligotrichea, which mainly comprise the Choreotrichida and Oligotrichida, are the dominant group of ciliates in the microzooplankton (Agatha, 2011). Oligotrich ciliates are important consumers in the microbial loop and play a key role in linking microbial food webs to the traditional grazing food chain (Azam et al., 1983; Gifford, 1991; Pierce and Turner, 1992; Liu et al., 2005). The growth and grazing responses of oligotrich ciliates are very important issues in studies of marine ecosystems, inasmuch as they are among the factors that affect energy transfer (Verity, 1985; Calbet and Saiz, 2005). The composition of oligotrich ciliates often changes greatly depending on both biotic and abiotic factors (Fuhrman et al., 2006). Hence, studies of the relationship between a single species of oligotrich ciliate and its prey provide fundamental data for understanding the physiological differences between different kinds of prey.
Some studies suggest that food size (Fenchel, 1986; Jonsson, 1986; Bernard and Rassoulzadegan, 1990; Hansen et al., 1994) and food quality (Chen et al., 2010; Montagnes et al., 2011) determine the feeding behavior of ciliates (Löder et al., 2011). However, size may only be a physical constraint (Thurman et al., 2010) while other features of the prey are also likely to play an important role in the ciliates grazing process. Yang et al. (2015), for example, suggested that the swimming mode of algal cells is important in affecting the prey selectivity of ciliates.
Although most oligotrich ciliates ingest mainly nanoflagellates, some of them such as Strombidium sulcatum also have the ability to consume bacteria (Rivier et al., 1985; Bernard and Rassoulzadegan, 1990). Strombidium sulcatum occurs in many marine areas and is easy to culture (Jiang et al., 2011; Wickham et al., 2011; Xu et al., 2011). As a result, it has come to serve as a model organism (Fenchel and Jonsson, 1988). Previous studies of bacterial consumption by this species have used pico-sized prey, including live bacteria, heat-killed bacteria, fluorescently labeled bacteria (FLB), and non-living particles (Fenchel and Jonsson, 1988; Allali et al., 1994; Dolan and Šimek, 1997; Christaki et al., 1998). Studies of ciliate grazing on algae have also been done, but without controlling for the presence of bacteria (Montagnes, 1996; Gismervik, 2005). The only effective way to reduce the effect of bacteria and to make the culture sterile is to add antibiotics to the medium, but this method can affect the health of both the predator and the prey (Turner and Lloyd, 1971; Hagenbuch and Pinckney, 2012).
The presence of bacteria in the algal culture medium will inevitably affect the results of growth and grazing experiments of ciliates feeding on nanoflagellates. Yang et al. (2015) found that cultured Strombidium cf. sulcatum did not ingest algae significantly but still showed growth; they considered that this was due to the appearance of bacteria in the algal culture medium. Ignoring bacteria ingested during the experiments could result in underestimates of the ingestion rates of the ciliates as well as overestimates of their growth rates and gross growth efficiencies (GGE). At low relative algal concentrations, Chen et al. (2010) reported greatly random values of GGE, with some being remarkably high.
In order to understand (1) the grazing selection of ciliates on haptophytes (T-ISO) versus bacteria, and (2) the influence of the presence of bacteria in grazing experiments of ciliates on haptophytes, we studied the growth of the bacterivorous oligotrich ciliate Strombidium sp., isolated from the coastal waters of northeastern Taiwan, under two culture conditions, viz., (I) grazing on the haptophyte Isochrysis galbana (T-ISO) with bacteria present in the water column and (II) grazing only on bacteria isolated from the rice-grain-raised water column.
Materials and Methods
Cultures
Strombidium sp. was collected from coastal waters of northeastern Taiwan. A single-cell culture of Strombidium sp. in a 6-well culture plate was fed on bacteria and maintained at 25°C in a 12:12 light:dark cycle at 100 μmol photons m–2 s–1 in 0.2 μm filtered seawater at a salinity of 30 (practical salinity scale). Before culturing, a petri dish (Boeco, about 9 cm across; water depth about 1 cm) with several raw rice grains in 0.2 μm-filtered seawater was prepared and set aside for a week under the same light and temperature conditions as the ciliates in order to grow bacteria and kept in exponential growth phase. The haptophyte Isochrysis galbana (T-ISO) was maintained at 20°C in a 12:12 light:dark cycle at 100 μmol photons m–2 s–1 in f/2-filtered seawater (Guillard and Ryther, 1962) at a salinity of 30 in a culture flask (BD Falcon) and kept in exponential growth phase.
Growth, Ingestion and GGE
Growth and ingestion experiments at different food concentrations were conducted in six-well cell culture plates (GeneDireX) using T-ISO (in non-germ free condition, i.e., with bacteria in the water column) and bacteria (isolated from the rice-grain-raised water column) as prey, respectively, as the two culture conditions. Algal and bacterial food ranged from 150–2 × 104 cells mL–1 and 104–106 cells mL–1, respectively, with values chosen to represent a range of saturated and unsaturated conditions based on preliminary experiments. Before the experiment, individual Strombidium sp. cells were isolated with a capillary tube (Kibble, AK71900-00100), transferred to a well of 0.2-μm-filtered sterile seawater, washed gently, and transferred to another two wells in succession in order to remove or substantially dilute any bacteria on the cell surface or in the water. Afterwards, each Strombidium sp. cell was maintained in 0.2-μm-filtered sterile seawater and allowed to starve for 1 day to empty the residual food vacuoles in each cells for the standardization of the growth and ingestion experiments. Light and temperature conditions were the same as for culture maintenance of ciliates, as described above.
For use in experiments and as controls, T-ISO were placed in triplicate 12 mL f/2 filtered seawater (GeneDireX) in concentrations of 150, 300, 625, 1250, 2500, 5000, 10000, and 20000 cells mL–1. Before transferring Strombidium sp. cells into the wells, a 2 mL water subsample was collected from each well in a cryogenic tube (Nalgene), fixed by 1% PFA (paraformaldehyde) and stored in a −80°C freezer for later analysis with a flow cytometer (BD) to obtain the initial prey concentrations. Ten starved Strombidium sp. cells were transferred into each experimental well; none were introduced to the control wells. Light and temperature conditions for the experiments were the same as for the culturing ciliates as described above. After 3 days of culture, another 2 mL water subsample was collected from each well in order to check the final prey concentrations by flow cytometer as above. The remaining 8 mL of water in each well was fixed with Lugol’s iodine to a final concentration of 5% in order to count the Strombidium sp. cells under an inverted microscope (NIKON Optiphot-2, JAPAN). The growth and ingestion experiments for Strombidium sp. on bacteria alone were conducted the same way as those on T-ISO with initial bacteria concentrations of 104, 105, and 106 cells mL–1.
Specific growth rates of Strombidium sp., μ (d–1), were calculated between the initial and final sampling points:
where S0 is the measured concentration of Strombidium sp. at the beginning of incubation, St is the measured concentration at the end of incubation, and t is the time of incubation in days.
Ingestion rates of Strombidium sp., I (ng C ciliate–1 d–1), were calculated according to the equation of Frost (1972) as modified by Heinbokel (1978), which accounts for depletion of prey and any change in predator number over the incubation period as:
where g (specific grazing rate) = Vn–Vw, the difference between prey net growth rate without predator (Vn) and with predator (Vw), mean prey concentration P = (Pt–P0) × [ln(Pt × P0–1)]–1 and mean predator concentration S = (St–S0) × [ln(St × S0–1)]–1.
Growth and ingestion rate data, as a function of prey concentration, were fit to a modified Michaelis-Menten equation (Montagnes, 1996) using the trial and error method (Paasche, 1973) to get the best regression analysis by estimating x0 (prey concentration threshold) from x (prey concentration) in advance:
where V is the ciliate growth or ingestion rate, Vmax is the maximum rate, x is the prey concentration, x0 is the feeding threshold (x-intercept), and Km is the half-saturation constant (where μ = 1/2 Vmax), which describes how rapidly V approaches its maximum.
The gross growth efficiency (GGE) of Strombidium sp. was calculated as the ratio of ciliate growth in terms of carbon to ingested prey carbon. The carbon contents per cell for Strombidium sp., Isochrysis galbana (T-ISO) and bacteria are 2.62 ng C cell–1 according to the C : vol ratios of 0.19 pg μm–3 (Putt and Stoecker, 1989), 15.4 pg C cell–1 (Chen et al., 2010) and 149 fg C cell–1 according to its mean volume of 1 μm3 observed under an epifluorescence microscope and its carbon-volume ratios from 51 to 241 fg C μm–3 (Vrede et al., 2002), respectively.
Protistan growth rate can be modeled as linear functions of temperature (Atkinson et al., 2003; Montagnes et al., 2003; Kimmance et al., 2006). In the present study, the growth rates of free-living protists were normalized to 25°C using linear regression (0.07 d–1 °C–1, Montagnes et al., 2003) in Table 1. However, Kimmance et al. (2006) suggested that ingestion increases linearly with increasing temperature between 8 and 15°C, not in the range of culture temperature in Table 2. The difference in ingestion rates between oligotrich ciliates might be related to the size of grazers and the temperature used in the studies (Yang et al., 2015). Hence, the grazing rates were not normalized in the present study.
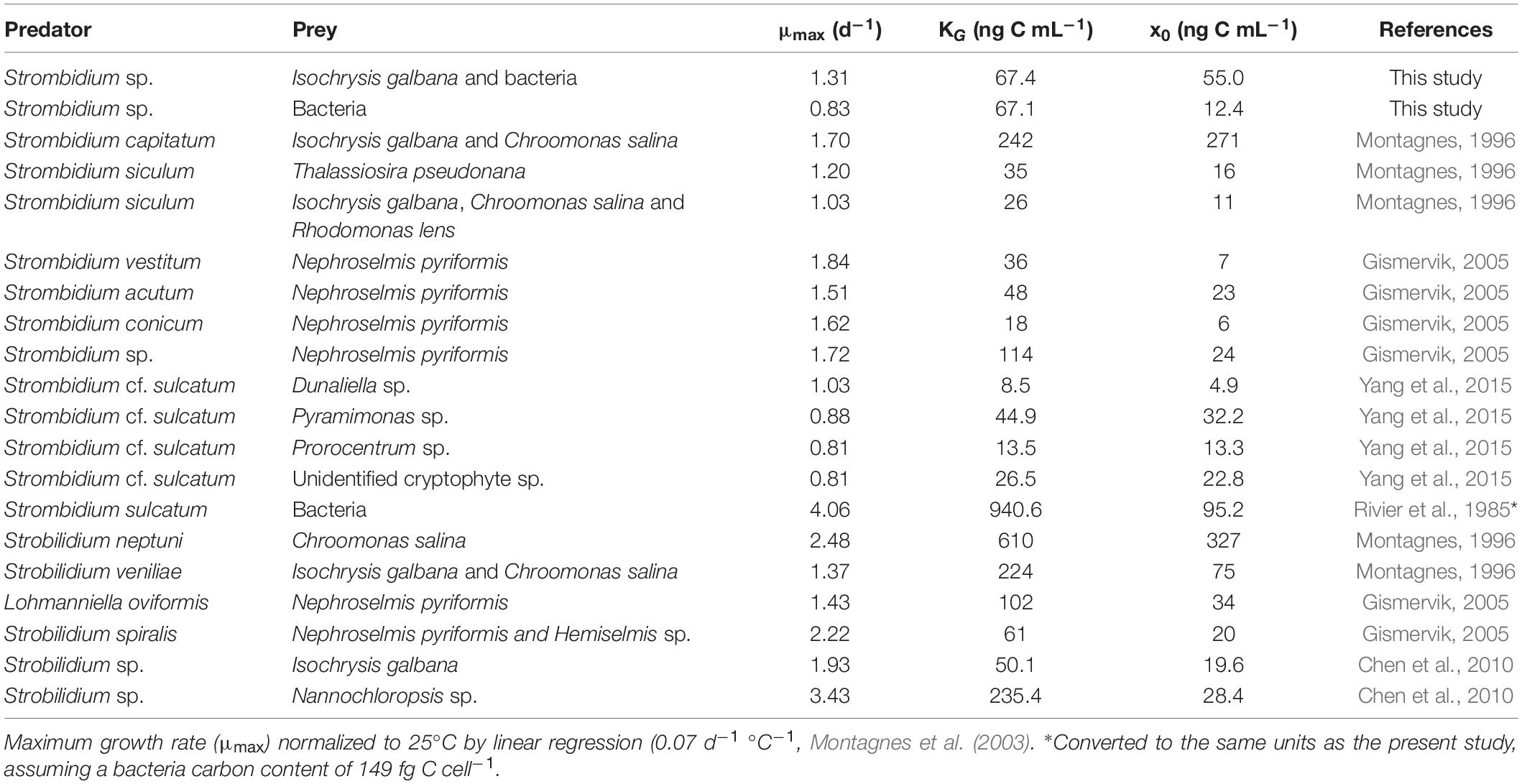
Table 1. Parameters of growth rates for oligotrich ciliates in previous studies (laboratory culture).
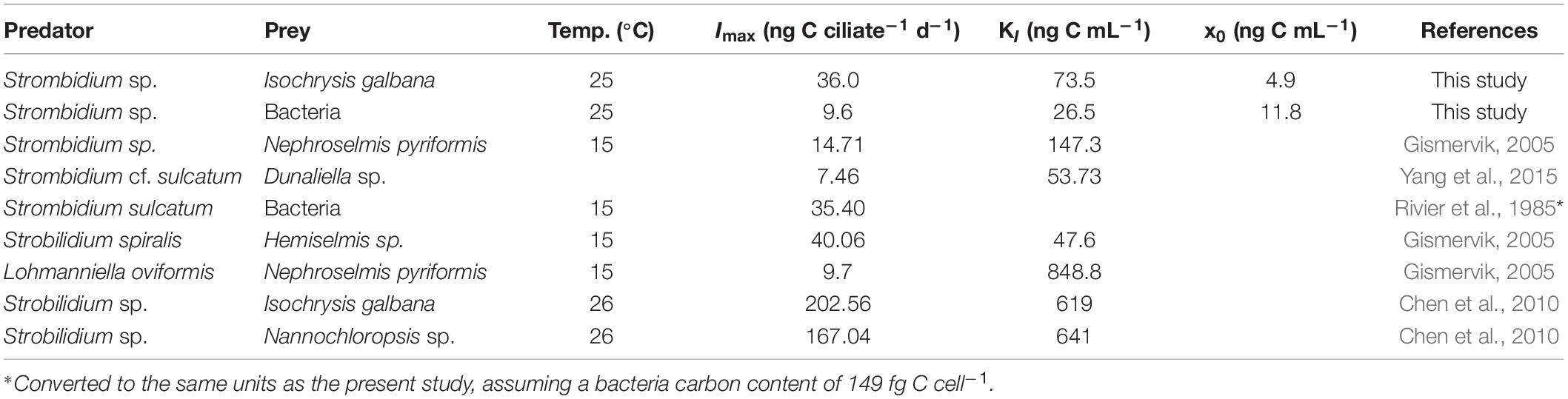
Table 2. Parameters of ingestion rates for oligotrich ciliates in previous studies (laboratory culture).
Results
Growth rate of Strombidium sp.
The numerical response of Strombidium sp. feeding on T-ISO (in the presence of bacteria) and on bacteria alone are shown in Figure 1. The hyperbolic regression equation of each growth curve was obtained using the trial-and-error method, in which μmax, KG and x0 were 1.31 d–1, 67.4 ng C mL–1 and 55.0 ng C mL–1, respectively, when grazing on T-ISO (in the presence of bacteria) and 0.83 d–1, 67.1 ng C mL–1 and 12.4 ng C mL–1, respectively, when grazing on bacteria only.
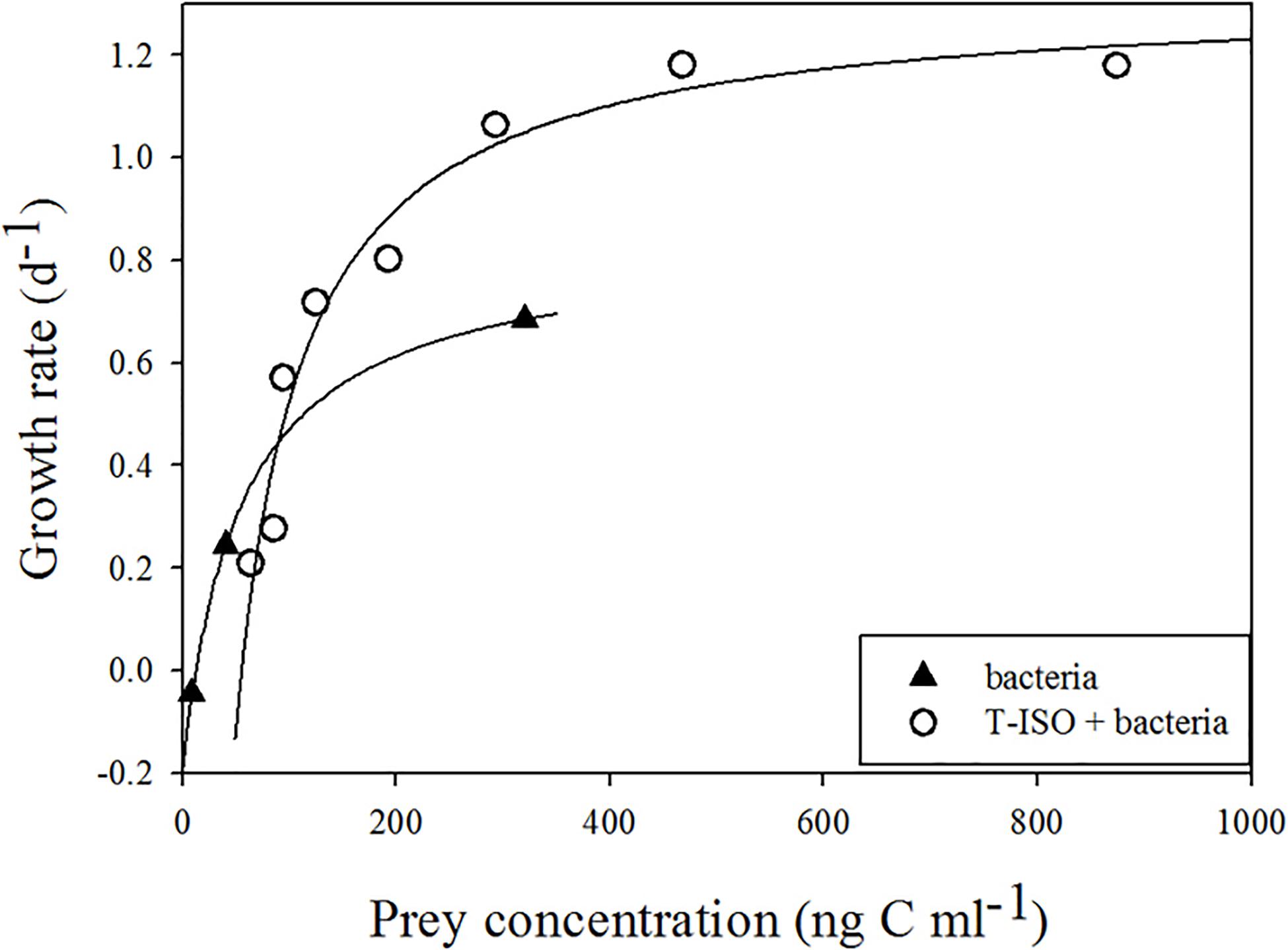
Figure 1. Growth rate of Strombidium sp. The fitted equations are μ = 1.31(x–55)/ [67.4 + (x–55)] (r2 = 0.96) for grazing on the haptophyte Isochrysis galbana (in the presence of bacteria) and μ = 0.83(x–12.4)/ [67.1 + (x–12.4)] (r2 = 1) for grazing on bacteria only.
The maximum growth rate (μmax) of Strombidium sp. on T-ISO (in the presence of bacteria) was higher than that on bacteria alone, showing that T-ISO is a better prey than bacteria for growing Strombidium sp. The half-saturation constants (KG) for two culture conditions were similar, indicating the same requirement of prey concentration to achieve half of the maximum growth rate. The threshold value (x0) of Strombidium sp. grazing on bacteria was lower than that for T-ISO (in the presence of bacteria), but the growth rate was nonetheless positive even under low bacterial concentrations, demonstrating an important role for bacteria.
Ingestion Rate of Strombidium sp.
Figure 2 shows the functional response of Strombidium sp. on different preys. For Strombidium sp. grazing on T-ISO (in the presence of bacteria), the curve matched hyperbolic distributions produced using the trial-and-error method, with Imax = 36.0 ng C ciliate–1 d–1, KI = 73.5 ng C mL–1 and x0 = 4.9 ng C mL–1. In contrast, the ingestion rate of Strombidium sp. on bacteria in the algal medium could not be fitted to a modified Michaelis-Menten equation.
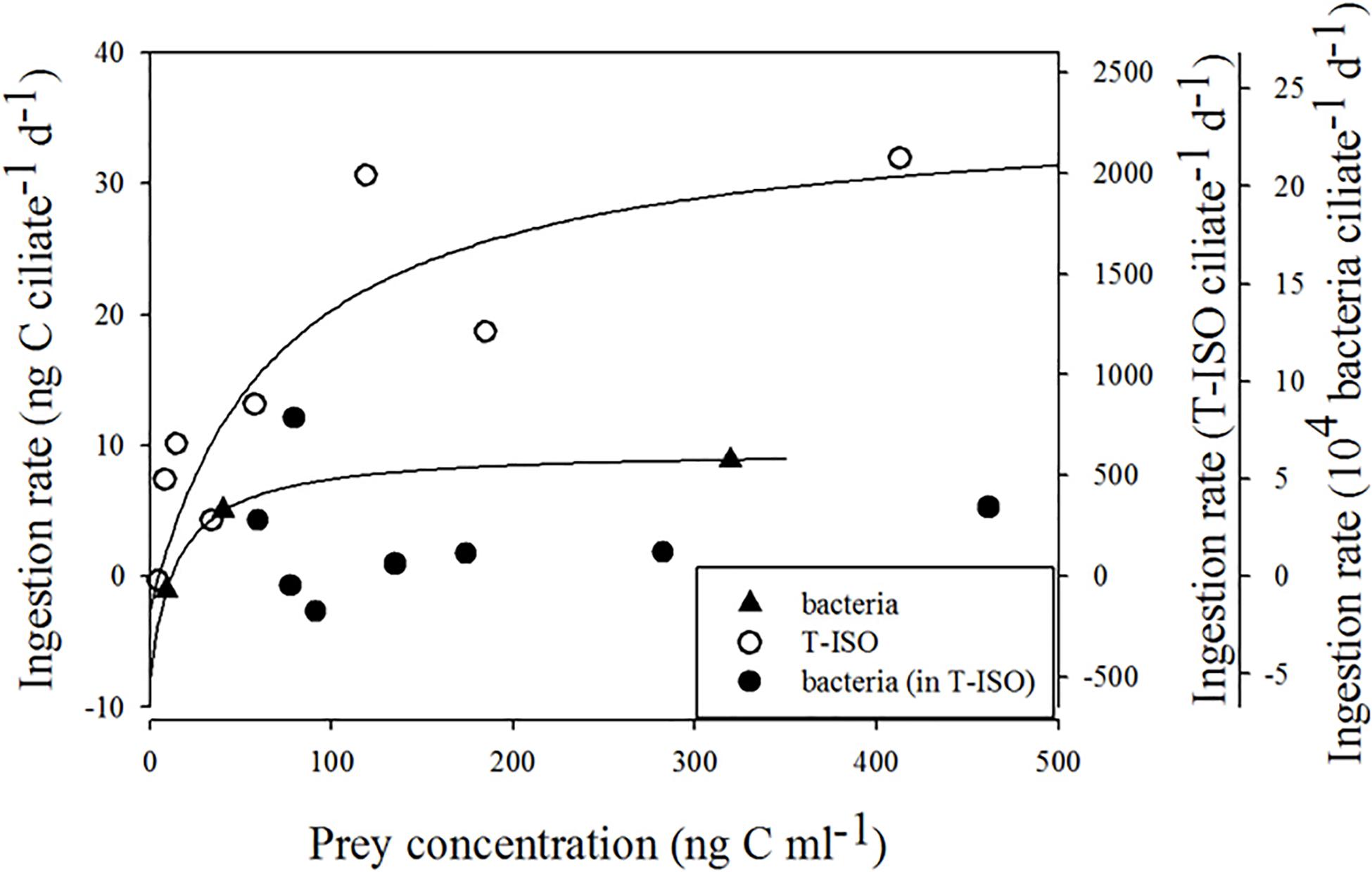
Figure 2. Ingestion rate of Strombidium sp. The fitted equation are I = 36.03 (x–4.9) / [73.5 + (x–4.9)] (r2 = 0.76) for grazing on the haptophyte Isochrysis galbana (in the presence of bacteria) and I = 9.64 (x–11.8) / [26.5 + (x–11.8)] (r2 = 0.999) for grazing on bacteria only.
As for Strombidium sp. feeding on bacteria alone, the ingestion rate did fit a modified equation produced by the trial-and-error method, with Imax = 9.6 ng C ciliate–1 d–1, KI = 26.5 ng C mL–1 and x0 = 11.8 ng C mL–1. The maximum ingestion rate of Strombidium sp. grazing on Isochrysis galbana (T-ISO) (in the presence of bacteria) was higher than that on bacteria alone.
Gross Growth Efficiency (GGE) of Strombidium sp.
The gross growth efficiency (GGE) of Strombidium sp. was between 6 and 15% when grazing on T-ISO (in the presence of bacteria), but between 12 and 20% when grazing bacteria only (Figure 3). The GGE at low prey concentration with negative growth and ingestion rates is shown as 0.
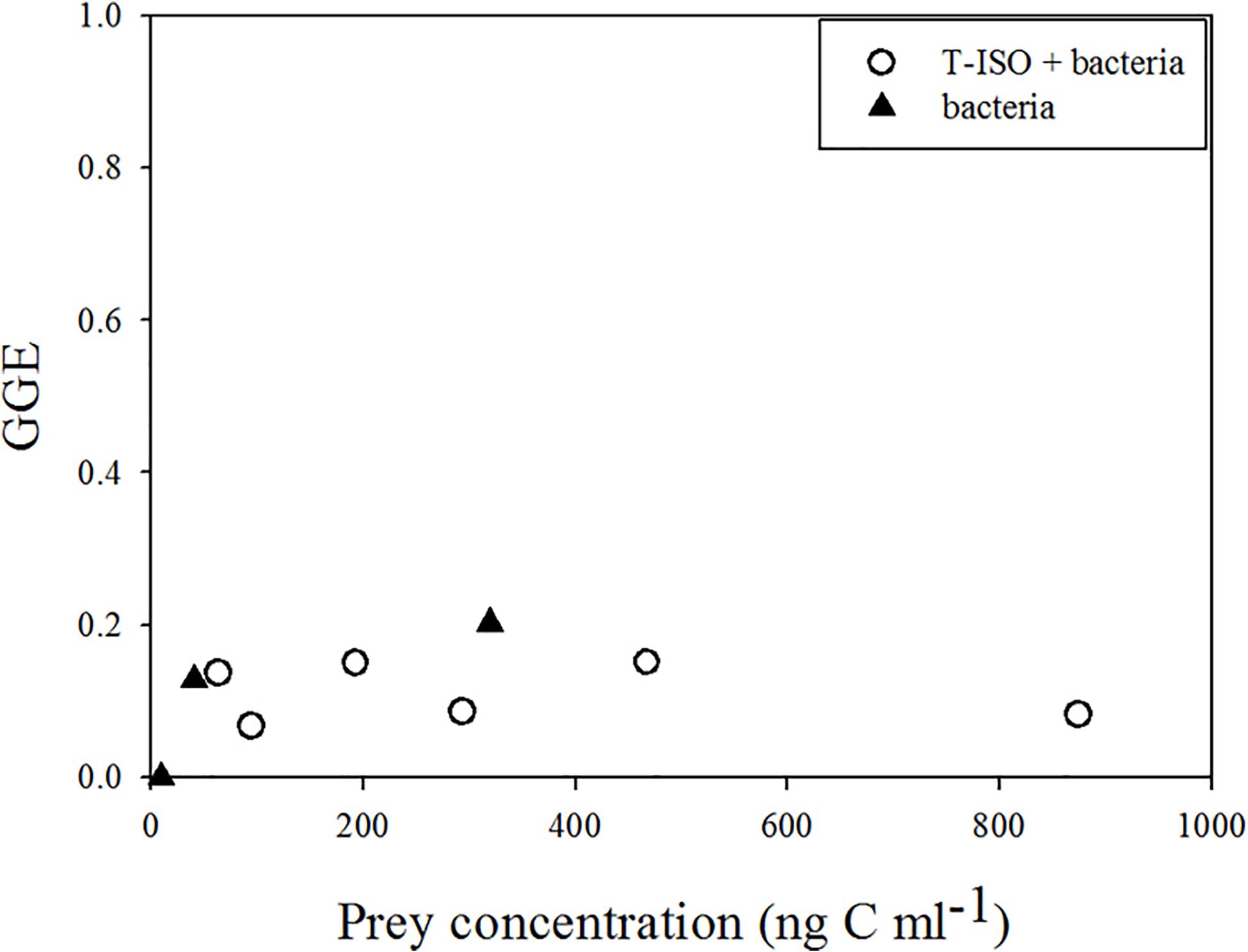
Figure 3. Gross growth efficiency (GGE) of Strombidium sp. when (circle) grazing on the haptophyte Isochrysis galbana (in the presence of bacteria) and (triangle) grazing on bacteria only.
Discussion
Growth Rate of Strombidium sp.
Ciliates can generally undergo 1–2 binary fissions per day (Pierce and Turner, 1992; Perez et al., 1997), which means that they can grow as fast as, or even faster, than their preys such as phytoplankton (Kamiyama, 2015). Studies of the relationship between growth rate and various environmental factors will help us to understand the dynamic changes of ciliates in the natural environment (Müller and Geller, 1993). In the present study, the growth rate parameters fitted to the curves of the modified Michaelis-Menten equations were within the range of those recorded all over the world (Table 1), with a maximum growth rate (μmax) of 0.11–3.50 d–1, a half-saturation constant (KG) of 8.5–940.6 ng C mL–1 and a threshold value (x0) of 6–327 ng C mL–1 at culture temperatures between 15 and 26°C. For Strombidium sp. grazing on T-ISO (in the presence of bacteria) at a temperature of 25°C, these parameters were similar to those of Chen et al. (2010), who used the same prey Isochrysis galbana (T-ISO) at a temperature of 26°C in a similar study of the choreotrich ciliate Strobilidium sp. Both species of ciliate appear to have similar numerical responses at the same prey (T-ISO) concentrations.
The growth rate parameters for Strombidium sp. grazing on bacteria were lower than those observed by Rivier et al. (1985), who fed bacteria to Strombidium sulcatum. Strombidium sp. could maintain its basic metabolism and showed a positive growth rate at low bacterial concentrations, and in that respect was superior to Strombidium sulcatum at low prey concentrations. However, as the concentration of bacteria increased, the maximum growth rate of Strombidium sp. was lower than that of Strombidium sulcatum, which suggests that bacteria are not the most suitable food for Strombidium sp.
Ingestion Rate of Strombidium sp.
The ingestion rate parameters for oligotrich ciliates as recorded by previous studies are shown in Table 2, with a maximum ingestion rate (Imax) between 7.5 and 202.6 ng C ciliate–1 d–1 and a half-saturation constant (KI) between 26.5 and 848.8 ng C mL–1 at temperatures between 15 and 26°C. Imax and KI of Strombidium sp. grazing on T-ISO (in the presence of bacteria) were lower than those of Strobilidium sp. from Chen et al. (2010), i.e., 36.0 vs. 202.6 ng C ciliate–1 d–1 for Imax and 73.5 vs. 619 ng C mL–1 for KI, with the same prey Isochrysis galbana (Table 2). The gross growth efficiency (GGE) of Strobilidium sp. was between 10 and 70% at low prey concentrations, but about 10% at high prey concentrations (Chen et al., 2010). Since these two ciliates have similar growth curves with the same prey (T-ISO) as mentioned above (Table 1), the high GGE at low prey concentrations makes it reasonable to speculate that Strobilidium sp. has the ability to ingest bacteria.
Imax was 9.6 ng C ciliate–1 d–1 and KI was 26.5 ng C mL–1 when Strombidium sp. grazed on bacteria only. The half-saturation constant (KI) of Strombidium sp. was the lowest in Table 2, and the maximum ingestion rate was lower than that of Strombidium sulcatum grazing on bacteria as observed by Rivier et al. (1985), implying that if ingestion rate increases linearly with increasing temperature, the maximum ingestion rates of Strombidium sulcatum could be larger from 15 to 25°C. Since Strombidium sp. had a higher growth rate when grazing on T-ISO than when grazing on bacteria only, bacteria appear not to be the main prey of Strombidium sp., especially at high prey concentrations, and were only an option when no better prey was available.
Prey Selectivity of Strombidium sp.
In Figure 4, the data points, where other two points representing negative ingestion rate were avoided in advance, showing the proportion of ingested carbon derived from bacteria versus the proportion of the carbon content in the environment (i.e., the culture vessel) represented by bacteria did not fall on the diagonal line of equivalency. This shows that the ciliate Strombidium sp. engages in prey selectivity.
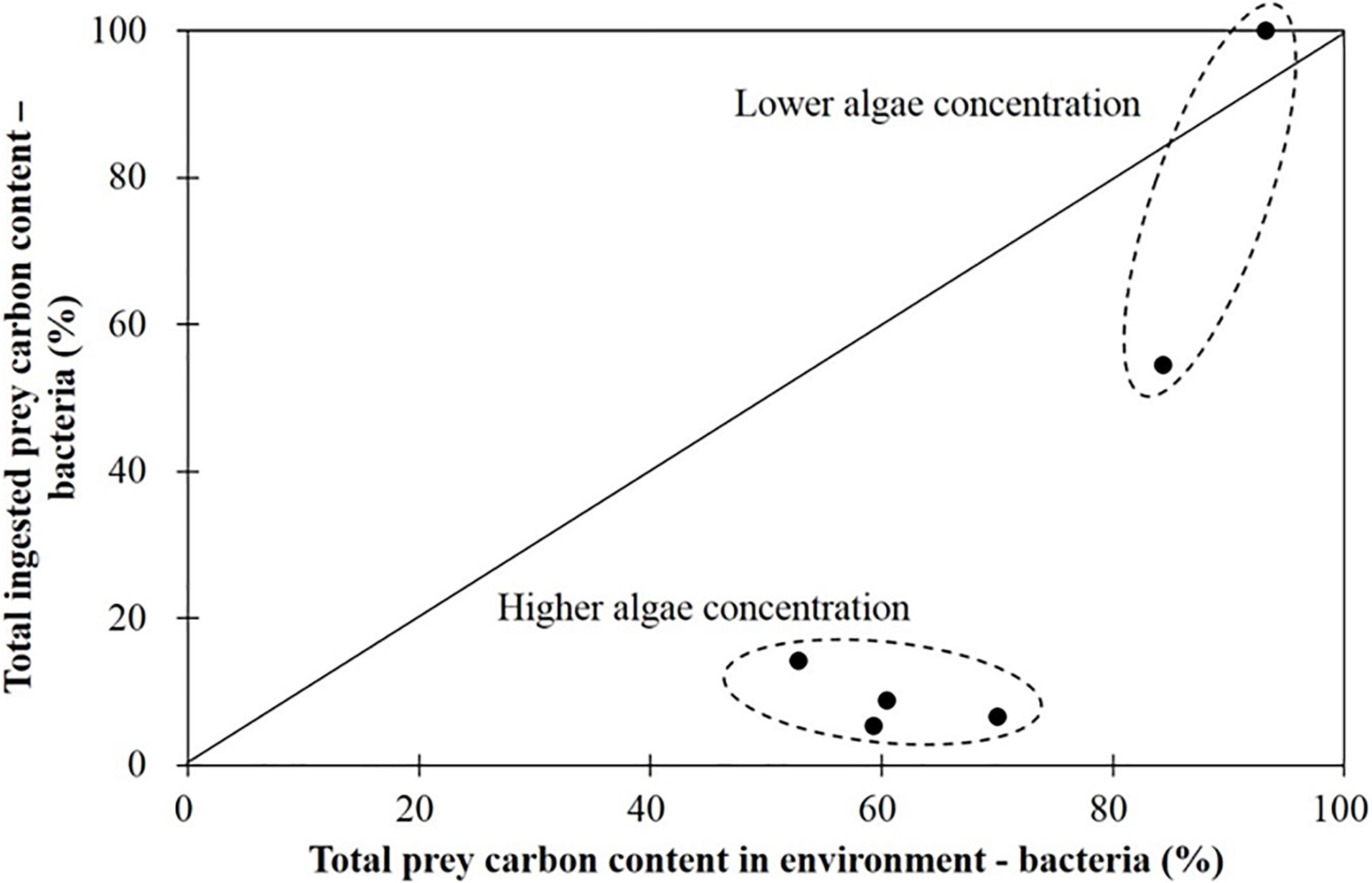
Figure 4. Percentage of total ingested prey carbon content of bacteria when grazing on the haptophyte Isochrysis galbana (in the presence of bacteria). The diagonal line means 1:1.
The bacteria in the culture medium accounted for 52–93% of the total prey carbon content in different treatments. Strombidium sp. mainly grazed on bacteria when the bacteria accounted for more than 80% of the total prey carbon content in the culture environment, a situation corresponding to a low relative algal concentration, and under those circumstances bacterial ingestion ranged from 60 to 100% of the total (Figure 4). In contrast, when bacteria constituted less than 80% of the total prey carbon content in the culture environment, a situation corresponding to a high relative algal concentration, the proportion of bacteria in the prey ingested by Strombidium sp. was less than 20% (Figure 4). Briefly stated, Strombidium sp. mainly grazed on bacteria at low relative algal concentrations, but on algae at high relative algal concentrations.
Gross Growth Efficiency (GGE) of Strombidium sp.
In early studies, ciliates have demonstrated a gross growth efficiency (GGE) of between 10 and 45%, with a mean of 30% (Straile, 1997). With the difficult of achieving a totally axenic algal culture and researchers’ inattention to the ability of oligotrich ciliates to graze on bacteria in previous studies, it is reasonable to speculate that GGEs at low relative algal concentrations have been substantially overestimated (as when bacteria accounted for more than 80% of the total prey carbon content in the present study), and also slight overestimated at high relative algal concentrations (as when bacteria accounted for less than 80% of the total prey carbon content in the present study), making the overestimation in commonly employed models of carbon flux.
Gross growth efficiencies of the choreotrich ciliate Strobilidium sp. grazing on Isochrysis galbana was found to be significantly different at different prey concentrations (Chen et al., 2010). At low relative algal concentrations it was inconstant, and unreasonable high (nearly 80%), but it was relatively stable (about 10%) at high relative algal concentrations. It is reasonable to assume that these authors did not control for the presence of bacteria in the algal culture medium (Figure 5). In the present study with grazing on both T-ISO and bacteria taken into account, GGE stayed in a fairly narrow range (6–15%) with no significant overestimates (Figure 5).
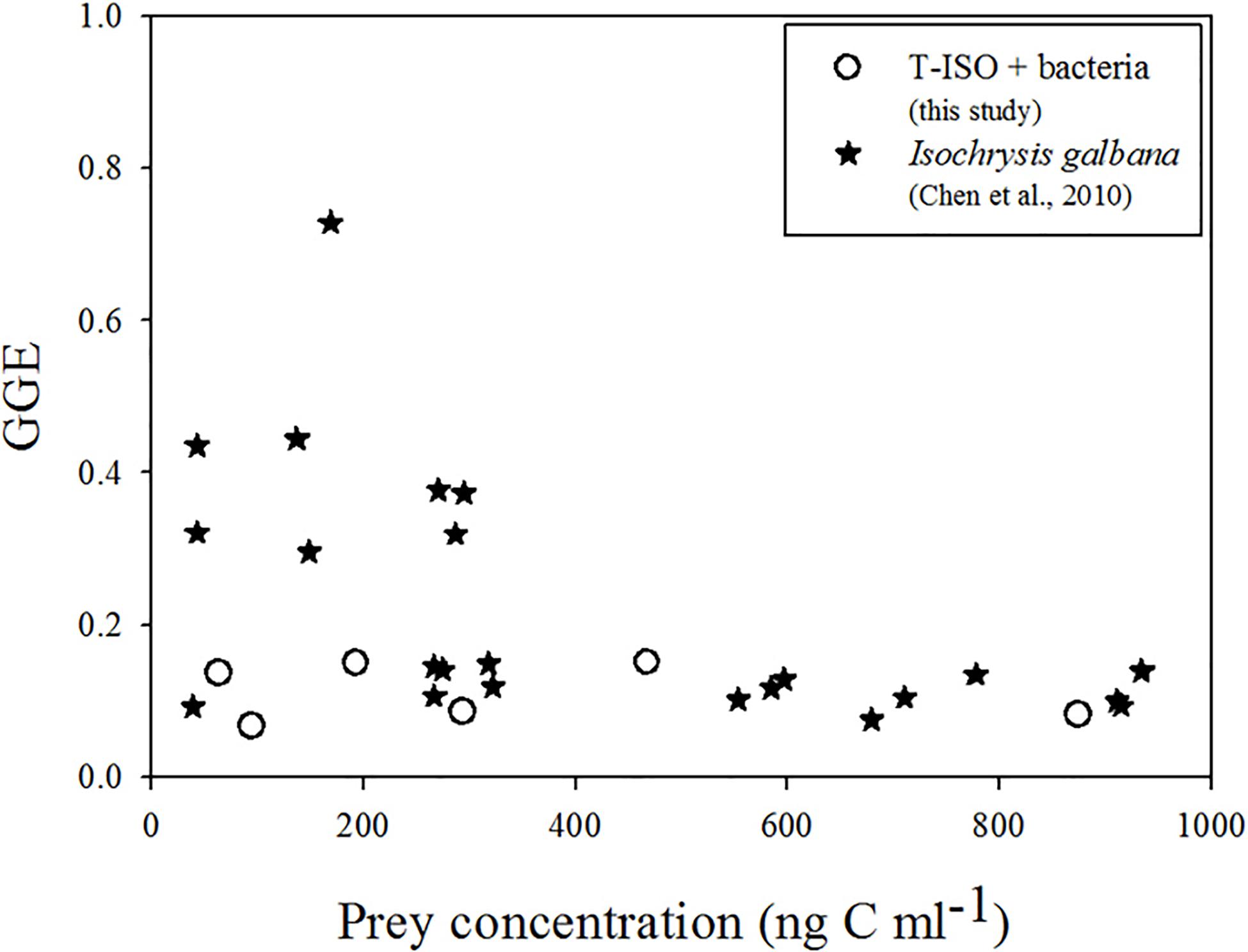
Figure 5. Gross growth efficiency (GGE) of Strombidium sp. grazing on T-ISO (in the presence of bacteria) and of Strobilidium sp. grazing on Isochrysis galbana (Chen et al., 2010).
Carbon Transfer by Grazing of Strombidium sp.
Lee and Fuhrman (1987) reported the coefficient of carbon content conversion of marine bacteria as approximately 20 fg C cell–1 and Kroer (1994) found that the carbon content of estuarine bacteria is 117 fg C cell–1. Bacteria show different coefficients of carbon content conversion in different environments, ranging from 7 to 149 fg C cell–1 (Bjørnsen, 1986; Fagerbakke et al., 1996; Trousselier et al., 1997; Theil-Nielsen and Sondergaard, 1998; Vrede et al., 2002). Bacteria with a carbon content of 149 fg C cell–1 were used in the present study.
Figure 6, in which a conversion coefficient of 20 fg C cell–1 for the bacteria was used, in accord with general practice in marine microbial ecology (Lee and Fuhrman, 1987), shows the same result as in Figure 4 (where bacterial carbon content 149 fg C cell–1). However, the gross growth efficiency (GGE) shown in Figure 7 with the bacterial carbon content set at 20 fg C cell–1 shows an unrealistically high GGE of about 95% at the lowest T-ISO concentration, indicating that the carbon content conversion of bacteria did not fit the value of GGE. In the present study, large bacteria were collected from coastal water, not small bacteria like those in the open ocean, and cultivated in a non-nutrient-limited environment. This makes as estimate of 149 fg C cell–1 for bacterial cell carbon content is more appropriate than one of 20 fg C cell–1.
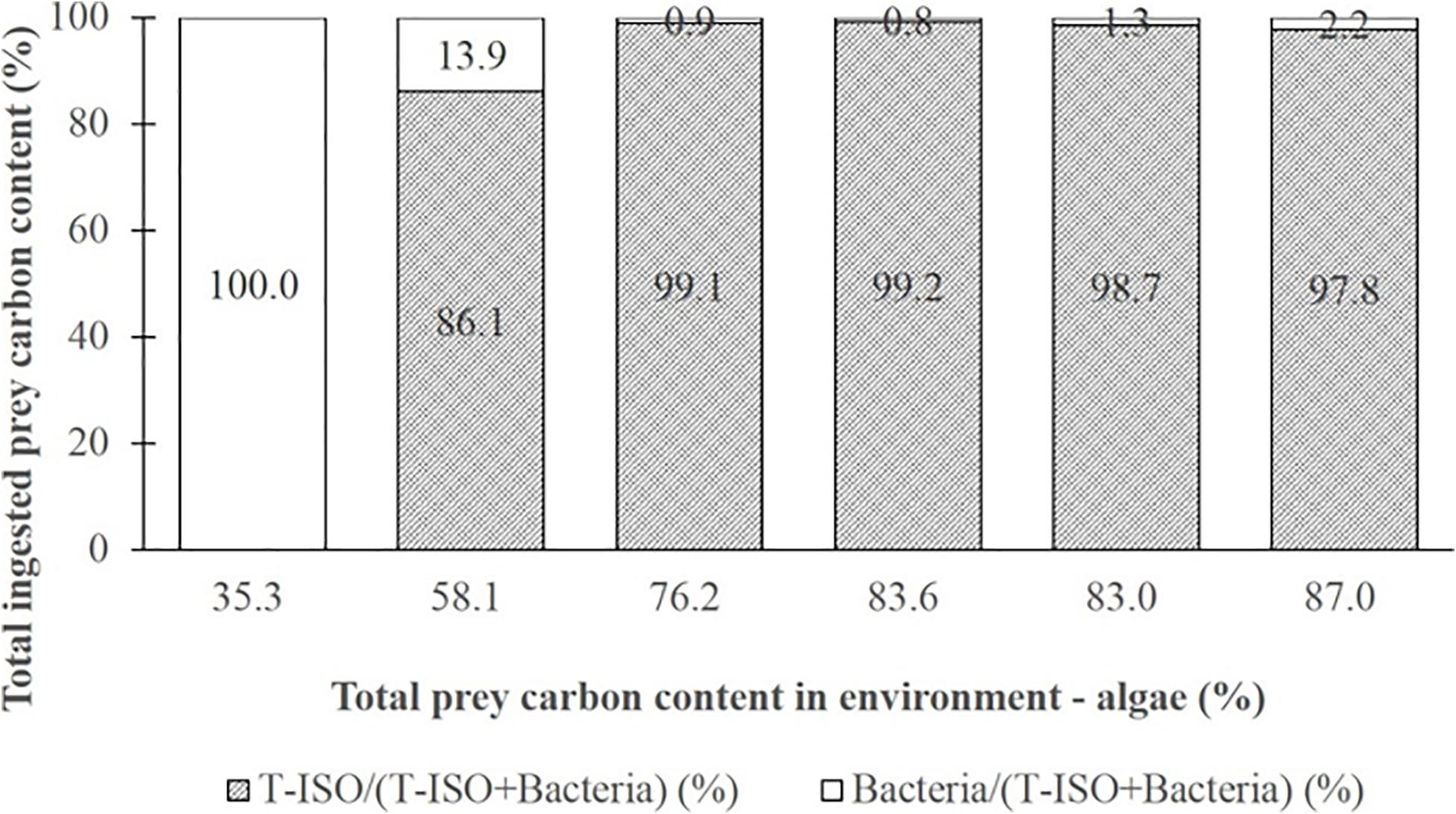
Figure 6. Percentage composition of total ingested prey carbon content for Strombidium sp. under the first culture condition (grazing on haptophyte Isochrysis galbana (T-ISO), in the presence of different proportions of bacteria) (calculated with a bacteria cell carbon content of 20 fg C cell–1).
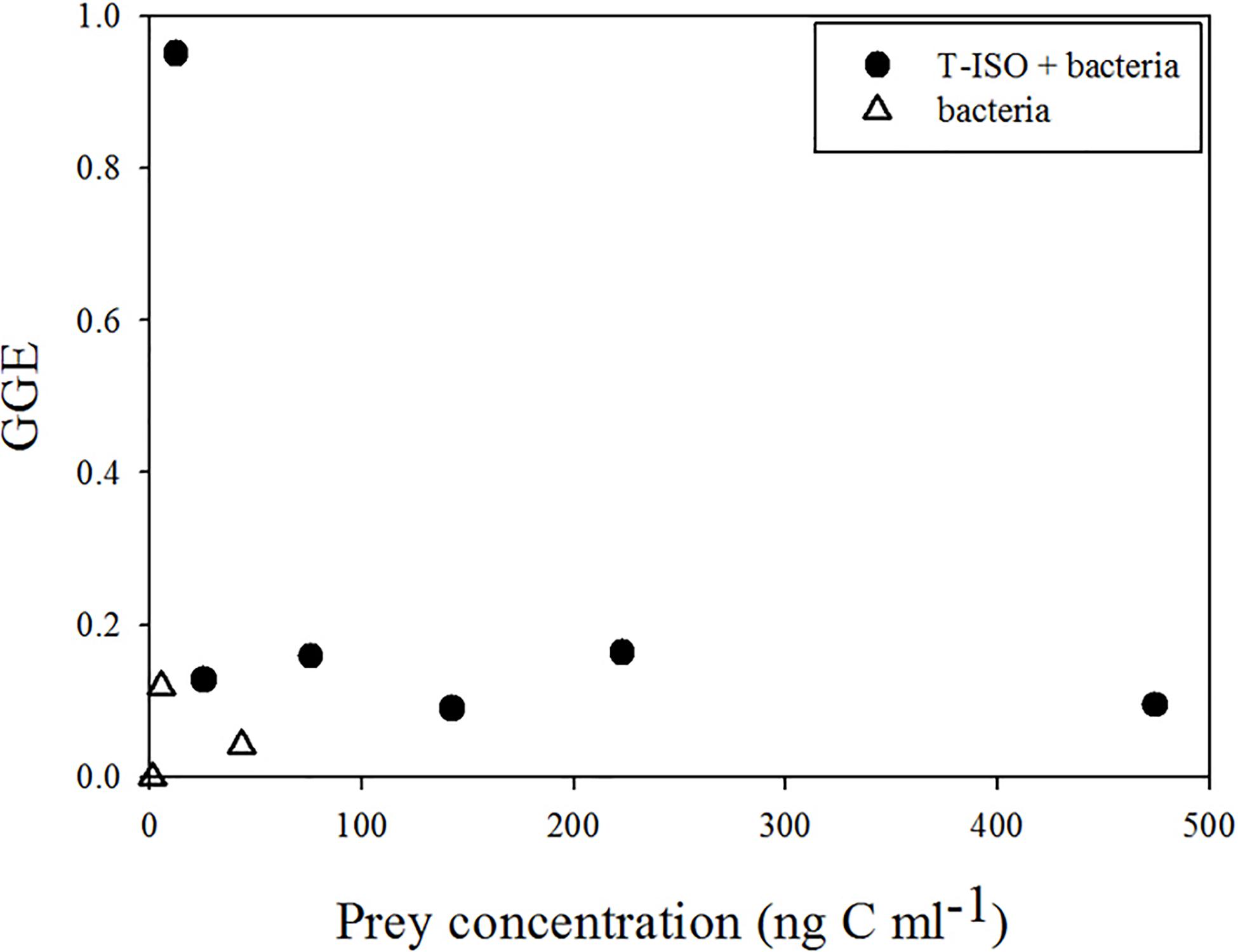
Figure 7. Gross growth efficiency (GGE) of Strombidium sp. under two culture conditions: (circle) grazing on the haptophyte Isochrysis galbana (T-ISO) in the presence of bacteria, (triangle) grazing on bacteria only (calculated with a bacteria cell carbon content of 20 fg C cell–1).
Previous studies have speculated that the concentration of bacteria in the natural environment is not enough to provide sufficient energy for ciliates even if the latter are capable of ingesting them (Fenchel, 1980; Rassoulzadegan and Etienne, 1981; Capriulo and Carpenter, 1983; Jonsson, 1986; Fenchel and Jonsson, 1988), and have therefore assumed that bacteria are not the main source of prey for ciliates (Kamiyama, 2015). However, bacterial concentrations in coastal waters and lakes are high enough to provide ciliates with sufficient energy for growth (Sherr et al., 1989). With the discovery that smaller ciliates (<20 μm; Sherr et al., 1986) and other ciliates do graze on bacteria (Borsheim, 1984; Gast, 1985; Rivier et al., 1985), it appears that bacteria do play an important role in carbon transformation, especially in coastal waters.
In the open ocean, the low biomass of bacteria may not be enough to support the growth of ciliates, whose dietary carbon mainly comes from grazing on nano-sized plankton. However, in the coastal zone and especially in culture, carbon obtained from grazing on bacteria should be taken into account. Lack of attention to this can have a significant impact on the results of quantitative studies of food webs and carbon flow.
Conclusion
The greatly random values of GGE, with some being remarkably high, at low relative algal concentrations observed by other researches can be explained in the present study by the prey selective behavior of Strombidium sp., which mainly grazed on bacteria at low relative algal concentrations, but on algae at high relative algal concentrations. The presence of bacteria in the algal culture medium affects the growth and grazing rates of ciliates grazing on nanoflagellates, resulting in an overestimate of gross growth efficiency at low relative algal concentrations.
Data Availability Statement
The raw data supporting the conclusions of this article will be made available by the authors, without undue reservation.
Author Contributions
S-FT and K-PC designed the research. W-LC carried out the experiment. All authors analyzed data. S-FT and W-LC wrote the manuscript.
Funding
This study was supported by grants from the Ministry of Science and Technology, Taiwan, ROC (106-2611-M-019-023, 107-2611-M-019-008, and 107-2611-M-019-002-MY3).
Conflict of Interest
The authors declare that the research was conducted in the absence of any commercial or financial relationships that could be construed as a potential conflict of interest.
Acknowledgments
Many thanks are given to Mark J. Grygier (Center of Excellence for the Oceans, National Taiwan Ocean University, Taiwan) for his constructive criticism and linguistic improvements.
References
Agatha, S. (2011). Global diversity of aloricate Oligotrichea (Protista, Ciliophora, Spirotricha) in marine and brackish sea water. PLoS One 6:e22466. doi: 10.1371/journal.pone.0022466
Allali, K., Dolan, J., and Rassoulzadegan, F. (1994). Culture characteristics and orthophosphate excretion of a marine oligotrich ciliate, Strombidium sulcatum, fed heat-killed bacteria. Mar. Ecol. Prog. Ser. 105, 159–165. doi: 10.3354/meps105159
Atkinson, D., Ciotti, B. J., and Montagnes, D. J. S. (2003). Protists decrease in size linearly with temperature: ca. 2.5% oC-1. Proc. R. Soc. B Biol. Sci. 270, 2605–2611. doi: 10.1098/rspb.2003.2538
Azam, F., Fenchel, T., Field, J. G., Gray, J. S., Meyer-Reil, L., and Thingstad, F. (1983). The ecological role of water-column microbes in the sea. Mar. Ecol. Prog. Ser. 10, 257–263. doi: 10.3354/meps010257
Bernard, C., and Rassoulzadegan, F. (1990). Bacteria or microflagellates as a major food source for marine ciliates: possible implications for the microzooplankton. Mar. Ecol. Prog. Ser. 64, 147–155. doi: 10.3354/meps064147
Bjørnsen, P. K. (1986). Automatic determination of bacterioplankton biomass by image analysis. Appl. Environ. Microbiol. 51, 1199–1204. doi: 10.1128/AEM.51.6.1199-1204.1986
Borsheim, K. Y. (1984). Clearance rates of bacteria-sized particles by freshwater ciliates, measured with monodisperse fluorescent latex beads. Oecologia 63, 286–288. doi: 10.1007/bf00379891
Calbet, A., and Saiz, E. (2005). The ciliate-copepod link in marine ecosystems. Aquat. Microb. Ecol. 38, 157–167. doi: 10.3354/ame038157
Capriulo, G. M., and Carpenter, E. J. (1983). Abundance, species composition and feeding impact of tintinnid micro-zooplankton in central Long Island Sound. Mar. Ecol. Prog. Ser. 10, 277–288. doi: 10.3354/meps010277
Chen, B. Z., Liu, H. B., and Lau, M. T. S. (2010). Grazing and growth responses of a marine oligotrichous ciliate fed with two nanoplankton: does food quality matter for micrograzers? Aquat. Ecol. 44, 113–119. doi: 10.1007/s10452-009-9264-5
Christaki, U., Dolan, J. R., Pelegri, S., and Rassoulzadegan, F. (1998). Consumption of picoplankton-size particles by marine ciliates: effects of physiological state of the ciliate and particle quality. Limnol. Oceanogr. 43, 458–464. doi: 10.4319/lo.1998.43.3.0458
Dolan, J. R., and Šimek, K. (1997). Processing of ingested matter in Strombidium sulcatum, a marine ciliate (Oligotrichida). Limnol. Oceanogr. 42, 393–397. doi: 10.4319/lo.1997.42.2.0393
Fagerbakke, K. M., Heldal, M., and Norland, S. (1996). Content of carbon, nitrogen, oxygen, sulfur and phosphorus in native aquatic and cultured bacteria. Aquat. Microb. Ecol. 10, 15–27. doi: 10.3354/ame010015
Fenchel, T. (1980). Relation between particle size selection and clearance in suspension-feeding ciliates. Limnol. Oceanogr. 25, 733–738. doi: 10.4319/lo.1980.25.4.0733
Fenchel, T., and Jonsson, P. R. (1988). The functional biology of Strombidium sulcatum, a marine oligotrich ciliate (Ciliophora, Oligotrichina). Mar. Ecol. Prog. Ser. 48, 1–15. doi: 10.3354/meps048001
Frost, B. (1972). Effects of size and concentration of food particles on the feeding behavior of the marine planktonic copepod Calanus pacificus. Limnol. Oceanogr. 17, 805–815. doi: 10.4319/lo.1972.17.6.0805
Fuhrman, J. A., Hewson, I., Schwalbach, M. S., Steele, J. A., Brown, M. V., and Naeem, S. (2006). Annually reoccurring bacterial communities are predictable from ocean conditions. PNAS 103, 13104–13109. doi: 10.1073/pnas.0602399103
Gast, V. (1985). Bacteria as a food source for microzooplankton in the Schlei Fjord and Baltic Sea with special reference to ciliates. Mar. Ecol. Prog. Ser. 22, 107–120. doi: 10.3354/meps022107
Gifford, D. J. (1991). The protozoan-metazoan trophic link in pelagic ecosystems. J. Protozool. 38, 81–86. doi: 10.1111/j.1550-7408.1991.tb04806.x
Gismervik, I. (2005). Numerical and functional responses of choreo- and oligotrich planktonic ciliates. Aquat. Microb. Ecol. 40, 163–173. doi: 10.3354/ame040163
Guillard, R. R. L., and Ryther, J. H. (1962). Studies of marine planktonic diatoms: I. Cyclotella nana Hustedt, and Detonula confervacea (Cleve). Gran. Can. J. Microbiol. 8, 229–239. doi: 10.1139/m62-029
Hagenbuch, I. M., and Pinckney, J. L. (2012). Toxic effect of the combined antibiotics ciprofloxacin, lincomycin, and tylosin on two species of marine diatoms. Water Res. 46, 5028–5036. doi: 10.1016/j.watres.2012.06.040
Hansen, B., Bjørnsen, P. K., and Hansen, P. J. (1994). The size ratio between planktonic predators and their prey. Limnol. Oceanogr. 39, 395–403. doi: 10.4319/lo.1994.39.2.0395
Heinbokel, J. F. (1978). Studies on the functional role of tintinnids in the Southern California Bight. I. Grazing and growth rates in laboratory cultures. Mar. Biol. 47, 177–189. doi: 10.1007/BF00395638
Jiang, Y., Xu, H., Al-Rasheid, K. A. S., Warren, A., Hu, X., and Song, W. (2011). Planktonic ciliate communities in a semi-enclosed bay of Yellow Sea, northern China: annual cycle. J. Mar. Biol. Assoc. 91, 97–105. doi: 10.1017/S002531541000175X
Jonsson, P. R. (1986). Particle size selection, feeding rates and growth dynamics of marine planktonic oligotrichous ciliates (Ciliophora: Oligotrichina). Mar. Ecol. Prog. Ser. 33, 265–277. doi: 10.3354/meps033265
Kamiyama, T. (2015). “Planktonic ciliates: diverse ecological function in seawater,” in Marine Protists: Diversity and Dynamics, eds S. Ohtsuka, T. Suzaki, T. Horiguchi, N. Suzuki, and F. Not, (Tokyo: Springer), 277-309. doi: 10.1007/978-4-431-55130-0
Kimmance, S. A., Atkinson, D., and Montagnes, D. J. S. (2006). Do temperature-food interactions matter? Responses of production and its components in the model heterotrophic flagellate Oxyrrhis marina. Aquat. Microb. Ecol. 42, 63–73. doi: 10.3354/ame042063
Kroer, N. (1994). Relationships between biovolume and carbon and nitrogen content of bacterioplankton. FEMS Microbiol. Ecol. 13, 217–223. doi: 10.1111/j.1574-6941.1994.tb00068.x
Lee, S., and Fuhrman, J. A. (1987). Relationships between biovolume and biomass of naturally derived marine bacterioplankton. Appl. Environ. Microbiol. 53, 1298–1303. doi: 10.1128/AEM.53.6.1298-1303.1987
Liu, H. B., Dagg, M. J., Wu, C.-J., and Chiang, K.-P. (2005). Mesozooplankton consumption of microplankton in the Mississippi River plume, with special emphasis on planktonic ciliates. Mar. Ecol. Prog. Ser. 286, 133–144. doi: 10.3354/meps286133
Löder, M. G. J., Meunier, C., Wiltshire, K. H., Boersma, M., and Aberle, N. (2011). The role of ciliates, heterotrophic dinoflagellates and copepods in structuring spring plankton communities at Helgoland Roads, North Sea. Mar. Biol. 158, 1551–1580. doi: 10.1007/s00227-011-1670-2
Montagnes, D. J. S. (1996). Growth responses of planktonic ciliates in the genera Strobilidium and Strombidium. Mar. Ecol. Prog. Ser. 130, 241–254. doi: 10.3354/meps130241
Montagnes, D. J. S., Kimmance, S. A., and Atkinson, D. (2003). Using Q10: can growth rates increase linearly with temperature? Aquat. Microb. Ecol. 32, 307–313. doi: 10.3354/ame032307
Montagnes, D. J. S., Lowe, C. D., Martin, L., Watts, P. C., Downes-Tettmar, N., Yang, Z., et al. (2011). Oxyrrhis marina growth, sex and reproduction. J. Plankton Res. 33, 615–627. doi: 10.1093/plankt/fbq111
Müller, H., and Geller, W. (1993). Maximum growth rates of aquatic ciliated protozoa: the dependence on body size and temperature reconsidered. Arch. Hydrobiol. 126, 315–327.
Paasche, E. (1973). Silicon and the ecology of marine plankton diatoms. II. Silicate-uptake kinetics in five diatom species. Mar. Biol. 19, 262–269. doi: 10.1007/bf02097147
Perez, M. T., Dolan, J. R., and Fukai, E. (1997). Planktonic oligotrich ciliates in the NW Mediterranean: growth rates and consumption by copepods. Mar. Ecol. Prog. Ser. 155, 89–101. doi: 10.3354/meps155089
Pierce, R. W., and Turner, J. T. (1992). Ecology of planktonic ciliates in marine food webs. Rev. Aquat. Sci. 6, 139–181.
Putt, M., and Stoecker, D. K. (1989). An experimentally determined carbon: volume ratio for marine “oligotrichous” ciliates from estuarine and coastal waters. Limnol. Oceanogr. 34, 1097–1103. doi: 10.4319/lo.1989.34.6.1097
Rassoulzadegan, F., and Etienne, M. (1981). Grazing rate of the tintinnid Stenosemella ventricosa (Clap. & Lachm.) Jorg. on the spectrum of the naturally occurring particulate matter from a Mediterranean neritic area. Limnol. Oceanogr. 26, 258–270. doi: 10.4319/lo.1981.26.2.0258
Rivier, A., Brownlee, D. C., Sheldon, R. W., and Rassoulzadegan, F. (1985). Growth of microzooplankton: a comparative study of bactivorous zooflagellates and ciliates. Mar. Microb. Food Webs 1, 51–60.
Sherr, E. B., Rassoulzadegan, F., and Sherr, B. F. (1989). Bacterivory by pelagic choreotrichous ciliates in coastal waters of the NW Mediterranean Sea. Mar. Ecol. Prog. Ser. 55, 235–240. doi: 10.3354/meps055235
Sherr, E. B., Sherr, B. F., Fallon, R. D., and Newell, S. Y. (1986). Small, aloricate ciliates as a major component of the marine heterotrophic nanoplankton. Limnol. Oceanogr. 31, 177–183. doi: 10.4319/lo.1986.31.1.0177
Straile, D. (1997). Gross growth efficiencies of protozoan and metazoan zooplankton and their dependence on food concentration, predator-prey weight ratio, and taxonomic group. Limnol. Oceanogr. 42, 1375–1385. doi: 10.4319/lo.1997.42.6.1375
Theil-Nielsen, J., and Sondergaard, M. (1998). Bacterial carbon biomass calculated from biovolumes. Arch. Hydrobiol. 141, 195–207. doi: 10.1127/archiv-hydrobiol/141/1998/195
Thurman, J., Parry, J. D., Hill, P. J., and Laybourn-Parry, J. (2010). The filter-feeding ciliates Colpidium striatum and Tetrahymena pyriformis display selective feeding behaviours in the presence of mixed, equally-sized, bacterial prey. Protist 161, 577–588. doi: 10.1016/j.protis.2010.04.001
Trousselier, M., Bouvy, M., Courties, C., and Dupuy, C. (1997). Variation of carbon content among bacterial species under starvation condition. Aquat. Microb. Ecol. 13, 113–119. doi: 10.3354/ame013113
Turner, G., and Lloyd, D. (1971). The effect of chloramphenicol on growth and mitochondrial function of the ciliate protozoon Tetrahymena pyriformis strain ST. Microbiology 67, 175–188. doi: 10.1099/00221287-67-2-175
Verity, P. G. (1985). Grazing, respiration, excretion and growth rates of tintinnids. Limnol. Oceanogr. 30, 1268–1282. doi: 10.4319/lo.1985.30.6.1268
Vrede, K., Heldal, M., Norland, S., and Bratbak, G. (2002). Elemental composition (C, N, P) and cell volume of exponentially growing and nutrient-limited bacterioplankton. Appl. Environ. Microbiol. 68, 2965–2971. doi: 10.1128/AEM.68.6.2965-2971.2002
Wickham, S. A., Steinmair, U., and Kamennaya, N. (2011). Ciliate distributions and forcing factors in the Amundsen and Bellingshausen Seas (Antarctic). Aquat. Microb. Ecol. 62, 215–230. doi: 10.3354/ame01468
Xu, K., Choi, J. K., Lei, Y., and Yang, E. J. (2011). Marine ciliate community in relation to eutrophication of coastal waters in the Yellow Sea. Chin. J. Oceanol. Limnol. 29, 118–127. doi: 10.1007/s00343-011-9106-x
Keywords: axenic algal culture, carbon flux, functional response, grazing pressure, growth efficiency, numerical response, prey selectivity
Citation: Chen W-L, Chiang K-P and Tsai S-F (2020) Neglect of Presence of Bacteria Leads to Inaccurate Growth Parameters of the Oligotrich Ciliate Strombidium sp. During Grazing Experiments on Nanoflagellates. Front. Mar. Sci. 7:569309. doi: 10.3389/fmars.2020.569309
Received: 03 June 2020; Accepted: 04 September 2020;
Published: 24 September 2020.
Edited by:
Stelios Katsanevakis, University of the Aegean, GreeceReviewed by:
Tian Xiao, Institute of Oceanology (CAS), ChinaKrzysztof Rychert, Pomeranian University of Slupsk, Poland
Copyright © 2020 Chen, Chiang and Tsai. This is an open-access article distributed under the terms of the Creative Commons Attribution License (CC BY). The use, distribution or reproduction in other forums is permitted, provided the original author(s) and the copyright owner(s) are credited and that the original publication in this journal is cited, in accordance with accepted academic practice. No use, distribution or reproduction is permitted which does not comply with these terms.
*Correspondence: Sheng-Fang Tsai, stsai@mail.ntou.edu.tw