- 1Instituto de Estudos Costeiros, Universidade Federal do Pará, Bragança, Brazil
- 2Núcleo de Ecologia Aquática e Pesca da Amazônia, Universidade Federal do Pará, Belém, Brazil
- 3ICHTUS Soluções em Meio Ambiente, Salvador, Brazil
- 4Instituto Bicho D’água, Conservação Socioambiental, Belém, Brazil
Trawling is a controversial fishing method due to the perceived lack of selectivity of the net and the resulting capture of a large quantity and diversity of non-target species. Here, we used DNA barcode methods to identify the composition of the bycatch produced by the trawl fishery of the Brazilian North coast. A total of 182 species belonging to 18 orders and 62 families were captured, including 17 species under some degree of threat in the wild according to the IUCN Red List of Threatened Species (IUCN). These results highlight the impact on the marine biodiversity of northern Brazil caused by the bycatch of small-scale industrial and unregulated fishery operations, and support the application of DNA-based methods for the identification of the bycatch species taken by data-poor fisheries, as a powerful tool for the improvement of the quality of fishery catch statistics and more precise bycatch records.
Introduction
Fisheries that trawl for shrimp in tropical regions take an extremely diverse bycatch fauna, but generally provide few historical or biological data for the quantitative assessment of stocks. The constant mortality of bycatch species caused by the fishery industry has a serious impact on the ecosystem and on the ongoing decline of the populations of many marine vertebrate species (Lewison et al., 2004), a pressure that threatens the stability of fish stocks through the overexploitation of many species (Pauly et al., 1998, 2002; Harrington et al., 2006; Worm et al., 2006). Bycatch commonly consists of (a) non-target species that are kept to be eaten or sold, and (b) discards, which are a subset of organisms that represent a wasted fishery resource, and thus attract significant public awareness, especially when including endangered, threatened, or protected species (Gray and Kennelly, 2018). Many groups of species are highly susceptible to bottom trawl fisheries, in particular, commercial shrimping, which unavoidably harvest bottom-dwelling species, such as elasmobranchs and catfishes. Many of these species are endangered or vulnerable, such as the Largetooth Sawfish Pristis pristis, the Long-tail stingray Hypanus longus, and the Gillbacker sea catfish Sciades parkeri (Chee, 1996; Willems et al., 2016; Schmid and Giarrizzo, 2017).
The poor management of non-target stocks compromises efforts to guarantee sustainability, and may result in a substantial, undocumented removal of biomass. On the northern coast of Brazil, industrial shrimp trawling fleets operate over the continental shelf, leading to the bycatch of a range of fish species from the region’s marine fauna, which is still poorly documented (Marceniuk et al., 2019). The recent discovery of a reef system off the mouth of the Amazon River has greatly increased our understanding of the region’s marine biodiversity (Moura et al., 2016). The discovery of the occurrence of 73 species of the Elasmobranchii and Actinopterygii in this region reinforces that idea that it does not function simply as a migratory corridor between the Brazilian and Caribbean geographic provinces, but rather that it provides a subsistence habitat for many of these fish, which highlights the functional importance of this Amazonian reef system (Moura et al., 2016). Although a recent study (Marceniuk et al., 2019) provided the first checklist of the bony fish caught by the industrial shrimp trawling operations off the northern coast of Brazil, many of the species (over 15% of the total diversity) were identified through taxonomic keys and photographs taken by onboard observers with no specimens being collected or deposited in museum collections. These authors also overlooked the impact of the trawling operations on the Chondrichthyes, a group of fish that of threatened shark and ray species that are marketed unregulated in Brazil.
Fishery management can be hampered by a lack of reliable species identification (Bornatowski et al., 2014). The traditional morphological approach to species identification has recently been reinforced by the inclusion of DNA-based approaches, such as DNA barcoding. This technique is based on the diversity of the ∼650 bp region of the mitochondrial Cytochrome C Oxidase I (COI) gene, which has been used widely to improve the accuracy of fish species identification (Hebert et al., 2003b; Ratnasingham and Hebert, 2007). The DNA barcoding approach has progressed rapidly, and is now widely used, due primarily to its low cost, combined with the need to address critical conservation issues and fishery management questions. In the present study, a DNA barcoding library was compiled in order to investigate the composition of fish species caught as bycatch by the shrimp trawling fleet operating off the North Coast of Brazil, in order to provide a comprehensive update of the region’s fish fauna (Chondrichthyes and Teleostei), with comments on the conservation of the species and the unrecognized diversity. The bycatch species were identified at both morphological and molecular levels, generating new data on the fish biodiversity of the study region.
Materials and Methods
General Characteristics of the Study Area
The northern coast of Brazil encompasses the region between the Oiapoque River estuary (4°16 ″N), in Amapá state, and the Parnaíba River Delta (3°S), in Maranhão state (Figure 1; Isaac and Barthem, 1995; Ekau and Knoppers, 1999). This region has an irregular coastline, being mostly straight in Amapá, but highly indented in Pará and Maranhão, due to the presence of numerous estuaries, interspersed with tidal plains and the largest continuous tract of mangroves on the planet, with a total area of approximately 8,900 km2 (Lara and Dittmar, 1999). This region is dominated by the Amazon and Orinoco rivers (Briggs, 1974; Floeter et al., 2008; Briggs and Bowen, 2012), whose total mean annual freshwater discharge into the Atlantic Ocean is 120 × 103 m3/s to 300 × 103 m3/s (Ward et al., 2015). This combined discharge results in a plume of low salinity water with a high sediment concentration at the surface, and a muddy-bottomed continental platform stretching approximately 2,500 km of the coast off northwestern South America (Collette and Rützler, 1977; Meade et al., 1985), constituting one of the largest freshwater barrier zones in the Western Atlantic, which is known to form a biogeographic barrier between the coral-dwelling fish faunas of the Caribbean and Brazilian provinces (Briggs, 1995; Floeter and Gasparini, 2000; Rocha, 2003).
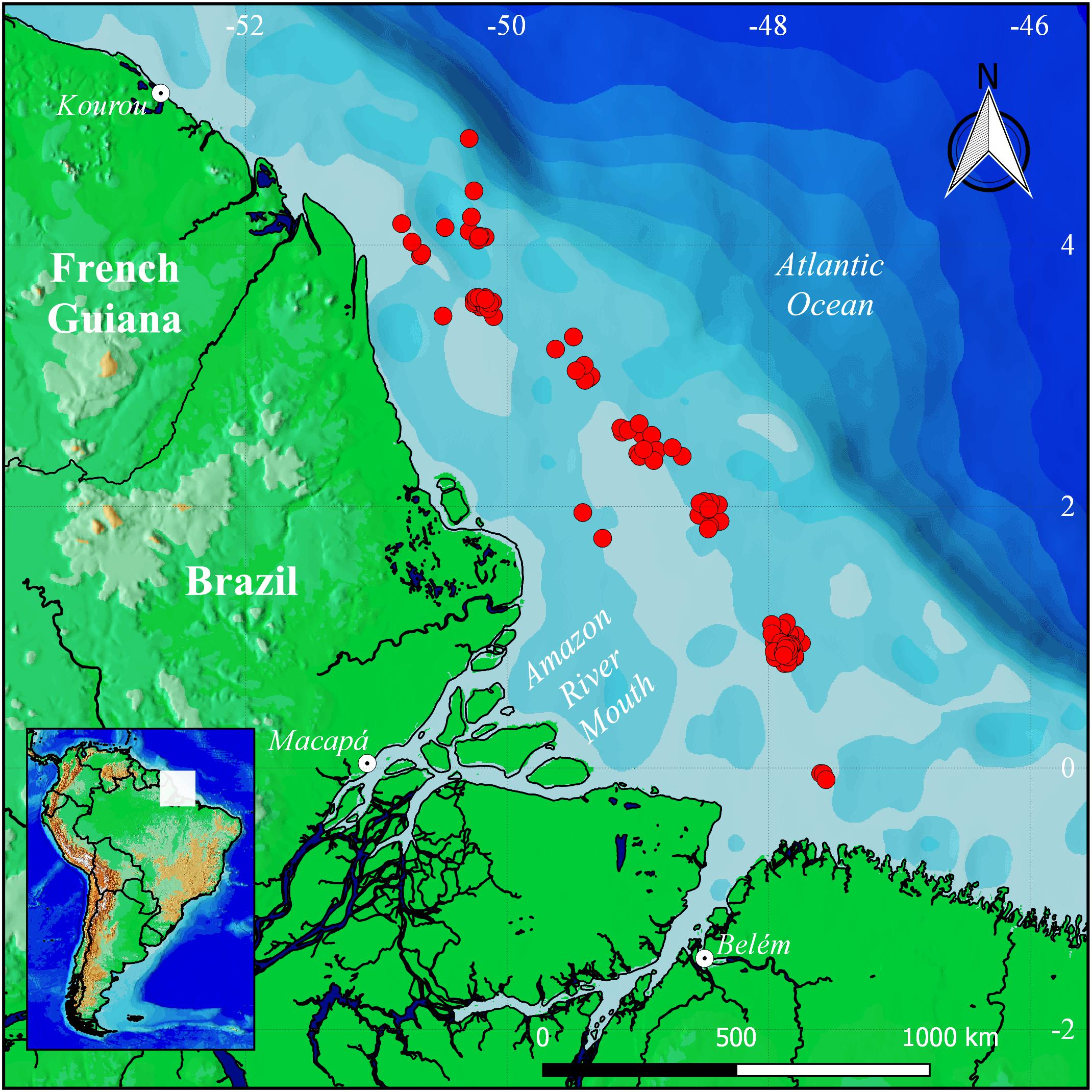
Figure 1. The northern coast of Brazil, showing the location of the sampling sites at which the fish specimens were obtained from the bycatch of shrimp trawling. Map created using QGIS 3.4.0 (Geographic Information System. Open Source Geospatial Foundation) software (https://qgis.org/en/site/).
Sampling and Morphological Identification
The bycatch specimens were collected during the shrimp trawling operations by onboard technicians of the National Center for the Research and Conservation of the Marine Biodiversity of the North Coast of Brazil (CEPNOR), based in Belém, Pará. This monitoring operation accompanied 229 trawls between July 2015 and May 2017 during bimonthly excursions with a mean duration of 15 days. Were used vessels of the industrial shrimping fleet operating on the North Brazilian Coast, between Marajó Bay (08° S, 47.85° W) and the mouth of the Oiapoque River (4.7° N, 51.17°W), at an approximate mean distance of 80 km from the coast, at depths of 40–80 m. This fleet uses vessel-shaped outboard bottom trawl nets, with a 30 mm × 21 mm mesh size (Figure 1). Specimen collection was authorized by ICMBIO/MMA (permit 69486-1), a branch of the Brazilian Environment Ministry (MMA).
A random subsample of ca. 90 kg of the catch of each monitored trawl was frozen at −18°C and sent to the fish collection of the Aquatic Ecology Group (GEA), at the Federal University of Pará in Belém, Brazil. In the laboratory, the fish species were identified to the lowest possible taxonomic level based on Figueiredo and Menezes (1978, 1980a, 1980b), Carvalho-Filho (1999), and Carpenter et al. (2002a,b), with the scientific names, authority, and year of description following Fricke et al. (2020). The common names used by crew members to refer to the fish were not considered, as they could bias the identification. A small fragment of muscle tissue was taken from between one and eight specimens per species and stored in 96% ethanol for the DNA barcode analysis. The voucher specimens were fixed in a 10% formalin solution in the field, and then stored in a 70% ethanol solution in the laboratory, where they were deposited in the fish collection of the GEA (the GEA catalog is available in Supplementary Table S3). All the material is available to interested researchers on request.
DNA Extraction, Amplification, and Sequencing of the Genomic Regions
We extracted DNA from the muscle tissue or other parts of the fish using the Wizard Genomic Purification DNA kit (Promega Corporation, Madison, WI, United States), following the instructions provided by the manufacturer. All tissue samples were stored in 95% ethyl alcohol at −20°C and deposited in the fish collection of the GEA at the Federal University of Pará, in Belém (Supplementary Table S1). Approximately 650 base pairs (bps) of the COI gene, which was used as the DNA barcode, was amplified via Polymerase Chain Reaction (PCR), using the following primers, described by Ward et al. (2005): FishF1 5′-TCAACCAACCACAAAGACATTGGCAC-3′ and FishR1 5′-TAGACTTCTGGGTGGCCAAAGAATCA-3′. The samples were amplified in a final volume of 25 μL, containing 4 μL of dNTP (Deoxyribonucleotide Triphosphate; 1.25 mM), 2.5 μL of 10× buffer solution, 1 μL of MgCl2 (25 mM), 0.25 μL of each primer (200 ng/μL), 1–1.5 μL of genomic DNA (100 ng/μL), 1 U of Taq DNA polymerase (5 U/μL), and purified water to complete the final reaction volume. The amplification conditions were: initial denaturing at 95°C for 5 min; 35 cycles of denaturing at 94°C for 40 s, annealing at 55°C for 35 s, and extension at 72°C for 1 min, with a final extension step at 72°C for 5 min. The PCR products were purified using the ExoSAP-IT enzyme and were sequenced in an ABI 3500 automatic sequencer using the Big-Dye Terminator Cycle Sequencing Kit (Applied Biosystems).
DNA-Based Species Identification
As described above, morphological identification was conducted based on specific literature. In addition, we used a DNA-based species identification methods were employed for the taxonomic identification of the specimens. The sequences were aligned automatically using the ClustalW software (Thompson et al., 1994), implemented in Bioedit 7.0.9 (Hall, 1999). Following the morphological identification, the sequences of each specimen were compared with those of fish species obtained from the GenBank1 and BOLD2 databases.
The Barcode of Life Data Systems (BOLDSystems) tool (Ratnasingham and Hebert, 2007) was used to assign existing Barcode Index Numbers (BINs) to all the sequences of the specimens analyzed in the present study. The sequence files, institutional data, taxonomic information, images, and collection numbers were all submitted to this platform (see Supplementary Material). This permitted the calculation of the number of clusters, to determine the number of BINs, the levels of divergence within and between species, genera, and families, the barcode gap, and to construct a Neighbor-Joining (NJ) tree based on the Kimura 2-parameter (K2P) approach (Kimura, 1980), using 1,000 bootstrap pseudoreplicates. This analysis was run in the BOLD Workbench application (version 3.6).
In addition, phylogenetic relationships were analyzed using Bayesian Inference and the Maximum Likelihood approach. The BI approach was run in MrBayes 3.1.2 (Huelsenbeck and Ronquist, 2001), while the ML analyses were performed in RAxML 7.2.7 (Stamatakis, 2006). A priori, the best nucleotide substitution model was selected by jModeltest (Darriba et al., 2012). The BI approach had two independent runs, four chains, and 10 million generations, for which one tree was archived every 100 generations, with the first 25% of the trees being discarded as burn-in. The performance of the runs was evaluated using Tracer 1.5 (Rambaut and Drummond, 2009), and the a posteriori probability was calculated as the percentage of samples recovered in a clade (≥95% significant support; Huelsenbeck and Ronquist, 2001). The ML analysis was run using the GTRGAMMA model, and the confidence of the branches of the best tree was analyzed in greater depth, based on 1,000 bootstrap replicas.
Species Delimitation Analysis
The DNA barcoding analysis was complemented by performing species-delimitation procedures based on a single locus. These methods have been useful in resolving relationships among groups of species that have cryptic taxa and hence, taxonomic uncertainty (Conte-Grand et al., 2017; Araújo et al., 2019). We selected three widely used methods to delimit species based on a single locus: the Generalized Mixed Yule Coalescent (GMYC) (Pons et al., 2006), AGBD (Automatic Barcode Gap Discovery) (Puillandre et al., 2012), and BPTP (Bayesian Poisson Tree Processes) methods (Zhang et al., 2013).
For the GMYC approach, we used the topology inferred by MrBayes for single-locus species delimitation. The analyses were performed using the Splits package (Ezard et al., 2009) in R 3.5.2 (R Core Team, 2018), and by using a single temporal threshold. The bPTP was run on the webserver3 and was based on the best unrooted ML tree identified by RaxML as the input, over 500,000 generations (thinning = 500) and with other default parameters. The ABGD analysis was run online at the http://wwwabi.snv.jussieu.fr/public/abgd/abgdweb.html interface, using the.mega format matrix of the pairwise K2P distances between the Sparisoma specimens. This analysis had a relative gap width of 1.5 and interspecific divergence ranging from 0.001 to 0.1.
Results
Composition and Geographical Ranges
A total of 182 fish species were recognized, representing two subclasses, 26 taxonomic orders, 62 families, and 123 genera (Table 1). These species included 20 elasmobranchs and 162 teleosts. The most diverse fish families represented in the bycatch were Sciaenidae (21 species), Carangidae (14 species), Haemulidae (10 species), Ariidae (8 species), and Tetradontidae (7 species), while none of the other families were represented by more than six species (Table 1 and Figure 2).
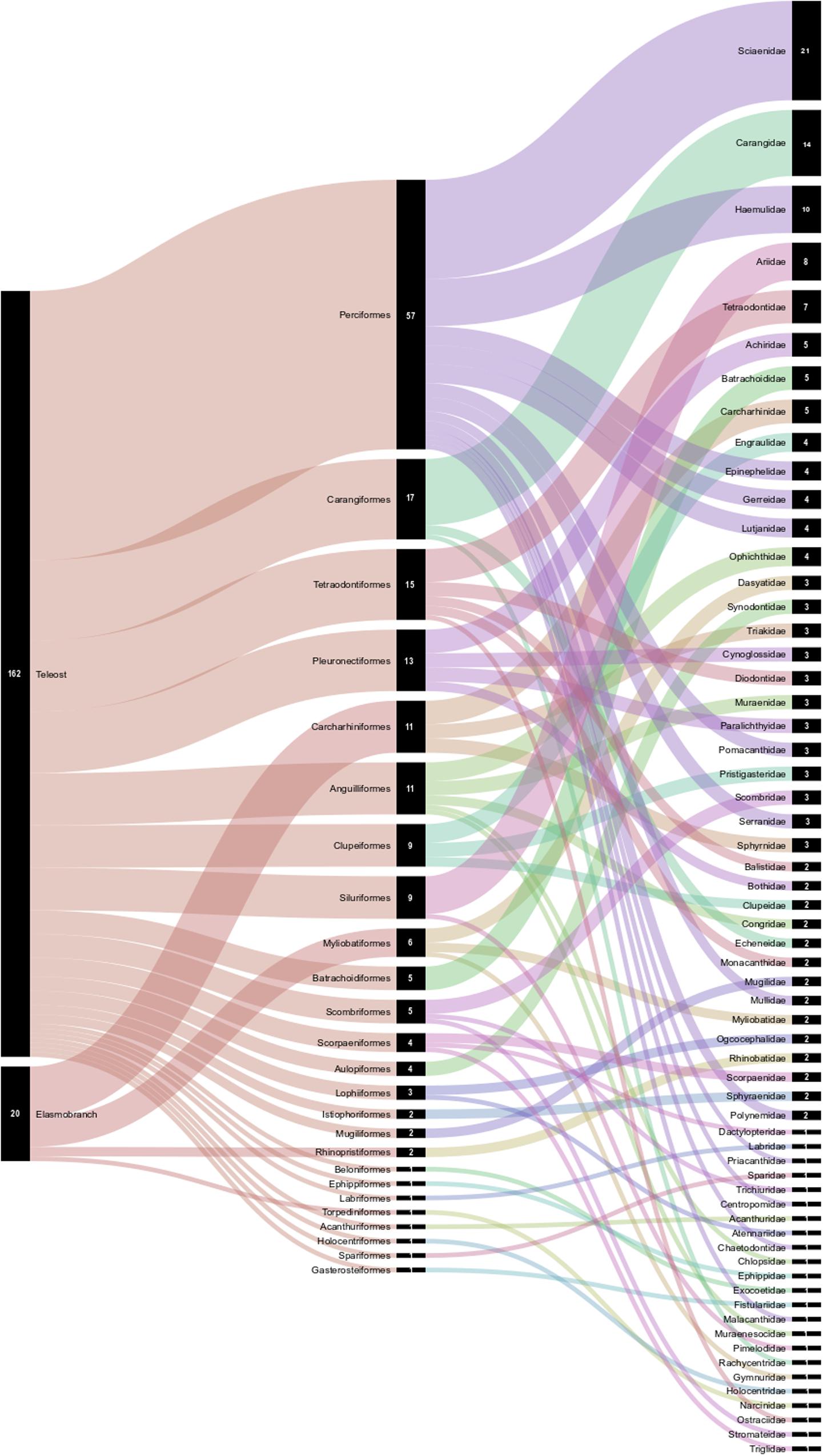
Figure 2. Orders and families of teleosts and elasmobranchs taken as bycatch by the northern Brazilian shrimp trawl fisheries. The numbers in the black columns represents the richness of the taxa in each order and family. The chart was created using the free online software RAWGraphs (https://rawgraphs.io/).
According to Fricke et al. (2020), the composition of the bycatch was dominated by species with a wide geographical distribution: 20 species, including sharks (Carcharhinus falciformis, Carcharhinus leucas, Sphyrna mokarran, and Sphyrna lewini), rays (Aetobatus narinari), and members of the families Carangidae (Selar crumenophthalmus, Seriola rivoliana, and Decapterus tabl), Scombridae (Auxis rochei), Sphyraenidae (Sphyraena barracuda), Exocoetidae (Cheilopogon cyanopterus), Echeneidae (Remora australis and Echeneis naucrates), and Monacanthidae (Aluterus monoceros and Aluterus scriptus) have a circumtropical distribution (see Table 1). In addition, 57 species identified are distributed throughout the Western Atlantic Ocean, while another 23 species are amphi-Atlantic, including species also distributed in the Mediterranean, the Black Sea (Priacanthus arenatus and Caranx crysos), and in the Western Indian Ocean (Kaupichthys hyoproroides). We identified some species that also occur in both the Atlantic and the eastern Pacific, including the shark Sphyrna tiburo (Sphyrnidae), the hamulid Haemulon steindachneri, the mugilids Mugil hospes and Mugil curema, and the engraulid, Anchoa spinifer. Finally, the remainder species have a more restricted distribution, with 27 occurring in the southwestern and western central Atlantic, four in the northwestern and central Atlantic, 11 only in the western central Atlantic, and 15 found only in the southwestern Atlantic, including 13 new occurrences for the North coast of Brazil (Table 1). Species that have an affinity with the freshwater system were also recorded, including Brachyplatystoma vaillantii, Cathorops agassizii, Rhinosardinia amazonica, and Pellona flavipinnis, which are found in the Amazon basin and coastal rivers of South America.
DNA Barcoding
We were able to obtain usable DNA sequences from 112 of the 182 morphospecies identified during the present study. We obtained 624 bp of the COI gene from 557 fish specimens, and no deletions, insertions, and stop codons were detected in the final alignment. All the sequences were submitted to BOLD Systems, and details of the specimen list and BINs are provided in Supplementary Table S2. The Kimura 2-Parameter (K2P) model revealed a hierarchical increase in nucleotide divergence, with a mean divergence of 0.10% between members of the same species, 12.84% between species of the same genus, and 19.10% between species of the same family (Table 2).
The lowest mean interspecific divergence was observed between Carcharhinus acronotus and Carcharhinus falciformis (4.47%), while the highest value was recovered between Prionotus punctatus and Aluterus monoceros (23.41%). In general, the nucleotide divergence between congeners was 1.3 times greater than that between specimens of the same species, which confirms the existence and magnitude of the Barcode Gap (Table 2 and Figure 3).
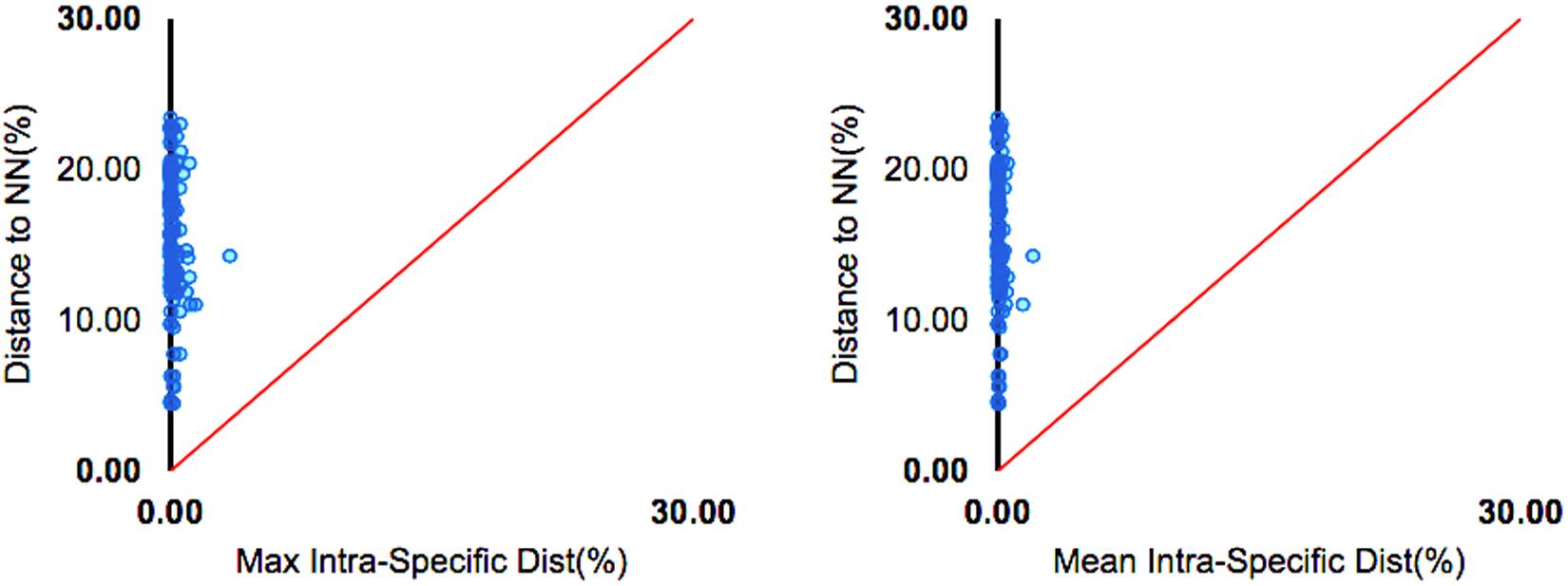
Figure 3. Barcode gap analysis of the COI sequences of the fish taken as bycatch by shrimp trawling vessels off the North Coast of Brazil. The dot plot shows the maximum intra-specific distances vs. the inter-specific (nearest neighbor) distances.
The Bayesian phylogenetic tree (Figure 4) was effective for the separation of all the identified sequences of the different species into 112 clusters, or OTUs (Supplementary Tables S1, S2) supported by high bootstrap values (>90), although the three methods of species delimitation (ABGD, GMYC, and bPTP) indicated the formation of 113 clusters. These methods indicated that the nine specimens identified as Notarius grandicassis, based on their external morphology, may actually belong to two different groups, that is, OTUs 92 and 93 (Supplementary Table S1).
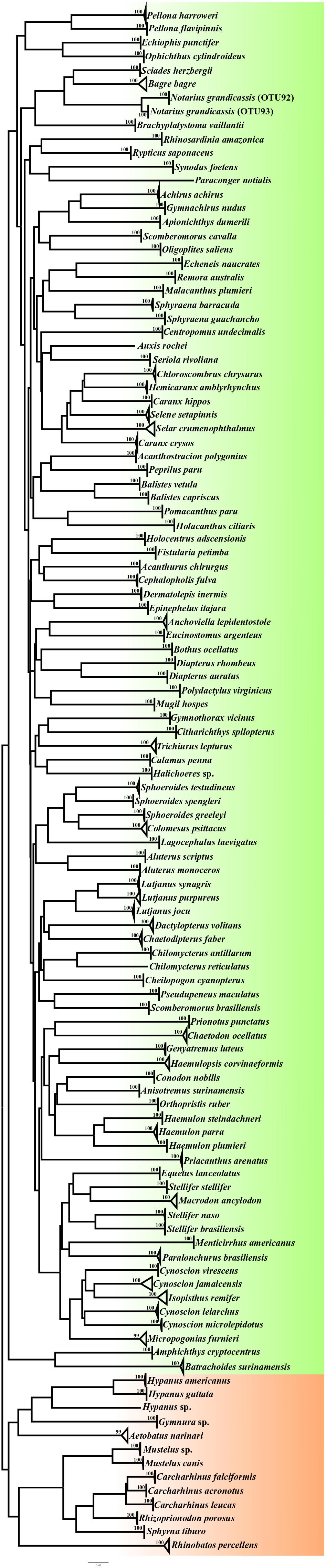
Figure 4. Neighbor-Joining (NJ) tree based on the COI barcode, showing the diversity of fish from the North Coast of Brazil.
New Occurrences on the Northern Coast of Brazil and in the Western Atlantic
We also recorded species not previously known to occur on the northern coast of Brazil or in the western Atlantic. In particular, we identified Paraconger notialis (Congridae), a demersal conger eel, originally assumed to be endemic to the coastal regions of the eastern Atlantic Ocean, between Senegal and Angola (Kanazawa, 1961; Sylla et al., 2016). Our molecular diagnosis was confirmed using the ultrametric topology (Figure 5) generated in the BEAST program, in which our specimens grouped in the P. notialis cluster of the sequences from the complete mitochondrial genome of this species downloaded from the BOLD Systems and GenBank databases. All three species delimitation methods (GMYC, ABGD, and bPTP) also confirmed the formation of the P. notialis cluster.
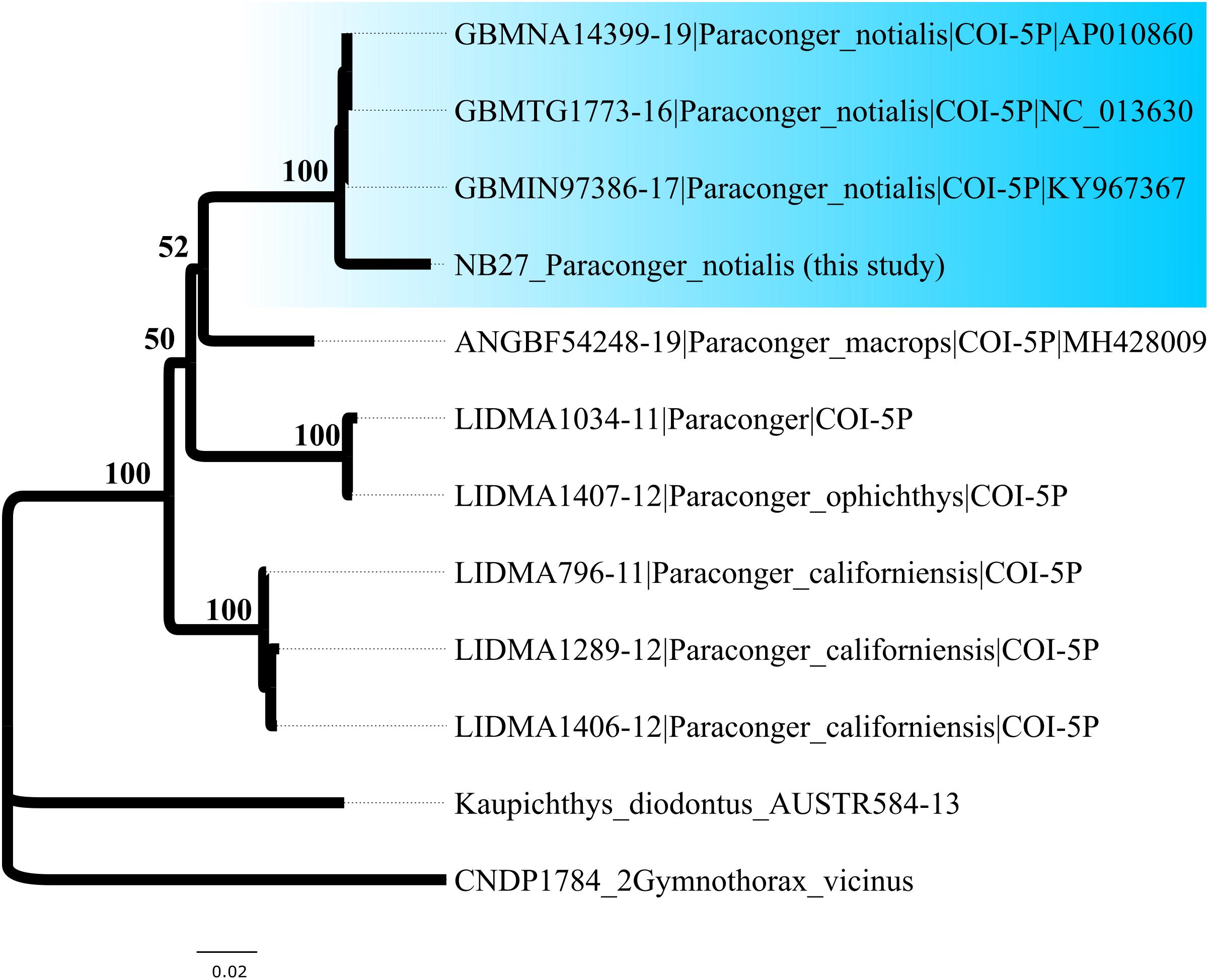
Figure 5. New occurrence of Paraconger notialis in the western Atlantic. The topology of the genus Paraconger generated in RAxML indicates the position (blue clade) of the specimen NB27 captured at the mouth of the Amazon in the P. notialis cluster. The delimitations based on the GMYC, ABGD, and bPTP approaches all confirmed the validity of this cluster.
Other species known to occur in the Atlantic Ocean were also recorded for the first time on the northern coast of Brazil (see previous records in Table 1). The teleosts Umbrina canosai (Sciaenidae), Dermatolepis inermis (Serranidae), Syacium gunteri (Paralichthyidae), Synodus foetens (Synodontidae), Scorpaena bergii (Scorpaenidae), Gymnothorax nigromarginatus (Muraenidae), Trichopsetta ventralis (Bothidae), and Symphurus rhytisma (Cynoglossidae). Three elasmobranchs, Mustelus canis (Triakidae), Narcine brasiliensis (Narcinidae), and Rhinobatos horkelii (Rhinobatidae), were also recorded on the northern Brazilian coast for the first time. These species have been recorded previously mainly in the southwestern Atlantic, south of the Brazilian state of Rio de Janeiro, southwards to Argentina (Fricke et al., 2020).
Species Delimitation of the Genus Isopisthus in the Bycatch
The different species delimitation approach produced unexpected results for the COI sequences of the Isopisthus (Sciaenidae) bycatch specimens, when compared with those downloaded from BOLDSystems. The GMYC (single threshold), bPTP, and ABGD analyses (P = 0.035938) recovered three species-level Isopisthus clusters. Cluster 1 was composed of Isopisthus parvipinnis, a species found in the Atlantic Ocean (Figure 6). However, cluster 2 was formed by Isopisthus remifer, a species known previously to occur in the eastern Pacific Ocean grouped specimens identified as I. remifer, which were collected in the Atlantic (collected by C. O. Data available at Boldsystems)4.
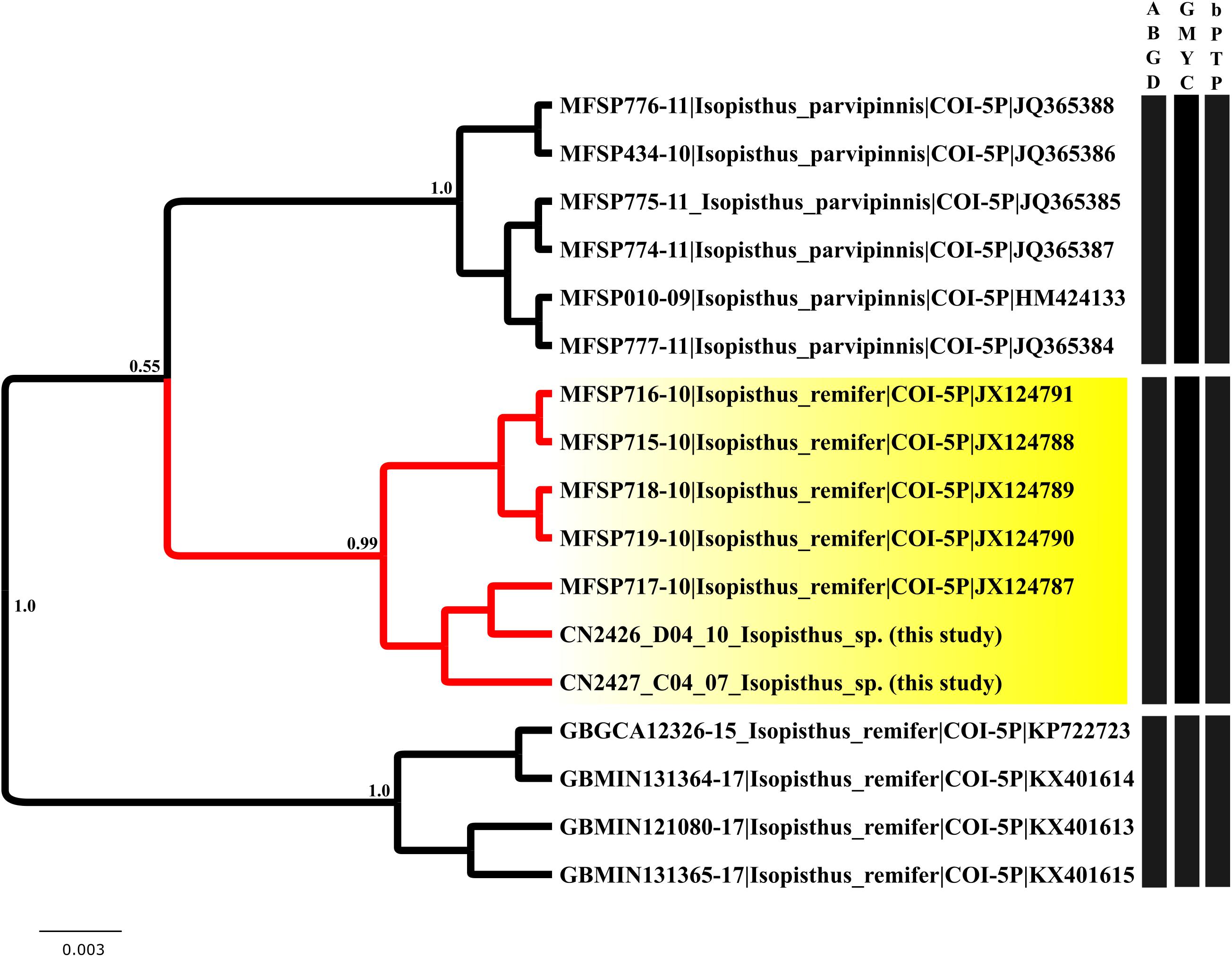
Figure 6. Ultrametric Bayesian topology obtained in BEAST, representing the species delimitation of the Isopisthus specimens analyzed in the present study. The analysis formed three clusters in all three species delimitation methods (GMYC, bPTP, and ABGD). The Isopisthus bycatch specimens formed a cluster distinct from the valid species of the genus, and was grouped with specimens identified as I. remifer, collected in the Atlantic Ocean.
Conservation Status
The IUCN Red List classifies the 20 elasmobranchs identified in the present study in different categories, including five species as Data Deficient (DD) and three as Least Concern (LC). However, six other species—Aetobatus narinari (Aetobatidae), Carcharhinus acronotus, C. leucas (Carcharhinidae), Mustelus canis (Triakidae), Rhinoptera bonasus (Rhinopteridae), and Pseudobatos percellens (Rhinobatidae)—are Near Threatened (NT). The Silky Shark Carcharhinus falciformis is classified as Vulnerable (VU), while Sphyrna lewini (Sphyrnidae) and Pseudobatos horkelii are Critically Endangered (CR) in the wild (Figure 7).
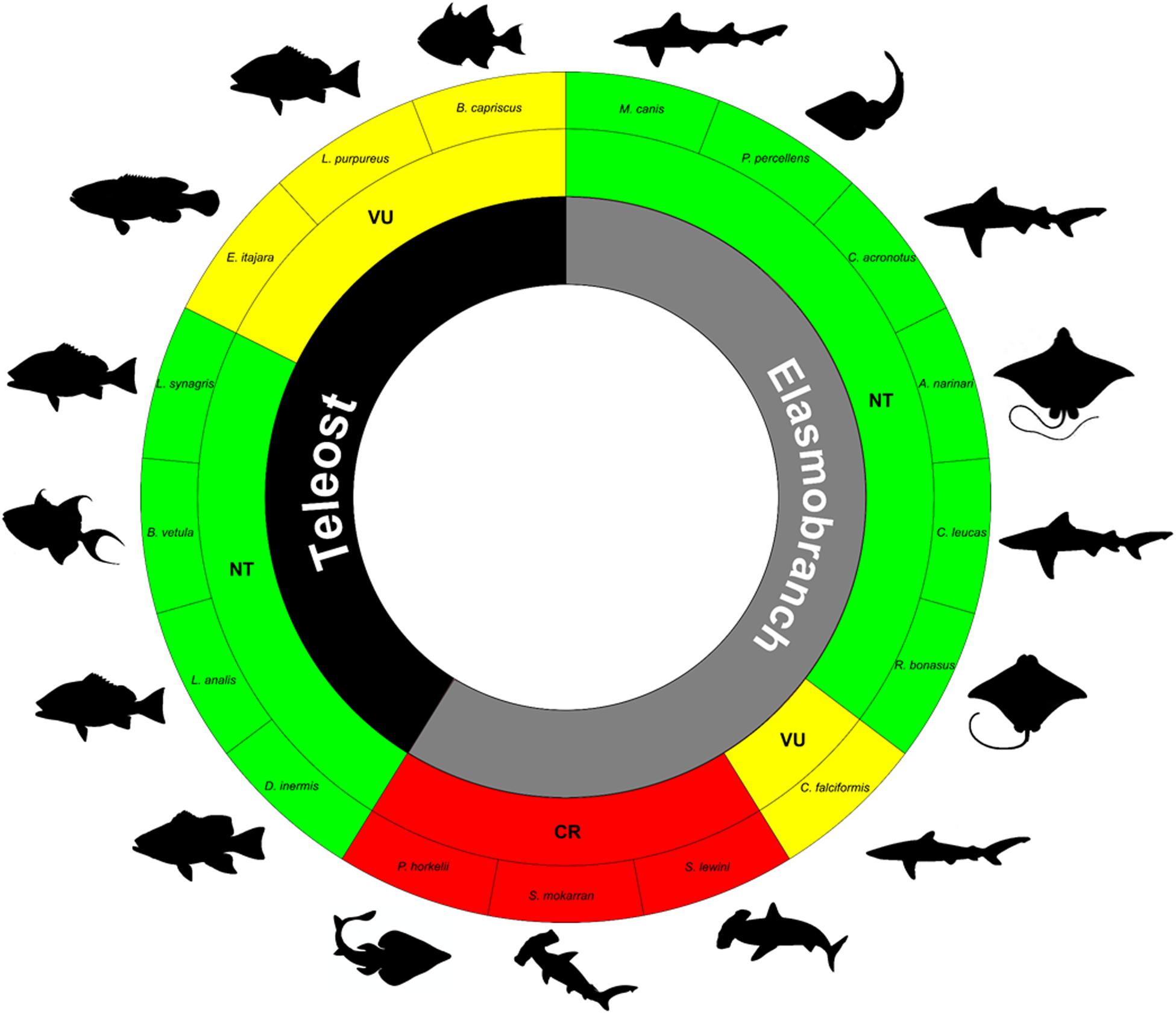
Figure 7. Conservation status of the fish species caught as bycatch of the North coast of Brazil. The classifications were obtained from the IUCN Red List of Threatened Species (https://www.iucnredlist.org/).
The vast majority of the teleost species identified in the bycatch in the present study are classified as Least Concern (LC) by the IUCN (Figure 7). However, some epinephelids, lutjanids, and balistids have been assigned to the Near Threatened (Dermatolepis inermis, Lutjanus synagris, L. analis, and Balistes vetula) or Vulnerable (Balistes capriscus, Epinephelus itajara, and Lutjanus purpureus) categories.
The Brazilian List of Endangered Fauna (MMA ordinance numbers 444/2014 and 445/2014) classifies the elasmobranchs M. canis and S. mokarran as Endangered (EN), and S. tiburo, S. lewini, P. horkelii as Critically Endangered (CR). This list also includes the teleost L. purpureus as Vulnerable (VU) and E. itajara as Critically Endangered (CR).
Discussion
DNA Barcoding
The COI gene (Barcoding region) was effective for the taxonomic delimitation of the 557 fish specimens analyzed in the present study using the most popular species delimitation methods. Our results revealed details of the little-known diversity of the biogeographic region located between the Caribbean and Brazilian provinces (Briggs and Bowen, 2012). The combined analysis of morphological characteristics and COI sequences nevertheless resulted in a slight divergence, given that, whereas 112 morphospecies were identified in the BOLD analysis, 113 potential species were recovered by the three species delimitation methods. This divergence was due to the separation of the Notarius grandicassis (Ariidae) specimens into two OTUs, 92 and 93 (Supplementary Table S1) with a 3.2% genetic divergence, which supports the existence of cryptic species in this region, which require further assessment.
The effectiveness of the COI gene for the delimitation of species is related directly to the Barcode Gap, that is, the difference between the maximum and minimum intraspecific distances in the COI sequences (Meyer and Paulay, 2005). In the present study, we did not detect any overlap between the maximum intraspecific distance (3.40%) and the minimum interspecific distance (4.47%), which is consistent with the existence of the Barcode Gap. The clusters of the NJ tree were supported by high bootstrap values (>90). With the gene used in the present study for cluster analyses, we were able to observe a phylogenetic signal for the delimitation of some monophyletic groups, such as the Siluriformes, Tetraodontidae, and Sciaenidae, an approach that appears to be effective, as discussed by Hebert et al. (2003a).
New Species Occurrences From the Northern Coast of Brazil
The findings of the present study contribute to a better understanding of the diversity and distribution of fish species in this poorly studied region of the Brazilian coast. In particular, the data resolve a distribution gap in the known occurrence of Mustelus canis (Triakidae) and Dermatolepis inermis (Serranidae), previously restricted to the northwestern Atlantic, between Massachusetts, the Gulf of Mexico, and the Caribbean, and southeastern Brazil, with a gap in the central Atlantic, which coincides with the Amazon coast (Conrath, 2009; Claro et al., 2015). We also confirmed (i) the expansion of the known geographic distribution of three species (Narcine brasiliensis, Rhinobatos horkelii, and Umbrina canosai) previously known only from southeastern Brazil, (ii) the occurrence of three species (Syacium gunteri, Synodus foetens, Scorpaena bergii, and Gymnothorax nigromarginatus) recorded previously only in the northern and central regions of the western Atlantic, but not on the northern coast of Brazil, and (iii) the occurrence in the region of a species (Trichopsetta ventralis) recorded previously only in the western North Atlantic (Table 1).
The bycatch analyzed in the present study also provided the first record of the Guinea conger Paraconger notialis (Congridae) in the western Atlantic. The genus Paraconger Kanazawa, 1961, currently includes seven recognized species of which only two—Paraconger caudilimbatus Poey, 1867 and Paraconger guianensis Kanazawa, 1961—had been recorded previously in the western Atlantic Ocean (Nolf and Aguilera, 1998; Aguilera and Lundberg, 2010). As only a single specimen of P. notialis was collected in the present study, however, it remains unclear whether the species is established in the western Atlantic Ocean.
One other important finding of the present study was the collection of an unknown species of Isopisthus (Sciaenidae). Two valid Isopisthus species are known to inhabit the coastal and estuarine waters of South America, I. parvipinnis, which is found in the western Atlantic, in Central and South America, and I. remifer, previously assumed to be endemic to the eastern Pacific. The molecular evidence from the present study indicates that two individuals of the genus Isopisthus formed a third, divergent cluster. All three species delimitation methods (GMYC, bPTP, and ABGD) applied in the present study confirmed the existence of this cluster (Figure 6). Although the geographic distribution of this new lineage is unclear, these findings indicate the existence of an as yet unknown Isopisthus species in the western Atlantic, which may further increase the diversity of one of the most diverse families of Perciformes. There is thus a clear need for the reassessment of the taxonomy and zoogeography of this group.
Few studies have evaluated the diversity of the fish bycatch taken by the shrimp trawling fleets of the Brazilian coast. While these operations appear to be decimating the fish diversity of Brazilian waters, there is little official monitoring or control of these activities. A larger number of taxa (182 species, 123 genera, and 62 families) were identified in the bycatch analyzed in the present study, which is consistent with the findings on other industrial fisheries, in northern (Marceniuk et al., 2019, N = 201 species), northeastern (Silva-Júnior et al., 2018, N = 51 species), and southern Brazil (Vianna and Almeida, 2005, N = 91 species; Branco et al., 2015, N = 124 species). This further highlights the impact of trawling fisheries on the biodiversity of the Brazilian coast. Trawling is the most common shrimping method used in the region, which impacts most the fauna of sciaenids and haemulids, the families that make up a large part of the fish bycatch taken off the coast of Brazil (see Vianna and Almeida, 2005; Branco et al., 2015; Silva-Júnior et al., 2013, 2015; Marceniuk et al., 2019). This pressure from shrimp trawling operations, combined with the overfishing of some of the commercially important species may lead to widespread ecological impacts and the decline of stocks.
In the present study, the bycatch fauna included 20 elasmobranch species, of which nine are subject to some level of global threat, as indicated by the IUCN Red List, while five species are also included in the Brazilian list of endangered fauna. Most of the bycatch harvested in this tropical region is made up of fish, which increases the fishing pressure on many of these species (Wassenberg and Hill, 1989). In addition to bycatch, the demand for shark fins has driven an increase in the commercial harvesting of these species in the world (Musick et al., 2000; Stobutzki et al., 2001; Feitosa et al., 2018).
The predominance of teleosts, in particular lutjanids, serranids, and balistids, should be a priority for research and management initiatives, given that these families tend to already suffer pressure from overfishing. A prime example is the Atlantic goliath grouper, E. itajara, which is classified globally as Vulnerable (VU), but in Brazil, this species has suffered a significant population decline due to fishing pressure, primarily on juvenile individuals, which has led to its classification as Critically Endangered (CR) in the Brazilian list of endangered fauna.
In addition to these considerations, the analysis of the composition of the shrimping bycatch provided important insights into the marine biodiversity of the northern coast of Brazil. This region encompasses the Amazon Plume, formed by sediment a persistent and massive discharge (Moura et al., 2016). The complexity of the local aquatic habitats accounts for the diversity of local fish assemblages, which include freshwater, estuarine, and marine species, some of which have extremely limited geographic distributions.
Conclusion
The DNA barcoding technique was highly effective for the delimitation of the fish species taken as bycatch by shrimp trawling operations off the northern coast of Brazil. This region not only has a rich fauna of widely distributed species, but the study also revealed the occurrence of a number of species in the western Atlantic for the first time. The analyses also revealed the presence in the bycatch of a number of elasmobranchs and teleosts that have an at risk conservation status.
On the Brazilian coast, there is no systematic monitoring of fisheries in terms of catches or biomass that would permit a more reliable evaluation of the potential threat of bycatch (see debate in Reis-Filho and Leduc, 2017; Reis-Filho, 2019). We thus conclude that local and regional measures of management and control will be more effective when supported by: (i) systematic surveillance to assess the intensity of the impact of bottom trawling on non-target species, and provide basic data on the impact of these fisheries; (ii) the implementation of continuous monitoring to determine the spatio-temporal patterns in catches and fishing effort, and (iii) the evaluation and implementation of Bycatch Reduction Devices (BRDs) to minimize the accidental catches in shrimp trawls. The need for collaboration and feedback between the trawling fleet and resource managers cannot be overstated, nor can the importance of more reliable data, such as those presented in this study. Clearly, the success of any initiative of this type will depend on the constant evaluation of management strategies and the trustworthy involvement of the stakeholders.
Data Availability Statement
The datasets generated for this study can be found in the online repositories. The names of the repository/repositories and accession number(s) can be found in the article/ Supplementary Material.
Ethics Statement
The animal study was reviewed and approved by the Instituto Chico Mendes de Conservação da Biodiversidade.
Author Contributions
TG: design and conception of the experiments. FM, MA, EC, and TG: data collection and specimen processing. AG-C, RA, and IS: molecular analyses. AG-C, FM, RA, JR-F, MA, EC, IS, and TG: production and review of the manuscript. All authors contributed to the article and approved the submitted version.
Funding
This work was supported by the “Rede cooperativa multidisciplinar para subsidiar o manejo da pesca dos estoques de camarões da região Norte e Nordeste do Brasil com foco ecossistêmico” (MCTI/MPA/CNPq number 2/2015), Ordenamento da Pesca Marinha Brasileira, Linha temática III: Camarões da Costa Norte/Nordeste (N/NE) (Process number 445766/2015-8). This publication was supported by Pró-Reitoria de Pesquisa e Pós-Graduação - PROPESP/UFPA (No. 01/2020 PAPQ).
Conflict of Interest
The authors declare that the research was conducted in the absence of any commercial or financial relationships that could be construed as a potential conflict of interest.
Acknowledgments
We would like to thank the Brazilian National Council for Scientific and Technological Development (CNPq) and the Coordination for Higher Education Personnel Training (CAPES) for financial support. TG received a productivity grant from CNPq (#311078/2019-2). Our thanks to the editor and reviewers for the constructive comments and recommendations which improved quality of the paper.
Supplementary Material
The Supplementary Material for this article can be found online at: https://www.frontiersin.org/articles/10.3389/fmars.2020.566021/full#supplementary-material
Footnotes
- ^ http://ncbi.nlm.nih.gov/
- ^ http://BOLDsystems.org/
- ^ https://species.h-its.org/ptp/
- ^ http://boldsystems.org/
References
Aguilera, O., and Lundberg, J. (2010). Venezuelan Caribbean and Orinocoan neogene fish. Urumaco and Venezuelan Paleontology. Bloomington: Indiana Press University.
Araújo, R. G., da Silva, R. D. F., Sampaio, I., and Guimarães-Costa, A. (2019). Does DNA barcoding offer meaningful insights into the diversity of the parrotfish of the genus Sparisoma (Scaridae)? J. Appl. Ichthyol. 35, 1029–1033. doi: 10.1111/jai.13932
Bornatowski, H., Braga, R. R., and Vitule, J. R. S. (2014). Threats to sharks in a developing country: the need for effective simple conservation measures. Nat. Conserv. 12, 11–18. doi: 10.4322/natcon.2014.003
Branco, J. O., Freitas Júnior, F., and Christoffersen, M. L. (2015). Bycatch fauna of seabob shrimp trawl fisheries from Santa Catarina State, southern Brazil. Biota Neotrop. 15, 1–14. doi: 10.1590/1676-06032015014314
Briggs, J. C., and Bowen, B. W. (2012). A realignment of marine biogeographic provinces with particular reference to fish distributions. J. Biogeogr. 39, 12–30. doi: 10.1111/j.1365-2699.2011.02613.x
Carpenter, K. E., FAO, and Europäische Kommission (2002a). Bony fishes, (Opistognathidae to Molidae), sea turtles and marine mammals. Rome: FAO.
Carpenter, K. E., FAO, and Europäische Kommission (2002b). Introduction, Molluscs, Crustaceans, Hagfishes, Sharks, Batoid Fishes And Chimaeras. Rome: FAO.
Chee, P. E. (1996). A review of the bycatch and discards in the fisheries of Southeast Asia - In Report on the Technical Consultation on Reduction of Wastage in Fisheries, FAO Fisheries Report. No 547, eds I. J. Clucas and D. James (Rome: FAO).
Claro, R., Zapp-Sluis, M., and Sedberry, G. (2015). Dermatolepis Inermis. The IUCN Red List of Threatened Species 2015: e.T39303A70317343. Available at: https://www.iucnredlistorg (accessed September 2018).
Collette, B. B., and Rützler, K. (1977). “Reef fishes over sponge bottoms off the mouth of the Amazon River,” in Proceedings of the Third International Coral Reef Symposium, Miami.
Conrath, C. (2009). Mustelus canis. The IUCN Red List of Threatened Species. Available online at: https://dx.doi.org/10.2305/IUCN.UK.2005.RLTS.T39359A10215463.en (accessed March 18, 2019).
Conte-Grand, C., Britz, R., Dahanukar, N., Raghavan, R., Pethiyagoda, R., Tan, H. H., et al. (2017). Barcoding snakeheads (Teleostei, Channidae) revisited: discovering greater species diversity and resolving perpetuated taxonomic confusions. PLoS One 12:e0184017. doi: 10.1371/journal.pone.0184017
Darriba, D., Taboada, G. L., Doallo, R., and Posada, D. (2012). jModelTest 2: more models, new heuristics and parallel computing. Nat. Methods 9:8. doi: 10.1038/nmeth.2109
Ekau, W., and Knoppers, B. (1999). An introduction to the pelagic system of the North-East and East Brazilian shelf. Arch. Fish. Mar. Res. 47, 113–132.
Ezard, T., Fujisawa, T., and Barraclough, T. G. (2009). SPLITS: SPecies’ LImits by Threshold Statistics. R package version 1.0-18/r45. Available at: http://R-Forge.R-project.org/projects/splits/ (accessed June 2013).
Feitosa, L. M., Martins, A. P. B., Giarrizzo, T., Macedo, W., Monteiro, I. L., Gemaque, R., et al. (2018). DNA-based identification reveals illegal trade of threatened shark species in a global elasmobranch conservation hotspot. Sci. Rep. 8, 1–11. doi: 10.1206/754.1
Figueiredo, J. L., and Menezes, N. (1978). Manual dos Peixes Marinhos do Sudeste do Brasil. II Teleostei 1. São Paulo: Museu de Zoologia da USP. doi: 10.5962/bhl.title.109986
Figueiredo, J. L., and Menezes, N. (1980a). Manual dos Peixes Marinhos do Sudeste do Brasil. III Teleostei 2. São Paulo: Museu de Zoologia da USP.
Figueiredo, J. L., and Menezes, N. (1980b). Manual dos Peixes Marinhos do Sudeste do Brasil. IV Teleostei 3. São Paulo: Museu de Zoologia da USP.
Floeter, S., Rocha, L. A., Robertson, D. R., Joyeux, J. C., Smith-Vaniz, W. F., Wirtz, P., et al. (2008). Atlantic reef fish biogeography and evolution. J. Biogeogr. 35, 22–47. doi: 10.1111/j.1365-2699.2007.01790.x
Floeter, S. R., and Gasparini, J. L. (2000). The southwestern Atlantic reef fish fauna: composition and zoogeographic patterns. J. Fish Biol. 56, 1099–1114. doi: 10.1111/j.1095-8649.2000.tb02126.x
Fricke, R., Eschmeyer, W. N., and Van der Laan, R. (eds) (2020). Eschmeyer’s Catalog Of Fishes: Genera, Species, References. Available at: http://researcharchive.calacademy.org/research/ichthyology/catalog/fishcatmain.asp (accessed March 13, 2020).
Gray, C. A., and Kennelly, S. J. (2018). Bycatches of endangered, threatened and protected species in marine fisheries. Rev. Fish Biol. Fish. 28, 521–541. doi: 10.1007/s11160-018-9520-7
Hall, T. A. (1999). BioEdit: a user-friendly biological sequence alignment editor and analysis program for Windows 95/98/NT. Nucleic Acids Symp. Ser. 41, 95–98. doi: 10.14601/Phytopathol_Mediterr-14998u1.29
Harrington, J. M., Myers, R. A., and Rosenberg, A. A. (2006). Wasted fishery resources: discarded by-catch in the USA. Fish Fish. 6, 350–361. doi: 10.1111/j.1467-2979.2005.00201.x
Hebert, P. D., Cywinska, A., Ball, S. L., and Dewaard, J. R. (2003a). Biological identifications through DNA barcodes. Proc. R. Soc. B-Biol. Sci. 270, 313–321. doi: 10.1098/rspb.2002.2218
Hebert, P. D., Ratnasingham, S., and Ward, J. R. (2003b). Barcoding animal life: cytochrome c oxidase subunit 1 divergences among closely related species. Proc. R. Soc. B-Biol. Sci. 270, S96–S99. doi: 10.1098/rsbl.2003.0025
Huelsenbeck, J. P., and Ronquist, F. (2001). MRBAYES: bayesian inference of phylogenetic trees. Bioinformatics 17, 754–755. doi: 10.1093/bioinformatics/17.8.754
Isaac, V. J., and Barthem, R. B. (1995). Os recursos pesqueiros da Amazônia Brasileira. Bol. Mus. Para. Emílio Goeldi. 11, 151–194.
Kanazawa, R. H. (1961). Paraconger, a new genus with three new species of eels (family Congridae). Proc. U. S. Natl. Mus. 113, 1–14. doi: 10.5479/si.00963801.113-3450.1
Kimura, M. (1980). A simple method for estimating evolutionary rates of base substitutions through comparative studies of nucleotide sequences. J. Mol. Evol. 16, 111–120. doi: 10.1007/BF01731581
Lara, R. J., and Dittmar, T. (1999). Nutrient dynamics in a mangrove creek (North Brazil) during the dry season. Mangroves Salt Marshes 3, 185–195. doi: 10.1023/A:1009903824243
Lewison, R. L., Freeman, S. A., and Crowder, L. B. (2004). Quantifying the effects of fisheries on threatened species: the impact of pelagic longlines on loggerhead and leatherback sea turtles. Ecol. Lett. 7, 221–231. doi: 10.1111/j.1461-0248.2004.00573.x
Marceniuk, A. P., Rotundo, M. M., Caires, R. A., Cordeiro, A. P. B., Wosiacki, W. B., Oliveira, C., et al. (2019). The bony fishes (Teleostei) caught by industrial trawlers off the Brazilian North coast, with insights into its conservation. Neotrop. Ichthyol. 17:e180038. doi: 10.1590/1982-0224-20180038
Meade, R. H., Dunne, T., Richey, J. E., Santos, U. M., and Salati, E. (1985). Storage and remobilization of suspended sediment in the lower Amazon River of Brazil. Science 228, 488–490. doi: 10.1126/science.228.4698.488
Meyer, C. P., and Paulay, G. (2005). DNA barcoding: error rates based on comprehensive sampling. PLoS Biol. 3:e422. doi: 10.1371/journal.pbio.0030422
Moura, R. L., Amado-Filho, G. M., Moraes, F. C., Brasileiro, P. S., Salomon, P. S., Mahiques, M. M., et al. (2016). An extensive reef system at the Amazon River mouth. Sci. Adv. 2:e1501252. doi: 10.1126/sciadv.1501252
Musick, J. A., Burgess, G., Cailliet, G., Camhi, M., and Fordham, S. (2000). Management of sharks and their relatives (Elasmobranchii). Fisheries 25, 9–13. doi: 10.1577/1548-84462000025<0009:MOSATR<2.0.CO;2
Nolf, D., and Aguilera, O. (1998). Fish otoliths from the Cantaure Formation (early Miocene of Venezuela). Bull. lInst. R. Sci. Nat. Belgique Sci. Terre 68, 237–262.
Pauly, D., Christensen, V., Dalsgaard, J., Froese, R., and Torres, F. Jr. (1998). Fishing Down Marine Food Webs. Science 279, 860–863. doi: 10.1126/science.279.5352.860
Pauly, D., Christensen, V., Guénette, S., Pitcher, T. J., and Walters, C. J. (2002). Towards sustainability in world fisheries. Nature 418, 689–695. doi: 10.1038/nature01017
Pons, J., Barraclough, T. G., Gomez-Zurita, J., Cardoso, A., Duran, D. P., Hazell, S., et al. (2006). Sequence-based species delimitation for the DNA taxonomy of undescribed insects. Syst. Biol. 55, 595–609. doi: 10.1080/10635150600852011
Puillandre, N., Lambert, A., Brouillet, S., and Achaz, G. (2012). ABGD, Automatic Barcode Gap Discovery for primary species delimitation. Mol. Ecol. 21, 1864–1877. doi: 10.1111/j.1365-294X.2011.05239.x
R Core Team (2018). R: A Language and Environment for Statistical Computing. Vienna: R Foundation for Statistical Computing.
Ratnasingham, S., and Hebert, P. D. (2007). BOLD: the Barcode of Life Data System (http://www. barcodinglife. org). Mol. Ecol. Notes 7, 355–364. doi: 10.1111/j.1471-8286.2007.01678.x
Reis-Filho, J. A. (2019). Historical perspective of artisanal encircling gillnet use at the Brazilian coast: changes in fishing behaviour is mirrored by dwindling stocks. Fish. Manag. Ecol. 27, 155–166. doi: 10.1111/fme.12393
Reis-Filho, J. A., and Leduc, A. O. H. C. (2017). Management-Challenged Brazilian Governance and the Low Relevance of National Fishery Management Policy: recommendations to promote viable Small-Scale Fisheries. Fish. Oceanogr. 2:555583. doi: 10.19080/OFOAJ.2017.02.555583
Rocha, L. A. (2003). Patterns of distribution and processes of speciation in Brazilian reef fishes. J. Biogeogr. 30, 1161–1171. doi: 10.1046/j.1365-2699.2003.00900.x
Schmid, K., and Giarrizzo, T. (2017). New record of a largetooth sawfish specimen from the Amazon river estuary in Northern Brazil. Fisheries 42, 254–255. doi: 10.1080/03632415.2017.1276357
Silva-Júnior, C. A. B. D., Araújo, M. E. D., and Feitosa, C. V. (2013). Sustainability of capture of fish bycatch in the prawn trawling in northeastern Brazil. Neotrop. Ichthyol. 11, 133–142. doi: 10.1590/S1679-62252013000100016
Silva-Júnior, C. A. B., Lira, A. S., Eduardo, L. N., Viana, A. P., Lucena-Frédou, F., and Frédou, T. (2018). Ichthyofaunabycatch of the artisanal fishery of penaeid shrimps in Pernambuco, Northeastern Brazil. Bol. Inst. Pesca 45:435. doi: 10.20950/1678-2305.2019.45.1.435
Silva-Júnior, C. A. B., Viana, A. P., Frédou, F. L., and Frédou, T. (2015). Aspects of the reproductive biology and characterization of Sciaenidae captured as bycatch in the prawn trawling in the northeastern Brazil. Acta Sci. Biol. Sci. 37, 1–8. doi: 10.4025/actascibiolsci.v37i1.24962
Stamatakis, A. (2006). RAxML-VI-HPC: maximum likelihood-based phylogenetic analyses with thousands of taxa and mixed models. Bioinformatics 22, 2688–2690. doi: 10.1093/bioinformatics/btl446
Stobutzki, I. C., Miller, M. J., Jones, P., and Salini, J. P. (2001). Bycatch diversity and variation in a tropical Australian penaeid fishery; the implications for monitoring. Fish. Res. 53, 283–301. doi: 10.1016/s0165-7836(00)00273-3
Sylla, S., Kouakou, K. F., Tia, C. B., Yao, S. S., and Atse, B. C. (2016). The spatial distribution of coastal fish assemblage in Côte d’Ivoire’s Exclusive Economic Zone (EEZ). West Africa. J. Appl. Biosci. 108, 10519–10530. doi: 10.4314/jab.v108i1.5
Thompson, J. D., Higgins, D. G., and Gibson, T. J. (1994). CLUSTAL W: improving the sensitivity of progressive multiple sequence alignment through sequence weighting, position-specific gap penalties and weight matrix choice. Nucleic Acids Res. 22, 4673–4680. doi: 10.1093/nar/22.22.4673
Vianna, M., and Almeida, T. (2005). Bony fish bycatch in the southern Brazil pink shrimp (Farfantepenaeus brasiliensis and F. paulensis) fishery. Braz. Arch. Biol. 48, 611–623. doi: 10.1590/S1516-89132005000500014
Ward, N. D., Krusche, A. V., Sawakuchi, H. O., Brito, D. C., Cunha, A. C., Moura, J. M. S., et al. (2015). The compositional evolution of dissolved and particulate organic matter along the lower Amazon River—Óbidos to the ocean. Mar. Chem. 177, 244–256. doi: 10.1016/j.marchem.2015.06.013
Ward, R. D., Zemlak, T. S., Innes, B. H., Last, P. R., and Hebert, P. D. (2005). DNA barcoding Australia’s fish species. Philos. Trans. R. Soc. Lond. B Biol. Sci. 360, 1847–1857. doi: 10.1098/rstb.2005.1716
Wassenberg, T. J., and Hill, B. J. (1989). The effect of trawling and subsequent handling on the survival rates of the by-catch of prawn trawlers in Moreton Bay, Australia. Fish. Res. 71, 99–110. doi: 10.1016/0165-7836(89)90010-6
Willems, T., Depestele, J., De Backer, A., and Hostens, K. (2016). Ray bycatch in a tropical shrimp fishery: do bycatch reduction devices and turtle excluder devices effectively exclude rays? Fish. Res. 175, 35–42. doi: 10.1016/j.fishres.2015.11.009
Worm, B., Barbier, E. B., Beaumont, N., Emmett, D. J., Folke, C., Halpern, B. S., et al. (2006). Impacts of biodiversity loss on ocean ecosystem services. Science 314, 787–790. doi: 10.1126/science.1132294
Keywords: conservation, DNA-barcode, genetic identification, bycatch composition, threatened species
Citation: Guimarães-Costa A, Machado FS, Reis-Filho JA, Andrade M, Araújo GR, Corrêa EMR, Sampaio I and Giarrizzo T (2020) DNA Barcoding for the Assessment of the Taxonomy and Conservation Status of the Fish Bycatch of the Northern Brazilian Shrimp Trawl Fishery. Front. Mar. Sci. 7:566021. doi: 10.3389/fmars.2020.566021
Received: 26 May 2020; Accepted: 08 September 2020;
Published: 29 September 2020.
Edited by:
Sophie von der Heyden, Stellenbosch University, South AfricaReviewed by:
Romina Henriques, Stellenbosch University, South AfricaJoseph D. DiBattista, Australian Museum, Australia
Giacomo Bernardi, University of California, Santa Cruz, United States
Copyright © 2020 Guimarães-Costa, Machado, Reis-Filho, Andrade, Araújo, Corrêa, Sampaio and Giarrizzo. This is an open-access article distributed under the terms of the Creative Commons Attribution License (CC BY). The use, distribution or reproduction in other forums is permitted, provided the original author(s) and the copyright owner(s) are credited and that the original publication in this journal is cited, in accordance with accepted academic practice. No use, distribution or reproduction is permitted which does not comply with these terms.
*Correspondence: Aurycéia Guimarães-Costa, auryceia@yahoo.com.br