- 1Department of Marine Sciences, College of Marine Sciences, Hainan University, Haikou, China
- 2Key Laboratory of Tropical Hydrobiology and Biotechnology of Hainan Province, Hainan University, Haikou, China
- 3State Key Laboratory of Marine Resource Utilization in South China Sea, Hainan Aquaculture Breeding Engineering Research Center, Hainan University, Haikou, China
Ammonium is the main nitrogen resources for scleractinian coral-Symbiodiniaceae symbiotic association, and there is urgent need to investigate the involvement of ammonium assimilation in the heat acclimation of the symbiotic association to heat stress. In the present study, symbiont density and chlorophyll content, as well as redox and ammonium assimilation enzyme activities, in two symbiotic partners of the scleractinian coral Pocillopora damicornis were firstly investigated after acute heat stress (32°C). Symbiont density and chlorophyll content decreased significantly at 24 h (1.81 × 106 cell cm–2, p < 0.05) and 36 h (23.25 pg cell–1, p < 0.05) after heat stress, respectively. We observed significant activity increases of coral nitric oxide synthase, superoxide dismutase and catalase, and symbiont superoxide dismutase after heat stress, but no alterations in the activities of symbiont nitric oxide synthase and catalase during the whole experiment period. The activities of coral glutamine synthetase and glutamine oxoglutarate aminotransferase began to increase significantly at 24 h, and reached the peak at 36 h after heat stress. As for coral glutamate dehydrogenase activity, a significant increase was observed only at 36 h after heat stress. The activities of symbiont glutamine synthetase and glutamine oxoglutarate aminotransferase did not change significantly after heat stress. Secondly, symbiont density, chlorophyll content, apoptosis rate, coral total antioxidant capacity, and caspase3 activation level were determined after glufosinate (glutamine synthetase inhibitor) treatment. Symbiont density decreased significantly at 6 h (1.25 × 106 cell cm–2, p < 0.05) after glufosinate treatment, and symbiont chlorophyll content also decreased significantly during 12–24 h after glufosinate treatment, with the lowest level at 12 h (16.69 pg cell–1, p < 0.05). Furthermore, the coral total antioxidant capacity and caspase3 activation level both increased significantly at 12 h (0.57 U mg–1, 2.08-fold, p < 0.05) after glufosinate treatment, while no significant change was observed for the symbiont apoptosis rate. These results suggest that the ammonium assimilation activity in the coral host P. damicornis, not its symbiont, was induced by acute heat stress, which might contribute to the acclimatization of the symbiotic association to high temperature through regulating coral antioxidant capacity and apoptosis.
Introduction
Scleractinian corals are main builders of coral reef, which provide habitats for one-quarter to one-third of all marine species (Plaisance et al., 2011). In order to thrive in oligotrophic reef environment, scleractinian corals have evolved the symbiotic relationship with unicellular, photosynthetic dinoflagellate algae of the family Symbiodiniaceae that are harbored within corals’ endodermal cells (Dubinsky and Jokiel, 1994). Scleractinian corals provide shelter and inorganic nutrients for their symbiotic Symbiodiniaceae. In return, Symbiodiniaceae supply the coral host with photosynthates and other organic nutrients, which can meet up to 95% of the corals’ energy requirements (Muscatine and Porter, 1977). The mutual nutrient exchange determines the stability and maintenance of the coral-Symbiodiniaceae symbiosis (Liu et al., 2018; Wall et al., 2020). However, it has been considered that the coral-Symbiodiniaceae symbiosis is vulnerable to environmental changes, especially for elevated seawater temperature (Vidal-Dupiol et al., 2011).
The rise of the mean annual sea surface temperature due to global warming has been threating the symbiosis between scleractinian corals and Symbiodiniaceae (Wooldridge, 2013). The typical physiological response of the symbiotic association to high temperature is the loss of symbiotic Symbiodiniaceae from coral host or the degradation of symbiont’s photosynthetic pigments, which will lead to the collapse of coral-Symbiodiniaceae symbiosis (Coles and Brown, 2003; Hughes et al., 2017). It has been considered that the symbiosis collapse can attribute to excessive oxidative pressure in the symbiotic association owing to induced production of oxygen free radicals under heat stress, which is able to result in the damage of D1 protein (a crucial component of the photosynthetic electron transport chain) in symbiont chloroplast PSII system and the activation of apoptosis in coral host (Lesser, 1997; Roberty et al., 2015). Subsequently, symbionts are expelled from the coral host, and photosynthates will reduce dramatically, causing the coral host not to get sufficient nutrient and energy supply from their symbionts (Pernice et al., 2011; Wein et al., 2019). The imbalance of the nutrient exchanges under heat stress finally results in the collapse of the symbiosis, coral bleaching, and even death.
Nitrogen nutrient can also be exchanged between scleractinian corals and symbiotic Symbiodiniaceae, which is of importance for the maintenance of their symbiosis (Radecker et al., 2015). Coral host takes up and assimilates limited ammonium as main nitrogen resource from oligotrophic seawater environment through two key pathways including glutamine synthetase (GS)/glutamine oxoglutarate aminotransferase (GOGAT) pathway, and glutamate dehydrogenase (GDH) pathway, and symbiotic Symbiodiniaceae assimilate ammonium from coral host through GS/GOGAT pathway (Pernice et al., 2012; Roberty et al., 2020). The assimilated ammonium is used to synthesize amino acid and protein in the symbiotic association, and further symbionts can provide coral host with essential amino acid and other nitrogen-containing organics (Shinzato et al., 2011). Generally, symbiotic Symbiodiniaceae requires more ammonium nutrient than the coral host, and therefore are under nitrogen limitation (Peng et al., 2012). It has been believed that nitrogen limitation of symbionts is beneficial for the maintenance of the symbiosis because it obliges symbionts to produce and translocate more photosynthates and restrain their own reproduction (Radecker et al., 2015; Morris et al., 2019; Xiang et al., 2020). However, the role of nitrogen nutrient exchange, especially ammonium assimilation, in the acclimation of the coral-Symbiodiniaceae symbiotic association to high temperature, is still not well understood.
Scleractinian coral Pocillopora damicornis belongs to the family Pocilloporidae and is widely distributed in the tropical and subtropical areas of the Indian and Pacific Oceans. In the present study, P. damicornis was employed as a model of scleractinian corals to explore the involvement of ammonium assimilation in the acclimation of coral-Symbiodiniaceae symbiosis to high temperature. Firstly, the symbiont density, chlorophyll content, redox and ammonium assimilation enzyme activities in two symbiotic partners after acute heat stress were measured to investigate the response of ammonium assimilation in the coral-Symbiodiniaceae symbiotic association to high temperature. Secondly, GS inhibitor was used to repress ammonium assimilation ability of the coral-Symbiodiniaceae symbiotic association to further explore the potential regulation of heat acclimation by ammonium assimilation. Our results will provide insights into the effect of ammonium assimilation on the maintenance of coral-Symbiodiniaceae symbiosis and the environment acclimation of scleractinian corals.
Materials and Methods
Coral
Three P. damicornis colonies were collected from a fringing reef at a depth 1.5 m in Wenchang, China, and have been shown to harbor Symbiodiniaceae within the genus Cladocopium as dominant symbionts (unpublished work). The branches in the colonies were split as nubbins, and these nubbins were attached on the plastic mesh plate located in the bottom of flow-through aquaria (ca. 500 L) filled with natural seawater (26°C). All nubbins were illuminated with white and blue cool fluorescent bulbs (Philips T5HO Activiva Active 54 W) at a light intensity of about 500 μmol photons m–2 s–1 in a 12 h/12 h light–dark cycle for 1 month to acclimatize to laboratory conditions.
Acute Heat Treatment Experiment
Forty-eight coral nubbins from three colonies (16 nubbins each colony) were employed in the heat treatment experiment. Twenty-four nubbins were transferred into the heated seawater (32°C), which were employed as the heat group. The rest of 24 nubbins were kept in 26°C seawater as the control group. Coral nubbins were randomly sampled in the heat and control groups after 0, 12, 24, and 36 h of incubation. Six nubbins (2 nubbins each colony, 3 colonies giving rise to 6 biological replicates) were sampled from each group at each time point to measure the density and chlorophyll content of the symbionts, as well as the enzyme activities of the two symbiotic partners.
Glufosinate Treatment Experiment
Water-soluble glufosinate was used to inhibit GS activity in the coral-Symbiodiniaceae symbiotic association under high temperature (32°C). A total of 48 coral nubbins from three colonies (16 nubbins each colony) were used in the glufosinate treatment experiment and divided into two groups, including glufosinate and control groups. Twenty-four nubbins in the glufosinate group were transferred into glufosinate-containing and heated seawater (final concentration 10 μmol L–1, 32°C), and 24 nubbins in the control group were only placed into the heated seawater (32°C). To measure the density, apoptosis and chlorophyll content of the symbionts and enzyme activities of the two symbiotic partners, 6 nubbins (2 nubbins each colony, 3 colonies giving rise to 6 biological replicates) were sampled randomly from the glufosinate and control groups at 0, 6, 12, and 24 h after the treatment.
Determination of Symbiont Density
The density variation of symbionts was measured following a previous method with few modifications (Higuchi et al., 2008; Higuchi et al., 2015). Briefly, coral tissues were homogenized in 10 mL phosphate buffered saline (PBS, 377 mmol L–1 NaCl, 2.7 mmol L–1 KCl, 8.09 mmol L–1 Na2HPO4, 1.47 mmol L–1 KH2PO4, pH 7.4); 1-mL homogenates were then centrifuged at 5000 rpm, 4°C for 15 min, and the symbiont pellets were resuspended in PBS and counted using a Neubauer hemocytometer (QIUJING, China). The surface area of the nubbins was determined using the aluminum foil method (Johannes et al., 1970). The symbiont density was defined as its number per unit surface area of the coral nubbins (cells cm–2).
Measurement of Chlorophyll Content
Chlorophyll content of symbionts was determined as previously reported (Hedouin et al., 2016). Briefly, 2-mL homogenates were centrifuged at 12,000 rpm, 4°C for 3 min. The harvested symbionts were resuspended in PBS and further centrifuged at 12,000 rpm, 4°C for 3 min. Chlorophyll a and c2 were extracted for 24 h at 4°C in 2 mL of 100% acetone, followed by the centrifugation at 12,000 rpm, 4°C for 3 min. The absorbance values of the extracts were measured at 630 and 663 nm. The total content of chlorophyll a + c2 was computed according to the equations of Jeffrey and Humphrey (1975). The total quantity of chlorophyll a + c2 was divided by the number of symbionts to yield chlorophyll content (pg cell–1).
Activity Assays of Ammonium Assimilation Enzymes in Coral and Symbiont
Six-milliliter homogenates were centrifuged at 5,000 rpm, 4°C for 15 min to collect the supernatants for the activity determination of enzymes in the coral host. The symbiont pellets were resuspended in 6 mL of PBS, following by a homogenate. After the centrifugation at 12,000 rpm, 4°C for 3 min, the supernatants were harvested for the activity detection of symbiont enzymes.
GS (BC0915, Solarbio, China), GDH (A125, JIANCHENG, China), and GOGAT (BC0070, Solarbio, China) kits were employed to determine their activities in the coral and symbiont supernatants according to manufacturer’s protocol. After the total enzyme activities were obtained, the concentrations of total protein in the supernatants were quantified using bicinchoninic acid assay (BCA) method (Zhou et al., 2018) and used to normalize the measured activities of GS, GDH, and GOGAT to U mg–1 protein.
Determination of the Redox Parameters in Coral and Symbiont
The activities of superoxide dismutase (SOD), catalase (CAT), nitric oxide synthetase (NOS), and total antioxidant capacity in the coral and symbiont supernatants were measured using commercial kits (A001, A007, A014, and A015, JIANCHENG, China), following the manufacturer’s recommendations. The measured enzyme activity or capacity was divided by the total protein to yield specific activity expressed as U mg–1 protein.
Detection of Caspase3 Activation Level in Coral
The caspase3 activity in the coral supernatant was measured by Caspase-3 Colorimetric Assay Kit (KeyGEN, China) according to the instruction. Briefly, the supernatants of all nubbin samples were diluted firstly to the same protein concentration. Then, 50 μL supernatant was added in the reaction mixture containing 50 μL reaction buffer and 5 μL substrate, following incubation for 4 h in the dark at 37°C. Finally, the color change of the mixture was detected spectrophotometrically at the wavelength of 405 nm. The activity of caspase3 was defined as the absorbance of the reaction solution at 405 nm (A405), and the activation level of caspase3 in the coral was defined as the ratio of A405 in samples to that of the control group at 0 h.
Symbiont Apoptosis Assay
The apoptosis of symbionts was detected using the Apoptosis-Hoechst staining kit and the Tubulin-Tracker Red fluorescent probe (C0003, C1050, Beyotime, China). Briefly, about 3.0 × 105 symbionts were fixed by the fixative supplied in the apoptosis kit for 10 min, following by washing three times with PBS containing 0.05% Tween-20 (PBS-T). Then, 0.5 mL Hoechst 33258 staining solution was added to incubate for 5 min, and the symbionts were washed three times with PBS-T. After incubation with 200 μL Tubulin-tracker Red staining solution in the dark for 30 min and three washing, the double-stained symbionts were transferred to a glass slide and observed under a fluorescence microscope. The cell nucleus of healthy symbionts was stained blue, whereas the nucleus of apoptotic symbionts was not stained blue. Symbiont apoptosis rate was evaluated by counting the number of apoptotic symbionts in 100 cells, and each counting was repeated three times.
Statistical Analysis
All data was presented as means ± standard deviation (SD) from biological replicates. A paired samples t-test was applied using SPSS v22.0 (SPSS Inc., Chicago, Illinois) to determine significant differences of physiological parameters between the treatment and control groups in the acute heat and glufosinate treatment experiments at each time point. Differences were considered significant at p < 0.05.
Results
Symbiont Density and Chlorophyll Content After Acute Heat Treatment
The density and chlorophyll a + c2 content of symbionts were determined in P. damicornis after heat treatment. The symbiont density decreased significantly at 24 h (1.81 ± 0.65 × 106 cell cm–2, p < 0.05), in comparison with that in the control group, and returned to the control level at 36 h after heat treatment (Figure 1A). The content of symbiont chlorophyll a + c2 also declined significantly after heat treatment, and it reached the lowest level at 36 h of treatment (23.25 ± 8.37 pg cell–1, p < 0.05) (Figure 1B).
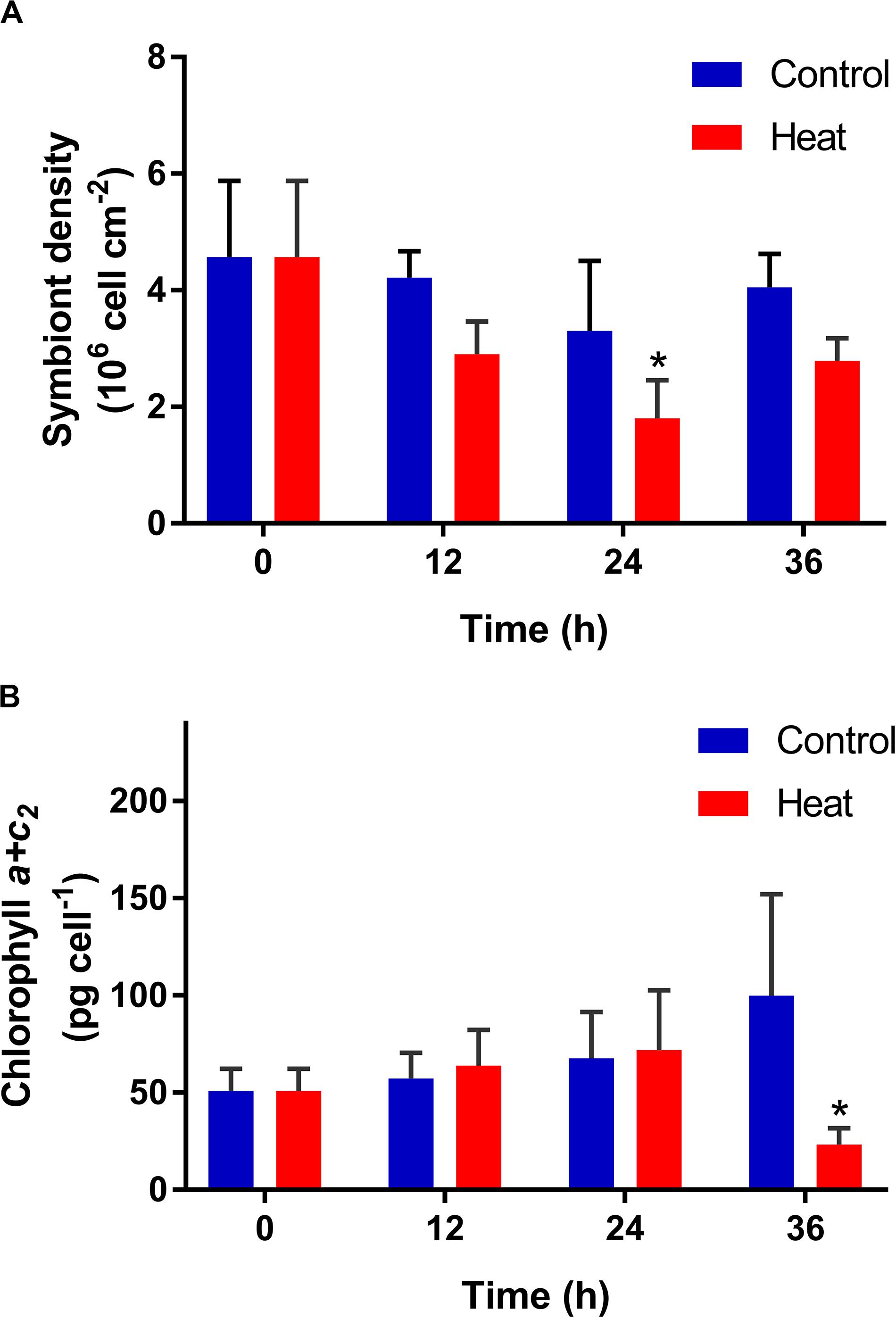
Figure 1. Temporal variation of symbiont density (A) and chlorophyll content (B) in the scleractinian coral Pocillopora damicornis after heat treatment. Data points represent means, and error bars represent standard deviations (N = 6). Asterisks depict significant differences between the heat and control groups (p < 0.05).
Temporal Alterations of Redox Parameters in the Symbiotic Association After Acute Heat Treatment
Coral NOS, SOD, and CAT activities in the heat group all showed significant increases, compared to those in the control group. NOS activity began to increase significantly at 12 h (2.27 ± 0.57 U mg–1, p < 0.05), and reached the peak at 36 h (5.64 ± 0.21 U mg–1, p < 0.05) after heat treatment (Figure 2A). SOD and CAT activities both increased significantly during 24–36 h after heat treatment, with the highest level at 36 h (329.90 ± 94.68 U mg–1 and 22.22 ± 5.48 U mg–1, respectively, p < 0.05) (Figures 2B,C).
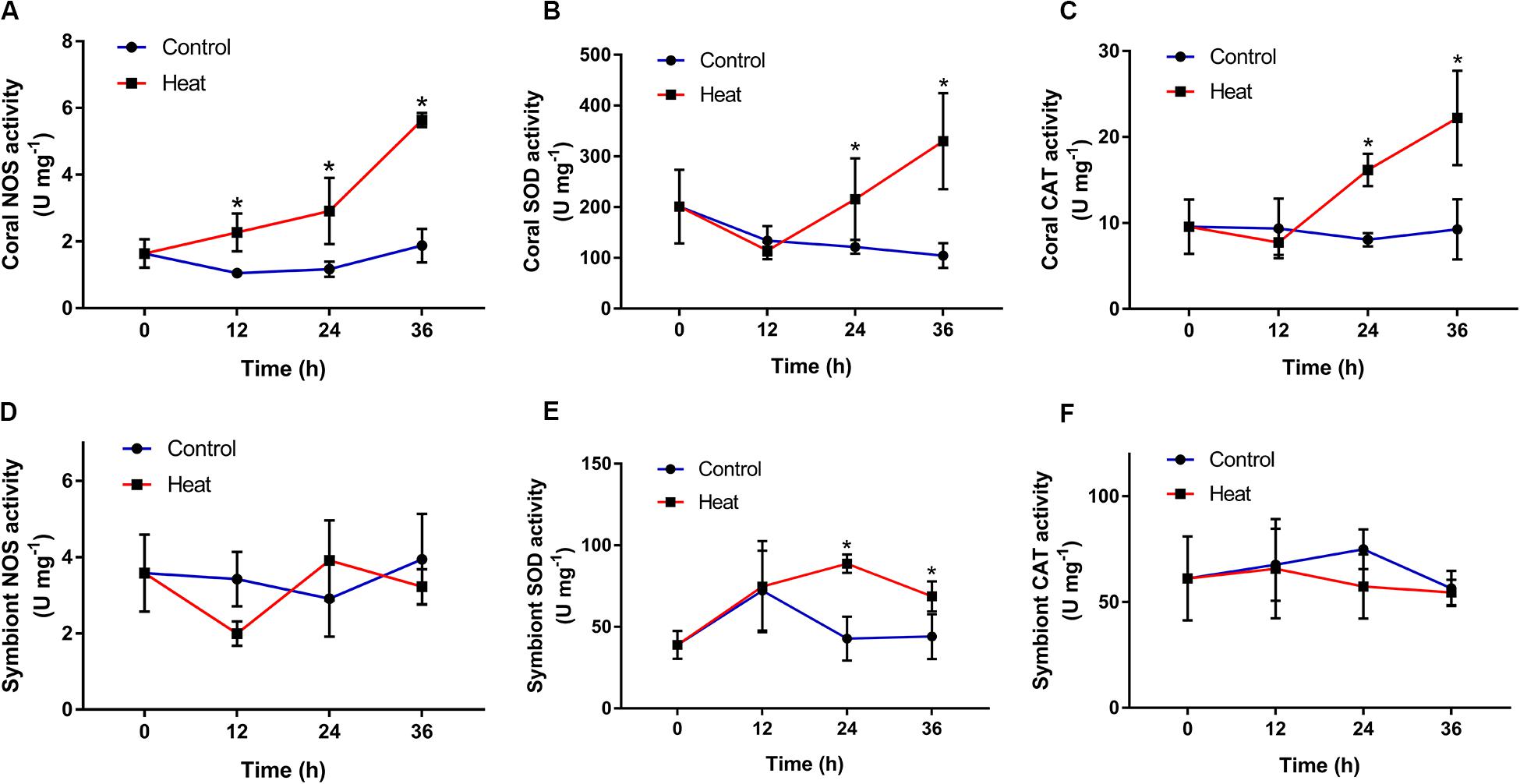
Figure 2. Temporal patterns of redox-related enzyme activities in the scleractinian coral Pocillopora damicornis and its symbionts after heat treatment. (A) Nitric oxide synthetase (NOS) in the coral. (B) Superoxide dismutase (SOD) in the coral. (C) Catalase (CAT) in the coral. (D) NOS in the symbionts. (E) SOD in the symbionts. (F) CAT in the symbionts. Data points represent means and error bars represent standard deviations (N = 6). Asterisks depict significant differences between the heat and control groups (p < 0.05).
Symbiont SOD activity underwent a significant rise during 24–36 h and peaked at 24 h after heat treatment (88.68 ± 5.63 U mg–1, p < 0.05) (Figure 2E). However, symbiont NOS and CAT activities in the heat group showed no significant changes throughout the experiment process, compared to those in the control group (Figures 2D,F).
Temporal Activities of Ammonium Assimilation Enzymes in the Symbiotic Association After Acute Heat Treatment
GS, GDH and GOGAT activities also increased significantly in the coral host after heat treatment, in comparison with those in the control group. GS and GOGAT both began to increase significantly at 24 h (2.73 ± 1.32 U mg–1, 131.27 ± 16.53 U mg–1, p < 0.05), and reached the peak level at 36 h (5.03 ± 1.48 U mg–1, 167.13 ± 51.39 U mg–1, p < 0.05) after heat treatment (Figures 3A,C). For GDH activity, a significant increase was observed only at 36 h (17.31 ± 6.82 U mg–1, p < 0.05) after heat treatment (Figure 3B). The activities of symbiont GS and GOGAT both did not change significantly after heat treatment during the whole treatment process (Figures 3D,E).
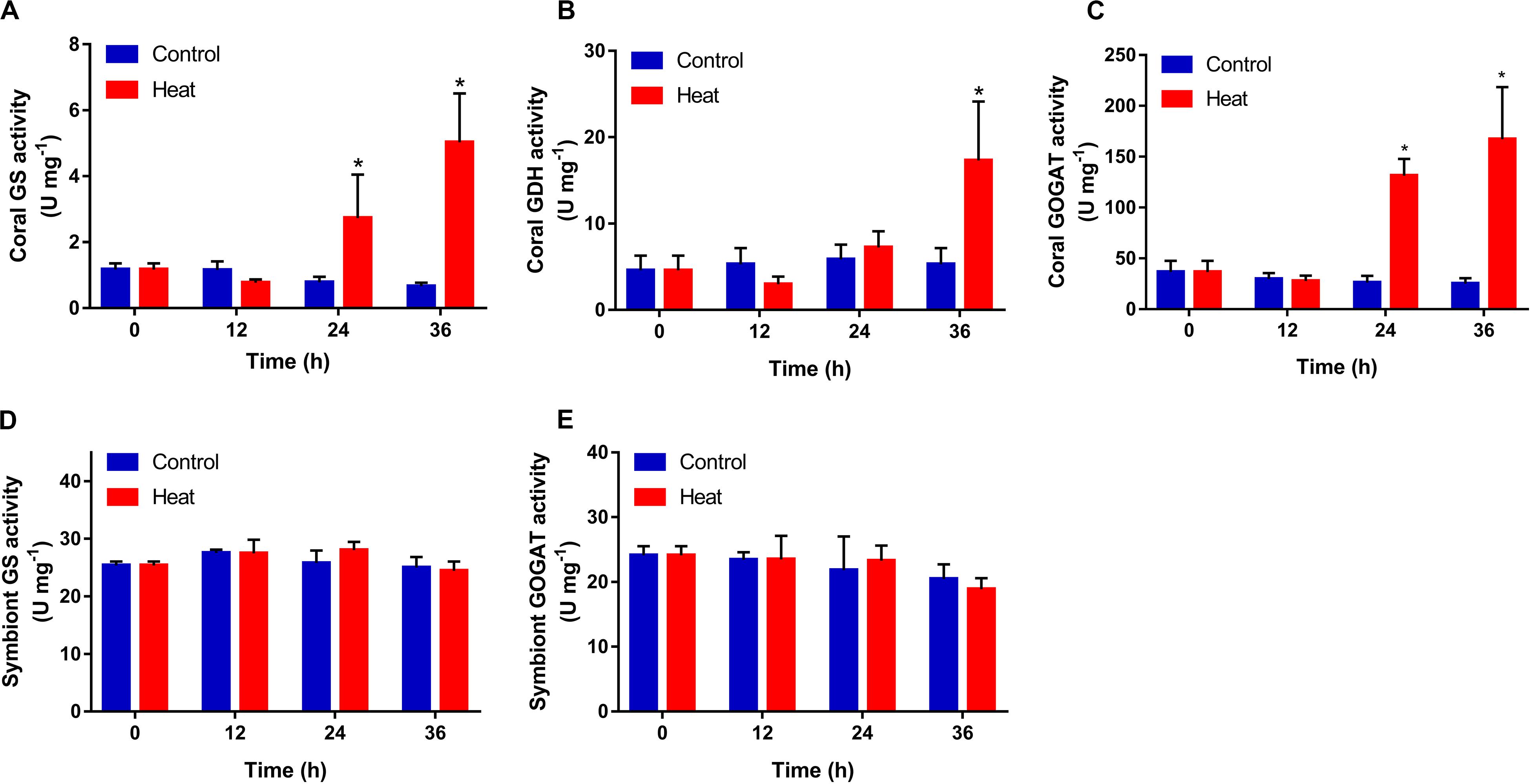
Figure 3. Temporal activities of ammonium assimilation enzymes in the scleractinian coral Pocillopora damicornis and its symbionts after heat treatment. (A) Glutamine synthetase (GS) in the coral. (B) Glutamate dehydrogenase (GDH) in the coral. (C) Glutamine oxoglutarate aminotransferase (GOGAT) in the coral. (D) GS in the symbionts. (E) GOGAT in the symbionts. Data points represent means and error bars represent standard deviations (N = 6). Asterisks depict significant differences between the heat and control groups (p < 0.05).
Symbiont Density and Chlorophyll Content After Glufosinate Treatment
The density and chlorophyll a + c2 content of symbiont were determined after glufosinate treatment. The symbiont density decreased significantly at 6 h (1.25 ± 0.28 × 106 cell cm–2, p < 0.05) after glufosinate treatment, compared by that in the control group (Figure 4A). The content of chlorophyll a + c2 per symbiont cell decreased significantly during 12–24 h after glufosinate treatment, with the lowest level at 12 h (16.69 ± 3.66 pg cell–1, p < 0.05) (Figure 4B).
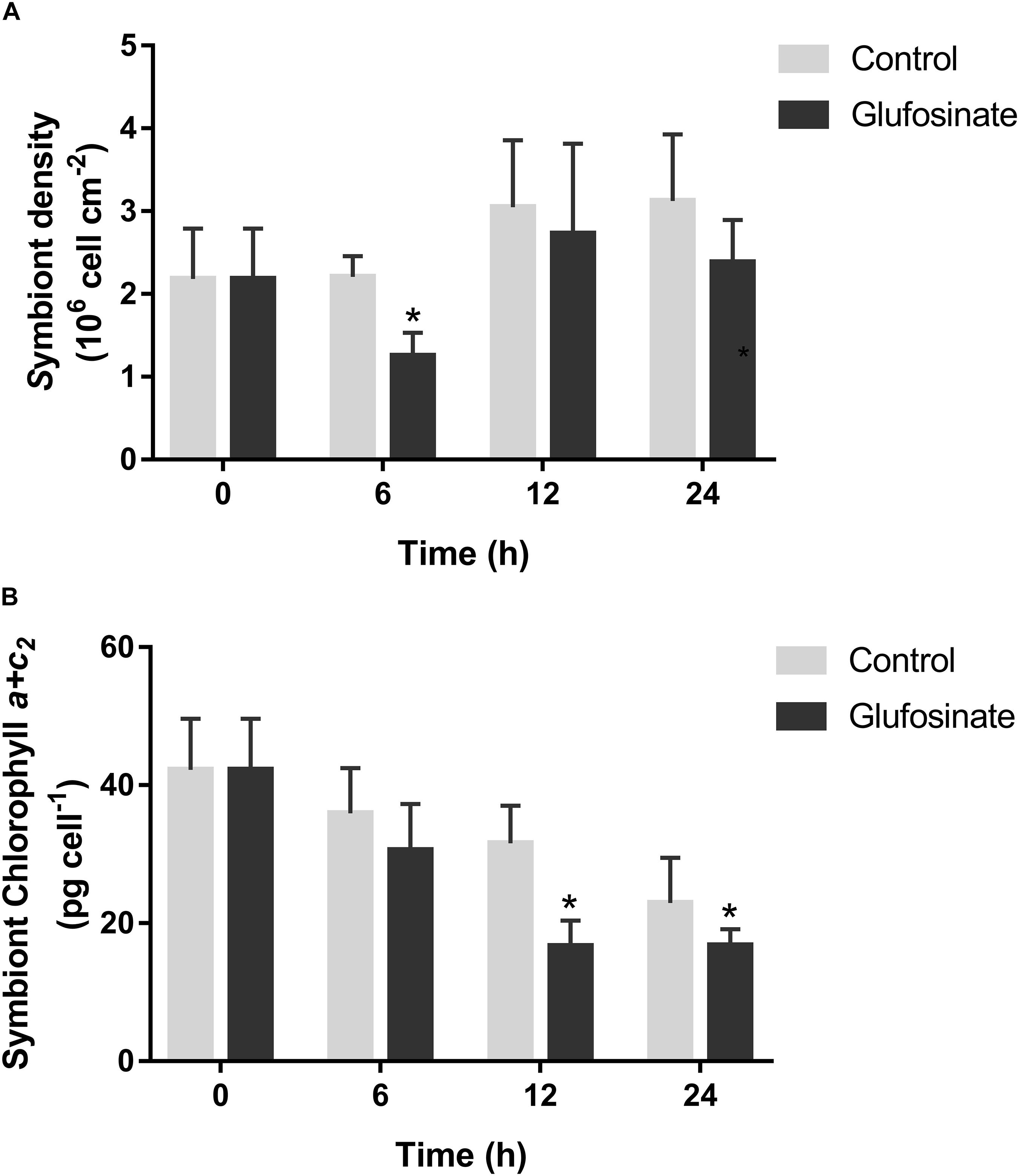
Figure 4. Temporal symbiont density (A) and chlorophyll content (B) in the scleractinian coral Pocillopora damicornis after glufosinate treatment. Data points represent means and error bars represent standard deviations (N = 6). Asterisks depict significant differences between the glufosinate and control groups (p < 0.05).
Antioxidant Capacity and Apoptosis of the Symbiotic Association After Glufosinate Treatment
The total antioxidant capacity of the coral host increased significantly at 12 h (0.57 ± 0.18 U mg–1, p < 0.05) after glufosinate treatment (Figure 5A), and its caspase3 activation level also rise significantly at 12 h (2.08 ± 0.37-fold, p < 0.05) after glufosinate treatment (Figure 5B), compared by those in the control group. In addition, no significant change was observed for the symbiont apoptosis rate in the glufosinate group at 12 and 24 h after the treatment of glufosinate (Figure 5C).
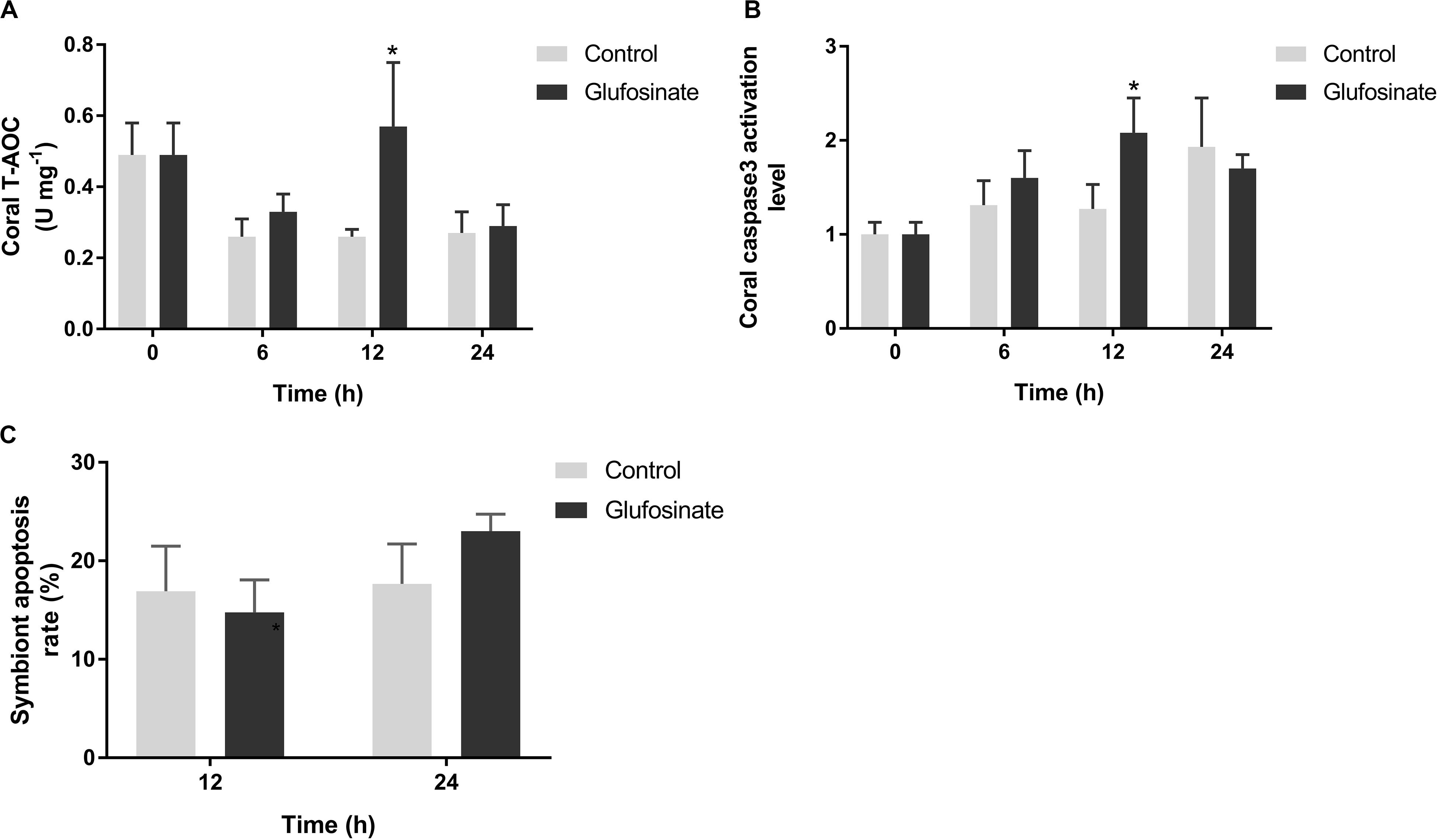
Figure 5. Total antioxidant capacity (T-AOC) (A) and caspase3 activation level (B) in Pocillopora damicornis and symbiont apoptosis rate (C) after glufosinate treatment. Data points represent means and error bars represent standard deviations (N = 6). Asterisks depict significant differences between the glufosinate and control groups (p < 0.05).
Discussion
Coral-Symbiodiniaceae symbiotic association assimilates ammonium as main inorganic nitrogen resource, which further forms a nitrogen cycling to sustain the symbiosis (Pernice et al., 2012; Radecker et al., 2015). However, little is known about the role of ammonium assimilation in heat acclimation of the coral-Symbiodiniaceae symbiotic association. In the present study, we found that the activities of GS, GDH, and GOGAT in the coral host P. damicornis all increased significantly under high temperature, along with the decrease of symbiont density and chlorophyll content. Furthermore, the treatment of glufosinate (GS inhibitor) under high temperature also caused the decline of density and chlorophyll content in the symbionts and the rise of total antioxidant capacity and apoptosis level in the coral host.
We employed firstly the exposure of the scleractinian coral P. damicornis to high temperature to simulate its acute heat stress, and subsequently determined the density and chlorophyll content of symbiotic Symbiodiniaceae. The symbiont density and chlorophyll a + c2 content decreased significantly at 24 and 36 h after the exposure to high temperature, respectively. The results revealed that high temperature (32°C) induced the decline of symbiont density and chlorophyll content in the scleractinian coral P. damicornis. The loss of symbionts and the degradation of its photosynthetic pigments are both typical characterizations of coral bleaching (Coles and Brown, 2003), and therefore, the present results demonstrated that the heat exposure could activate the heat stress response of the symbiotic association, and therefore impair the symbiotic relationship between the coral host and symbionts. These results could also attribute to the excessive oxidative pressure in symbiotic association and subsequent D1 protein damage in symbiont chlorophyll PSII system under high temperature (Flores-Ramirez and Linan-Cabello, 2007; Weis, 2008; Lesser, 2011). However, the symbiont density reverted to the control level at 36 h after heat exposure, indicating the possible real-time regulation of heat acclimatization of the symbiotic association through the symbiont acquisition of the coral host from seawater environment and/or the propagation of symbionts in the coral’s endodermal cells. It suggests that the acute heat stress induced the loss of symbionts and the degradation of its symbiont chlorophyll, and further resulted in the collapse of coral-Symbiodiniaceae symbiosis in the scleractinian coral P. damicornis.
To ascertain the mediation of redox system to the above symbiosis collapse, we monitored the activities of several enzymes including NOS, SOD, and CAT in the symbiotic association of P. damicornis after acute heat stress. The activities of NOS, SOD, and CAT in the coral host all increased significantly, while only SOD activity showed significant increase in the symbionts after heat stress. Because SOD and CAT are main antioxidases in the redox system of most organisms such as coral and Symbiodiniaceae (Levy et al., 2006), and their significant increases in the present study demonstrated that the acute heat stress induced the antioxidant capacity of the coral host and its symbionts. The rise of antioxidant capacity in the symbiotic association could result from elevated production of reactive oxygen species (ROS) owing to their heat stress response (Yakovleva et al., 2004; Levy et al., 2006; Teixeira et al., 2013). Furthermore, the significant increase of NOS activity in the coral host revealed the upregulation of nitric oxide (NO) production, which could bind with ROS to form reactive nitrogen species (RNS) with stronger oxidation ability, hinting excessive oxidative stress in the symbiotic association after heat stress. The excessive oxidative stress would activate the apoptosis of the coral host through the mediation of caspase3, damage the D1 protein in symbionts, and induce the substantial reduction of symbiont density and chlorophyll content after heat stress to trigger the collapse of coral-Symbiodiniaceae symbiosis (Lesser, 1997; Downs et al., 2002; Hawkins et al., 2014). These results might further suggest the collapse of coral-Symbiodiniaceae symbiosis in the scleractinian coral P. damicornis, owing to excessive oxidative pressure after heat stress.
Meanwhile, the activities of GS, GDH, and GOGAT in the two symbiotic partners were determined to understand the involvement of ammonium assimilation in their acute heat stress response. In the present study, the activities of GS, GDH and GOGAT all increased significantly in the coral host P. damicornis, whereas its symbiont GS and GOGAT activities did not change significantly after heat stress. The results demonstrated that heat stress induced the ability of ammonium assimilation in the coral host, not the symbionts. The induction of GS expression by heat stress was also observed in the Pacific oyster Crassostrea gigas (Meistertzheim et al., 2007), and similar results were reported that elevated temperature did not change significantly the expression level of GS gene in the symbiont of the scleractinian coral P. damicornis (Hoadley et al., 2015). Therefore, the activity rises of the three enzymes related to ammonium assimilation could also result from the upregulation of their gene expression level in the coral host. Furthermore, the rises of ammonium assimilation ability revealed that coral host could need more inorganic nitrogen for the repair of heat damage and the acclimatization of high temperature. However, it was reported that heat stress decreased ammonium uptake of the scleractinian coral Stylophora pistillata (Godinot et al., 2011). Because symbiotic Symbiodiniaceae was reported to increase nitrogen availability from host coral under heat stress (Radecker et al., 2015; Cui et al., 2019), there was a contradiction among the increased nitrogen availability of symbionts, the increased ammonium assimilation ability and decreased ammonium uptake of coral host when suffering from high temperature. We speculated that the contradiction could be resolved by different expression and activity of ammonium transporter in the interface of coral/seawater and coral/symbiont interface, because ammonium transporter in thermotolerant symbiont Durusdinium trenchii was expressed differentially under heat stress (Bellantuono et al., 2019). However, more experimental evidences are needed to confirm the speculation and uncover the detailed mechanism in future study. Together, these results suggest that acute heat stress could induce the ability of ammonium assimilation in the coral host P. damicornis, which might be involved in the heat acclimation of the coral-Symbiodiniaceae symbiotic association of P. damicornis.
To further reveal the effect of ammonium assimilation on the coral-Symbiodiniaceae symbiosis under acute heat stress, the alteration of symbiont density and chlorophyll content were detected after the glufosinate (GS inhibitor) treatment to the scleractinian coral P. damicornis. The symbiont density and chlorophyll content decreased significantly after glufosinate treatment, which was earlier than that only under heat stress. It demonstrated that glufosinate treatment accelerated the collapse of coral-Symbiodiniaceae symbiosis after acute heat stress. Glufosinate is a GS inhibitor, and also functions in the scleractinian coral (Su et al., 2018). In the present study, glufosinate could repress the GS activities and corresponding ammonium assimilation ability in the coral host and symbionts, and caused inadequate acquisition of inorganic nitrogen resource for the symbiotic association and earlier collapse of the symbiosis under heat stress, which implied that available ammonium could contribute to the acclimatization of the symbiotic association to heat stress. This was also manifested in some observation that ammonium availability could reduce the negative effect of heat stress on scleractinian corals (Beraud et al., 2013; Zhou et al., 2017; Fernandes de Barros Marangoni et al., 2020). However, the significant decline of symbiont density was only observed at 6 h after the treatment, which could result from the compensation effect of GDH pathway in the coral host. We will explore the compensation effect and regulation mechanism in our further studies. To further understand the potential effect of ammonium assimilation under heat stress, we determined the total antioxidant capacity and caspase3 activation level in the coral host and symbiont apoptosis rate after glufosinate treatment. The total antioxidant capacity and caspase3 activation level of the coral host increased significantly, while symbiont apoptosis did not change significantly. It revealed that the repression of ammonium assimilation could facilitate the collapse of the coral-Symbiodiniaceae symbiosis through the induction of excessive oxidative pressure and apoptosis in the coral host after heat stress. All results imply that ammonium assimilation might be involved in the response and acclimatization of the coral-Symbiodiniaceae symbiosis to high temperature, and the effect of high temperate on scleractinian coral might depend on the nitrogen nutrient status of its symbiotic association.
Data Availability Statement
The raw data supporting the conclusions of this article will be made available by the authors, without undue reservation.
Ethics Statement
All animal-involving experiments of this study were approved by the Ethics Committee of Hainan University and local government.
Author Contributions
JT and ZZ conceived and designed the experiments. JT, XN, and JW performed the experiments. JT and ZZ analyzed the data. LW and JL contributed to the reagents, materials, and analysis tools. JT and ZZ contributed to the discussion and wrote the manuscript. All authors read and approved the final manuscript.
Funding
This research was supported by the National Key R&D Program of China (Grant 2018YFC1406504), the National Natural Science Foundation of China (Grants 31772460 and 42076145), the Major Science and Technology Program (ZDKJ2019011), and Natural Science Foundation of Hainan Province (2019RC067).
Conflict of Interest
The authors declare that the research was conducted in the absence of any commercial or financial relationships that could be construed as a potential conflict of interest.
Acknowledgments
The authors were grateful to all of the laboratory members for their continuous technical advice and helpful discussions.
References
Bellantuono, A. J., Dougan, K. E., Granados-Cifuentes, C., and Rodriguez-Lanetty, M. (2019). Free-living and symbiotic lifestyles of a thermotolerant coral endosymbiont display profoundly distinct transcriptomes under both stable and heat stress conditions. Mol. Ecol. 28, 5265–5281. doi: 10.1111/mec.15300
Beraud, E., Gevaert, F., Rottier, C., and Ferrier-Pages, C. (2013). The response of the scleractinian coral Turbinaria reniformis to thermal stress depends on the nitrogen status of the coral holobiont. J. Exp. Biol. 216, 2665–2674. doi: 10.1242/jeb.085183
Coles, S. L., and Brown, B. E. (2003). Coral bleaching–capacity for acclimatization and adaptation. Adv. Mar. Biol. 46, 183–223. doi: 10.1016/s0065-2881(03)46004-5
Cui, G., Liew, Y. J., Li, Y., Kharbatia, N., Zahran, N. I., Emwas, A. H., et al. (2019). Host-dependent nitrogen recycling as a mechanism of symbiont control in Aiptasia. PLoS Genet. 15:e1008189. doi: 10.1371/journal.pgen.1008189
Downs, C. A., Fauth, J. E., Halas, J. C., Dustan, P., Bemiss, J., and Woodley, C. M. (2002). Oxidative stress and seasonal coral bleaching. Free Radic. Biol. Med. 33, 533–543. doi: 10.1016/s0891-5849(02)00907-3
Dubinsky, Z., and Jokiel, P. L. (1994). Ratio of energy and nutrient fluxes regulates symbiosis between zooxanthellae and corals. Pacific Sci. 48, 313–324.
Fernandes de Barros Marangoni, L., Ferrier-Pages, C., Rottier, C., Bianchini, A., and Grover, R. (2020). Unravelling the different causes of nitrate and ammonium effects on coral bleaching. Sci. Rep. 10:11975.
Flores-Ramirez, L. A., and Linan-Cabello, M. A. (2007). Relationships among thermal stress, bleaching and oxidative damage in the hermatypic coral, Pocillopora capitata. Comp. Biochem. Physiol. C. Toxicol Pharmacol 146, 194–202. doi: 10.1016/j.cbpc.2006.09.008
Godinot, C., Houlbreque, F., Grover, R., and Ferrier-Pages, C. (2011). Coral uptake of inorganic phosphorus and nitrogen negatively affected by simultaneous changes in temperature and pH. PLoS One 6:e25024. doi: 10.1371/journal.pone.0025024
Hawkins, T. D., Krueger, T., Becker, S., Fisher, P. L., and Davy, S. K. (2014). Differential nitric oxide synthesis and host apoptotic events correlate with bleaching susceptibility in reef corals. Coral Reefs 33, 141–153. doi: 10.1007/s00338-013-1103-4
Hedouin, L. S., Wolf, R. E., Phillips, J., and Gates, R. D. (2016). Improving the ecological relevance of toxicity tests on scleractinian corals: influence of season, life stage, and seawater temperature. Environ. Pollut. 213, 240–253. doi: 10.1016/j.envpol.2016.01.086
Higuchi, T., Fujimura, H., Arakaki, T., and Oomori, T. (2008). “Activities of antioxidant enzymes (SOD and CAT) in the coral Galaxea fascicularis against increased hydrogen peroxide concentrations in seawater,” in Proceeding of the 11th International Coral Reef Symposium, Ft. Lauderdale, FLA.
Higuchi, T., Yuyama, I., and Nakamura, T. (2015). The combined effects of nitrate with high temperature and high light intensity on coral bleaching and antioxidant enzyme activities. Reg. Stud. Mar. Sci. 2, 27–31. doi: 10.1016/j.rsma.2015.08.012
Hoadley, K. D., Pettay, D. T., Grottoli, A. G., Cai, W. J., Melman, T. F., Schoepf, V., et al. (2015). Physiological response to elevated temperature and pCO2 varies across four Pacific coral species: understanding the unique host+symbiont response. Sci. Rep. 5:18371.
Hughes, T. P., Kerry, J. T., Alvarez-Noriega, M., Alvarez-Romero, J. G., Anderson, K. D., and Baird, A. H. (2017). Global warming and recurrent mass bleaching of corals. Nature 543, 373–377.
Jeffrey, S. W., and Humphrey, G. F. (1975). New spectrophotometric equations for determining chlorophylls a, b, c1 and c2 in higher plants, algae and natural phytoplankton. Biochem. Physiol. Pflanzen 167, 191–194. doi: 10.1016/s0015-3796(17)30778-3
Johannes, R. E., Coles, S. L., and Kuenzel, N. T. (1970). The role of zooplankton in the nutrition of some scleractinian Corals1. Limnol. Oceanogr. 15, 579–586. doi: 10.4319/lo.1970.15.4.0579
Lesser, M. P. (1997). Oxidative stress causes coral bleaching during exposure to elevated temperatures. Coral Reefs 16, 187–192. doi: 10.1007/s003380050073
Lesser, M. P. (2011). “Coral bleaching: causes and mechanisms,” in Coral Reefs: An Ecosystem in Transition, eds Z. Dubinsky and N. Stambler (Dordrecht: Springer Netherlands), 405–419. doi: 10.1007/978-94-007-0114-4_23
Levy, O., Achituv, Y., Yacobi, Y. Z., Stambler, N., and Dubinsky, Z. (2006). The impact of spectral composition and light periodicity on the activity of two antioxidant enzymes (SOD and CAT) in the coral Favia favus. J. Exp. Mar. Biol. Ecol. 328, 35–46. doi: 10.1016/j.jembe.2005.06.018
Liu, H., Stephens, T. G., Gonzalez-Pech, R. A., Beltran, V. H., Lapeyre, B., Bongaerts, P., et al. (2018). Symbiodinium genomes reveal adaptive evolution of functions related to coral-dinoflagellate symbiosis. Commun. Biol. 1:95.
Meistertzheim, A. L., Tanguy, A., Moraga, D., and Thebault, M. T. (2007). Identification of differentially expressed genes of the Pacific oyster Crassostrea gigas exposed to prolonged thermal stress. FEBS J. 274, 6392–6402. doi: 10.1111/j.1742-4658.2007.06156.x
Morris, L. A., Voolstra, C. R., Quigley, K. M., Bourne, D. G., and Bay, L. K. (2019). Nutrient availability and metabolism affect the stability of coral-symbiodiniaceae symbioses. Trends Microbiol. 27, 678–689. doi: 10.1016/j.tim.2019.03.004
Muscatine, L., and Porter, J. W. (1977). Reef corals: mutualistic symbioses adapted to nutrient-poor environments. BioScience 27, 454–460. doi: 10.2307/1297526
Peng, S. E., Chen, C. S., Song, Y. F., Huang, H. T., Jiang, P. L., Chen, W. N., et al. (2012). Assessment of metabolic modulation in free-living versus endosymbiotic Symbiodinium using synchrotron radiation-based infrared microspectroscopy. Biol. Lett. 8, 434–437. doi: 10.1098/rsbl.2011.0893
Pernice, M., Dunn, S. R., Miard, T., Dufour, S., Dove, S., and Hoegh-Guldberg, O. (2011). Regulation of apoptotic mediators reveals dynamic responses to thermal stress in the reef building coral Acropora millepora. PLoS One 6:e16095. doi: 10.1371/journal.pone.0016095
Pernice, M., Meibom, A., Van Den Heuvel, A., Kopp, C., Domart-Coulon, I., Hoegh-Guldberg, O., et al. (2012). A single-cell view of ammonium assimilation in coral-dinoflagellate symbiosis. ISME J. 6, 1314–1324. doi: 10.1038/ismej.2011.196
Plaisance, L., Caley, M. J., Brainard, R. E., and Knowlton, N. (2011). The diversity of coral reefs: what are we missing? PLoS One 6:e25026. doi: 10.1371/journal.pone.0025026
Radecker, N., Pogoreutz, C., Voolstra, C. R., Wiedenmann, J., and Wild, C. (2015). Nitrogen cycling in corals: the key to understanding holobiont functioning? Trends Microbiol. 23, 490–497. doi: 10.1016/j.tim.2015.03.008
Roberty, S., Beraud, E., Grover, R., and Ferrier-Pages, C. (2020). Coral productivity is Co-limited by bicarbonate and ammonium availability. Microorganisms 8:640. doi: 10.3390/microorganisms8050640
Roberty, S., Fransolet, D., Cardol, P., Plumier, J. C., and Franck, F. (2015). Imbalance between oxygen photoreduction and antioxidant capacities in Symbiodinium cells exposed to combined heat and high light stress. Coral Reefs 34, 1063–1073. doi: 10.1007/s00338-015-1328-5
Shinzato, C., Shoguchi, E., Kawashima, T., Hamada, M., Hisata, K., Tanaka, M., et al. (2011). Using the Acropora digitifera genome to understand coral responses to environmental change. Nature 476, 320–323. doi: 10.1038/nature10249
Su, Y., Zhou, Z., and Yu, X. (2018). Possible roles of glutamine synthetase in responding to environmental changes in a scleractinian coral. Mol. Biol. Rep. 45, 2115–2124. doi: 10.1007/s11033-018-4369-3
Teixeira, T., Diniz, M., Calado, R., and Rosa, R. (2013). Coral physiological adaptations to air exposure: heat shock and oxidative stress responses in Veretillum cynomorium. J. Exp. Mar. Biol. Ecol. 439, 35–41. doi: 10.1016/j.jembe.2012.10.010
Vidal-Dupiol, J., Ladriere, O., Destoumieux-Garzon, D., Sautiere, P. E., Meistertzheim, A. L., Tambutte, E., et al. (2011). Innate immune responses of a scleractinian coral to vibriosis. J. Biol. Chem. 286, 22688–22698. doi: 10.1074/jbc.m110.216358
Wall, C. B., Kaluhiokalani, M., Popp, B. N., Donahue, M. J., and Gates, R. D. (2020). Divergent symbiont communities determine the physiology and nutrition of a reef coral across a light-availability gradient. ISME J. 14, 945–958. doi: 10.1038/s41396-019-0570-1
Wein, T., Romero Picazo, D., Blow, F., Woehle, C., Jami, E., Reusch, T. B. H., et al. (2019). Currency, exchange, and inheritance in the evolution of symbiosis. Trends Microbiol. 27, 836–849. doi: 10.1016/j.tim.2019.05.010
Weis, V. M. (2008). Cellular mechanisms of Cnidarian bleaching: stress causes the collapse of symbiosis. J. Exp. Biol. 211, 3059–3066. doi: 10.1242/jeb.009597
Wooldridge, S. A. (2013). Breakdown of the coral-algae symbiosis: towards formalising a linkage between warm-water bleaching thresholds and the growth rate of the intracellular zooxanthellae. Biogeosciences 10, 1647–1658. doi: 10.5194/bg-10-1647-2013
Xiang, T., Lehnert, E., Jinkerson, R. E., Clowez, S., Kim, R. G., Denofrio, J. C., et al. (2020). Symbiont population control by host-symbiont metabolic interaction in Symbiodiniaceae-cnidarian associations. Nat. Commun. 11:108.
Yakovleva, I., Bhagooli, R., Takemura, A., and Hidaka, M. (2004). Differential susceptibility to oxidative stress of two scleractinian corals: antioxidant functioning of mycosporine-glycine. Comp. Biochem. Physiol. B. Biochem. Mol. Biol 139, 721–730. doi: 10.1016/j.cbpc.2004.08.016
Zhou, Z., Zhang, G., Chen, G., Ni, X., Guo, L., Yu, X., et al. (2017). Elevated ammonium reduces the negative effect of heat stress on the stony coral Pocillopora damicornis. Mar. Pollut. Bull. 118, 319–327. doi: 10.1016/j.marpolbul.2017.03.018
Keywords: nitrogen nutrient, nitrogen limitation, heat acclimation, symbiosis breakdown, scleractinian coral
Citation: Tang J, Ni X, Wen J, Wang L, Luo J and Zhou Z (2020) Increased Ammonium Assimilation Activity in the Scleractinian Coral Pocillopora damicornis but Not Its Symbiont After Acute Heat Stress. Front. Mar. Sci. 7:565068. doi: 10.3389/fmars.2020.565068
Received: 23 May 2020; Accepted: 11 September 2020;
Published: 29 September 2020.
Edited by:
Hajime Kayanne, The University of Tokyo, JapanReviewed by:
Daniel Aagren Nielsen, University of Technology Sydney, AustraliaAnthony William Larkum, University of Technology Sydney, Australia
Copyright © 2020 Tang, Ni, Wen, Wang, Luo and Zhou. This is an open-access article distributed under the terms of the Creative Commons Attribution License (CC BY). The use, distribution or reproduction in other forums is permitted, provided the original author(s) and the copyright owner(s) are credited and that the original publication in this journal is cited, in accordance with accepted academic practice. No use, distribution or reproduction is permitted which does not comply with these terms.
*Correspondence: Zhi Zhou, zhouzhi@hainanu.edu.cn