- 1College of Sciences and Engineering, Institute for Marine and Antarctic Studies, University of Tasmania, Hobart, TAS, Australia
- 2IMOS Animal Tracking Facility – Satellite Tagging, Sydney Institute of Marine Science, Mosman, NSW, Australia
- 3Department of Biological Sciences, Faculty of Science and Engineering, Macquarie University, Sydney, NSW, Australia
- 4Centre d’Études Biologiques de Chizé, UMR 7372 CNRS- La Rochelle Université, Villiers-en-Bois, France
- 5Australian Antarctic Division, Kingston, TAS, Australia
Identifying factors influencing survivorship is key to understanding population persistence. Although satellite telemetry is a powerful tool for studying remote animal ecology and behaviour it is rarely used for demographic studies because distinguishing the death of the animal (individual mortality) from failure of the tag (mechanical tag failure) has proven difficult. Southern elephant seals present an opportunity to separate tag failure from animal mortality thanks to the availability of large tracking datasets, broad knowledge of demographic rates, and because for these large animals, satellite tags are known not to influence mortality rates. A key rationale for investigating satellite telemetry to estimate mortality as compared to using traditional Capture-Mark-Recapture methods is the potential for obtaining spatially and temporally specific information, particularly while the animals are at sea and largely unobservable. We used satellite tag data from 182 seals from Isles Kerguelen, deployed between 2004 and 2018. Of these, 76 (42%) tags transmitted for the full post-moult foraging trip (max. 265 days for females and max. 305 days for sub-adult males) with the remaining 107 tags (58%) ceasing transmission at sea. We found that contrary to expectations, behavioural choices seem not to influence tag failure rates by mechanical means, rather the signals we detected seemed to align with previously described variation in mortality between groups. There was evidence, albeit limited, for an increase in tag failure for adult females in years with negative Southern Annular Mode (lower Southern Ocean productivity). We speculate that this increase in failure may suggest higher mortality in these years. Also, males using the Kerguelen Plateau had higher tag failure rates than those in the sea-ice zone, perhaps indicative of higher mortality. We suspect that these differences in tag failure rates between groups reflect variation in predator exposure and foraging success. This suggests satellite telemetry could be used to infer mortality events for southern elephant seals while they are at sea.
Introduction
Knowing when and why animals die provides key insights into the processes that shape populations. Capture-Mark-Recapture (CMR) approaches provide excellent information on time structured mortality, most often at annual scales (Lebreton et al., 1992). Finer scale temporal estimates of mortality are more challenging due to the need to regularly monitor a population’s status which is especially difficult for animals that perform long migrations into remote regions. Tracking studies, however, have the potential to quantify individual animal mortality even in remote regions (Hays et al., 2016). For instance, tracking studies were used to estimate mortality in three raptor species by quantifying how animal migration routes and behaviour affected individual survival and then calculating the effect of these factors on population growth rates (Klaassen et al., 2016). Tracking data also showed how the cumulative influence of multiple stressors reduced the rate of successful migration for the sockeye salmon (Oncorhynchus nerka) (Naughton et al., 2005). The main obstacle in the use of tracking data to estimate mortality rates is that even in the absence of catastrophic battery failure (Smith et al., 2018), an unknown proportion of tags will stop transmitting simply due to failure of the tag itself, which can be as high as 25%, at least in terrestrial studies (Hofman et al., 2019).
Southern elephant seals provide an opportunity to investigate whether high spatially and temporally resolved at-sea mortality information can be inferred from satellite tracking data due to the availability of large multi-year tracking datasets (Hindell et al., 2016), broad knowledge of demographic rates (Le Boeuf and Laws, 1994; McMahon et al., 2005), and the knowledge that the deployment of tags does not in itself, affect survival (McMahon et al., 2008). Southern elephant seals are meso-predators that range widely across the Southern Ocean (Hindell et al., 2016). They have two annual haul-outs when they come ashore on sub-Antarctic Islands, coastal South America, or continental Antarctica, to breed and moult. Most populations exhibited significant declines from the 1950s, with some now stabilising or growing (Hindell et al., 2016). The Kerguelen southern elephant seal population is now growing after a 20-year stabilisation period (Authier et al., 2011; Guinet pers. comm) although current adult survival estimates are not known for this population.
As is the case for many long-lived species, adult southern elephant seals have low annual mortality [approximately 21% (Pistorius et al., 2004, 2008)]. At-sea mortality is thought to be largely due to starvation (Pistorius et al., 2011) dictated by an individual’s ability to forage successfully, or to predation (Guinet et al., 1999). Climate-driven inter-annual variation on the availability of prey has a measurable effect on annual survival rates and reproductive output (van den Hoff et al., 2014; Oosthuizen et al., 2015; Clausius et al., 2017; McMahon et al., 2017, 2015) and we may see this reflected in tag failure rates. Long-term changes to Southern Ocean foraging conditions are linked to population trends (Hindell et al., 2017), so identifying when and where southern elephant seals die during their annual life cycle would provide valuable insight into the factors that influence mortality.
The primary aim of this study was to test whether satellite telemetry could be used to detect high spatially and temporally resolved at-sea mortality for southern elephant seals. To achieve this, we; (i) model tag failure rates, (ii) quantify the role of potential mechanical factors (such as exposure to ice, high pressure, or contact with the benthos) to the tag failure rate and (iii) assess the role of other, biological factors, in tag failure rates. We use the relationships between the factors assessed and tag failure rates to infer patterns of at-sea mortality.
Materials and Methods
Data Analysis
Over 14-years (2004–2018) 182 southern elephant seals were captured and sedated (McMahon et al., 2000) on sub-Antarctic Kerguelen Island toward the end of their post-moult haul-out. The same SMRU-SRDL tag type and attachment methods were used each year. Once attached, tags remain attached to the animal until the moult in the following season and given that the few tags that are recovered all remain securely fixed to moulted hair, there is no reason to believe they fall off during deployments (Guinet pers. comm.). Details on the instruments, capture, attachment and recovery techniques (Boehme et al., 2009; Field et al., 2012), and the negligible effects these have on animal growth, reproduction and survival can be found elsewhere (McMahon et al., 2008; Mazzaro and Dunn, 2009).
Data from the tag is transmitted via the ARGOS satellite network, relaying location and behavioural information (Myers et al., 2006). Location estimates were filtered using a state-space model with a correlated random walk and a 6-h time step (using R package BSAM), for details see Jonsen et al. (2019). For each seal position, we attributed a bathymetric depth, based on the ETPOPO1 bathymetric data set,1 and a temporally explicit sea ice concentration at that location on that day using the blended optimal interpolation Version 2 ECDC AMSR + AVHRR 0.25-degree global ice charts from the NASA Coastwatch site.2 Only tracks which extended beyond a 30 km radius of the deployment location were included in this study, to remove seals from the analysis which did not begin a foraging trip.
There were two possible outcomes for each tag; either, it continued to transmit until it returned to land after the foraging trip (censored), or transmissions terminated at sea (failed). Transmission duration was calculated as the time from the start of the trip (in water locations) to returning to breed (females) or moult (males) for censored tags, and until the last received at-sea transmission for failed tags.
Transmission Rate and Surface Duration
These tags are expected to produce a total of 50,000 transmission attempts before they succumb to battery exhaustion (SMRU Instrumentation). We investigated the daily transmission rate to determine if rates differed between sex and/or outcome (failed or censored) perhaps indicating some tags were malfunctioning and transmitting incorrectly. The total number of transmissions (TX_NUMBER) made by the tag (irrespective of being received by the ARGOS satellite network) was recorded and periodically transmitted during deployment. As the TX_NUMBER can reset (i.e., the tag resetting itself in response to an error), the mean daily transmission rate was calculated as the change in the max TX_NUMBER for each seal from 1 day to the next.
Correctly functioning tags only transmit while the seal is at the sea surface, and so surfacing behaviour could be linked to the transmission rate. Surface duration is transmitted by the tag at the completion of dives, and we used this to calculate the mean surface duration per day (individually for each seal). A Linear Mixed-Effects (LME) model (Lindstrom and Bates, 1988) with a random variable (individual seals) and CorAR1 autocorrelation term was used to determine whether failed tags had; (i) higher daily transmission rates or (ii) longer mean daily surface duration [lme(surface duration/transmission rate ∼ outcome (failed or censored) ∗ sex, random = ∼1| individual seal, corr = corAR1(), data = d)]. The model was simplified based on results from likelihood model selection using function dredge().
Modelling Tag Failure
Survival models are commonly used to estimate the effectiveness of treatments and the risk of behavioural choices in human health studies (Collett, 2015). For our study, we used Cox Proportional Hazard (CPH) models (a type of survival model) as they do not assume a specific hazard function and are thus robust to hazards typically encountered by wildlife populations (Murray, 2006). We used R packages: survival (Singer and Willett, 1991) and timereg (Martinussen and Scheike, 2007). A null CPH model [coxph(transmission duration (days), outcome) ∼ 1, data] was used to define the failure rate (a comparison of track duration, Figure 3). We assumed all individuals in each sex class had equal probabilities of mortality when they left for their winter foraging trip. Individuals were chosen for tagging based on a pseudorandom approach so there is no a priori reason to assume that tagged animals differed from the population as a whole (Boehme et al., 2009; Field et al., 2012; McMahon et al., 2008).
Mechanical Factors That May Influence Tag Failure
Three a priori factors were identified as potentially influencing tag failure rates; exposure to sea-ice (aerial abrasion), high pressures (damage to sensors and housings), or impacting the benthos (aerial abrasion) (Table 1). For each factor, individual seals were assigned as either having (a) increased or (b) reduced exposure to the hazard. Increased exposure to sea-ice and the benthos is proposed to heighten the risk of aerial damage, potentially preventing tag transmissions. Sea-ice is also an indicator for cooler ambient temperatures potentially reducing battery life. We also investigated whether repeated exposure to high pressures at depth could potentially cause cracks to the tag casings and/or force water ingress leading ultimately to electronic failure (Note, this has not been recorded in any recovered tags; Guinet pers. comm.).
Environmental Influences on Seal Mortality
The Southern Annular Mode (SAM) is a large-scale climatic index (Lovenduski and Gruber, 2005) which influences variability in annual foraging success of a suite of Southern Ocean marine predators (Trathan et al., 2007; Bost et al., 2015), including southern elephant seals (Oosthuizen et al., 2011). For southern elephant seals, female fecundity (van den Hoff et al., 2014), pup survival (Clausius et al., 2017), and pup weaning mass (McMahon et al., 2015, 2017; Oosthuizen et al., 2015) are all positively correlated with the SAM. Accordingly, if the failure rate differs between years of contrasting SAM, and we assume mechanical tag failure rate is independent of SAM, these differences could be attributed to a difference in relative at-sea mortality among years (Table 1).
Because males and females differ in both foraging strategies and haul-out routines (Le Boeuf and Laws, 1994; Bailleul et al., 2007; Hindell et al., 2016), we constructed separate CPH survival models for tags worn by males and female seals including, pressure + ice + benthos + SAM as fixed effects (SAM data accessed at http://www.nerc-bas.ac.uk/icd/gjma/sam.html). To test whether our variables complied with the multiplicative hazard assumption of the CPHs model, we used a scaled Schoenfeld residuals test [cox.zph(model fit)] (Therneau and Grambsch, 2000).
Results
Locations of At-Sea Tag Failure
A total of 182 individual tag deployments were included in the analysis. Seventy-six (42%) of these were censored, (i.e., they transmitted data throughout the deployment period) while the remaining 107 (58%) failed at sea. For our study, sub-adult male seals (n = 75) predominantly used shelf areas on the Kerguelen Plateau (exposure to benthos) or the Antarctic continental shelf (exposure to the benthos and sea-ice). In contrast, female seals (n = 107) predominantly used oceanic regions and performed more deep dives resulting in greater exposure to high pressures (Figure 1). The locations of the final transmission of failed tags did not appear to be associated with sea-ice extent (exposure to ice, purple frequency distribution), exposure to the benthos (blue locations) or high pressures (green locations, Figure 1). There however did appear to be a cluster of failed locations around the Kerguelen Plateau (−49°S, 69°E) for males (blue locations, Figure 1).
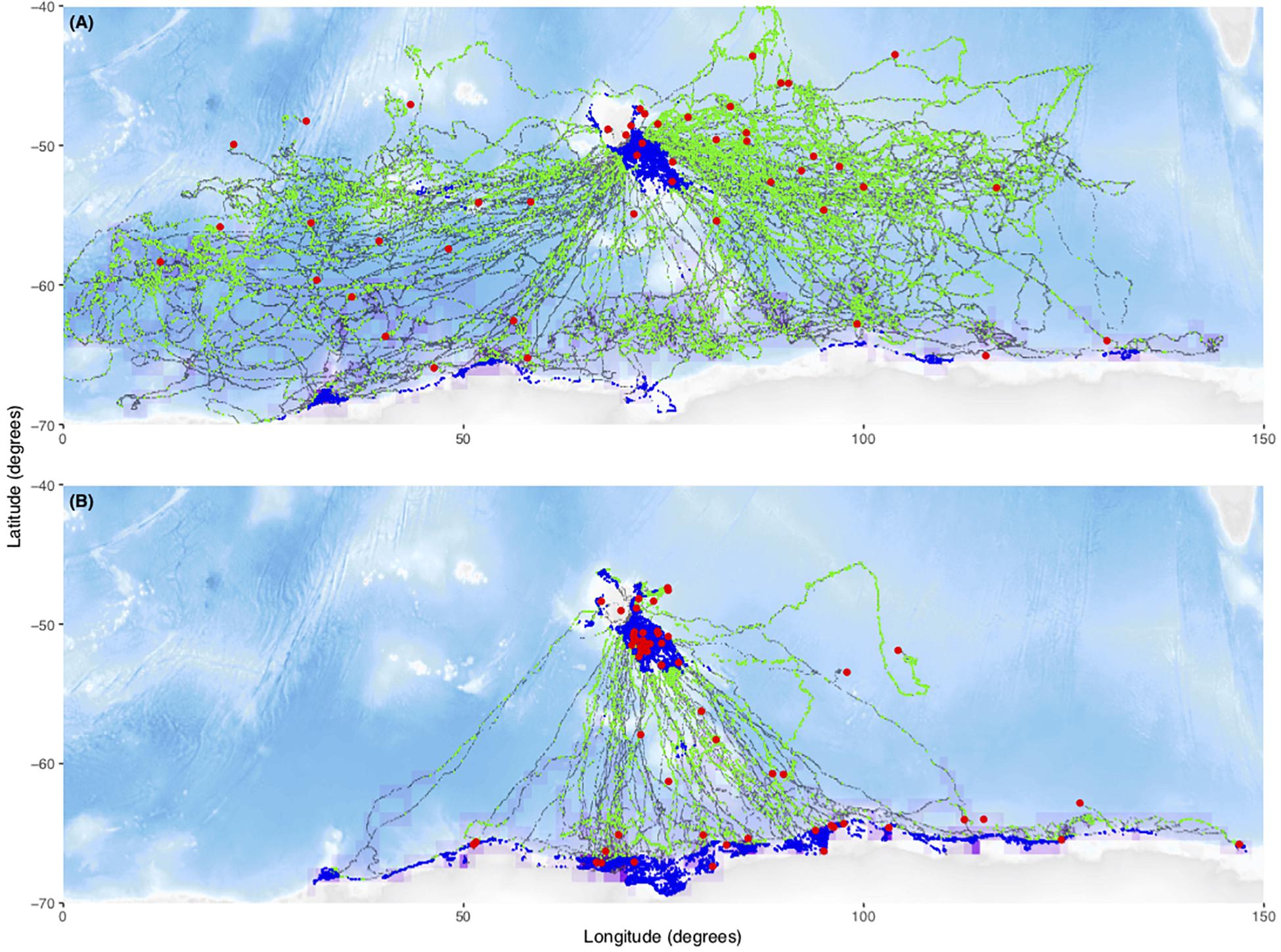
Figure 1. Plot of all tracks faceted by sex. Male movements were concentrated in the Kerguelen and Antarctic shelf regions (B) while female movements were focussed in pelagic regions (A). Green locations are deep dives (90% quantile of max dive depths) (exposure to high pressure) and blue locations are dives on the shelf (>–1000 m bathymetry) (exposure to the benthos). Coarse-scale frequency distribution of locations interacting with sea-ice (>15% concentration) (purple) and fail locations are plotted (red). Fail locations seemed to cluster around areas of high use, not potential tag hazards, with the possible exception of males foraging on the Kerguelen Plateau (–49°S, 69°E). Black locations are dives without increased exposure to proposed mechanical tag failure mechanisms. The final locations of censored tracks have been omitted.
Transmission Rate and Surface Duration
The mean number of transmissions per day was higher (LME: d.f. = 167, p = 0.003) for tags which failed (Figure 2A). However, failed tags had the same mean surface durations as the censored tags (d.f. = 169, p = 0.713, Figure 2B). No significant relationship was found for sex or the interaction term (sex:outcome) in either transmission rate (p = 0.223, 0.535, respectively) or surface duration (p = 0.075, 0.060, respectively). We are confident the interaction term (sex:outcome) is not inhibiting interpretation of these results, as the dataset is large, there was no strong evidence for an interaction term contributing to model fit and p-values remained high (p ≥ 0.06). Testing of the residuals suggested assumptions of normal distribution were met.
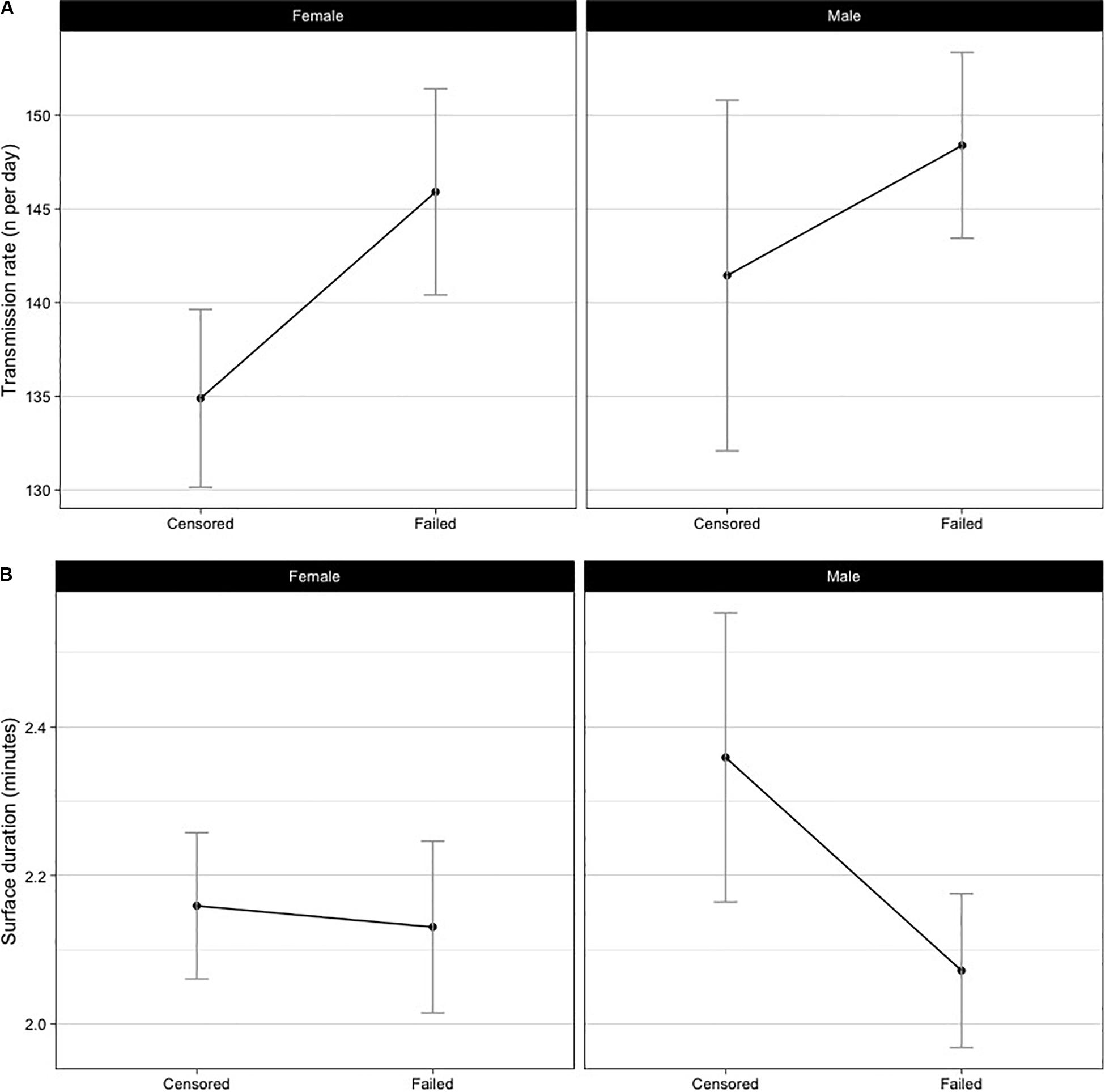
Figure 2. Sex and outcome (failed or censored) specific mean daily transmission rates (A) and surface durations (B). Failed tags had more transmissions per day (d.f. = 169, p = 0.003), but there were no differences in the surface durations (d.f. = 169, p = 0.5502).
Tag Failure Rates
The failure rate was initially constant for the first 200 days at 0.2% of units per day (Figure 3A). There was a cluster of censored tracks in association with the median female breeding haul-out at 236 days at sea (solid vertical line, Figure 3A). After this, the tag failure rate of the remaining tags (mostly sub-adult males) increased rapidly (Figure 3A) [and the associated cumulative hazard increased exponentially (Figure 3B)]. The mean failure rate between 200 days and the final transmitting tag at 305 days was 0.34% units per day (Figure 3A). The final remaining transmitting tag stopped transmitting at 265 days for females (red vertical line) and 305 days for sub-adult males (blue vertical line, Figure 3A).
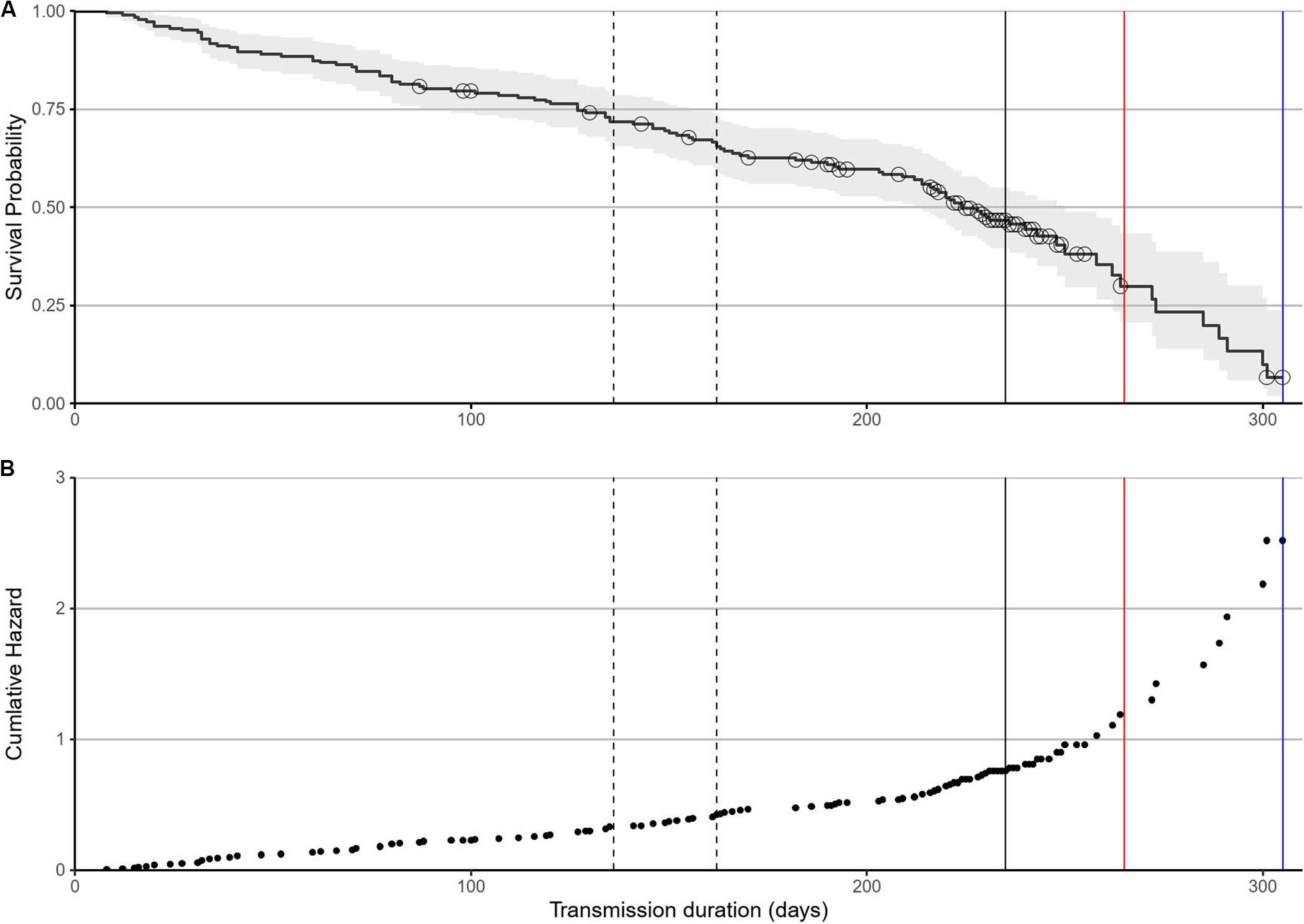
Figure 3. Failure rate determined by a CPH survival model. (A) Tag failure rates for the first 200 days were constant over time, with no association with haul-outs when tags were likely to be damaged. The median male first haul-out was at 136 days with median departure at 162 days (dashed lines). The median female breeding haul-out (solid line) was at 236 days. The range of time adjusted mortality for males (blue line) and females (red line) are plotted. Black circles are censored tracks and grey areas represent 95% confidence intervals. (B) The associated cumulative hazard function remained constant before accelerating after 200 days.
The Influence of Behavioural Choices and Environmental Variables on Tag Failure Rates
Increased exposure to the high pressure had little effect on tag failure for either male or female seals (Table 2). Increased exposure to ice was significant for sub-adult males and suggested this behaviour reduced tag failure rates. Specifically, sub-adult male seals that foraged in ice areas (exposure to ice) i.e., south of ≈60°, predominantly in Antarctic waters (Figure 1) (n = 38) had reduced tag failure rates with a 0.36 multiplicative reduction in the hazard (Table 2), compared to those foraging in ice-free areas mainly on the Kerguelen Plateau (Figure 1). Similarly, sub-adult males which had a high percentage of benthic dives (exposure to the benthos, Table 1) (n = 36) had reduced tag failure rates compared to those making fewer benthic dives with a 0.38 multiplicative reduction in the hazard (Table 2). Assumption testing suggested the multiplicative hazard assumption was met (Schoenfeld: d.f. 1–4, p > 0.18).
For females, tag failure was lower during positive SAM seasons (Figure 4) when foraging conditions are better (Lovenduski and Gruber, 2005) (0.41 multiplicative reduction in the hazard). Tag failure rates of the positive and negative SAM groups separated early in the winter foraging trip and continued to diverge until the final tag terminated at 265 days (Figure 5). However, the 95% confidence intervals remained wide (16–63%) encompassing the positive SAM tag survival estimate of 59% (Figure 5B). The SAM variable was not significant for males. Assumption testing suggested the multiplicative hazard assumption was met (Schoenfeld: d.f. 1–4, p > 0.17) except for exposure to depth variable for female seals (Schoenfeld: d.f. 1, p > 0.001). Graphical representation showed residuals plotted around zero with no discernible pattern with time.
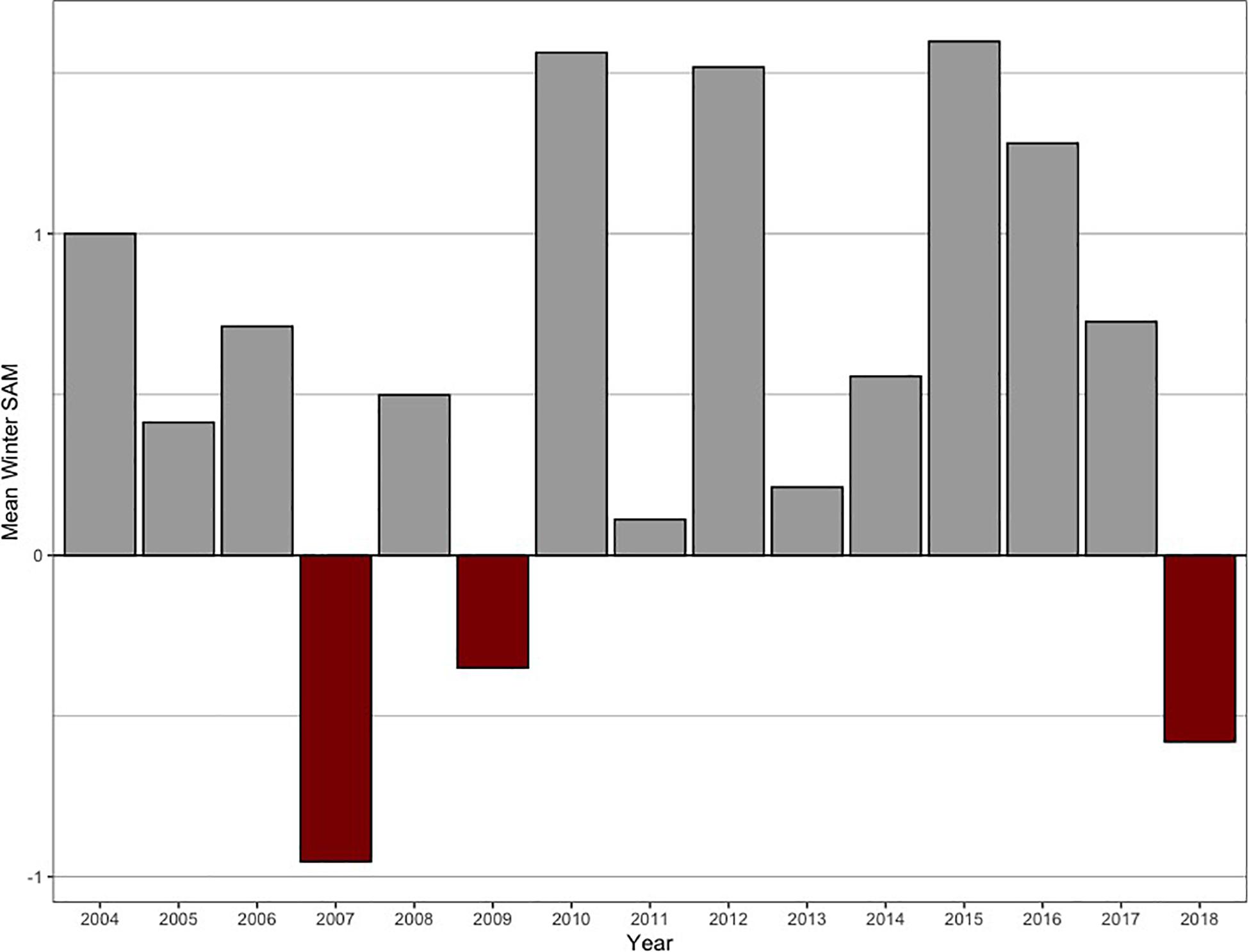
Figure 4. Mean winter SAM index during the study period (2004–2018). For the mean winter foraging phase (March–August), the SAM index was mostly in its positive phase (136 tags deployed), with 2007, 2009, and 2018 contributing to the negative SAM years (47 tags deployed).
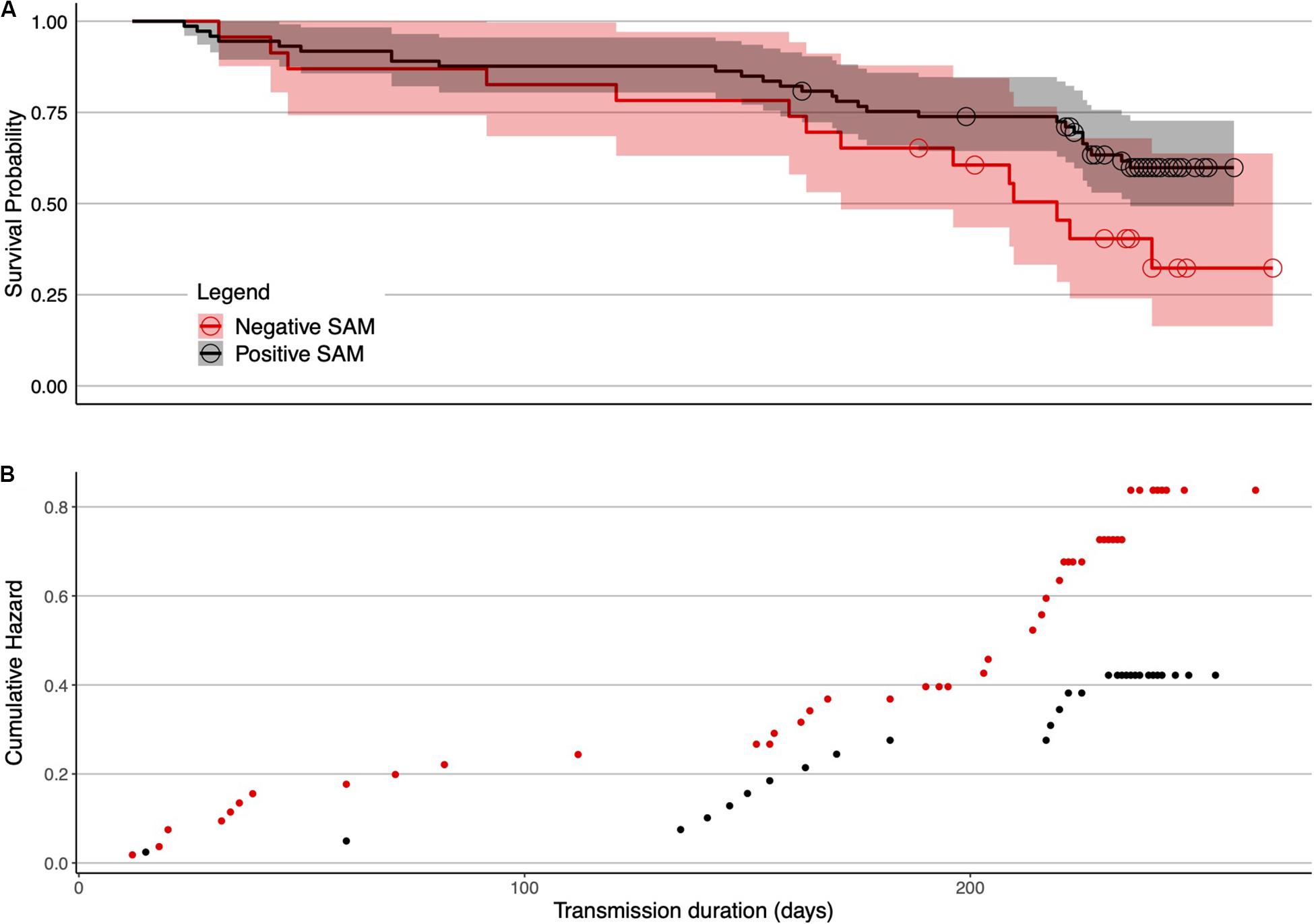
Figure 5. Female SAM survival curves in isolation of other non-significant factors. (A) Deployments in positive SAM years were associated with reduced tag failure probabilities in females. Separation of tag failure probabilities between the two groups was evident, beginning around 70 days. For females, there were 78 deployments made in positive SAM years (2004–2006, 2008, and 2010–2017), and 29 in the remaining negative SAM years (2007, 2009, and 2018). Shaded areas are 95% confidence intervals. The hazards plot (B) suggests an increased hazard associated with seals deployed in negative SAM years (red points).
Discussion
Mechanical Tag Failure
We found a tag failure rate of 40% for the first 200 days of deployment which increased to 70% at day 265 (adult female breeding) and 90% at day 305 (sub-adult male moult). We found no evidence that seal behavioural choices increased mechanical causes of tag failure. We also found no evidence in the published literature nor in the reported occurrences of recovered elephant seal tags showing signs of physical damage or detrimental fouling (Reisinger and Bester, 2010a,b; McIntyre et al., 2011; Field et al., 2012). Consequently, the daily tag failure rate of one unit failing every 7–8 days per 100 tags deployed before 200 days may at least in part be due to intrinsic software or hardware failure.
After 200 days of deployment, daily failure rates increased dramatically with an additional 30% tag failure observed over a period of only 65 days. Hanson et al. (2013) observed a similar pattern, with a dramatic decrease in harbour seal (Phoca vitulina) “tag survival” after 250 days, which they attributed to failure of the tag. This may be due to increasing battery malfunction as the tags reach the end of their functional life. We used transmission rates as a proxy for power consumption of the tag and found evidence that failed tags produced, on average, more transmissions per day, thereby using more power than those that were censored. This relationship held for both sexes but was not related to surface durations associated with diving behaviour. This suggests that failed tags may have malfunctioned, e.g., transmitting below the sea surface or at a slightly higher rate than the predefined 45 s repetition rate, causing excessive power consumption and premature tag failure while at sea. Similarly, Hofman et al. (2019) found that for some terrestrial deployments, failed tags transmitted more often than programmed and consequently drained their batteries leading to early termination. The conditions tags are exposed to while attached to wild animals are far different to those experienced under laboratory conditions, and for southern elephant seals, this may significantly reduce the tag’s functional lives. Further analysis of the ultimate fate of deployed tags would be aided by the inclusions of battery voltage in diagnostic data and the advancement of technology to allow tags to record the transmissions that have been successfully received by the satellite array.
Few other studies have quantified tag failure rates, and more work is needed to draw generalised conclusions. Satellite tags deployed on northern fur seal pups (Callorhinus ursinus) showed a tag failure rate of 7% (tag type and transmission duration not specified) (Lea et al., 2009). For marine turtles, tag failure rates were estimated to be 78–100% (transmission duration range = 83–486 days, n = 14), largely due to fouling of the saltwater switch (Hays et al., 2007). However, fouling is almost non-existent in polar deployments, especially with long, deep diving predator such as southern elephant seals (Reisinger and Bester, 2010a,b; McIntyre et al., 2011), as cooler water temperatures and limited surface duration inhibits algae growth (Hays et al., 2007). A review of 167 satellite tag deployments on 62 terrestrial species estimated a tag failure rate of 25% (Hofman et al., 2019). It’s difficult to make further comparisons between these studies and ours, as physical realms, the tag type, species and the objectives vary.
Mortality rates for female southern elephant seals are known to be higher during post-breeding foraging trips compared to post-moult foraging trips (the focus of this study) due to the demands of reproduction (Pistorius et al., 2008). It is not known if sub-adult males experience a strong fluctuation in mortality during the year. The accelerated rate of tag failure at ≈200 days could be attributed to changes in mortality. Mortality is unlikely to be constant across the foraging trip, and a higher rate may potentially be associated with the return to haul-out locations, for two reasons. First, it’s possible that seals which have not foraged successfully die on the energetically expensive return journey (Cox et al., 2020). Alternatively, seals may be exposed to high rates of predation as they approach haul-out locations (Isles Kerguelen) (Van Den Hoff and Morrice, 2008; Tixier et al., 2018; Cox et al., 2020). The role of mortality and predation is further discussed below.
Role of Habitat and Behaviour
The presence of sea-ice has in the past been linked to tag failure in Weddell seals as the aerials become damaged (Andrews-Goff et al., 2010). Our results suggest this is not the case for southern elephant seals. Rather, sub-adult males which foraged in ice-covered areas had lower tag failure rates than those on sub-adult males using the Kerguelen Plateau. The Kerguelen Plateau is an area of high productivity and known foraging focus for about 50% of sub-adult males (Authier et al., 2012a). However, this may also be an area of increased mortality (Authier et al., 2012a,b; Chaigne et al., 2013). As they age, the proportion of males foraging on sub-Antarctic prey (on the Kerguelen Plateau) decreases, while the proportion of male foraging further south on Antarctic prey increases (Authier et al., 2012a,b; Chaigne et al., 2013). At 4 years, according to their stable isotope signature, 70% of sub-adult males were assigned to sub-Antarctic foraging habitat and 30% to Antarctic foraging habitat. This proportion switches (35% sub-Antarctic, 65% Antarctic) by the age of 10 (Chaigne et al., 2013), despite the fact that teeth δ13C signatures reveal that male southern elephant seals became faithful to their foraging habitat by the age of 4−5 (Martin et al., 2011; Authier et al., 2012a,b). These changes are interpreted as a differential survival probability between the seals foraging in sub-Antarctic waters (exhibiting higher mortality rates), compared to those foraging in Antarctic waters (Authier et al., 2012b; Chaigne et al., 2013). A 20% difference in annual survival rate between these two foraging habitats would lead to this observed change in the proportion of male southern elephant seals using sub-Antarctic (Kerguelen Plateau), versus Antarctic shelf foraging habitat. The simultaneous failure of both tags for 9 of 20 double-tagged yearling elephant seals on the Kerguelen Plateau may further indicate a higher rate of predation events (Orgeret et al., 2019; Cox et al., 2020). The Kerguelen Plateau is home to elephant seal predators such as sleeper sharks and killer whales (Orcinus orca) (Van Den Hoff and Morrice, 2008; Tixier et al., 2018; Cox et al., 2020). Therefore, the cluster of failures (Figure 1B) and higher failure rate of ice-free foraging individuals (Table 2) may indicate higher mortality due to predation and/or lower foraging opportunities on the Kerguelen Plateau. Note, however, that these factors are convoluted and often confounded, and signals may be masked by the interplay of variables. For example, a slight increase in tag failure associated with foraging in sea-ice may be masked by a larger increase in survival probabilities associated with Antarctic foraging. It is impossible to know conclusively if individuals successfully returned to Isles Kerguelen as there is no regular systematic search for flipper bands.
Sub-adult males which performed a high portion of dives over shelf waters (>200 m deep, in <−1000 bathymetry, Table 1) had reduced tag failure probabilities, which suggests that this behaviour may also reduce risk of mortality. It is well known that population trajectories are intimately linked to foraging success in southern elephant seals (Hindell et al., 2017), and that shelf habitats provide a highly productive habitat on which to forage, particularly for sub-adult males (Labrousse et al., 2018). We speculate that reduced tag failure in association with lower probable foraging effort in shelf habitats may be due to reduced mortality. This work represents an exciting first step in the detection of at-sea mortality for southern elephant seals, and our findings align with much of the current knowledge of the species.
The SAM is the largest driver of inter-season and inter-annual variation in the Southern Ocean (Thompson and Wallace, 2000). The SAM influences the strength of the westerly winds that drive ocean circulation which in turn influences the biogeochemical cycling and phytoplankton abundance (Lovenduski and Gruber, 2005) and the foraging behaviour and success of higher trophic levels (Bost et al., 2015). The downstream demographic effects of reduced resource availability in negative SAM years for Southern Ocean predators is well documented (Proffitt et al., 2007; Trathan et al., 2007; Forcada and Trathan, 2009; van den Hoff et al., 2014). Starvation is thought to be an important source of at-sea mortality for southern elephant seals (Pistorius et al., 2011) and our results suggest that reduced foraging success in relatively low SAM years may increase female mortality rates. This may be further investigated in future studies using at-sea body condition metrics (Biuw et al., 2003).
Low annual adult mortality rates characterise long-lived species, as individuals minimise the cost of reproduction to optimise lifetime reproductive output (Desprez et al., 2018). This is true for southern elephant seals where maternal resource allocation (McMahon et al., 2017) and reproductive output (van den Hoff et al., 2014; McMahon et al., 2015; Oosthuizen et al., 2015; Clausius et al., 2017) are compromised by poor foraging conditions. The cost of reproduction to females is high, and they lose an average of 35% of their mass over the 24-day lactation period (Fedak et al., 1996). The ability of females to subsequently recover these resources will be challenged if available foraging resources are limited (as in years of negative SAM) which may cause high rates of mortality in these years. We suggest that increased tag failure may be indicative of increased mortality in negative SAM years for females, although the high confidence intervals overlapped (Figure 5).
An accompanying CMR dataset of the Isles Kerguelen southern elephant seal population during the study period would provide further valuable insights into tag failure and mortality. Research using bespoke surgically implanted mortality tags (LHX) has revealed evidence of at-sea mortality (Horning and Mellish, 2014). However, this technique is not feasible in many situations, necessitating surgery in remote areas, and may in itself influence subsequent survival (Walker et al., 2009; Horning et al., 2019). By contrast, the approach undertaken here has no measurable effect on short-term seal health or long-term seal survival and is value adding to existing and ongoing long-term studies of seals and their environment (McMahon et al., 2008; Mazzaro and Dunn, 2009; Harcourt et al., 2019).
Adult survival of southern elephant seals is strongly linked to population trends (Pistorius et al., 2004, 2005, 2011), which are in turn linked to changes in habitat characteristics (Hindell et al., 2016, 2017). Currently, the few ongoing CMR studies (e.g., Marion Island) provide highly accurate annual survival information. However, southern elephant seal mortality fluctuates across their annual cycle (Pistorius et al., 2008), which CMR studies cannot quantify in migratory marine mammals. Future studies that combine this work with CMR data of the same population may provide further insights into spatial and temporal patterns of mortality, thereby clarifying the drivers of southern elephant seal population trends and deepening our understanding of the processes that shape the productivity of the Southern Ocean.
Data Availability Statement
The seal data are available on the IMOS Australian Ocean Data Network (AODN) portal at: https://portal.aodn.org.au/search?uuid=06b09398-d3d0-47dc-a54a-a745319fbece.
Ethics Statement
The study was carried out under approval from and in compliance of the Australian Antarctic Programme Animal Ethics Committee for AAS project 4344.
Author Contributions
MH, CM, CG, and RH conducted the data collection. BP collected the data. MH, CM, and SW conceived the study. AH analysed and interpreted the data under the guidance of MH, CM, and SW. AH wrote manuscript with guidance from MH and CM. SW, CG, BP and RH reviewed the manuscript. All authors contributed to the article and approved the submitted version.
Funding
This work was supported by the French Polar Institute (programme 109: PI. H. Weimerskirch and 1201: PI. C. Gilbert and CG), the SNO-MEMO and CNES-TOSCA. In collaboration with The Integrated Marine Observing System. Australia’s Integrated Marine Observing System (IMOS) is enabled by the National Collaborative Research Infrastructure Strategy (NCRIS). It is operated by a consortium of institutions as an unincorporated joint venture, with the University of Tasmania as Lead Agent.
Conflict of Interest
The authors declare that the research was conducted in the absence of any commercial or financial relationships that could be construed as a potential conflict of interest.
Acknowledgments
We wish to thank all the people who helped with tag deployment in the field.
Footnotes
References
Andrews-Goff, V., Hindell, M., Field, I., Wheatley, K., and Charrassin, J. (2010). Factors influencing the winter haulout behaviour of Weddell seals: consequences for satellite telemetry. Endanger Species Res. 10, 83–92. doi: 10.3354/esr00257
Authier, M., Bentaleb, I., Ponchon, A., Martin, C., and Guinet, C. (2012a). Foraging fidelity as a recipe for a long life: foraging strategy and longevity in male southern elephant seals. PLoS One 7:e32026. doi: 10.1371/journal.pone.0032026
Authier, M., Delord, K., and Guinet, C. (2011). Population trends of female elephant seals breeding on the Courbet Peninsula, îles Kerguelen. Polar Boil 34, 319–328. doi: 10.1007/s00300-010-0881-1
Authier, M., Martin, C., Ponchon, A., Steelandt, S., Bentaleb, I., and Guinet, C. (2012b). Breaking the sticks: a hierarchical change−point model for estimating ontogenetic shifts with stable isotope data. Methods Ecol. Evol. 3, 281–290. doi: 10.1111/j.2041-210x.2011.00162.x
Bailleul, F., Charrassin, J.-B., Ezraty, R., Girard-Ardhuin, F., McMahon, C. R., Field, I. C., et al. (2007). Southern elephant seals from Kerguelen Islands confronted by Antarctic Sea ice. Changes in movements and in diving behaviour. Deep Sea Res. Part II Top. Stud. Oceanogr. 54, 343–355. doi: 10.1016/j.dsr2.2006.11.005
Biuw, M., McConnell, B., Bradshaw, C. J., Burton, H., and Fedak, M. (2003). Blubber and buoyancy: monitoring the body condition of free-ranging seals using simple dive characteristics. J. Exp. Biol. 206, 3405–3423. doi: 10.1242/jeb.00583
Boehme, L., Lovell, P., Biuw, M., Roquet, F., Nicholson, J., Thorpe, S. E., et al. (2009). Animal-borne CTD-Satellite relay data loggers for real-time oceanographic data collection. Ocean Sci. 5, 685–695. doi: 10.5194/os-5-685-2009
Bost, C. A., Cotté, C., Terray, P., Barbraud, C., Bon, C., Delord, K., et al. (2015). Large-scale climatic anomalies affect marine predator foraging behaviour and demography. Nat. Commun. 6:8220.
Carlini, A. R., Daneri, G. A., Marquez, M. E. I., Soave, G. E., and Poljak, S. (1997). Mass transfer from mothers to pups and mass recovery by mothers during the post-breeding foraging period in southern elephant seals (Mirounga leonina) at King George Island. Polar Biol. 18, 305–310. doi: 10.1007/s003000050192
Chaigne, A., Authier, M., Richard, P., Cherel, Y., and Guinet, C. (2013). Shift in foraging grounds and diet broadening during ontogeny in southern elephant seals from Kerguelen Islands. Marine Biol. 160, 977–986. doi: 10.1007/s00227-012-2149-5
Clausius, E., McMahon, C., Harcourt, R., and Hindell, M. (2017). Effect of climate variability on weaning mass in a declining population of southern elephant seals Mirounga leonina. Mar. Ecol. Prog. Ser. 568, 249–260. doi: 10.3354/meps12085
Clinton, W. L., and Le Boeuf, B. J. (1993). Sexual Selection’s effects on male life history and the pattern of male mortality. Ecology 74, 1884–1892. doi: 10.2307/1939945
Collett, D. (2015). Modelling Survival Data in Medical Research. Boca Raton, FL: Chapman and Hall/CRC, doi: 10.1201/b18041
Cox, S. L., Authier, M., Orgeret, F., Weimerskirch, H., and Guinet, C. (2020). High mortality rates in a juvenile free-ranging marine predator and links to dive and forage ability. Ecol. Evol. 10, 410–430. doi: 10.1002/ece3.5905
Desprez, M., Gimenez, O., McMahon, C. R., Hindell, M. A., and Harcourt, R. G. (2018). Optimizing lifetime reproductive output: intermittent breeding as a tactic for females in a long-lived, multiparous mammal. J. Anim. Ecol. 87, 199–211. doi: 10.1111/1365-2656.12775
Desprez, M., Harcourt, R., Hindell, M. A., Cubaynes, S., Gimenez, O., and McMahon, C. R. (2014). Age-specific cost of first reproduction in female southern elephant seals. Biol. Lett. 10:20140264. doi: 10.1098/rsbl.2014.0264
Fedak, M. A., Arnbom, T., and Boyd, I. L. (1996). The relation between the size of southern elephant seal mothers, the growth of their pups, and the use of maternal energy, fat, and protein during lactation. Physiol. Zool. 69, 887–911. doi: 10.1086/physzool.69.4.30164234
Field, I. C., Bradshaw, C. J., Burton, H. R., and Hindell, M. A. (2005). Juvenile southern elephant seals exhibit seasonal differences in energetic requirements and use of lipids and protein stores. Physiol. Biochem. Zool. 78, 491–504. doi: 10.1086/430227
Field, I. C., Harcourt, R. G., Boehme, L., Bruyn, P. N., De Charrassin, J.-B., McMahon, C. R., et al. (2012). Refining instrument attachment on phocid seals. Mar. Mammal Sci. 28, 325–332.
Forcada, J., and Trathan, P. N. (2009). Penguin responses to climate change in the Southern Ocean. Glob. Change Biol. 15, 1618–1630. doi: 10.1111/j.1365-2486.2009.01909.x
Guinet, C., Jouventin, P., and Weimerskirch, H. (1999). Recent population change of the southern elephant seal at îles Crozet and îles Kerguelen: the end of the decrease? Antarctic Sci. 11, 193–197. doi: 10.1017/s0954102099000255
Hanson, N., Thompson, D., Duck, C., Moss, S., and Lonergan, M. (2013). Pup mortality in a rapidly declining harbour seal (Phoca vitulina) population. PLoS One 8:e80727. doi: 10.1371/journal.pone.0080727
Harcourt, R., Sequeira, A. M. M., Zhang, X., Roquet, F., Komatsu, K., Heupel, M., et al. (2019). Animal-Borne Telemetry: an integral component of the ocean observing toolkit. Front. Mar. Sci. 6:326. doi: 10.3389/fmars.2019.00326
Hays, G. C., Bradshaw, C. J. A., James, M. C., Lovell, P., and Sims, D. W. (2007). Why do Argos satellite tags deployed on marine animals stop transmitting? J. Exp. Mar. Biol. Ecol. 349, 52–60. doi: 10.1016/j.jembe.2007.04.016
Hays, G. C., Broderick, A. C., Godley, B. J., Luschi, P., and Nichols, W. J. (2003). Satellite telemetry suggests high levels of fishing-induced mortality in marine turtles. Mar. Ecol. Prog. Ser. 262, 305–309. doi: 10.3354/meps262305
Hays, G. C., Ferreira, L. C., Sequeira, A. M. M., Meekan, M. G., Duarte, C. M., Bailey, H., et al. (2016). Key questions in marine megafauna movement ecology. Trends Ecol. Evol. 31, 463–475. doi: 10.1016/j.tree.2016.02.015
Hindell, M. A., McMahon, C. R., Bester, M. N., Boehme, L., Costa, D., and Fedak, et al. (2016). Circumpolar habitat use in the southern elephant seal: implications for foraging success and population trajectories. Ecosphere 7:e01213.
Hindell, M. A., Sumner, M., Bestley, S., Wotherspoon, S., Harcourt, R. G., Lea, M.-A., et al. (2017). Decadal changes in habitat characteristics influence population trajectories of southern elephant seals. Glob. Change Biol. 23, 5136–5150. doi: 10.1111/gcb.13776
Hofman, M. P. G., Hayward, M. W., Heim, M., Marchand, P., Rolandsen, C. M., Mattisson, J., et al. (2019). Right on track? Performance of satellite telemetry in terrestrial wildlife research. PLoS One 14:e0216223.
Horning, M., Andrews, R. D., Bishop, A. M., Boveng, P. L., Costa, D. P., Crocker, D. E., et al. (2019). Best practice recommendations for the use of external telemetry devices on pinnipeds. Anim. Biotelemetry 7:20. doi: 10.1186/s40317-019-0182-6
Horning, M., Haulena, M., Tuomi, P. A., Mellish, J. A., Goertz, C. E., Woodie, K., et al. (2017). Best practice recommendations for the use of fully implanted telemetry devices in pinnipeds. Anim. Biotelem. 5:13.
Horning, M., and Mellish, J. A. (2014). In cold blood: evidence of Pacific sleeper shark (Somniosus pacificus) predation on Steller sea lions (Eumetopias jubatus) in the Gulf of Alaska. Fish. Bullet. 112:4.
Jonsen, I. D., Basson, M., Bestley, S., Bravington, M. V., Patterson, T. A., Pedersen, M. W., et al. (2013). State-space models for bio-loggers: a methodological road map. Deep Sea Res. Part II Top. Stud. Oceanogr. 88, 34–46. doi: 10.1016/j.dsr2.2012.07.008
Jonsen, I. D., McMahon, C. R., Patterson, T. A., Auger−Méthé, M., Harcourt, R., Hindell, M. A., et al. (2019). Movement responses to environment: fast inference of variation among southern elephant seals with a mixed effects model. Ecology 100:e02566. doi: 10.1002/ecy.2566
Klaassen, R. H. G., Hake, M., Strandberg, R., Koks, B. J., Trierweiler, C., Exo, K.-M., et al. (2016). When and where does mortality occur in migratory birds? Direct evidence from long-term satellite tracking of raptors. J. Anim. Ecol. 16, 176–184. doi: 10.1111/1365-2656.12135
Kooyman, G. L., McDonald, B. I., and Goetz, K. T. (2015). Why do satellite transmitters on emperor penguins stop transmitting? Anim. Biotelem. 3:54. doi: 10.1186/s40317-015-0091-2
Kooyman, G. L., and Ponganis, P. J. (2004). The icing of external recorders during the polar winter. Mem. Natl Inst. Polar Res. 58, 183–187.
Labrousse, S., Williams, G., Tamura, T., Bestley, S., Sallée, J.-B., Fraser, A. D., et al. (2018). Coastal polynyas: winter oases for subadult southern elephant seals in East Antarctica. Sci. Rep. 8:3183.
Le Boeuf, B. J., and Laws, R. M. (1994). Elephant Seals: Population Ecology, Behavior, and Physiology. Berkeley, CA: University of California Press.
Lea, M.-A., Johnson, D., Ream, R., Sterling, J., Melin, S., and Gelatt, T. (2009). Extreme weather events influence dispersal of naive northern fur seals. Biol. Lett. 5, 252–257. doi: 10.1098/rsbl.2008.0643
Lebreton, J.-D., Burnham, K. P., Clobert, J., and Anderson, D. R. (1992). Modeling survival and testing biological hypotheses using marked animals: a unified approach with case studies. Ecol. Monogr. 62, 67–118. doi: 10.2307/2937171
Lindstrom, M. J., and Bates, D. M. (1988). Newton—raphson and EM algorithms for linear mixed-effects models for repeated-measures data. J. Am. Statist. Assoc. 83, 1014–1022. doi: 10.2307/2290128
Lovenduski, N. S., and Gruber, N. (2005). Impact of the Southern Annular Mode on Southern Ocean circulation and biology. Geophys. Res. Lett. 32:L11603.
Martin, C., Bentaleb, I., Steelandt, S., and Guinet, C. (2011). Stable carbon and nitrogen isotope variations in canine dentine growth layers of Kerguelen southern elephant seals. Mar. Ecol. Prog. Ser. 439, 295–305. doi: 10.3354/meps09331
Martinussen, T., and Scheike, T. H. (2007). Dynamic Regression Models for Survival Data. Berlin: Springer Science & Business Media.
Mazzaro, L. M., and Dunn, J. L. (2009). Descriptive account of long-term health and behavior of two satellite-tagged captive harbor seals Phoca vitulina. Endanger Species Res. 10, 159–163. doi: 10.3354/esr00190
McConnell, B. J., Chambers, C., and Fedak, M. A. (1992). Foraging ecology of southern elephant seals in relation to the bathymetry and productivity of the Southern Ocean. Antarct. Sci. 4, 393–398. doi: 10.1017/S0954102092000580
McIntyre, T., Postma, M., Van der Merwe, D. S., Wege, M., and Bester, M. N. (2011). Hitchhiking goose barnacles and their potential implications on the functioning of animal-borne instruments. South Afr. J. Wildl. Res. 41, 218–223. doi: 10.3957/056.041.0210
McMahon, C. R., Bester, M. N., Burton, H. R., Hindell, M. A., and Bradshaw, C. J. (2005). Population status, trends and a re-examination of the hypotheses explaining the recent declines of the southern elephant seal Mirounga leonina. Mammal Rev. 35, 82–100. doi: 10.1111/j.1365-2907.2005.00055.x
McMahon, C. R., Burton, H., McLean, S., Slip, D., and Bester, M. (2000). Field immobilisation of southern elephant seals with intravenous tiletamine and zolazepam. Vet. Rec. 146, 251–254. doi: 10.1136/vr.146.9.251
Mcmahon, C. R., Burton, H. R., and Bester, M. N. (2003). A demographic comparison of two southern elephant seal populations. J. Anim. Ecol. 72, 61–74. doi: 10.1046/j.1365-2656.2003.00685.x
McMahon, C. R., Field, I. C., Bradshaw, C. J., White, G. C., and Hindell, M. A. (2008). Tracking and data–logging devices attached to elephant seals do not affect individual mass gain or survival. J. Exp. Mar. Biol. Ecol. 360, 71–77. doi: 10.1016/j.jembe.2008.03.012
McMahon, C. R., Harcourt, R. G., Burton, H. R., Daniel, O., and Hindell, M. A. (2017). Seal mothers expend more on offspring under favourable conditions and less when resources are limited. J. Anim. Ecol. 86, 359–370. doi: 10.1111/1365-2656.12611
McMahon, C. R., New, L. F., Fairley, E. J., Hindell, M. A., and Burton, H. R. (2015). The effects of body size and climate on post−weaning survival of elephant seals at Heard Island. J. Zool. 297, 301–308. doi: 10.1111/jzo.12279
Murray, D. L. (2006). On improving telemetry-based survival estimation. J. Wildl. Manag. 70, 1530–1543. doi: 10.2193/0022-541
Myers, A. E., Lovell, P., and Hays, G. C. (2006). Tools for studying animal behaviour: validation of dive profiles relayed via the Argos satellite system. Anim. Behav. 71, 989–993. doi: 10.1016/j.anbehav.2005.06.016
Naughton, G. P., Caudill, C. C., Keefer, M. L., Bjornn, T. C., Stuehrenberg, L. C., and Peery, C. A. (2005). Late-season mortality during migration of radio-tagged adult sockeye salmon (Oncorhynchus nerka) in the Columbia River. Can. J. Fish. Aquat. Sci. 62, 30–47. doi: 10.1139/f04-147
Oosthuizen, W. C., Bester, M. N., Altwegg, R., McIntyre, T., and de Bruyn, P. N. (2015). Decomposing the variance in southern elephant seal weaning mass: partitioning environmental signals and maternal effects. Ecosphere 6, 1–22.
Oosthuizen, W. C., Bester, M. N., Tosh, C. A., Guinet, C., Besson, D., and De Bruyn, P. N. (2011). Dispersal and dispersion of southern elephant seals in the Kerguelen province, Southern Ocean. Antarct. Sci. 23, 567–577. doi: 10.1017/s0954102011000447
Orgeret, F., Cox, S. L., Weimerskirch, H., and Guinet, C. (2019). Body condition influences ontogeny of foraging behavior in juvenile southern elephant seals. Ecol. Evol. 9, 223–236. doi: 10.1002/ece3.4717
Parker, D. M., and Balazs, G. H. (2014). Variability in reception duration of dual satellite tags on sea turtles tracked in the pacific ocean. Micronesica 3, 1–8.
Pistorius, P. A., Bester, M. N., Hofmeyr, G. J., Kirkman, S. P., and Taylor, F. E. (2008). Seasonal survival and the relative cost of first reproduction in adult female southern elephant seals. J. Mammal. 89, 567–574. doi: 10.1644/07-mamm-a-219r.1
Pistorius, P. A., Bester, M. N., and Kirkman, S. P. (1999). Survivorship of a declining population of southern elephant seals, Mirounga leonina, in relation to age, sex and cohort. Oecologia 121, 201–211. doi: 10.1007/s004420050922
Pistorius, P. A., Bester, M. N., Lewis, M. N., Taylor, F. E., Campagna, C., and Kirkman, S. P. (2004). Adult female survival, population trend, and the implications of early primiparity in a capital breeder, the southern elephant seal (Mirounga leonina). J. Zool. 263, 107–119. doi: 10.1017/s0952836904004984
Pistorius, P. A., Bester, M. N., and Taylor, F. E. (2005). Pubescent southern elephant seal males: population changes at Marion Island and the food limitation hypothesis. South Afr. J. Wildl. Res. Delayed Open Access 35, 215–218.
Pistorius, P. A., De Bruyn, P. J. N., and Bester, M. N. (2011). Population dynamics of southern elephant seals: a synthesis of three decades of demographic research at Marion Island. Afr. J. Mar. Sci. 33, 523–534. doi: 10.2989/1814232x.2011.637357
Proffitt, K. M., Garrott, R. A., Rotella, J. J., Siniff, D. B., and Testa, J. W. (2007). Exploring linkages between abiotic oceanographic processes and a top-trophic predator in an Antarctic ecosystem. Ecosystems 10:120. doi: 10.1007/s10021-006-9003-x
Reisinger, R. R., and Bester, M. N. (2010a). Goose barnacles hitchhike on satellite-tracked southern elephant seals. Polar Biol. 33, 561–564. doi: 10.1007/s00300-009-0724-0
Reisinger, R. R., and Bester, M. N. (2010b). Goose barnacles on seals and a penguin at Gough Island. Afr. Zool. 45, 129–132. doi: 10.3377/004.045.0109
Richard, G., Cox, S. L., Picard, B., Vacquié-Garcia, J., and Guinet, C. (2016). Southern elephant seals replenish their lipid reserves at different rates according to foraging habitat. PLoS One 11:e0166747. doi: 10.1371/journal.pone.0166747
Rivière, P., Jaud, T., Siegelman, L., Klein, P., Cotté, C., Le Sommer, J., et al. (2019). Sub−mesoscale fronts modify elephant seals foraging behavior. Limnol. Oceanogr. Lett. 4, 193–204. doi: 10.1002/lol2.10121
Singer, J. D., and Willett, J. B. (1991). Modeling the days of our lives: using survival analysis when designing and analyzing longitudinal studies of duration and the timing of events. Psychol. Bull. 110:268. doi: 10.1037/0033-2909.110.2.268
Smith, B. J., Hart, K. M., Mazzotti, F. J., Basille, M., and Romagosa, C. M. (2018). Evaluating GPS biologging technology for studying spatial ecology of large constricting snakes. Anim. Biotelem. 6:1.
Swimmer, Y., Empey Campora, C., Mcnaughton, L., Musyl, M., and Parga, M. (2014). Post-release mortality estimates of loggerhead sea turtles (Caretta caretta) caught in pelagic longline fisheries based on satellite data and hooking location: POST-RELEASE MORTALITY OF LOGGERHEAD SEA TURTLES. Aquat. Conserv. Mar. Freshw. Ecosyst. 24, 498–510. doi: 10.1002/aqc.2396
Therneau, T. M., and Grambsch, P. M. (2000). Testing Proportional Hazards. InModeling Survival Data: Extending the Cox model 2000. New York, NY: Springer, 127–152.
Thompson, D. W., and Wallace, J. M. (2000). Annular modes in the extratropical circulation. Part I J. Clim. 13, 1000–1016. doi: 10.1175/1520-0442(2000)013<1000:amitec>2.0.co;2
Tixier, P., Lea, M. A., Hindell, M. A., Guinet, C., Gasco, N., Duhamel, G., et al. (2018). Killer whale (Orcinus orca) interactions with blue-eye trevalla (Hyperoglyphe antarctica) longline fisheries. PeerJ 6:e5306. doi: 10.7717/peerj.5306
Trathan, P. N., Forcada, J., and Murphy, E. J. (2007). Environmental forcing and Southern Ocean marine predator populations: effects of climate change and variability. Philos. Trans. R. Soc. Lond. B Biol. Sci. 362, 2351–2365. doi: 10.1098/rstb.2006.1953
van den Hoff, J., McMahon, C. R., Simpkins, G. R., Hindell, M. A., Alderman, R., and Burton, H. R. (2014). Bottom-up regulation of a pole-ward migratory predator population. Proc. R. Soc. Lond. B Biol. Sci. 281:20132842. doi: 10.1098/rspb.2013.2842
Van Den Hoff, J., and Morrice, M. G. (2008). Sleeper shark (Somniosus antarcticus) and other bite wounds observed on southern elephant seals (Mirounga leonina) at Macquarie Island. Mar. Mamm. Sci. 24, 239–247. doi: 10.1111/j.1748-7692.2007.00181.x
Keywords: satellite telemetry, Southern Ocean, ARGOS, Mirounga leonina, survival %, migration, SES, IMOS animal tracking facility
Citation: Henderson AF, McMahon CR, Harcourt R, Guinet C, Picard B, Wotherspoon S and Hindell MA (2020) Inferring Variation in Southern Elephant Seal At-Sea Mortality by Modelling Tag Failure. Front. Mar. Sci. 7:517901. doi: 10.3389/fmars.2020.517901
Received: 06 December 2019; Accepted: 31 August 2020;
Published: 24 September 2020.
Edited by:
Peter H. Dutton, Southwest Fisheries Science Center (NOAA), United StatesReviewed by:
Tommy Clay, University of Liverpool, United KingdomPaige Eveson, Commonwealth Scientific and Industrial Research Organisation (CSIRO), Australia
Copyright © 2020 Henderson, McMahon, Harcourt, Guinet, Picard, Wotherspoon and Hindell. This is an open-access article distributed under the terms of the Creative Commons Attribution License (CC BY). The use, distribution or reproduction in other forums is permitted, provided the original author(s) and the copyright owner(s) are credited and that the original publication in this journal is cited, in accordance with accepted academic practice. No use, distribution or reproduction is permitted which does not comply with these terms.
*Correspondence: Angus F. Henderson, YW5ndXMuaGVuZGVyc29uQHV0YXMuZWR1LmF1